- 1Department of Agronomy, Tamil Nadu Agricultural University, Coimbatore, India
- 2International Rice Research Institute South Asia Regional Centre, Varanasi, India
- 3International Rice Research Institute, Los Baños, Philippines
- 4Department of Soil and Crop Sciences, Texas A&M University, College Station, TX, United States
- 5Department of Biological and Agricultural Engineering, Texas A&M University, College Station, TX, United States
- 6Department of Crop Physiology, Tamil Nadu Agricultural University, Coimbatore, India
- 7Centre for Water and Geospatial Studies, Tamil Nadu Agricultural University, Coimbatore, India
- 8Department of Farm Machinery and Power Engineering, Tamil Nadu Agricultural University, Coimbatore, India
Unmanned aerial vehicles (UAVs) represent a cutting-edge technology that holds the promise of revolutionizing the conventional tasks carried out in the realm of agriculture. On a global scale, UAVs are gaining prominence for pesticide applications, particularly with a focus on utilizing low spray volumes. Nevertheless, there remains a notable gap in research concerning the impacts of employing low spray volumes on herbicide efficacy. Hence, multi-location field studies were conducted at Agricultural Research Station, Bhavanisagar and Wetland Station, Coimbatore, Tamil Nadu, India in 2022 to evaluate the impact of different spray volumes using UAV and Knapsack Manual Sprayer (KMS) on droplet deposition, droplet density, and weed control efficacy. The treatments included UAV sprays at 30 and 45 L ha-1, as well as KMS at 500 L ha-1, with a weedy check as a control. Bispyribac-sodium 10% SC was applied at a rate of 35 g a.i ha-1 during the 2-to 3-leaf stage of the crops. The results revealed that droplet deposition, area coverage, and volume median diameter were affected by sprayer type (UAV and KMS). However, the two spray volumes tested using the UAV method provided similar droplet parameters. The KMS system at 500 L ha-1 exhibited the highest droplet deposition, while the UAV system achieved better droplet distribution at a spray volume of 30 L ha-1. Remarkably, the UAV system at 30 L ha-1 demonstrated effective weed control, which was statistically comparable to the KMS system at 500 L ha-1. These findings emphasize that the UAV spraying system with a spray volume of 30 L ha-1, delivering effective weed control while utilizing less carrier volume compared to the manual knapsack spraying method. Thus, the UAV spray system has great potential as a viable alternative to manual knapsack spraying for herbicide application in direct-seeded rice.
1 Introduction
Rice is one of the principal food sources for over 50% of the global human population and provides more than 20% of the calories in the diet (Fukagawa and Ziska, 2019). In India, rice is one of the most important staple food crops, producing 129.5 million metric tons from a cultivated area of 46.3 million ha with an average yield of 2.79 t ha-1 (Indiastat, 2023). The projected demand for rice is expected to rise by 26 million tons between 2020 and 2030 Anno Domini (AD) in India (Mondal et al., 2022).
Conventional transplanted rice production faces a range of challenges, including skilled labor shortages, reduced access to irrigation availability, and increased severe weather (Bhushan et al., 2007; Kumar et al., 2021). Transplanted rice production is associated with higher production costs, increased energy demand, greater nutrient loss, greenhouse gas emissions, soil structure degradation, and extended maturity period (Farooq et al., 2011a; Chauhan et al., 2012; Chaudhary et al., 2022). These factors collectively delayed the sowing of subsequent non-rice upland crops within the cropping system (Kumar and Ladha, 2011). Consequently, there is a growing trend toward the adoption of direct-seeded rice (DSR) as a viable alternative approach to address these challenges. DSR saves 33% of irrigation water and reduces labor input by 97% for planting compared to transplanted rice (Haindavi et al., 2018).
Despite several benefits, DSR is highly susceptible to weed infestation compared to transplanted rice due to the absence of water ponding in the field (Farooq et al., 2011b). Additionally, the weed flora in DSR is more diverse compared to transplanted rice (Tomita et al., 2003). Among the various factors influencing the productivity of DSR, weeds play a pivotal role. Studies have reported yield losses of up to 90% in the absence of effective weed control measures in DSR (Chauhan and Johnson, 2011). Therefore, timely and efficient weed management is essential to enhance the productivity of DSR. The critical period for crop-weed competition in DSR occurs between 15 to 45 days after sowing (DAS) (Singh et al., 1999). However, during the peak season, the unavailability of skilled labor for hand weeding results in delayed field operations. These delays have severe consequences, including a significant loss in rice yield. Furthermore, to ensure the timely completion of field operations, farmers are compelled to pay higher wages, which adds an economic burden on them. Hence, the use of herbicides emerges as the most effective and economically viable option to control weeds in DSR when compared to the other available alternatives (Dass et al., 2017).
Conventionally, herbicides are applied using manually operated or battery-powered knapsack sprayers (Li et al., 2017). These sprayers often lead to non-uniform application, increased application costs, human drudgery, difficulties in navigating through muddy soil while carrying the load, and uneven crop stands due to the deep pressing of seeds into the wet soil within spraying person footprint areas (Vijayakumar et al., 2022). Besides that, these methods pose a higher risk of exposure to toxic chemicals for the personnel spraying the herbicides, when compared to herbicide applications using unmanned aerial vehicles (UAVs) (Cao et al., 2017; Meivel et al., 2016).
An Unmanned Aerial Vehicle (UAV) is a multispecialty device which is employed for many agricultural applications such as soil analysis, seeding, bird control, pesticide spraying, monitoring of groundwater quality, and farming systems (Ahirwar et al., 2019; Rejeb et al., 2022). Globally, interest in UAV technology has increased for agricultural operations (Telli et al., 2023). UAV crop protection applications can be helpful in cropping systems where ground applications are difficult, such as wet fields and plantation crops (Arthanari and Paul, 2022). Therefore, the utilization of UAVs for pesticide spraying is increasingly gaining popularity in the field of agriculture (Wen et al., 2018; Meng et al., 2020; Vijayakumar et al., 2020).
The efficacy of pesticides applied through UAV is significantly influenced by factors such as, spray volume, droplet size, and deposition rate, which are, in turn, affected by weather parameters, including wind velocity and direction (Fritz, 2006; Legleiter and Johnson, 2016). Increasing contact with the target weeds is necessary to achieve improved control efficiency for post-emergence herbicides. Ferguson et al. (2018) reported that the reduction in spray droplets size affected the efficacy of contact herbicides (amitrole and paraquat) but not of systemic herbicides (imazapyr, glyphosate, and clodinafop). Chen et al. (2019) used two carrier volumes (15 and 22.5 L ha-1) for the UAV application of systemic herbicide and found that reduced carrier volume did not affect the weed control efficacy. According to Jeevan et al. (2023a), droplet deposition and coverage were increased with spray volumes from 25 to 50 L ha-1; however, the greater weed control efficacy for bispyribac-sodium at 25 g a.i ha-1 was achieved at a volume greater than 37.5 L ha-1.
Qin et al. (2016) studied the effect of flying height (0.8 and 1.5 m) and speed (3 and 5 m s-1) on deposition uniformity and found that the application of pesticides with a flying height of 1.5 m and speed of 5 m s-1 showed a greater uniformity of droplets than conventional sprayer in rice. The droplet density and coverage were increased with increasing flow rate at a constant flying height (2 m); however, the droplet density was reduced with increased flying height and speed under the constant flow rate (1.08 L min-1) (Wang et al., 2017).
UAVs can fly and hover close to plant canopy to apply the herbicides safely. Ahmad et al. (2020) reported that the UAV operational parameters of a flying height 2 m and a flight speed of 2 m s-1 provided the highest average herbicide deposition on weed canopy. Paul et al. (2023a) conducted an experiment to evaluate the efficacy of herbicides on rice and found that no significant difference in herbicide efficacy between treatments using UAV and backpack sprayer. Similarly, Pranaswi et al. (2024) found that the effectiveness of systemic herbicides on wheat remained unaffected by UAV and backpack application. Jeevan et al. (2023b) also reported that applying herbicides with UAVs led to a significant reduction in weed density and weed dry weight on transplanted rice, which was comparable to conventional application methods. The application of post-emergence systemic herbicides like rinskor, cyhalofop and bispyribac sodium through UAV yielded similar herbicide efficacy and weed control as conventional applicator in rice (Abd Ghani et al., 2024). Several researchers reported that UAVs can implement site-specific herbicide applications, which can reduce the quantity of herbicide used. For instance, the adoption of site-specific herbicide application through UAV under moderate weed patches in a clumped distribution reduced herbicide use by 90% in maize and 43% in sugar beet (Mink et al., 2018). Hence, the adoption of UAVs can be an alternative to conventional systems.
The management of emerged weeds in DSR is convenient with UAV-based herbicide applications (Paul et al., 2023b). However, it is unclear whether UAV-based herbicide application influences weed control ratings while saving carrier volume in the DSR system. Addressing these knowledge gaps is essential for the development of standardized practices for herbicide spraying in DSR. The specific objectives of this study were to (i) compare the efficacy of UAV (hexacopter)-based herbicide application with the KMS system and (ii) effective spray volume for herbicide application through UAVs, aiming to achieve maximum weed control efficacy.
2 Materials and methods
2.1 Experimental site
The field experiments were conducted at South Block, Agricultural Research Station, Bhavanisagar (11°29’ N, 77°08’ E) (study 1) and Wetland Station, Coimbatore (11°54’ N, 76°56’ E) (study 2), Tamil Nadu Agricultural University, Coimbatore, India. The description of soil and weather parameters for the experimental sites are provided in Tables 1, 2.
2.2 Crop husbandry
The experimental field was plowed using a tractor-drawn disc harrow, followed by puddling using a tractor-drawn cage wheel. The puddled field was then leveled using a wooden plank. The rice variety used for the experiment was ADT 43. The rice seeds were soaked in water for a duration of 10 h, followed by incubation in darkness for 24 h to promote seed sprouting. The sprouted seeds were sown on the puddled soil using a drum seeder with a row spacing of 0.2 m. The seeds were sown on May 19, 2022 (Study 1) and May 23, 2022 (Study 2), and a seed rate of 60 kg ha-1 was used. Water was drained from the field one day after sowing, up to a week to encourage early and uniform germination. After the establishment of seedlings, the water depth was maintained at 5 cm. The recommended dose of fertilizer, comprising 150:50:50 kg ha-1 of nitrogen, phosphorus, and potassium, was applied in the form of urea, single superphosphate, and muriate of potash. Prior to sowing, 100% of the phosphorus and 25% each of the nitrogen and potassium were applied as a basal application, while the remaining 75% of the nitrogen and potassium were applied in three equal splits at 21 DAS, panicle initiation, and flowering.
2.3 Equipment
A hexacopter UAV (Kisan drone V.2, Garuda Aerospace Pvt Ltd, Chennai, India) equipped with various spraying components (Figure 1A) was utilized for the study. The UAV sprayer was equipped with 180 kV Brushless Direct Current (BLDC) motors, 0.57 m foldable propeller, two Lipo batteries, a flight controller, receiver, GPS unit, and a pesticide tank with a capacity of 10 L and four nozzles with a spacing of 0.70 m. The BLDC motor pump was used to pressurize the spray liquid with the pressure of 3.4 kg cm-2. The flight planner was employed to control the flying height and speed, while the flow rate of the UAV sprayer was adjusted to achieve the desired application flow rate, ranging from 100% to 75%. Prior to the flight, pre-flight calibration of the UAV spraying system was conducted. In contrast, the battery-operated knapsack manual sprayer utilized in the study consisted of a 16 L tank to hold the spray solution, a pump, a filter, a flow control valve, a delivery hose, and a spray gun with nozzle with the pressure of 2.1 kg cm-2. Detailed information on different components of both the knapsack manual spraying (KMS) (KK-BBS-199, KisanKraft Limited, India as shown in Figure 1B) and UAV spraying systems are provided in Table 3.
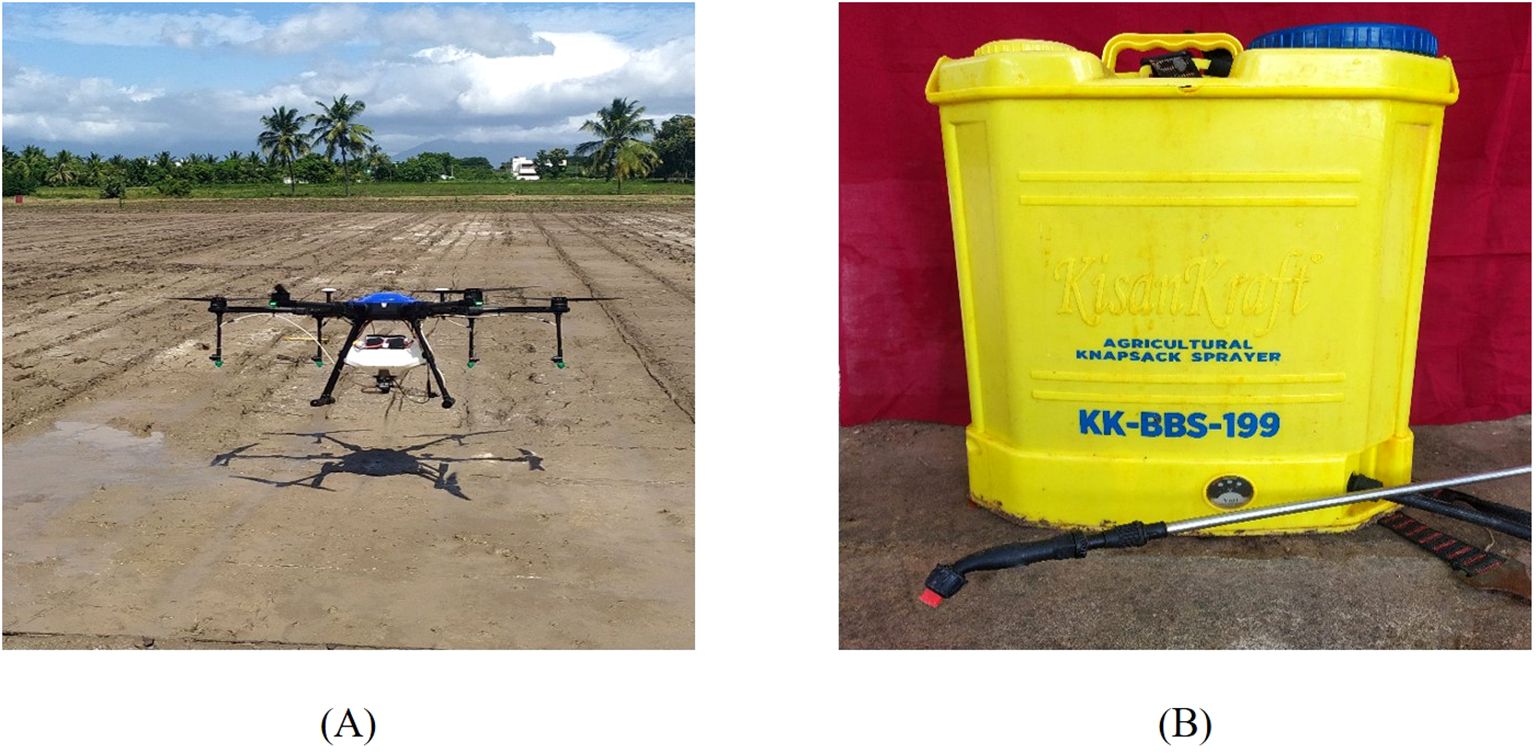
Figure 1. Different sprayers used in the study (A) Hexacopter UAV sprayer, (B) Knapsack manual sprayer.
2.4 Application rate
The application rate was determined following the standard set by the American Society of Agricultural and Biological Engineers standard (ASABE) (S386.2, 2018). The travel speed, effective spray width, and discharge rate values were measured, and the application rate was calculated using the following equation:
Where,
R= Application rate, L ha-1
Q= Output rate, L min-1
K= Constant, 600
S= Travel speed, km h-1
W= Effective spray width, m
2.5 Herbicide application
A systemic early post-emergence herbicide bispyribac-sodium (Nominee Gold, 10% SC, PI Industries Limited, Udaipur, India) was applied at a rate of 35 g a.i ha-1. The herbicide was applied as an early post-emergence treatment at 2-to 3-leaf stage of the rice plant using both KMS and UAV (Figure 2A). The treatments comprised spraying with the UAV at the rate of 30 and 45 L ha-1, along with KMS at a rate of 500 L ha-1, with a weedy check serving as a control. The randomized complete block design was used to arrange the experimental units with a plot size of 40 × 16 m and three replications for each treatment. The operational parameters for the UAV and KMS are presented in Table 4. During the herbicide application, various meteorological parameters such as temperature, relative humidity, and rainfall were obtained from the meteorological observatory (Table 5). A portable hand-held anemometer (LUTRON AM 4202, Vane type, range: 0.4 to 30.0 m s-1) was used to measure the continuous wind velocity during the herbicide application (Yang et al., 2018).
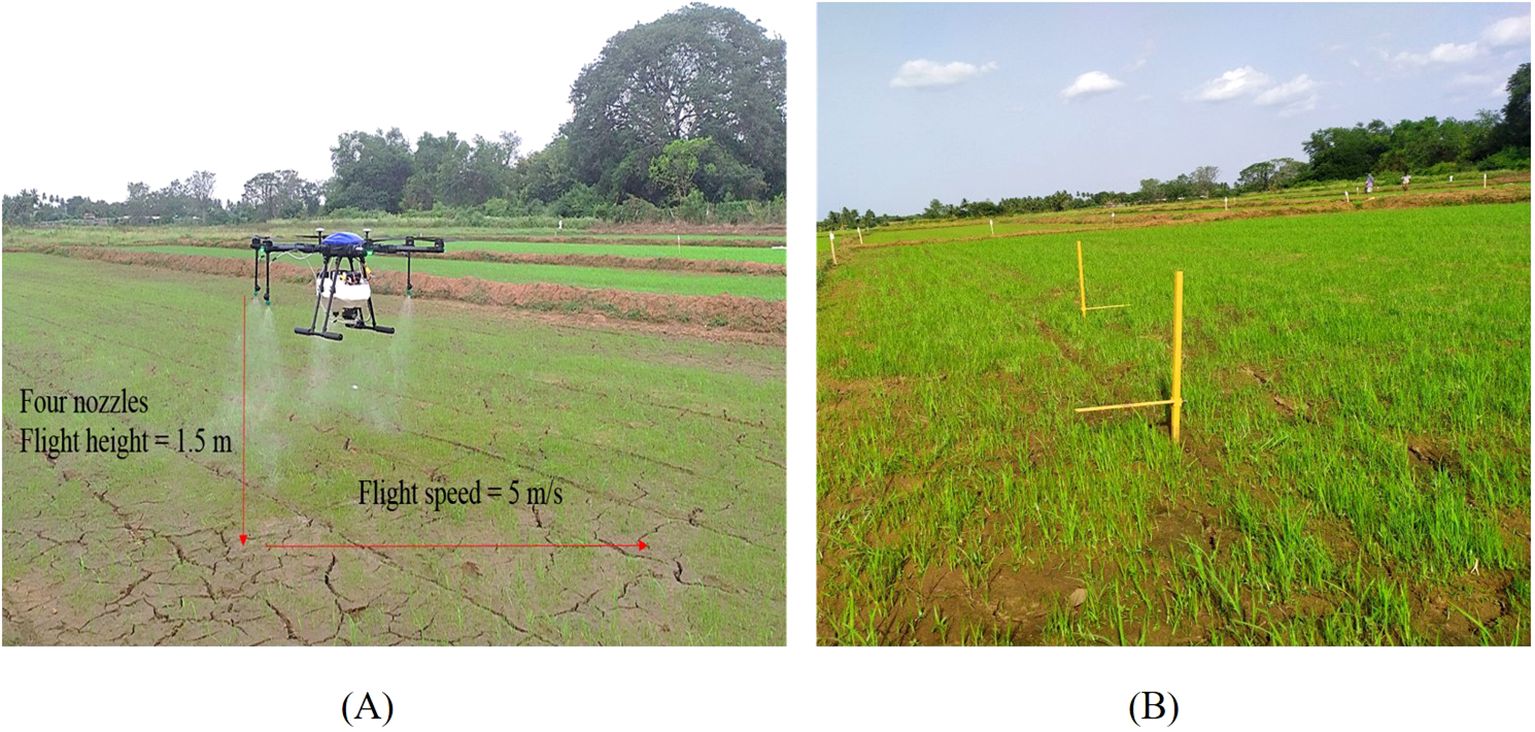
Figure 2. Spraying. (A) UAV herbicide spray operation, (B) placement of water sensitive paper (WSP) on iron poles in the experimental field.
2.6 Sampling of spray deposition
Water-sensitive papers (WSP) with the dimension of 2.6 cm x 7.6 cm (Spray Check, SC-20301, USA) were clipped to an iron platform (0.04 m × 0.30 m) to ensure that they remained flat during the experiment (Figure 2B) and placed above to the crop canopy within 2 cm. A total of eight samplers were placed randomly within the experimental plots. The first sampler was deployed at a distance of 4 m from the border row. Subsequent samplers were placed randomly in different lines within the row (Figure 3) and samplers were collected and replaced between the flights.
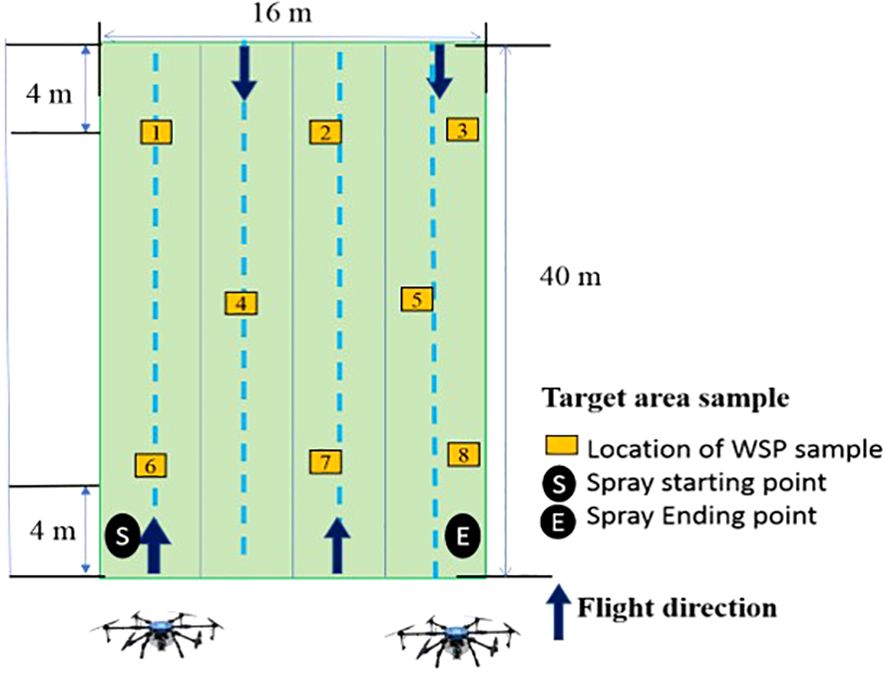
Figure 3. Schematic representation for the location of water sensitive paper (WSP) cards during the spray.
Following the herbicide application, the WSPs were left to dry completely. Once dried, they were carefully placed in zip-tie bags and transported to the laboratory. Afterwards, DropletScan software (USDA, Wooster, OH, USA) was used to measure droplet diameter, droplet density, and coverage area on the WSPs (Whitney and Gardisser, 2003).
2.7 Droplet distribution
In order to characterize the droplet distribution between the various collection points, the coefficient of variation (CV) for droplet deposition at each collection point was computed. Smaller CV values result in more uniform droplet deposition and better efficacy (Zhang et al., 2020).
2.8 Biometric observations on weed
Weed density (number of weeds m-2) and dry weight (g m-2) of the weeds were recorded in each experimental plot at 40 DAS. Weed density was observed in four quadrants (0.5 × 0.5 m) placed in each plot. To determine weed dry weight, the weeds were removed at ground level and allowed to dry in the shade for six days. Subsequently, they were oven-dried at a temperature of 78 ± 2°C until a constant weight was attained (Jabran et al., 2012). Weed control efficiency was calculated as per the procedures given by Mani et al. (1973) and expressed in percentage.
2.9 Statistical analysis
Due to the high variability observed in the data recorded from the WSP for spray deposition, droplet density, and area coverage, transformations were applied to stabilize the wide variation and meet the assumption of normality. A variable refers to traits, either quantitative or qualitative, that vary across observations within the same treatment. The data expressed as a percentage of area coverage was subjected to arcsine transformation . The other parameters obtained from the WSPs data were transformed using the log transformation log(x+1). Additionally, the weed density and weed dry weight data were transformed using square root transformation to stabilize the wide variation and meet the normality assumptions. The transformed data were used to perform the Shapiro-wilk normality test and Bartlett test for analyzing homogeneity of variance. Then, the data were analyzed by using one-way ANOVA and multiple comparisons were performed using Tukey’s Honest significant difference test at 5% probability using IBM SPSS statistics software version 26.0 (Steel et al., 1997).
3 Results
3.1 Spray droplet spectra on WSPs
3.1.1 Volume median diameter
The volume median diameter (VMD) increased with the spray volume in UAV treatments (Table 6). The VMD of the droplets was significantly higher (613.6 ± 27.39 μm and 601.9 ± 29.35 μm for study 1 and study 2, respectively) for the KMS compared to the UAV treatments (p=0.000), which were operated at low pressure (2.1 kg cm-2) (Table 6). Among the UAV treatments, VMD increased with the tested spray volumes of 30 and 45 L ha-1. However, there was no significant difference in VMD across the UAV treatments (p>0.05), indicating that carrier volume had minimal impact on VMD when using the same spraying system.
3.1.2 Area coverage
The percentage of spray area coverage is an important parameter for evaluating droplet deposition and sprayer effectiveness. The different spray volumes had an impact on the percentage of area coverage on the WSPs (Table 6). In both studies, the highest area coverage on WSPs was recorded with the KMS treatment at 500 L ha-1, which was significantly higher from UAV treatments (p=0.000) and the highest values of area coverage on WSPs was recorded in study 1 (35.68%) as compared to study 2 (34.23%). There was no significant difference in the spray area coverage between the UAV treatments with the tested spray volume of 30 and 45 L ha-1 (p>0.05).
3.1.3 Droplet density
The highest spray droplet density per unit area was recorded with the KMS treatment at 500 L ha-1. There was no significant difference in droplet density among the UAV treatments. On the WSP samplers, the average spray droplet density (Figure 4) were 35.1 ± 2.58, 38.6 ± 4.50 and 45.4 ± 8.70 droplets cm-2 in study 1, and 30.9 ± 4.12, 34.1 ± 5.63 and 44.3 ± 7.83 droplets cm-2 in study 2 for UAV at 30, 45 L ha-1 and the KMS treatments, respectively (Figure 5).
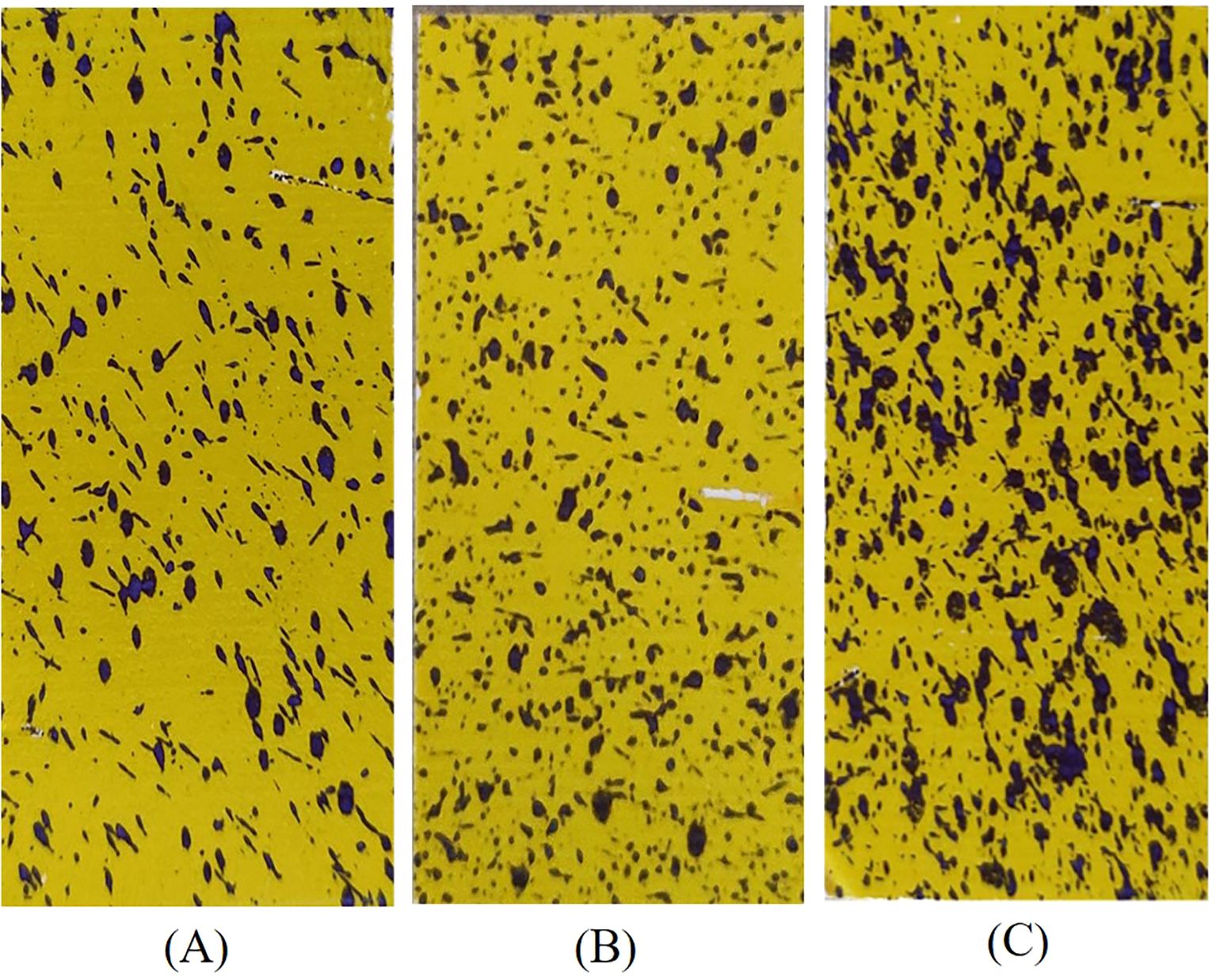
Figure 4. Illustration of spray droplets on water sensitive paper. (A) UAV-30 L ha-1, (B) UAV-45 L ha-1, (C) KMS-500 L ha-1.
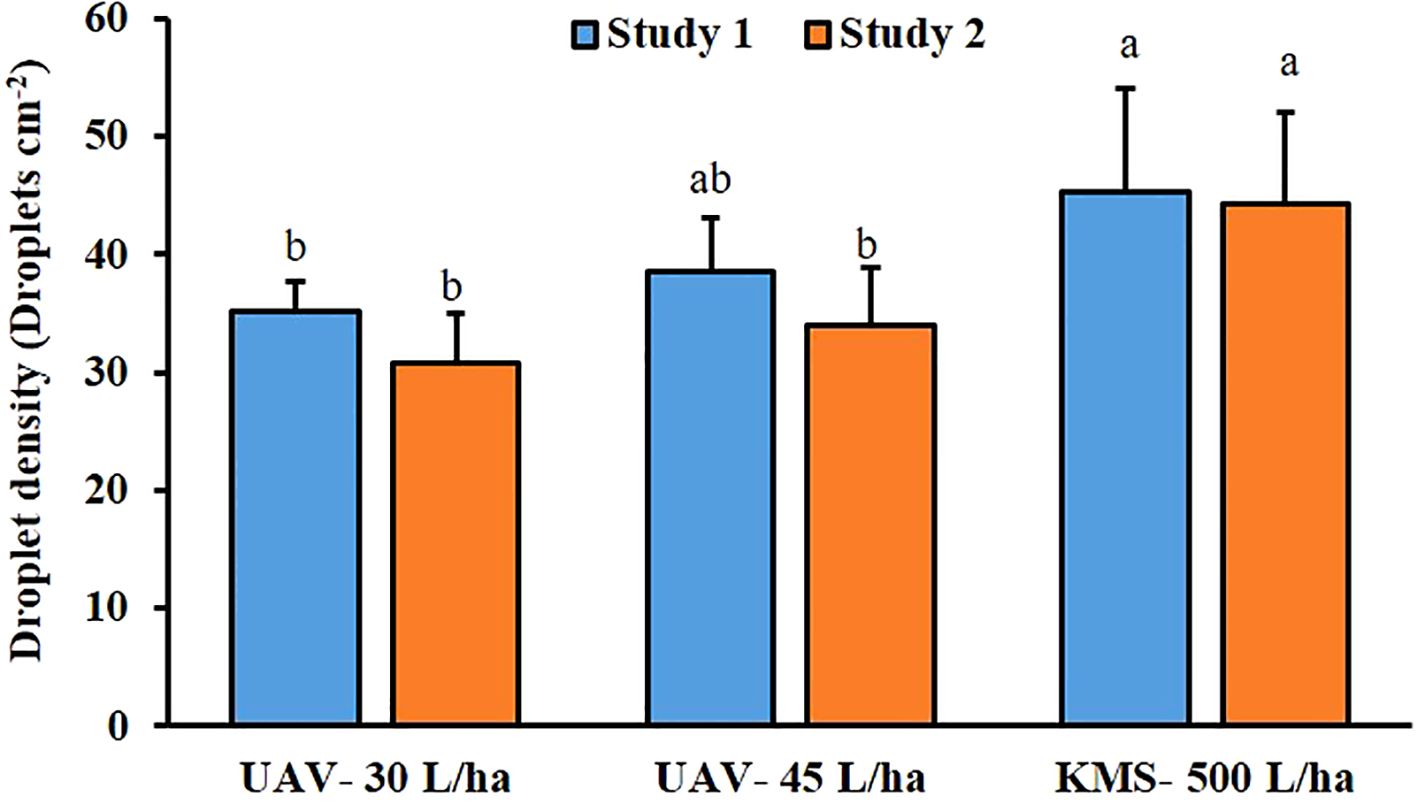
Figure 5. Droplet density on WSPs by sprayer and volume. Treatments sharing the same letter were not significantly different according to Tukey’s HSD test (p>0.05).
3.1.4 Droplet distribution
Spray droplet distribution is another important parameter to evaluate spray efficacy. A smaller CV indicates a uniform distribution of droplets. The results show that the UAV treatments demonstrated lower CV values compared to the KMS treatment, indicating better spray distribution across the experimental plots. The uniformity of droplet distribution on the WSPs for UAV at 30, 45 L ha-1 and KMS at 500 L ha-1 were 8.64%, 17.21%, and 21.23% in study 1 and 11.76%, 19.21%, and 19.68% in study 2, respectively (Figure 6).
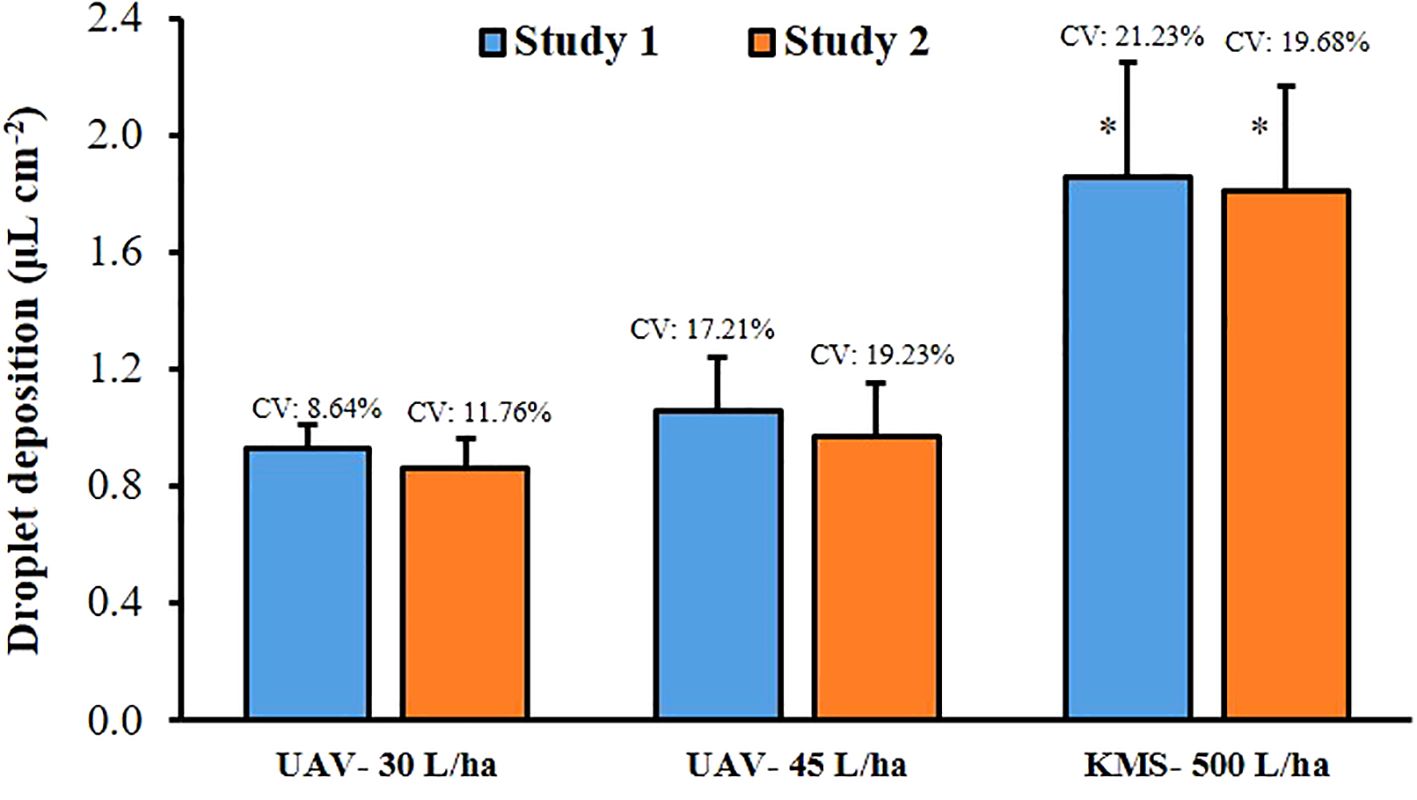
Figure 6. Droplet deposition and coefficient of variation on WSPs by two sprayers at three spray volumes. Asterisks represent that the treatment was significantly differ from other treatments with the p value <0.05 at Tukey’s HSD test.
3.1.5 Droplet deposition
Tested spray volumes had a significant effect on droplet deposition (Figure 6). The highest deposition was recorded in the KMS treatment with a spray volume of 500 L ha-1 (1.86 ± 0.39 μL cm-2 and 1.81 ± 0.35 μL cm-2 for study 1 and study 2, respectively), and it was significantly different from the UAV treatments (p=0.000).
3.2 Weed control efficacy
The major weed flora found in the experimental fields comprised of Leptochloa chinensis, Echinochloa colona, Cyperus difformis, Cyperus iria, Marsilea quadrifolia, Bergia capensis, and Ludwigia parviflora. In addition, Sphenoclea zeylanica and Monochoria vaginalis were also observed in study 1 experimental plot. The application of herbicide using both UAV and KMS sprayers resulted in significantly lower total weed density and weed dry weight compared to the weedy check (p<0.001 and p=0.000, respectively). The Shapiro-Wilk normality test confirmed that the data sets were normally distributed, while the Bartlett test demonstrated that the variance was homogeneous across all data sets without any bias. The results for normality and homogeneity of variance are presented in Supplementary Table 1. The total weed density recorded in the test plot were 75.5 ± 13.36, 63.5 ± 19.80, 47.5 ± 18.38, and 220.5 ± 27.04 numbers m-2 in study 1 and 83.5 ± 16.26, 88.0 ± 17.97, 62.5 ± 27.58, and 201.5 ± 31.82 numbers m-2 in study 2 for UAV sprayer at 30, 45 L ha-1, KMS treatments, and weedy check, respectively (Figure 7A). Numerically lower weed dry weight was recorded in the herbicide-treated plots using the KMS (15.17 ± 2.95 and 14.70 ± 7.32 for study 1 and study 2, respectively) (Figure 7B), and it was on par with UAV treatments. A higher WCE of 87.4% in study 1 and 84.7% in study 2 were recorded in the KMS. The WCE values for the UAV treatments at spray volume for 30 and 45 L ha-1 were 85.7% and 79.7%, 86.1% and 77.0%, for study 1 and study 2, respectively.
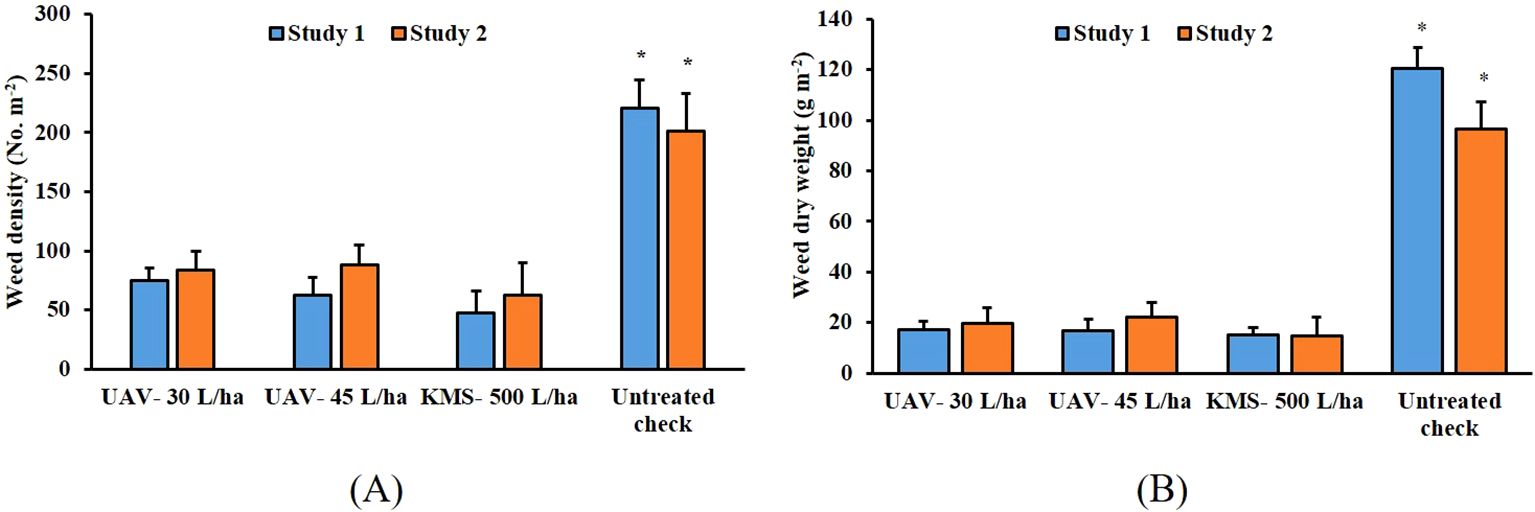
Figure 7. Control efficacy of different sprayer and spray volume on weed density (A) and weed dry weight (B). Asterisks represent that the treatment was significantly differ from other treatments with the p value <0.05 at Tukey’s HSD test.
4 Discussion
4.1 Spray droplet parameters
Our study evaluated the effective spray volume for herbicidal weed management in DSR using UAV applicator. We analyzed the droplet spray parameters for UAV with two tested volumes in comparison with KMS applicator. Increasing carrier volume from 30 to 45 L ha-1 with UAV increased the VMD value only by 4.6% in study 1 and 4.3% in study 2. Creech et al. (2015a) concluded that increasing the carrier volume from 47 to 187 L ha-1 increased the VMD value only by 5% at constant height (1.4 m), which indicates that the droplet size of the herbicide was not dependent on carrier volume. Similarly, there was no significant difference in the spray area coverage between the UAV treatments with the tested spray volumes. This can be attributed to the fact that increasing spray volume under the same spraying system does not have much influence on the spray area coverage, especially when the weather condition is the least concern particularly environmental wind. Wind velocity changes during flight passes were relatively narrow (0.62 to 0.78 m s-1 and 0.81 to 0.95 m s-1 for study 1 and study 2, respectively), indicating minimal impact on deposition. Similar results were reported by Martin et al. (2020) in an experiment on UAV application of spray mixture on palmer amaranth and morning glory, where a spray volume of 18.7 and 37.4 L ha-1 yielded similar area coverage under the same spraying system.
The number of spray droplets is a critical factor that affects weed control efficacy. The droplet density was not significantly different between UAV treatment with tested spray volumes. Previous studies have shown that the number of droplets tends to increase as the droplet size decreases (Adams and Hall, 1990; Bouse et al., 1992). However, smaller droplets can lead to serious drift problems (Chen et al., 2020). Additionally, increasing the spray volume did not influence the droplet density when the droplet size remained the same (Shan et al., 2021). Another possible reason could be increasing spray volume caused the droplets to overlap and resemble as a single droplet. Increasing the number of droplets can enhance contact with weeds and improve herbicide efficacy, particularly with highly concentrated herbicide formulations. Syngenta Crop Protection AG (Basel, Switzerland) recommended a minimum 30 to 40 droplets cm-2 for post-emergence herbicide application to achieve effective weed control (Zhu et al., 2011).
The uniformity of droplet distribution can be affected by sprayer operating parameters (Qin et al., 2016) and meteorological conditions (Hussain et al., 2019). In both studies, the fluctuations of wind velocity were relatively narrow (0.62 to 0.78 m s-1 in study 1 and 0.81 to 0.95 m s-1 in study 2), resulting in minimal influence on droplet deposition. At the same time, UAV rotor downwash air assisted in the uniform distribution of spray droplets at lower spray volumes (Shengde et al., 2017). The higher uniformity of spray deposition observed in the UAV at 30 L ha-1, can be attributed to ideal flight parameters such as flight height (1.5 m) and forward speed (5 m s-1) for the autonomous navigation of the UAV sprayer. However, the efficiency of UAV was not uniform to all models, and it may vary with changes in nozzles, flight parameters, and UAV models. Researchers have reported that an increase in flight altitude and flight speed can result in insufficient and poor uniformity of droplet deposition due to drift and evaporation (Zhang et al., 2020; Hunter et al., 2020). Poor uniformity of the spray distribution can negatively affect the efficacy of herbicide application and lead to poor weed control. Flight operational parameters in this study were consistent with the result of Qin et al. (2016). In contrast, the poor uniformity recorded in the KMS treatment may be attributed to the operator’s unsteady walking speed and arm movement as well as instances of missing and overlapping operations (Wang et al., 2019). The larger droplet size and higher spray volume used in the KMS treatment resulted in higher droplet deposition on the WSP samplers. Conventional sprayers operating at low pressures produce larger droplets, which tend to deposit more quickly on target weed plants. This is due to the increased gravitational pull on larger droplets compared to smaller ones, helping to reduce both droplet drift and evaporation losses (Chen et al., 2020).
4.2 Weed control efficacy
The greater weed control was achieved at a spray volume of 500 L ha-1 using the KMS, which was comparable to the UAV treatments. The efficacy of herbicides was not influenced by carrier volumes, particularly for UAVs. This can be attributed to uniform droplet deposition on weed plants and the major role of UAV rotor downwash in penetrating droplets deep into dense crop canopy systems. Martin et al. (2020) reported that UAV-based spraying with a spray volume of 37.4 L ha-1 resulted in a 4-fold increase in droplet deposition on the abaxial surface of weed foliage compared to a conventional sprayer with a spray volume of 140 L ha-1. The application of systemic herbicide (bispyribac-sodium) using UAV and KMS methods significantly reduced weed density compared to untreated check, which confirms that the efficacy of herbicide was not affected by the application methods. The application of bispyribac-sodium resulted in effective weed control in all treated plots as it inhibited the biosynthesis of the enzyme acetolactate synthase, thereby arresting weed growth. Similarly, Jeevan et al. (2023b) also stated that there was no significant difference between the efficacy of systemic herbicide in UAV and conventional applications.
In both studies, the application of herbicide resulted in a significant reduction in weed dry weight under KMS, which was comparable with UAV treatments. Similar results were reported by Pranaswi et al. (2024) and confirmed that the application of systemic herbicide metribuzin on wheat through UAV resulted in similar weed control as compared to backpack application (Pranaswi et al., 2024). Similarly, Kumar et al. (2022) also reported no significant difference in weed control efficacy between low and high volume application of systemic herbicides applied through UAV and knapsack sprayers in wheat. In both studies, WCE under UAV treatments was similar to KMS, with a narrow variation of 1.7% to 5.1% WCE recorded between the KMS and UAV at 30 L ha-1. This confirms that the application method and carrier volume did not affect herbicide efficacy. Chen et al. (2019) reported that herbicide mixtures (isoproturon + clodinafop-propargyl + mesosulfuron) applied through UAV on wheat resulted in similar WCE compared to a conventional knapsack sprayer. However, the effects of spray volume on herbicide efficacy can vary depending on the physicochemical properties of the herbicides, such as solubility, droplet distribution, retention capacity, and chemical stability. For instance, contact herbicides require more carrier volume than systemic herbicides for UAV application to achieve desirable droplet distribution and retention on target weed (Shan et al., 2021). Creech et al. (2015b) reported that the carrier volume requirement to improve efficacy for different herbicides (glyphosate, glufosinate, lactofen, fluazifop-P, and 2,4-D) was not uniform in field and greenhouse experiments, indicating the need to consider specific application environments when assessing the effects of spray volume on herbicidal efficacy.
5 Conclusions
The results of this study demonstrate that droplet parameters such as VMD, spray area coverage, droplet density and droplet deposition were increased with higher spray volumes. Greater uniformity of droplet distribution was observed with the UAV at 30 L ha-1. Furthermore, UAV-based herbicide spraying in DSR system shows great potential for reducing weed density and weed dry weight, achieving similar levels of effectiveness comparable to the KMS method. Overall, these findings suggest that improved weed control efficacy was achieved with UAV (hexacopter) application at lower carrier volumes (30 L ha-1). This approach has the potential to limit human exposure to harmful chemicals. Future research advancements will include optimizing the spray volume for distinct contact herbicides in UAV applications, as well as refining UAV operating parameters. These parameters include factors like application height under varying wind conditions, UAV sprayer speed, nozzle configurations, and the utilization of adjuvants and their impact on droplet deposition. Additionally, studies on herbicidal spray drift from UAVs and its impact on sensitive non-target crops under small land holding applications can be assessed to maintain the georeferenced buffer zones. Such dedicated research endeavors will undoubtedly contribute to the evolution and enhancement of UAV spraying systems, solidifying their role as a sustainable and versatile solution for modern agricultural weed management.
Data availability statement
The datasets presented in this study can be found in online repositories. The names of the repository/repositories and accession number(s) can be found in the article/Supplementary Material.
Author contributions
RAIP: Data curation, Formal Analysis, Investigation, Validation, Writing – original draft. MAP: Conceptualization, Funding acquisition, Supervision, Writing – review & editing. PP: Conceptualization, Funding acquisition, Supervision, Writing – review & editing. VK: Conceptualization, Funding acquisition, Supervision, Writing – review & editing. MB: Conceptualization, Supervision, Writing – review & editing. BG: Writing – review & editing. SV: Writing – review & editing. MD: Writing – review & editing. SP: Resources, Writing – review & editing. RK: Resources, Writing – review & editing.
Funding
The author(s) declare that financial support was received for the research, authorship, and/or publication of this article. The authors would like to thank the International Rice Research Institute (IRRI), Philippines and Tamil Nadu Agricultural University (TNAU), Coimbatore for providing funding support.
Acknowledgments
The authors acknowledge the International Rice Research Institute and Tamil Nadu Agricultural University for their valuable assistance in conducting this research as well as support received from INSPIRE, facilitated by the Department of Science and Technology, Government of India.
Conflict of interest
The authors declare that the research was conducted in the absence of any commercial or financial relationships that could be construed as a potential conflict of interest.
The author(s) declared that they were an editorial board member of Frontiers, at the time of submission. This had no impact on the peer review process and the final decision.
Publisher’s note
All claims expressed in this article are solely those of the authors and do not necessarily represent those of their affiliated organizations, or those of the publisher, the editors and the reviewers. Any product that may be evaluated in this article, or claim that may be made by its manufacturer, is not guaranteed or endorsed by the publisher.
Supplementary material
The Supplementary Material for this article can be found online at: https://www.frontiersin.org/articles/10.3389/fagro.2024.1491842/full#supplementary-material
References
Abd Ghani M. A., Juraimi A. S., Su A. S. M., Ahmad-Hamdani M. S., Islam A. M., Motmainna M. (2024). Chemical weed control in direct-seeded rice using drone and mist flow spray technology. Crop Prot. 184, 106853. doi: 10.1016/j.cropro.2024.106853
Adams A. J., Hall F. R. (1990). Initial behavioural responses of Aphis gossypii to defined deposits of bifenthrin on chrysanthemum. Crop Prot. 9, 39–43. doi: 10.1016/0261-2194(90)90044-8
Ahirwar S., Swarnkar R., Bhukya S., Namwade G. (2019). Application of drone in agriculture. Int. J. Cur Micro Appl. Sci. 8, 2500–2505. doi: 10.20546/ijcmas.2019.801.264
Ahmad F., Qiu B., Dong X., Ma J., Huang X., Ahmed S., et al. (2020). Effect of operational parameters of UAV sprayer on spray deposition pattern in target and off-target zones during outer field weed control application. Comput. Electron Agr. 172, 1–10. doi: 10.1016/j.compag.2020.105350
Arthanari P. M., Paul R. A. I. (2022). “Herbicide application using drones: advantage and constraints,” in Technological glimpses on weeds and their management. Eds. Mishra J. S., Sushilkumar, Rao A. N. (Indian Society of Weed Science., Jabalpur), 79–81.
Bhushan L., Ladha J. K., Gupta R. K., Singh S., Tirol-Padre A., Saharawat Y., et al. (2007). Saving of water and labor in a rice-wheat system with no-tillage and direct seeding technologies. Agron. J. 99, 1288–1296. doi: 10.2134/agronj2006.0227
Bouse L. F., Whisenant S., Carlton J. B. (1992). Aerial spray deposition on mesquite. T ASAE 35, 51–59. doi: 10.13031/2013.28569
Cao L., Cao C., Wang Y., Li X., Zhou Z., Li F., et al. (2017). Visual determination of potential dermal and inhalation exposure using allura red as an environmentally friendly pesticide surrogate. ACS Sustain Chem. Eng. 5, 3882–3889. doi: 10.1021/acssuschemeng.6b03050
Chaudhary A., Venkatramanan V., Mishra A. K., Sharma S. (2022). Agronomic and environmental determinants of direct seeded rice in South Asia. Circ. Econ Sust. 3, 253–290. doi: 10.1007/s43615-022-00173-x
Chauhan B. S., Johnson D. E. (2011). Ecological studies on Echinochloa crus-galli and the implications for weed management in direct-seeded rice. Crop Prot. 30, 1385–1391. doi: 10.1016/j.cropro.2011.07.013
Chauhan B. S., Mahajan G., Sardana V., Timsina J., Jat M. L. (2012). Productivity and sustainability of the rice-wheat cropping system in the Indo-Gangetic Plains of the Indian subcontinent: problems, opportunities, and strategies. Adv. Agron. 117, 315–369. doi: 10.1016/B978-0-12-394278-4.00006-4
Chen S., Lan Y., Zhou Z., Ouyang F., Wang G., Huang X., et al. (2020). Effect of droplet size parameters on droplet deposition and drift of aerial spraying by using plant protection UAV. Agronomy 10, 1–15. doi: 10.3390/agronomy10020195
Chen Y., Qi H., Li G., Lan Y. (2019). Weed control effect of unmanned aerial vehicle (UAV) application in wheat field. Int. J. Precis Agric. Aviat. 2, 25–31. doi: 10.33440/j.ijpaa.20190202.45
Creech C. F., Henry R. S., Fritz B. K., Kruger G. R. (2015a). Influence of herbicide active ingredient, nozzle type, orifice size, spray pressure, and carrier volume rate on spray droplet size characteristics. Weed Technol. 29, 298–310. doi: 10.1614/WT-D-14-00049.1
Creech C. F., Henry R. S., Werle R., Sandell L. D., Hewitt A. J., Kruger G. R. (2015b). Performance of post emergence herbicides applied at different carrier volume rates. Weed Technol. 29, 611–624. doi: 10.1614/WT-D-14-00101.1
Dass A., Shekhawat K., Anil K., Sepat S., Rathore S. S., Mahajan G., et al. (2017). Weed management in rice using crop-competition. Crop Prot. 95, 45–52. doi: 10.1016/j.cropro.2016.08.005
Farooq M., Flower K., Jabran K., Wahid A., Siddique K. H. (2011b). Crop yield and weed management in rainfed conservation agriculture. Soil Till Res. 117, 172–183. doi: 10.1016/j.still.2011.10.001
Farooq M., Siddique K. H., Rehman H., Aziz T., Lee D. J., Wahid A. (2011a). Rice direct seeding: experiences, challenges and opportunities. Soil Till Res. 111, 87–98. doi: 10.1016/j.still.2010.10.008
Ferguson J. C., Chechetto R. G., Adkins S. W., Hewitt A. J., Chauhan B. S., Kruger G. R., et al. (2018). Effect of spray droplet size on herbicide efficacy on four winter annual grasses. Crop Prot. 112, 118–124. doi: 10.1016/j.cropro.2018.05.020
Fritz B. (2006). Meteorological effects on deposition and drift of aerially applied sprays. T ASABE 49, 1295–1301. doi: 10.13031/2013.22038
Fukagawa N. K., Ziska L. H. (2019). Rice: Importance for global nutrition. J. Nutr. Sci. Vitaminol 65, S2–S3. doi: 10.3177/jnsv.65.S2
Haindavi P., Chandrasekhar K., Lakshmi N. V., Prasad P. R. (2018). Assessment of yield, consumptive use and water use efficiency of dry sown rice (Oryza sativa L.) influenced by irrigation schedules and weed management options. Bull. Env. Pharmacol. Life Sci. 7, 62–66.
Hunter J. E., Gannon T. W., Richardson R. J., Yelverton F. H., Leon R. G. (2020). Coverage and drift potential associated with nozzle and speed selection for herbicide applications using an unmanned aerial sprayer. Weed Technol. 34, 235–240. doi: 10.1017/wet.2019.101
Hussain S., Cheema M. J., Arshad M., Ahmad A., Latif M. A., Ashraf S., et al. (2019). Spray uniformity testing of unmanned aerial spraying system for precise agro-chemical applications. Pak J. Agr Sci. 56, 897–903. doi: 10.21162/PAKJAS/19.8594
Indiastat (2023). Agricultural rice production. Available online at: https://www.Indiastat.com/data/agriculture/rice (Accessed July 27, 2024).
Jabran K., Hussain M., Farooq M., Baba M., Dogan M. N., Lee D. J. (2012). Application of bispyribac-sodium provides effective weed control in direct-planted rice on a sandy loam soil. Weed Biol. Manag 12, 136–145. doi: 10.1111/j.1445-6664.2012.00446.x
Jeevan N., Pazhanivelan S., Kumaraperumal R., Ragunath K., Arthanari P. M., Sritharan N., et al. (2023a). Effect of different spray volumes on deposition characteristics of a fuel-operated UAV sprayer using herbicides in transplanted rice (Oryza sativa). Indian J. Agr Sci. 93, 720–725. doi: 10.56093/ijas.v93i7.133995
Jeevan N., Pazhanivelan S., Kumaraperumal R., Ragunath K., Arthanari P. M., Sritharan N., et al. (2023b). Effect of different herbicide spray volumes on weed control efficiency of a battery-operated unmanned aerial vehicle sprayer in transplanted rice (Oryza sativa L.). J. Appl. Nat. Sci. 15, 972–977. doi: 10.31018/jans.v15i3.4753
Kumar N., Chhokar R., Meen R., Kharub A., Gill S., Tripathi S., et al. (2021). Challenges and opportunities in productivity and sustainability of rice cultivation system: a critical review in Indian perspective. Cereal Res. Commun. 50, 573–601. doi: 10.1007/s42976-021-00214-5
Kumar S., Singh M., Singh S. K., Bhullar M. S. (2022). Droplet distribution and weed control efficacy of unmanned aerial vehicle sprayer in wheat crop. J. Agricul Eng. 59, 126–136. doi: 10.52151/jae2022592.1770
Kumar V., Ladha J. K. (2011). Direct seeding of rice: recent developments and future research needs. Adv. Agron. 111, 297–413. doi: 10.1016/B978-0-12-387689-8.00001-1
Legleiter T. R., Johnson W. G. (2016). Herbicide coverage in narrow row soybean as influenced by spray nozzle design and carrier volume. Crop Prot. 83, 1–8. doi: 10.1016/j.cropro.2016.01.009
Li Y., Li Y., Pan X., Li Q., Chen R., Li X., et al. (2017). Comparison of spray deposition, loss and residue of azoxystrobin and tebuconazole in sunlit greenhouse tomato and field cucumber of a new air-assisted sprayer and two conventional sprayers. Pest Mang Sci. 74, 448–455. doi: 10.1002/ps.4728
Mani V., Malla M., Gautam K. C. (1973). Weed-killing chemicals in potato cultivation. Indian Farming 23, 17–18.
Martin D., Singh V., Latheef M. A., Bagavathiannan M. (2020). Spray deposition on weeds (Palmer amaranth and Morningglory) from a remotely piloted aerial application system and backpack sprayer. Drones 3, 1–21. doi: 10.3390/drones4030059
Meivel S., Maguteeswaran R., Gandhiraj N., Srinivasan G. (2016). Quadcopter UAV based fertilizer and pesticide spraying system. Int. Acad. Res. J. Eng. Sci. 1, 8–12.
Meng Y., Su J., Song J., Chen W. H., Lan Y. (2020). Experimental evaluation of UAV spraying for peach trees of different shapes: Effects of operational parameters on droplet distribution. Comput. Electron Agr 170, 1–12. doi: 10.1016/j.compag.2020.105282
Mink R., Dutta A., Peteinatos G. G., Sokefeld M., Engels J. J., Hahn M., et al. (2018). Multi-Temporal Site-Specific weed control of Cirsium arvense (L.) Scop. and Rumex crispus L. @ in maize and sugar beet using unmanned aerial vehicle based mapping. Agriculture 8, 1–14. doi: 10.3390/agriculture8050065
Mondal B., Bisen J., Jambhulkar N. N., Tripathi R. (2022). Rice supply, demand and exportable surplus in India: Present vis-à-vis thirty years ahead. Oryza 59, 504–511. doi: 10.35709/ory.2019.56.2
Paul R. A. I., Arthanari P. M., Pazhanivelan S., Kavitha R., Djanaguiraman M. (2023a). UAV-based herbicide application for efficient weed management in direct-seeded rice. Agr Sci. Dig. 44, 295–300. doi: 10.18805/ag.D-5826
Paul R. A. I., Arthanari P. M., Pazhanivelan S., Kavitha R., Djanaguiraman M. (2023b). Drone-based herbicide application for energy saving, higher weed control and economics in direct-seeded rice (Oryza sativa). Indian J. Agr Sci. 93, 704–709. doi: 10.56093/ijas.v93i7.137859
Pranaswi D., Jagtap M. P., Shinde G. U., Khatri N., Shetty S., Pare S. (2024). Analyzing the synergistic impact of UAV-based technology and knapsack sprayer on weed management, yield-contributing traits, and yield in wheat (Triticum aestivum L.) for enhanced agricultural operations. Comput. Electron Agr. 219, 108796. doi: 10.1016/j.compag.2024.108796
Qin W. C., Qiu B. J., Xue X. Y., Chen C., Xu Z. F., Zhou Q. Q. (2016). Droplet deposition and control effect of insecticides sprayed with an unmanned aerial vehicle against plant hoppers. Crop Prot. 85, 79–88. doi: 10.1016/j.cropro.2016.03.018
Rejeb A., Abdollahi A., Rejeb K., Treiblmaier H. (2022). Drones in agriculture a review and bibliometric analysis. Comput. Electron Agr. 198, 107017. doi: 10.1016/j.compag.2022.107017
S386.2, ASABE Standards (2018). Calibration and Distribution Pattern Testing of Agricultural Aerial Application Equipment (St.Joseph, MI, USA: ASABE) 2018.
Shan C., Wang G., Wang H., Wang H., Wang S., Chen S., et al. (2021). Effects of droplet size and spray volume parameters on droplet deposition of wheat herbicide application by using UAV. Int. J. Agr Biol. Eng. 14, 74–81. doi: 10.25165/j.ijabe.20211401.6129
Shengde C., Lan Y., Jiyu L., Zhiyan Z., Aimin L., Yuedong M. (2017). Effect of wind field below unmanned helicopter on droplet deposition distribution of aerial spraying. Int. J. Agr Biol. Eng. 10, 67–77. doi: 10.3965/j.ijabe.20171003.3078
Singh G., Singh R., Singh V., Singh B., Nayak R. (1999). Effect of crop weed competition on yield and nutrient uptake by direct-seeded rice (Oryza sativa) in rainfed lowland situation. Indian J. Agron. 44, 722–727. doi: 10.59797/ija.v44i4.3577
Steel R., Torrie J., Dickey D. (1997). Principles and Procedures of Statistics: A Biometrical Approach. 3rd edn (New York: McGraw-Hill Book Company), 352–358.
Telli K., Kraa O., Himeur Y., Ouamane A., Boumehraz M., Atalla S., et al. (2023). A comprehensive review of recent research trends on unmanned aerial vehicles (UAVs). Systems 11, 400. doi: 10.3390/systems11080400
Tomita S., Miyagawa S., Kono Y., Noichana C., Inamura T., Nagata Y., et al. (2003). Rice yield losses by competition with weeds in rainfed paddy fields in north-east Thailand. Weed Biol. Manag 3, 162–171. doi: 10.1046/j.1445-6664.2003.00101.x
Vijayakumar S., Madireddy H., Bhusarapu S. C., Kumar R., Sundaram R. (2022). Drone application in rice cultivation: Experiences from ICAR-IIRR trails. Indian Farming. 72, 3–6.
Vijayakumar S., Nayak A. K., Poonam A., Aravindan S., Khanam R. (2020). Unmanned aerial vehicle (UAV) and its application in Indian agriculture: A perspective. Indian Farming. 70, 34–37.
Wang D. S., Zhang J. X., Zhang S. L., Xiong B., Qu F., Li X., et al. (2017). Spraying parameters and droplet deposition distribution analysis of CD-15 unmanned helicopter. Int. Agricul Eng. J. 26, 41–50.
Wang G., Lan Y., Yuan H., Qi H., Chen P., Ouyang F., et al. (2019). Comparison of spray deposition, control efficacy on wheat aphids and working efficiency in the wheat field of the unmanned aerial vehicle with boom sprayer and two conventional knapsack sprayers. Appl. Sci-Basel 9, 1–16. doi: 10.3390/app9020218
Wen S., Zhang Q., Deng J., Lan Y., Yin X., Shan J. (2018). Design and experiment of a variable spray system for unmanned aerial vehicles based on PID and PWM control. Appl. Sci-Basel 8, 1–22. doi: 10.3390/app8122482
Whitney R., Gardisser D. (2003). DropletScan Operators Manual (Stillwater, OK, USA: WRK of Oklahoma and WRK of Arkansas).
Yang F., Xue X., Cai C., Sun Z., Zhou Q. (2018). Numerical simulation and analysis on spray drift movement of multirotor plant protection unmanned aerial vehicle. Energies 11, 1–20. doi: 10.3390/en11092399
Zhang X. Q., Song X. P., Liang Y. J., Qin Z. Q., Zhang B. Q., Wei J. J., et al. (2020). Effects of spray parameters of drone on the droplet deposition in sugarcane canopy. Sugar Tech 22, 583–588. doi: 10.1007/s12355-019-00792-z
Keywords: drone, direct-seeded rice (DSR), flat fan nozzle, knapsack manual sprayer (KMS), remotely piloted aircraft systems (RPAS)
Citation: Paul RAI, Palanisamy MA, Peramaiyan P, Kumar V, Bagavathiannan M, Gurjar B, Vijayakumar S, Djanaguiraman M, Pazhanivelan S and Ramasamy K (2024) Spray volume optimization with UAV-based herbicide application for effective droplet deposition and weed control in direct-seeded rice. Front. Agron. 6:1491842. doi: 10.3389/fagro.2024.1491842
Received: 05 September 2024; Accepted: 06 November 2024;
Published: 22 November 2024.
Edited by:
Simerjeet Virk, Auburn University, United StatesReviewed by:
Sheikh Muhammad Masum, Sher-e-bangla Agricultural University, BangladeshAmar Godar, University of Arkansas, United States
Copyright © 2024 Paul, Palanisamy, Peramaiyan, Kumar, Bagavathiannan, Gurjar, Vijayakumar, Djanaguiraman, Pazhanivelan and Ramasamy. This is an open-access article distributed under the terms of the Creative Commons Attribution License (CC BY). The use, distribution or reproduction in other forums is permitted, provided the original author(s) and the copyright owner(s) are credited and that the original publication in this journal is cited, in accordance with accepted academic practice. No use, distribution or reproduction is permitted which does not comply with these terms.
*Correspondence: Murali Arthanari Palanisamy, YWdyb25tdXJhbGlAdG5hdS5hYy5pbg==