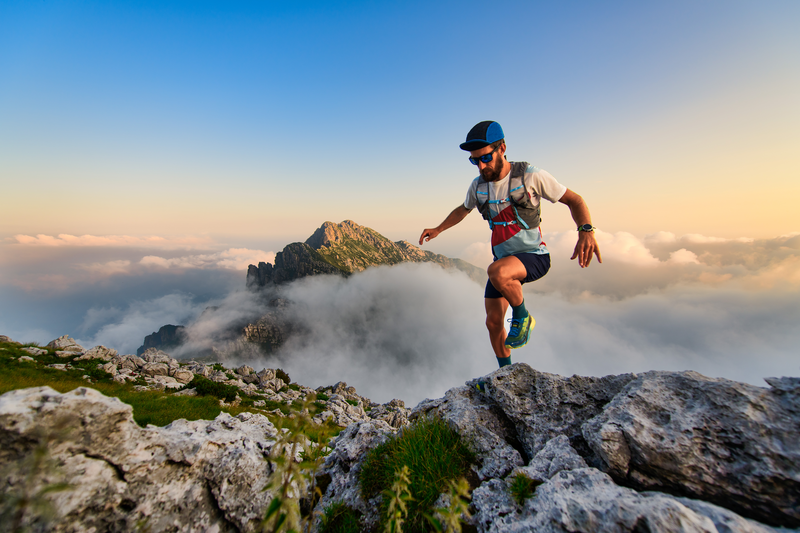
94% of researchers rate our articles as excellent or good
Learn more about the work of our research integrity team to safeguard the quality of each article we publish.
Find out more
ORIGINAL RESEARCH article
Front. Amphib. Reptile Sci. , 29 August 2023
Sec. Phylogenetics and Paleobiology
Volume 1 - 2023 | https://doi.org/10.3389/famrs.2023.1217025
The basal alligatoroid crocodylian Diplocynodon kochi erected for incomplete three-dimensional skull from the late Eocene (Priabonian) Cluj Limestone Formation in Romania, represents one of the easternmost distributed European members of Diplocynodontidae. New isolated cranial and postcranial remains provide new insights into the diagnostic features, phylogenetic relationships and lifestyle of this taxon, extending its fossil record to four new localities situated on the north-western side of the Transylvanian Basin. Diagnostic traits of the holotype include an extended insertion surface of jaw adductors on the parietal and squamosal, whereas the newly referred mandibles possess an enlarged and procumbent first dentary tooth, and the posterior teeth and alveoli are mediolaterally compressed. These attributes might have been related to the prey capture approach of D. kochi, involved undoubtedly in the food chains of both continental (fluvial or marshy-lacustrine) and shallow marine environments, as indicated by the taphonomic settings of the surveyed fossil bearing deposits. The warm and humid climate is indicated for the late Eocene (Priabonian) of the Transylvanian Basin based on palynomorphs, however, the presence of “growth rings” and “lines of arrested growth” on the prezygapophyses of a dorsal vertebra referred to D. kochi, points to a seasonal climate, installed well before the Eocene–Oligocene terminal event. Positioned between western and eastern European faunas, the diplocynodontid populations from the Transylvanian Basin survived probably the Eocene–Oligocene transition, as suggested by the early Oligocene (Rupelian) fossil record from the area.
Diplocynodontidae represent a basal alligatoroid crocodylian clade exclusively known from Europe and their fossil record covers the late Paleocene–middle Miocene interval (Buscalioni et al., 1992; Brochu, 1999; Delfino et al., 2007; Martin, 2010; Martin and Gross, 2011; Delfino and Smith, 2012; Díaz Aráez et al., 2017; Chroust et al., 2019; Luján et al., 2019; Rio et al., 2020; Chroust et al., 2021; Massonne and Böhme, 2022; Venczel and Codrea, 2022). Until present, 12 valid species of Diplocynodon have been erected (D. buetikonenis, D. darwini, D. deponiae, D. elavericus, D. hantoniensis, D. muelleri, D. ratelii, D. remensis, D. tormis, D. ungeri) (Rio et al., 2020; Massonne and Böhme, 2022); the list includes also D. kochi, described recently from the late Eocene (Priabonian) of Romania (Venczel and Codrea, 2022) and D. levantinicum from the late Oligocene (Chattian) of Bulgaria; the latter has been revised by Massonne and Böhme (2022).
Diplocynodon kochi represents the first example of a diplocynodontid crocodylian discovered in marine sedimentary environment (Codrea and Venczel, 2020; Venczel and Codrea, 2022). The bioclastic limestone block enclosing the incomplete skull (holotype) of D. kochi, recovered more than 130 years ago from the former Cluj-Mănăştur limestone quarry (Koch, 1894), is part of the Cluj Limestone Formation and corresponds to a shallow marine taphonomic setting. Previously, apart from the partial skull, described briefly by Koch (1894) from the Cluj-Mănăştur open pit, indetermined cranial remains and isolated teeth of late Eocene (Priabonian) age were also reported from the localities of Someş Dam and Rădaia (Koch, 1894; Pávay, 1871; Codrea and Fărcaş, 2002), and Treznea (Fărcaş, 2011; Codrea and Venczel, 2020).
In few recent years, despite the scarcity of Paleogene crocodylian remains, we recognized further crocodylian fossils from several localities of the Transylvanian Basin, Romania, corresponding to three distinct crocodylian groups, as it follows: (1) planocraniids from the late Paleocene (Thanetian) of Jibou (Venczel et al., 2023), (2) gavialoids from the middle Eocene of Turnu Roşu (Venczel and Codrea, 2022), and (3) diplocynodontids from the late Eocene and early Oligocene of Gilău and Meseş sedimentary areas, NW of the Transylvanian Basin (Fărcaş, 2011; Codrea and Venczel, 2020; Sabău et al., 2021; Venczel and Codrea, 2022).
The present study is devoted to a series of newly recognized isolated diplocynodontid remains, recovered from the late Eocene of Gilău and Meseş sedimentary areas. The fossils, consisting of isolated cranial, vertebral and appendicular skeletal parts, bear diagnostic traits of D. kochi, and therefore are referable to that taxon. Part of the studied localities represent shallow marine settings (Cluj-Mănăştur and Leghia), while others were deposited in fluviatile or marshy-lacustrine sedimentary environment (Rădaia and Treznea). Apart from the recently described holotype skull of D. kochi (Venczel and Codrea, 2022), the newly referred specimens include among others a partial left jugal from Rădaia, listed in the Antal Koch’s collection (Babeş-Bolyai University, Cluj-Napoca) as “Crocodylus sp.” (Codrea and Venczel, 2020: Figure 4A), another fragmentary jugal and the anterior part of a dentary from Treznea (Fărcaş, 2011) and a nearly complete lower jaw, associated with a posterior mandible, from the limestone quarry of Leghia-Tabără (see below). The new information derived from phenotypic traits of the newly identified isolated specimens, expectantly will provide further details on diagnostic features, phylogenetic relationships and lifestyle of this peculiar alligatoroid crocodylian, apparently able to survive the Eocene–Oligocene transition.
The Transylvanian Basin, a geomorphological unit surrounded by the Carpathian Mountains, started its development in the Upper Cretaceous and include four tectonostratigraphic megacycles (Krézsek and Bally, 2006). The Paleogene sediments, sometimes with rare terrestrial vertebrate remains, mostly occur in marginal areas of the Transylvanian Basin (Krézsek and Bally, 2006), however, the most important finds are located in the northwestern parts of that basin (Koch, 1894; Codrea et al., 1997; Codrea and Venczel, 2020; Sabău et al., 2021) (Figure 1).
Figure 1 Map of the crocodylian-bearing Paleogene fossil localities from NW Transylvania, Romania (modified after Răileanu and Saulea, 1967). 1. Treznea (late Eocene, early Priabonian); 2. Rădaia (late Eocene, early Priabonian); 3. Suceag (early Oligocene, Rupelian); 4. Someş Dam, Cluj-Napoca (late Eocene, late Priabonian); 5. Cetăţuia, Cluj-Napoca (early Oligocene, Rupelian); 6. Cluj-Mănăştur (late Eocene, late Priabonian); 7. Leghia-Tabără (late Eocene, early Priabonian).
During the Paleocene and early Eocene, coarse to fine-grained reddish alluvial-fan and fluvial sediments accumulated in subsidiary sag basins (Hosu, 1999), sometimes with short episodes of lacustrine interbedding (Gheerbrant et al., 1999; Codrea and Hosu, 2001). These continental deposits (Jibou Formation), ranging in thickness from several meters to more than 500 meters, were coined out first by Hofmann (1879), also known in the old stratigraphic nomenclature as “lower variegated red shales” (Koch, 1894) or lower variegated complex (Răileanu and Saulea, 1956).
The middle to late Eocene sedimentary succession corresponds to two transgressive–regressive cycles that constitute the Călata and Turea Groups, each composed of evaporites, shallow-marine carbonates, outer shelf marls, shallow-marine sands and on the top fine-grained fluvial deposits (Koch, 1894; Proust and Hosu, 1996; Mészáros, 2000; Krézsek and Bally, 2006). The first marine series of Călata Group ends with the Viştea Limestone Formation (Rusu, 1995), known also as “lower coarse limestone” (Koch, 1894), and with the Rakoczi Sandstone (Mészáros, 2000). The fine-grained fluviatile deposits of the Valea Nadăşului Formation (Popescu, 1976), also known as “upper variegated clay series” (Koch, 1894), mark the reestablishment of the continental sedimentary environment. The second marine series of Turea Group (Rusu, 1995), or “upper marine series” (Răileanu and Saulea, 1956) include the Jebucu Formation (Bombiță, 1984), followed by the Cluj Limestone Formation, or “upper coarse limestone” (Koch, 1894), and the Brebi Formation, where the Gigantostrea gigantea level marks the Eocene–Oligocene boundary (Mészáros, 2000). The Hoia Limestone Formation and the Mera Formation close the marine series of the Turea Group (Mészáros et al., 1989). The continental series was re-established again with the deposition of the Moigrad Formation and it was continued with the brackish deposits of Gruia and Dâncu formations (Mészáros, 2000). The late Eocene localities yielding fossil remains of Diplocynodon correspond to both marine and continental taphonomic settings (see below).
The fossiliferous outcrop is situated on the southwestern vicinity of Leghia village (Gilău sedimentary area, Cluj County) within an active limestone quarry (closed today), where the limestone of about five meters in thickness was extracted as block-shaped pieces; subsequently the blocks were cut into slices and used for building decorations (Sabău et al., 2021). The sediments outcropping in the quarry are part of the Viştea Limestone Formation (late Eocene, early Priabonian) and are composed of compact packstone, bioclastic calcarenite with crushed bioclasts composed of calcareous algae, echinoderms, and foraminifers including Alveolina elongata and Nummulites fabianii (Popescu et al., 1978), biomicrite with some terrigenous inputs (quartz, feldspar, muscovite, biotite, clay minerals, limonite) that originate from nearby land surfaces (Sabău et al., 2021). The fossils identified from this formation include mollusks (Panopea oppenhaimi, Thracia stenochora, Tellina sp., Cardiopsis sp., Corbulla sp., C. gallica, Lucina sp., L. mutabilis, Chlamys subimbricatus, Ostrea cymbula, Terebellum sp.) (Mészáros et al., 1987), the sea urchin Sismondia occitana (Mészáros et al., 1987) and isolated shark teeth (Koch, 1894; Koch, 1900).
The fossil locality is situated within the village of Rădaia (Gilău sedimentary area, Cluj County). The sedimentary succession, consisting of fine-grained fluviatile deposits that might correspond to a flood plain, belongs to the Valea Nadăşului Formation (late Eocene, early Priabonian). Rădaia is the type locality of the rhinocerotoid Prohyracodon orientale Koch, 1897 and of the brontothere Brachydiastematherium transilvanicum Böck and Matyasowski, 1876 (Böck, 1876). Beside mammals, the fossil locality has yielded also carapace and plastron fragments of the turtle Trionyx clavatomarginatus (Fărcaş, 2011) and isolated teeth of crocodylians (Koch, 1894).
The fossil locality is situated in the Şanţului Valley near the locality of Treznea (Meseş sedimentary area, Sălaj County). The continental sediments belong to Turbuţa Formation (late Eocene, early Priabonian), that may be considered a lateral correspondent of the Valea Nadăşului Formation (Fărcaş, 2011). The taphonomic setting indicates a marshy-lacustrine paleoenvironment with occurrence of charophytes and a series of palynomorphs representing gymnosperms (Cupressaceae and Taxodiaceae) and angiosperms (Fagaceae, Juglandaceae, Arecaceae and Nyssaceae) that corresponds to a paleoclimatic curve with mean annual temperature of around 20°C and mean annual precipitation of about 1,200 mm (Petrescu and Balintoni, 2004). The faunal association, after Fărcaş (2011) has yielded, among others lepisosteid fish, freshwater turtles (?Mauremys), isolated teeth of alligatoroid crocodylians, marsupial mammals (Peratherium lavergnense) and cricetid rodents (Atavocricetodon cf. atavus, cf. Pseudocricetodon sp.).
The geologic strata of the presently abandoned limestone quarry belong to the Cluj Limestone Formation (Gilău sedimentary area, late Priabonian) (Mészáros, 2000). These strata consist of three coarse bioclastic limestone beds, being rich in calcareous algae, ostracods, gastropods and bivalve shells (e.g., Anomia tenuistriata) and alternate with calcareous or clayey marls (Koch, 1894), accumulated in a shallow marine carbonate platform. Occasionally, the carbonate platform emerged and exposed to atmospheric erosion (Codrea et al., 1997). After Koch (1894), a partial skull of a small crocodylian, reminiscent of Crocodylus communis (i.e., C. niloticus), was recovered by quarry workers; that specimen actually embodies the holotype of Diplocynodon kochi (Venczel and Codrea, 2022). In addition, the former Cluj-Mănăştur open pit is the type locality of the ardeiform bird Eostega lebedinskyi Lambrecht, 1929.
The fossil material examined in this study consists of an incomplete skull, isolated jugals, lower jaws, isolated teeth, isolated femora and a dorsal vertebra. The incomplete three-dimensionally preserved skull from Cluj-Mănăştur open pit (UBB V. 1453, holotype of Diplocynodon kochi), originally embedded in coarse limestone and broken into three pieces of rock, was prepared mechanically using an air-scribe and chisels, then strengthened and reunited using mowilith (Venczel and Codrea, 2022). Several specimens originate from Leghia-Tabără limestone quarry as sliced limestone plates of variable thickness (from 9 mm to 55 mm), where the fragmentary specimens are partly embedded or exposing only sections of bones when the rock was sliced up; these specimens were further prepared under a stereomicroscope using chisels and then strengthened. Unfortunately, the counterparts of the holotype specimen or those derived from limestone plates from the Leghia-Tabără open pit, potentially bearing further morphological details, were not retained and subsequently are lost. The isolated jugal specimen from Rădaia (UBB V. 1449) was listed as “Crocodylus sp.” by Koch (1894). The specimens from Treznea, resulted from screen-washing of about 500 kg marshy-lacustrine sediments, were cleaned, dried and strengthened using mowilith. The digital images were captured with a Canon Eos digital camera using tilt-shift and macro-objective. Cladistic analyses were conducted with the phylogenetic software package TNT version 1.6 of Goloboff and Morales (2023). The skeleto-chronological methodology used for crocodylian vertebrae follows Venczel et al. (2015). Common English terms and the standard anatomical orientation system are used throughout this paper. The fossil material described herein belongs to the collections of the Geological Department Paleontological Museum of Babeş-Bolyai University, Cluj-Napoca, Romania and to Ţării Crişurilor Museum, Oradea, Romania.
IRSNB – Institut royal des Sciences naturelles de Belgique, Bruxelles; MNHN – Muséum national d’Histoire naturelle, Paris, France; MTC – Ţării Crişurilor Museum, Oradea; NHMUK – Natural History Museum, London; UBB – Babeş-Bolyai University, Cluj-Napoca.
Eusuchia Huxley, 1875, sensu Brochu, 2003
Crocodylia Gmelin, 1789, sensu Benton & Clark, 1988
Alligatoroidea Gray, 1844, sensu Brochu, 2003
Diplocynodontinae Brochu, 1999
Genus Diplocynodon Pomel, 1847
Diplocynodon kochi Venczel & Codrea, 2022
UBB V.1453, a three-dimensionally preserved incomplete skull (Figure 2A), missing from its right side the anterolateral margin of premaxilla, most part of the posterior margin of maxilla, anterior margin of the jugal, also lacking from its left side the postorbital, squamosal, jugal, quadratojugal and ventrally the posterior part of the palatine and left ectopterygoid; most parts of the cranial openings are infilled by matrix.
Figure 2 Fossil remains of Diplocynodon kochi from the late Eocene (Priabonian) of the Transylvanian Basin. (A) Holotype (UBB V.1453) in right lateral view; (B, C) partial left jugal from the late Eocene of Rădaia, Valea Nadăşului Formation, Romania (UBB V. 1449) in lateral and medial views; (D) mirrored image of left jugal (MTC 26015) in lateral view; (E) left jugal in medial view and with interpretive outlines; (F). fr, frontal; ju, jugal; juf, jugal foramen; la, lacrimal; ma, maxilla; na, nasal; pa, parietal; pf, prefrontal; pm, premaxilla; po, postorbital; pob, postorbital bar; qj, quadratojugal; qjjc, quadratojugal–jugal contact; qu, quadrate; so, supraoccipital; sq, squamosal. Arrows point at the synapomorphies discussed in the text. Scale bar = 10 mm.
Leghia: paired dentaries (UBB V. 1004/1), posterior part of right mandible (UBB V. 1004/2), isolated tooth (UBB V. 1008), trunk vertebra (UBB V. 1007), femora (UBB V. 1005, 1006); Treznea: left jugal (MTC. 26015), isolated dentary (MTC. 26016), three isolated teeth (UBB V. uncatalogued); Rădaia: partial left jugal (UBB V. 1449).
Diplocynodon kochi is a small-sized late Eocene member of Diplocynodontidae with an estimated total body length of about 1.6–1.8 m. D. kochi is diagnosed by the following unique combination of characters (autapomorphy marked by *): (1) premaxillary surface with deep notch lateral to naris; (2) incisive foramen larger than half of the greatest width of premaxillae; (3) occlusion pits on the premaxillae positioned medially to alveoli; (4) bony ridge on the lacrimal is prominent and wide with lateral overhang; (5) dermal bones of skull roof overhang rim of supratemporal fenestra; (6*) medial wall of parietal with deep recess; (7) anterior process of quadratojugal short along lower temporal bar; (8) margin of otic aperture inset from paroccipital process; (9) lateral carotid foramen opens dorsally to basisphenoid at maturity; (10) posterior series of 12th–16th maxillary teeth and alveoli, and 14th–19th dentary teeth and alveoli mediolaterally compressed, (11*) first dentary tooth enlarged and procumbent; (12) dentary symphysis short, extending to the posterior margin of the 4th alveoli, and (13) splenial excluded from the symphysis.
Jugal (Figures 2D–F). The isolated jugals are elongate and anteriorly widening bones that in life delimited lateroventrally the orbit and the infratemporal fenestra. In lateral view, the MTC 26015 specimen, corresponding to a left jugal (Figure 2D, represented as a mirror image), has both the anteriormost margin (in life, articulating with the lacrimal) and the posteriormost termination (in life, contacting the quadratojugal) are damaged. The outer surface of the bone is moderately convex, its anteroventral portion slightly bowed medially and covered by a strong sculpture consisting of a network of ridges delimiting rounded or elongated pits. The anterior ramus is widened with its dorsal margin more elevated and produced into a sharp dorsal crest, corresponding to the lower periorbital crest (Andrade and Hornung, 2011). In medial view, the deepest part of the anterior ramus is reached in the anterior vicinity of the postorbital bar. The ascending process of the postorbital bar is broken off, but the preserved part indicates that it was detached medially from the body of the jugal by the dorsal crest, similarly to that in the holotype of D. kochi. Anterior to the remnants of the ascending process of the jugal, there are two foramina preserved, of which the anterior one is larger and the posterior one is smaller. The posterior ramus of the jugal is rod-like and somewhat damaged, however, it exposes the articulating surface for the quadratojugal along the posterodorsal margin. Medially to the latter, there are two shallow grooves that may represent the contact surface with the extremely short anterior process of the quadratojugal. On the posterior vicinity of the ascending process of the jugal, there are two small foramina preserved in a shallow concavity. The specimen from Rădaia (UBB V. 1449), was identified by Koch (1894: p. 225) as a flattened and 1.5 cm wide crocodylian bone, sculptured labially by deep hemispherical pits. It resembles closely the above specimens in possessing a complete periorbital crest (i.e., the postorbital bar is inset from the lateral jugal surface) and a relatively large jugal foramen (filled with sediment). Unfortunately, it possesses a more fragmentary posterior ramus (Figures 2B, C), and therefore the imprint of the quadratojugal’s anterior process is not preserved.
Dentary (Figures 3A–E). The MTC 26016 specimen (Figures 3A–D) represents the anterior part of a right dentary, preserving a series of nine alveoli. The dorsal mandibular margin is preserved completely showing a dorsal convexity at the level of the first alveolus and at the level of the third and fourth alveoli. However, the bone is somewhat crashed mediolaterally, especially near to the dorsal alveolar margin, caused probably by lithostatic pressure. The lateral surface is strongly sculptured, whereas the medial surface exposes widely the Meckelian groove. The splenial is broken off, however, part of its posterior lamina is preserved in the embedding matrix. The anterior limit of the splenial on the ventral side is marked by a slanting groove that reaches the level of the fifth alveolus; the imprint on the dorsal side is observed somewhat posterior to that of the ventral side. The above morphology indicates that the splenial is excluded from the symphysis. The first alveolus is situated at the anterior margin of the dentary near the symphysial limit, and similar to the UBB V. 1004/1 specimen (Figure 3E), the first tooth was probably procumbent. The second alveolus is smaller, whereas the third one is hidden by the broken part of the outer mandibular margin, shift over that alveolus. The fourth alveolus, preserving at its base a tooth remnant, was probably as large as the third alveolus. The remaining alveoli are relatively small and placed in a straight line. The symphysis extends backwards to the level of the posterior margin of the fourth alveolus (Figure 3D).
Figure 3 Isolated lower jaw bones of Diplocynodon kochi. (A–D) Right dentary from the late Eocene (Priabonian) of Treznea, Romania (MTC 26016) in lateral (A), dorso-medial (B), medial (C) and with interpretive outlines (D), arrows point to levels of the symphysial posterior margin; (E) partial paired dentary from the late Eocene (Pribonian) of Leghia, Romania (UBB V. 1004/1), arrows point to replacement teeth; (F) partial sectioned right postdentary bones from the late Eocene (Pribonian) of Leghia, Romania (UBB V. 1004/2). an, angular; ar, articular; de, dentary; isp, imprint of the splenial; mg, Meckelian groove; rp, retroarticular process; sp, splenial; su, surangular; sy, symphysis; 1, 3, 4, 7, 14 – tooth positions/alveoli in the dentary. Arrow points at the synapomorphy discussed in the text. Scale bars = 10 mm.
The UBB V. 1004/1 specimen (Figure 3E), partly embedded in a limestone slab, represents a nearly horizontally sectioned surface of a fused pair of dentaries, exposing on both sides 19 alveoli. The dentary gently curves between the fourth and tenth alveoli, and it is slightly festooned at the level of the third and fourth largest alveoli. The symphysis extends backwards to the level of the posterior margin of the fourth alveoli; the paired dentaries share an articulating angle of about 30°. The pair of first alveoli have a gently anterolaterally oriented, elongate-oval shape indicating that the pair of first mandibular teeth were strongly inclined anteriorly (i.e., procumbent). The second alveoli are situated in the near posterior vicinity of the first pair, however in life, when measured on the dorsal surface of the dentary, the distance between the first and second alveoli might have been the same as the interval between the second and third alveoli. The third and fourth alveoli are enlarged, almost equal in size and confluent, and slightly oriented laterally. The next largest alveoli are the 11th and the 12th (better exposed on the left side), whereas the last six alveoli are smaller in size and are compressed medio-laterally. At the base of several tooth sockets small replacement teeth have been preserved. The tooth crown in these replacement teeth appears slightly compressed and conical, provided with mesiodistal carina and devoid of any trace of striations (Figure 3E). The splenial is strongly damaged, but the remaining parts and the impression left on the dentary indicates that its anterior tip reached the level of the fifth alveoli, and as a consequence it was excluded from the mandibular symphysis; the anterior tip of the splenial passes ventral to Meckelian groove.
Posterior mandible fragment (Figure 3F). The UBB V. 1004/2 specimen, represents the posterior fragment of the mandible that has been detached probably from the right dentary and preserved on the same limestone slab with the fused pair of dentaries (Sabău et al., 2021: Figure 2). The mandible fragment lays on its medial side, the sectioned surface preserving parts of the splenial, angular, surangular, articular and the retroarticular process; part of the sculptured surface of the angular is also preserved. The external mandibular window was present, but only the trace of its posteroventral side is preserved. The ventral part of the surangular is visible on the posterolateral side of the external mandibular window, contacting the angular laterally and the articular medially. The imprint of the retroarticular process suggests that it was oriented posterodorsally. Apparently, both the surangular and angular reached posteriorly the tip of the retroarticular process.
Isolated teeth (Figures 4A–C). Based on the tooth morphology the available specimens may be partitioned in two morphotypes: (1) caniniform and (2) conical.
Figure 4 Isolated specimens of Diplocynodon kochi from the late Eocene of the Transylvanian Basin. (A) Isolated tooth (UBB V. 1008) from the late Eocene of Leghia, Romania; (B, C) isolated teeth (UBB V. uncatalogued) from the late Eocene of Treznea, Romania; (D) isolated dorsal vertebra (UBB V. 1007), arrows point to the dorsal surfaces of the prezygapophyses displaying “growth rings” (1–10); (E, F) partial, sectioned femurs from the late Eocene (Priabonian) of Leghia, Romania; (G) posterior side of dorsal vertebra (UBB V. 1007). ct, cotyle; ftr, fourth trochanter; ns, neural spine; pre, proximal epiphysis; tp, transverse process. Scale bars = 10 mm.
The single specimen from Leghia-Tabără, partly embedded in the matrix (Figure 4A), and some of the available tooth crowns from Treznea (Figure 4B) are of caniniform morphotype and may represent the anterior series of D. kochi. The crown surfaces exhibit mesiodistal carinae and are devoid of serrations. Some of the specimens bear few gentle ridges near the base of the crown.
The teeth of the second morphotype are distinctly smaller in size and have a conical shape (Figure 4C), representing probably the posterior series on the maxilla or dentary. These are provided with mesiodistal carinae and are devoid of serrations. Some weakly defined irregular carinae extend along both the lingual and labial sides of the tooth crown.
Dorsal vertebra (Figures 4D, G). The specimen UBB V. 1007, is the only partial dorsal vertebra that has been recovered from a limestone slab in the open pit of Leghia-Tabără. The cutting plane affected the anterior margins of the prezygapophyses only, whereas the whole posterior half of the vertebra was cut out, including the postzygapophyseal area; the dorsal tip of the neural spine is also missing. The vertebral centrum appears triangular with the lateral surface apparently smooth; the cotyle is moderately deep. The prezygapophyses are of moderate width, gently inclined medially and bearing on their dorsal surface ten or eleven well-defined annual growth lines (Figure 4D). The transverse processes are extremely long, about twice the width of the centrum, and are almost horizontal (Figure 4G). The neural spine is high, whereas the neural canal is circular.
Femur (Figures 4E, F). Two femora have been identified among the already cut limestone slabs. Both the available specimens are of sigmoidal outline. However, the larger specimen (UBB V. 1005), embedded partially in the matrix is strongly eroded, whereas the cutting plane in the smaller specimen (UBB V. 1006), exhibits on the proximal metaphysis the insertion surface of M. pubo-ischio-femoralis internus and part of the fourth trochanter.
In our previous cladistic analysis (Venczel and Codrea, 2022), the family Diplocynodontidae was recovered as a monophyletic clade, where the relationships of D. kochi, based on phenotypic characters of the holotype specimen, with the included seven congeners appear fully resolved. In the present analysis, I have reviewed all the scorable characters and have completed with those of the newly referred specimens of D. kochi (jugals, mandible, teeth and vertebra). I added all the scored 98 phenotypic characters (52% from the character list) to the character–taxon matrix of Massonne and Böhme (2022), altogether consisting of 105 operational taxonomic units and 187 phenotypic characters (see Supplementary files); all characters were treated as unordered. The new character–taxon matrix includes 11 species of Diplocynodon (D. darwini, D. deponiae, D. elavericus, D. hantoniensis, D. kochi, D. levantinicum, D. muelleri, D. ratelii, D. remensis, D. tormis and D. ungeri) (Massonne and Böhme, 2022). In the parsimony analysis, using the TNT version 1.6 (Goloboff and Morales, 2023), the character–taxon matrix was first analyzed using the “New Technology search” option with the sectorial search, ratchet, tree drift and tree fusing options as default parameters. A search for suboptimal trees ten steps longer than that of the most parsimonious tree was also completed in order to calculate the decay indices of Bremer (1994), and the common synapomorphies for all trees were also identified.
In the analysis, the TNT returned eight most parsimonious trees of 758 steps from a total of 43.808.463 rearrangements examined (consistency index = 0.311; retention index = 0.784; homoplasy index = 0.689; rescaled consistency index = 0.243) (Figure 5). The strict consensus tree represents Diplocynodontidae as a monophyletic clade, situated in a basal position within Alligatoroidea, and where Leidyosuchus canadensis is the basalmost alligatoroid and sister-taxon to all other alligatoroid clades. Within Diplocynodontidae, the pair of D. deponiae and D. darwini are recovered in an unresolved nesting position, and as sister-taxa to the remaining congeners. Among the latter group, the relationships of D. tormis, D. ratelii, D. muelleri, D. levantinicum and D. hantoniensis are unresolved, whereas D. remensis, D. kochi, D. ungeri and D. elavericus form an unnamed clade with D. remensis situated stem ward, and D. ungeri + D. elavericus are positioned crownward, as sister-taxon of each other. Nodal support (calculated from 94.041 trees) remains weak for both Alligatoroidea and Diplocynodontidae (decay index = 2), and moderate to Alligatoridae (decay index = 3).
Figure 5 Relationships of Diplocynodon kochi, based on phylogenetic analysis. Strict consensus tree resulting from eight most parsimonious trees generated by TNT, based on 105 operational taxonomic units with 187 phenotypic characters, derived from Massone and Böhme (2022). Arrow points to clade of Alligatoroidea. The numbers above nodes indicate decay indices of Bremer (= extra steps).
The clade of Alligatoroidea is supported by five common synapomorphies: the third and fourth dentary alveoli are nearly the same size and confluent (ch. 47-0), the anterior processes of surangular are sub-equal to equal (ch. 61-1), the foramen aëreum is inset from the margin of the retroarticular process (ch. 70-1), the maxilla separates broadly the ectopterygoid from the maxillary tooth row (ch. 103-1) and the quadrate foramen aëreum is located on the dorsal surface of the quadrate (ch. 175-1). The clade of Diplocynodontidae is supported also by five common synapomorphies: axial hypapophysis located toward the midpoint of centrum (ch. 15-0), the posterior tip of the dorsal margin of iliac blade is very deep (ch. 34-4), presence of paired ventral ossifications that suture together (ch. 42-2), frontoparietal suture is linear between supratemporal fenestrae (ch. 149-1), and one row of postoccipital osteoderms (ch. 181-1). Finally, within Diplocynodontidae, the unnamed clade clustering D. remensis, D. kochi, D. ungeri and D. elavericus, is supported by three common synapomorphies: posterior teeth and alveoli in the maxilla and/or dentary laterally compressed (ch. 79-1), fourth dentary tooth occludes in notch between the premaxilla and maxilla early in ontogeny (ch. 91-0), and the postorbital-squamosal suture oriented ventrally (ch. 144-0). The unresolved intrageneric relationships of Diplocynodon are linked probably to the relatively high number of missing characters in some of the congeners (e.g., D. levantinicum, D. kochi and D. elavericus).
In the second analysis, I added separately to the CTM the scored characters resulted from the Leghia-Tabără specimen (11 characters) and I used for D. kochi only the scored characters derived from the holotype. As in the previous analysis, all characters were treated as unordered. As expected, the TNT recovered the Diplocynodontidae as a monophyletic assemblage, where the Leghia specimen appears as the sister taxon of D. kochi (Supplementary file 1).
The new isolated specimens, referred here to Diplocynodon kochi, come from both marine and continental late Eocene (Priabonian) sediments of a rather limited sedimentary area, located on the northwestern border of the Transylvanian Basin. In fact, these examples are the only crocodylian fossils of that age, originating presumably from the same source area. The new specimens share with the holotype of D. kochi a combination of derived character states that allows their assignment to this species.
The jugal specimen from Trezenea (MTC 26015) exposes the imprint of an extremely short anterior process of the quadratojugal, a derived character state shared with the holotype of D. kochi (ch. 142-1), and also with D. elavericus, known from the late Eocene of France (Martin, 2010) and D. ungeri, known from the middle Miocene of Austria (Martin and Gross, 2011). In the holotype and in both the isolated jugals the lower periorbital crest (sensu Andrade and Hornung, 2011) is produced into a sharp and posteriorly descending crest that separates the postorbital bar from the jugal’s dorsolateral margin (ch. 133-1), whereas the medial jugal foramen, situated at the base of postorbital bar, is relatively large (i.e., at least half of the thickness of the postorbital bar) (ch. 101-1).
In the fused pair of dentaries, the last six dentary tooth positions, similarly to the last five maxillary alveoli in the holotype of D. kochi, are compressed mediolaterally (ch. 79-1). Within the genus, this character state is shared only with D. remensis, known from the late Paleocene of Mont de Berru, Marne, France (Martin et al., 2014), and with D. elavericus (see above).
The isolated tooth crowns, the femora and the dorsal vertebra are reminiscent of other members within the genus Diplocynodon. However, these elements were found in the same locality with the more diagnostic specimens, and therefore may be attributed to D. kochi, the only known late Eocene crocodylian from the Transylvanian Basin.
Venczel and Codrea (2022) reported a single autapomorphy preserved in the skull of D. kochi: (1*) the medial wall of parietal forms a deep recess in the supratemporal fenestra. This feature might have been associated with an extended insertion surface on the parietal and squamosal of the external jaw adductor muscles (i.e., musculus adductor mandibulae externus profundus), being in control for rapid closure of the lower jaws in a palinal type jaw movement (Holliday and Witmer, 2007; Ősi, 2014). This capability could be useful in ambush-type capture of fast-moving prey, like smaller fish from fluviatile, lacustrine or shallow marine waters, frequented potentially by D. kochi. In the remaining members, where the medial wall of the supratemporal fenestra is preserved, that recess is lacking or only weakly developed. In D. hantoniensis, the medial wall of the supratemporal fenestra is steep, faces dorsolaterally, and the parietal rim slightly overhangs the supratemporal fenestra (Rio et al., 2020). However, in D. hantoniensis a shallow fossa occurs at the anterior corner of the supratemporal fenestra (Rio et al., 2020), which is also the case in D. ungeri (Martin and Gross, 2011). In D. deponiae (Delfino and Smith, 2012: Figure 2A), D. ratelii (Dı́az Aráez et al., 2017: Figures 2 A, G; 5A) and D. tormis (Serrano-Martı́nez et al., 2019: Figure 1E), the parietal wall is well-exposed from above and devoid of deep recess. In D. muelleri, a well-defined sub-horizontal medial parietal shelf has been reported as a distinctive feature (Piras and Buscalioni, 2006), moreover, a somewhat similar structure was reported in Diplocynodon cf. D. muelleri by Macaluso et al. (2019: Figure 3D).
A second autapomorphic feature is preserved in the referred UBB V. 1004/1 specimen, representing a fused pair of dentaries: (2*) the enlarged first dentary tooth is steeply inclined anteriorly (procumbent), whereas the remaining anterior teeth are directed dorsally; this condition may be considered a local autapomorphy of D. kochi. In UBB V. 1004/1, the nearly horizontally applied cutting plane in the limestone slab revealed a pair of elongated alveoli in the fused dentary, that in life might correspond to an enlarged and strongly anteriorly inclined insertion (i.e., procumbent) of the first dentary tooth. The only other available specimen from Treznea displays a similarly positioned and enlarged first tooth alveolus, whereas the second one is much smaller, positioned posteriorly at some distance from the first tooth and oriented dorsally. The NHMUK OR 30394 specimen, representing a well-preserved isolated dentary of D. hantoniensis (Rio et al., 2020: Figure 10A, B) and the MNHN F BR 1645 and IRSNB R289 dentaries of D. remensis (Martin et al., 2014: Figures 5E, F, 7A), appear also reminiscent of D. kochi. Nevertheless, closer examination of those specimens would confirm probably the procumbent nature of the first tooth insertion, a feature that was probably more widely distributed in diplocynodontids then considered before.
The premaxillary dorsal surface in D. kochi displays a deep notch extending parallel to the lateral and posterolateral narial margin, condition present in D. hantoniensis, D. tormis and Alligator (Rio et al., 2020), as well in D. ratelii (Díaz Aráez et al., 2017: Figure 6) and Orientalosuchus naduongensis (Massonne et al., 2019).
In D. kochi, the anteroposteriorly wide and mediolaterally shallow premaxillary-maxillary notch is bordered by a bony ridge extending on the dorsolateral surface of the posterior premaxillary process. The anterior margin of that notch stands roughly at an angle of 30° with the sagittal plane, whereas in D. remensis it becomes perpendicular to the sagittal plane (Martin et al., 2014: Figures 1A, B). In D. muelleri, D. deponiae and D. hantoniensis the premaxillary-maxillary notch is rather small or absent (Piras and Buscalioni, 2006; Delfino and Smith, 2012; Macaluso et al., 2019; Rio et al., 2020).
The holotype of D. kochi possesses on the lacrimal a prominent and widened bony ridge with a lateral overhang (Venczel and Codrea, 2022), a character shared with D. hantoniensis (Rio et al., 2020), and therefore it should be considered a local autapomorphy of D. kochi and D. hantoniensis. In D. remensis, a preorbital ridge has been reported by Martin et al. (2014), but considered as weakly developed. Outside diplocynodontids, other alligatoroids, like Orientalosuchus, Krabisuchus and Mourasuchus, and some crocodylids (e.g., Osteolaemus and Crocodylus porosus) possess a prominent preorbital ridge on the lacrimal, connected posteriorly to a ridge developed on the prefrontal and anteriorly to a ridge presenton the maxilla (Massone et al., 2019). Therefore, the homology of this character seems equivocal with the above mentioned diplocynodontid taxa (Massonne and Böhme, 2022: Supplementary File 2).
The anterior process of quadratojugal is short (ch. 142-1), extending onto the medial surface of the jugal as a triangular process (Venczel and Codrea, 2022), a character shared with D. elavericus and D. ungeri. In the remaining members of Diplocynodon, where it is known, the anterior process of the quadratojugal is long, extending along the medial margin of the infratemporal fenestra (e.g., D. darwini, D. deponiae, D. hantoniensis, D. ratelii and D. tormis).
The posterior margin of the otic aperture in D. kochi is inset, similarly to D. darwini (Massone et al., 2019), D. hantoniensis (Rio et al., 2020: Figures 3A, B) and D. tormis (Serrano-Martínez et al., 2019: Figure 1A). This condition contrasts with that in the remaining members of the genus, where the posterior margin of the otic aperture is flush with the paroccipital process, as it is exposed in D. deponiae (Delfino and Smith, 2012: Figure 4) and D. remensis (Martin et al., 2014: Figures 3A, B, D).
In the holotype of D. kochi, the last five maxillary alveoli (between the 12th and 16th tooth positions) and those of the last six dentary tooth positions (between the 14th and 19th tooth positions) are slightly compressed mediolaterally (ch. 79-1), a condition present also in D. remensis, D. elavericus (Martin et al., 2014) and Brachychampsa montana (Mook, 1925).
The holotype of D. kochi, represented by an almost completely preserved skull, was recovered from the Cluj-Mănăştur open limestone pit, corresponding to a marine taphonomic setting (Venczel and Codrea, 2022). The specimen, beyond collecting shortages (the sample was broken in three parts and subsequently the counterparts of the exposed portions of skull were abandoned by quarry workers), is well-preserved, devoid of signs of destruction on the external surface, suggesting that prior to its burial it experienced a short transport and subsequently deposited in shallow marine sediments. The referred pair of dentaries, recovered from marine sedimentary rocks in Leghia-Tabără open pit, are also well-preserved and with intact symphysial connection; the right postdentary mandible was found detached in the close vicinity of the fused dentaries. Unfortunately, part of the specimen was lost when the limestone tile was shaped through cutting (Sabău et al., 2021). Few other isolated specimens (femurs, a tooth and a dorsal vertebra), partly sectioned and without their counterparts, are devoid of signs of abrasion or scavenging, even if the presence of sharks was known from the sedimentary rocks of Viştea Limestone Formation (Koch, 1894; Koch, 1900). Especially the isolated vertebra is remarkably well-preserved, still possessing a delicate neural spine and elongated transverse processes.
Sabău et al. (2021) proposed two reliable scenarios for the presence of freshwater diplocynodontids in marine sediments. In the first scenario, it is presumed that the crocodylian carcasses reached the oryctocenosis by a transport from the terrestrial milieu. After a period of floating the carcasses decomposed gradually, their fragments sank separately to the bottom and were covered by bioclasts of various invertebrates and algae (Sabău et al., 2021). In the second scenario, it is assumed that adult individuals of D. kochi arrived actively into the sea, similarly to large adults of recent Alligator mississippiensis, observed occasionally in the sea at a considerable distance from the coastline (more than 60 km; Grigg and Kirshner, 2015). Nevertheless, for the second scenario it remains an open question if diplocynodontids were able or not to tolerate normal salinity conditions comparable to recent crocodylids (e.g., Crocodylus porosus). It is known, that members of the latter group possess salt glands (Taplin, 1988), and are able to tolerate even hypersaline environments (Taplin, 1984). Conversely, it is assumed that alligatoroids might have lost their ability of osmoregulation (i.e., by reduction of their salt glands) during the Mesozoic (Wheatley, 2010; Wheatley et al., 2012). An indirect evidence of possible salt gland reduction in diplocynodontids might be their limited capability of transoceanic colonization (Delfino et al., 2007).
Despite to its putative deficiency in osmoregulation, D. kochi still possessed important adaptations of hunting in water environments (acting probably as an ambush or sit-and-wait predator), including lakes and rivers that were part of the land surfaces that bordered the northwestern part of the Transylvanian Basin during the late Eocene. The fossil records from the localities of Rădaia (fluviatil taphonomic context) and Treznea (marshy-lacustrine taphonomic context) are direct evidence for the presence of this predator in terrestrial paleoenvironments. Furthermore, we may presume that fully grown adults of D. kochi, similarly to large individuals of present-day alligators, occasionally could pass through coastal seawaters in search of prey. The elongated platyrostral skull of D. kochi possessed an expanded buccal cavity, bordered anteriorly by an enlarged and procumbent first dentary tooth, and anterolaterally by a pair of enlarged and slightly laterally inclined third and fourth dentary teeth, fitting into the anteroposteriorly wide and mediolaterally shallow premaxillary-maxillary constriction. Both the posterior series of maxillary and dentary teeth were compressed mediolaterally and provided with well-defined mesiodistal carina, enabling probably the capture of fast-moving prey. The putatively well-developed jaw adductors, suggested by their extended shelf-like insertion surface on the parietal and squamosal, controlled probably the quick closure of the lower jaws (Holliday and Witmer, 2007; Ősi, 2014).
Reconstructed body size estimation of D. kochi, based on Hurlburt et al. (2003) and Godoy et al. (2019), indicates a total length of 1.76 m, while based on the dorsal cranial length (0.23 m) to body length ratio of 1:7 (Whitaker and Whitaker, 2008; Massonne and Böhme, 2022), it could be even smaller (1.61 m). The minuscule size of D. kochi, however, being in range of D. darwini and D. tormis (1.4–1.8 m), might be related to a reduced food availability amid insular conditions, where some members of that crocodylian population were forced occasionally to survey coastal areas in search of food. The alternating food availability in terrestrial habitats might have been related to a seasonal climate, as indicated by the irregular “growth rings” and “lines of arrested growth” (Castanet et al., 1993; Venczel et al., 2015), preserved on the prezygapophyseal surfaces of the only dorsal vertebra (Figure 4D), recovered from Leghia-Tabără open pit. The “growth rings” indicate periods of functional osteogenesis, whereas the “lines of arrested growth” show the temporary halting of that process (Venczel et al., 2015). The prezygapophyseal facet on the right prezygapophysis displays at least ten pairs of “growth rings”/“lines of arrested growth”, that might correspond to ten seasonal growths in that small-sized specimen. On the other hand, that mark may be indicative of the already installed fluctuating climatic conditions, previous to the so called “Terminal Eocene Event” corresponding to global cooling and glacio-eustatic regression (Sotak, 2010). Furthermore, this climatic signal shows a clear disparity with the late Eocene paleoclimatic curve from the Transylvanian Basin (mean annual temperature of around 20°C and mean annual precipitation of about 1,200 mm), resulting from the approximation based on palynomorphs (Petrescu and Balintoni, 2004). Nevertheless, caution is needed when interpreting the “growth rings”/“lines of arrested growth” on the zygapophyses of crocodylomorphs, especially because detailed studies in recent populations, especially those inhabiting the equatorial zones, still are lacking.
The fossil record of the European endemic family Diplocynodontidae started in the late Paleocene (Thanetian) of France (Martin et al., 2014) and came to an end in the middle Miocene of Central Europe (Böhme, 2003; Böhme and Ilg, 2003). Most of the fossil occurrences of diplocynodontids are known from Western and Central Europe (e.g., Díaz Aráez et al., 2017; Chroust et al., 2019; Luján et al., 2019), however, recent reports extend the distribution of this group to more eastern European territories. These reports include Diplocynodon kochi, from the late Eocene (Priabonian) of the Transylvanian Basin, Romania (Venczel and Codrea, 2022), D. levantinicum from the late Oligocene (Chattian) of Bulgaria (Massonne and Böhme, 2022) and cf. Diplocynodon sp. from the middle Eocene (early Lutetian) of Ikovo, Ukraine (Kuzmin and Zvonok, 2021). Likely, the basal alligatoroid ancestors of the European diplocynodontids immigrated from North America and that event might have occurred during the Paleocene (Brochu, 1999; Martin et al., 2014). The dispersal of diplocynodontids from western into more eastern territories was controlled by an extremely high eustatic level during most of the Eocene, when Europe looked like an archipelago, covered by extensive epicontinental seas and with temporarily interconnected landmasses (Rage and Roček, 2003). An intriguing report of Kuzmin and Zvonok (2021), refers to the presence of Diplocynodon-like fossils in some Late Cretaceous (Cenomanian–Santonian) localities from Central Asia (Kazakhstan, Tadzhikistan, and Uzbekistan). The still unpublished material from the Turonian of Uzbekistan, mostly resembling D. remensis, include specimens with equally enlarged fourth and fifth maxillary teeth, enlarged and confluent third and fourth dentary teeth and the splenials being involved in the dentary symphysis (Kuzmin and Zvonok, 2021: Figures 12D, F). If the above assignments are confirmed, we should take into account a second possible scenario for their origin (i.e., the existence of a dispersal route for the ancestors of diplocynodontids from Central Asia into Europe), that might be functional during the Paleocene (Danian), when some terrestrial routes were attainable (Kuzmin and Zvonok, 2021: Figure 10).
Regardless of the above dispersal scenarios proposed, the diplocynodontid populations from the Transylvanian Basin represented probably an important zoogeographical link between the western and eastern European congeners. Despite of deteriorating climatic conditions across the Eocene/Oligocene boundary, the survival of Central European diplocynodontid populations was conceivable, since their fossils have been recovered from the early Oligocene (Rupelian) localities of Suceag and Cetăţuia Hill, Cluj-Napoca, part of Gilău sedimentary area (Fărcaş, 2011; Codrea and Venczel, 2020).
The Eocene fossil record of Diplocynodontidae from Romania was restricted to the late Eocene (Priabonian), being represented by the diminutive Diplocynodon kochi from four fossil localities in the northwestern margin of the Transylvanian Basin. The fossil bearing localities are part of Meseş and Gilău sedimentary areas and represent both marine (carbonate platform) and continental (marshy-lacustrine) taphonomic settings. Diagnostic traits preserved in the holotype (extended insertion surface of jaw adductors on the parietal) and in the newly referred specimens (enlarged and procumbent first dentary tooth, posterior alveoli and teeth mediolaterally compressed) might have been connected to its peculiar lifestyle. The presence of “growth rings” and “lines of arrested growth” exposed on the prezygapophyseal surfaces, may indicate a seasonal paleoclimate installed well before the Eocene–Oligocene terminal event. D. kochi represented certainly an important zoogeographical link between the western and more eastward distributed European congeners, and the genus survived the Eocene–Oligocene transition, as suggested by the early Oligocene (Rupelian) fossil record from the surveyed sedimentary area.
The original contributions presented in the study are included in the article/Supplementary Material. Further inquiries can be directed to the corresponding author.
MV contributed to conception and design of the study, performed the cladistical analysis, wrote sections of the manuscript, revised the manuscript, read, and approved the submitted version.
The research was supported by a grant of the Ministry of Research, Innovation and Digitization, CNCS – UEFISCDI, project number PN-III-P4-PCE-2021-0351, within PNCDI III.
The geological map with crocodylian bearing Paleogene deposits from NW Transylvania was produced by Drd Marian Bordeianu. Prof. Vlad A Codrea organized numerous collecting trips to the fossil localities of Treznea, Rădaia and Leghia-Tabără and provided useful information on those sedimentary environments. The isolated crocodile specimens from Leghia-Tabără open pit were recovered by Mr. Adrian Oltean and donated subsequently to the Museum of Paleontology and Stratigraphy in the Babeş-Bolyai University. Engineer Sergiu Hossu kindly indicated the origin of the limestone with crocodylian content. VAC also helped procuring the relevant literature. A grant of the Ministry of Research, Innovation and Digitization, CNCS – UEFISCDI, project number PN-III-P4-PCE-2021-0351, within PNCDI III was provided to MV. The author thanks Àngel H. Luján, Ivan T. Kuzmin and the review editor Jeremy Martin for their critical comments and suggestions that greatly improved the paper.
The author declares that the research was conducted in the absence of any commercial or financial relationships that could be construed as a potential conflict of interest.
The author MV declared that he was an editorial board member of Frontiers, at the time of submission. This had no impact on the peer review process and the final decision.
All claims expressed in this article are solely those of the authors and do not necessarily represent those of their affiliated organizations, or those of the publisher, the editors and the reviewers. Any product that may be evaluated in this article, or claim that may be made by its manufacturer, is not guaranteed or endorsed by the publisher.
The Supplementary Material for this article can be found online at: https://www.frontiersin.org/articles/10.3389/famrs.2023.1217025/full#supplementary-material
Andrade M. B., Hornung J. J. (2011). A new look into the periorbital morphology of Goniopholis (Mesoeucrocodylia: Neosuchia) and related forms. J. Vertebr. Paleontol. 31, 352–368. doi: 10.1080/02724634.2011.550353
Böck J. (1876). Brachydiastematherium transylvanicum Böckh et Maty, ein Neues Pachydermen Genus aus den eocänen Schichten Siebenburgens. Mitt. Jahrb. König. Ungarn. Geol. 4, 121–150.
Böhme M. (2003). The Miocene climatic optimum: evidence from ectothermic vertebrates of Central Europe. Palaeogeogr. Palaeoclimatol. Palaeoecol. 195 (3–4), 389–401. doi: 10.1016/S0031-0182(03)00367-5
Böhme M., Ilg A. (2003) FosFARbase. Available at: www.wahre-staerke.com/ (Accessed 20.04.2023).
Bremer K. (1994). Branch support and tree stability. Cladistics 10, 295e304. doi: 10.1111/j.1096-0031.1994.tb00179.x
Brochu C. A. (1999). Phylogenetics, taxonomy, and historical biogeography of Alligatoroidea. J. Vertebr. Paleontol. 19 (S2), 9–100. doi: 10.1080/02724634.1999.10011201
Buscalioni A. D., Sanz J. L., Casanovas M. L. (1992). A new species of the eusuchian crocodile Diplocynodon from the Eocene of Spain. Neues Jahrb. Geol. Palaontol. Abh. 187 (1), 1–29.
Castanet J. D. G., Francillon-Vieillot H., Meunier F. J., Ricqles A. D. (1993). “Bone and individual aging,” in Bone. Ed. Hall B. K. (Boca Raton, Fl: CRC Press), 245–283.
Chroust M., Mazuch M., Ivanov M., Ekrt B., Luján À.H. (2021). First remains of Diplocynodon cf. ratelii from the early Miocene sites of Ahníkov (Most Basin, Czech Republic). Bull. Geosci. 96 (2), 123–138. doi: 10.3140/bull.geosci.1803
Chroust M., Mazuch M., Luján À.H. (2019). New crocodylian material from the Eocene–Oligocene transition of the NW Bohemia (Czech Republic): an updated fossil record in Central Europe during the Grande Coupure. Neues Jahrb. Geol. Palaontol. Abh 293 (1), 73–82. doi: 10.1127/njgpa/2019/0832
Codrea V., Fărcaş C. (2002). Principalele asociaţii de tetrapode continentale paleogene din Transilvania: distribuţie stratigrafică şi semnificaţii paleoambientale. Armonii Naturale 4, 80–90.
Codrea V., Hosu A. (2001). “The Paleocene-Eocene formations and the Eocene/Oligocene boundary in the Jibou area (Sălaj Country),” in Field trip guide” in 4th regional meeting of IFAA. Eds. Bucur I. I., Filipescu S., Săsăran E. (Cluj-Napoca: Babeş-Bolyai University), 93–107.
Codrea A. V., Venczel M. (2020). The fossil record of Palaeogene crocodylians in Romania: preliminary data. Nymphaea 46-47, 67–82.
Codrea V., Vremir M., Dica P. (1997). Calcarul de Cluj de la Someş-Dig (Cluj-Napoca): semnificaţii paleoambientale şi impactul activităţilor antropice asupra aflorimentului. Compl. Muz. jud. Bistriţa-Năsăud Stud. Cerc. 3, 31–39.
Delfino M., Böhme M., Rook L. (2007). First European evidence for transcontinental dispersal of Crocodylus (late Neogene of southern Italy). Zool. J. Linn. Soc. 149 (3), 239–307. doi: 10.1111/j.1096-3642.2007.00248.x
Delfino M., Smith T. (2012). Reappraisal of the morphology and phylogenetic relationships of the middle Eocene alligatoroid Diplocynodon deponiae (Frey, Laemmert, and Riess 1987) based on a three-dimensional specimen. J. Vertebr. Paleontol. 32 (6), 1358–1369. doi: 10.1080/02724634.2012.699484
Díaz Aráez J. L., Delfino M., Luján A. H., Fortuny J., Bernardini F., Alba D. M. (2017). New remains of Diplocynodon (Crocodylia: Diplocynodontidae) from the early miocene of the Iberian Peninsula. C. R. Palevol. 16 (1), 12–26. doi: 10.1016/j.crpv.2015.11.003
Fărcaş C. (2011). Studiul formaţiunilor continentale eocen terminale şi oligocen timpurii din NV-ul Depresiunii Transilvaniei - biostratigrafie şi reconstituiri ambientale, pe baza asociaţiilor de vertebrate continentale (Cluj-Napoca: Babeş-Bolyai Univesity).
Gheerbrant E., Codrea V., Hosu A., Sen S., Guernet C., de Lapparent de Broin F., et al. (1999). Découverte de vertébrés dans les Calcaires de Rona (Thanétien ou Sparnacien), Transylvanie, Roumanie: les plus anciens mammiferes cénozoiques d’Europe Orientale. Eclogae Geol. Helv. 92, 517–535.
Godoy P. L., Benson R. B. J., Bronzati M., Butler R. J. (2019). The multi-peak adaptive landscape of crocodylomorph body size evolution. BMC Evol. Biol. 167. doi: 10.1186/s12862-019-1466-4
Goloboff P. A., Morales M. E. (2023). TNT version 1.6, with a graphical interface for MacOS and Linux, including new routines in parallel. Cladistics 39 (2), 144–153. doi: 10.1111/cla.12524
Grigg G., Kirshner D. (2015). Biology and evolution of crocodylians (Clayton South: CSIRO Publishing).
Hofmann K. (1879). Jelentés az 1878 nyarán Szilágymegye keleti részében tett földtani részletes felvételekről. M. Kir. Földt. Int. Évkönyve 9(5, 6), 167–212.
Holliday C., Witmer L. M. (2007). Archosaur adductor chamber evolution: integration of musculoskeletal and topological criteria in jaw muscle homology. J. Morphol. 268 (6), 457–484. doi: 10.1002/jmor.10524
Hosu A. (1999). Arhitectura sedimentaţiei depozitelor eocene din nord-vestul Depresiunii Transilvaniei (Cluj-Napoca: Presa Univ Clujeană).
Hurlburt G. R., Heckert A. B., Farlow J. O. (2003). “Body mass estimates of phytosaurs (Archosauria: Parasuchidae) from the Petrified Forest Formation (Chinle group: Revueltian) based on skull and limb bone measurements,” in Paleontology and geology of the snyder quarry, vol. 24 . Eds. Zeigler K. E., Heckert A. B., Lucas S. G., 105–114. New Mexico Mus. Nat. Hist. Sci. Bull. (Albuquerque: New Mexico Museum of Natural History).
Koch A. (1894). Az Erdélyrészi medencze harmadkori képződményei. I. Paleogén csoport. M. Kir. Földt. Int. Évkönyve 10 (6), 161–356.
Koch A. (1900). A Magyar Korona országai kövült gerinczesállat maradványainak rendszeres átnézete. Magy. Orv. Természetvizsg. Vándorgy. 30, 526–560.
Krézsek C., Bally A. W. (2006). The Transylvanian Basin (Romania) and its relation to the Carpathian fold and thrust belt: insights in gravitational salt tectonics. Mar. Pet. Geol. 23 (4), 405–442. doi: 10.1016/j.marpetgeo.2006.03.003
Kuzmin I. T., Zvonok E. A. (2021). Crocodylian assemblage from the middle Eocene Ikovo locality (Lugansk Province, Ukraine), with a discussion of the fossil record and geographic origins of crocodyliform fauna in the Paleogene of Europe. Geobios 65, 7–27. doi: 10.1016/j.geobios.2021.02.002
Luján À.H., Chroust M., Čerňanský A., Fortuny J., Mazuch M., Ivanov M. (2019). First record of Diplocynodon ratelii Pomel 1847 from the early Miocene site of Tušimice (Most Basin, Northwest Bohemia, Czech Republic). C. R. Palevol 18 (7), 877–889. doi: 10.1016/j.crpv.2019.04.002
Macaluso L., Martin J. E., Del Favero L., Delfino M. (2019). Revision of the crocodylians from the Oligocene of Monteviale, Italy, and the diversity of European eusuchians across the Eocene-Oligocene boundary. J. Vertebr. Paleontol. 39 (2), e160109. doi: 10.1080/02724634.2019.1601098
Martin J. E. (2010). A new species of Diplocynodon (Crocodylia, Alligatoroidea) from the Late Eocene of the Massif Central, France, and the evolution of the genus in the climatic context of the Late Palaeogene. Geol. Mag. 147 (4), 596–610. doi: 10.1017/S0016756809990161
Martin J. E., Gross M. (2011). Taxonomic clarification of diplocynodon pomel 1847 (Crocodilia) from the miocene of Styria, Austria. Neues Jahrb. Geol. Palaontol. Abh. 261 (2), 177–193. doi: 10.1127/0077-7749/2011/0159
Martin J. E., Smith T., de Lapparent de Broin F., Escuillié F., Delfino M. (2014). Late Palaeocene eusuchian remains from Mont de Berru, France, and the origin of the alligatoroid Diplocynodon. Zool. J. Linn. Soc 172 (4), 867–891. doi: 10.1111/zoj.12195
Massone T., Vasilyan D., Rabi M., Böhme M. (2019). A new alligatoroid from the Eocene of Vietnam highlights an extinct Asian clade independent from extant Alligator sinensis. PeerJ 7, e7562. doi: 10.7717/peerj.7562
Massonne T., Böhme M. (2022). Re-evaluation of the morphology and phylogeny of Diplocynodon levantinicum Huene & Nikoloff 1963 and the stratigraphic age of the West Maritsa coal field (Upper Thrace Basin, Bulgaria). PeerJ 10, e14167. doi: 10.7717/peerj.14167
Mészáro N., Moga V., Ianoliu C. (1987). “Studying the various groups of fossil organisms of Leghia – Leghia-Băi”, in The Eocene from the Transylvanian Basin. Eds Petrescu I., Ghergari L., Mészáros N., Nicorici E. (Cluj-Napoca: Babeş-Bolyai University), 143–150.
Mészáros N. (2000). Correlation of the paleogene and neogene deposits from Northern Transylvania. Studia Univ. Babeş-Bolyai Geol. 45 (2), 9–12. doi: 10.5038/1937-8602.45.2.1
Mészáros N., Moisescu V., Rusu A. (1989). “The Merian, a new substage of the Mesogean Oligocene,” in The oligocene of the Transylvanian Basin. Ed. Petrescu I. (Cluj-Napoca: Babeş-Bolyai University), 31–54.
Mook C. C. (1925). A revision of the Mesozoic Crocodilia of North America (preliminary report). Bull. Am. Mus. Nat. Hist. 51, 319–432.
Ősi A. (2014). The evolution of jaw mechanism and dental function in heterodont crocodyliforms. Hist. Biol. 26 (3), 279–414. doi: 10.1080/08912963.2013.777533
Petrescu I., Balintoni I. (2004). Paleoclimate and paleorelief in Romania during the Tertiary period. An. Şt. Univ.”Al. I. Cuza” Iaşi 49-50, 183–189.
Piras P., Buscalioni A. D. (2006). Diplocynodon muelleri comb. nov., an Oligocene diplocynodontine alligatoroid from Catalonia (Ebro Basin, Lleida province, Spain). J. Vertebr. Paleontol. 26 (3), 608–620. doi: 10.1671/0272-4634(2006)26[608:DMCNAO]2.0.CO;2
Popescu B. (1976). Lithostratigraphy of the cyclic continental to marine Eocene deposits in NW Transylvania. Romania. Arch. Sci. 37 (1), 37–73.
Popescu B., Bombiţă G., Rusu A., Iva M., Gheţa N., Olteanu R., et al. (1978). The eocene of the Cluj-Huedin area. Dări Seamă ale Şedinţelor 64 (1976-1977), 295–357.
Proust J. N., Hosu A. (1996). Sequence stratigraphy and Paleogene tectonic evolution of the Transilvanian Basin (Romania, Eastern Europe). Sediment. Geol. 105, 117–140. doi: 10.1016/0037-0738(95)00144-1
Rage J.-C., Roček Z. (2003). Evolution of anuran assemblages in the Tertiary and Quaternary of Europe, in the context of palaeoclimate and palaeogeography. Amphibia-Reptilia 24, 133–167. doi: 10.1163/156853803322390408
Răileanu G., Saulea E. (1956). Paleogenul din regiunea Cluj şi Jibou (NV bazinului Transilvaniei). An. Comit. Geol. 29, 272–308.
Răileanu G., Saulea E. (1967). Harta geologică L-34-XVIII scara 1:200 000. Foaia 18 Turda (Bucureşti: Institutul Geologic al României).
Rio J. P., Mannion P. D., Tschopp E., Martin J. E., Delfino M. (2020). Reappraisal of the morphology and phylogenetic relationships of the alligatoroid crocodylian Diplocynodon hantoniensis from the late Eocene of the United Kingdom. Zool. J. Linn. Soc 188 (2), 579–629. doi: 10.1093/zoolinnean/zlz034
Rusu A. (1995). Eocene formations in the Călata region (NW Transylvania): a critical review. Roum. J. Tecton. Reg. Geol. 76, 59–72.
Sabău I., Venczel M., Codrea A. V., Bordeianu M. (2021). Diplocynodon: A salt water Eocene Crocodile from Transylvania? North West. J. Zool. 17 (1), 117–121.
Serrano-Martínez A., Knoll F., Narváez I., Ortega F. (2019). Brain and pneumatic cavities of the braincase of the basal alligatoroid Diplocynodon tormis (Eocene, Spain). J. Vertebr. Paleontol. 39 (1), e1572612. doi: 10.1080/02724634.2019.1572612
Sotak J. (2010). Paleoenvironmental changes across the Eocene-Oligocene boundary: insights from the Central-Carpathian Paleogene Basin. Geol. Carpath. 61 (5), 393–418. doi: 10.2478/v10096-010-0024-1
Taplin L. E. (1984). Homeostasis of plasma electrolytes, sodium and water pools in the estuarine crocodile, Crocodylus porosus, from fresh, saline and hypersaline waters. Oecologia 63 (1), 63–70. doi: 10.1007/BF00379786
Taplin L. E. (1988). Osmoregulation in crocodylians. Biol. Rev. Camb. Philos. Soc 63 (3), 333–377. doi: 10.1111/j.1469-185X.1988.tb00721.x
Venczel M., Codrea V. A., Trif N. (2023). Eocene gavialoid teeth from southern Transylvania with notes on the diversity of Paleogene crocodilians from Romania. North West. J. Zool. 19 (1), 71–79.
Venczel M., Codrea V. A. (2022). A new late Eocene alligatoroid crocodyliform from Transylvania. C. R. Palevol 21 (20), 411–429. doi: 10.5852/cr-palevol2022v21a20
Venczel M., Vasile S., Csiki-Sava Z. (2015). A Late Cretaceous madtsoiid snake from Romania associated with a megaloolithid egg nest - Paleoecological inferences. Cretac. Res. 55, 152–163. doi: 10.1016/j.cretres.2015.02.009
Wheatley P. V. (2010). Understanding salt water tolerance and marine resource use in the Crocodylia: a stable isotope approach (Santa Cruz: University of California).
Wheatley P. V., Peckham H., Newsome S. D., Koch P. L. (2012). Marine resource use in the American crocodile (Crocodylus acutus) in Southern Florida. Mar. Ecol. Prog. Ser. 447, 211–229. doi: 10.1016/j.cretres.2015.02.009
Keywords: alligatoroid, fossil record, lifestyle, paleobiogeography, paleogene, survival
Citation: Venczel M (2023) Updating the fossil record of the alligatoroid crocodylian Diplocynodon from the late Eocene of Transylvanian Basin. Front. Amphib. Reptile Sci. 1:1217025. doi: 10.3389/famrs.2023.1217025
Received: 04 May 2023; Accepted: 01 August 2023;
Published: 29 August 2023.
Edited by:
Jeremy Martin, CNRS, FranceReviewed by:
Àngel Hernández Luján, Universitat Autònoma de Barcelona, SpainCopyright © 2023 Venczel. This is an open-access article distributed under the terms of the Creative Commons Attribution License (CC BY). The use, distribution or reproduction in other forums is permitted, provided the original author(s) and the copyright owner(s) are credited and that the original publication in this journal is cited, in accordance with accepted academic practice. No use, distribution or reproduction is permitted which does not comply with these terms.
*Correspondence: Márton Venczel, bXZlbmN6ZWxAZ21haWwuY29t
Disclaimer: All claims expressed in this article are solely those of the authors and do not necessarily represent those of their affiliated organizations, or those of the publisher, the editors and the reviewers. Any product that may be evaluated in this article or claim that may be made by its manufacturer is not guaranteed or endorsed by the publisher.
Research integrity at Frontiers
Learn more about the work of our research integrity team to safeguard the quality of each article we publish.