- 1Food and Agriculture Organization (FAO) Regional Office in Uzbekistan, Tashkent, Uzbekistan
- 2Tashkent State Agrarian University, Tashkent, Uzbekistan
- 3International Strategic Center for Agri-Food Development (ISCAD), Tashkent, Uzbekistan
Rainfed agriculture is primarily limited by unstable low precipitation, poor soil fertility and monocropping, which are the main factors leading to decreased crop production. This long-term research was conducted under a rainfed agroecosystem from 2019 to 2023 on the sierozem soil of the Karshi steppe, Uzbekistan. Along with winter wheat (WW) which was the main crop covering 50% of each proposed cropping pattern, chickpea (CH), safflower (SA), flax (FL), barley (BA) and canola (CA) were evaluated to find the most suitable rotation systems under no-till (NT) i.e. NT1: WW–CH–WW–FL, NT2: CH–WW–SA–WW, NT3: WW–SA–WW–BA and NT4: SA–WW–CA–WW compared against continuous WW produced with conventional tillage (CT). Results showed that the integrated effect of NT x crop diversification x residue retention positively affected crop productivity; however, their impact were significantly higher under the NT2 treatment, but not with continuous WW under CT. The highest grain yield of WW in the 2020-2021 growing season was recorded under NT2 and NT4 treatments with values of 1.47 and 1.30 Mg ha-1, while the lowest index (1.02 Mg ha-1) was found at the CT treatment. The grain yield in the NT treatments increased with the improvement of soil chemical and physical parameters, i.e. NPK and humus content. When comparing NT2 to CT treatment at the project end, the total N, P, and K values at the 0–20 cm soil profile were 27.9%, 13.9%, and 33.9% higher, respectively. This study concluded that implementation of NT along with strategic selection of legumes incorporated into the cropping system and residue management can be prioritized as rehabilitation measures in rainfed croplands.
Introduction
Soil quality is deteriorating in arable land because of frequent tillage, which often causes soil degradation, environmental damage, economic and resource use inefficiencies (Nurbekov et al., 2016). Particularly, land degradation in the dryland ecosystem of Uzbekistan intensified during the past 15-20 years, which requires the use of sustainable land management practices to restore soil productivity and nutritional balance (Nurbekov et al., 2023). The situation is being further exacerbated by the overexploitation of natural resources and the increasing scarcity of water resources due to human-induced climate change. Population growth alongside increased food demand put pressure on agriculture, requiring over 50% more food, feed, and biofuel by 2050 than the current level (FAO, SOLAW 2021). Moreover, climate constraints challenged crop production not only in rainfed areas of Uzbekistan but also in all Central Asian countries’ agroecosystems (Opitz-Stapleton et al., 2022; Grigorieva et al., 2023).
Despite, the shortage of resources, unstable precipitation and climate variability are significant constraints to the sustainability of agricultural production in rainfed locations (Kaye and Quemada, 2017; Khaitov et al., 2023), technological advancements, including water-saving technologies, conservation tillage, and drought-resistant crop production practices, primarily showed positive effects. However, adoption of conservation agriculture practices in Central Asia including Uzbekistan is slow compared to other part of the world (Nurbekov et al., 2022). For examples, Brazil and USA are leading countries on the adoption of conservation agriculture practices in arid rainfed conditions (Cárceles Rodríguez et al., 2022). Several studies have been done on this direction in these regions but with limited emphasis on the combined three principles of conservation agriculture (Kassam et al., 2019). Optimization models could guide growers in strategically selecting cropping systems, but these tools are often considered a single land management solution. Priorities should be directed to applying the combined use of proper crop rotation, NT, and mulching practices which showed an effective synergy in both agronomic and economic aspects under rainfed conditions (Nurbekov et al., 2023).
Introduction and development of cutting-edge techniques, i.e. alternative cropping systems and tillage practices, are in great demand in these rainfed areas, considering drought risk assessment (Islam and Reeder, 2014). Growing drought-tolerant crops, including cereals and legumes, based on resource-saving agrotechnologies might promote sustainable and efficient use of resources in these harsh ecosystems (Rustamova et al., 2023). Spring crops are of no importance in rainfed drylands because the rainfall during the growing season is low and unstable. Therefore, current farming systems in this region are based on the cultivation of winter cereals relying on over-winter and early spring rainfalls.
Winter wheat (Triticum aestivum L.) is an essential crop for food security, with a yearly grain production of 8 million tons from nearly 1.5 million hectares in Uzbekistan. Arable land in Uzbekistan totals 4 million hectares, including the total rainfed area of 755,900 hectares or 2.9% of agricultural land. Kashkadarya, as a southern region, has 258,500 hectares of arable rainfed land covered by grain crops (160,000 ha; 61.5%), legumes (40,000 ha; 15.4%), oil crops (35,000 ha; 13.5%), fodder and pulse crops (25,000 ha; 9.6%) (Statistical yearbook of the Republic of Uzbekistan, 2024).
This study hypothesized that applying NT along with diversified cropping systems and residue retention techniques might provide a significant advantage in rainfed arid regions. More suitable crop rotation patterns in rainfed environments can be tested by analyzing several cropping systems that contain multiple crops to maximize agricultural production and financial profitability. This study focused on the crop productivity and soil fertility of a four-field crop rotation system, including crops such as winter wheat (Triticum aestivum), chickpea (Pisum sativum), safflower (Carthamus tinctorius), flax (Linum usitatissimum), barley (Hordeum vulgare) and canola (Brassica napus). The sustainability of rainfed agricultural production depends on proactively implementing an action plan for drought management that supports incentive measures to use land and water resources more rationally. Initiatives were directed to incorporate drought risk management into agricultural production and food security strategies by disseminating appropriate sustainable agriculture technologies. Therefore, the objectives of this study were to evaluate crop productivity and soil quality in response to the land management of combined agrotechnologies, i.e. NT practices, diversified cropping system, and residue management under unstable low rainfed dryland agroecosystem.
Materials and methods
Research initiative
This study was part of the project funded by the Global Environment Facility entitled “Integrated Natural Resources Management in Drought-prone and Salt-affected Agricultural Production Landscapes in Central Asia and Turkey” (CACILM-2). The Food and Agriculture Organization of the United Nations (FAO) has been implementing this regional project since 2018 at the demonstration site located in Kashkadarya region, Uzbekistan, from 2019 to 2023 with a target to improve crop yield and soil quality in rainfed areas. The project aims to increase integrated natural resources management (INRM) techniques to mitigate the effects of climate change, water scarcity, soil salinization, and land degradation in rainfed arid areas. A part of the project results describing improved land management practices in saline irrigated soils has been recently reported (Nurbekov et al., 2023).
This initiative project promotes sustainable land management techniques that lessen risks and vulnerabilities, increase resilience, and limit pressures and adverse effects on natural resources. Additionally, the project helps implement and scale up the National Action Plans and the United Nations Convention to Combat Desertification (UNCCD). These goals are to be achieved by implementing a legume-based cropping system and conservation agriculture techniques like NT and residue management. This study provided empirical support and valuable recommendations for enhancing soil quality and crop productivity in rainfed desert environments.
Climate and soil condition
This open field experiments under rainfed conditions were conducted during 2019–2023 in southwest Uzbekistan (38.45° latitude and 65.7° longitude). The climate in this area is arid, with an average annual precipitation of 100-250 mm. These indices are also unstable and highly variable due to climate change. For example, very rainy periods occurred in 2019 with 336.7 mm rainfall, while it only reached 107.0 mm in 2021, presenting inadequate rainfall patterns (Figure 1). The driest period is prolonged between June and September, with almost no precipitation in the study area. The lowest average air temperature is observed in January at 2.8°C, and the highest indicator is 30.8°C in July. During the experiment period, 2019 had higher weather variability when the air temperature in July reached 32.9°C.
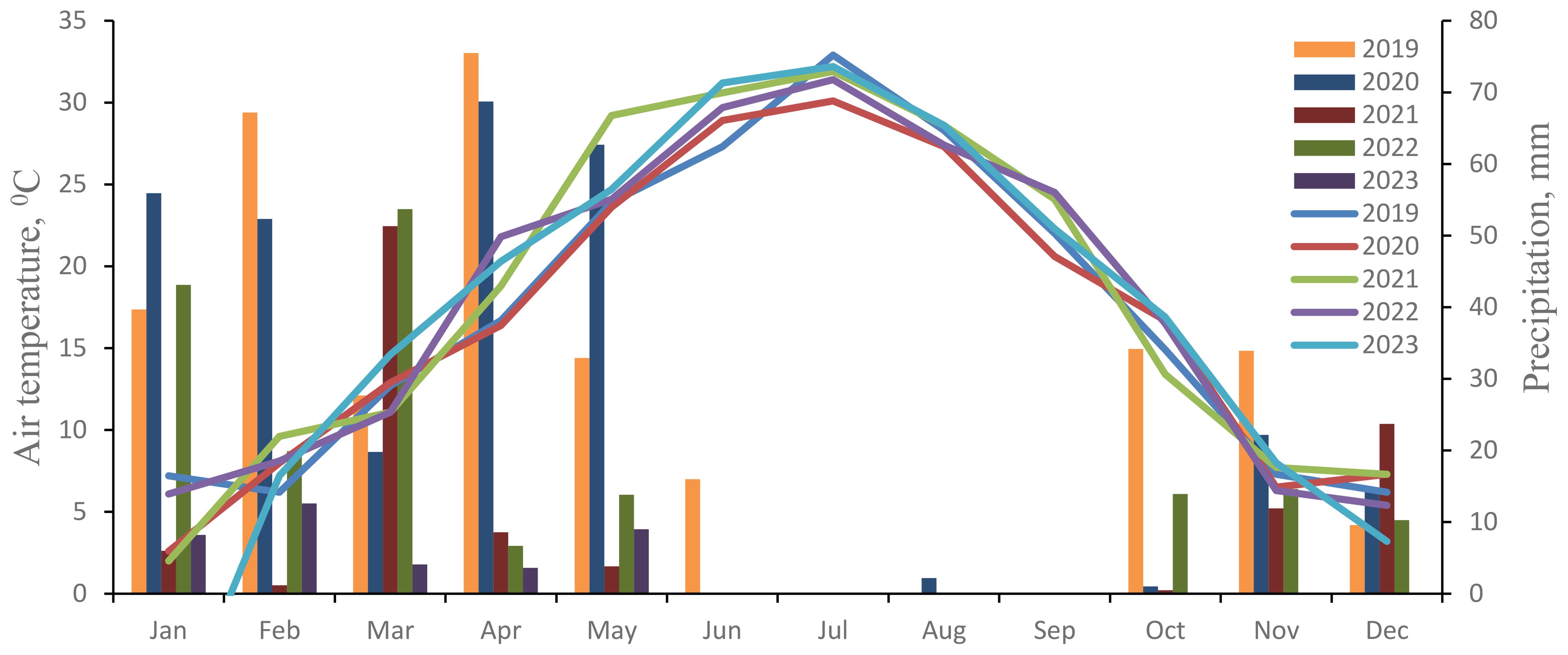
Figure 1. Air temperature (in curves) and precipitation data (in columns) were recorded in the study area, Kamashi district, Kashkadarya region (2019–2023 years long-term data).
The mechanical composition of soils in the experiment area has a heavy loam texture and is characterized by typical sierozem. Soil nutrient content is poor with 1.0% humus, 23-25 mg kg-1 NO3, 18.1-21.8 mg kg-1 P2O5, 104-127 mg kg-1 K2O in the 0-20 soil profile. These indicators were lower in the 20-40 cm soil profile consisting of 0.8-1.0% humus, 17-24 mg kg-1 NO3, 8-11 mg kg-1 P2O5, and 92-103 mg kg-1 K2O.
Experiment design
This crop rotation system was designed as a mixture of oil, legume and cereal crops, whereas the share of winter wheat was 50% for each proposed crop rotation system. In this rainfed environment, crops are seeded only once per year in autumn as a winter crop and harvested next summer. A strip plot design was employed in this trial in three replications; each plot size was 72.0 m² (4.80 x 15.0 m). The experiment was set up in 3 replications and a total study area was 1080 m².
Six different crops were studied in this experiment: WW – Winter wheat, CH – Chickpea, FL – Flax, SA – Safflower, BA – Barley, CA – Canola. These crops in this study were selected based on their abilities to tolerate drought, improve soil fertility and market demand. The treatments included five crop rotations i.e. continuous winter wheat under CT, NT1: WW–CH–WW–FL, NT2: CH–WW–SA–WW, NT3: WW–SA–WW–BA and NT4: SA–WW–CA–WW. WW (cv. Qayroqtosh) seeding rate of 120 kg/ha, BA (cv. Lalmikor) seeding rate of 100 kg/ha, Safflower (cv. Gallayaral) seeding rate of 20 kg/ha, Chickpea (cv. Jakhongir) seeding rate of 60 kg/ha, CA (cv. Taras) seeding rate of 30 kg/ha and Flax (cv. Bakhorikor) were used in this study. These winter-tolerant crops (for WW and BA) were seeded from 20 September to 15 October, whereas CA, CH and SA were planted from 1 to 15 November (for) each year. Flax has been planted from 15 February to 10 March during the research years. If winter crop sowing is delayed, it has a significant influence on the occurrence of the crop’s critical phenological stages.
A no-till multiple seed drill (SA-14600) was used for the NT treatments. Whereas the CT treatment is associated with several field operations including moldboard ploughing (30 cm depth), discing + mechanical hoeing treatments + seed planted with disc openers and a precise seed furrow-closing mechanism.
Applied agrotechnology
Ammonium nitrate - (NH3)2NO3 was applied in early spring at a rate of 50-60 kg/ha for WW. Urea - (NH2)2CO, fertilizers in the rate of a suspension were top dressed in two equal splits at jointing and boot stages. The application of ammophos (NH4H2PO4) and potassium chloride (KCl) was done with seeding under NT treatments while the CT treatment received the fertilizers prior to tillage.
Chickpea, safflower and canola received ammonium nitrate ((NH3)2NO3) during the vegetation period at 30, 40 and 50 kg/ha, respectively. Ammophos (NH4H2PO4) was applied with seeding under NT and before tillage under CT at 30, 20 and 30 kg/ha for chickpea, safflower and canola, respectively. Potassium chloride (KCl) was applied with seeding in NT and tossed prior to tillage at 20 kg/ha for chickpea, safflower and canola. Flax received 40 kg/ha N in the form of ammonium nitrate ((NH3)2NO3), 20 kg/ha P2O5 in the form of ammophos (NH4H2PO4), 20 kg/ha of potassium chloride (KCl).
Following harvest, crop residues were methodically crushed and mixed into the soil. Root remains were determined on 50×15 cm soil monoliths. While shoot residues were recorded on 1 m2 in each plot following standard techniques. In the middle of June, each plot was harvested to calculate the grain yield. Afterwards, grain yields were adjusted to match standard moisture content (15% for cereals, 9% for oil crops, and 14% for legume crops) to make comparisons. Every crop’s concentration of grain oil and protein content was methodically tested. Keeping an identical application method to all plots, pesticide treatments and efficacy were weekly determined by visual assessments of weeds, insects, and illnesses.
Soil chemical analysis
Based on the envelop approach, soil samples were gathered from the experimental plots at two specified depths of 0–20 and 20–40 cm in sealable plastic bags. The gathered soil samples were grounded and sieved through a 2-mm mesh screen for two weeks at room temperature before being subjected to chemical analysis. The physical and chemical properties of the soil (such as its bulk density, pH, EC parameters, and texture) were ascertained using the standard procedures devised by Ryan et al. (2001) (NPK, humus content) (NIAST, 2000). 0.5 g of soil samples were put in a tube, and 10 ml of 50% percholic acid was added. The mixture was then shaken for a minute, and 1 ml of concentrated sulfuric acid was added, and heated on a hot plate to cause decomposition. Total N, P2O5, and K2O were analyzed using the Kjeldahl distillation, Vanadate technique, and inductively coupled plasma spectro-photometer. Soil organic matter was measured using the Tyurin method. The bulk density (g cm−3) of the soil in each soil stratum was determined using the conventional core method. These taken soil samples were also sourced to assess soil moisture content after drying at 105°C for 6 hours. The following equation was employed to determine soil moisture content:
Statistic analysis
The collected data, which included crop yield, residue retention, soil nutrient content, and soil physical and chemical parameters, were subjected to an analysis of variance (ANOVA) using the CropSTAT 2.7 statistical software program, (2015). The Tukey test was used to determine whether there was a trait difference at P ≤ 0.05.
Results
Grain yield and quality
During the four growing seasons, WW grain yields were consistently higher under NT coupled with the diversified cropping system and residue management practices than continued monocropping WW with CT practices in the open field rainfed environment (Table 1). Results showed that WW experienced better moisture and nutrient levels under NT management, facilitating biomass accumulation and yield formation. Also, the NT management practices caused a significant shift in yield-related qualities of WW and other crops used in the rotation system.
At the 2019-2020 growing season, the lowest grain yield of WW was 1.25 Mg ha-1 under the CT. WW yield increased by 9.6 and 8.8% under the NT1 and NT3 treatments, respectively, compared to the CT treatment. Chickpea and safflower under NT2 and NT4 treatments yielded 0.92 and 0.85 Mg ha-1, respectively.
The highest grain yield of WW in the 2020-2021 growing season was recorded under NT2 and NT4 treatments with values of 1.47 and 1.30 Mg ha-1, while the lowest index (1.02 Mg ha-1) was observed at the CT treatment. Barley and safflower produced 0.87 and 0.84 Mg ha-1 grain yields under the NT1 and NT3 treatments, respectively.
NT exhibited higher grain yield for all tested crop rotation treatments than the monoculture WW under CT. There was a general trend of increasing crop productivity under NT; however, in the 2021-2022 season, there was more precipitation, which positively influenced the growth, development and grain formation of all tested crops compared to the other experiment years. The grain yield of WW was 1.43 Mg ha-1 under CT, while the NT1 and NT3 treatments exhibited 18.75% and 7.0% higher yields, respectively.
The trend of increasing crop productivity surpassed in the 2022-2023 growing season, where WW yields were 84.8% and 67.4% greater under the NT2 and NT4 treatments than the CT value. Flax and barley gave 0.90 and 0.97 Mg ha-1 yield under the NT1 and NT3 treatments, respectively.
Introducing chickpeas into this rotation resulted in a more pronounced increase in crop productivity. The highest grain yield was observed in the NT2 treatment, where the crop rotation sequence was CH–WW–SA–WW. The grain yield among the tested treatments formulated in the following order: NT2: CH-WW-SA-WW > NT1: WW-BA-WW-FL > NT3: WW-SA-WW-BA > NT4: SA-WW-CA-WW > CT: WW-WW-WW-WW.
Implementation of NT, along with crop diversification and residue retention techniques, facilitated the achievement of a total grain yield to the rate of 5.22 Mg ha−1 under the NT2 treatment during the four-year experiment period. Even though crop yield was greater under the NT2 treatment with a significant difference than the other treatments, non-significant differences were detected between NT4 (4.79 Mg ha-1) and NT1 (4.9 Mg ha-1) or NT1 (4.9 Mg ha-1) with NT3 (4.7 Mg ha-1).
The number of empty spikelets increased in WW under CT, most likely due to moisture deficiency and high temperatures during the grain filling period. Significant statistical differences were observed in the grain yield values between CT and NT treatments, which strongly suggests that, in the typical arid zone climate, the implementation of NT, along with legume-based crop rotation and crop residue retention, has the potential to maximize yield while conserving limited soil moisture. Thus, introducing chickpeas into the wheat-based cropping system under NT combined with residue retention brought benefits such as increased crop production under rainfed conditions.
Plant residues and soil moisture content
The root and phytoresidues increased significantly in response to the implementation of NT practice than that of the CT technique (Table 2). The total residue biomass was in the order of NT3 > NT1 > NT2 > NT4 > CT. While applying these advanced techniques increased residue retention in the soil, specific crop rotation systems also had a crucial role in this regard. In contrast, continuous WW mono-cropping in the CT field produced substantially lower residues as compared the NT treatments.
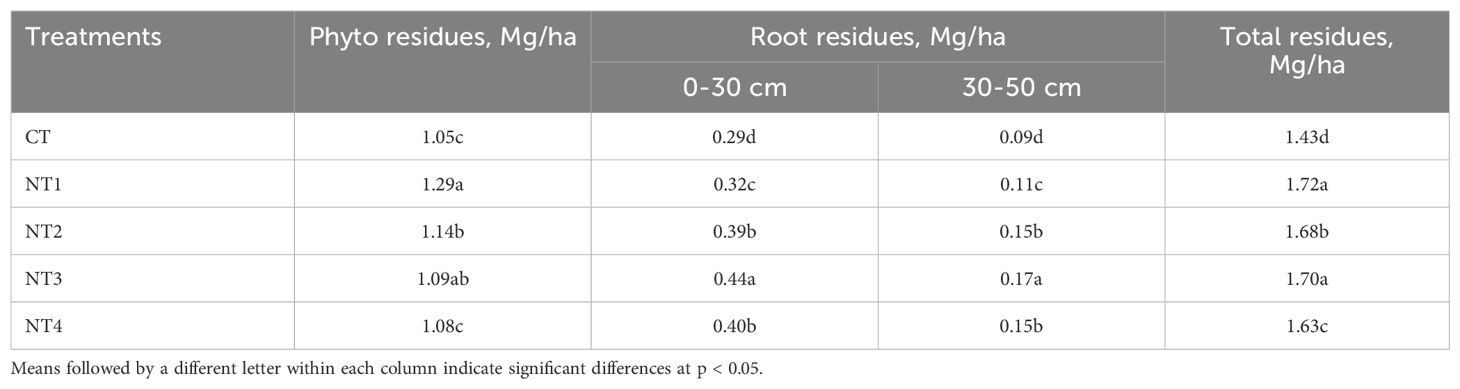
Table 2. Phyto and root residues under different crop rotation and tillage practices (averaged across 2019 and 2023 growth seasons).
The highest crop residue retention was observed in the NT1 and NT3 treatments, totaling 3.09 and 3.08 Mg ha-1, respectively. At the same time, there were no significant differences between these indices of the treatments. Over the experiment years, the tested NT treatments tended to produce higher crop residues than in the CT system under a rainfed agroecosystem. Within the NT system, lower residue biomass was measured in the NT2 and NT4 plots. However, the NT2 and NT4 treatments had similar residue retention without significant differences, exhibiting 1.72 and 1.70 Mg ha-1 values, respectively. In the meantime, the lowest crop residue in this experiment was detected under CT treatment with a total accumulated residue of 1.43 Mg ha-1.
During the experiment, the ambient temperature totals remained constant, with minimal deviations from the long-term norm. However, there was a substantial yearly fluctuation in the precipitation intensity. There were no significant differences between the four tested innovative methods regarding soil moisture content. The average soil water content dropped over time in both compared treatments (CT versus NT) starting in the early hot season (Figure 2).
In contrast to CT, the combined treatments conserved the soil water content more effectively. When crops reached the growth stage (April-May), soil water status under NT was significantly higher than that of CT. A similar pattern was observed during the soil moisture critical period from May to November. Notably, the soil water storage tended to be higher under the combined treatment by 82.7% in May, 28% in June, 64.6% in July, 107.7% in August, 111.8% in September, and 92.3% in October in comparison to CT.
Soil physical and chemical analysis
As shown in Table 3, the soil under NT was improved in terms of stability and total porosity. In contrast, CT decreased air-filled porosity, increasing bulk density and decreasing total porosity. Soil bulk density decreased by 14.2% and 7.9% in the NT1 and NT2 treatments, respectively, compared to the CT parameter after the four-year experiment period. Electrical conductivity indices were approximately 12.8-15% lower in the NT treatments compared to the CT value, decreasing salt concentrations in the soil profiles followed the same trend. Total porosity was ~13-20% higher in the NT treatment combinations than CT, depending on the soil depths. As expected, soil moisture content increased by 37.9-42.7% at 0-20 and 20-40 cm soil profiles for the NT groups compared to the CT treatment parameters. No significant differences were observed between the NT groups in the same soil depth.
The results presented in Table 4 show that the pH level was slightly higher under NT, even though no significant difference was observed. This gives insight that short crop rotation under NT might not have a short-term impact on the soil pH. Because humus content naturally declined as soil depth increased, there was a significant difference between the 0-20 cm and 20-40 cm soil profiles. The highest increase of soil humus content at the 0–20 soil depth was detected at the NT2 treatment, exhibiting a 20.2% increase compared to the control. Similarly, NT3, NT1 and NT4 treatments showed higher soil humus content. Synergetic land management practices had a significant positive impact on soil humus content.
The combined application of NT x diversified cropping system x residue management resulted in a considerable increase in the overall quantities of N, P and K. Compared to the CT treatment, total N and P indices at the 0–20 cm soil profile were 13.9% and 12.5% higher with NT. When comparing NT2 to CT treatment, the total N, P, and K values at the 0–20 cm soil profile were 27.9%, 13.9%, and 33.9% higher, respectively. The NT3 treatment yielded the highest P values, indicating a 15.4% increase under the NT3 practice compared to CT. Although the studied land management strategies impacted the overall K content, this index did not significantly differ.
Applying NT treatment in conjunction with crop rotation and mulching techniques substantially (p < 0.05) impacted the available nutrients, specifically NO3, P2O5, and K2O. For example, averaged across the soil profiles, NO3 was increased by 7.1% under NT2 compared to CT during the four-year experiment period. Likewise, P2O5 and K2O indices were significantly higher under the NT practices than under CT, indicating the effectiveness of the implemented NT treatments on the soil nutrient balance.
Results showed that the increased soil residue retention due to the implemented NT x crop diversification x residue management methods played a significant role in improving soil physical stability, biological efficiency and chemical equilibrium, proving the positive effects of these advanced technologies in combination.
Discussion
Impact of no-till on crop productivity
Innovative and advanced land management approaches can be considered as a solution to drought preparedness planning and climate crisis (Islam et al., 2021). Under the challenging environment of the study area, diversification of drought-resistant crops, resource-saving technologies and mulch management practices have been found as incentives for investments in water-saving technologies. To date, several studies have compared diversified crop rotation systems in response to uncertain precipitation under rainfed conditions (Namozov et al., 2022). Perennials and annuals may serve as cover crops, and appropriate crop rotations should be designed considering the crops that are good predecessors (Ahmed et al., 2019). In agreement with this study, these authors emphasized the importance of using combined resource-saving agrotechnologies in rainfed agriculture because of positive impacts such as enhancing water retention, restoration of nutrient cycling and soil biological processes.
The impact of applied practices, i.e. NT, crop diversification and residue retention on grain yield was more pronounced in the CH-WW-SA-WW crop rotation cycle (chickpea – winter wheat – safflower – winter wheat). In agreement with this outcome, recent studies highlighted that the integration of innovative and comprehensive methods of conservation tillage, crop diversification with cover crops, and soil amendments can assist in restoring, maintaining, and improving soil quality (Islam et al., 2021). As an essential part of conservation tillage, NT maintains an undisturbed soil environment that positively impacts soil processes i.e. air, water, and thermal exchanges, enhances biodiversity and efficiency, controls chemical balancing, buffering, and equilibrium, maintains physical stability, increases soil organic matter accumulation and soil carbon sequestration (Daryanto et al., 2018; Mestelan et al., 2021).
As the drought level increases, crop productivity decreases; drought tolerance is plant-specific (Ilyas et al., 2021). The crops used in this study (WW – Winter wheat, CH – Chickpea, FL – Flax, SA – Safflower, BA – Barley, CA – Canola) are well adapted in the harsh climatic environment, showing characteristics such as withstanding drought, improving soil fertility, and meeting market demand. This phenomenon gives an insight into how proper crop rotation is strategically important under challenging climate conditions. Although crop growers have access to a wide range of crop varieties, their financial and material resources (labor, machinery, water, loans) are restricted in arid regions. Sustainable land management practices will help increase the area under crop production in producing areas plagued by salt or drought, considering the site-specificity of these techniques for every agroecosystem (Tian et al., 2021). It will raise the value of marginal water and soils for alternative livelihood systems, improve irrigation efficiency, and reverse the salinization trends in irrigated regions. Futhermore, resource saving technologies refer to methods and tools that help farmers adapt to climate change, lower greenhouse gas emissions, and sustainably boost crop productivity on marginal soils (Hussain et al., 2021).
Adopting climate-smart agricultural practices that mitigate and adapt to climate challenges and enhance agroecosystem functions can be ensured by implementing proper cropping patterns, conservation agriculture, and efficient integrated farming systems.
Residue management
The importance of studying phyto and root residues is that this organic biomass is the primary source of soil humus formation, macro and microelements after decomposition (Shukla et al., 2022). Furthermore, planting follow-up crops after WW requires a lot of fuel and additional costs for land preparation activities when CT is used. Our research agrees with previous studies indicating crops differ in their ability to absorb nutrients from the soil, and the amount of residue they return can have varying nutrient inputs (Ashworth et al., 2018; Hammac et al., 2016). While studying the effect of mulch, Allanov et al. (2019) highlighted the importance of crop residue as mulch, which can be considered as a soil amendment to maximize water and nutrient use efficiency as well as crop productivity in degraded soils of arid regions. Wheat residue has a relatively high C/N ratio (Lin and Chen, 2014); and decomposes slowly (Islam and Reeder, 2014) which probably resulted in a considerable increase in the overall quantities of N, P, and K as compared to CT. Soil nutrients in available forms (NO3; Р2О5: К2О) were influenced consistently and positively by the tested land management practices.
Previous studies also confirmed that the high residue biomass in the soil ensures the constant enrichment of the soil with humus and improves the soil’s water-physical, physical, and chemical properties (Fu et al., 2021). The adverse effects of soil erosion processes are limited as a result of the covering of the soil surface with crop residue throughout the year, resulting in the creation of favorable conditions for the following year’s crops. Furthermore, in well-developed soil cover, the humus layer thickens and increases the fertility of these soils. This study also revealed the increase of soil moisture content in the early spring by 37.9-42.7% at 0-20 and 20-40 cm soil profiles for the NT groups compared to the CT treatment parameters (Table 3). However, the difference of soil moisture status between these two treatments was substantially higher during the hot periods (Figure 2). More importantly, the soil under NT improved stability and microporosity due to the crop residue retention and incorporation into the soil, which is more beneficial in arid rainfed regions than in other conditions (Namozov et al., 2022). Recent studies also confirmed that residue management is the key element of moisture conservation, nutrient cycling, soil health and structure maintenance processes (Fu et al., 2021; Nurbekov et al., 2023; Qureshi et al., 2023).
This study showed that mulching soil surfaces reduces water evaporation, which helps to conserve water. Meanwhile, drought, heat stress, salinity, and limited water throughout the cropping season are the main threats to agricultural sustainability in dryland contexts. Many studies indicated that mulching is effective in situations when water scarcity becomes a limiting factor of agricultural production (El-Beltagi et al., 2022). A long-term study of mulching effects researched by Zhang et al. (2021) also found much more moisture storage, enhanced soil microbial biomass and nutrient cycling activity in the crop root zone under an arid environment. Yu et al. (2021) investigated the impact of mulch in the dryland cropping system and discovered that mulch significantly reduces soil temperature and water evaporation.
Under the conventional tillage system, the soil becomes more vulnerable to wind and water erosion, followed by a number of negative impacts, i.e. soil compaction, degradation, and infertility, ultimately affecting the yield (Jahangir et al., 2021).
Crop diversification
The rotation effect was substantial in this rainfed system since chickpeas, as a legume crop, improved soil quality with appropriate treatment. Under rainfed land management, chickpeas appear as an essential crop to increase the productivity and income of farmers and should be considered at different levels of decision-making. Despite relatively low production (0.92 Mg ha–1) as a consequence of decreasing soil moisture content from booting/stem elongation to flowering, chickpeas are fully integrated into the cropping system, exhibiting potential properties when water resources are limited. This indigenous crop is well known for its capacity to grow in saline soil under hot and dry environments (Khaitov and Abdiev, 2018). In addition, this ancient leguminous crop is highly regarded for its N fixation ability, which facilitates increased soil fertility in nutrient-depleted poor soils (Abdiev et al., 2019). As the obtained results suggest, the best conservation techniques, along with a legume-based diversified cropping system, must be tailored for this rainfed environment. As expected, introducing chickpeas/legumes into the conservation system has enhanced soil health by adding organic matter, enhancing nutrient availability and stimulating microbial biomass.
High crop productivity and sustainability in the face of changing climate circumstances are associated with plant adaptation dynamics to diverse stress situations in terms of physiological, genetic, and molecular mechanisms (Chen et al., 2011; Yadav et al., 2021). As this study showed that implementing diversified crop rotation systems that include legumes can resolve the production constraints the area faces. Adopting resource-saving and soil-protecting technologies in agriculture supports biological life in the soil (Jarvan et al., 2014; Lal et al., 2017). Soil flora and fauna increase land productivity by breaking down plant residues and improving soil fertility, nutrient exchange, soil structure, water absorption, moisture retention and soil aeration (Lin and Chen, 2014). These techniques have a positive effect on the soil environment and help to rid of pests and disease-causing organisms (Rzaliyev et al., 2023).
Rainfed, low-input farming systems were a good fit for WW, BA, SA, FL and CH due to their more stable yields under limited soil moisture levels. However, the prospective yield targets must be adjusted in rainfed agricultural systems. Along with crop diversification and residue retention, NT is needed to provide adaptability and resilience in arid regions. These techniques complemented each other by exerting beneficial effects on crop yield capacities and soil health.
Conclusion
The results of this four-year-long experiment under the rainfed arid condition showed that the integrated effect of NT along with legume-based crop rotation and mulching had a significant positive impact, improving soil quality and crop productivity in a sustainable way. The crop rotation system consisting of WW–CH–WW–SA exhibited a more pronounced effect under NT in this harsh soil and climatic condition when the productivity of WW was evaluated as a primary outcome. It also turned out that precipitation variability was a decisive factor impacting crop yield, whereas higher crop residues under NT improved soil moisture content by 37.9-42.7% at 0-20 and 20-40 cm soil profiles compared to the CT treatment parameters. A general trend of increasing crop productivity under NT was likely associated with the increase in soil moisture and fertility parameters.
The adverse effects of climatic challenges on crop production were substantially mitigated through the advancement of these innovative climate-smart agronomic practices that synergize NT, crop diversification and residue management and have the potential to be considered as a critical land management strategy in this rainfed agroecosystem.
Data availability statement
The original contributions presented in the study are included in the article/supplementary material. Further inquiries can be directed to the corresponding authors.
Author contributions
AN: Writing – original draft, Writing – review & editing, Conceptualization, Formal analysis, Methodology, Project administration, Supervision. BK: Data curation, Writing – original draft, Writing – review & editing. MK: Formal analysis, Funding acquisition, Writing – original draft, Writing – review & editing. SI: Data curation, Writing – original draft, Writing – review & editing. DQ: Data curation, Investigation, Writing – original draft, Writing – review & editing. ZY: Data curation, Investigation, Writing – original draft, Writing – review & editing. JK: Data curation, Investigation, Writing – original draft, Writing – review & editing. KE: Data curation, Formal analysis, Writing – original draft, Writing – review & editing. RN: Data curation, Formal analysis, Writing – original draft, Writing – review & editing.
Funding
The author(s) declare financial support was received for the research, authorship, and/or publication of this article. This study was carried out within the GEF-funded project entitled “Integrated natural resources management in drought-prone and salt-affected agricultural production landscapes in Central Asia and Turkey” (CACILM-2).
Acknowledgments
The authors thank the farm named “Javli bobo ugli Bakhtiyor” in Kamashi district, Kashkadarya region, Uzbekistan, for providing land, technical support and collaboration.
Conflict of interest
The authors declare that the research was conducted in the absence of any commercial or financial relationships that could be construed as a potential conflict of interest.
Publisher’s note
All claims expressed in this article are solely those of the authors and do not necessarily represent those of their affiliated organizations, or those of the publisher, the editors and the reviewers. Any product that may be evaluated in this article, or claim that may be made by its manufacturer, is not guaranteed or endorsed by the publisher.
References
Abdiev A., Khaitov B., Toderich K., Park K. W. (2019). Growth, nutrient uptake and yield parameters of chickpea (Cicer arietinum L.) enhance by Rhizobium and Azotobacter inoculations in saline soil. J. Plant Nutr. 42, 2703–2714. doi: 10.1080/01904167.2019.1655038
Ahmed N., Masood S., Ahmad S., Bashir S., Hussain S., Hassan I. R., et al. (2019). “Soil management for better crop production and sustainable agriculture,” in Agronomic Crops: Volume 2: Management Practices (Belgium: Springer).
Allanov K., Sheraliev K., Ulugov C., Ahmurzayev S., Sottorov O., Khaitov B., et al. (2019). Integrated effects of mulching treatment and nitrogen fertilization on cotton performance under dryland agriculture. Commun. Soil Sci. Plant Anal. 50, 1907–1918. doi: 10.1080/00103624.2019.1648496
Ashworth A. J., Allen F. L., DeBruyn J. M., Owens P. R., Sams C. (2018). Crop rotations and poultry litter affect dynamic soil chemical properties and soil biota long term. J. Environ. Qual. 47, 1327–1338. doi: 10.2134/jeq2017.12.0465
Cárceles Rodríguez B., Durán-Zuazo V. H., Soriano Rodríguez M., García-Tejero I. F., Gálvez Ruiz B., Cuadros Tavira S. (2022). Conservation agriculture as a sustainable system for soil health: A review. Soil Syst. 6, 87–95. doi: 10.3390/soilsystems6040087
Chen C., Han G., He H., Westcott M. (2011). Yield, protein, and remobilization of water soluble carbohydrate and nitrogen of three spring wheat varietys as influenced by nitrogen input. Agron. J. 103, 786–795. doi: 10.2134/agronj2010.0424
CropStat 2.7. Statistical software program (2015). (Philippines: International Rice Research Institute). Available online at: www.bbi.irri.org/products (Accessed April 21, 2024).
Daryanto S., Fu B., Wang L., Jacinthe P. A., Zhao W. (2018). Quantitative synthesis on the ecosystem services of cover crops. Earth-Sci. Rev. 185, 357–373. doi: 10.1016/j.earscirev.2018.06.013
El-Beltagi H. S., Basit A., Mohamed H. I., Ali I., Ullah S., Kamel E. A., et al. (2022). Mulching as a sustainable water and soil saving practice in agriculture: A review. Agron 12, 1881. doi: 10.3390/agronomy12081881
FAO, SOLAW, 2021. FAO SOLAW Synthesis Report. Available online at: www.fao.org/land-water/solaw2021/en/.
Fu B., Chen L., Huang H., Qu P., Wei Z. (2021). Impacts of crop residues on soil health: A review. Environ. pollut. Bioavail. 33, 164–173. doi: 10.1080/26395940.2021.1948354
Grigorieva E., Livenets A., Stelmakh E. (2023). Adaptation of agriculture to climate change: a scoping review. Climate 11.10, 202. doi: 10.3390/cli11100202
Hammac W. A., Stott D. E., Karlen D. L., Cambardella C. A. (2016). Crop, tillage, and landscape effects on near-surface soil quality indices in Indiana. Soil Sci. Soc Am. J. 80, 1638–1652. doi: 10.2136/sssaj2016.09.0282
Hussain M. I., Abideen Z., Qureshi A. S. (2021). Soil degradation, resilience, restoration and sustainable use. Sustain. Agr. Rev. 52, 335–365.
Ilyas M., Nisar M., Khan N., Hazrat A., Khan A. H., Hayat K., et al. (2021). Drought tolerance strategies in plants: a mechanistic approach. J. Plant Growth Reg. 40, 926–944. doi: 10.1007/s00344-020-10174-5
Islam R., Reeder R. (2014). No-till and conservation agriculture in the United States: An example from the David Brandt farm, Carroll, Ohio. Int. Soil Water Conserv. Res. 2, 97–107. doi: 10.1016/S2095-6339(15)30017-4
Islam K. R., Roth G., Rahman M. A., Didenko N. O., Reeder R. C. (2021). Cover crop complements flue gas desulfurized gypsum to improve no-till soil quality. Commun. Soil Sci. Plant Anal. 52, 926–947. doi: 10.1080/00103624.2021.1872594
Jahangir M. M. R., Islam S., Nitu T. T., Uddin S., Kabir A. K. M. A., Meah M. B., et al. (2021). Bio-compost-based integrated soil fertility management improves post-harvest soil structural and elemental quality in a two-year conservation agriculture practice. Agron. 11, 2101. doi: 10.3390/agronomy11112101
Jarvan M., Edesi L., Adamson A., Vosa T. (2014). Soil microbial communities and dehydrogenase activity depending on farming systems. Plant Soil Environ. 60, 459–463. doi: 10.17221/410/2014-PSE
Kassam A., Friedrich T., Derpsch R. (2019). Global spread of conservation agriculture. Int. J. Environ. Stud. 76, 29–51. doi: 10.1080/00207233.2018.1494927
Kaye J. P., Quemada M. (2017). Using cover crops to mitigate and adapt to climate change. A review. Agron. Sustain. Dev. 37, 4. doi: 10.1007/s13593-016-0410-x
Khaitov B., Abdiev A. (2018). Performance of chickpea (Cicer arietinum L.) to bio-fertilizer and nitrogen application in arid condition. J. Plant Nutt. 41, 1980–1987. doi: 10.1080/01904167.2018.1484134
Khaitov B., Karimov A., Kodirov A., Yuldasheva R., Kim Y. C. (2023). Identification, characterization and domestication of new sorghum (Sorghum bicolor L.) genotypes to saline environments of the Aral sea regions. Plant Sci. Tod. 10, 48–56.
Lal R., Mohtar R. H., Assi A. T., Ray R., Baybil H., Jahn M. (2017). Soil as a basic nexus tool: soils at the center of the food–energy–water nexus. Curr. Sustainable/Renewable Energy Rep. 4, 117–129. doi: 10.1007/s40518-017-0082-4
Lin R., Chen C. (2014). Tillage, crop rotation, and nitrogen management strategies for wheat in central Montana. Agron. J. 106, 475–485. doi: 10.2134/agronj2013.0316
Mestelan S., Sprunger N. S., Dyck C., Dick W. (2021). Four decades of continuously applied tillage or no-tillage on soil properties and soil morphology. Agrosystems Geosci. Environ. 4, e20195. doi: 10.1002/agg2.20195
Namozov F., Islamov S., Atabaev M., Allanov K., Karimov A., Khaitov B., et al. (2022). Agronomic performance of soybean with bradyrhizobium inoculation in double-cropped farming. Agricul. 12, 855. doi: 10.3390/agriculture12060855
NIAST. (2000). Method of Soil and Plant Analysis (Suwon, Korea: National Institute of Agricultural Science and Technology (NIAST).
Nurbekov A., Akramkhanov A., Kassam A., Sydyk D., Ziyadaullaev Z., Lamers J. P. A. (2016). Conservation Agriculture for combating land degradation in Central Asia: a synthesis. AIMS Agricul. Food 1, 144–156. doi: 10.3934/agrfood.2016.2.144
Nurbekov A., Kosimov M., Shaumarov M., Khaitov B., Qodirova D., Mardonov H., et al. (2023). Short crop rotation under no-till improves crop productivity and soil quality in salt affected areas. Agron 13, 2974. doi: 10.3390/agronomy13122974
Nurbekov A., Mirzabayev A., Karabayev M., Asozoda N., Khalilov N., Sydyk D. (2022). “Adoption of conservation agriculture in central asia: present trends and future prospects,” in Advances in Conservation Agriculture: Volume 3: Adoption and Spread, 1st ed. Ed. Kassam A. (Germany: Burleigh Dodds Science Publishing). doi: 10.19103/AS.2021.0088.11
Opitz-Stapleton S., Borodyna O., Nijhar I., Panwar V., Nadin R. (2022). Managing climate risks to protect net-zero energy goals: net-zero transition opportunities in Kyrgyzstan, Tajikistan and Uzbekistan, (London: Overseas Development Institute).
Qureshi A. S., Tesfaye E. M., Melese M. (2023). “Prospects of alternative agricultural systems to improve the productivity of marginal lands in Ethiopia,” in Biosaline Agriculture as a Climate Change Adaptation for Food Security. (Springer International Publishing, Cham).
Rustamova I., Primov A., Karimov A., Khaitov B., Karimov A. (2023). Crop diversification in the aral sea region: long-term situation analysis. Sustain. 15, 10221. doi: 10.3390/su151310221
Ryan J., Estefan G., Rashid A. (2001). Soil and plant analysis laboratory manual. 2nd ed (Allepo, Syria: International Center for Agricultural Research in the Dry Areas (ICARDA).
Rzaliyev A., Goloborodko V., Bekmuhametov S. H., Ospanbayev Z. H., Sembayeva A. (2023). Influence of tillage methods on food security and its agrophysical and water-physical properties. Food Sci. Tech. 43, 1–9. doi: 10.1590/fst.76221
Shukla A., Kumar M., Shukla A. (2022). Effect of conservation tillage and precision nitrogen management on wheat: A review. Int. J. Plant Soil Sci. 34, 87–97. doi: 10.9734/ijpss/2022/v34i2131245
Statistical yearbook of the Republic of Uzbekistan (Accessed 08 February 2024). stat.uz/en/publications/3627-statistical-yearbook-of-the-republic-of-Uzbekistan.
Tian Z., Wang J. W., Li J., Han B. (2021). Designing future crops: challenges and strategies for sustainable agriculture. Plant J. 105, 1165–1178. doi: 10.1111/tpj.v105.5
Yadav G. S., Das A., Kandpal B. K., Babu S., Lal R., Datta M., et al. (2021). The food-energy-water-carbon nexus in a maize-maize-mustard cropping sequence of the Indian Himalayas: An impact of tillage-cum-live mulching. Renew. Sustain. Energy Rev. 151, 111602. doi: 10.1016/j.rser.2021.111602
Yu Y., Zhang Y., Xiao M., Zhao C., Yao H. (2021). A meta-analysis of film mulching cultivation effects on soil organic carbon and soil greenhouse gas fluxes. Catena 206, 105483. doi: 10.1016/j.catena.2021.105483
Keywords: arid region, no-till, crop rotation, residue retention, rainfed condition, soil moisture, crop yield
Citation: Nurbekov A, Kosimov M, Islamov S, Khaitov B, Qodirova D, Yuldasheva Z, Khudayqulov J, Ergasheva K and Nurbekova R (2024) No-till, crop residue management and winter wheat-based crop rotation strategies under rainfed environment. Front. Agron. 6:1453976. doi: 10.3389/fagro.2024.1453976
Received: 24 June 2024; Accepted: 25 September 2024;
Published: 14 October 2024.
Edited by:
Othmane Merah, Université de Toulouse, FranceReviewed by:
Bharat Prakash Meena, Indian Institute of Soil Science (ICAR), IndiaAmit Anil Shahane, Central Agricultural University, India
Yuanye Ping, Zhengzhou Normal University, China
Copyright © 2024 Nurbekov, Kosimov, Islamov, Khaitov, Qodirova, Yuldasheva, Khudayqulov, Ergasheva and Nurbekova. This is an open-access article distributed under the terms of the Creative Commons Attribution License (CC BY). The use, distribution or reproduction in other forums is permitted, provided the original author(s) and the copyright owner(s) are credited and that the original publication in this journal is cited, in accordance with accepted academic practice. No use, distribution or reproduction is permitted which does not comply with these terms.
*Correspondence: Aziz Nurbekov, YXppei5udXJiZWtvdkBmYW8ub3Jn; Botir Khaitov, Ym90aXIua2hhaXRvdkBmYW8ub3Jn