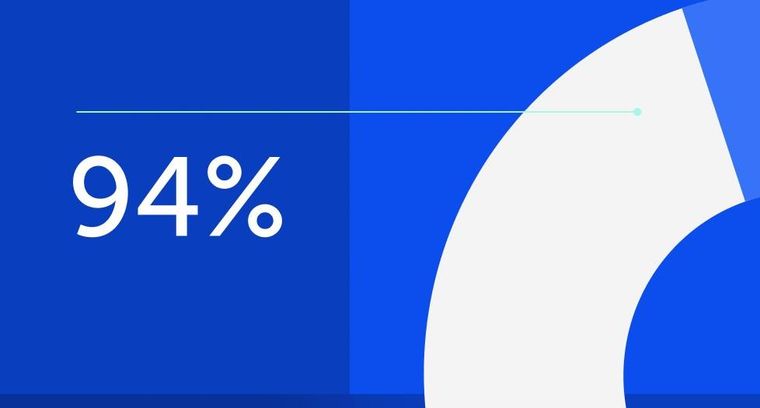
94% of researchers rate our articles as excellent or good
Learn more about the work of our research integrity team to safeguard the quality of each article we publish.
Find out more
ORIGINAL RESEARCH article
Front. Amphib. Reptile Sci., 19 January 2024
Sec. Physiology and Health
Volume 1 - 2023 | https://doi.org/10.3389/famrs.2023.1320803
This article is part of the Research TopicReproductive Physiology, Reproductive Technologies, and Biobanking to Assist Amphibian and Reptile ConservationView all 4 articles
Due to the concentrated nature and small volume of spermic milt that is typically produced from internally-fertilizing salamanders, an extender is needed to analyze the sperm for quality and quantity metrics. Techniques for diluting salamander sperm for analysis have thus far utilized low osmolality media, which are likely physiologically distinct from the salamander female reproductive tract. Application of mammalian sperm extenders could maintain salamander sperm motility and morphology in storage longer due to the similar nature of internal fertilization in both taxa. Several sperm extenders were tested including: Multipurpose Handling Medium, Sperm Washing Medium, Modified Ham’s F-10 Basal Medium (HAM’s), Refrigeration Medium, and 10% Holtfreter’s solution as a control. The experimental treatments were divided into two groups: diluted (90 mOsm, the approximate osmolality of salamander sperm) and undiluted (280-320 mOsm). Milt samples were collected from male tiger salamanders (N=11) and diluted 1:50 with each of the treatments and measured for sperm progressive motility, total motility, and normal morphology every 10 minutes for one hour. When comparing the diluted versus undiluted treatments of the same extender, there was no difference (p>0.05) for any of the response variables. In addition, we found that 10% Holtfreter’s solution is best for maintaining progressive motility, while diluted HAM’s is best for retaining total motility and normal morphology over time. Overall, we found that osmolality is not a key driver influencing tiger salamander sperm motility, but rather other factors such as ionic composition or pH could be affecting sperm form and function.
In the order Caudata, 109 species of aquatic salamander belonging to the families Cryptobranchidae, Hynobiidae, and Sirenidae exhibit external fertilization, whereas the remaining seven families (704 species) exhibit internal fertilization (Sever, 1991; Kahrl et al., 2022; AmphibiaWeb, 2023). The difference between the two modes of fertilization can be observed in several evolutionary adaptations unique to spermatozoa from salamander and newt families. As opposed to releasing free-swimming sperm into an aquatic environment directly on eggs, principally seen in anurans and a limited number of caudates, most male salamanders deposit one or more spermatophores (Sever, 2000). These spermatophores are gelatinous protein capsules containing a mass of sperm, which are typically deposited in small, shallow waterbodies such as vernal pools, ephemeral wetlands, streams, springs, or seeps. Most females utilize a strong sense of smell and pheromone recognition to locate mates and will subsequently accept or decline the spermatophores based on the success of the males’ courtship behaviors (Houck et al., 2007). Once the female accepts the spermatophore into her cloaca, it is kept in a structure called the spermatheca for short to long-term storage until ovulation, after which the eggs are fertilized by the sperm as they pass through the reproductive tract for oviposition (Adams et al., 2005). Research has indicated that the salamander spermatheca contains glycoproteins as well as zinc that assists sperm in the storage process like in some mammal species (Sever, 2002). As opposed to external fertilizers, internally-fertilizing species have evolved sperm cells that can be kept for long periods within the female reproductive tract where signal transduction is thought to control sperm movement and the buildup of reactive oxygen species (Holt & Fazeli, 2016). These differences in reproductive modes influence the reproductive physiology of species as well as the approaches to implementing assisted reproductive technologies (ART) (Comizzoli et al., 2012).
For aquatic breeding and externally-fertilizing anurans, the development and application of ART, such as exogenous hormone treatment for gamete collection and in-vitro fertilization (IVF), is fairly straightforward. Sperm evaluation and fertilization do not require any complicated media formulation, as the process occurs in a simple aqueous environment, similar to their natural reproductive niche (McDiarmid, 1994). Moreover, anuran sperm is typically expressed in their urine, which lowers osmolality to around 40 mOsm (Arregui et al., 2021), thereby activating forward motility (Mansour et al., 2010). The expression of anuran sperm into urine typically results in lower concentrations and higher volumes that favor a low osmolality (<100 mOsm) fertilization environment (Arregui et al., 2021). In comparison, hormonally induced internally-fertilizing salamanders produce both spermic urine and small volumes of milt (Chen et al., 2022; Chen et al., 2023), that more closely resembles the appearance of fish milt or semen in mammalian species. Furthermore, salamander sperm motility may not be activated by lowering the osmolality and can be difficult to observe as the sperm velocity can be low and the spermatozoa tend to spin in circles with little to no forward motion (Gillis et al., 2021). Thus, evaluating salamander sperm requires an extender for measuring quality (e.g., motility) and quantity metrics.
Techniques for diluting salamander sperm in preparation for evaluation, cryopreservation, and IVF have so far utilized low osmolality extenders such as Holtfreter’s solution (Peng et al., 2011; Hayashi et al., 2013; Marcec, 2016). While this approach may be appropriate for externally-fertilizing amphibians whose sperm is activated by a drop in osmolality (Mansour et al., 2010), the internal fertilization environment of the salamander female reproductive tract is biochemically complex and osmotically distinct from an aquatic environment (Hardy and Dent, 1986). For example, research suggests that the female newt spermatheca has a high osmolality similar to that of the male vas deferens where sperm is stored, and sperm cells have a higher and more persistent motility over time when placed in high osmolality solutions (Hardy and Dent, 1986). Hence, the application of sperm extenders with higher osmolality, such as those used for mammal sperm and other internal fertilizers, may preserve salamander sperm motility and morphology over time. The high osmolality environment of the mammalian oviduct, referred to as the ‘holding pen’ for spermatozoa before fertilization (Holt, 2011), and the presumed high osmolality of the female salamander spermatheca point to possible similarities between the reproductive physiology of these two taxa. Furthermore, higher osmolality extenders with additives such as antioxidants would likely benefit the development of salamander ART by maintaining higher sperm quality in preparation for freezing or use in IVF as has been observed for mammalian sperm (Anastas et al., 2023).
Similar to salamander sperm, mammalian sperm is typically produced in high concentrations sometimes requiring the use of extenders to dilute the sample for evaluation, cryopreservation, and subsequent assisted breeding purposes. Mammalian semen extenders typically have a higher osmolality (300-400 mOsm/kg), so as to prevent osmotic shock and swelling of sperm cells (Saragusty et al., 2009). Several commercially available media have been developed for the evaluation, storage, and manipulation of mammalian gametes that may have applications for use in extending salamander sperm (typically ~90 mOsm/kg), including Multipurpose Handling Medium (MHM), Sperm Washing Medium (SWM), Refrigeration Medium, and HAM’s F-10 culture medium (HAM’s). As an example, Multipurpose Handling Medium has been used to process sperm in mice (Helmy et al., 2023) and humans (Al-Saleh et al., 2019), whereas Sperm Washing Medium has been used with macaque (Strelchenko et al., 2020) and human (Kim et al., 2015) sperm. In addition, Refrigeration Medium has been used to extend sperm samples and increase storage time for sperm from margays (Erdmann et al., 2020), Asiatic black bears (Jeong et al., 2019), and mangabeys (Gadea et al., 2019), while HAM’s F-10 solution has been used for Iberian lynx (Gañán et al., 2009) and giant panda (Santiago-Moreno et al., 2016) sperm extension, to name a few. These particular extenders were chosen since they are widely available, have been successful in a variety of mammal species, and do not contain milk or egg products (with the exception of Refrigeration Medium) that stimulate formation of micelles when diluting that prevent visual and quantitative analysis; the drop in osmolality following dilution stimulates the formation of these obstructive and sticky micelles within egg yolk. The higher osmolality of the commercial mammalian extenders (ranging from 280-320 mOsm/kg) and their additional ingredients (e.g., glucose, thioctic acid) may support salamander sperm motility and morphology over time better than low osmolality solutions that have been utilized previously in amphibians (Marcec, 2016; Gillis, 2020). For example, tiger salamander sperm has been observed to be motile at a range of osmolalities between 5-300 mOsm/kg (Gillis et al., 2021), which at the upper end is similar to the osmolality of many of the commercially available sperm extenders described above.
To date, 10% Holtfreter’s solution has primarily been used to dilute and extend salamander sperm in preparation for evaluation and cryopreservation (Marcec, 2016; Gillis, 2020). While a 10% Holtfreter’s solution is meant to mimic pond or stream water and has a very low osmolality (<10 mmol/kg), it may not be the most appropriate extender for internally-fertilizing amphibian species. Thus, the objective of this research was to compare four different extenders against 10% Holtfreter’s solution on their ability to preserve salamander sperm motility and morphology over time. In addition, we diluted each experimental extender to about 90 mOsm/kg, the approximate osmolality of artificially collected tiger salamander sperm, and measured the same impacts on sperm motility and morphology. Overall, nine different extender treatments were evaluated for their impacts on sperm parameters during storage at 0°C in an ice bath. We hypothesized that higher osmolality extenders would result in sperm samples maintaining higher progressive motility, total motility, and normal morphology over time and be more appropriate for internal fertilization as they contain a variety of energy substrates and antioxidants. By maintaining sperm with higher motilities and normal morphology during processing stages, higher fertilization rates should be obtainable, which will enhance breeding efforts for at-risk caudate species in conservation breeding programs. The eastern tiger salamander (Ambystoma tigrinum) is an internally-fertilizing salamander species that was chosen for this study as it has previously been used as a model for salamander sperm cryopreservation and IVF (Marcec, 2016; Gillis et al., 2021; Chen et al., 2022).
Captive-bred, sexually mature male eastern tiger salamanders (mean mass = 51.8 ± 2.9 g) were housed in 30 x 46 x 66 cm enclosures, with 5 cm of coconut fiber as bedding, in groups of 2–6 animals. Salamanders were kept on a 12-hour light cycle and provided with water baths as well as clay and polyvinyl chloride hides and were fed a diet of crickets (Gryllodes sigillatus), mealworms (Tenebrio molitor), and Dubia roaches (Blaptica dubia) dusted with a calcium supplement (Zoo Med Laboratories, Inc., CA, USA) and vitamin mix (Supervite, Repashy Ventures Inc., CA, USA). To obtain sperm for the experimental treatments, males were administered a priming dose of gonadotropin-releasing hormone analogue (GnRH) (0.025 μg/g body weight), followed 24 hours later by a resolving dose of GnRH (0.1 μg/g body weight) via intramuscular injection with a 32-gauge syringe (Marcec, 2016). The GnRH (Ala6, des-Gly10 ethylamide LHRH; Sigma-Aldrich® catalog #L4513) was reconstituted 1:1 in phosphate buffered saline to a concentration of 1 μg/μL. During sperm collection, males were kept individually in 28 x 15 x 13 cm plastic tubs with 200 mL of water for 36 hours or less. Following the resolving dose, males were abdominally massaged to express spermic milt every hour for up to six hours (Gillis et al., 2021). After collecting a sufficient volume of milt from an individual male (~10 μL) to run all treatment groups, the individual was returned to their original group housing. Sperm samples were kept cold on ice at 0°C in 0.5 mL microcentrifuge Eppendorf tubes for the duration of the experiment to mimic the procedure of storing tiger salamander sperm prior to use for in-vitro fertilization. All research was approved under IACUC #20-160 at Mississippi State University.
Four commercially available sperm extenders were tested (Table 1), including Multipurpose Handling Medium® (MHM; FUJIFILM Irvine Scientific®, Catalog No. 90163), Sperm Washing Medium (SWM; FUJIFILM Irvine Scientific®, Catalog No. 9983), Modified Ham’s F-10 Basal Medium with 4-(2-hydroxyethyl)-1-piperazineethanesulfonic acid (HAM’s; FUJIFILM Irvine Scientific®, Catalog No. 99168), Refrigeration Medium Test Yolk Buffer with Gentamicin (FUJIFILM Irvine Scientific®, Catalog No. 90129), and 10% Holtfreter’s solution as a control (10% Holt; Sigma-Aldrich® NaCl, KCl, CaCl2, NaHCO3). The 10% Holtfreter’s serves as a control since it has been typically used as a tiger salamander sperm extender (Marcec, 2016; Gillis, 2020), and salamander milt without an extender cannot usually be counted accurately due to high concentrations and cell crowding. The four experimental extenders were divided into two experimental groups each: diluted (90 mOsm/kg, the approximate osmolality of salamander sperm) and undiluted (280-320 mOsm/kg) (Figure 1), resulting in eight experimental extender treatments and one Holtfreter’s solution treatment (n=11 males/treatment). To achieve diluted extenders, a portion of each medium was diluted with sterile embryo transfer water (Sigma-Aldrich®, Catalog No. W1503) to ~90 mOsm/kg. Osmolality values were measured with a Vapro® 5520 osmometer at room temperature. All extenders were stored in the fridge for a maximum of seven months at 4°C until use.
Table 1 Chemical components, osmolality, and pH of experimental extenders used to dilute tiger salamander (Ambystoma tigrinum) sperm.
Figure 1 Experimental procedure including: (1) Hormonal administration of GnRH to stimulate sperm expression in tiger salamanders (Ambystoma tigrinum; n = 11); (2) Sperm collection of milt (thick, white spermic material) and subsequent dilution; (3) Extender treatments including a diluted and undiluted version of each extender and a control; and (4) Data collection of sperm metrics every 10-min for one hour. Stock images by Marie Jeffs, Coprid, kwanchaichaiudom \ Adobe Stock.
Milt samples from each salamander (n = 11 males/treatment) were diluted 1:50 (1 µL sperm/49 µL extender) with each of the extender treatments in a random order and evaluated. Immediately after mixing (Time 0) and every 10 minutes thereafter (up to 60 minutes), a 7 μL sample from each treatment group was pipetted onto a slide and analyzed under 200x magnification using an Olympus® CX41 compact phase-contrast microscope. Each treatment group was measured for progressive motility (sperm actively rotating in a circle), stationary motility (sperm tail membrane undulating, but no circular rotation of cells observed), total motility (progressive motility + stationary motility), normal morphology (no broken sperm tails or heads), sperm cells with abnormal tails, sperm with abnormal heads, and sperm with abnormal heads and tails. Swollen sperm cells were not observed, thus abnormal (i.e., curled, bent, broken, or missing) heads and tails were measured as in Burger et al. (2022). Motility and morphology metrics were each directly measured every 10 minutes via visual inspection by a single observer out of 100 random cells for each male and each extender treatment.
Sperm samples were evaluated across all extender treatments over time (0, 10, 20, 30, 40, 50, and 60 min) to evaluate the effect of treatment, time, and a treatment by time interaction. A one-way ANOVA or Kruskal-Wallis rank sum test was used to compare the initial effect of each treatment on motility and morphology immediately upon diluting (Time 0). In addition, the rate of change per hour for each of the sperm parameters, as a measure of toxicity, was calculated for each extender treatment by finding the slope of the line of each parameter, for each male, over time and used as the response variable. A positive rate of change indicates an increase in the specific variable, while a negative rate indicates a decrease in the variable (e.g., a negative rate in sperm morphology over time means an accrual in proportion of abnormalities). This approach was employed to standardize the response due to sperm samples from different males having different starting motilities and morphologies. A Shapiro-Wilk test was run to analyze the normality of the data and variance was tested through the Levene’s test. General Additive Models in the package ‘gamlss’ in R (R Core Team, 2022) were used to analyze sperm motility and sperm abnormalities, where treatment was the fixed effect and individual and salamander cohort were the random effects. A normal, t-distribution, or skew t-type 1 family was used depending on the response variable. Model fit was checked by testing the normality of the residuals and using Q-Q plots. Additionally, paired t-tests were implemented to directly compare the diluted and undiluted versions of each extender for the sperm parameters measured. The Refrigeration Medium formed large concentrations of hydrophobic micelles that attached to the sperm cells when diluted, and it was impossible to evaluate the sperm visually. Thus, we removed the diluted Refrigeration Medium extender from the experiment, resulting in a total of eight extender treatments that could be evaluated, instead of nine. Data are expressed as mean ± SEM and an alpha level of 0.05 was set for significance testing.
When directly comparing the diluted versus undiluted treatments of the same extender medium, there was no difference (p > 0.05) in rates of change over the 60 minutes for any of the motility or morphology response variables. This suggests that osmolality may not have a direct impact on salamander sperm physiology over the range of osmolalities tested (90-310 mOsmol/kg). Rather, other factors such as extender composition could have an effect.
We examined the immediate effect that each extender treatment had on sperm samples at Time 0 upon dilution and found that there was no effect of treatment on initial motility; however, we did find that there was an immediate impact on sperm normal morphology, where MHM (61.0 ± 5.2%) had significantly lower (p < 0.05) normal morphology at Time 0 compared to diluted SWM (82.4 ± 2.6%) and Refrigeration Medium (82.2 ± 3.2%) but was no different (p > 0.05) from the other treatments. Samples were analyzed in a random order with each diluent, mitigating the effect of time on starting motility and morphology for each individual extender.
When comparing the progressive and total motility values across time points for each treatment, we found that there were significant (p < 0.05) effects of extender treatment and time, but there was no treatment by time interaction (p > 0.05). For normal morphology values, there were significant (p < 0.03) effects of treatment and time, and there was one significant (p < 0.05) interaction between the diluted MHM treatment and the 30-minute time point, indicating that the effect of diluted MHM on sperm morphology depended on the time of analysis.
We found that extender affected sperm motility and morphology rates of change over time. For example, 10% Holtfreter’s (-11.5 ± 2.6%), diluted HAM’s (-14.4 ± 2.2%), and HAM’s (-18.0 ± 5.1%) resulted in significantly slower (p < 0.05) rates of change compared to the other treatments, indicating their ability to better retain progressive motility of sperm cells (Figure 2). The diluted MHM solution (-29.3 ± 4.0%) lost progressive motility the fastest of all the treatments. The 10% Holtfreter’s (-5.2 ± 1.0%) and Refrigeration Medium (-5.7 ± 2.6%) had significantly different (p < 0.03) rates of change in stationary motility compared to diluted MHM (7.0 ± 3.2%), diluted SWM (3.7 ± 2.8%), and SWM (3.1 ± 2.3%). There were some differences in total motility based on extender treatment (Figure 2). For example, sperm in diluted HAM’s (-12.9 ± 2.0%) had a slower (p < 0.03) rate of change in total motility compared to all other extenders except for HAM’s (-17.4 ± 3.4%) and 10% Holtfreter’s (-16.7 ± 2.5%), which indicates that these three extenders retained sperm function longer than the other treatments. A summary of the motility results for each treatment can be found in Table 2.
Figure 2 Rate of change (%/hr) of (A) Progressive sperm motility for each of the extender treatments; (B) Average change in progressive motility from 0 to 60 min post mixing with extender; (C) Rate of change (%/hr) of total sperm motility for each of the extender treatments; (D) Average change in total sperm motility from 0 to 60 min post mixing with extender. Treatments with different letters indicate significant (p < 0.05) differences. Yellow dots indicate the mean, while horizontal lines represent the median.
Table 2 The mean rate of change (%/hr) ± SEM of progressive motility, stationary motility, and total motility of tiger salamander (Ambystoma tigrinum) sperm samples for each of the eight extender treatments (n=11 males/treatment).
In terms of sperm structure, the diluted HAM’s (-4.8 ± 4.2%) resulted in the slowest change in normal morphology (Figure 3), with 10% Holtfreter’s (-18.9 ± 3.6%), diluted MHM (-15.9 ± 2.4%), and MHM (-20.1 ± 5.7%) accruing a greater (p < 0.04) number of abnormal sperm over time. HAM’s (-8.4 ± 4.7%) also had a slower rate of change (p < 0.05) than the 10% Holtfreter’s and MHM treatments for normal morphology. The MHM extender was the overall worst at retaining normal sperm morphology, where approximately 1 in 100 sperm cells became abnormal every three minutes. In general, abnormal heads was the least common abnormality type identified with an average change of 0.24 to 0.72% per minute. When examining differences between treatments for the change in cells with abnormal heads, there was no difference (p > 0.05) between extenders. As for abnormal tails, diluted HAM’s (2.7 ± 2.2%) resulted in a significantly slower (p < 0.04) accrual of deformed sperm tails over time compared to 10% Holtfreter’s (10.8 ± 2.1%) and MHM (12.0 ± 3.8%), but was not different (p > 0.05) from the other treatments. This indicates that the sperm tail is the most prone to changes based on the solution it is stored in; to note, tail abnormalities are one of the most common responses of healthy mammalian sperm cells to the hypoosmotic test (Jeyendran et al., 1984). A summary of the morphology results for each treatment can be found in Table 3. Overall, 10% Holtfreter’s, HAM’s, and diluted HAM’s were the most effective at preserving sperm quality over time, while SWM and MHM were the least effective.
Figure 3 Rate of change (%/hr) of (A) Normal morphology for each of the extender treatments; (B) Average change in normal morphology from 0 to 60 min post mixing with extender; (C) Rate of change (%/hr) of abnormal sperm tails for each of the extender treatments; (D) Average change in abnormal sperm tails from 0 to 60 min post mixing with extender. Treatments with different letters indicate significant (p < 0.05) differences. Yellow dots indicate the mean, while horizontal lines represent the median.
Table 3 The mean rate of change (%/min) ± SEM for percent of tiger salamander (Ambystoma tigrinum) sperm with normal morphology, abnormal heads, abnormal tails, and both abnormal heads and tails in samples for each of the eight extender treatments (n=11 males/treatment) to analyze the impact of media on sperm over time.
In this study, four different mammalian extenders in either diluted or undiluted conditions were compared to 10% Holtfreter’s solution in their ability to maintain tiger salamander sperm quality over an hour when stored at 0°C. The results showed that progressive motility, total motility, and normal morphology of tiger salamander sperm deteriorated over the hour, regardless of extender used. This observed change in motility and morphology is likely due to sperm cells using up energy stores and buildup of reactive oxygen species (Aziz et al., 2004). Stationary motility generally increased over time as cells transitioned from progressive motility to a non-motile state. The deterioration in sperm quality was slowest in the HAM’s, diluted HAM’s, and Holtfreter’s extenders, revealing that these three solutions were superior in preserving sperm motility and morphology compared to Refrigeration Medium, MHM, and SWM extenders. It is possible that additives present in the other extenders may have had a slightly toxic effect on sperm parameters we measured (motility and morphology), or that HAM’s and 10% Holtfreter’s had beneficial components, which may be considered important for fertilization in caudates. Interestingly, osmolality did not seem to affect the rate of decline in motility or normal morphology, further supporting reports that caudate sperm motility from internally-fertilizing species is not activated by osmolality (Ukita et al., 1999; Marcec, 2016), as it is in anurans where a drop in osmolality stimulates movement (Kouba et al., 2003; Watanabe and Onitake, 2003).
Previous research studying the changes in sperm motility over time for tiger salamanders found that progressive motility decreased from 51.4% to 22.3% in the first 60 minutes when stored neat at 0°C (Gillis et al., 2021) for both spermic urine and milt combined. Similarly, Marcec (2016) observed tiger salamander sperm motility decreased by approximately half in 60 minutes when stored at 4°C. In comparison, we found that when tiger salamander sperm was stored in 10% Holtfreter’s, progressive motility was lost by approximately 33% in the first 60 minutes, while the progressive motility was lost by over 80% when stored in MHM at 0°C. Non-extended sperm from the sharp-ribbed newt, Pleurodeles waltl (Uteshev et al., 2015), and eastern hellbender, Cryptobranchus alleganiensis (McGinnity et al., 2021), were observed to have a drop in motility following cold storage after 24 hours. The difference between these previous studies and here, was that previous studies did not extend the sperm until analysis, whereas we diluted the sperm with different extenders and followed the impact on several sperm metrics over time. Sperm were exposed to extenders for 60 minutes to mimic the processing time necessary during IVF or sperm cryopreservation protocols. Our data suggest certain extenders can help retain motility for longer periods of time compared to non-extended samples, which would be useful when conducting IVFs where gamete collection is often asynchronous (Kouba et al., 2009).
We found salamander sperm motility and morphology declined at the same rate regardless of osmolality. While not shown here, tiger salamander sperm motility has been observed in solutions with >1,400 mOsm/kg. Other taxa such as birds and mammals have also been shown to maintain motility in a range of isosmotic to hyperosmotic extenders (Blanco et al., 2008; Martínez-Pastor et al., 2019). For externally-fertilizing amphibians and freshwater fish, sperm motility is activated by a drop in osmolality (Mansour et al., 2010). However, a dramatic drop in osmolality is typically harmful to sperm from internally-fertilizing taxa such as mammals (González-Fernández et al., 2012). For example, it has been shown that incubating boar and ram sperm in 90 mOsm NaCl solution resulted in significant reduction in sperm viability (Tsikis et al., 2018). Similarly, river stingray (Potamotrygon motoro) sperm motility decreases when exposed to a hypotonic solution (e.g., freshwater) (Dzyuba et al., 2019). We found that tiger salamander sperm motility and morphology are not significantly impacted by either low or high osmolalities, indicating its versatility in changing environmental conditions and the potential for a wide range of extender types. The resilience of tiger salamander sperm in a range of low and high osmolality solutions could be due to the adaptation of the sperm having to travel through various environments including the internal female reproductive tract and into aquatic locations such as ponds within a spermatophore. Other research has shown that tiger salamander sperm (Gillis et al., 2021) as well as newt sperm (Hardy and Dent, 1986) can be found motile in a range of low and high osmolalities, which is helpful for the development of assisted reproduction techniques.
We examined the media components (Supplementary Table) that were similar between the three best extenders (10% Holtfreter’s, HAM’s, and diluted HAM’s) compared to the other five and found it difficult to speculate on benefits of media composition due to proprietary company information on ingredients. One possibility may be the calcium chloride concentration within the extenders, as calcium is an important cation that is critical in the activation of vertebrate sperm motility through ion channels (Pereira et al., 2017). For example, research comparing sperm extenders in tropical catfish (Mystus nemurus) found that the medium containing calcium ions maintained sperm motility the best (Muchlisin et al., 2004). While the calcium channel, CatSper, has been found to be the main gate for the entry of calcium ions into the mammalian sperm cell to promote activation and the acrosome reaction, the CatSper channel is absent in amphibians (Lishko and Kirichok, 2010). However, calcium signaling is still a key component in controlling amphibian sperm motility, where flagellum movement is stimulated by voltage-dependent calcium channels (Sato et al., 2021). Within caudates, calcium is one of the major ions that can be found in the jelly layers coating the outside of the newt egg, which can help activate sperm movement (Ukita et al., 1999). The sustained contact of sperm with calcium ions is also important for the acrosome reaction in amphibians (Ishihara et al., 1984). Additionally, the presence of calcium ions can cause sperm motility to become hyperactivated in newts, though it does not cause initial motility on its own (Ukita et al., 1999). Whereas monovalent ions such as sodium and potassium found within egg jelly can initiate newt sperm motility in high concentrations (Ukita et al., 1999).
Other factors in the extenders such as energy sources, pH, vitamins, and antioxidants could be affecting sperm motility for species that display internal fertilization. For example, when comparing different sperm extenders in mammals, it was found that antioxidant presence could improve sperm quality by blocking oxidative stress in felines (Thuwanut et al., 2010; Angrimani et al., 2017), canines (Neagu et al., 2010; Mahiddine and Kim, 2021), and fishes (Mansour et al., 2006; Martínez-Páramo et al., 2012). In addition, higher pH has been associated with increased sperm motility in fish (Pérez et al., 2022), which might explain why the 10% Holtfreter’s, with the highest pH, had the slowest rate of progressive motility loss. Watanabe et al. (2003) found that Japanese fire-bellied newt (Cynops pyrrhogaster) sperm had over 90% motility when in a solution with a pH of 8.5, while sperm in a solution with a pH of 7.8 only reached a motility of 55%. The sperm-activating egg jelly of newts has been found to have an alkaline pH of 8.5, which could explain why 10% Holtfreter’s better maintained motility over time (Ukita et al., 1999). We found that tiger salamander milt has a pH of 6.4, which is similar to findings showing the pH of newt sperm to be 6.5 (Hardy and Dent, 1986). The change of pH of sperm samples to a more alkaline environment could be affecting hydronium concentrations and subsequent sperm motility. For example, Hardy and Dent (1986) found that newt sperm samples had extensively higher motility when initially placed into a solution of pH 8-9. However, sperm motility was negatively impacted when stored at these higher pH levels for more than 12 hours (Hardy and Dent, 1986).
Alternatively, HAM’s was the only extender to contain an antioxidant (i.e., thioctic acid), which could explain the higher sperm motility and morphology over time compared to the other extenders. Previous work in humans has shown that antioxidants, such as thioctic acid, can significantly increase sperm motility and normal morphology by reducing reactive oxygen species accumulation (Canepa et al., 2018). Additives such as biotin within the HAM’s extender could also be a cause for the sustained quality since previous research with humans found biotin increased sperm motility post-freezing (Kalthur et al., 2012). Comparative research with mammal sperm extenders has found that HAM’s F-10 is a useful treatment for maintaining sperm quality in both coatis, Nasua nasua (da Paz and dos Santos Avila, 2015), and African lions, Panthera leo (Lueders et al., 2012), which agrees with our findings in tiger salamanders. Conversely, research with sand cats (Felis margarita) and black-footed cats (Felis nigripes) observed significant decreases in sperm motility for samples stored in HAM’s F-10 as opposed to a customized medium (Herrick et al., 2010), suggesting that sperm media for salamanders could be further tailored for optimal storage conditions.
Mammalian sperm have evolutionarily adapted the ability to withstand some changes in osmolality through their path from the male epididymis to the female oviduct through adaptations such as aquaporins to regulate water uptake (Chen and Duan, 2011). This relationship appears to be reflected in internally-fertilizing salamander species, where the sperm has to undergo several osmolality changes (e.g., deposition of spermatophores in freshwater) until finally fertilizing an egg. Both mammal and caudate sperm can undergo sperm storage within the female reproductive tract where signal transduction plays a key role in processes such as sperm capacitation; further research has found that heat shock proteins, which are highly conserved across taxa including salamanders, can influence sperm survival in the mammalian female reproductive tract (Holt and Lloyd, 2010). The lack of osmotic shock of tiger salamander sperm to low osmolality conditions could also be a relic of their phylogenetic history as external fertilizers in the evolutionary past (Jia et al., 2021), or the adaptation of spermatophores to withstand osmotic shock when released into water before a female picks it up. This adaptability in both low and high osmolality conditions is advantageous for creating ART protocols for salamanders, as different media can be used to manipulate sperm and possibly increase fertilization potential.
Here, we chose to focus on mammalian sperm extenders, because there is a broader range of commercially available extenders for research on mammalian assisted reproduction. This study looked at four common mammalian sperm diluents, but there are untested diluents that could be more beneficial for salamander sperm, such as those used for avian or reptilian species (Johnston et al., 2014; Burilo and Kashoma, 2023). Furthermore, a mid-range osmolality could be tested in the future, such as those approximating the osmolality of salamander blood or egg jelly (Watanabe and Onitake, 2003). We acknowledge that morphology analysis included only visual head and/or tail abnormalities, and further testing such as morphological staining for acrosome damage could add depth to future studies.
Osmolality is not likely the key driver of sperm motility for internally-fertilizing salamanders as is seen in other externally-fertilizing amphibian species (e.g., frogs and toads). We found that the 10% Holtfreter’s solution is best for maintaining progressive motility, while diluted HAM’s is best for maintaining total motility and normal morphology over time. Other amphibians, such as frogs, have sperm that is activated by a drop in osmolality due to their aquatic, externally-fertilizing reproductive strategy. In contrast, tiger salamanders are internal fertilizers similar to mammals, where sperm motility is largely influenced by pH and calcium ion fluctuations. It is plausible that salamander sperm is impacted more by these other factors, such as ionic composition or pH changes, which could be affecting sperm form and function. Future research projects should continue to use and optimize 10% Holtfreter’s or diluted HAM’s F-10 as extenders for ambystomid sperm cryopreservation and IVF. Increasing the health and functionality of salamander sperm during the processing stages before cryopreservation or IVF would potentially provide a higher motility and morphology, leading to improved fertilization rates and increased offspring production. Ultimately, greater production of genetically diverse offspring may enhance reintroduction and recovery efforts for some portion of the more than 50% threatened caudate species.
The raw data supporting the conclusions of this article will be made available by the authors, without undue reservation.
The animal study was approved by Institutional Animal Care & Use Committee #20-160 at Mississippi State University. The study was conducted in accordance with the local legislation and institutional requirements.
DC: Conceptualization, Formal Analysis, Investigation, Visualization, Writing – original draft. CK: Conceptualization, Funding acquisition, Project administration, Supervision, Writing – review & editing. NS: Conceptualization, Supervision, Writing – review & editing. TR: Conceptualization, Supervision, Writing – review & editing. PA: Conceptualization, Supervision, Writing – review & editing. AK: Conceptualization, Funding acquisition, Project administration, Supervision, Visualization, Writing – review & editing.
The author(s) declare financial support was received for the research, authorship, and/or publication of this article. This work was supported by the Institute of Museum and Library Services (IMLS) National Leadership Grant [grant number MG-30-17-0052-17] for the sustainability and improvement of collections care. In addition, this project was supported by the Mississippi State Forest and Wildlife Research Center (FWRC), Mississippi State Agricultural and Forestry Experiment Station (MAFES), the National Institute of Food and Agriculture, U.S. Department of Agriculture, Hatch project under accession number W3173, and the U.S. Department of Agriculture, Agricultural Research Service, Biophotonics project 6066-31000-015-00D.
Thank you to fellow members of the Mississippi State University Conservation Physiology Lab, including Li-Dunn Chen and Trinity Kassuhn for their assistance.
The authors declare that the research was conducted in the absence of any commercial or financial relationships that could be construed as a potential conflict of interest.
All claims expressed in this article are solely those of the authors and do not necessarily represent those of their affiliated organizations, or those of the publisher, the editors and the reviewers. Any product that may be evaluated in this article, or claim that may be made by its manufacturer, is not guaranteed or endorsed by the publisher.
The Supplementary Material for this article can be found online at: https://www.frontiersin.org/articles/10.3389/famrs.2023.1320803/full#supplementary-material
Adams E. M., Jones A. G., Arnold S. J. (2005). Multiple paternity in a natural population of a salamander with long-term sperm storage. Mol. Ecol. 14 (6), 1803–1810. doi: 10.1111/j.1365-294X.2005.02539.x
Al-Saleh I., Coskun S., Al-Doush I., Al-Rajudi T., Al-Rouqi R., Abduljabbar M., et al. (2019). Exposure to phthalates in couples undergoing in vitro fertilization treatment and its association with oxidative stress and DNA damage. Environ. Res. 169, 396–408. doi: 10.1016/j.envres.2018.11.018
AmphibiaWeb Species Lists (2023) The regents of the University of California. Available at: https://amphibiaweb.org/lists/index.shtml (Accessed 2023 Jul 29).
Anastas Z. M., Byrne P. G., O’Brien J. K., Hobbs R. J., Upton R., Silla A. J. (2023). The increasing role of short-term sperm storage and cryopreservation in conserving threatened amphibian species. Animals 13 (13), 2094. doi: 10.3390/ani13132094
Angrimani D. D. S. R., Barros P. M. H., Losano J. D. D. A., Cortada C. N. M., Bertolla R. P., Guimarães M. A. B. V., et al. (2017). Effect of different semen extenders for the storage of chilled sperm in Tigrina (Leopardus tigrinus). Theriogenology 89, 146–154. doi: 10.1016/j.theriogenology.2016.10.015
Arregui L., Kouba A. J., Germano J. M., Barrios L., Moore M., Kouba C. K., et al. (2021). Fertilization potential of cold-stored Fowler’s toad (Anaxyrus fowleri) spermatozoa: temporal changes in sperm motility based on temperature and osmolality. Reproduction Fertility Dev. 34 (5), 461–469. doi: 10.1071/RD21037
Aziz N., Saleh R. A., Sharma R. K., Lewis-Jones I., Esfandiari N., Thomas A. J. Jr., et al. (2004). Novel association between sperm reactive oxygen species production, sperm morphological defects, and the sperm deformity index. Fertility Sterility 81 (2), 349–354. doi: 10.1016/j.fertnstert.2003.06.026
Blanco J. M., Long J. A., Gee G., Donoghue A. M., Wildt D. E. (2008). Osmotic tolerance of avian spermatozoa: Influence of time, temperature, cryoprotectant and membrane ion pump function on sperm viability. Cryobiology 56 (1), 8–14. doi: 10.1016/j.cryobiol.2007.09.004
Burger I. J., Lampert S. S., Kouba C. K., Morin D. J., Kouba A. J. (2022). Development of an amphibian sperm biobanking protocol for genetic management and population sustainability. Conservation Physiology 10 (1), coac032.
Burilo A., Kashoma I. (2023). Effect of semen extenders, dilution rates and storage periods on spermatozoa quality of Horasi chicken ecotype. Appl. Veterinary Res. 2 (4), 2023020–2023020. doi: 10.31893/avr.2023020
Canepa P., Dal Lago A., De Leo C., Gallo M., Rizzo C., Licata E., et al. (2018). Combined treatment with myo-inositol, alpha-lipoic acid, folic acid and vitamins significantly improves sperm parameters of sub-fertile men: a multi-centric study. Eur. Rev. Med. Pharmacol. Sci. 22 (20), 7078–7085.
Chen D., Kouba C., Kouba A. (2022). 36 Evaluating the toxicity of several sperm diluents over time and at varying osmolalities in an internally fertilising salamander species. Reproduction Fertility Dev. 35 (2), 143–144. doi: 10.1071/RDv35n2Ab36
Chen D., Kouba C., Songsasen N., Roth T., Allen P., Kouba A. (2023). Oral administration of GnRH via a cricket vehicle stimulates spermiation in tiger salamanders (Ambystoma tigrinum) bioRxiv 2023-08. doi: 10.1101/2023.08.01.551446
Chen Q., Duan E. K. (2011). Aquaporins in sperm osmoadaptation: an emerging role for volume regulation. Acta Pharmacologica Sin. 32 (6), 721–724. doi: 10.1038/aps.2011.35
Comizzoli P., Songsasen N., Hagedorn M., Wildt D. E. (2012). Comparative cryobiological traits and requirements for gametes and gonadal tissues collected from wildlife species. Theriogenology 78 (8), 1666–1681. doi: 10.1016/j.theriogenology.2012.04.008
da Paz R. C. R., dos Santos Avila H. B. (2015). Coatis (Nasua nasua) semen cryopreservation. Braz. J. Veterinary Res. Anim. Sci. 52 (2), 151–157. doi: 10.11606/issn.1678-4456.v52i2p151-157
Dzyuba V., Sampels S., Ninhaus-Silveira A., Kahanec M., Veríssimo-Silveira R., Rodina M., et al. (2019). Sperm motility and lipid composition in internally fertilizing ocellate river stingray Potamotrygon motoro. Theriogenology 130, 26–35. doi: 10.1016/j.theriogenology.2019.02.029
Erdmann R. H., Blank M. H., Ribeiro R. N., de Oliveira M. J., Cubas Z. S., Pradiee J., et al. (2020). Cryopreservation of margay (Leopardus wiedii) spermatozoa: Effects of different extenders and frozen protocols. Theriogenology 143, 27–34.
Gadea J., Toledano-Díaz A., Navarro-Serna S., Jiménez-Movilla M., Soriano P., Matás C., et al. (2019). Assessment and preservation of liquid and frozen-thawed Black crested mangabey (Lophocebus aterrimus) spermatozoa obtained by transrectal ultrasonic-guided massage of the accessory sex glands and electroejaculation. Anim. Reprod. Sci. 210, 106176. doi: 10.1016/j.anireprosci.2019.106176
Gañán N., González R., Garde J. J., Martínez F., Vargas A., Gomendio M., et al. (2009). Assessment of semen quality, sperm cryopreservation and heterologous IVF in the critically endangered Iberian lynx (Lynx pardinus). Reproduction Fertility Dev. 21 (7), 848–859. doi: 10.1071/RD08226
Gillis A. B. (2020). Assisted reproductive technologies in male ambystoma tigrinum with application to threatened newt species (Mississippi State University).
Gillis A. B., Guy E. L., Kouba A. J., Allen P. J., Marcec-Greaves R. M., Kouba C. K. (2021). Short-term storage of tiger salamander (Ambystoma tigrinum) spermatozoa: The effect of collection type, temperature and time. PloS One 16 (1), e0245047. doi: 10.1371/journal.pone.0245047
González-Fernández L., Morrell J. M., Peña F. J., Macías-García B. (2012). Osmotic shock induces structural damage on equine spermatozoa plasmalemma and mitochondria. Theriogenology 78 (2), 415–422. doi: 10.1016/j.theriogenology.2012.02.021
Hardy M. P., Dent J. N. (1986). Regulation of motility in sperm of the red-spotted newt. J. Exp. Zoology 240 (3), 385–396. doi: 10.1002/jez.1402400313
Hayashi T., Yokotani N., Tane S., Matsumoto A., Myouga A., Okamoto M. (2013). Molecular genetic system for regenerative studies using newts. Development, growth & differentiation 55 (2), 229–236.
Helmy A., Halawa A., Montaser A., Zaabel S., Tawfik W., Gohar M., et al. (2023). The effects of fructose on the developmental competence, biochemical biomarkers and apoptotic gene expression by murine oocytes. Egyptian J. Veterinary Sci. 54 (3), 323–336. doi: 10.21608/ejvs.2023.177857.1412
Herrick J. R., Campbell M., Levens G., Moore T., Benson K., D’Agostino J., et al. (2010). In vitro fertilization and sperm cryopreservation in the black-footed cat (Felis nigripes) and sand cat (Felis margarita). Biol. Reprod. 82 (3), 552–562. doi: 10.1095/biolreprod.109.081034
Holt W. V. (2011). Mechanisms of sperm storage in the female reproductive tract: an interspecies comparison. Reprod. Domest. Anim. 46, 68–74. doi: 10.1111/j.1439-0531.2011.01862.x
Holt W. V., Fazeli A. (2016). Sperm storage in the female reproductive tract. Annu. Rev. Anim. Biosci. 4, 291–310. doi: 10.1146/annurev-animal-021815-111350
Holt W. V., Lloyd R. E. (2010). Sperm storage in the vertebrate female reproductive tract: how does it work so well? Theriogenology 73 (6), 713–722. doi: 10.1016/j.theriogenology.2009.07.002
Houck L. D., Palmer C. A., Watts R. A., Arnold S. J., Feldhoff P. W., Feldhoff R. C. (2007). A new vertebrate courtship pheromone, PMF, affects female receptivity in a terrestrial salamander. Anim. Behav. 73 (2), 315–320. doi: 10.1016/j.anbehav.2006.07.008
Ishihara K., Hosono J., Kanatani H., Katagiri C. (1984). Toad egg-jelly as a source of divalent cations essential for fertilization. Dev. Biol. 105 (2), 435–442. doi: 10.1016/0012-1606(84)90300-2
Jeong D. H., Yang J. J., Seo M. K., Lee A. N., Lim Y. K. (2019). Effectiveness of urethral catheterization under ultrasound guidance for semen collection from Asiatic black bears (Ursus thibetanus). Theriogenology 129, 154–159. doi: 10.1016/j.theriogenology.2019.02.032
Jeyendran R. S., van der Ven H. H., Perez-Pelaez M., Crabo B. G., Zaneveld L. J. D. (1984). Development of an assay to assess the functional integrity of the human sperm membrane and its relationship to other semen characteristics. Reproduction 70 (1), 219–228. doi: 10.1530/jrf.0.0700219
Jia J., Anderson J. S., Gao K. Q. (2021). Middle Jurassic stem hynobiids from China shed light on the evolution of basal salamanders. iScience 24 (7). doi: 10.1016/j.isci.2021.102744
Johnston S. D., Lever J., McLeod R., Qualischefski E., Brabazon S., Walton S., et al. (2014). Extension, osmotic tolerance and cryopreservation of saltwater crocodile (Crocodylus porosus) spermatozoa. Aquaculture 426, 213–221. doi: 10.1016/j.aquaculture.2014.02.014
Kahrl A. F., Snook R. R., Fitzpatrick J. L. (2022). Fertilization mode differentially impacts the evolution of vertebrate sperm components. Nat. Commun. 13 (1), 6809. doi: 10.1038/s41467-022-34609-7
Kalthur G., Salian S. R., Keyvanifard F., Sreedharan S., Thomas J. S., Kumar P., et al. (2012). Supplementation of biotin to sperm preparation medium increases the motility and longevity in cryopreserved human spermatozoa. J. Assisted Reprod. Genet. 29, 631–635. doi: 10.1007/s10815-012-9760-8
Kim E. K., Kim E. H., Kim E. A., Lee K. A., Shin J. E., Kwon H. (2015). Comparison of the effect of different media on the clinical outcomes of the density-gradient centrifugation/swim-up and swim-up methods. Clin. Experiment. Reprod. Medicine. 42 (1), 22 .
Kouba A. J., Vance C. K., Frommeyer M. A., Roth T. L. (2003). Structural and functional aspects of Bufo americanus spermatozoa: effects of inactivation and reactivation. J. Exp. Zoology Part A: Comp. Exp. Biol. 295 (2), 172–182. doi: 10.1002/jez.a.10192
Kouba A. J., Vance C. K., Willis E. L. (2009). Artificial fertilization for amphibian conservation: current knowledge and future considerations. Theriogenology 71 (1), 214–227. doi: 10.1016/j.theriogenology.2008.09.055
Lishko P. V., Kirichok Y. (2010). The role of Hv1 and CatSper channels in sperm activation. J. Physiol. 588 (23), 4667–4672. doi: 10.1113/jphysiol.2010.194142
Lueders I., Luther I., Scheepers G., Van der Horst G. (2012). Improved semen collection method for wild felids: urethral catheterization yields high sperm quality in African lions (Panthera leo). Theriogenology 78 (3), 696–701. doi: 10.1016/j.theriogenology.2012.02.026
Mahiddine F. Y., Kim M. J. (2021). Overview on the antioxidants, egg yolk alternatives, and mesenchymal stem cells and derivatives used in canine sperm cryopreservation. Animals 11 (7), 1930. doi: 10.3390/ani11071930
Mansour N., Lahnsteiner F., Patzner R. A. (2010). Motility and cryopreservation of spermatozoa of European common frog, Rana temporaria. Theriogenology 74 (5), 724–732. doi: 10.1016/j.theriogenology.2010.03.025
Mansour N., McNiven M. A., Richardson G. F. (2006). The effect of dietary supplementation with blueberry, α-tocopherol or astaxanthin on oxidative stability of Arctic char (Salvelinus alpinus) semen. Theriogenology 66 (2), 373–382. doi: 10.1016/j.theriogenology.2005.12.002
Marcec R. M. (2016). Development of assisted reproductive technologies for endangered North American salamanders (Mississippi State University).
Martínez-Páramo S., Diogo P., Dinis M. T., Herráez M. P., Sarasquete C., Cabrita E. (2012). Incorporation of ascorbic acid and α-tocopherol to the extender media to enhance antioxidant system of cryopreserved sea bass sperm. Theriogenology 77 (6), 1129–1136. doi: 10.1016/j.theriogenology.2011.10.017
Martínez-Pastor F., Álvarez M., Guerra C., Chamorro C. A., Anel-López L., de Paz P., et al. (2019). Extender osmolality, glycerol and egg yolk on the cryopreservation of epididymal spermatozoa for gamete banking of the Cantabric Chamois (Rupicapra pyrenaica parva). Theriogenology 125, 109–114. doi: 10.1016/j.theriogenology.2018.10.022
McDiarmid R. W. (1994). Amphibian diversity and natural history: an overview. Measuring and monitoring biological diversity: Standard methods for amphibians.
McGinnity D., Reinsch S. D., Schwartz H., Trudeau V., Browne R. K. (2021). Semen and oocyte collection, sperm cryopreservation and IVF with the threatened North American giant salamander Cryptobranchus alleganiensis. Reproduction, Fertility and Development 34 (5), 470–477.
Muchlisin Z. A., Hashim R. A. S. C., Chong A. S. C. (2004). Preliminary study on the cryopreservation of tropical bagrid catfish (Mystus nemurus) spermatozoa; the effect of extender and cryoprotectant on the motility after short-term storage. Theriogenology 62 (1-2), 25–34. doi: 10.1016/j.theriogenology.2003.05.006
Neagu V. R., García B. M., Sandoval C. S., Rodríguez A. M., Ferrusola C. O., Fernández L. G., et al. (2010). Freezing dog semen in presence of the antioxidant butylated hydroxytoluene improves postthaw sperm membrane integrity. Theriogenology 73 (5), 645–650. doi: 10.1016/j.theriogenology.2009.10.021
Peng L. Y., Xiao Y. M., Liu Y. (2011). Effect of cryopreservation and short-term storage of Chinese giant salamander sperm. Acta Hydrobiologica Sin. 35 (2), 325–331. doi: 10.3724/SP.J.1035.2011.00325
Pereira R., Sá R., Barros A., Sousa M. (2017). Major regulatory mechanisms involved in sperm motility. Asian J. Androl 19 (1), 5. doi: 10.4103/1008-682X.167716
Pérez L., Asturiano J. F., Yoshida M., Gallego V. (2022). Ionic control of sperm motility and trials for the improvement of pufferfish (Takifugu alboplumbeus) sperm extenders. Aquaculture. 554, 738146. doi: 10.1016/j.aquaculture.2022.738146
R Core Team (2022). R: A language and environment for statistical computing (Vienna, Austria: R Foundation for Statistical Computing). Available at: https://www.R-project.org/.
Santiago-Moreno J., Esteso M. C., Pradiee J., Castano C., Toledano-Díaz A., O’Brien E., et al. (2016). Giant panda (Ailuropoda melanoleuca) sperm morphometry and function after repeated freezing and thawing. Andrologia 48 (4), 470–474. doi: 10.1111/and.12468
Saragusty J., Hildebrandt T. B., Behr B., Knieriem A., Kruse J., Hermes R.. (2009). Successful cryopreservation of Asian elephant (Elephas maximus) spermatozoa. Animal Reproduction Science 115 (1-4), 255–266.
Sato T., Arimura T., Murata K., Kawamura M., Obama W., Suzuki M., et al. (2021). Differences of extracellular cues and Ca2+ permeable channels in the signaling pathways for inducing amphibian sperm motility. Zoological Sci. 38 (4), 343–351. doi: 10.2108/zs200159
Sever D. M. (1991). Comparative anatomy and phylogeny of the cloacae of salamanders (Amphibia: Caudata). II. Cryptobranchidae, Hynobiidae, and Sirenidae. J. Morphol 207 (3), 283–301. doi: 10.1002/jmor.1052070306
Sever D. M. (2000). “Sperm storage in female plethodontids with especial reference to the Desmognathinae,” in The biology of Plethodontid Salamanders (Boston, MA: Springer), 345–369.
Sever D. M. (2002). Female sperm storage in amphibians. J. Exp. Zoology 292 (2), 165–179. doi: 10.1002/jez.1152
Strelchenko N. S., Schmidt J. K., Mean K. D., Schotzko M. L., Golos T. G., Slukvin I. I. (2020). Cryopreservation of Mauritian cynomolgus macaque (Macaca fascicularis) sperm in chemically defined medium. J. Am. Assoc. Lab. Anim. Sci. 59 (6), 681–686. doi: 10.30802/AALAS-JAALAS-20-000059
Thuwanut P., Chatdarong K., Johannisson A., Bergqvist A. S., Söderquist L., Axnér E. (2010). Cryopreservation of epididymal cat spermatozoa: effects of in vitro antioxidative enzymes supplementation and lipid peroxidation induction. Theriogenology 73 (8), 1076–1087. doi: 10.1016/j.theriogenology.2010.01.007
Tsikis G., Reynaud K., Ferchaud S., Druart X. (2018). Seminal plasma differentially alters the resistance of dog, ram and boar spermatozoa to hypotonic stress. Anim. Reprod. Sci. 193, 1–8. doi: 10.1016/j.anireprosci.2018.01.012
Ukita M., Itoh T., Watanabe T., Watanabe A., Onitake K. (1999). Substances for the initiation of sperm motility in egg-jelly of the Japanese newt, Cynops pyrrhogaster. Zoological Sci. 16 (5), 793–802. doi: 10.2108/zsj.16.793
Uteshev V. K., Kaurova S. A., Shishova N. V., Stolyarov S. D., Browne R. K., Gakhova E. N. (2015). In vitro fertilization with hormonally induced sperm and eggs from sharp-ribbed newts Pleurodeles waltl. Russian J. Herpetol 22 (1), 1–6.
Keywords: amphibian, caudate, diluent, HAM’s, Holtfreter’s, milt, morphology, motility
Citation: Chen DM, Kouba CK, Songsasen N, Roth TL, Allen PJ and Kouba AJ (2024) Comparing novel sperm extenders for the internally-fertilizing tiger salamander (Ambystoma tigrinum). Front. Amphib. Reptile Sci. 1:1320803. doi: 10.3389/famrs.2023.1320803
Received: 12 October 2023; Accepted: 29 December 2023;
Published: 19 January 2024.
Edited by:
Alfredo Medrano, National Autonomous University of Mexico, MexicoReviewed by:
Alma Lilia Álvarez Guerreo, National Autonomous University of Mexico, MexicoCopyright © 2024 Chen, Kouba, Songsasen, Roth, Allen and Kouba. This is an open-access article distributed under the terms of the Creative Commons Attribution License (CC BY). The use, distribution or reproduction in other forums is permitted, provided the original author(s) and the copyright owner(s) are credited and that the original publication in this journal is cited, in accordance with accepted academic practice. No use, distribution or reproduction is permitted which does not comply with these terms.
*Correspondence: Andrew J. Kouba, YS5rb3ViYUBtc3N0YXRlLmVkdQ==
Disclaimer: All claims expressed in this article are solely those of the authors and do not necessarily represent those of their affiliated organizations, or those of the publisher, the editors and the reviewers. Any product that may be evaluated in this article or claim that may be made by its manufacturer is not guaranteed or endorsed by the publisher.
Research integrity at Frontiers
Learn more about the work of our research integrity team to safeguard the quality of each article we publish.