- School of Earth, Atmospheric and Life Sciences, University of Wollongong, Wollongong, NSW, Australia
Reproductive technologies are increasingly being adopted to improve the conservation management of threatened species. The storage and transport of sperm is a vital aspect of the practical implementation of reproductive technologies, however, bacterial contamination during the storage and transport of sperm samples presents a biosecurity risk and can contribute to a reduction in sperm longevity during storage. The present study: 1) characterised the urinary microbiome (bacterial species composition and abundance) using culture-independent 16S rRNA sequencing; 2) quantified the effect of various doses of gentamicin and streptomycin-penicillin on bacterial abundance (colony-forming units; CFUs) and; 3) quantified the effect of antibiotic supplementation on the sperm viability (proportion live/dead) of spermic urine during a 12-day cold-storage period, using the common eastern froglet, Crinia signifera. Overall, urine samples were found to host a diverse array of bacteria, dominated by the phyla Proteobacteria, Firmicutes, and Bacteroidetes. Bacterial abundance was significantly reduced in all antibiotic treatment groups compared to the control group. Antibiotic supplementation had no effect on sperm viability between day 0 and day 6 of storage, however both antibiotic treatments significantly improved sperm viability from days 9 to 12 of storage compared to the control group. Overall, the results of this study provide novel insight into the urinary microbiome, being the first to use a metagenomics approach to characterise the bacterial community present in the urine of an amphibian. Importantly, this study provides evidence that antibiotic supplementation with either gentamicin or streptomycin-penicillin, minimises bacterial proliferation and improves sperm viability during cold storage. These findings will contribute to the development of biosecurity protocols aimed at reducing the risk of disease transmission and cross-infection from unwanted bacteria and infectious agents in amphibian captive breeding programs.
1 Introduction
Species declines are impacting a diversity of taxa globally (IUCN, 2022). Though the cause of biodiversity loss varies among taxa, common drivers include habitat degradation and fragmentation, pollution, climate-change, and accelerated global movement of living organisms (including humans and agricultural animals), all of which have contributed to an increased emergence of infectious disease (Daszak et al., 2001; Aguirre and Tabor, 2008). One example of a catastrophic infectious disease, is the amphibian Chytrid fungus, caused by the fungal pathogen Batrachochytrium dendrobatidis (Bd), which is considered the worst wildlife disease in recorded history, having contributed to the decline of at least 500 amphibian species globally (Scheele et al., 2019). In response to this threat, controlling infectious disease remains a priority for both in situ and ex situ conservation efforts, and has resulted in the creation of specialised protocols to maximise biosecurity (Murray et al., 2011; Pessier and Mendelson, 2017). Whilst these protocols aim to minimise contamination through hygiene protocols implemented into wildlife husbandry and whole animal transport, there has been far less emphasis on minimising potential contaminants in the transport and storage of genetic material (including sperm/semen suspensions), despite sample biosecurity being routine practise within commercial livestock and aquaculture industries (Contreras et al., 2020, Contreras et al., 2022).
Reproductive technologies (RTs), which include a suite of tools aimed at improving reproductive outcomes, such as hormone therapies to induce gamete release, biobanking and assisted fertilisation (AF, also referred to as artificial fertilisation or in vitro fertilisation), are increasingly being recognised for their value in assisting wildlife conservation (Cseh and Solti, 2000; Pukazhenthi and Wildt, 2004; O’Brien et al., 2009; Silla and Byrne, 2019; Holt and Comizzoli, 2021; Anastas et al., 2023). The integration of RTs has the potential to bolster propagation, improve genetic management, and facilitate the establishment of repositories of genetic resources (known as genome resource banks, GRBs) (Lueders and Allen, 2020; Holt and Comizzoli, 2021; Silla and Kouba, 2022). It has been proposed that the use of RTs may function in a similar way to a wildlife corridor, with the collection, storage and transport of germplasm from both ex situ and in situ populations facilitating gene flow among otherwise isolated populations (Bennett, 2001; Holt and Comizzoli, 2021; Byrne and Silla, 2022). However, the collection and transport genetic material also brings with it the risk of introducing novel bacteria, parasites and infectious diseases. Infectious diseases can impact population dynamics and viability, whilst also compromising the welfare of infected individuals (Kirkwood and Colenbrander, 2001). There is evidence of a diversity of infectious agents that may be present within sperm suspensions and several accounts of the transmission of infectious disease through artificial insemination (Kirkwood and Colenbrander, 2001; Maes et al., 2008; Givens, 2018).
Across externally fertilising taxa, including several species of fish and amphibians, sperm may be exposed to cutaneous bacteria, internal bacteria and environmental bacteria, and each provides a potential source of contamination. In addition to sperm becoming contaminated, there is increasing evidence that a dynamic reproductive microbiome may exist within the reproductive tract in a range of species (Comizzoli and Power, 2019; Molina et al., 2021; Contreras et al., 2023). Bacteria present within sperm samples may contain pathogens or other novel bacteria that can disrupt the host-associated microbiomes of other individuals, including both conspecifics and heterospecifics, that may come into contact with them during transport or use in AF (Jani and Briggs, 2014). In addition to this, bacterial contamination of sperm samples can directly affect sperm longevity during storage in a range of taxa, and therefore limit subsequent AF success, by inducing morphological damage, reducing motility and fertility, and increasing DNA fragmentation (Stoss and Refstie, 1983; Saad et al., 1988; Christensen and Tiersch, 1996; Sanocka-Maciejewska et al., 2005; Haines et al., 2013; Nimrat et al., 2022). Therefore, controlling the proliferation of bacteria within sperm samples may be required to both minimise the risk of disease transmission and increase the potential for sperm storage (Silla et al., 2015), and this may be achieved by supplementing the sample with antibiotics.
Antibiotic supplementation is routinely used in the aquaculture industry to control bacterial contamination in sperm samples, with a streptomycin-penicillin combination the most widely used antibiotic (Contreras et al., 2020), in addition to gentamicin (Segovia et al., 2000; Zidni et al., 2023). Antibiotics such as these are used as they are classed as broad-spectrum antibiotics, which are effective at controlling the proliferation of both gram-positive and gram-negative bacteria (Ory and Yow, 1963). Previous studies have indicated that a diverse array of bacteria may be present within sperm samples (Andrabi et al., 2016; Contreras et al., 2023), and thus broad-spectrum antibiotics are required. Both sperm motility and viability has been shown to be maintained significantly longer during storage with the addition of antibiotics compared to without in a range of commercially valuable fish (Stoss and Refstie, 1983; Saad et al., 1988; Christensen and Tiersch, 1996; Segovia et al., 2000; Boonthai et al., 2016; Rahimi et al., 2016; Zidni et al., 2023) and invertebrate species (Nimrat et al., 2005, 2022). For example, in the critically endangered Caspian brown trout (Salmo trutta caspius), sperm motility and longevity was improved in sperm samples stored in a medium containing streptomycin-penicillin compared to the antibiotic-free control for the duration of storage (Rahimi et al., 2016). Furthermore, the reduction of bacterial abundance has been demonstrated with antibiotic supplementation (Nimrat et al., 2022), minimising biosafety concerns associated with bacterial contamination.
In amphibians, the effect of antibiotic supplementation has only been tested in five studies across four frog species, with equivocal results (Anastas et al., 2023). Of these studies, sperm was sourced from testes extraction and maceration (Love, 2011; Silla et al., 2015), from spermic urine following hormonal induction of spermiation (Germano et al., 2013; Kaurova et al., 2022), or from both sources (Keogh et al., 2017). Where testes extraction and maceration is performed, it is recommended that sterile dissection equipment and suspender solutions are used to reduce bacterial contamination. However, bacterial contamination can still occur from contact with tissues and bodily fluids, particularly from the bladder and intestine if these organs are perforated during dissection and removal of the testes (Keogh et al., 2017). In addition to sample contamination, there is potential for the testes to harbour their own unique bacterial signature, as was recently found to be the case within human testes (Molina et al., 2021). Obtaining sperm as spermic urine, following the hormonal induction of spermiation (see Silla and Langhorne, 2022), is increasingly being applied to threatened species, but this method of sperm collection exposes sperm samples to additional sources of bacterial contamination. Sperm pass through the cloaca, where they are exposed to bacteria from the gut, faeces and the environment, resulting in comparatively higher levels of bacterial contamination compared to sperm obtained as testes macerates (Germano et al., 2013; Keogh et al., 2017). Antibiotic supplementation is therefore even more imperative in controlling bacteria during the storage of spermic urine samples.
When considering antibiotic supplementation, appropriate doses must be determined. The optimal dose of antibiotic is likely to depend on several factors, including the source of the sperm samples (testes maceration versus spermic urine), and the source of the animal that sperm are extracted from (captive versus wild), both of which will influence the amount of bacterial contamination in the sperm suspension (Anastas et al., 2023). In addition to the abundance of bacteria in the sperm sample influencing the dose of antibiotic required to reduce or eliminate bacteria, species-specificity in the resilience of sperm to the potential toxic effects of antibiotics may influence the optimal antibiotic dose to use to maintain or enhance sperm quality during storage. Results of the previous studies have suggested that high concentrations of antibiotics may exhibit toxic effects on sperm (Silla et al., 2015; Keogh et al., 2017; Kaurova et al., 2022), likely due to impaired mitochondrial function (Segovia et al., 2000). However, the addition of comparatively lower doses may have beneficial outcomes. For example, in the European common frog (Rana temporaria), treatment of sperm samples with gentamicin at a concentration of 0.04 mg/mL resulted in sperm motility being maintained for a minimum of 32 days, compared to 12 days for untreated samples (Kaurova et al., 2022). Although, the effects of antibiotic treatment appear to vary between species. In the Booroolong frog (Litoria booroolongensis), comparatively moderate doses of gentamicin (1-2 mg/mL) resulted in no effect on sperm motility during storage compared to untreated samples. Importantly, however, antibiotic treatment significantly reduced bacterial abundance (Silla et al., 2015; Keogh et al., 2017). There may also be an effect of antibiotic type, with sperm longevity negatively affected by the addition of streptomycin-penicillin to sperm samples in Fowler’s toad (Anaxyrus baxteri) (Germano et al., 2013). Irrespective of the variable effect of antibiotics on sperm performance and viability, the potential for antibiotic supplementation to minimise bacterial proliferation is an important consideration in order to minimise the risk of disease transfer. To date, however, bacterial abundance during storage has only been quantified in a single published study (Keogh et al., 2017). Additionally, no study to date has identified bacterial species present within samples, which may be important for determining the most appropriate antibiotic type and dose to use to control the diversity of bacterial species present.
The present study aimed to: 1) characterise the urinary microbiome (identify bacterial species composition and abundance) using culture-independent 16S rRNA sequencing; 2) quantify the effect of various doses of gentamicin and streptomycin-penicillin on bacterial abundance and; 3) quantify the effect of antibiotic supplementation on sperm viability during cold storage of spermic urine samples. The study was conducted using the common eastern froglet, Crinia signifera as a model species.
2 Methods
2.1 Ethical note
All procedures were performed following approval by the University of Wollongong’s Animal Ethics Committee (approval number AEPR22/12) in accordance with the Australian Code for the Care and Use of Animals for Scientific Purposes. Animals were collected under NSW Scientific Licence SL 102672.
2.2 Study species
The common eastern froglet is a small (14 to 29 mm in snout-vent length) ground-dwelling Australian frog species of the family Myobatrachidae (Figure 1A). During breeding, which may occur throughout the year but mainly occurs in Austral autumn, winter and spring (March to November), males aggregate in shallow ephemeral water bodies (typically at the edge of creeks and streams), and attract females using advertisement calls (Anstis, 2017). Eggs are deposited onto vegetation or substrate, and fertilisation occurs when males release sperm at the site of egg deposition during amplexus (Byrne et al., 2003).
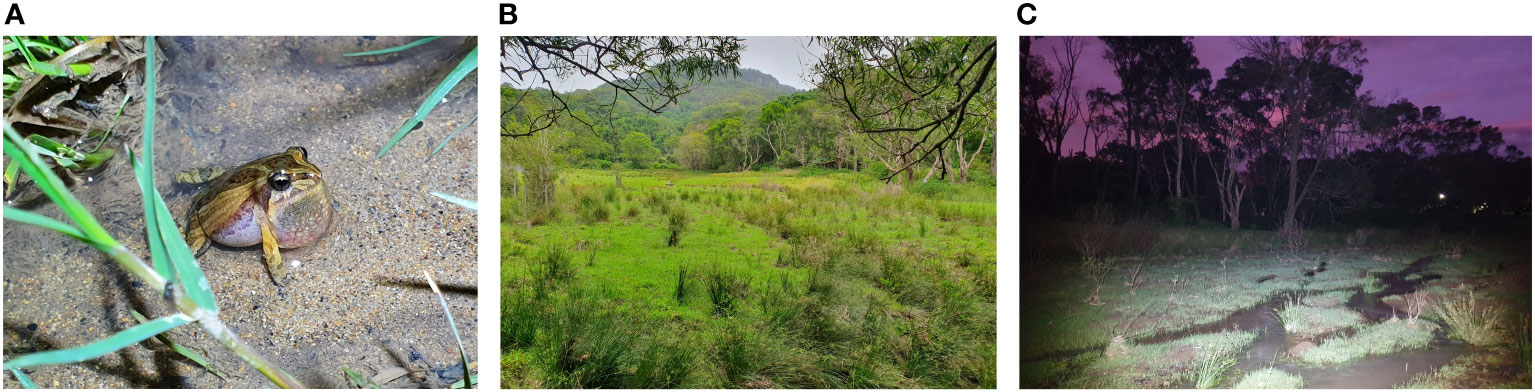
Figure 1 (A) Adult male common eastern froglet, Crinia signifera; vocalisations were observed prior to the collection of males. (B, C) study site located at the University of Wollongong, NSW. Photographs courtesy of Aimee J. Silla.
2.3 Study site and animal collection
All frogs were collected from a single, large population, located adjacent to the Ecological Research Centre (ERC) at the University of Wollongong (34.4048° S, 150.8717° E) (Figures 1B, C). This site is a known breeding site for our study species, and is characterised by shallow ephemeral water bodies surrounded by remnant riparian vegetation.
Collections occurred on October 19 and 26, 2022, during Austral spring after periods of light rainfall when calling activity increased. All collections were performed at night, between 17:00 and 21:00 hours. To eliminate the risk of cross-contamination of bacteria from captured individuals, latex examination gloves were worn at all times, and changed between individuals. Frogs were caught by hand after tracking their vocalisations (Figure 1A), and each individual was then confirmed to be male by checking for the presence of a darkened vocal sac. Immediately after capture, frogs were placed in individual sealed plastic zip-lock bags containing 2-3 Kimwipes (bags were pre-prepared prior to collecting any individuals). At the time of collection, the Kimwipes were wetted with water from the exact sight of capture of each individual. At the end of the collection period all frogs were immediately transported on foot to an isolated constant-temperature room in the ERC. The room was located within a biosecurity corridor, and no other individuals of any species were housed in the corridor during the study period.
2.4 Animal husbandry
Frogs were housed in the ERC at the University of Wollongong, Australia. To maintain independence and eliminate cross-contamination of bacteria, frogs remained isolated and were housed in individual terraria (27 x 17 x 16 cm) with an open-lattice plastic lid for adequate air supply. Each terraria contained 2 cups aquarium gravel, a 300 mL rectangular plastic water dish (10 x 6 x 5 cm), and a section of PVC piping (3cm in diameter, 5cm long) placed on the gravel. Additionally, equally sized pieces of plastic aquarium plant and gutter guard were placed in the water dish to allow the frogs to move in and out of the water. For drainage, each terrarium had 8 holes (~0.5cm in diameter) in the base. One side of each terrarium was covered in black dampcourse (16 cm × 9 cm) to prevent visual contact between males and the possibility that male–male interactions would influence sperm production or sperm performance (Lüpold et al., 2017; Magris, 2021).
Frogs were kept in a constant temperature room maintained at 22°C, simulating the average maximum temperature for the study area in October (data obtained from Bellambi weather station from 1997-2021 (BOM 2021)). An artificial 13: 11 hour light: dark cycle was maintained to simulate natural conditions during spring in the Illawarra region. Broad spectrum UV light was supplied by Reptisun 36” high output fluorescent strip bulbs (Pet Pacific Pty Ltd, Sydney) suspended approximately 20cm above the terrariums. During their time in captivity, all frogs were monitored daily. Frogs were fed a diet of two hatchling (first instar) live crickets (Archeta domestica) once per week, with food withheld for three days prior to urine collection to reduce faecal contamination of samples. To prevent metabolic bone disease caused by a calcium and/or vitamin D3 deficient diet, crickets were dusted with approximately 200 mg of calcium powder with D3 (Repti calcium; Zoo Med, USA) immediately prior to feeding (Ferrie et al., 2014). To prevent the build-up of waste, terrariums were completely flushed with reverse osmosis (RO) water (Sartorius Stedim biotech, Australia) (~1000 mL) once a week. Additionally, water was changed in all water dishes in each terrarium every three to four days, and water dishes were topped up with RO water on days that water was not changed. Frogs remained in captivity for a maximum of 14 days after which time all frogs were returned to the field at the site of collection, with the exception of those used in experiment three as they were euthanised as part of experimental procedures.
2.5 Experiment one: characterisation of the urinary microbiome (bacterial composition and abundance)
To identify the composition and abundance of bacterial species within urine, urine samples were collected from 14 individual male frogs within 4-5 days of collection from the field. Urine was collected from 14 male frogs according to protocols previously developed (Silla and Roberts, 2012), whereby a fire-polished microcapillary tube was inserted into the cloaca to stimulate urination. Each urine sample was absorbed onto a sterile dry rayon-tip swab (CLASSIQ swabs 167KS01, Copan Diagnostics, Inc., USA), placed in a 1.5 mL Eppendorf tube, and stored in a -80°C freezer until sample processing (microbiome extraction and profiling). Five negative controls (sterile rayon-tip swabs) were similarly stored and submitted for processing. Frozen samples were stored for 23 weeks before being transported on dry ice to the Australian Genome Research Facility in Urrbrae, South Australia for genome sequencing and species identification. DNA extraction was then performed using the DNeasy® 96 PowerSoil® Pro Kit (QIAGEN) as per the manufacturer’s instructions, for manual high-throughput isolation of microbial genomic DNA. PCR amplification was then performed, and PCR amplicons were generated. The V3–V4 region of the bacterial 16S rRNA gene was amplified using 341F (CCTAY GGG RBG CAS CAG) and 806R (GGACT ACN NGG GTA TCTAAT) primers. Conditions were as follows; cycle 30, initial 98°C for 30 sec, disassociate 98°C for 10 sec, anneal 60°C for 10 sec, extension 72°C for 30 sec, finish 72°C for 5 min. The first stage PCR was cleaned using magnetic beads, and samples were visualised on 2% Sybr Egel (Thermo-Fisher). A secondary PCR to index the amplicons was performed with Platinum SuperFi II mastermix (Life Technologies, Australia). The resulting amplicons were cleaned again using magnetic beads, quantified by fluorometry (Promega Quantifluor) and normalised. The eqimolar pool was cleaned a final time using magnetic beads to concentrate the pool and then measured using a High-Sensitivity D1000 Tape on an Agilent 2200 TapeStation. The pool was diluted to 5nM and molarity was confirmed again using a Qubit High Sensitivity dsDNA assay (ThermoFisher). This was followed by sequencing on an Illumina MiSeq (San Diego, CA, USA) with a V3, 600 cycle kit (2 x 300 base pairs paired-end).
Output generated included absolute abundance and relative abundance of bacterial species in each sample, identified using the SILVA rRNA database.
2.6 Experiment two: effect of antibiotic treatment on bacterial abundance in urine
2.6.1 Experimental design
To test the effect of various doses of antibiotics on bacterial abundance in urine, a split-sample experimental design was used, whereby a total of ten urine samples were evenly divided among seven experimental treatments (1, 2 or 3 mg mL-1 gentamicin, 0.5 mg mL-1 + 500 IU mL-1, 1 mg mL-1 + 1000 IU mL-1 or 1.5 mg mL-1 + 1500 IU mL-1 streptomycin-penicillin, and a ‘no antibiotics’ control) (Figure 2). Antibiotic concentrations were selected based on previous research (Silla et al., 2015; Keogh et al., 2017). Urine was collected from 20 male frogs according to methods described in section 2.5. Urine was collected every one to two hours for four hours, and was pooled for each individual. Samples were kept on ice between collection times. At the end of the urine collection period, the urine from two individuals was pooled to generate 10 unique urine samples, and the final volume of each urine sample ranged from 46 – 58 μL. Each urine sample (n=10) was then homogenised by gently agitating the Eppendorf tube, and split into seven 5 μL subsamples. Each sub-sample was then diluted in 45 μL of 1:4 simplified amphibian Ringer (SAR) containing varying concentrations of either gentamicin or streptomycin + penicillin such that the final concentration in each subsample was 1, 2 or 3 mg mL-1 gentamicin, or 0.5 mg mL-1 + 500 IU mL-1, 1 mg mL-1 + 1000 IU mL-1 or 1.5 mg mL-1 + 1500 IU mL-1 streptomycin-penicillin. The control treatment (no antibiotics) was diluted with 45 μL 1:4 SAR. The dilution ratio for each sub-sample was then 1:10.
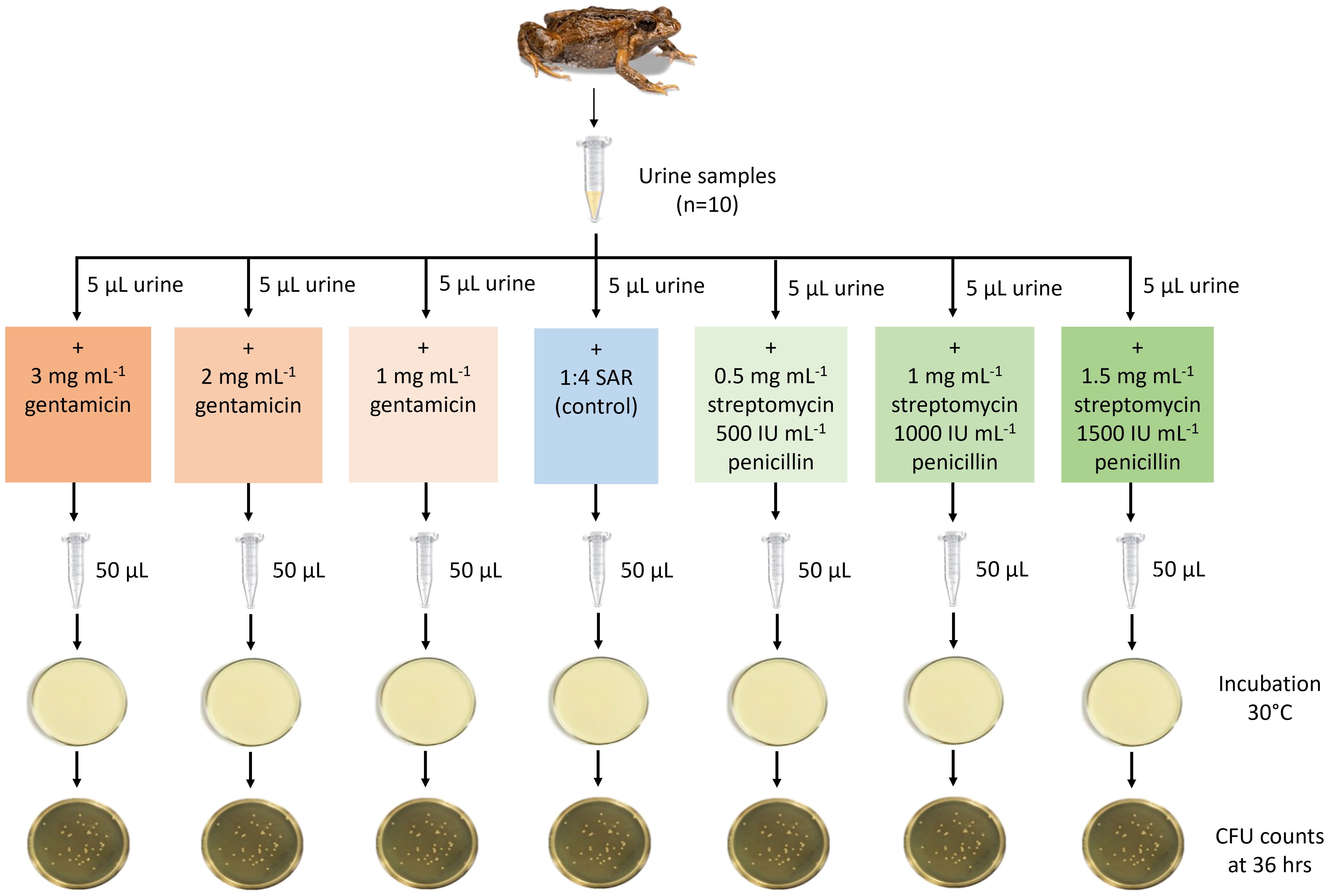
Figure 2 Experimental design used to test the effect of antibiotic treatment on bacterial abundance (CFU) in urine. Shown is a split-sample experimental design, whereby urine samples (n = 10) were split between seven experimental antibiotic treatments (1, 2 or 3 mg mL-1 gentamicin, 0.5 mg mL-1 + 500 IU mL-1, 1 mg mL-1 + 1000 IU mL-1 or 1.5 mg mL-1 + 1500 IU mL-1 streptomycin-penicillin, and a ‘no antibiotics’ control). Samples were plated on nutrient agar and bacterial abundance (colony forming units, CFU) determined after an incubation period of 36 hours at 30°C. Figure courtesy of Aimee J. Silla.
2.6.2 Assessment of bacterial abundance
To determine bacterial abundance, each 50 μL subsample was spread on an individual nutrient agar plate (WestLab, Australia). The subsample was deposited onto the plate using a pipettor and disposable pipette tip. Plates were mounted on a dial rotating at a constant speed, and the sample was spread in a counter-clockwise direction from a marked start point for one full rotation using a disposable plastic spreader. Plates were then incubated at 30°C, and after 36 hours photographs were taken of each plate using a backlight to help illuminate individual colonies. The number of colony forming units (CFU) was determined for each plate using the manual count function in ImageJ software. For antibiotic treatment plates, CFU counts were determined by counting the total number of colonies that formed on the plate in its entirety. Due to the high density of colonies on the control plates, CFU counts for the control treatment plates were determined by evenly dividing each control plate image into four quarters using the program ImageJ, and counts were taken in the quadrant diagonally opposite from the starting-point quadrant. This count was then multiplied by four to give a CFU count for the entire plate. For each plate the CFU mL-1 was calculated by multiplying the CFU by the dilution factor. To ensure that any bacterial growth was the result of the sample added and not due to plate contamination or the methods used, an additional five plates were concurrently incubated without the addition of any sample, and after 36 hours incubation there was negligible bacterial growth (three plates with 0 CFU per plate, two plates with 1-2 CFU per plate). Plating for experiment two was conducted on October 31, 2022.
2.7 Experiment three: effect of antibiotic treatment on spermic urine storage
2.7.1 Experimental design
To test the effect of antibiotics on the storage of spermic urine, a split-sample experimental design was used, whereby sperm suspensions from 10 males were evenly divided among three experimental treatments (1 mg mL-1 gentamicin, 1 mg mL-1 + 1000 IU mL-1 streptomycin-penicillin and a control treatment with no antibiotics added) (Figure 3). The antibiotic doses were chosen based on the outcome of experiment two above. Urine was collected from male frogs (n=10) using a microcapillary tube as described above. Urine was collected every 1.5 hours for a total of seven hours, and was pooled across time points for each individual. Samples were kept on ice between collection times. Final volumes pooled within an individual ranged from 20 to 175 μL. Following urine collection, frogs were weighed to the nearest 0.001g (range = 0.595 to 0.849 g, mean ± SEM = 0.706 ± 0.00587, n=10) and photographed to measure snout-vent length (mm) using the program ImageJ. Snout-vent was measured from the apex of the head to the cloaca (range = 20.41 to 23.43 mm, mean ± SEM = 21.62 ± 0.0752 mm, n=10). Immediately after frogs were weighed and photographed, they were euthanised via double-pithing (destruction of the brain and brain stem) and both testes were removed by dissection and weighed to the nearest 0.0001 grams (range = 0.0005 to 0.0018 g, mean ± SEM = 0.0011 ± 0.0000315 g, n=10). Testes were then macerated in 60 μL of urine of the corresponding frog, except in the case of two frogs where the total urine volumes obtained were less than the required 60 μL volume, and instead testes were macerated in 50% own urine and 50% different frog urine. This method of macerating testes within urine was used to simulate spermic urine (normally collected following hormonal stimulation; see Silla and Langhorne (2022). This method was chosen to ensure high concentrations of sperm for the accurate assessment of sperm viability at each time-point. After the imitation 'spermic urine' samples were generated, these sperm suspensions were homogenised and split into three 20 μL sub-samples, which were then diluted in 25 μL of 1:1 SAR containing either no antibiotic, gentamicin or streptomycin-penicillin, such that the final concentration in each subsample was either no antibiotic (control), 1 mg mL-1 gentamicin, or 1 mg mL-1 + 1000 IU mL-1 streptomycin penicillin.
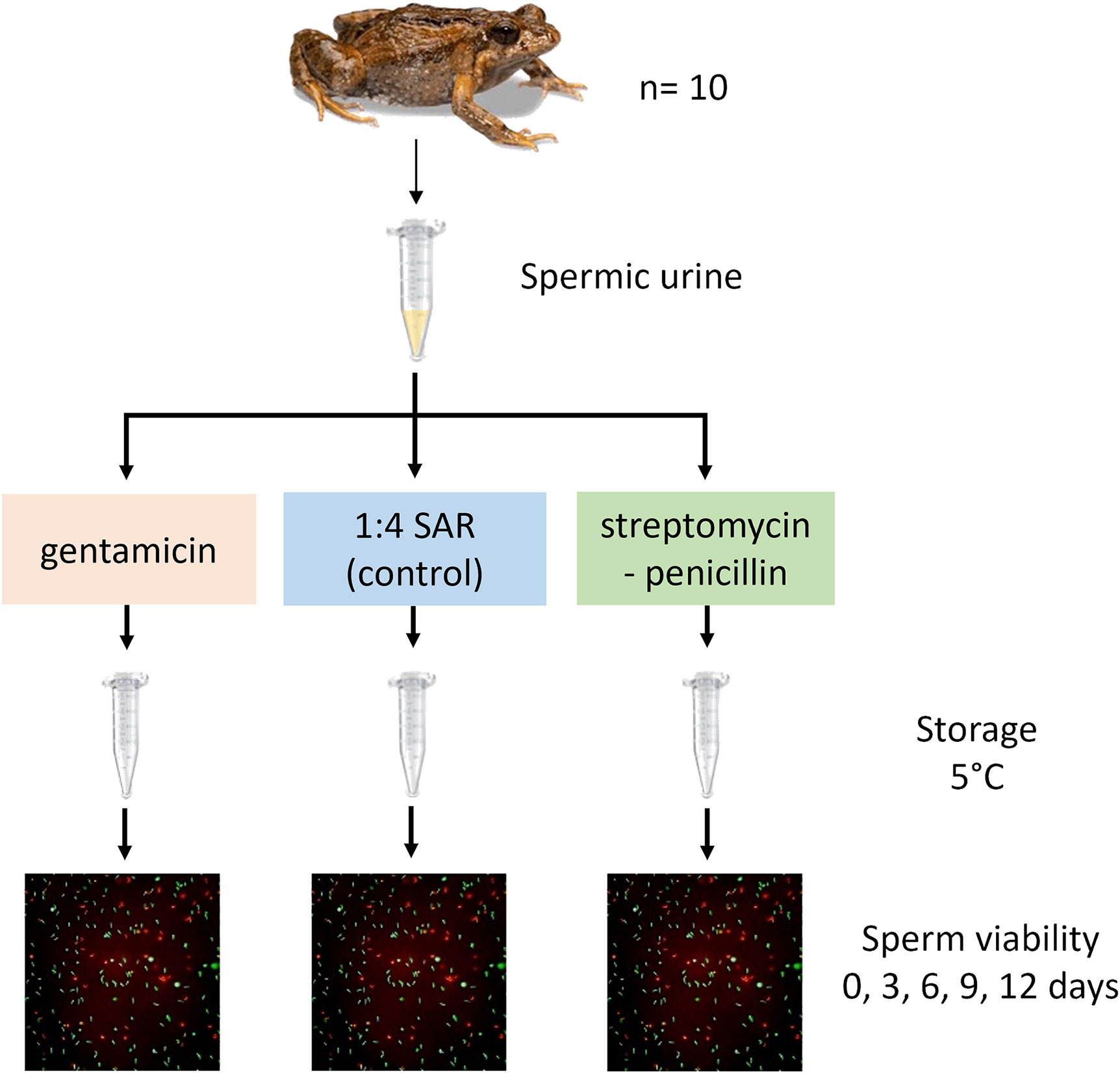
Figure 3 Experimental design used to test the effect of antibiotic treatment on percent sperm viability over a 12-days sperm storage period. Shown is a split-sample experimental design, whereby a spermic urine sample from each male (n=10) was split between three experimental treatments (1 mg mL-1 gentamicin, 1 mg mL-1 + 1000 IU mL-1 streptomycin-penicillin and a ‘no antibiotics’ control) and cold-stored to test the effect of antibiotic treatment on sperm viability over 12 days of storage. Figure courtesy of Aimee J. Silla.
For each sperm suspension subsample, sperm concentration was quantified using an Improved Neubauer Haemocytometer (Bright line, Optik Labor, Germany). Briefly, a 2 μL aliquot was taken from each sub-sample, diluted in 7 μL 1:1 SAR, homogenised and loaded into a haemocytometer chamber using a pipette. The number of sperm cells present within 25 quadrats was recorded and used to calculate sperm concentration, and this count was repeated twice per suspension and averaged. Sperm concentration in each subsample ranged from 6.53 x 105 to 1.56 x 107 sperm cells mL-1 (mean ± SEM = 4.78 x 106 ± 0.65 x 106 sperm cells mL-1; n=10). All sperm suspensions were stored in capped 1.5mL Eppendorf tubes and refrigerated at 5°C for a total of 12 days, with viability assessments occurring on days 0, 3, 6, 9 and 12 of storage and bacterial abundance assessments occurring on days 0, 6 and 12 of storage. Sperm suspensions were uncapped and gently agitated for 10 seconds every day during the 12-day storage period, to aerate the suspensions and reduce sperm sedimentation (Germano et al., 2013; Silla et al., 2020).
2.7.2 Assessment of sperm viability
To assess sperm viability, a live/dead sperm viability assay was conducted, following procedures previously described (Silla, 2013). Briefly, a 2 μL aliquot of each sperm suspension was mixed with 5 μL of a 1:50 dilution of SYBR-14, followed by 2 μL of propidium iodide (Invitrogen, L-7011). The sample was incubated in the dark for 7 minutes following the addition of each solution, before being pipetted into a haemocytometer chamber. The haemocytometer was then mounted on a fluorescent microscope, and sperm viability was determined by counting sperm cells fluorescing green as viable (live), and those fluorescing red were considered non-viable (dead). To ensure accurate viability data, counts were performed for a minimum total of 100 sperm cells, and from this the percent viability was determined for each sample. This experiment was conducted from November 8 to November 20, 2022, and all viability assessments were conducted between 13:00 and 18:00.
2.8 Statistical analysis
To evaluate the effect of antibiotic treatment on bacterial abundance in urine, a Linear mixed-effects (LME) model was used. Within the model, treatment (1, 2 or 3 mg mL-1 gentamicin, 0.5 mg mL-1 + 500 IU mL-1, 1 mg mL-1 + 1000 IU mL-1 or 1.5 mg mL-1 + 1500 IU mL-1 streptomycin-penicillin, or control [no antibiotic added]) was a fixed factor, and the response variable was CFU mL-1, with frog ID included as a random effect to account for repeated sampling of the same individuals across the experiment. To evaluate the effect of antibiotic treatment on sperm viability and bacterial abundance in sperm suspensions during storage, two separate LME models were conducted with either percent sperm viability or CFU mL-1 as the response variable. In each model, treatment (1 mg mL-1 gentamicin, 1 mg mL-1 - 1000 IU ml-1 streptomycin-penicillin and control [no antibiotic added]), storage time, and the interaction between treatment and storage time were added as fixed effects, and frog ID was included as a random effect. In models where significant effects were detected, post hoc comparisons were made using Tukey-Kramer Honestly Significantly Different (HSD) tests. Prior to analysis, CFU mL-1 was Log10 transformed (after the addition of 0.0001 to all values), and percent sperm viability data was arcsine square root (sin-1 [√x]) transformed, due to violations of normality as determined by a Shapiro-Wilk goodness of fit test. Prior to analysis, regression models were conducted between the response variables (CFU mL-1 or sperm viability) and frog mass (g), frog snout-vent length (mm), testes mass (g) and sperm concentration. No significant correlations were found. As such, these variables were not included in any subsequent statistical models. All statistical analyses were performed using JMP Pro 17.0 software package (SAS Institute Inc. North Carolina, USA). For all tests in this study, statistical significance was accepted at P < 0.05.
3 Results
3.1 Experiment one: characterisation of the urinary microbiome (bacterial composition and abundance)
Overall, a total of 160 unique bacterial species were isolated from urine samples of 14 males (Supplementary Table S1). Species within the genera Burkholderia-Caballeronia-Paraburkholderia, Coprobacillus, [Eubacterium] fissicatena group and Ruminiclostridium 9, and within the families Desulfovibrionaceae, Enterobacteriaceae, Erysipelotrichaceae, Lachnospiraceae and Ruminococcaceae were the most prevalent, occurring in approximately 79-93% of samples (n = 11-13/14) (Supplementary Table S1). The most abundant phylum was Proteobacteria (39%), followed by Firmicutes (37%), Bacteroidetes (12%), Deferribacteres (5%), Actinobacteria (3%) and Verrucomicrobia (3%), with other phyla constituting approximately 0.7% relative abundance (Figure 4). The most abundant taxonomic order was Clostridiales (32%), and the following five most abundant taxonomic orders were Desulfovibrionales, Bacteroidales, Enterobacteriales, Betaproteobacteriales and Rickettsiales, each constituting between 7 and 12% relative abundance.
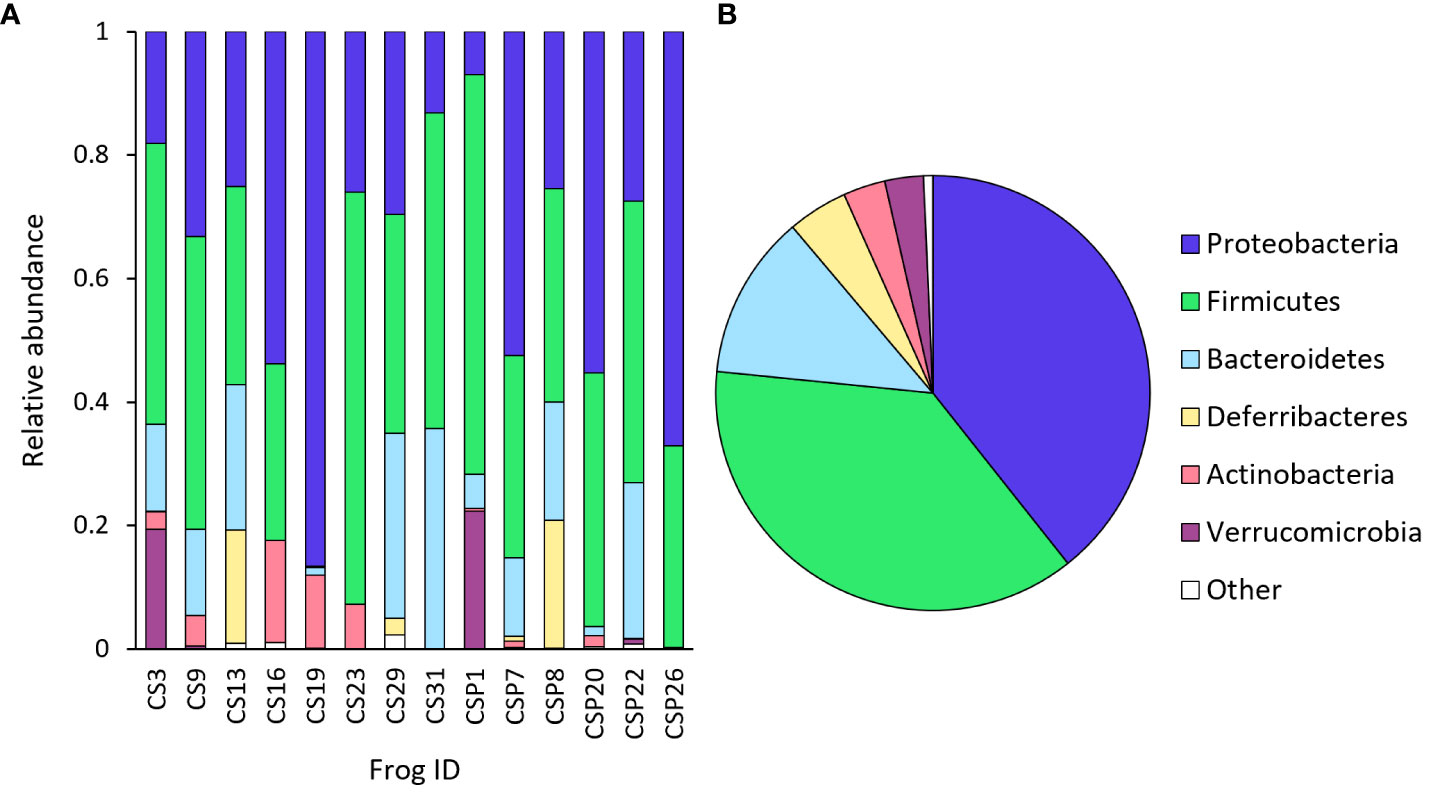
Figure 4 Relative abundance of dominant bacteria phyla identified from urine samples from frogs, showing (A) taxonomic composition per sample (individual frog), and (B) mean taxonomic composition across all samples (n=14).
3.2 Experiment two: effect of antibiotic treatment on bacterial abundance in urine
There was a significant effect of antibiotic treatment on bacterial abundance (CFU mL-1) (LME; F6, 54 = 19.480, P < 0.0001), whereby bacterial abundance was significantly lower in all antibiotic treatments (1, 2 or 3 mg mL-1 gentamicin and 0.5 mg mL-1 + 500 IU mL-1, 1 mg mL-1 + 1000 or 1.5 mg mL-1 + 1500 IU mL-1 streptomycin-penicillin) compared to the control treatment after incubation for 36 hours (Table 1). Whilst bacterial abundance was statistically similar among antibiotic treatments, bacterial abundance was lowest at a concentration of 1 mg mL-1 among gentamicin treated samples, and was lowest at a concentration of 1 mg mL-1 – 100 IU mL-1 among streptomycin-penicillin treated samples (Table 1).
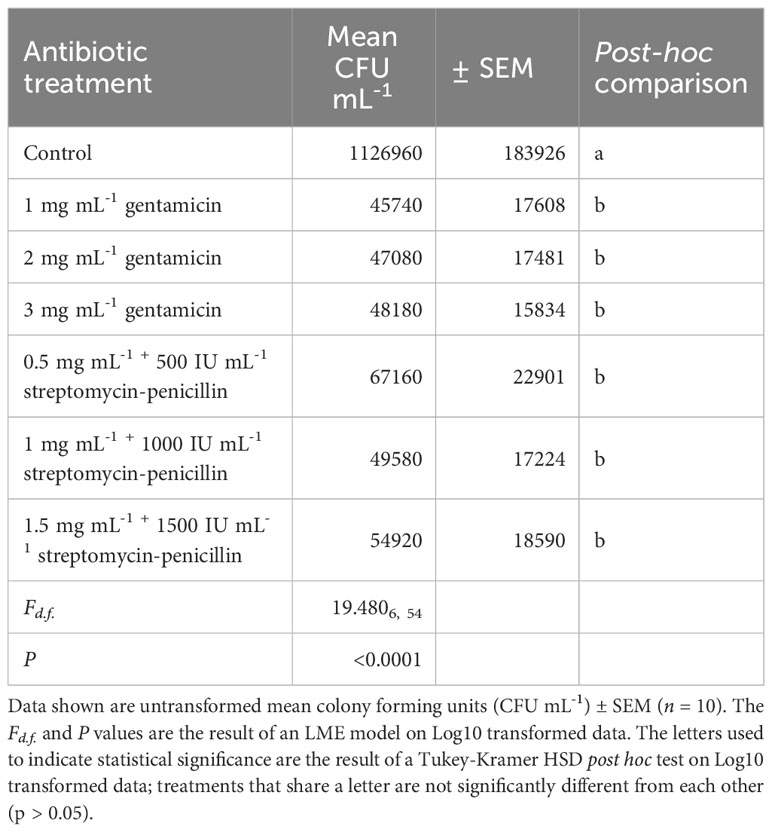
Table 1 Effect of antibiotic treatments (1, 2 or 3 mg mL-1 gentamicin and 0.5 mg mL-1 + 500 IU mL-1, 1 mg mL-1 + 1000 or 1.5 mg mL-1 + 1500 IU mL-1 streptomycin-penicillin) versus a control treatment (no antibiotics added) on bacterial abundance in urine after 36 hours of incubation.
3.3 Experiment three: effect of antibiotic treatment on sperm viability in spermic urine during storage
Percent sperm viability significantly declined over the 12-day storage period (LME; F4, 126 = 78.9, P <0.0001) in all treatments (Figure 5). Overall, treatment did not have a significant effect on percent sperm viability (LME; F4, 126 = 0.0199, P = 0.9803), but there was a significant treatment by time interaction (LME; F8, 126 = 3.86, P < 0.0001). The percent sperm viability of samples was similar on days 0, 3 and 6, after which time, treatment means diverged and on days 9 and 12 samples treated with either gentamicin (1 mg mL-1) or streptomycin-penicillin (1 mg mL-1 + 1000 IU mL-1) had significantly higher sperm viability compared to control samples which did not receive antibiotics (Tukey HSD < 0.05; Figure 5). By the end of the storage period, percent sperm viability in the control treatment was less than 10%, while samples treated with antibiotics retained over 30% viability (Figure 5).
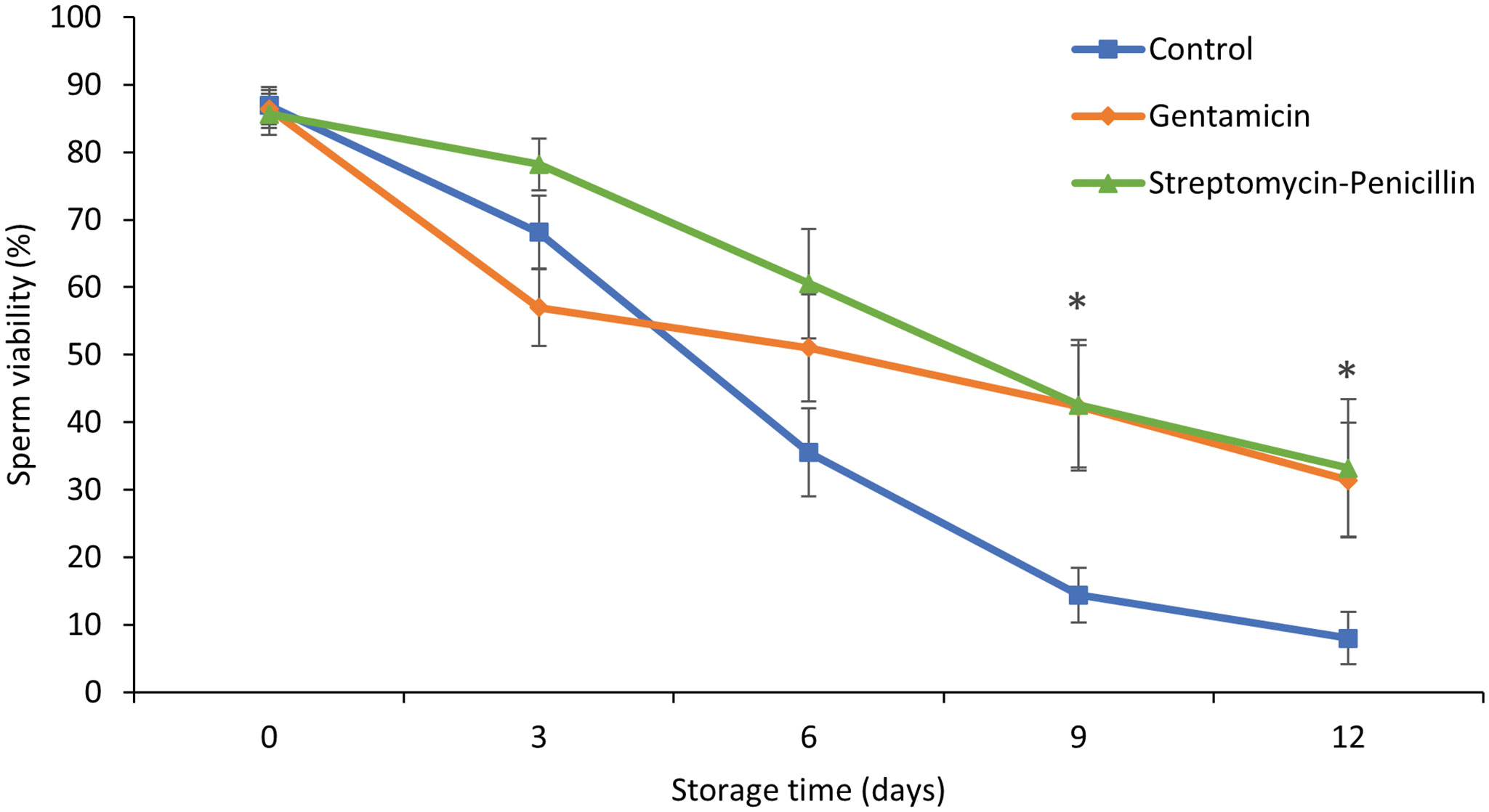
Figure 5 Effect of antibiotic treatment (1 mg mL-1 gentamicin, 1 mg mL-1 + 1000 IU ml-1 streptomycin-penicillin, or no antibiotic control; n=10 sperm suspensions per treatment) on percentage sperm viability over 12 days of cold storage. Data shown are untransformed mean % sperm viability ± SEM. The symbol '*' denotes storage days where treatments are significantly different (p > 0.05) according to Tukey-Kramer HSD post hoc tests on arcsine transformed percent viability data.
4 Discussion
Sperm samples across a range of taxa have been found to contain a diversity of bacteria. The presence of bacteria in sperm samples is of concern for two reasons; 1) because of the risk of disease transmission and cross-infection from unwanted bacteria and infectious agents, and 2) because of the deleterious effects of bacteria on sperm quality and capacity for fertilisation. Despite this, the addition of antibiotics to amphibian sperm samples to control bacterial proliferation has previously only been studied in four species (Booroolong frog, European common frog, Fowler’s toad and Southern brown tree frog). Furthermore, characterisation of the amphibian urinary microbiome was yet to be determined for any species, despite the routine collection of spermic urine of amphibians. The present study: 1) characterised the urinary microbiome (bacterial species composition and abundance); 2) quantified the effect of various doses of gentamicin and streptomycin-penicillin on bacterial abundance and; 3) quantified the effect of antibiotic supplementation on sperm viability during cold storage of spermic urine samples, from the common eastern froglet. Overall, whilst bacterial composition and relative abundance differed among individuals, the phyla Proteobacteria (39%) and Firmicutes (37%) in combination made up over 75% of the relative abundance across sampled individuals. As predicted, antibiotic supplementation resulted in a significant reduction in bacterial abundance across treatments. However, antibiotic type and dose did not have an effect on the magnitude of bacterial inhibition. Furthermore, antibiotic supplementation with either gentamicin or streptomycin-penicillin improved sperm viability from nine to twelve days of cold storage compared to the antibiotic-free control.
4.1 Characterisation of the urinary microbiome
In the present study, the urinary microbiome of an amphibian was characterised for the first time, adopting a metagenomic approach. This approach is increasingly being used over traditional culturing methods because it allows for the identification of a broad spectrum of species, including otherwise underrepresented, difficult-to-culture species (Contreras et al., 2023). The bacterial communities identified within urine samples in the present study were found to contain a diverse array of both gram-negative and gram-positive bacterial species, with 160 unique species identified across 74 families, and 16 phyla. The dominant bacterial taxa reported herein, including Proteobacteria, Firmicutes and Bacteroidetes, have similarly been found to be among the dominant phyla present in the cutaneous microbiomes (Becker et al., 2014; Bataille et al., 2016; Bates et al., 2018) and gut microbiomes (Mashoof et al., 2013; Zhou et al., 2020) of other amphibian species. In anurans, Wolffian ducts serve as the urogenital tract and thus transport both sperm and urine into the cloaca (Oielska, 2009). Therefore, the amphibian reproductive, excretory, and digestive systems are all inherently connected, and so too by extension are the microbiomes present within each. Additionally, there are opportunities for exchange of microbiota between the cutaneous and gut microbiomes, with gut bacteria excreted through the cloaca serving as a source of cutaneous bacteria (Wiggins et al., 2011), and cutaneous bacteria ingested during feeding, including the ingestion of their own shed skin, serving as a source of gut bacteria (Colombo et al., 2015; Tong et al., 2020). As such, there may be similarities between the microbiomes of the cloaca, gut and skin.
In amphibians, it has been shown that cutaneous and gut microbiomes are dynamic and shaped by a number of abiotic and biotic factors including, but not limited to, diet and nutrition, contact with conspecifics and heterospecifics, habitat type, seasonality or climatic conditions (Jiménez and Sommer, 2017; Tong et al., 2020). Of these extrinsic factors, environmental conditions imposed during captivity are increasingly being recognised as a driver of microbiome disruption and loss of bacterial diversity (Loudon et al., 2014; Bataille et al., 2016; Kueneman et al., 2016; Sabino-Pinto et al., 2016; Passos et al., 2018; Bates et al., 2019; Hernández-Gómez et al., 2019; Korpita et al., 2023). Whilst the urinary microbiome of both wild and captive individuals is yet to be characterised and compared for any amphibian species, the same trend as has been reported for the gut and skin microbiomes is expected. In following, sperm sampled from wild amphibians (as spermic urine) is predicted to be a source of high bacterial species richness and abundance, and therefore poses a high risk of introducing novel bacterial species, and along with this, novel pathogens, to other populations or captive colonies. Following, bacterial abundances reported herein (mean 11.3 x 105 ± 1.84 x 105 CFU mL-1), were two orders of magnitude higher than those reported by Keogh et al. (2017), where the mean initial bacterial abundance within spermic urine samples of the Booroolong frog was 0.72 x 105 ± 0.143 x 105 CFU mL-1. Differences in the bacterial abundance reported is likely linked to the source of study animals, with male frogs in the present study collected from the wild, while Keogh et al. (2017) employed F1 Booroolong frogs that were bred and reared in captivity. The introduction of novel bacteria and pathogens can result in disease in the recipient population not only through direct transmission, but also through the alteration of recipient microbiomes which can compromise immunity (Redford et al., 2012). It is therefore recommended that strict biosecurity measures, including the use of antibiotic treatment, be implemented when collecting, storing, and transporting sperm samples to minimise this risk.
4.2 Effect of antibiotic supplementation on bacterial abundance within urine
Overall, unsupplemented urine samples exhibited high bacterial abundance, compared to samples supplemented with antibiotics, which exhibited bacterial abundances several orders of magnitude lower across all treatments. Whilst bacteria were not completely eliminated from any samples in the present study, the reduction in abundance was significant across all antibiotic treatments, with gentamicin and streptomycin-penicillin exhibiting similar effects, suggesting both are appropriate in reducing the bacteria present within amphibian urine samples. Both gentamicin and streptomycin-penicillin are broad-spectrum antibiotics that are known to be effective against a range of gram-positive and gram-negative bacteria, as were identified in our study. A past study similarly found that treatment of amphibian sperm samples with antibiotics reduces bacterial abundance, with the addition of gentamicin at concentrations ranging 2 to 4 mg mL-1 to spermic urine samples resulting in a significant decrease in CFU mL-1 in the Booroolong frog (Keogh et al., 2017). In contrast to our study, Keogh et al. (2017) found that a lower antibiotic dose (1 mg mL-1 gentamicin) was less effective at reducing bacterial abundance than higher doses (2, 3, or 4 mg mL-1). Our study found that antibiotic treatment resulted in statistically similar bacterial abundance regardless of antibiotic type or concentration. Whilst not significantly different, it was found that relatively low or moderate doses of antibiotics resulted in the greatest reduction in bacterial abundance, at concentrations of 1 mg mL-1 gentamicin and 1 mg mL-1 + 1000 IU ml-1 streptomycin-penicillin, respectively. As bacterial responses to antibiotics are dose-dependent, with a minimum dose required to have lethal effects on bacteria (Bernier and Surette, 2013), results of this study suggest that low to moderate doses are sufficient to reduce bacterial proliferation within urine samples.
4.3 Effect of antibiotic supplementation on sperm longevity
Antibiotic supplementation was found to improve sperm longevity, as significantly higher sperm viability was maintained in treated samples at 9- and 12- days of cold storage. Similar results on the beneficial effects of antibiotic supplementation on sperm longevity have also been reported in several fish species (Stoss and Refstie, 1983; Saad et al., 1988; Christensen and Tiersch, 1996; Segovia et al., 2000; DeGraaf and Berlinsky, 2004; Rahimi et al., 2016; Zidni et al., 2023), a shrimp species (Nimrat et al., 2022) and another amphibian (Kaurova et al., 2022). In a study on the European common frog (Rana temporaria), the effects of several antibiotic types on sperm motility were tested. The study reported that the addition of enrofloxacin, a combination antibiotic, and gentamicin at doses ranging 0.03 to 0.4 mg mL-1, all significantly increased sperm motility throughout cold storage (Kaurova et al., 2022). The greatest improvement to sperm longevity was observed following the addition of gentamicin at 0.1 mg mL-1, with greater than 80% motility maintained for 30 days of storage, whereas sperm were completely immotile by day 14 within the antibiotic-free control treatment (Kaurova et al., 2022). In contrast, studies in the Booroolong frog, Fowler’s toad and the Southern brown tree frog have reported no effect of antibiotic supplementation on sperm longevity during cold storage (Love, 2011; Germano et al., 2013; Silla et al., 2015; Keogh et al., 2017). There may be several explanations for the beneficial effect of antibiotic supplementation on sperm longevity observed in the present study. Firstly, the bacterial load within the present study was comparatively high, and this is likely to have exacerbated the detrimental effects of bacterial contamination on sperm. It is known that bacteria in high concentrations compete with sperm for resources such as oxygen (Stoss and Refstie, 1983), and this competition may have resulted in sample aeration being insufficient to replenish oxygen required for sperm cell functioning over the storage period within the untreated control samples. Additionally, a relatively high ratio of bacteria to sperm cells increases the chances of bacteria adhering to sperm cells and causing deleterious effects (Auroux et al., 1991; Yániz et al., 2010; Keogh et al., 2017).
A second explanation for improved sperm longevity is that the doses tested were effective in minimising bacteria without compromising sperm functioning. Based on the current literature, high doses of antibiotics have been found to be toxic to sperm, whereas low doses may be insufficiently effective (Kaurova et al., 2022). Whilst the present study cannot ascertain whether the antibiotic doses administered exerted toxic effects on the sperm, the results indicate that an overall net benefit was achieved through the minimisation of bacterial proliferation. Additionally, whilst not significant, this study found that sperm samples supplemented with streptomycin-penicillin had a higher proportion of viable sperm on days three and six of storage compared to gentamicin, possibly indicating that this antibiotic type is comparatively less toxic. However, by day nine of storage, both antibiotic types were equally effective at maintaining sperm viability. Future studies should aim to quantify sperm quality alongside measures of bacterial abundance throughout the storage period.
4.4 Implications for amphibian conservation
With the increasing implementation of reproductive technologies to improve ex situ amphibian conservation, decreasing the risk of disease transmission is of paramount importance. Furthermore, the collection of sperm samples from wild populations to capture valuable genetic material and facilitate genetic exchange between captive and wild populations is being increasingly emphasised (Silla and Kouba, 2022). In following, the risk of disease transmission and cross-infection from unwanted bacteria and infectious agents is of heightened concern, and thus there is an urgent need to establish rigorous biosecurity protocols for amphibian sperm suspensions.
Results of the present study suggest that antibiotic supplementation has the two-fold benefit of both reducing bacterial abundance (and therefore potentially lowering the risk of disease transfer) and improving sperm longevity, both of which have important implications for the success of conservation breeding programs. This beneficial effect of antibiotic supplementation may also be true for other amphibian species, as discussed above, particularly in species or populations that have high bacterial diversity and abundance. Biosecurity protocols that effectively control bacteria in sperm suspensions are not only important for short-term cold storage, but also for the long-term biobanking of samples within genome resource banks (GRBs), as many bacterial species survive the cryopreservation process (Murray et al., 2016) and continue to pose a risk to biosecurity. With cryopreserved sperm samples increasingly being transferred between facilities for genetic management purposes (Kouba and Julien, 2022), reducing the risk of the introduction of novel bacteria is of equal importance in this case.
While biosecurity measures are of utmost importance for reducing the risk of disease transmission, we also recognise that the reduction of bacteria, through antibiotic supplementation and aseptic techniques, is indiscriminate and can also lead to the elimination of beneficial bacteria. It is becomingly increasingly apparent that amphibian microbiomes play a role in host immunity, with higher bacterial diversity and the presence of disease-inhibiting bacterial species associated with higher host survival (Harris et al., 2009; Ellison et al., 2019; Harrison et al., 2019). In particular, various bacterial species have been found to have antifungal properties and are reported to be effective at inhibiting Bd infection (Becker et al., 2009; Harris et al., 2009; Kueneman et al., 2016; Ellison et al., 2019). The inoculation of amphibian hosts with probiotics (bioaugmentation) therefore serves as a means to successfully restore bacterial diversity and introduce beneficial bacteria to improve host immunity (Bletz et al., 2013). Bioaugmentation has successfully been applied to a range of amphibian species to improve outcomes, and inoculation has occurred through dietary supplementation (Pereira et al., 2017, 2018), through exposure to bacterial isolates (Kueneman et al., 2016), or through direct or indirect horizontal transmission among inoculated individuals (Rebollar et al., 2016). This method has the potential to additionally be employed in offspring produced through AF where sperm samples are treated with antibiotics, to introduce beneficial probiotic bacteria that may have otherwise been eliminated. Due to the increasing threat of disease to amphibian biodiversity, we recommend that biosecurity measures are implemented to reduce or eliminate bacteria within sperm samples, followed by targeted inoculation of embryos/offspring with beneficial bacteria to restore bacterial diversity. As an alternative to inoculation with bacterial isolates, the microbiomes of embryos/offspring can also potentially be reinvigorated through transmission from conspecifics, or when introduced to source inoculum naturally (through reintroductions or exposure to natural substrates) (Rebollar et al., 2020; Korpita et al., 2023).
4.5 Conclusions
In conclusion, urine from the common eastern froglet was found to host a diverse array of bacterial species, and the addition of both gentamicin and streptomycin-penicillin at varied doses was effective at reducing bacterial proliferation. Antibiotic supplementation was also shown to improve sperm viability during cold storage. This study is the first to use metagenomics to characterise the bacterial community of amphibian urine, and only the second to show that antibiotic supplementation can improve amphibian sperm longevity. Our results highlight the benefit of antibiotic supplementation for minimising bacteria within sperm samples and improving sperm viability during storage. The findings of this study will contribute to the development of biosecurity protocols aimed at reducing the risk of disease transmission and cross-infection from unwanted bacteria and infectious agents, which will benefit amphibian captive breeding programs globally.
Data availability statement
The bacterial identification and relative abundance data presented in this study are deposited in the DRYAD data repository: Silla, Aimee; Anastas, Zara; Byrne, Phillip (2024). Characterisation and relative abundance of bacteria present in the urine of a frog [Dataset]. Dryad. https://doi.org/10.5061/dryad.vt4b8gv0b. All other original data contributions presented in this study are included in the article/Supplementary Material. Further inquiries can be directed to the corresponding author.
Ethics statement
The animal study was approved by the University of Wollongong’s Animal Ethics Committee (AEPR22/12) and authorised by New South Wales National Parks and Wildlife Service (SL102672). The study was conducted in accordance with the local legislation and institutional requirements.
Author contributions
AS was responsible for conceptualization of the study, acquired animal ethics approval and the scientific license. AS and PB were responsible for funding acquisition and supervision. AS and ZA collected the study animals, and ZA was responsible for animal monitoring and husbandry. AS prepared all antibiotic solutions, collected the urine samples, generated sperm suspensions, and collected all sperm viability data. ZA cultured the bacteria plates and collected the CFU data. ZA and PB analysed the data. ZA wrote the manuscript with contributions from AS and PB. Each author contributed to the review and editing of the manuscript and approved the submitted version.
Contributions Summary: ZA: Data curation, Formal analysis, Investigation, Writing – original draft, Writing – review & editing. PB: Conceptualization, Formal analysis, Funding acquisition, Resources, Supervision, Visualization, Writing – review & editing. AS: Conceptualization, Data curation, Funding acquisition, Investigation, Methodology, Project administration, Resources, Supervision, Visualization, Writing – review & editing.
Funding
The author(s) declare financial support was received for the research, authorship, and/or publication of this article. This study was funded by a grant from the Australian Research Council, Discovery Early Career Researcher Award (DE210100812) awarded to AS. The study was conducted while ZA was in receipt of an Australian Government Research Training Program (AGRTP) Scholarship.
Acknowledgments
The authors would like to acknowledge the Wodi Wodi people as the Traditional Custodians and knowledge holders of the lands on which this research was conducted and acknowledge their connection to Country.
Conflict of interest
The authors declare that the research was conducted in the absence of any commercial or financial relationships that could be construed as a potential conflict of interest.
Publisher’s note
All claims expressed in this article are solely those of the authors and do not necessarily represent those of their affiliated organizations, or those of the publisher, the editors and the reviewers. Any product that may be evaluated in this article, or claim that may be made by its manufacturer, is not guaranteed or endorsed by the publisher.
Supplementary material
The Supplementary Material for this article can be found online at: https://www.frontiersin.org/articles/10.3389/famrs.2024.1329888/full#supplementary-material
References
Aguirre A. A., Tabor G. M. (2008). ‘Global factors driving emerging infectious diseases. Ann. N. Y. Acad. Sci. 1149, 1–3. doi: 10.1196/annals.1428.052.
Anastas Z. M., Byrne P. G., O’Brien J. K., Hobbs R. J., Upton R., Silla A. J. (2023). ‘The increasing role of short-term sperm storage and cryopreservation in conserving threatened amphibian species. Animals 13, 2094. doi: 10.3390/ani13132094.
Andrabi S. M. H., Khan L. A., Shahab M. (2016). ‘Isolation of bacteria in semen and evaluation of antibiotics in extender for cryopreservation of buffalo (Bubalus bubalis) bull spermatozoa. Andrologia 48, 1166–1174. doi: 10.1111/and.2016.48.issue-10.
Auroux M. R., Jacques L., Mathieu D., Auer J. (1991). ‘Is the sperm bacterial ratio a determining factor in impairment of sperm motility: an in-vitro study in man with Escherichia coli. Int. J. Androl. 14, 264–270. doi: 10.1111/j.1365-2605.1991.tb01091.x.
Bataille A., Lee-Cruz L., Tripathi B., Kim H., Waldman B. (2016). Microbiome variation across amphibian skin regions: implications for chytridiomycosis mitigation efforts. Microb. Ecol. 71, 221–232. doi: 10.1007/s00248-015-0653-0.
Bates K. A., Clare F. C., O’Hanlon S., Bosch J., Brookes L., Hopkins K., et al. (2018). ‘Amphibian chytridiomycosis outbreak dynamics are linked with host skin bacterial community structure’, Nat. Commun. vol. 9 no. 1 p, 693. doi: 10.1038/s41467-018-02967-w
Bates K. A., Shelton J. M. G., Mercier V. L., Hopkins K. P., Harrison X. A., Petrovan S. O., et al. (2019). Captivity and infection by the fungal pathogen batrachochytrium salamandrivorans perturb the amphibian skin microbiome’. Front. Microbiol. 10, 1834. doi: 10.3389/fmicb.2019.01834.
Becker M. H., Brucker R. M., Schwantes C. R., Harris R. N., Minbiole K. P. C. (2009). Bacterially produced metabolite violacein is associated with survival of amphibians infected with a lethal fungus’. Appl. Environ. Microbiol. 75, 6635–6638. doi: 10.1128/AEM.01294-09.
Becker M. H., Richards-Zawacki C. L., Gratwicke B., Belden L. K. (2014). The effect of captivity on the cutaneous bacterial community of the critically endangered Panamanian golden frog (Atelopus zeteki). Biol. Conserv. 176, 199–206. doi: 10.1016/j.biocon.2014.05.029.
Bennett P. M. (2001). “Establishing animal germplasm resource banks for wildlife conservation: genetic, population and evolutionary aspects,” in Cryobanking the genetic resource. Eds. Watson P. F., Holt W. V. (Taylor & Francis, London, UK), 47–68.
Bernier S. P., Surette M. G. (2013). Concentration-dependent activity of antibiotics in natural environments. Front. Microbiol. 4, 20. doi: 10.3389/fmicb.2013.00020
Bletz M. C., Loudon A. H., Becker M. H., Bell S. C., Woodhams D. C., Minbiole K. P. C., et al. (2013). ‘Mitigating amphibian chytridiomycosis with bioaugmentation: characteristics of effective probiotics and strategies for their selection and use. Ecol. Lett. 16, 807–820. doi: 10.1111/ele.12099.
Boonthai T., Khaopong W., Sangsong J., Nimrat S., Vuthiphandchai V. (2016). ‘Effect of antibiotic supplementation on the quality of cryopreserved fish sperm of silver barb (Barbodes gonionotus): Sperm motility and viability, bacterial quality and fertilization’. Anim. Reprod. Sci. 166, 36–46. doi: 10.1016/j.anireprosci.2016.01.002.
Byrne P. G., Silla A. J. (2022). “Genetic management of threatened amphibians: using artificial fertilisation technologies to facilitate genetic rescue and assisted gene flow,” in Reproductive technologies and biobanking for the conservation of amphibians. Eds. Silla A. J., Kouba A. J., Heatwole H. (CSIRO Publishing, Melbourne, Australia).
Byrne P. G., Simmons L. W., Dale Roberts J. (2003). Sperm competition and the evolution of gamete morphology in frogs’. Proc. R. Soc B. 270, 2079–2086. doi: 10.1098/rspb.2003.2433.
Christensen J. M., Tiersch T. R. (1996). Refrigerated storage of channel catfish sperm. J. World Aquac. Soc 27, 340–346. doi: 10.1111/j.1749-7345.1996.tb00617.x.
Colombo B. M., Scalvenzi T., Benlamara S., Pollet N. (2015). Microbiota and mucosal immunity in amphibians. Front. Immunol. 6, 111. doi: 10.3389/fimmu.2015.00111.
Comizzoli P., Power M. (2019). ‘Reproductive microbiomes in wild animal species: A new dimension in conservation biology. Adv. Exp. Med. Biol. 1200, 225–240. doi: 10.1007/978-3-030-23633-5.
Contreras M. J., Núñez-Montero K., Bruna P., García M., Leal K., Barrientos L., et al. (2022). Bacteria and boar semen storage: progress and challenges. Antibiotics 11, 1796. doi: 10.3390/antibiotics11121796.
Contreras M. J., Núñez-Montero K., Bruna P., Zárate A., Pezo F., García M., et al. (2023). ‘Mammals’ sperm microbiome: current knowledge, challenges, and perspectives on metagenomics of seminal samples’. Front. Microbiol. 14, 1167763. doi: 10.3389/fmicb.2023.1167763.
Contreras P., Dumorné K., Ulloa-Rodríguez P., Merino O., Figueroa E., Farías J. G., et al. (2020). ‘Effects of short-term storage on sperm function in fish semen: a review’. Rev. Aquac. 12, 1373–1389. doi: 10.1111/raq.12387.
Cseh S., Solti L. (2000). ‘Importance of assisted reproductive technologies in the conservation of wild, rare or indigenous ungulates: review article. Acta Vet. Hung. 48, 313–323. doi: 10.1556/avet.48.2000.3.8.
Daszak P., Cunningham A. A., Hyatt A. D. (2001). ‘Anthropogenic environmental change and the emergence of infectious diseases in wildlife’. Acta Trop. 78, 103–116. doi: 10.1016/S0001-706X(00)00179-0.
DeGraaf J. D., Berlinsky D. L. (2004). ‘Cryogenic and refrigerated storage of rainbow smelt Osmerus mordax spermatozoa’. J. World Aquac. Soc 35, 209–216. doi: 10.1111/j.1749-7345.2004.tb01076.x.
Ellison S., Knapp R. A., Sparagon W., Swei A., Vredenburg V. T. (2019). ‘Reduced skin bacterial diversity correlates with increased pathogen infection intensity in an endangered amphibian host’. Mol. Ecol. 28, 127–140. doi: 10.1111/mec.14964.
Ferrie G. M., Alford V. C., Atkinson J., Baitchman E., Barber D., Blaner W. S., et al. (2014). ‘Nutrition and health in amphibian husbandry. Zoo Biol. 33, 485–501. doi: 10.1002/zoo.21180.
Germano J. M., Arregui L., Kouba A. J. (2013). ‘Effects of aeration and antibiotics on short-term storage of Fowler’s toad (Bufo fowleri) sperm. Aquaculture 396-99, 20–24. doi: 10.1016/j.aquaculture.2013.02.018.
Givens M. D. (2018). Review: Risks of disease transmission through semen in cattle. Animal 12, s165–ss71. doi: 10.1017/S1751731118000708.
Haines M. D., Parker H. M., McDaniel C. D., Kiess A. S. (2013). Impact of 6 different intestinal bacteria on broiler breeder sperm motility in vitro. Poult. Sci. 92, 2174–2181. doi: 10.3382/ps.2013-03109.
Harris R. N., Brucker R. M., Walke J. B., Becker M. H., Schwantes C. R., Flaherty D. C., et al. (2009). Skin microbes on frogs prevent morbidity and mortality caused by a lethal skin fungus. ISME J. 3, 818–824. doi: 10.1038/ismej.2009.27.
Harrison X. A., Price S. J., Hopkins K., Leung W. T. M., Sergeant C., Garner T. W. J. (2019). ‘Diversity-stability dynamics of the amphibian skin microbiome and susceptibility to a lethal viral pathogen. Front. Microbiol. 10, 2883. doi: 10.3389/fmicb.2019.02883.
Hernández-Gómez O., Briggler J. T., Williams R. N. (2019). ‘Captivity-induced changes in the skin microbial communities of hellbenders (Cryptobranchus alleganiensis). Microb. Ecol. 77, 782–793. doi: 10.1007/s00248-018-1258-1.
Holt W. V., Comizzoli P. (2021). PERSPECTIVE: genome resource banking for wildlife conservation: promises and caveats. Cryo-Lett. 42, 309–320.
Jani A. J., Briggs C. J. (2014). ‘The pathogen Batrachochytrium dendrobatidis disturbs the frog skin microbiome during a natural epidemic and experimental infection. Proc. Natl. Acad. Sci. 111, 16645. doi: 10.1073/pnas.1412752111.
Jiménez R. R., Sommer S. (2017). ‘The amphibian microbiome: natural range of variation, pathogenic dysbiosis, and role in conservation. Biodivers. Conserv. 26, 763–786. doi: 10.1007/s10531-016-1272-x.
Kaurova S. A., Browne R. K., Uteshev V. K. (2022). ‘Antibiotics for the refrigerated storage at 4°C of hormonally induced European сommon frog (Rana temporaria) spermatozoa. Theriogenology Wild 1, 100009. doi: 10.1016/j.therwi.2022.100009.
Keogh L. M., Byrne P. G., Silla A. J. (2017). ‘The effect of gentamicin on sperm motility and bacterial abundance during chilled sperm storage in the Booroolong frog. Gen. Comp. Endocrinol. 243, 51–59. doi: 10.1016/j.ygcen.2016.11.005.
Kirkwood J. K., Colenbrander B. (2001). “Disease control measures for genetic resource banking,” in Cryobanking the genetic resource; wildlife conservation for the future. Ed. Watson P. F. (Taylor & Francis, New York, NY, USA), 69–84.
Korpita T. M., Muths E. L., Watry M. K., McKenzie V. J. (2023). Captivity, reintroductions, and the rewilding of amphibian-associated bacterial communities. Microb. Ecol. 86, 2271–2281. doi: 10.1007/s00248-023-02229-3.
Kouba C. K., Julien A. R. (2022). “Linking in situ and ex situ populations of threatened amphibian species using genome resource banks,” in Reproductive technologies and biobanking for the conservation of amphibians. Eds. Silla A. J., Kouba A. J., Heatwole H. (CSIRO Publishing, Melbourne, Australia).
Kueneman J. G., Woodhams D. C., Harris R., Archer H. M., Knight R., McKenzie V. J. (2016). Probiotic treatment restores protection against lethal fungal infection lost during amphibian captivity. Proc. R. Soc B 283 (1839), 20161553. doi: 10.1098/rspb.2016.1553
Loudon A. H., Woodhams D. C., Parfrey L. W., Archer H., Knight R., McKenzie V., et al. (2014). Microbial community dynamics and effect of environmental microbial reservoirs on red-backed salamanders (Plethodon cinereus). ISME J. 8, 830–840. doi: 10.1038/ismej.2013.200.
Love E. (2011). Hormonal induction of spermiation and short-term sperm storage in two Australian tree frogs.’, Honours thesis. Silla A. J., Byrne P. G., Mohideen M. (Supervisors). Melbourne, Australia: Monash University.
Lueders I., Allen W. R. T. (2020). Managed wildlife breeding-an undervalued conservation tool? Theriogenology 150, 48–54. doi: 10.1016/j.theriogenology.2020.01.058.
Lüpold S., Jin L., Liao W. B. (2017). ‘Population density and structure drive differential investment in pre- and postmating sexual traits in frogs. Evolution 71, 1686–1699. doi: 10.1111/evo.13246.
Maes D., Nauwynck H., Rijsselaere T., Mateusen B., Vyt P., de Kruif A., et al. (2008). ‘Diseases in swine transmitted by artificial insemination: An overview. Theriogenology 70, 1337–1345. doi: 10.1016/j.theriogenology.2008.06.018.
Magris M. (2021). Strategic adjustment of ejaculate quality in response to variation of the socio-sexual environment. Behav. Ecol. Sociobiol. 75, 91. doi: 10.1007/s00265-021-03032-1.
Mashoof S., Goodroe A., Du C. C., Eubanks J. O., Jacobs N., Steiner J. M., et al. (2013). ‘Ancient T-independence of mucosal IgX/A: Gut microbiota unaffected by larval thymectomy in Xenopus laevis’. Mucosal Immunol. 6, 358–368. doi: 10.1038/mi.2012.78.
Molina N. M., Plaza-Díaz J., Vilchez-Vargas R., Sola-Leyva A., Vargas E., Mendoza-Tesarik R., et al. (2021). ‘Assessing the testicular sperm microbiome: a low-biomass site with abundant contamination. Reprod. BioMed. 43, 523–531. doi: 10.1016/j.rbmo.2021.06.021.
Murray K., Skerratt L. F., Marantelli G., Berger L., Hunter D. A., Mahony M. J., et al. (2011). Hygiene protocols for the control of diseases in Australian frogs (Canberra, Australia: Australian Government Department of Sustainability, Environment, Water, Population and Communities).
Murray K. N., Varga Z. M., Kent M. L. (2016). ‘Biosecurity and health monitoring at the zebrafish international resource center. Zebrafish 13, S–3-S-38. doi: 10.1089/zeb.2015.1206.
Nimrat S., Sangnawakij T., Vuthiphandchai V. (2005). Preservation of black tiger shrimp Penaeus monodon spermatophores by chilled storage. J. World Aquac. Soc 36, 76–86. doi: 10.1111/j.1749-7345.2005.tb00133.x.
Nimrat S., Sonwat S., Matharatanukul B., Boonthai T., Vuthiphandchai V. (2022). ‘Chilled and cryopreserved spermatophores of banana shrimp (Fenneropenaeus merguiensis): Effects of antibiotics on sperm quality and bacterial abundance. Aquaculture 560, 738551. doi: 10.1016/j.aquaculture.2022.738551.
O’Brien J. K., Steinman K. J., Robeck T. R. (2009). ‘Application of sperm sorting and associated reproductive technology for wildlife management and conservation. Theriogenology 71, 98–107. doi: 10.1016/j.theriogenology.2008.09.052.
Oielska M. (2009). “Reproduction of amphibians,” in Biological systems in vertebrates (CRC Press, Milton). doi: 10.1201/9781482280135.
Ory E. M., Yow E. M. (1963). ‘The use and abuse of the broad spectrum antibiotics. JAMA 185, 273–279. doi: 10.1001/jama.1963.03060040057022.
Passos L. F., Garcia G., Young R. J. (2018). ‘Comparing the bacterial communities of wild and captive golden mantella frogs: Implications for amphibian conservation. PloS One 13, e0205652–e. doi: 10.1371/journal.pone.0205652.
Pereira S. A., Jerônimo G. T., Costa Marchiori N., Oliveira H. M., Owatari M. S., Jesus G. F. A., et al. (2017). Autochthonous probiotic Lactobacillus sp. in the diet of bullfrog tadpoles Lithobates catesbeianus improves weight gain, feed conversion and gut microbiota. Aquac. Nutr. 23, 910–916. doi: 10.1111/anu.2017.23.issue-5.
Pereira S. A., Jerônimo G. T., Marchiori N. C., Oliveira H. M., Jesus G. F. A., Schmidt E. C., et al. (2018). ‘Tadpoles fed supplemented diet with probiotic bacterium isolated from the intestinal tract of bullfrog Lithobates catesbeianus: Haematology, cell activity and electron microscopy. Microb. Pathog. 114, 255–263. doi: 10.1016/j.micpath.2017.11.033.
Pessier A. R., Mendelson J. R. III. eds. (2017). A manual for control of infectious diseases in amphibian survival assurance colonies and reintroduction programs, ver. 2.0 (Apple Valley, MN: IUCN/SSC Conservation Breeding Specialist Group).
Pukazhenthi B. S., Wildt D. E. (2004). ‘Which reproductive technologies are most relevant to studying, managing and conserving wildlife? Reprod. Fertil. Dev. 16, 33–46. doi: 10.1071/RD03076.
Rahimi R., Hajirezaee S., Shaluei F., Kaboutari Katadj J. (2016). ‘Antibiotics, Penicillin and Sreptomycin improve semen quality indices of endangered Caspian brown trout, Salmo trutta caspius (Kessler 1870) during in vitro short-term storage. Aquac. Res. 47, 3662–3666. doi: 10.1111/are.2016.47.issue-11.
Rebollar E. A., Martínez-Ugalde E., Orta A. H. (2020). The amphibian skin microbiome and its protective role against chytridiomycosis. Herpetologica 76, 167–177. doi: 10.1655/0018-0831-76.2.167.
Rebollar E. A., Simonetti S. J., Shoemaker W. R., Harris R. N. (2016). ‘Direct and indirect horizontal transmission of the antifungal probiotic bacterium Janthinobacterium lividum on green frog (Lithobates clamitans) tadpoles. Appl. Environ. Microbiol. 82, 2457–2466. doi: 10.1128/AEM.04147-15.
Redford K. H., Segre J. A., Salafsky N., del Rio C. M., McAloose D. (2012). ‘Conservation and the microbiome. Conserv. Biol. 26, 195–197. doi: 10.1111/j.1523-1739.2012.01829.x.
Saad A., Billard R., Theron M. C., Hollebecq M. G. (1988). ‘Short-term preservation of carp (Cyprinus carpio) semen. Aquaculture 71, 133–150. doi: 10.1016/0044-8486(88)90280-3.
Sabino-Pinto J., Bletz M. C., Islam M. M., Shimizu N., Bhuju S., Geffers R., et al. (2016). ‘Composition of the cutaneous bacterial community in Japanese amphibians: effects of captivity, host species, and body region. Microb. Ecol. 72, 460–469. doi: 10.1007/s00248-016-0797-6.
Sanocka-Maciejewska D., Ciupińska M., Kurpisz M. (2005). Bacterial infection and semen quality. J. Reprod. Immunol. 67, 51–56. doi: 10.1016/j.jri.2005.06.003.
Scheele B. C., Pasmans F., Skerratt L. F., Berger L., Martel A., Beukema W., et al. (2019). Amphibian fungal panzootic causes catastrophic and ongoing loss of biodiversity. Science 363, 1459–1463. doi: 10.1126/science.aav0379.
Segovia M., Jenkins J. A., Paniagua-Chavez C., Tiersch T. R. (2000). Flow cytometric evaluation of antibiotic effects on viability and mitochondrial function of refrigerated spermatozoa of nile tilapia. Theriogenology 53, 1489–1499. doi: 10.1016/S0093-691X(00)00291-0.
Silla A. J. (2013). ‘Artificial fertilisation in a terrestrial toadlet (Pseudophryne guentheri): effect of medium osmolality, sperm concentration and gamete storage. Reprod. Fertil. Dev. 25, 1134–1141. doi: 10.1071/RD12223.
Silla A. J., Byrne P. G. (2019). ‘The role of reproductive technologies in amphibian conservation breeding programs’, annu. Rev. Anim. Biosci. vol. 7 pp, 499–519. doi: 10.1146/annurev-animal-020518-115056.
Silla A. J., Keogh L. M., Byrne P. G. (2015). ‘Antibiotics and oxygen availability affect the short-term storage of spermatozoa from the critically endangered booroolong frog, Litoria booroolongensis. Reprod. Fertil. Dev. 27, 1147–1153. doi: 10.1071/RD14062.
Silla A. J., Kouba A. J. (2022). “Integrating reproductive technologies into the conservation toolbox for the recovery of amphibian species,” in Reproductive technologies and biobanking for the conservation of amphibians. Eds. Silla A. J., Kouba A. J., Heatwole H. (CSIRO Publishing, Melbourne, Australia).
Silla A. J., Langhorne C. J. (2022). “Protocols for hormonally induced spermiation, and the cold storage, activation, and assessment of amphibian sperm,” in Reproductive technologies and biobanking for the conservation of amphibians. Eds. Silla A. J., Kouba A. J., Heatwole H. (CSIRO Publishing, Melbourne, Australia).
Silla A. J., Roberts J. D. (2012). ‘Investigating patterns in the spermiation response of eight Australian frogs administered human chorionic gonadotropin (hCG) and luteinizing hormone-releasing hormone (LHRHa). Gen. Comp. Endocrinol. 179, 128–136. doi: 10.1016/j.ygcen.2012.08.009.
Silla A. J., Roberts J. D., Byrne P. G. (2020). The effect of injection and topical application of hCG and GnRH agonist to induce sperm-release in the roseate frog, Geocrinia rosea. Conserv. Physiol. 8, 1–coaa104. doi: 10.1093/conphys/coaa104.
Stoss J., Refstie T. (1983). ‘Short-term storage and cryopreservation of milt from Atlantic salmon and sea trout. Aquaculture 30, 229–236. doi: 10.1016/0044-8486(83)90165-5.
Tong Q., Hu Z.-F., Du X.-P., Bie J., Wang H.-B. (2020). Effects of seasonal hibernation on the similarities between the skin microbiota and gut microbiota of an amphibian (Rana dybowskii)’. Microb. Ecol. 79, 898–909. doi: 10.1007/s00248-019-01466-9.
Wiggins P. J., Smith J. M., Harris R. N., Minbiole K. P. C. (2011). Gut of red-backed salamanders (Plethodon cinereus) may serve as a reservoir for an antifungal cutaneous bacterium. J. Herpetol. 45, 329–332. doi: 10.1670/10-231.1.
Yániz J. L., Marco-Aguado M. A., Mateos J. A., Santolaria P. (2010). ‘Bacterial contamination of ram semen, antibiotic sensitivities, and effects on sperm quality during storage at 15°C. Anim. Reprod. Sci. 122, 142–149. doi: 10.1016/j.anireprosci.2010.08.006
Zhou J., Nelson T. M., Rodriguez Lopez C., Sarma R. R., Zhou S. J., Rollins L. A. (2020). ‘A comparison of nonlethal sampling methods for amphibian gut microbiome analyses. Mol. Ecol. Resour. 20, 844–855. doi: 10.1111/1755-0998.13139.
Zidni I., Lee H.-B., Yoon J.-H., Park J.-Y., Jang H.-S., Co Y.-S., et al. (2023). Intermediate-term storage of spotted halibut (Verasper variegatus) sperm: effects of storage methods, extenders supplemented with antibiotics and antioxidants on sperm quality. Antioxidants 12, 122. doi: 10.3390/antiox12010122.
Keywords: amphibian, antibiotics, biosecurity, bacterial identification, spermic urine, microbiome, reproductive technologies, conservation breeding program
Citation: Anastas ZM, Byrne PG and Silla AJ (2024) Characterisation of the urinary microbiome of a frog, and the effect of antibiotics on bacterial abundance and sperm viability during refrigerated storage. Front. Amphib. Reptile Sci. 2:1329888. doi: 10.3389/famrs.2024.1329888
Received: 30 October 2023; Accepted: 08 February 2024;
Published: 08 March 2024.
Edited by:
Trenton Garner, Zoological Society of London, United KingdomReviewed by:
Volker Schmidt, Leipzig University, GermanyDuminda Dissanayake, University of Canberra, Australia
Copyright © 2024 Anastas, Byrne and Silla. This is an open-access article distributed under the terms of the Creative Commons Attribution License (CC BY). The use, distribution or reproduction in other forums is permitted, provided the original author(s) and the copyright owner(s) are credited and that the original publication in this journal is cited, in accordance with accepted academic practice. No use, distribution or reproduction is permitted which does not comply with these terms.
*Correspondence: Aimee J. Silla, YXNpbGxhQHVvdy5lZHUuYXU=