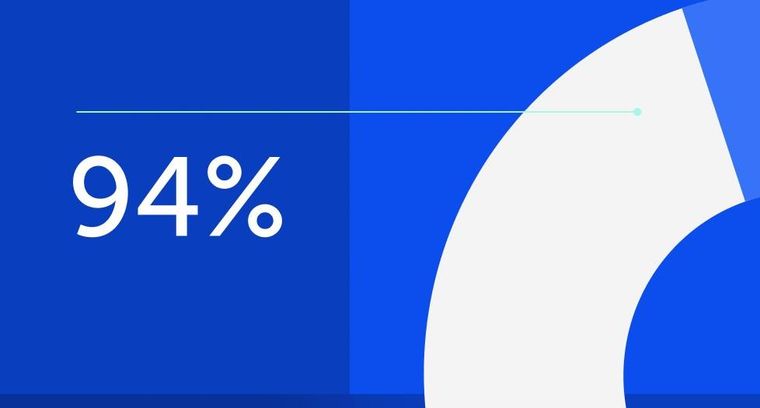
94% of researchers rate our articles as excellent or good
Learn more about the work of our research integrity team to safeguard the quality of each article we publish.
Find out more
ORIGINAL RESEARCH article
Front. Anesthesiol., 07 August 2023
Sec. Neuroanesthesiology
Volume 2 - 2023 | https://doi.org/10.3389/fanes.2023.1169961
Introduction: Intraoperative hypotension is common following general anaesthesia induction with propofol, but its impact on cerebral autoregulation (CA) remains unclear. We investigate the incidence and risk factors of impaired CApost-propofol induction and its recovery after a mean arterial pressure (mAP) challenge.
Methods: We included 40 non-emergency neuroradiology surgery patients [58 (47, 58)years old., 57% women]. We recorded mAP, mean blood flow velocity in the mean cerebral artery (MCAvmean), and regional cerebral oxygen saturation (rSO2). We computed the mean flow index (Mxa) pre and post mAP challenge. Mxa > 0.3 defined poor CA.
Results: After anaesthesia induction, 21 (53%) had impaired CBF autoregulation (CA−, Mxa > 0.3). The average mAP was 66 ± 9 mmHg, average MCAv was 39 ± 12 cm.s−1, and rSO2 was 63 ± 7%. We found no significant difference in age, norepinephrine infusion rate, and cardiovascular risks factors were similar between CA− and CA+ (Mxa ≤ 0.3) patients. Among the 22 patients (CA−: n = 14; CA+: n = 8) undergoing mAP challenge, there was a significant Mxa improvement and MCAv increase among CA− patients, (CA−: 0.63 ± 0.18 vs. 0.28 ± 0.20, p < 0.001), and [absolute variation: 1 (0.7–1.5) vs. 7 (3–9) cm.sec−1], respectively.
Conclusion: After induction of general anaesthesia for neuroradiology procedure, 53% of the patients had an impaired CA, regardless of age or medical history. Importantly, a mAP challenge effectively restored CA and improved CBF.
Clinical Trial Registration: identifier, NCT04288869
Intraoperative hypotension is associated with increased risks of postoperative complications (1), mortality (2), and can worsen pre-existing neurocognitive fragility (3). It often occurs following administration of anaesthetic drugs, particularly propofol (4). For this reason, to restore appropriate organ perfusion, a mean arterial pressure (mAP) challenge is commonly performed after anaesthesia induction. In healthy patients, intraoperative hypotension is thought to only slightly affect cerebral blood flow (CBF) due to the active mechanism of autoregulation (CA), which involves modulation of microvascular resistances.
However, this mechanism can be overridden during general anaesthesia (GA). Autoregulation is strongly influenced by the rate at which mAP changes (5). The slower the change in mAP, the smaller the impact on CBF. However, rapid changes in mAP, which are common during GA, progressively diminish the buffering capacity, leading to significant changes in CBF (6–8). Additionally, autoregulation can also be overridden for degrees of hypotension that depend on several factors such as age or patient's vascular health (9, 10), resulting in a lack of consensus on the optimal mAP target during surgery (11). Studies have shown that blood pressure alone doesn't reliably indicate brain perfusion quality (12, 13). Therefore, evaluating the effectiveness of a mAP challenge in restoring or optimising organ perfusion, especially brain perfusion, presents a significant challenge.
In a previous study (9), we demonstrated that increasing mAP using a norepinephrine bolus during GA improves cerebral perfusion. This was measured by an increase in mean blood flow velocity in the mean cerebral artery (MCAvmean) in patients with cardiovascular morbidities. In the present study, we aimed to investigate whether increasing mAP with norepinephrine restores CA, as measured by Mxa, and whether it also enhances CBF by increasing MCAvmean.
Mxa is an index defined as the correlation coefficient between mAP and MCAvmean measured in the middle cerebral artery during spontaneous, steady state changes in arterial blood pressure (14). In the context of cardiac surgeries, the Mxa index has been used to determine the optimal mAP and subsequently prevent post-operative delirium (15).
In this study, we investigated the existence of a significant prevalence of impaired CA of patients following GA induction for neuroradiology procedures. This assessment aimed to identify patients who would potentially benefit from a brain-guided hemodynamic optimisation. Our secondary objectives were to investigate the association between pre-operative variables, such as age or comorbidities, and the occurrence of a post-induction impaired CA. Furthermore, we compared changes in MCAvmean and mAP in response to a mAP challenge between patients with and without impaired CA.
This study obtained approval from the institutional board (CE SRLF 11-356) of the Société de Réanimation de Langue Française Written informed consent was waived by the IRB, and patients were provided with an information letter and gave verbal consent before anaesthesia. The study was retrospectively registered on http://www.ClinicalTrials.gov (NCT 04288869).
From February 2019 to July 2020, we conducted a prospective, observational, mono-centric study at Lariboisière Hospital (Paris, France). The study included adult patients undergoing interventional neuro-radiology surgery under general anaesthesia. Inclusion criteria were elective surgery, propofol intravenous anaesthesia, and French-speaking adults (>18 years old). Exclusion criteria were pregnancy, history of bleeding aneurysm (incidental finding following either cephalea or tinnitus complaints) or intracranial hypertension, emergency procedures for subarachnoid haemorrhage, and a Body Mass Index (BMI) >35 kg/m2 (conditioned by Schnider's model). Patient demographics were collected during the anaesthesia consultation, and Doppler signal measurements were taken on the opposite side of the head from the aneurysm location.
Cardiovascular risk factors were defined based on the concept of “early vascular aging” used in cardiology, which is related to the structural and functional state of the arterial system (16, 17). Non-modifiable risk factors included age >50 and history of a cardiovascular events, while modifiable risk factors consisted of current smoking, diabetes mellitus, dyslipidaemia, and arterial hypertension (18). Patients were classified as high-risk (Hi-risk) if they had a non-modifiable risk factor or two or more modifiable risk factors, and as low-risk (Lo-risk) if they had no or only one modifiable risk factor.
Standard monitoring, including pulse oxygen saturation (SpO2), heart rate (HR), systolic (sBP), diastolic (dBP) and mean (mAP) arterial blood pressure, temperature, and end tidal CO2 (EtCO2), along with electroencephalogram (EEG) monitoring using the Masimo Sedline monitor (SEF95, Patient State Index: PSI and burst-suppression), were recorded. Total intravenous anaesthesia was standardly induced using Remifentanil (Minto's model) followed by Propofol (Schnider's model). Atracurium besilate (0.5 mg.kg−1) was administered to induce paralysis before intubation, and mechanical ventilation was maintained with a tidal volume of 6–8 ml.kg−1. EtCO2 was maintained between 35 and 38 mmHg, FiO2 was set to 40%, and body temperature was kept between 36°C and 37°C. Propofol target-controlled infusion (TCI) was set to 5–6 µg.ml−1 for oral tracheal intubation, then reduced to 2.5–3.5 µg.ml−1 for maintenance. At any time, the anaesthesiologist in charge could adjust drug infusion rates to maintain stable hypnosis characterised by no burst suppression and a PSI between 25 and 50.
Intraoperative arterial blood pressure was continuously monitored with the Clearsight® device (Edwards Lifesciences, Irvine, CA) from the middle finger, starting before anaesthesia (19). The analog signal was transmitted to the IntelliVue MP60 monitor (Philips, Eindhoven, The Netherlands) at a 125 Hz sampling frequency and recorded using the ixTrend software (ixellence, Wildau, Germany).
Cerebral blood flow velocity was measured in the middle cerebral artery using a 1.5 MHz ultrasound Doppler probe placed on the side of the head opposite to the intervention site by the same trained physician. The probe was positioned only during anaesthesia induction and for the subsequent 30 min, but not during the neuroradiology procedure. The probe settings were power at 100 mW, gate at 9 mm for a depth of 45–55 mm, and a gain ranging from 4 to 8. Maximal velocity envelopes were acquired at a sampling rate of 100 Hz, then visualised in real time using ADMS (Atys Data Management Software), and exported as.csv file.
In this study, diluted norepinephrine at 5 µg/ml−1 was the only vasoconstrictor used. It was administered either by bolus of 10 µg or continuously via intravenous injection using syringe pumps. The infusion rate was set to maintain mAP above hypotension threshold (20). In our anaesthesia department, the intraoperative hypotension was defined as a decrease in mAP equal to or greater than 20% of its reference value. The reference value was determined in the operating room after 5 min of resting position and prior to the induction of general anaesthesia (21). Patients who experienced hypotensive episodes following induction were resuscitated with a 10 μg bolus of diluted norepinephrine at 5 µg/ml−1, followed immediately by a continuous infusion of diluted norepinephrine at 5 µg/ml−1, a rate of 40 ml/h (equivalent to 200 µg/h or 10 µg/10 min). If a continuous infusion was already ongoing, an additional increase of 40 ml/h was added.
Mxa was computed using the methodology developed by Czosnyka et al. (22), which involves calculating the Pearson correlation coefficient between mAP and MCAvmean from 30 non-overlapping epochs, each lasting 10 s. This results in a time averaging that removes pulse and respiratory frequency waveforms unrelated to cerebral autoregulation phenomena. Additionally, during these periods, mAP, propofol, and remifentanil TCI remained steady. Each Mxa evaluation yielded a single Pearson correlation coefficient.
Steady measurements of MCAvmean and mAP were collected following the induction of GA to compute the first Mxa score (Mxa1, Figure 1). Based on Mxa1, patients were separated into two groups: CA+ (Mxa1 < 0.3) and CA− otherwise. The threshold of 0.3 was based on previous studies conducted on patients with traumatic brain injury (23). Subsequently, a second Mxa score was calculated after the mAP challenge (Mxa2, Figure 1).
We analysed the changes in MCAvmean and mAP to estimate the cerebral blood flow during a mAP challenge induced by a therapeutically administered bolus followed by a continuous infusion of diluted norepinephrine. The variation in cerebral blood flow (ΔCBF) was defined as the ratio of MCAvmean and mAP changes, expressed in both absolute units (cm.s−1.mmHg−1) and percentage changes (%.mmHg−1 or %/%), facilitating comparison with other published studies. To estimate these changes, we established reference values before mAP challenge and measured the response values after the challenge, using the same inputs as for Mxa (mAP, MCAvmean). The reference values (mAPref, MCAvmeanref) were obtained by averaging signals within the 5 min time window used to compute Mxa1. The second evaluation was obtained after the mAP challenge by averaging signals within the 5 min time window used to compute the Mxa2.
A significance threshold of α = 0.05 was used for all reported statistical tests. Normally distributed data are presented as mean (standard deviation) and tested using the Shapiro-Wilk test. Non-normal distributions (resp. categorical variables) are described as median [interquartile range] IQR [resp. as count (percentage)]. Covariates were compared with Fisher, t-test or Mann-Whitney tests as appropriate, and intra-patient variable testing was done in paired fashion. Based on previous data (24), we conservatively hypothesised that more than 20% of patients undergoing general anaesthesia with propofol would exhibit poor CA following induction. Considering a power (1-β) = 0.8 and assuming normality, we estimated a minimal sample size requirement of n = 21 to detect a proportion larger than 5%. Patients exceeding this sample size requirement were included to reduce linear regression variance. The variability of the percentage was estimated using a 95% confidence interval. Areas under the curve (AUC) for receiver operating characteristic (ROC) curves were computed from logistic models. Optimal ΔCBFcut-off values were derived from the logistic models as the negative ratio between intercept and coefficient. Analyses were performed using R.
Seventy patients scheduled for non-emergency neuroradiology interventions were prospectively included. Fifteen patients were excluded due to poor bone window preventing blood flow velocity measurements, while 11 (resp. 4) patients had a poor MCAvmean (resp. mAP) signal. As a result, a total of 40 patients [age = 58(47, 68) years old, 57% women] were included for analysis (see Table 1; Figure 1).
After induction and before the mAP challenge, the average mAP in the cohort was 67(9) mmHg, with a MCAvmean of 39(12) cm.s−1 and rSO2 of 63 (7) % (Table 2). We investigated the differences in comorbidities and intraoperative data between the CA+ [Mxa ≤ 0.3, n = 19(47%)] and CA− [Mxa > 0.3, n = 21(53%)] patients. Patients in both groups had similar mAP [CA+: 70(12) vs. 63(7) mmHg, p = NS], CO2 [CA+: 32(1) vs. 33(2) mmHg, p = NS], and temperature [CA + 36.2(0.3) vs. 36.3(0.4), p = NS] during acquisition period (period B in Figure 1). Mxa calculations were performed under equivalent depths of anaesthesia, as indicated by the PSI [CA+: PSI = 28 (5) vs. 27 (6), p = NS], propofol TCI targets [CA+: 3.5(3, 3.8) vs. 3.5(3, 3.5) µg/ml, p = NS], and remifentanil targets [CA+: 3.5 (3, 3.8) vs. 3.5(3, 3.5) ng/ml, p = NS] in both groups. There were no significant differences in age between the CA− and CA+ groups (CA+: 59 [48–69] vs. 56[40, 66], p = NS). Cardiovascular risks factors (CA+: 12 (63%) vs. 16(76%), p = NS) and norepinephrine infusion rate (CA+: 0.05[0.03, 0.07] vs. 0.04[0.03, 0.06] µg.kg−1.min−1, p = NS) did not differ between the two groups (see Table 1). Interestingly, there were no significant differences in rSO2 values between the two groups [CA+: 64 (7) vs. 63(7) %, p = 0.080, Table 2]. In summary, no significant differences were observed in demographic, comorbidity, or intraoperative variables between CA+ and CA− patients, despite 53% of our patients showing post-induction impaired CA.
In our cohort, 22 patients [mean age: 56(15) years old, 12 (55%) female] required a mAP challenge following GA induction, allowing for the assessment of CA with a second Mxa (see Mxa2 in Figure 2) and calculation of ΔCBF (Figure 3). The mAP challenge resulted in an median increase in mAP of 16 [12–18] mmHg. Out of these 22 patients, 14 (64%) had an Mxa >0.3 (poor CA) following induction. In these patients, the mAP challenge resulted in a significant increase in MCAvmean [43 (12) vs. 50 (14) cm.sec−1, p < 0.001, see Table 3; Figure 4] and a significant decrease in Mxa [CA−: 0.63 (0.18) vs. 0.28 (0.20), p < 0.001, see Table 3; Figure 5]. Among the 22 patients, 8 had an Mxa < 0.3 (CA+, preserved CA) following induction. For these patients, the mAP challenge did not result in significant changes in either MCAvmean [CA+: 33 (9) vs. 34 (9) cm.sec−1, p = 0.11] or in Mxa (CA+: 0.04 (0.25) vs. 0.13 (0.39), p = NS; see Table 3; Figure 5). Notably, CA− patients had a higher ΔCBF compared to CA+ patient [CA+: percentage change ratio of 0.24 (0.09) vs. CA− 0.55 (0.24), p < 0.001, Table 3; Figure 4].
Figure 2. Schematic representation of mAP challenge following GA induction. Drop in mAP follows propofol bolus administration (induction), then mAP reaches a new stationary state (period B). During period B, mAP is stabilised at a lower level than before GA induction (period A) allowing for computation of a first Mxa value (Mxa1) to be computed. If mAP meets the hypotension criteria (i.e. a decrease in mAP equal to or larger than 20% of baseline mAP), a mAP challenge is performed. After a new stationary state in mAP is reached, a second Mxa is computed (Mxa2, period C).
Figure 3. Cerebral blood flow variation (ΔCBF). Example of ΔCBF computation for a patient presenting an impaired cerebral blood flow autoregulation from cerebral blood flow velocity (A) and mAP (B). (A) Changes in mean blood flow velocity during the mAP challenge process: averaged values of baseline MCAvmean are computed before noradrenaline administration (dashed red, Baseline) and after the administration (red cross, Response) and used to compute MCAvmean variations. (B) Changes in MAP during the same mAP challenge period as described in panel A. The obtained changes in MCAvmean (A) and mAP (B) are used to compute ΔCBF, as indicated by the ratio on the right.
Figure 4. Boxplot distributions between CA+ (blue, Mxa1 < 0.3) and CA− (red, Mxa1 > 0.3) patients. (A–B) Boxplot comparing distributions of mAP and MCAvmean between the two groups before and after the mAP challenge. A significant difference was found for MAP in both groups, whereas a significant difference was found for MCAvmean only in CA− group. (C) Boxplot comparing distributions of ΔCBF between the two groups. mAP, mean arterial pressure; MCAvmean, mean blood flow velocity flow velocity; ΔCBF, neurovascular response; Mxa, mean flow index.
Figure 5. Paired changes in Mxa before and after mAP challenge. (A) Changes in Mxa among patient with preserved CA (CA+, blue lines). The thick arrow represents the average response within the CA+ group: ΔMxa = +0.08. Two patients whose Mxa2 increased after mAP challenge are shown with red star. (B) A similar description is shown for patients with an impaired CA (CA−, grey lines), where the average response (thick arrow) within the CA− group was ΔMxa = −0.34.
We then investigated the relationship between ΔCBF and the CA state of patients following GA induction, as represented by the Mxa1 score. Our analysis revealed a linear relationship between ΔCBF and Mxa1, showing a significant positive correlation (R = 0.730, p < 0.001, Figure 6). To compare the performance of ΔCBF in identifying an impaired CA state (Mxa1 > 0.3, Figure 1), we evaluated two hypotension variables based on mAP: absolute mAP before the mAP challenge and the relative drop compared to a preoperative baseline value. Univariate logistic models of CA state (see Method) confirmed that a larger ΔCBF was significantly associated with CA− [AUC = 0.88(0.71, 0.99), p = 0.035, Figure 2B]. Interestingly, our logistic model indicated that the optimal ΔCBF value for separating CA+ from CA− patients was 0.299. However, we found no significant association using the two mAP-based variables (Figure 2B). In summary, relying solely on mAP as an absolute value or a baseline percentage to define intraoperative hypotension is not a reliable indicator for evaluating cerebral blood flow autoregulation. In contrast, ΔCBF captures post-induction CA status more accurately.
Figure 6. Association of ΔCBF with poor Mxa1. (A) Scatterplot showing a significant positive relationship between Mxa1 scores and ΔCBF (black curve, r = 0.73, p < 0.001), where blue dots are patients who required a hemodynamic optimisation. (B) Predicting CA using ΔCBF and mAP-based definition of hypotension. ROC AUC curves computed from three distinct univariate logistic models of poor Mxa1 (Mxa1 values dichotomised based on 0.3 threshold). The ROC AUC values and their confidence intervals computed from bootstrap are shown using as predictors ΔCBF (red), the average mAP during Mxa1 period (yellow) and % mAP drop from baseline considered before induction of general anaesthesia (dashed blue). AUC, area under curve; CI, confidence interval; mAP, mean arterial pressure; ΔCBF, cerebral blood flow variation index.
Following the induction of general anaesthesia for neuroradiology procedures, mAP typically decreased to an average of 67 mmHg, resulting in an impaired CA in 53% of patients. Surprisingly, no significant differences in demographics or common intraoperative variables were found between CA+ and CA− patients. A mAP challenge, motivated by insufficient mAP values, improved cerebral blood flow (as measured by MCAvmean) in patients with impaired CA. To assess the impact of the mAP challenge on cerebral blood flow and CA, we calculated the ΔCBF. The analysis indicated a strong agreement between the ΔCBF and the CA status, as determined by two successive Mxa scores.
Interpreting Mxa can pose challenges due to its considerable intra-patient variability (25). Over the past decade, consensus has emerged on the parameters used to compute the Mxa correlation coefficient (10, 15, 26). A time window of 10 s to filter out physiological events unrelated to CA (8), and the number of windows has been set at 30 (5 min) to ensure correct interpretation of the Pearson correlation statistics. A significant positive association is denoted by an Mxa above 0.3, below which correlation coefficients become increasingly inconsistent. Consequently, an Mxa of 0.3 has been established, aligning with retrospective studies where Mxa has shown strong correlations with clinical outcomes after head injury (23). Note that some studies average several successive correlations coefficients to reduce variability. Yet, even with single values for CA evaluation, Mxa appears to respond to mAP variations as expected physiologically (13).
In our study, the mAP challenge resulted in a decrease in Mxa in CA− patients, indicating that they were below their lower limit of autoregulation (LLA). Two patients from the CA+ group (those with preserved CA, Mxa ≤ 0.3) experienced a rise in Mxa above the 0.3 threshold following a mAP challenge. These 2 patients, with mAP of 95 and 100 mmHg after hemodynamic optimization, respectively were potentially above their upper limit of autoregulation (27) (Figure 3A, indicated by arrows in the upper-right corner). The ΔCBF (%/%) for these two patients were 0.3 and 0.4, which agrees with a poor CA. This highlights the necessity of testing CA at a different level of mAP, to determine whether the patient is above the upper or below the lower limit of autoregulation. Our findings revealed no significant association between Mxa1 and age or medical history, which agree with the existing literature (28, 29). This highlights the importance of considering individual variability when characterising intraoperative hypotension based on CA, instead of relying solely on population statistics. For instance, in our cohort, all patients had a neurovascular pathology, which could be a major risk factor of CA impairment. That being said, these patients might require higher blood pressure targets than typically recommended, as shown by our results on mAP challenge. We suggest integrating data on cerebral blood flow autoregulation into intraoperative hypotension definitions, providing clinicians with a tool to personalise intraoperative blood pressure management to protect the brain during general anaesthesia.
Mxa provides information about CA but does not provide direct quantification on the amplitude of CBF changes in response to a mAP challenge. However, in this study we demonstrate that static CA (measured by ΔCBF) and dynamic CA (measured by Mxa) are positively correlated (R = 0.73, p < 0.001). In other words, the more CA is altered according to the Mxa, the more CBF increases in response to a mAP increase (i.e., the more ΔCBF is high). It is an important result since studies that systematically compare static and dynamic autoregulation in the same individuals are quite rare (30, 31). Also it confirms the idea that the amplitude of CBF changes in response to an mAP challenge could be approximated and predicted by the Mxa value.
Our study has several limitations. Firstly, it is a single-centre study, and focuses exclusively on neuroradiology interventions. Therefore, further studies are needed to validate and expand upon the present results. Secondly, despite maintaining a median mAP of 67 mmHg, we observed a relatively high percentage of patients (53%) with an impaired CA after the induction of general anaesthesia. This could be attributed to the fact that our selected patient population consisted of individuals with neurovascular pathologies, such as unruptured aneurysm, arteriovenous malformations, and meningioma, which may have pre-existing alteration in CA. However, to our knowledge, there is currently no scientific evidence indicating that the presence of an unruptured aneurysmal sac affects cerebral autoregulation in the healthy hemisphere. Lastly, the study conducted by Cardim et al. found a similar proportion of patients with an Mxa > 0.3 during intraoperative hypotension induced by beach chair position (13). This suggests that our findings may have broader applicability to other patient populations.
Of the initial group of 70 eligible patients, only 40 could be analysed, largely due to issues such as missing or poor-quality signals. This highlights the practical challenge of systematically conducting such monitoring in the operating room. Moreover, the relatively small sample size limited our ability to perform multivariate analyses. It is important to remark that this study only explored CA during hemodynamic optimisation through increasing mAP. As a result, the results cannot be extrapolated to other hemodynamic scenarios, such as a decreasing mAP challenge. Indeed, previous studies have reported a hysteresis effect in brain autoregulation, where the response differs between increasing or decreasing mAP (32–34). To gain a better understanding of this effect, future studies should explore both upward and downward changes in mAP. Another limitation is that averaging signal in the five minutes after norepinephrine bolus administration might not allow enough time to the MCA diameter to stabilise. Future studies should consider a longer time frame post administration to ensure a stable measurement.
The accuracy of Mxa as a measure of CA remains unclear. However, correlation-based indices are widely used and considered as the most reliable approach for assessing static autoregulation in the literature (35). This holds particularly true for Prx, which relies on intracranial pressure and mAP. Therefore, Mxa, being a non-invasive equivalent to Prx, appears to be currently a reasonable approach for assessing CA during steady states in intra-operative settings.
We conducted an original approach for evaluating dynamic and static CA following induction of general anaesthesia. The results of our study strongly suggest that a substantial number of patients would benefit from hemodynamic optimization and underline the fact that ‘one size fits all’ is no longer admissible in patients’ care. However, to confirm these findings, future studies should investigate larger cohorts.
Our study indicates that more than 50% of patients undergoing general anaesthesia for neuroradiology procedures have impaired cerebral blood flow autoregulation. Nonetheless, we were able to demonstrate that by optimising blood pressure through mAP challenges, we could also optimise CA and CBF in this group of patients.
The minimal data set underlying the findings and conclusion of this article will be made available by the authors, without undue reservation.
The studies involving human participants were reviewed and approved by Société de Réanimation de Langue Française. The ethics committee waived the requirement of written informed consent for participation.
EM, Contribution: This author contributed to the conception, design, analysis, and interpretation of the data; drafted the manuscript; and critically revised the manuscript for content. HR, Contribution: This author contributed to the conception, design, analysis, and interpretation of the data; drafted the manuscript. JS, Contribution: This author contributed to the collection and the analysis of the data. TC, Contribution: This author contributed to structuring and gathering the data. JJ, Contribution: This author contributed to structuring and gathering the data. EG, Contribution: This author contributed to the analysis and the interpretation of the data, and critically revised the manuscript for content. FV, Contribution: This author contributed to the conception, design, analysis, and interpretation of the data; drafted the manuscript; and critically revised the manuscript for content. JC, Contribution: This author contributed to the analysis, and interpretation of the data; drafted the manuscript; and critically revised the manuscript for content. All authors contributed to the article and approved the submitted version.
Bernoulli Lab (Laboratoire commun AP-HP - Inria); Fondation pour la Recherche Assistance Publique des hôpitaux de Paris.
The authors declare that the research was conducted in the absence of any commercial or financial relationships that could be construed as a potential conflict of interest.
All claims expressed in this article are solely those of the authors and do not necessarily represent those of their affiliated organizations, or those of the publisher, the editors and the reviewers. Any product that may be evaluated in this article, or claim that may be made by its manufacturer, is not guaranteed or endorsed by the publisher.
1. Wesselink EM, Kappen TH, Torn HM, Slooter AJC, van Klei WA. Intraoperative hypotension and the risk of postoperative adverse outcomes: a systematic review. Br J Anaesth. (2018) 121(4):706–21. doi: 10.1016/j.bja.2018.04.036
2. Sessler DI, Sigl JC, Kelley SD, Chamoun NG, Manberg PJ, Saager L, et al. Hospital stay and mortality are increased in patients having a “triple low” of low blood pressure, low bispectral index, and low minimum alveolar concentration of volatile anesthesia. Anesthesiology. (2012) 116(6):1195–203. doi: 10.1097/ALN.0b013e31825683dc
3. Futier E, Lefrant JY, Guinot PG, Godet T, Lorne E, Cuvillon P, et al. Effect of individualized vs standard blood pressure management strategies on postoperative organ dysfunction among high-risk patients undergoing major surgery: a randomized clinical trial. JAMA. (10 2017) 318(14):1346–57. doi: 10.1001/jama.2017.14172
4. Su H, Eleveld DJ, Struys MMRF, Colin PJ. Mechanism-based pharmacodynamic model for propofol haemodynamic effects in healthy volunteers⋆. Br J Anaesth. (2022) 128(5):806–16. doi: 10.1016/j.bja.2022.01.022
5. Claassen JAHR, Thijssen DHJ, Panerai RB, Faraci FM. Regulation of cerebral blood flow in humans: physiology and clinical implications of autoregulation. Physiol Rev. (2021) 101(4):1487–559. doi: 10.1152/physrev.00022.2020
6. Birch AA, Dirnhuber MJ, Hartley-Davies R, Iannotti F, Neil-Dwyer G. Assessment of autoregulation by means of periodic changes in blood pressure. Stroke. (1995) 26(5):834–7. doi: 10.1161/01.STR.26.5.834
7. Diehl RR, Linden D, Lücke D, Berlit P. Phase relationship between cerebral blood flow velocity and blood pressure. A clinical test of autoregulation. Stroke 26(10):1801–4. doi: 10.1161/01.STR.26.10.1801
8. Diehl RR, Linden D, Lücke D, Berlit P. Spontaneous blood pressure oscillations and cerebral autoregulation. Clin Auton Res Off J Clin Auton Res Soc. (1998) 8(1):7–12. doi: 10.1007/BF02267598
9. Chaix I, Manquat E, Liu N, Casadio MC, Ludes P, Tantot A, et al. Impact of hypotension on cerebral perfusion during general anesthesia induction: a prospective observational study in adults. Acta Anaesthesiol Scand. (2020) 64(5):592–601. doi: 10.1111/aas.13537
10. Burkhart CS, Rossi A, Dell-Kuster S, Gamberini M, Möckli A, Siegemund M, et al. Effect of age on intraoperative cerebrovascular autoregulation and near-infrared spectroscopy-derived cerebral oxygenation. Br J Anaesth. (2011) 107(5):742–8. doi: 10.1093/bja/aer252
11. Bijker JB, van Klei WA, Kappen TH, van Wolfswinkel L, Moons KGM, Kalkman CJ. Incidence of intraoperative hypotension as a function of the chosen definition: literature definitions applied to a retrospective cohort using automated data collection. Anesthesiology. (2007) 107(2):213–20. doi: 10.1097/01.anes.0000270724.40897.8e
12. Brady K, Joshi B, Zweifel C, Smielewski P, Czosnyka M, Easley RB, et al. Real-time continuous monitoring of cerebral blood flow autoregulation using near-infrared spectroscopy in patients undergoing cardiopulmonary bypass. Stroke. (2010) 41(9):1951–6. doi: 10.1161/STROKEAHA.109.575159
13. Cardim D, Robba C, Matta B, Tytherleigh-Strong G, Kang N, Schmidt B, et al. Cerebrovascular assessment of patients undergoing shoulder surgery in beach chair position using a multiparameter transcranial Doppler approach. J Clin Monit Comput. (2019) 33(4):615–25. doi: 10.1007/s10877-018-0211-7
14. Skow RJ, Brothers RM, Claassen JAHR, Day TA, Rickards CA, Smirl JD, et al. On the use and misuse of cerebral hemodynamics terminology using transcranial Doppler ultrasound: a call for standardization. Am J Physiol Heart Circ Physiol. (2022) 323(2):H350–7. doi: 10.1152/ajpheart.00107.2022
15. Brown CH, Neufeld KJ, Tian J, Probert J, LaFlam A, Max L, et al. Effect of targeting mean arterial pressure during cardiopulmonary bypass by monitoring cerebral autoregulation on postsurgical delirium among older patients: a nested randomized clinical trial. JAMA Surg. (2019) 154(9):819. doi: 10.1001/jamasurg.2019.1163
16. Nilsson P. Early vascular aging (EVA): consequences and prevention. Vasc Health Risk Manag. (2008) 4:547–52. doi: 10.2147/VHRM.S1094
17. Kotsis V, Stabouli S, Karafillis I, Nilsson P. Early vascular aging and the role of central blood pressure. J Hypertens. (2011) 29(10):1847–53. doi: 10.1097/HJH.0b013e32834a4d9f
18. Amar J, Ruidavets JB, Chamontin B, Drouet L, Ferriéres J. Arterial stiffness and cardiovascular risk factors in a population-based study. J Hypertens. (2001) 19(3):381–87. doi: 10.1097/00004872-200103000-00005
19. Petersen NH, Ortega-Gutierrez S, Reccius A, Masurkar A, Huang A, Marshall RS. Comparison of non-invasive and invasive arterial blood pressure measurement for assessment of dynamic cerebral autoregulation. Neurocrit Care. (2014) 20(1):60–8. doi: 10.1007/s12028-013-9898-y
20. Mets B. Should norepinephrine, rather than phenylephrine, be considered the primary vasopressor in anesthetic practice? Anesth Analg. (2016) 122(5):1707–14. doi: 10.1213/ANE.0000000000001239
21. Vallée F, Passouant O, Le Gall A, Joachim J, Mateo J, Mebazaa A, et al. Norepinephrine reduces arterial compliance less than phenylephrine when treating general anesthesia-induced arterial hypotension. Acta Anaesthesiol Scand. (2017) 61(6):590–600. doi: 10.1111/aas.12905
22. Czosnyka M, Smielewski P, Kirkpatrick P, Menon DK, Pickard JD. Monitoring of cerebral autoregulation in head-injured patients. Stroke. (1996) 27(10):1829–34. doi: 10.1161/01.STR.27.10.1829
23. Sorrentino E, Budohoski KP, Kasprowicz M, Smielewski P, Matta B, Pickard JD, et al. Critical thresholds for transcranial Doppler indices of cerebral autoregulation in traumatic brain injury. Neurocrit Care. (2011) 14(2):188–93. doi: 10.1007/s12028-010-9492-5
24. Manquat E, Ravaux H, Kindermans M, Joachim J, Serrano J, Touchard C, et al. Impact of impaired cerebral blood flow autoregulation on electroencephalogram signals in adults undergoing propofol anaesthesia: a pilot study. BJA Open. (2022) 1:100004. doi: 10.1016/j.bjao.2022.100004
25. Olsen MH, Riberholt CG, Mehlsen J, Berg RM, Møller K. Reliability and validity of the mean flow index (Mx) for assessing cerebral autoregulation in humans: a systematic review of the methodology. J Cereb Blood Flow Metab. (2022) 42(1):27–38. doi: 10.1177/0271678X211052588
26. Goettel N, Patet C, Rossi A, Burkhart CS, Czosnyka M, Strebel SP, et al. Monitoring of cerebral blood flow autoregulation in adults undergoing sevoflurane anesthesia: a prospective cohort study of two age groups. J Clin Monit Comput. (2016) 30(3):255–64. doi: 10.1007/s10877-015-9754-z
27. Petersen NH, Silverman A, Strander SM, Kodali S, Wang A, Sansing LH, et al. Fixed compared with autoregulation-oriented blood pressure thresholds after mechanical thrombectomy for ischemic stroke. Stroke. (2020) 51(3):914–21. doi: 10.1161/STROKEAHA.119.026596
28. Joshi B, Ono M, Brown C, Brady K, Easley RB, Yenokyan G, et al. Predicting the limits of cerebral autoregulation during cardiopulmonary bypass. Anesth Analg. (2012) 114(3):503–10. doi: 10.1213/ANE.0b013e31823d292a
29. Laflam A, Joshi B, Brady K, Yenokyan G, Brown C, Everett A, et al. Shoulder surgery in the beach chair position is associated with diminished cerebral autoregulation but No differences in postoperative cognition or brain injury biomarker levels compared with supine positioning: the anesthesia patient safety foundation beach chair study. Anesth Analg. (2015) 120(1):176–85. doi: 10.1213/ANE.0000000000000455
30. Tiecks FP, Lam AM, Aaslid R, Newell DW. Comparison of static and dynamic cerebral autoregulation measurements. Stroke. (1995) 26(6):1014–9. doi: 10.1161/01.STR.26.6.1014
31. Strebel S, Lam AM, Matta B, Mayberg TS, Aaslid R, Newell DW. Dynamic and static cerebral autoregulation during isoflurane, desflurane, and propofol anesthesia. Anesthesiology. (1995) 83(1):66–76. doi: 10.1097/00000542-199507000-00008
32. Willie CK, Tzeng YC, Fisher JA, Ainslie PN. Integrative regulation of human brain blood flow: integrative regulation of human brain blood flow. J Physiol. (2014) 592(5):841–59. doi: 10.1113/jphysiol.2013.268953
33. Tzeng YC, Willie CK, Atkinson G, Lucas SJE, Wong A, Ainslie PN. Cerebrovascular regulation during transient hypotension and hypertension in humans. Hypertension. (2010) 56(2):268–73. doi: 10.1161/HYPERTENSIONAHA.110.152066
34. Aaslid R, Blaha M, Sviri G, Douville CM, Newell DW. Asymmetric dynamic cerebral autoregulatory response to cyclic stimuli. Stroke. (2007) 38(5):1465–9. doi: 10.1161/STROKEAHA.106.473462
Keywords: cerebral autogregulation, mean arterial pressure challenge, transcranial Doppler, anesthesia, mean flow index
Citation: Manquat E, Ravaux H, Souilamas J, Chamoux T, Joaquim J, Gayat E, Vallée F and Cartailler J (2023) Cerebral autoregulation and cerebral blood flow response to mean arterial pressure challenge following induction of general anaesthesia for neuroradiology procedure. Front. Anesthesiol. 2:1169961. doi: 10.3389/fanes.2023.1169961
Received: 20 February 2023; Accepted: 24 July 2023;
Published: 7 August 2023.
Edited by:
Fabio Silvio Taccone, Université libre de Bruxelles, BelgiumReviewed by:
Umeshkumar Athiraman, Washington University in St. Louis, United States© 2023 Manquat, Ravaux, Souilamas, Chamoux, Joaquim, Gayat, Vallée and Cartailler. This is an open-access article distributed under the terms of the Creative Commons Attribution License (CC BY). The use, distribution or reproduction in other forums is permitted, provided the original author(s) and the copyright owner(s) are credited and that the original publication in this journal is cited, in accordance with accepted academic practice. No use, distribution or reproduction is permitted which does not comply with these terms.
*Correspondence: Elsa Manquat ZWxzYS5tYW5xdWF0QGdtYWlsLmNvbQ==
†These authors have contributed equally to this work
Disclaimer: All claims expressed in this article are solely those of the authors and do not necessarily represent those of their affiliated organizations, or those of the publisher, the editors and the reviewers. Any product that may be evaluated in this article or claim that may be made by its manufacturer is not guaranteed or endorsed by the publisher.
Research integrity at Frontiers
Learn more about the work of our research integrity team to safeguard the quality of each article we publish.