- 1Department of Anesthesiology, Division of Critical Care, Duke University School of Medicine, Durham, NC, United States
- 2Human Pharmacology and Physiology Laboratory (HPPL), Duke University School of Medicine, Durham, NC, United States
- 3Duke Cardiovascular Performance and Innovation Lab, Department of Medicine, Division of Cardiology, Duke University School of Medicine, Durham, NC, United States
- 4Department of Intensive Care Adults, Erasmus MC University Medical Center Rotterdam, Rotterdam, Netherlands
- 5Department of Targeted Intervention, Division of Surgery, University College London, London, United Kingdom
- 6Department of Clinical Research, InBody BWA Inc., Trooper, PA, United States
- 7Department of Medicine, Division of Cardiology, Duke University School of Medicine, Durham, NC, United States
- 8Department of Medicine, Pulmonary, Allergy and Critical Care Medicine, Duke University School of Medicine, Durham, NC, United States
- 9Department of Surgery, Division of Trauma, Acute and Critical Care, Duke University School of Medicine, Durham, NC, United States
- 10Departments of Radiology and Urology, Duke Cancer Institute Center for Prostate & Urologic Cancers, Duke University School of Medicine, Durham, NC, United States
- 11Department of Pulmonology and Critical Care, NYU School of Medicine, New York, NY, United States
- 12Department of Pulmonology and Critical Care, Columbia University Vagelos College of Physicians and Surgeons, New York, NY, United States
- 13Department of Intensive Care, Pontificia Universidad Católica de Chile, Santiago, Chile
Introduction: The COVID-19 pandemic brought unprecedented challenges to healthcare systems worldwide, particularly the often challenging physical recovery from critical illness. Among the myriad complications faced by these patients, ICU-acquired weakness (ICU-AW) stands out due to its significant impact on patient outcomes. ICU-AW, characterized by skeletal muscle atrophy and weakness, is linked to critical illness polyneuropathy (CIN), myopathy (CIM), and muscle atrophy. Notably, fasciculations, or involuntary muscle twitches, have been observed in patients with ICU-AW, suggesting altered muscle fiber excitability. This study aimed to describe the pattern of fasciculations in critically ill mechanically ventilated COVID-19 patients, assess clinical features associated with fasciculations, and evaluate their prognostic significance.
Methods: The study enrolled patients admitted to the Intensive Care Unit (ICU) with COVID-19 infection requiring intubation and mechanical ventilation. Quadriceps muscles were assessed using muscle skeletal (MSK) ultrasound and Phase Angle (PhA) through multifrequency segmental bioelectrical impedance analysis (MFBIA) every 72 h. PhA is considered a marker of cell membrane integrity and function, with lower PhA values indicating reduced skeletal muscle quality and increased risk of sarcopenia. Fasciculations were classified based on their appearance and frequency.
Results: A total of 35 intubated patients were studied, with 15 patients (43%) displaying scattered fasciculations exclusively in the vastus intermedius muscles. Upon admission, the study found no significant difference in PhA between the fasciculation and non-fasciculation groups. However, PhA decreased significantly from admission to discharge ICU only in the fasciculation group. Patients with fasciculations exhibited higher mortality rates, though not statistically significant, and this correlated with prolonged ICU and hospital lengths of stay.
Discussion: This study is the first to report scattered fasciculations in the vastus intermedius muscles of multiple patients with SARS-CoV-2 infection identified with MSK ultrasound. The findings suggest that the combination of impaired muscle cellular function (PhA) and the onset of fasciculations could serve as a potential biomarker of adverse outcomes and ICU-related muscle dysfunction and muscle loss.
Introduction
Although SARS-CoV-2 infection (COVID-19) most frequently results in respiratory symptoms, other major complications include persistent neuromuscular sequelae such as muscle weakness and myalgia (1–4). Critically ill patients with COVID-19 are at particular risk of developing ICU-acquired weakness (ICU-AW) (5–8). ICU-AW is defined as diffuse skeletal muscle wasting and weakness due to polyneuropathy (CIN) and/or myopathy (CIM) and muscle atrophy in the absence of another plausible etiology other than the critical illness (4, 9, 10). The etiology of ICU-AW is attributed to the combined effects of physical inactivity, systemic inflammation, hyperglycemia, and pharmacologic interventions (including corticosteroids and neuromuscular blocking agents). These factors are common in critically ill COVID-19 patients (11). Significant muscle weakness is reported in 25%–33% of patients mechanically ventilated for 4–7 days and in up to 60% in non-COVID acute respiratory distress syndrome (9, 12). ICU-AW has been reported in up to 72% of mechanically ventilated COVID-19 patients (11). COVID-19 infection is known to have significant detrimental effects on skeletal muscle, particularly in patients requiring ICU admission. Immune-mediated necrotizing myopathy, mitochondrial dysfunction, and myofiber damage due to the severe inflammatory response (cytokine storm) have been documented (13–15). Spontaneous depolarization of the lower motor neuron due to subacute partial muscle denervation occurs in ICU-AW and lower motor neuron diseases (MND) (16, 17). This partial muscle denervation can ultimately lead to involuntary muscle fiber excitations within one single motor unit, also known as fasciculations, known to occur in ICU-AW (16). These fasciculations represent a proxy of altered muscle fiber excitability, resulting from the reduced excitability of the nerve and muscle cell membrane, and may indicate an early stage of ICU-AW. Neuromuscular ultrasound (NMUS) has gained more acceptance in the neuromuscular physiology community. It can augment the clinician's diagnostic capabilities to assess MND, CIN, CIM, and muscle mass (thickness/cross-sectional area) from different muscle groups, such as the quadriceps and diaphragm (10, 18–23). Muscle fasciculations can be relatively quickly diagnosed with neuromuscular ultrasound (NMUS) with a sensitivity of 96% and specificity of 84% (16, 24). M-mode ultrasound captures movement over time on a single still image, allowing visualization of fasciculations' duration and frequency (temporal changes). This is a significant advantage over B-mode imaging, which only shows a cross-sectional view at one point (24). Fasciculations can easily be differentiated from other movements like voluntary contractions and (unifocal and rhythmic) arterial pulsations (25). Combining dynamic and static images in M-mode and B-mode enables the detection of spontaneous muscle activity. This way, ultrasound can assess muscle fasciculations with higher sensitivity than EMG measurements (18, 26, 27). The onset of fasciculations could be an early biomarker of ICU-AW, reflecting disease severity and impacting clinical outcomes in COVID-19 patients requiring critical care admission (18, 26). Our primary aim was to describe the pattern of and assess some pertinent clinical features associated with fasciculations in critically ill mechanically ventilated COVID-19 patients and study their prognostic significance.”
Methods
The Longitudinal Energy Expenditure and Metabolic Effects in Patients with COVID-19 (LEEP-COVID) study is a single-center prospective longitudinal cohort study of critically ill adult patients infected by the SARS-CoV-2 virus, beginning April 1, 2020. Patients with COVID-19 disease, 18 years of age, admitted to a Duke University Medical Center ICU. Already receiving or expected to require mechanical ventilation (MV) for >48 h were included in this study. Patients were excluded if their expected duration of ICU length of stay (LOS) or survival was <24 h if they had an implantable cardiac device (pacemaker, defibrillator, LVAD). The study was approved by the Duke Health Institutional Review Board in Durham, North Carolina (IRB# Pro00105221) (clinicaltrials.gov: NCT04350073), and patients were enrolled after obtaining a waiver of informed consent.
Musculoskeletal ultrasound images of the quadriceps muscles using the Philips Lumify linear array transducer L12-4 (Eindhoven, Netherlands) were obtained. Phase Angle (PhA) was measured using bioelectrical impedance analysis (BIA) (InBody S10, Biospace Co., Ltd, Korea). All assessments were done every 72 h. Electrodes were placed on the patient's hands and feet to pass a small electrical current through the body and assess body composition and phase angle while the patient was in the supine position.
We examined the rectus femoris (RF), vastus lateralis (VL), and vastus intermedius (VI) muscles bilaterally using the MSK preset mode. The midline between the origin and insertion of the rectus femoris muscle was used as standardized landmarks, with the patient in a supine position and legs extended. Long- and short-axis dicom images and loop videos were recorded for offline B-mode and M-mode analysis. Each left and right quadriceps muscle was assessed three times for 10 s without moving the probe. If the patient required prone positioning, the assessment was done after the rotation from prone to supine within the 72-hour time window. Muscle fasciculations were classified based on spontaneous activity and highly irregular patterns, needing to appear at least twice in B- and M-mode (16). BIA was used to assess body composition, particularly the distribution of body fluids. PhA is considered a valuable marker of muscle cellular health because it reflects the integrity and quality of muscle cell membranes (28–33).
Statistics
The BIA data were segmented individually and screened for measurement errors to ensure data quality. A linear regression model was fitted to each individual's data to account for the irregular assessment intervals. The initial and final values and the slopes were extracted from the fitted model to describe changes in PhA. This process enabled us to identify the intercepts, representing the time points of admission and discharge, and the slope, allowing us to extract the trend of how phase changes over time. The results from both the right and left sides of each individual were averaged to reduce variability and improve the accuracy of the measurements. Differences between the groups were evaluated with the Mann-Whitney U-test for continuous variables. A one-tailed t-test, with an alpha level of 0.1, was used to compare the initial and final values and the slopes of the non-fasciculation and fasciculation groups.
Results
35 consecutive intubated patients were enrolled. Patient demographics and results are presented in Table 1. Differences between the groups were evaluated with the Mann-Whitney U-test for continuous variables.
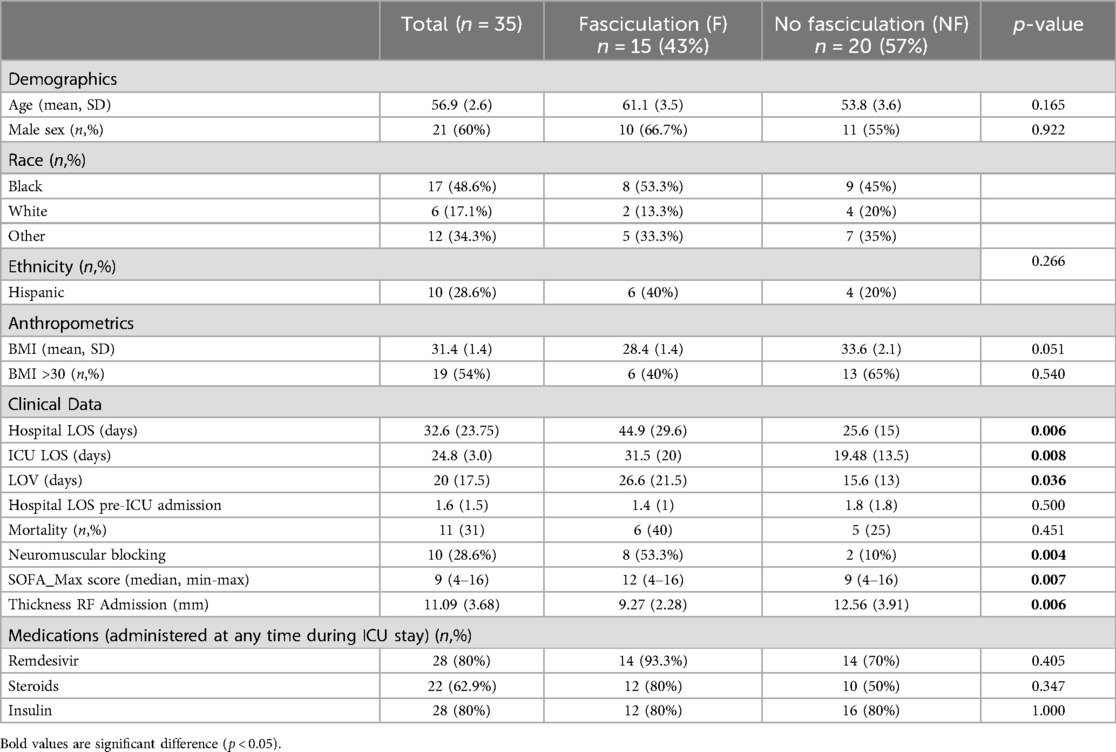
Table 1. Descriptive statistics: all data on all subjects are reported as mean ± standard deviation (D) or number (n) and percent. Differences between the groups were evaluated with the Mann-Whitney U-test.
Ultrasound
Scattered fasciculations, seen in both the left and right VI muscles only, were identified in 15/35 patients (43%) at any point in their ICU stay, and no fasciculations were seen in any of the remaining patients during their ICU stay. Figure 1 illustrates fasciculations as visualized in short and long-axis M-mode dicom images of the RF and VI. The oscillations visualize the scattered fasciculations in the VI only. The scattered fasciculations were demonstrated during every assessment of the entire ICU stay.
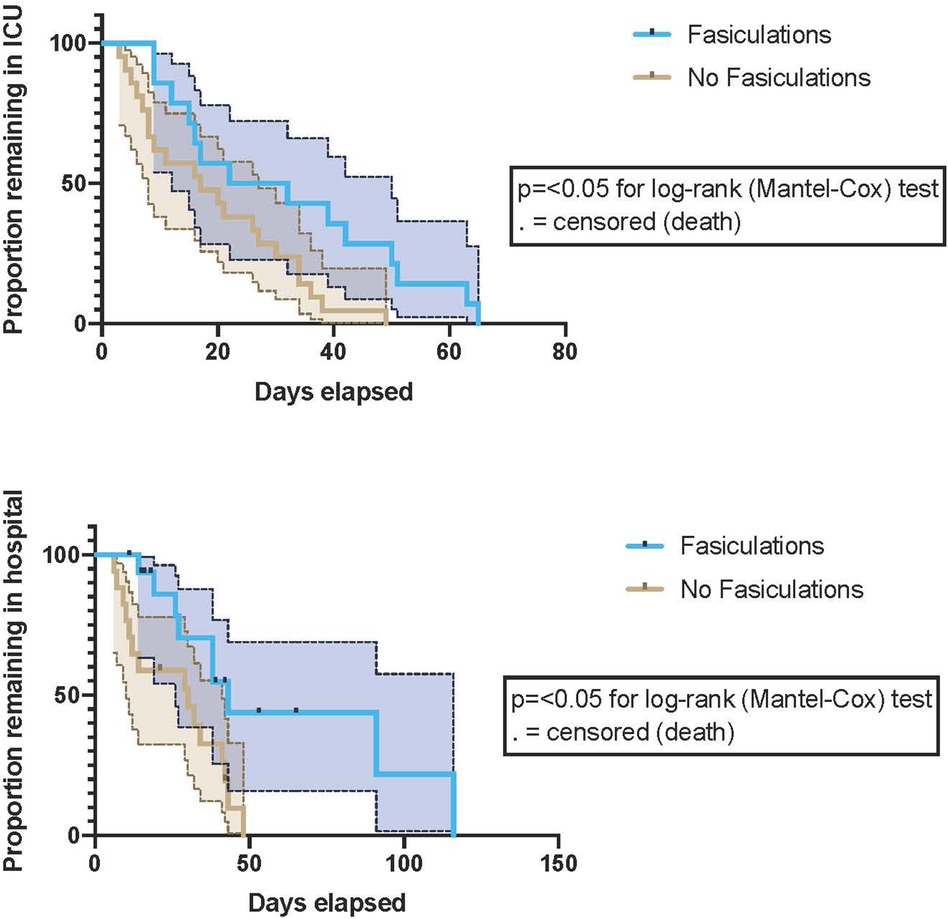
Figure 1. Proportions of patients with and without fasciculations remained in the ICU and the hospital during the study period. P-values are for the Log-rank (Mantel-Cox) test. 95% confidence intervals (CI) are represented by dotted lines and shading. Fasciculations were associated with both a prolonged ICU and hospital length of stay.
RF thickness assessed upon admission was significantly (p = 0.006) lower (9.27 mm). ± 2.28) in the fasciculation group compared to the non-fasciculation group (12.56 mm. ± 3.91.
Bioimpedance
PhA upon admittance to ICU in the fasciculation group was not different compared with the non-fasciculation group (4.31 ± 1.18 vs. 4.50 ± 1.67) (p = 0.36). PhA on discharge from ICU was decreased in patients who developed fasciculations compared to those who did not (3.61 ± 1.46 vs. 4.47 ± 1.73) (p = 0.09). PhA in patients who developed fasciculations decreased from ICU admittance to discharge (p = 0.09). In those patients who did not develop fasciculations, no difference in PhA was observed from admission to discharge (p = 0.48). During the course of ICU admission, patients who developed muscle fasciculations exhibited a negative PhA slope (−0.020 ± 0.043), indicating decreasing muscle cellular integrity over the course of the ICU admission. Conversely, the non-fasciculation group had a positive PhA slope (0.0014 ± 0.18), indicating improved tissue integrity over time. However, the difference in PhA slopes between the non-fasciculation and fasciculation groups was not statistically significant due to the study's power limitations.
During the study period, mortality was higher in patients who developed fasciculations, although not statistically significant (Hazard Ratio (log-rank) 1.5; 95% confidence interval [CI], 0.4–5.5; P = 0.5). Patients with fasciculations demonstrated prolonged ICU and hospital length of stay. [Log-rank P-value 0.02 for ICU LOS (p = 0.008) and 0.01 for Hospital LOS] p = 0.036), (Figure 2). Maximal SOFA scores were elevated in individuals who developed fasciculations (median (min-max) 9(4–16) vs. 12 (4–16); p = 0.007). Of those patients who developed scattered fasciculations, 60% developed them in week one of ICU stay; the remainder had developed fasciculations by the end of week 2. Patients who did not develop scattered fasciculations were more (p = 0.051) obese (Mann-Whitney U-test P = 0.05).
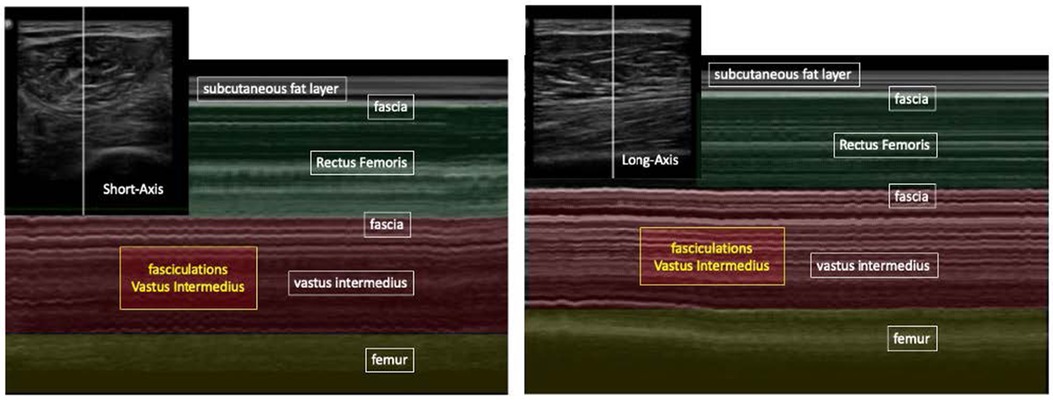
Figure 2. B-mode ultrasound (picutre in the left corner) and M-mode ultrasound, long- and short-axis, show RF and VI muscle. Temporal patterns of fasciculations are seen only in the VI.
The use of steroids during the treatment of COVID-19 in the ICU at any time point in the intensive care stay was associated with the development of scattered fasciculations (Relative Risk (Fisher’s exact test) 2.4; 95% confidence interval (CI), 0.95–7; P = 0.09).
In both groups, only propofol was used for sedation during mechanical ventilation; dosing was titrated to achieve a target Richmond-Agitation-Sedation Scale (RASS) score. Propofol is also known to reduce the incidence of fasciculations potentially (34). Neither remdesivir nor insulin administration was associated with the development of fasciculations (Table 1).
Discussion
This pilot study is the first to report fasciculations identified with musculoskeletal ultrasound in the VI muscles of multiple patients with SARS-CoV-2 infection. This finding was associated with increased severity of critical illness, increased length of ICU and hospital stay, and the usage of steroids and neuromuscular blocking agents (NMBAs). A non-depolarizing NMBA (cisatracurium) was used in all patients during the study, which is known not to induce muscle fasciculations (35). Patients who developed fasciculations also demonstrated deteriorations in Phase Angle, a proxy measure of muscle cellular health, over the course of their critical care stay.
These findings are congruent with known associations with the development of ICU-AW. The additional observations of sarcopenia at the time of admission and a trend toward increased patient age on admission to the ICU further support this association. A combination of worsening sarcopenia, deteriorations in Phase Angle, and the development of fasciculations might act as biomarkers of ICU-acquired muscle dysfunction and even ICU-AW.
Based on the sarcopenia cut-off values published by Fukumoto et al., the findings specifically highlight the importance of identifying sarcopenia in critically ill patients admitted to the ICU (36). Sarcopenia appears to be an independent predictor of poor outcomes and higher mortality rates in this patient population, regardless of the specific mortality timeframe analyzed (37, 38).
The presence of fasciculations exclusively in the vastus intermedius muscle is intriguing. Previous studies have reported neuromuscular complications such as myalgia, myopathy, and neuropathy in COVID-19 patients, but the specific pattern of fasciculations in the vastus intermedius has not been described before. The vastus intermedius is one of the quadriceps muscles important for knee extension. Weakness or dysfunction of this muscle could contribute to the profound weakness and fatigue reported by many COVID-19 survivors. Although not statistically significant, likely due to the small sample size, patients with fasciculations had higher mortality rates and longer ICU and hospital stays. This implies that fasciculations may be a marker of more severe disease. More extensive prospective studies are needed to confirm if fasciculations are an independent predictor of poor outcomes in critically ill COVID-19 patients (39).
The main limitations are the small sample size and lack of long-term follow-up to determine if fasciculations persist or resolve. Furthermore, no functional or electromyographic data is available to assess whether patients who developed fasciculations did indeed go on to develop ICU-AW. The different lengths of stay in critical care between groups may independently explain the association between reduced muscle size and the development of fasciculations. However, the difference in a gradient of Phase Angle decline between groups would suggest that those who did develop fasciculations were subject to deteriorations in muscle quality that would be expected to be associated with a reduction in function. The study did not include a control group of critically ill patients without COVID-19 to assess if fasciculations are unique to SARS-CoV-2 infection. Nevertheless, this pilot study provides important preliminary data to guide future research into neuromuscular complications of COVID-19 and perhaps in other critically ill patients. Future work should prospectively assess the combination of sarcopenia, deterioration in muscle size, and PhA combined with the development of fasciculations to further their utility as biomarkers and to elucidate any relationships with the development of ICU-AW. Future study design should consider a muscle biopsy to confirm or refute relationships with deterioration in muscle quality.
In conclusion, this study identifies a novel pattern of muscle fasciculations in the vastus intermedius of critically ill COVID-19 patients that correlates with impaired muscle cellular function and worse clinical outcomes. Serial musculoskeletal ultrasound may be a valuable tool to diagnose and monitor neuromuscular involvement in COVID-19 and potentially in other critically ill patients. More extensive prospective studies with longer follow-ups are warranted to validate these findings and determine the prognostic significance of fasciculations in COVID-19 patients.
Data availability statement
The raw data supporting the conclusions of this article will be made available by the authors, without undue reservation.
Ethics statement
The studies involving humans were approved by DUHS IRB Office. Suite 900 Erwin Square, 2200 West Main Street, Campus Box # 104026, Durham, NC 27705, Phone: (919) 668-5111. The studies were conducted in accordance with the local legislation and institutional requirements. The ethics committee/institutional review board waived the requirement of written informed consent for participation from the participants or the participants’ legal guardians/next of kin because The study would provide valuable data for decision-making during the COVID-19 pandemic. All assessments were noninvasive and FDA 510K approved.
Author contributions
JM: Writing – review & editing, Writing – original draft, Visualization, Validation, Supervision, Software, Resources, Project administration, Methodology, Investigation, Funding acquisition, Formal Analysis, Data curation, Conceptualization. JW: Writing – review & editing, Supervision, Methodology, Conceptualization. SC: Writing – review & editing, Methodology, Formal Analysis, Data curation, Conceptualization. DM: Writing – review & editing. MF: Writing – review & editing, Conceptualization. LU: Writing – review & editing. CB: Writing – review & editing. ZH: Writing – review & editing. KH: Writing – review & editing. RG: Writing – review & editing. MZ: Writing – review & editing, Methodology, Conceptualization. HE: Writing – review & editing, Supervision, Methodology, Conceptualization. DG: Writing – review & editing, Supervision, Methodology, Conceptualization. JB: Writing – review & editing, Supervision, Methodology, Conceptualization. PW: Writing – review & editing, Supervision, Methodology, Conceptualization.
Funding
The authors declare that this study received funding from Baxter International. The funder was not involved in the study design, collection, analysis, interpretation of data, the writing of this article or the decision to submit it for publication.
Acknowledgments
We warmly acknowledge the LEEP-COVID Research Taskforce: Anthony Sung MD, Lindsie Boerger RRT, Kathryn Lessig RD, Jessica Lumbard BS, Leslie C. Murray RD, Sue Steves RD, Jhana Parikh BS, Jacob Ribet BS, RRT LDN, Melanie Hollidge MD, Laura Niederer RD, Hilary Miller, MS, RD.
Conflict of interest
SC was employed by InBody BWA Inc. Trooper.
The remaining authors declare that the research was conducted in the absence of any commercial or financial relationships that could be construed as a potential conflict of interest.
Publisher's note
All claims expressed in this article are solely those of the authors and do not necessarily represent those of their affiliated organizations, or those of the publisher, the editors and the reviewers. Any product that may be evaluated in this article, or claim that may be made by its manufacturer, is not guaranteed or endorsed by the publisher.
Supplementary material
The Supplementary Material for this article can be found online at: https://www.frontiersin.org/articles/10.3389/fanes.2024.1440038/full#supplementary-material
References
1. Pinzon RT, Wijaya VO, Buana RB, Al Jody A, Nunsio PN. Neurologic characteristics in coronavirus disease 2019 (COVID-19): a systematic review and meta-analysis. Front Neurol. (2020) 11:565. doi: 10.3389/fneur.2020.00565
2. Bagnato S, Boccagni C, Marino G, Prestandrea C, D'Agostino T, Rubino F. Critical illness myopathy after COVID-19. Int J Infect Dis. (2020) 99:276–8. doi: 10.1016/j.ijid.2020.07.072
3. Nijholt W, Beek LT, Hobbelen JSM, van der Vaart H, Wempe JB, van der Schans CP, et al. The added value of ultrasound muscle measurements in patients with COPD: an exploratory study. Clin Nutr ESPEN. (2019) 30:152–8. doi: 10.1016/j.clnesp.2019.01.001
4. Lad H, Saumur TM, Herridge MS, Dos Santos CC, Mathur S, Batt J, et al. Intensive care unit-acquired weakness: not just another muscle atrophying condition. Int J Mol Sci. (2020) 21(21):7840. doi: 10.3390/ijms21217840
5. Hosey MM, Needham DM. Survivorship after COVID-19 ICU stay. Nat Rev Dis Primers. (2020) 6(1):60. doi: 10.1038/s41572-020-0201-1
6. Montalvan V, Lee J, Bueso T, De Toledo J, Rivas K. Neurological manifestations of COVID-19 and other coronavirus infections: a systematic review. Clin Neurol Neurosurg. (2020) 194:105921. doi: 10.1016/j.clineuro.2020.105921
7. Bax F, Lettieri C, Marini A, Pellitteri G, Surcinelli A, Valente M, et al. Clinical and neurophysiological characterization of muscular weakness in severe COVID-19. Neurol Sci. (2021) 42(6):2173–8. doi: 10.1007/s10072-021-05110-8
8. Hokkoku K, Erra C, Cuccagna C, Coraci D, Gatto DM, Glorioso D, et al. Intensive care unit-acquired weakness and positioning-related peripheral nerve injuries in COVID-19: a case series of three patients and the latest literature review. Brain Sci. (2021) 11(9):1177. doi: 10.3390/brainsci11091177
9. Vanhorebeek I, Latronico N, Van den Berghe G. ICU-acquired weakness. Intensive Care Med. (2020) 46(4):637–53. doi: 10.1007/s00134-020-05944-4
10. Molinger J, Pastva AM, Whittle J, Wischmeyer PE. Novel approaches to metabolic assessment and structured exercise to promote recovery in ICU survivors. Curr Opin Crit Care. (2020) 26(4):369–78. doi: 10.1097/MCC.0000000000000748
11. Van Aerde N, Van den Berghe G, Wilmer A, Gosselink R, Hermans G, Consortium C. Intensive care unit acquired muscle weakness in COVID-19 patients. Intensive Care Med. (2020) 46(11):2083–5. doi: 10.1007/s00134-020-06244-7
12. Herridge MS, Cheung AM, Tansey CM, Matte-Martyn A, Diaz-Granados N, Al-Saidi F, et al. One-year outcomes in survivors of the acute respiratory distress syndrome. N Engl J Med. (2003) 348(8):683–93. doi: 10.1056/NEJMoa022450
13. Dodig D, Tarnopolsky MA, Margeta M, Gordon K, Fritzler MJ, Lu JQ. COVID-19-associated critical illness myopathy with direct viral effects. Ann Neurol. (2022) 91(4):568–74. doi: 10.1002/ana.26318
14. Stevens S, Hendrickx P, Snijders T, Lambrichts I, Stessel B, Dubois J, et al. Skeletal muscles of patients infected with SARS-CoV-2 develop severe myofiber damage upon one week of admission on the intensive care unit. Appl Sci. (2022) 12(14):7310. doi: 10.3390/app12147310
15. Di Girolamo FG, Fiotti N, Sisto UG, Nunnari A, Colla S, Mearelli F, et al. Skeletal muscle in hypoxia and inflammation: insights on the COVID-19 pandemic. Front Nutr. (2022) 9:865402. doi: 10.3389/fnut.2022.865402
16. Grimm A, Teschner U, Porzelius C, Ludewig K, Zielske J, Witte OW, et al. Muscle ultrasound for early assessment of critical illness neuromyopathy in severe sepsis. Crit Care. (2013) 17(5):R227. doi: 10.1186/cc13050
17. Chan TW. Presentation of motor neuron disease in a patient with weight loss and acute-on-chronic respiratory failure. BMJ Case Rep. (2021) 14(4):e238789. doi: 10.1136/bcr-2020-238789.
18. Gonzalez NL, Hobson-Webb LD. Neuromuscular ultrasound in clinical practice: a review. Clin Neurophysiol Pract. (2019) 4:148–63. doi: 10.1016/j.cnp.2019.04.006
19. Shen J, Cartwright MS. Neuromuscular ultrasound in the assessment of polyneuropathies and motor neuron disease. J Clin Neurophysiol. (2016) 33(2):86–93. doi: 10.1097/WNP.0000000000000241
20. Duyndam A, Smit J, Heunks L, Molinger J MIJ, van Rosmalen J, van Dijk M, et al. Reference values of diaphragmatic dimensions in healthy children aged 0–8 years. Eur J Pediatr. (2023) 182(6):2577–89. doi: 10.1007/s00431-023-04920-6
21. Prado CM, Landi F, Chew STH, Atherton PJ, Molinger J, Ruck T, et al. Advances in muscle health and nutrition: a toolkit for healthcare professionals. Clin Nutr. (2022) 41(10):2244–63. doi: 10.1016/j.clnu.2022.07.041
22. van Ruijven IM, Stapel SN, Molinger J, Weijs PJM. Monitoring muscle mass using ultrasound: a key role in critical care. Curr Opin Crit Care. (2021) 27(4):354–60. doi: 10.1097/MCC.0000000000000846
23. Weijs PJM, Looijaard WGPM, Dekker IM, Memelink R, Stapel SN, Molinger J, et al. Imaging. In: Preiser J-C, Herridge M, Azoulay E, editors. Post-Intensive Care Syndrome. Cham: Springer International Publishing (2020). p. 109–24.
24. Walter U. Muscle ultrasound in clinical neurology: diagnostic uses and guidance of botulinum toxin injection. J Neurosonol Neuroimag. (2023) 15(1):38–53. doi: 10.31728/jnn.2023.00133
25. Arts IMP, Overeem S, Pillen S, Jurgen Schelhaas H, Zwarts MJ. Muscle changes in amyotrophic lateral sclerosis: a longitudinal ultrasonography study. Clin Neurophysiol. (2011) 122(3):623–8. doi: 10.1016/j.clinph.2010.07.023
26. Boon AJ, Smith J, Harper CM. Ultrasound applications in electrodiagnosis. PM R. (2012) 4(1):37–49. doi: 10.1016/j.pmrj.2011.07.004
27. Wijntjes J, van Alfen N. Muscle ultrasound: present state and future opportunities. Muscle Nerve. (2021) 63(4):455–66. doi: 10.1002/mus.27081
28. De Rosa S, Umbrello M, Pelosi P, Battaglini D. Update on lean body mass diagnostic assessment in critical illness. Diagnostics (Basel). (2023) 13(5):888. doi: 10.3390/diagnostics13050888
29. Wu H, Ding P, Wu J, Yang P, Tian Y, Zhao Q. Phase angle derived from bioelectrical impedance analysis as a marker for predicting sarcopenia. Front Nutr. (2022) 9:1060224. doi: 10.3389/fnut.2022.1060224
30. Geng J, Wei Y, Xue Q, Deng L, Wang J. Phase angle is a useful bioelectrical marker for skeletal muscle quantity and quality in hospitalized elderly patients. Medicine (Baltimore). (2022) 101(45):e31646. doi: 10.1097/MD.0000000000031646
31. Alves EAS, Salazar T, Silvino VO, Cardoso GA, Dos Santos MAP. Association between phase angle and adverse clinical outcomes in hospitalized patients with COVID-19: a systematic review. Nutr Clin Pract. (2022) 37(5):1105–16. doi: 10.1002/ncp.10901
32. Akamatsu Y, Kusakabe T, Arai H, Yamamoto Y, Nakao K, Ikeue K, et al. Phase angle from bioelectrical impedance analysis is a useful indicator of muscle quality. J Cachexia Sarcopenia Muscle. (2022) 13(1):180–9. doi: 10.1002/jcsm.12860
33. Yamada M, Kimura Y, Ishiyama D, Nishio N, Otobe Y, Tanaka T, et al. Phase angle is a useful indicator for muscle function in older adults. J Nutr Health Aging. (2019) 23(3):251–5. doi: 10.1007/s12603-018-1151-0
34. Kararmaz A, Kaya S, Turhanoglu S, Ozyilmaz MA. Effects of high-dose propofol on succinylcholine-induced fasciculations and myalgia. Acta Anaesthesiol Scand. (2003) 47:180–4. doi: 10.1034/j.1399-6576.2003.00052.x
35. Kim YS, Jeong CY, Park CJ. The effects of non - depolarizing neuromuscular blockers on the succinylcholine induced muscle fasciculation, postoperative musle pain and relaxation. Korean J Anesthesiol. (1991) 24(2):309–15. doi: 10.4097/kjae.1991.24.2.309
36. Fukumoto Y, Ikezoe T, Taniguchi M, Yamada Y, Sawano S, Minani S, et al. Cut-off values for lower limb muscle thickness to detect low muscle mass for sarcopenia in older adults. Clin Interv Aging. (2021) 16:1215–22. doi: 10.2147/CIA.S304972
37. Jiang T, Lin T, Shu X, Song Q, Dai M, Zhao Y, et al. Prevalence and prognostic value of preexisting sarcopenia in patients with mechanical ventilation: a systematic review and meta-analysis. Crit Care. (2022) 26(1):140. doi: 10.1186/s13054-022-04015-y
38. Zhang XM, Chen D, Xie XH, Zhang JE, Zeng Y, Cheng AS. Sarcopenia as a predictor of mortality among the critically ill in an intensive care unit: a systematic review and meta-analysis. BMC Geriatr. (2021) 21(1):339. doi: 10.1186/s12877-021-02276-w
Keywords: ICU-AW, SARS-coV, muscle fasciculations, muscle wasting, muscle ultrasound
Citation: Molinger J, Whittle J, Cha S, MacLeod D, Fudim M, Ulloa L, Barkauskas C, Healy Z, Haines KL, Gupta RT, Endeman H, van der Jagt M, Gommers D, Bakker J and Wischmeyer PE (2024) Prognostic significance of muscle fasciculations in critically Ill COVID-19 patients under mechanical ventilation. Front. Anesthesiol. 3:1440038. doi: 10.3389/fanes.2024.1440038
Received: 28 May 2024; Accepted: 15 August 2024;
Published: 4 September 2024.
Edited by:
Luigi La Via, Gaspare Rodolico Hospital, ItalyReviewed by:
Sebastian Isac, Carol Davila University of Medicine and Pharmacy, RomaniaAidos Konkayev, Astana Medical University, Kazakhstan
Copyright: © 2024 Molinger, Whittle, Cha, MacLeod, Fudim, Ulloa, Barkauskas, Healy, Haines, Gupta, Endeman, van der Jagt, Gommers, Bakker and Wischmeyer. This is an open-access article distributed under the terms of the Creative Commons Attribution License (CC BY). The use, distribution or reproduction in other forums is permitted, provided the original author(s) and the copyright owner(s) are credited and that the original publication in this journal is cited, in accordance with accepted academic practice. No use, distribution or reproduction is permitted which does not comply with these terms.
*Correspondence: Jeroen Molinger, jeroen.molinger@duke.edu