- 1Department of Plant Pathology and Weed Research, Newe Ya’ar Research Center, Agricultural Research Organization (ARO), Ramat Yishay, Israel
- 2The Robert H. Smith Institute of Plant Sciences and Genetics in Agriculture, The Robert H. Smith Faculty of Agriculture, Food and Environment, The Hebrew University of Jerusalem, Rehovot, Israel
- 3Southern Arava R & D, Hevel Eilot, Israel
- 4The Arava Institute for Environmental Studies, Ketura, Israel
- 5Laboratory for Pest Management Research, Institute of Agricultural Engineering, ARO - the Volcani Institute, Rishon LeZion, Israel
Cynanchum acutum L. (Asclepiadaceae) is a perennial vine weed widespread in the Mediterranean region. In Israel, it is native to areas such as the Golan Heights, Galilee, and the northern Negev. Recently, its range has expanded to the Arava Valley in the southern part of Israel. In this study, we investigate the biology and phenology of C. acutum plants originating from both native and invasive populations. To achieve this, we utilized rhizomes from the invasive area in the southern region characterized by a hyper-arid desert climate (Yahel), and from the northern region characterized by a temperate climate (Gvat). Additionally, we examine chemical-based weed management approaches for controlling C. acutum. Rhizomes collected from the northern region showed higher emergence rates at temperatures between 15-30°C, whereas at 35°C, rhizomes from the southern region exhibited a higher emergence rate. Phenology experiments conducted simultaneously at southern and northern locations revealed that plants from the northern location flowered first at both sites. However, flowering initiation occurred ~20 days earlier on average when plants from both populations were grown at the southern site. Pre-emergence herbicide treatments using indaziflam and pyroxasulfone were effective in inhibiting C. acutum root formation. According to the percentage of emergence, pendimethalin showed higher emergence (93% and 100%) in comparison to indaziflam (10% and 93%) and pyroxasulfone (72% and 70%) (2020 and 2021, respectively). Treatments including the herbicide fluroxypyr, as well as the treatment of glyphosate + saflufenacil + surfactant were highly effective in controlling C. acutum plants. These results offer valuable insights into effective weed management strategies for addressing C. acutum infestations. Moreover, our findings underscore the remarkable plasticity of this species and shed light on how populations originating from diverse habitats may have adapted to distinct environmental conditions.
Introduction
Cynanchum acutum L. (swallow-wort) is a perennial vine weed that belongs to the Asclepiadaceae family. Cynanchum acutum is highly prolific via sexual and vegetative propagation (Figures 1B, E). This species is mainly associated with wet habitats and can be found in cultivated fields, orchards, fence rows, natural areas, and roadsides (Shu, 1995). Cynanchum acutum is increasingly problematic, as an invasive weed in several countries around the world (Meighani et al., 2021). Native to Southern Europe, it has spread through Asia, and the Mediterranean region, and can be found from Spain to Iran, and in Northern African countries (Gilbert et al., 1995; Ollerton and Liede, 1997). The vast spread of this species can be attributed to the production of a high number of small seeds and the profound allelopathic effects on other species (Daehler, 2003). Further, the rise in C. acutum invasiveness may also be attributed to its high plasticity, i.e., its ability to adapt to different environmental conditions, such as high temperatures and high salinity conditions, in diverse habitats (El-Katony et al., 2018). In Israel, it is native to the Golan Heights, Galilee, and in the northern Negev. However, in the last years, its range has expanded to the Arava region in the southern part of Israel (Figure 1A), and it has become a major pest in the date palm orchards due to its unique phenological and biological characteristics.
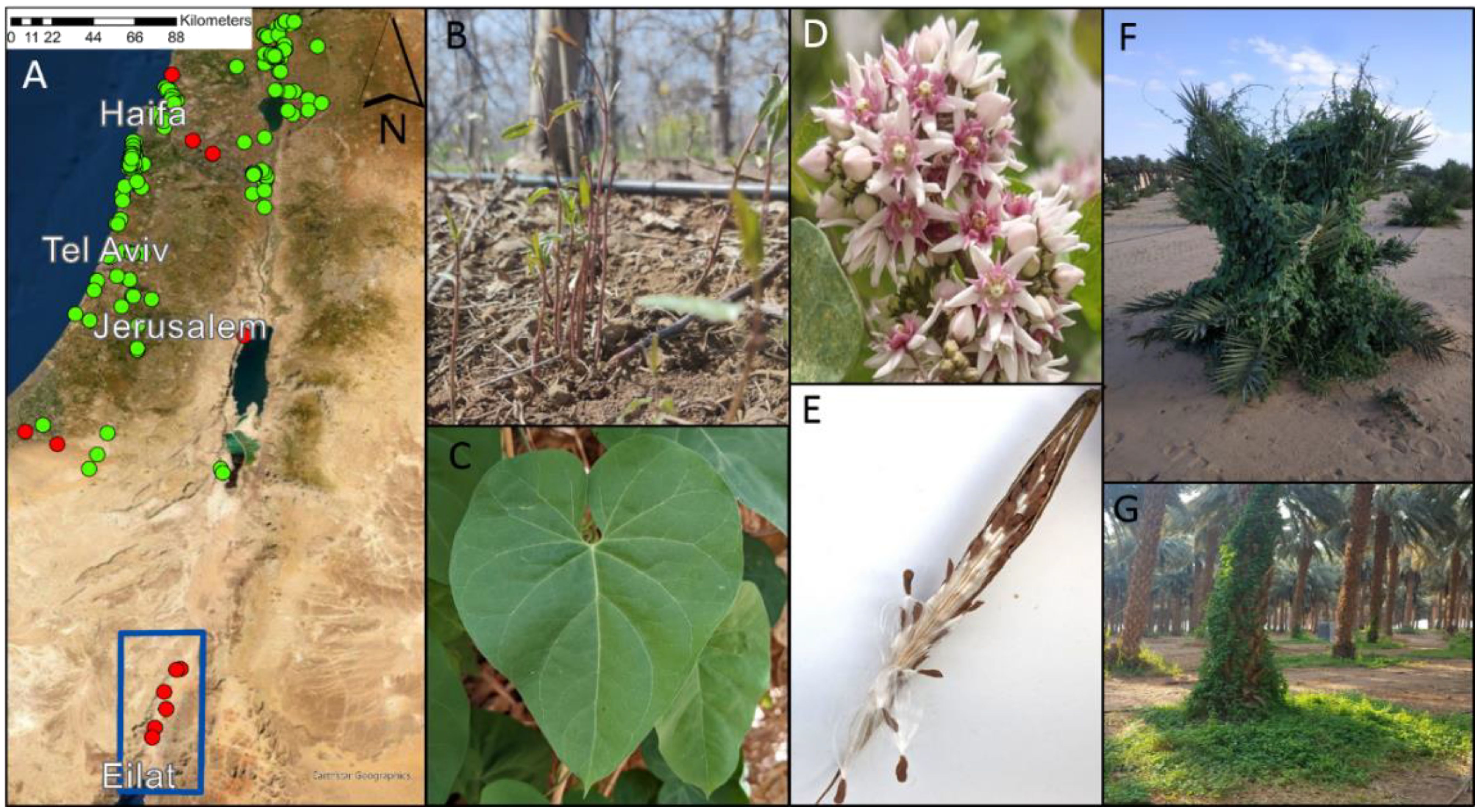
Figure 1. C. acutum distribution in Israel according to GBIF organization (GBIF.org (26 May 2022) GBIF Occurrence Download https://doi.org/10.15468/dl.n6rrq2) (green dots) and information collected during the current study (red dots). The blue frame outlines the invasion region in the southern Arava (A). C. acutum shoots sprouting from underground rhizomes (B), leaves (C) inflorescence (D), and seedpod (E). C. acutum infestation in a young (F) and mature orchard (G) in the southern Arava region.
Cynanchum acutum can climb on the tree trunk and produce many branches and woody stems at the base of the tree (Shu, 1995). This species is a strong competitor that can cause serious damage, mainly to young palm trees where it can fully cover the tree, its surroundings, and the fruit bunches (Figures 1F, G), inhibit their development, reduce yield production, and cause significant economic losses. In addition, C. acutum branches climbing on trees can interfere with the development of fruits and various operational work in the orchard, including care of trees and preparation for harvests. Under extreme scenarios, the infestation can result in the complete wilting of the young trees. In addition, Cohen et al. (1988) showed that C. acutum is a natural host plant for the whitefly-borne persistent virus, Tomato Yellow Leaf Curl Virus (TYLCV) that can be transmitted to other crops, including eggplants, potatoes, and peppers. Thus, indirect damages by this species may also influence yield quality and quantity.
Although C. acutum is considered a noxious pest in many countries it has invaded, studies describing the control of this weed are scarce. In Iran, both chemical and mechanical practices have been investigated for the control of C. acutum (Meighani et al., 2021). Results showed that systemic herbicides such as glyphosate, MCPA, and triclopyr provided adequate control and had high efficacy in reducing C. acutum density and biomass.
The alternative non-chemical control means for this species are limited. Hand weeding, mowing and collection of seedpods, and digging of rhizomes are some of the control measures aimed at limiting sexual and asexual plant reproduction of C. acutum. However, these tasks are tedious, expensive, and labor intensive and must be repeated throughout the growing season, mainly in the case of seedpod collection. Donkeys and camels currently being used in palm orchards are a biological weed control method, however, this method is not relevant for Cynanchum species as they contain high concentrations of toxic glycosides which are toxic and inedible to mammals (DiTommaso et al., 2005). These constraints emphasize the necessity for innovative ecology-based control strategies to complement existing and future control methods. However, the development of such approaches demands a profound understanding of weed biology and ecology (Bajwa et al., 2015).
Ecological control tools are based on data about key phases in the weed’s life cycle and phenology (Grundy et al., 2003). However, for C. acutum, this data is limited; only a few studies have examined the biology and ecology of this weed. Pahlevani et al. (2008) showed that C. acutum seeds may germinate under various temperatures and water potentials, with the ideal pH for germination being 4-6. Golzardi et al. (2014) showed that when C. acutum rhizomes were kept under high temperature (40C) for increasing time intervals (96h), their sprouting ratio was reduced. This was also the case for rhizomes stored under freezing temperatures for long time intervals, the increasing of the duration and decreasing of temperatures, initiated a reduction in the number of stalks and leaves obtained from each rhizome (Golzardi et al., 2015).
The overarching goal of our study was to obtain more data on the biology, and phenology of C. acutum and investigate possibilities for chemical weed management options to control the weed. Moreover, as C. acutum is considered native in the northern part of Israel and invasive in southern regions, we compared the competitive abilities and life cycle characteristics of plants originating from populations collected in both regions. Thus, plants from these populations were: 1) tested for rhizomes sprouting dynamics and magnitude under a wide range of temperatures; 2) phenological patterns for plants of C. acutum populations were examined; and 3) We explored new pre- and post-emergence herbicide treatments aimed at the effective control of C. acutum.
Materials and methods
Plant material
Because low seed germination was observed in preliminary studies (data not shown), we have focused our efforts on testing the sprouting and phenology of C. acutum plants originating from rhizomes. Plant material for all experiments was collected from orchards at two locations in the summer of 2021. Material from the first population was collected in a palm orchard owned by Kibbutz Yahel near Ramon International Airport (35.011855, 32.663539) in the Arava region, in which the weed is invasive. Material from the second population was collected from an olive grove at Kibbutz Gvat (32.663539, 35.205692) in the Jezreel Valley, a region in which the weed is native. For the Jezreel Valley, characterized by temperate climate, summers are hot with an average maximum daily temperature of 31°C, and winters are characterized by average maximum temperatures of 17°C, and rainfall is 450-650 mm (https://ims.gov.il/he). The southern Arava region has an arid-desert climate, with ~ 30 mm of rain on average every year. The average daily winter temperature in the Arava region is 21°C, however, maximum daily temperatures in the summer may reach an average of 44°C.
Rhizomes were washed and cleaned thoroughly, then cut into 5 cm segments and stored under a wet cloth. Rhizomes were kept at 4°C for 1-2 days after collection until they were used for phenological experiments, rhizome sprouting experiments, and to study the efficacy of various herbicides under controlled conditions.
Sprouting under different temperatures
Root segments from both Yahel and Gvat were cut into 5 cm long pieces and transplanted into 500 ml pots at a depth of 3 cm. Pots were filled with commercial potting mixture (Tuff, Marom Golan, Israel) enriched with slow-release fertilizer (Osmocote®). Emergence was evaluated at six constant temperatures: 10, 15, 20, 25, 30, and 35°C under dark conditions. Each pot contained five rhizomes. For each temperature treatment, seven posts were used. All experiments were conducted in growth chambers (Pol-Eko-Aparatura Incubator ISO:9001,2000, Pol-Eko-Aparatura Wodzisław, Pola). Each chamber was equipped with a temperature data logger (HOBO®, Data Logger; Onset Computer Corporation, Bourne, MA, USA). Each day, sprouting rhizomes were recorded and removed from each pot. The experiment continued for 33 days until no new rhizomes sprouted for five consecutive days.
Phenology of C. acutum
Phenology experiments were conducted in parallel at two sites, the Southern Arava R&D (29.886715, 35.075305) and Newe Ya’ar Research Center (32.707375, 35.179341). The experiment sites are within 20km distance from the site of the rhizome collection for each location and under the same climatic conditions. Experiments were conducted in nethouses with the average temperatures during the experiment in the Arava were 23/38.5°C (night/day), while average temperatures in Newe Ya’ar were 21/34°C. Rhizomes from the two populations described before, Yahel and Gvat, were planted in trays containing a commercial growth mixture. After emergence, on May 26 and 27, 2021, the plants were transferred to 2 litter pots at the Southern Arava R&D and Newe Ya’ar Research Center, respectively. For each experimental site, 50 plants were used for each population.
Plants were grown for 120 days until September 26 in the Arava and September 27 in Newe Ya’ar. Since C. acutum grow is a climbing vine, plants were trellised using a bamboo stick fixed in the center of each pot, to allow the vines to develop and to prevent them from tangling with other plants placed nearby. Irrigation was provided by a drip irrigation system. Plants were fertilized as needed using a commercial product (Ecogan®, nitrogen 20%: phosphate 20%: potassium20% + microelemnts).
Plant height was recorded weekly for the first five weeks of the experiment. The first flowering day was recorded for each plant. At the end of the experiment (the beginning of seed pod production), plants were harvested for further investigation. Therefore, the above soil shoots were separated from the root system. Roots were washed thoroughly from the growth mixture, and the two parts of the plant (shoot and root) were dried in an oven under 65°C for two days. Plant parts were then weighed separately.
Herbicide efficacy-net-house trials
Net-house experiments testing herbicide efficacy were carried out in the summer of 2020 and 2021 at the Newe Ya’ar Research Center in the Jezreel Valley. To have fresh C. acutum rhizomes for greenhouse experiments, they were collected from Gvat. Subsequently, on the day of harvest, rhizomes were washed, cut into 5 cm pieces and transplanted into 300 ml pots at a depth of one cm.
For pre-emergence herbicide trials, to ensure proper herbicide translocation through the soil, pots were filled with Newe Ya’ar soil (57% clay, 28.2% silt, 8.1% sand, and 1.63% organic matter). For post-emergence herbicide trials, the commercial potting mixture described above was used. Pots were maintained in a net-house under Israeli summer conditions in the Jezreel Valley, 19/28°C (night/day) average temperature, and 13 hours photoperiod. Pots were watered daily, with drip irrigation. All net-house experiments were arranged in a completely randomized design with 30 and 8 replicates of rhizomes for the pre-emergence and post-emergence trials, respectively. Experiments were conducted consequently in two separate trials.
Pre-emergence herbicide experiments
Experiments were conducted in consecutive years of 2020-2021. Herbicides were applied at the recommended labeled field rate as specified in Table 1 using a chain-driven sprayer delivering 300 L ha−1, with a flat-fan 8001E nozzle (TeeJet®, Spraying Systems Co., Wheaton, IL, USA). Three pre-emergence herbicides were tested (Table 1). Shoot fresh weight of each plant was recorded 30 days after treatment (DAT). The percentage of emerging plants was calculated for each treatment (one shoot per rhizome).
Post-emergence herbicide experiments
Seven post-emergence treatments were tested including a combination of five herbicides (Table 1). Plants were sprayed at two growth stages, 5-7 and 10-12 leaves. After the initial screening stage including all possible combinations (data not shown), seven herbicide combinations were chosen for further efficacy evaluation. Treatments that were used for post-emergence application: glufosinate + nonionic surfactant (Shatah 90®, ADAMA-Makhteshim, 0.05%), glufosinate + saflufenacil + nonionic surfactant, glufosinate + flumioxazin, fluroxypyr + surfactant, fluroxypyr + saflufenacil + nonionic surfactant, fluroxypyr + flumioxazin and glyphosate + saflufenacil + non-ionic surfactant (application rates are specified in Table 1). Shoot fresh weight and survival rate of each plant were recorded at 21 DAT. Plants were kept for an additional 21 days to evaluate regrowth after cutting for each plant, with plants that produced new shoots after cutting were considered as survivors.
Statistical analyses
Rhizome sprouting percent (f) was analyzed using a three-parameter log-logistic model:
where x is time (day), d is the upper limit, e is the time (days) to attain 50% of rhizome sprouting (ES50), and b is the relative slope around the ES50.
The number of flowering plants (y) was analyzed using a Weibull model with lag phase:
where M is the asymptote, which was fixed at 100%; k is the rate of increase; z is x of first emergence (or lag); and c is a curve shape parameter (Brown and Mayer, 1988). The Weibull function was fit to the flowering values using the nonlinear built-in NLS package in R (R Core Team 2023). Differences in plant elongation among populations compared at each of the five weeks of the experiment were analyzed using t-test (α = 0.05). The same test was used to analyze differences in mean dry shoot and root weight among populations.
For net-house herbicide application experiments, the data were transformed to biomass reduction (presentment of non-treated control). Thus, data were analyzed using a generalized linear mixed model (GLMM) with a beta distribution and logit family (GLMMTMB package; Brooks et al., 2017). Analysis of variance (ANOVA) was performed with the Anova.glmmTMB (GLMMTMB package) at α = 0.05, and followed by Tukey’s HSD test (α = 0.05) pairwise comparisons using the EMMEANS package version 1.5.4 (Lenth, 2020). To have a general assessment of C. acutum response, in pre-emergence herbicides efficacy, the year (2020 and 2021) and treatment (herbicides) were considered as fixed effect and the replicate as a random effect. In post-emergence herbicides efficacy, the treatment (herbicides), phenology stage (5-7 and 10-12 leaves) and time after treatment [(21 and 42 days after planting (DAP)] were considered as fixed effect whereas the replicate as random affect.
Results
Sprouting under different temperatures
Emergence under different temperatures was studied using rhizomes of C. acutum collected from both locations, Yahel and Gvat, recorded for 33 days to maximize rhizome sprouting potential. Emergence was recorded in the range of 15°C to 35°C, no emergence was observed in the rhizomes of both populations at a temperature of 40°C (Figure 2). For Gvat population maximum emergence was recorded at 15°C 100.03%, while for Yahel population it was at 20°C, 87.82% (Table 2). For both Gvat and Yahel populations, the optimal temperature for rhizome sprouting was 30 C, at which 50% emergence was achieved on average after 5.16 and 7.33 days, respectively (Table 2). The longest time to reach 50% emergence was measured at a temperature of 15 C, 18.73 and 21.98 days, for rhizomes from Gvat and Yahel populations, respectively. Differences were also observed in maximum emergence comparing rhizomes from the two populations. The final emergence rates were higher in the Gvat population at all temperatures from 15 to 30°C. The only temperature at which the Yahel population exhibited a higher final emergence rate, was 35°C, 41.58% vs. 32.30%, respectively (Table 2).
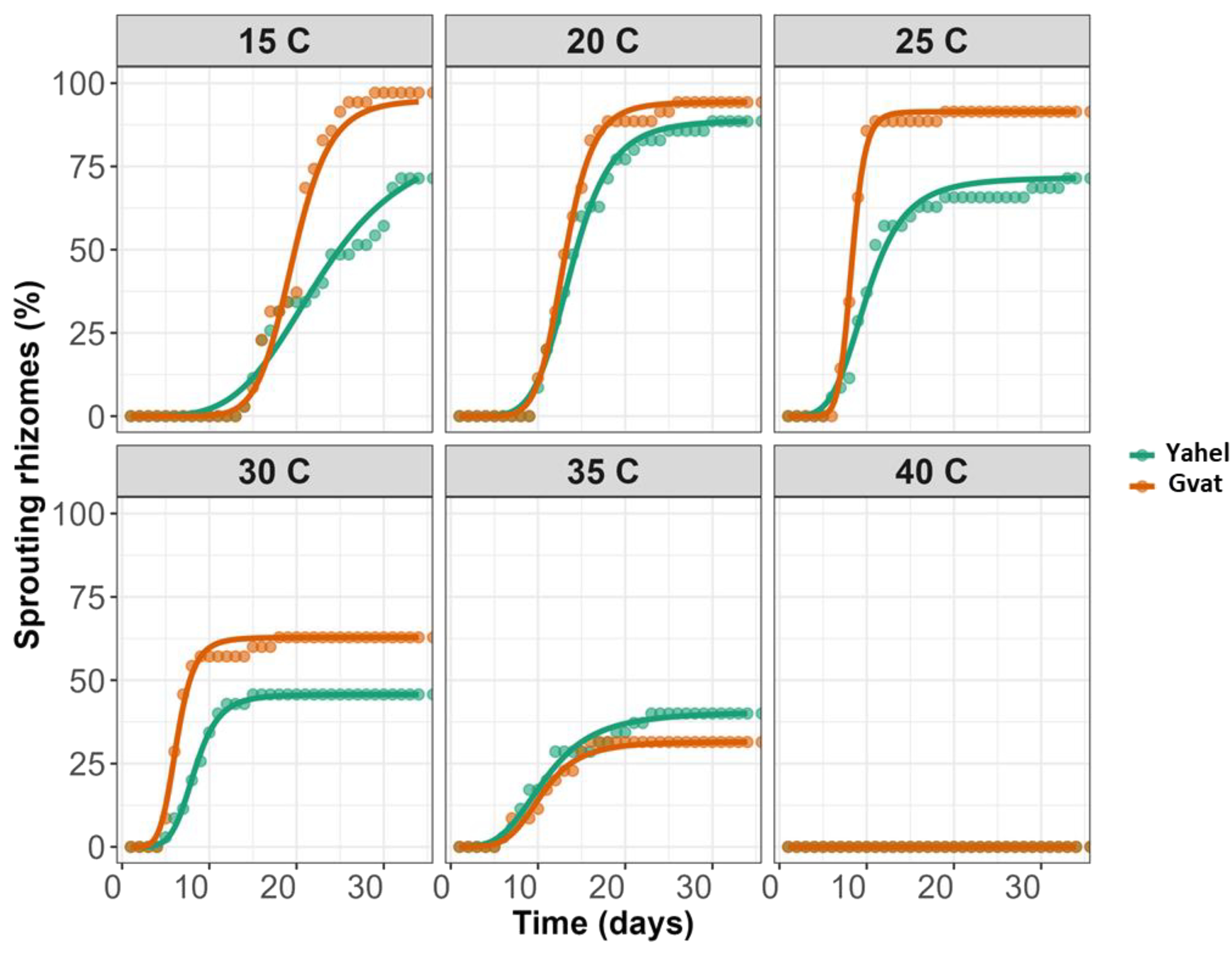
Figure 2. Emergence of plants generated from C. acutum rhizomes collected from Gvat (orange line) and Yahel (green line) and grown under different temperatures (n=5). Data were analyzed using a three-parameter log-logistic model.
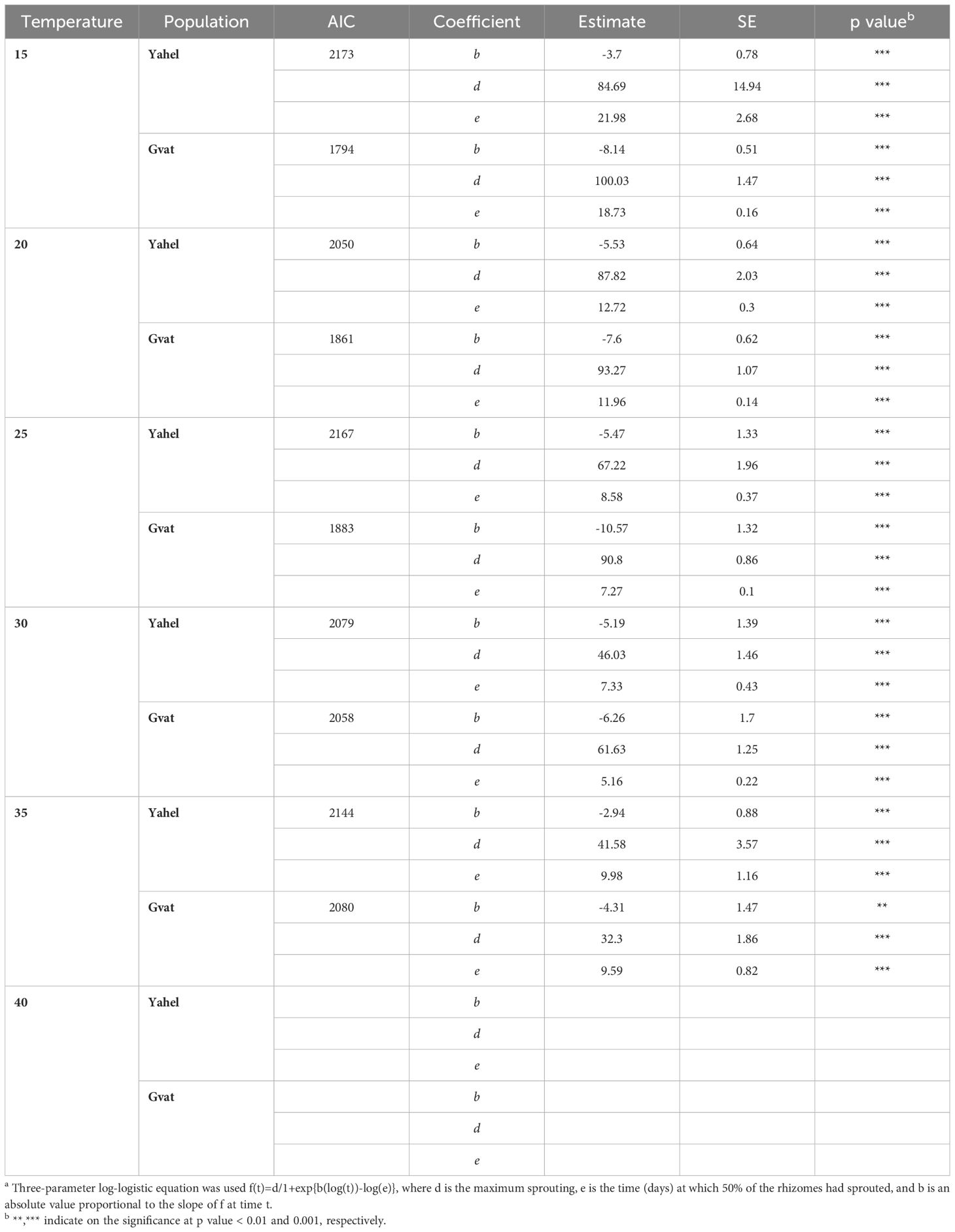
Table 2. Coefficients, their standard errors and p values describing the relationship a between C. acutum rhizomes sprouting rate and temperature (C) regimes of two populations collected at Gvat and Yahel.
Phenology of C. acutum
Growth rate
Several phenological parameters were measured during the growing season of C. acutum plants in Neve Ya’ar Research Center and the southern Arava R&D. The growth rate of plants from the two populations was similar at both sites. The plants elongated at an increasing rate, to the point where it was no longer possible to perform the measurements, in the fifth week of the experiment. We have assessed the differences in the relative elongation rate between populations during the first five weeks of the experiment. At the Arava site, by the fourth week, plants of Yahel population elongated significantly faster than those of Gvat population (Figure 3A). At the Neve Ya’ar site, plants from Gvat population elongated significantly faster in the second and third weeks of the experiment and maintained their advantage until the fifth week of the experiment (Figure 3B). The average plant length at the fifth week of the experiment of plants grown in the southern Arava R&D was 52.84 and 63.75, for Gvat and Yahel populations, respectively (p=0.009). At the same time, the average length of plants grown in Neve Ya’ar Research Center was 59.76 and 47.23, for Gvat and Yahel populations, respectively (p=0.005) (data not shown).
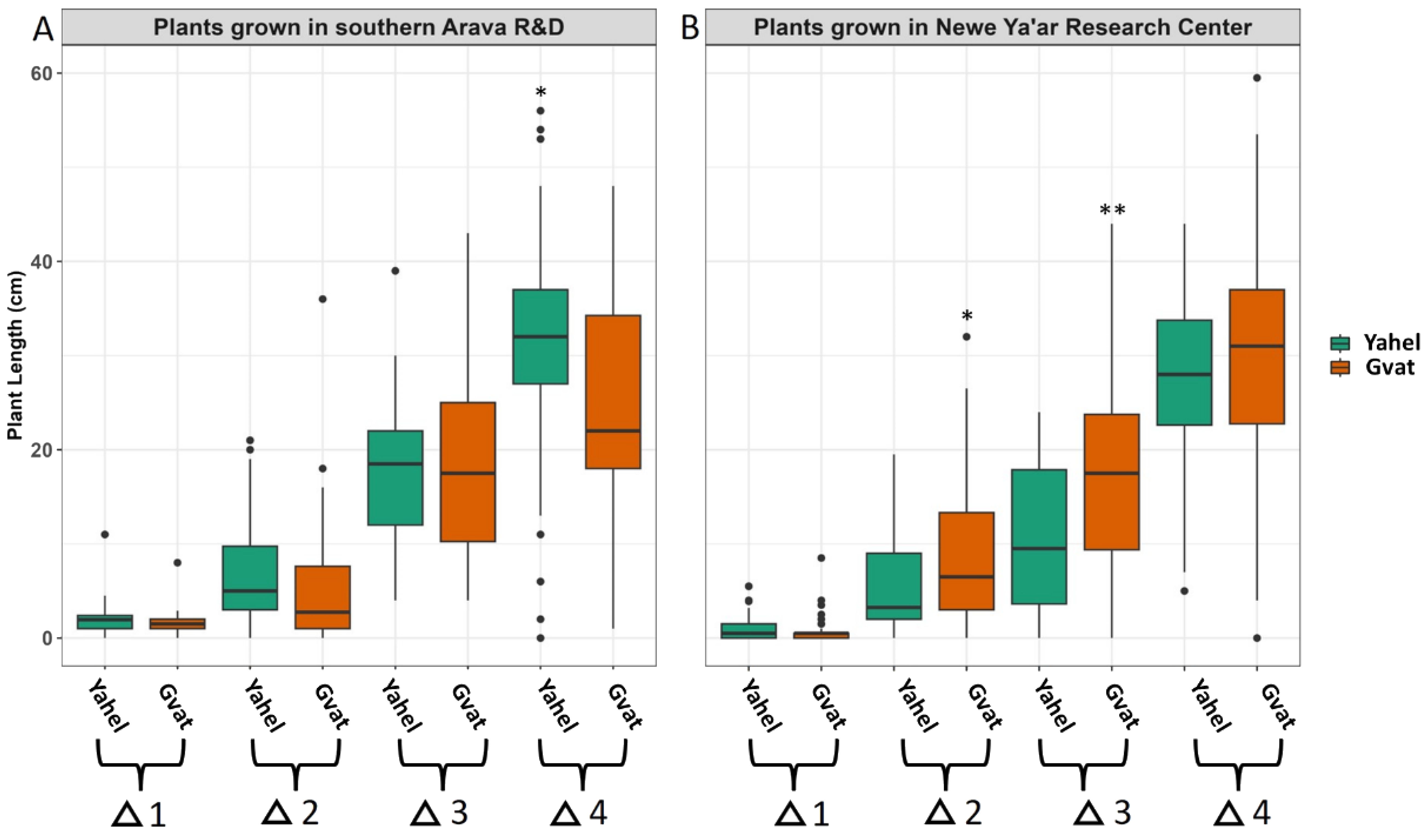
Figure 3. Differences in the relative elongation of plants generated from rhizomes of two C. acutum populations collected in Gvat and Yahel and grown in two locations, Arava R&D (A) and Newe Ya’ar Research Center (B). Data were recorded for the first five weeks after rhizome emergence and analyzed using t t-test (α = 0.05), *,** indicate on the significance at p value > 0.05 and 0.01, respectively (n=50).
Flowering
In addition to elongation, timing, and rate of flowering were recorded throughout the growing season. For both populations, plants grown in the Arava site reached the flowering growth stage faster than plants grown in the Neve Ya’ar site (Figure 4). The difference between sites for the day for flowering initiation was in favor of plants grown in the Arava site. The lag phase (z) where flowering initiated was recorded at the Arava site was 51.07 and 62.97 days (Table 3), for Gvat and Yahel populations, respectively. At the Neve Ya’ar site, z was recorded at 75.68 and 91.03 days for Gvat and Yahel populations, respectively. For plants grown in the Arava site, the final number of flowering plants (M) was similar comparing both Arava and Gvat populations, ~47 (Table 3). For plants grown at the Newe Ya’ar site, the final number of flowering plants was slightly higher for both population, ~49 (Table 3).
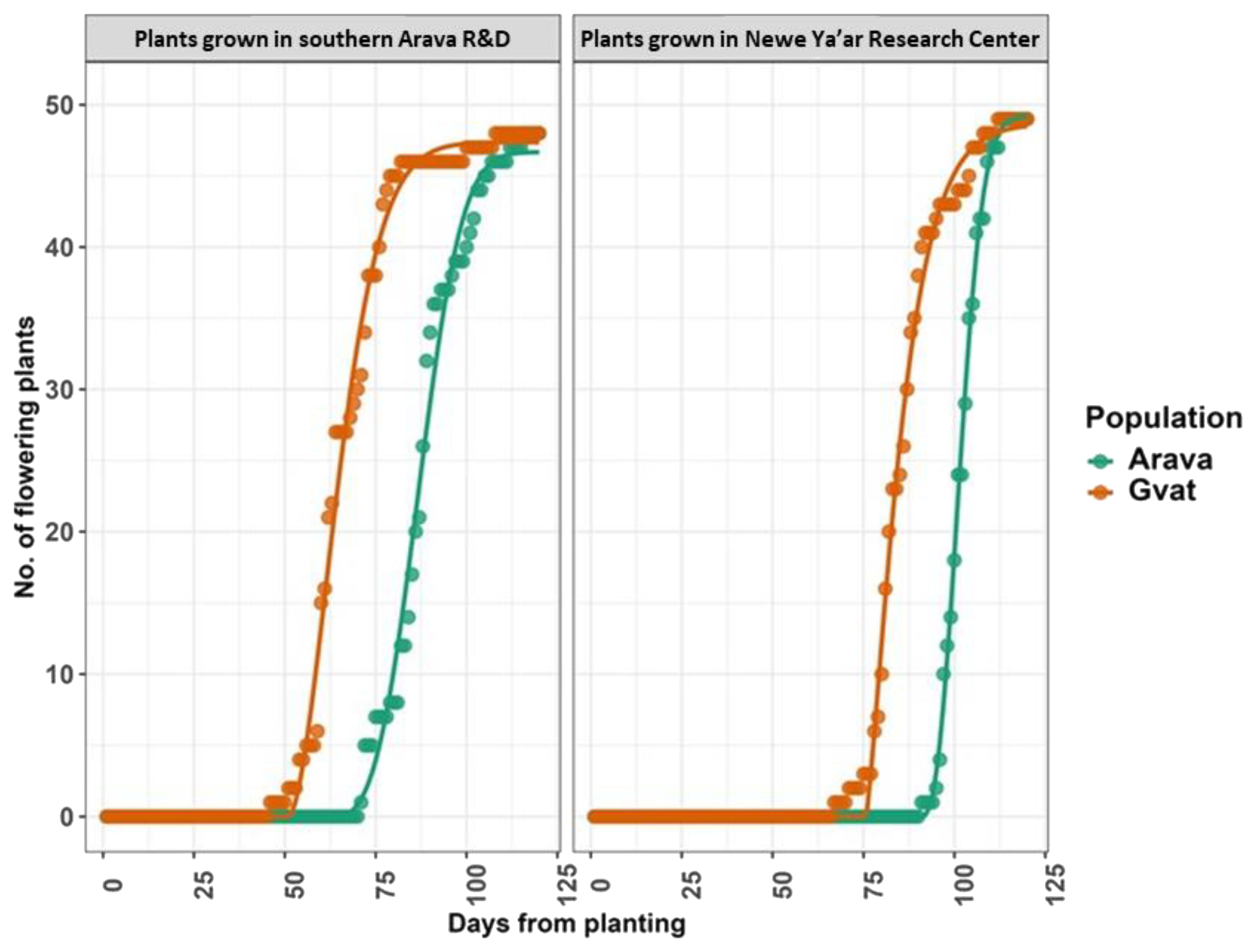
Figure 4. Flowering dynamics as recorded for plants generated from C. acutum rhizomes collected from Gvat (orange line) and Yahel (green line) and grown in two locations, Arava R&D and Newe Ya’ar Research Center. Data was recorded for 120 days (n=50). Data were analyzed using a four parameters Weibull model with lag phase.
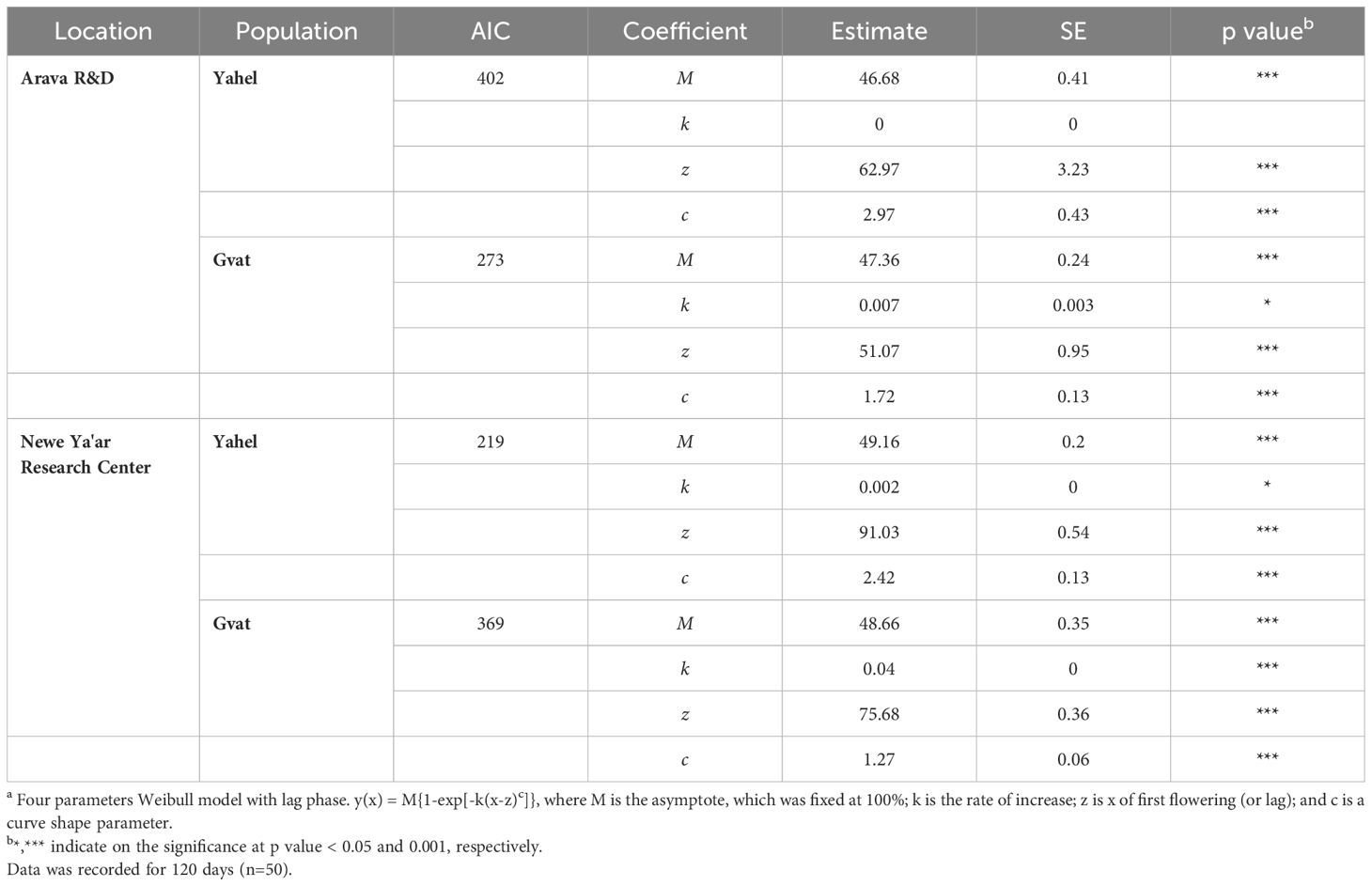
Table 3. Coefficients, their standard errors and p values describing the relationship a between C. acutum number of flowering plants and time (days) of two populations collected from Gvat and Yahel, while experiments were held at Newe Ya'ar Research Center and the Arava R&D.
Shoot and root dry weight were recorded 120 days after rhizome transplanting. Plants grown at the Arava site showed higher dry shoot weight in comparison to those grown at the Newe Ya’ar site, for both Yahel (42.1 vs. 26.3) and Gvat (33.2 vs. 21.4) populations, however, differences in dry root weight were not significant (Table 4). Differences among populations were observed for both shoot and root dry weight in both sites. Plants from Yahel population exhibited statistically significantly greater shoot (42.1 vs. 33.2, p < 0.001) and root (6.4 vs. 5.3, p < 0.003) dry weight compared to plants of the Gvat population when grown at the Arava site. The same trend was observed for plants grown at the Newe Ya’ar site with shoot (28.3 vs. 21.4, p < 0.0025) and root (6.8 vs. 5.8, p < 0.0196) dry weight of plants from the Yahel population outcompeting those of the Gvat population.
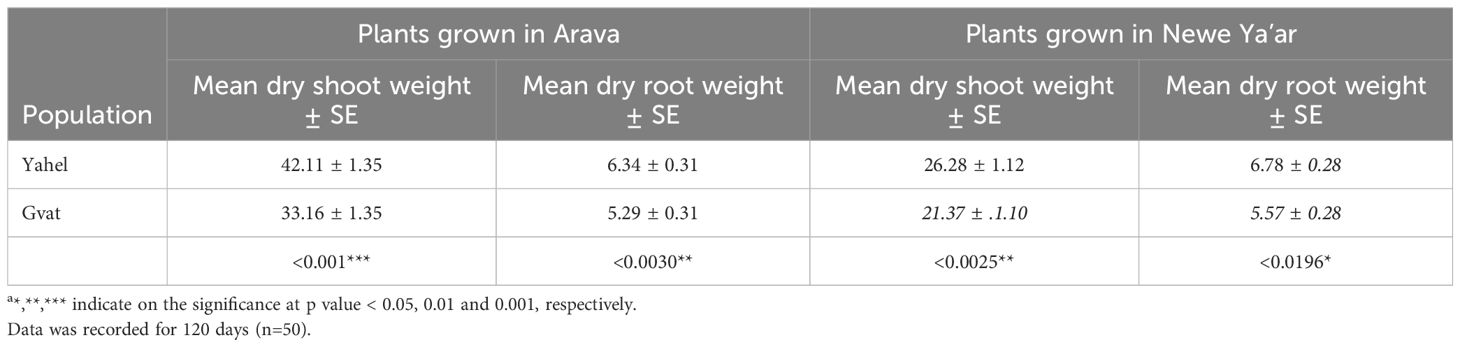
Table 4. Mean shoot and root dry weight (± SE) a indicate on the significance at p value < 0.05 and 0.001, respectively as recorded for plants generated from C. acutum rhizomes collected from Gvat and Yahel and grown in two locations, Arava R&D and Newe Ya’ar Research Center.
Herbicide efficacy-net-house trials
Pre-emergence herbicide experiments
For the pre-emergence herbicide treatments, the interaction between treatment and year was not observed (Supplementary Table S1), thus, the results for each year were analyzed and presented separately.
Our results visually demonstrate the efficacy of the tested herbicides on plant development (Figure 5A). Both indaziflam and pyroxasulfone reduced root formation of treated plants (Figure 5B), however, pendimethalin was less effective as not all tested plants responded to the same degree. According to the percentage of emergence, pendimethalin showed higher emergence (93% and 100%) in comparison to indaziflam (10% and 93%) and pyroxasulfone (72% and 70%) (2020 and 2021, respectively; Figure 5B). Differences in shoot development were also observed. The statistical analysis supported the visual observation, and all pre-emergence herbicide treatments significantly reduced shoot fresh weight compared with untreated control plants in both years (Figure 5). However, higher control efficacy was observed in 2020. Treatment with indaziflam excelled as it showed the best efficacy for both years with the lowest relative fresh weight [an average of less than 0.1% relative weight (Figure 5B), for 2020 and 2021 respectively]. Treatments with pendimethalin and pyroxasulfone were less efficient but maintained high activity in comparison to the untreated control treatment with an almost 80% reduction in shoot biomass. The percentage of emergence for indaziflam for 2020 and 2021 were 10% and 70% (respectively), while for pendimethalin it was over 90% for both years.
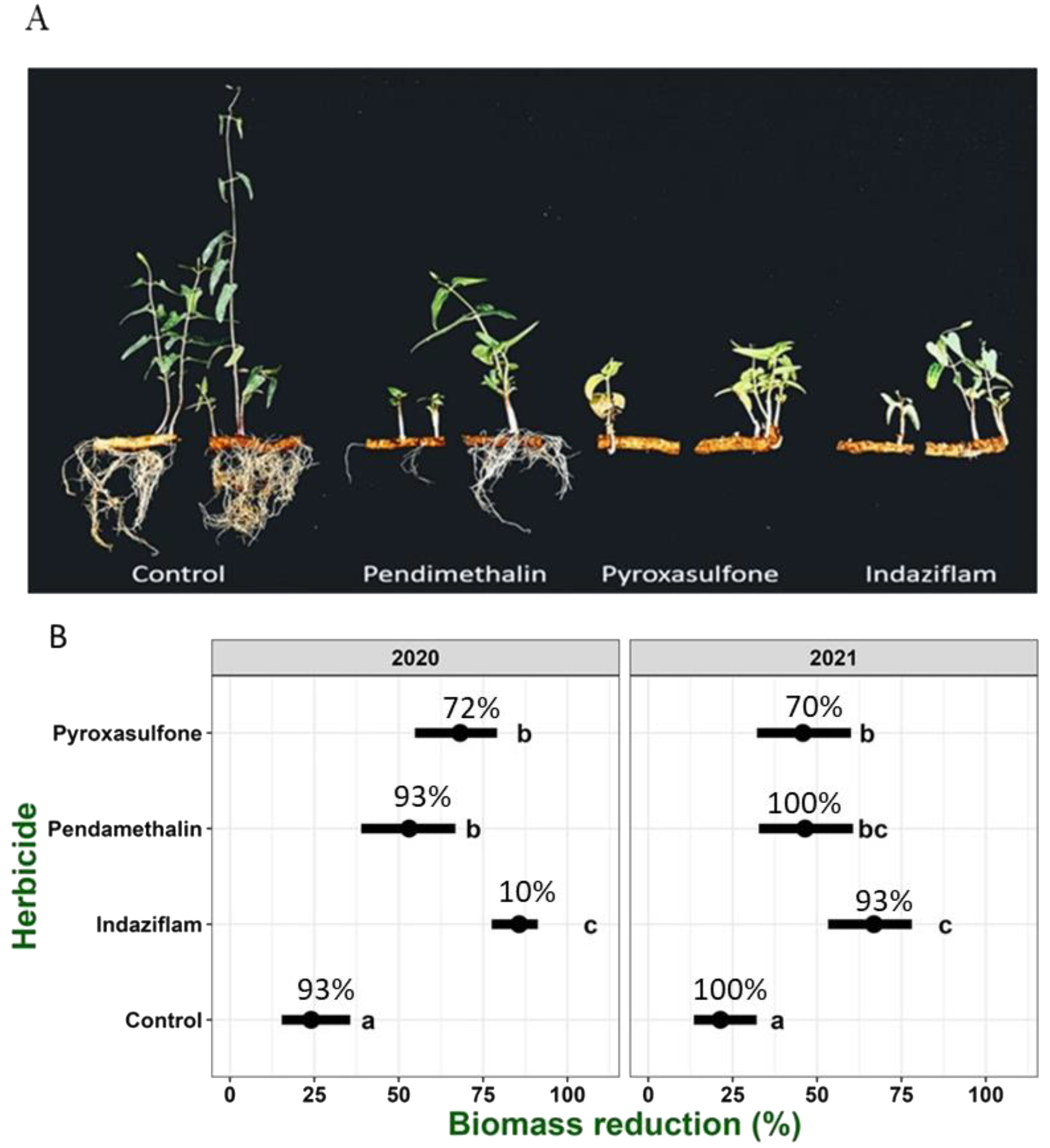
Figure 5. Biomass reduction and visual description of C. acutum plants following pre-emergence herbicides treatments. Visual description of the shoots and roots in treated plants (2021 experiment) (A). Relative biomass reduction following pre-emergence treatments, percentage of emergence for each treatment is shown for each bar (B). Herbicide application rates: pendimethalin– 1.8kg h-1, pyroxasulfone– 128g h-1 and indaziflam– 83g h-1. Experiments were conducted in the summer of 2020 and 2021. Data were analyzed using a generalized linear mixed model (GLMM), with two-way interaction between applied herbicide and year. Replicate were considered as a random effect. The black bar range represent the 95% confidence intervals. Treatments with the same letters did not differ according to Tukey’s honestly significant difference test at α = 0.05 (n=5).
Post-emergence herbicide experiments
Statistical analysis showed no significant differences comparing the two experimental runs; thus, data sets were pooled together (Supplementary Tables S2). All post-emergence treatments showed high efficacy and significantly reduced shoot fresh weight of C. acutum plants at both growth stages (Figure 6). However, an interaction between growth stage and treatment was observed (Supplementary Table S2), and fluroxypyr + surfactant was more effective on young plants compared to the mature ones.
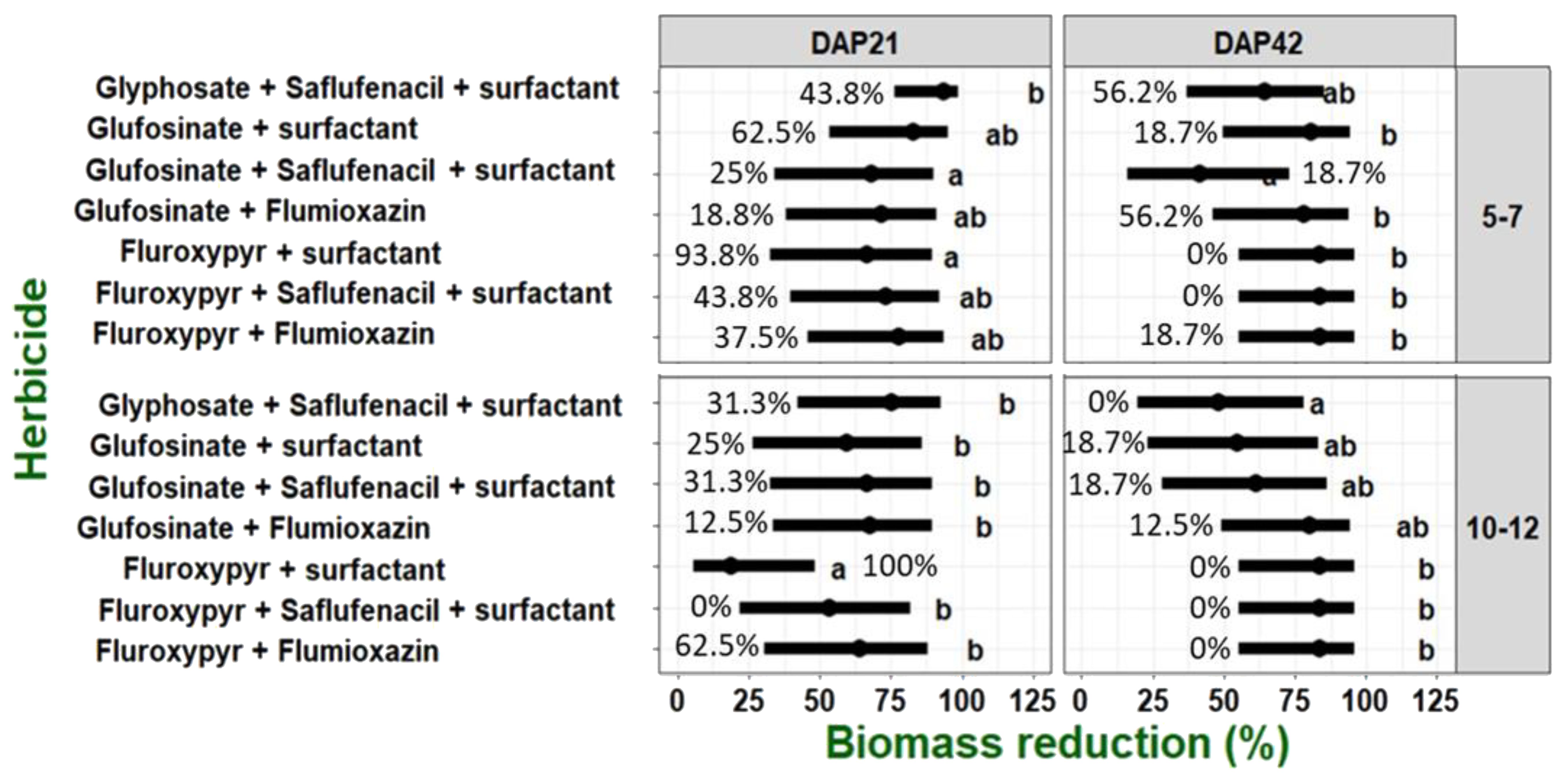
Figure 6. Relative biomass reduction of C. acutum plants following post-emergence treatments at growth stages of 5-7 and 10-12 leaf. Data were analyzed using a generalized linear mixed model (GLMM), with the three-way interaction between applied herbicide, phenology stage and time after treatment (21 and 42 DAP). Replicate were considered as a random effect. The black line range represent the 95% confidence intervals, percentage of emergence for each treatment is shown for each bar. Treatments with the same letters did not differ according to Tukey’s honestly significant difference test at α = 0.05 (n=16).
Treatments including flumioxazin (fluroxypyr + flumioxazin and glufosinate + flumioxazin) showed high efficacy in reducing plant shoot fresh weight; fluroxypyr + surfactant showed the lowest efficacy in reducing relative shoot fresh weight of C. acutum plants (Figure 6). However, this trend was overturned at 42 DAT, when no regeneration was observed for plants treated with the combination of fluroxypyr + surfactant (Figure 6). Furthermore, all treatments including fluroxypyr excelled at the 10-12 leaves growth stage with no regeneration abilities observed (Figure 6). For the 5-7 leaves growth stage, more than 50% regeneration was observed for treatment with glyphosate + saflufenacil + surfactant and glufosinate + flumioxazin. For both growth stages, all treatments with glufosinate had shown over 12% regeneration of C. acutum plants 42 DAT.
Discussion
The populations that were investigated in this study originated from two very distinctive climatic regions in Israel. While C. acutum is considered a native species in the northern part of the country, its presence in the southern Arava is alarming (Figure 1). Northern populations may be found in orchards, vineyards, as well as field margins, where water availability is scarce. However, in the Arava, plants are found only in irrigated private gardens and orchards, close to the irrigation system. The fact that C. acutum originates in tropical areas (Shu, 1995), may explain its vast distribution through the Arava date orchards, where both temperatures and water availability are high. As an invasive weed species, C. acutum may be found in several countries in the Mediterranean and Middle East regions such as Spain, Iraq etc. In Egypt, which borders the Arava region, this species was identified and categorized as an invasive weed (Ellmouni, 2019).
Phenological differences among populations of the same species may suggest different evolutionary processes under various environmental conditions (Mesgaran et al., 2021; Malka et al., 2023). Our data demonstrate the high plasticity of this species, and how populations of the same species grown in different habitats may have adapted to different environmental conditions. Rhizomes collected at the northern region (Gvat), originated in a temperate climate and had higher emergence rates at 15-30°C. For 35°C, the opposite trend was observed, and rhizomes collected at the southern region, hyper-arid desert, (Yahel) had a higher emergence rate (Table 2, Figure 2). Examining the changes in flowering time (Table 3, Figure 4), plants of Gvat population flowered first at both sites, however, flowering initiation was ~20 days earlier in average when plants of both populations were grown at the Arava site. Flowering initiation and plant development may be accelerated by high temperatures, as evident by climate change in recent decades (Preston and Fjellheim, 2022). When radiation is not restricted, high temperatures may induce photosynthetic assimilation and sugar production. Thus, hastening the transfer from the vegetative to the reproductive plant stage, resulting in faster flowering initiation.
At the end of the phenological experiment, the mean dry shoot and root weight of plants originating from rhizomes collected at the southern site (Yahel), was significantly higher than that of plants grown from rhizomes and collected at the northern site (Gvat), for both sites (Table 4). Invasive species may undergo phenotypic changes after being introduced into a new environment (Flores-Moreno et al., 2015). Caño et al. (2008) have investigated the fitness and plasticity of invasive (Spain) and native (South African) populations of Senecio pterophorus. Researchers have found that the invasive populations had higher biomass in different habitats but mainly when plants were grown under a disturbed environment. Leger and Rice (2003) showed that invasive Eschscholzia californica grows larger than native individuals under reduced competition. According to our results and others, we may assume that an adaptive shift in traits has occurred in the invasive population, enabling these plants to better maximize growth and reproduction characteristics.
Differences among populations may also be explained by their habitat adaptation. Rhizomes of Yahel were collected in a palm orchard and those of Gvat in an olive grove. We assume that to survive the competition with palm trees, which grow fast and tall, plants of the Yahel populations should possess high competitive abilities such as; fast growth, large leaf area surface, and vast biomass accumulation. In comparison, C. acutum plants grown in an olive grove, where more sunlight reaches the soil surface, and resource competition is low, may succeed even if possessing low competitive abilities. High competitive abilities of invasive species may be related to increased vigor and reduced herbivory in introduced plant populations (Bossdorf et al., 2005; Leishman et al., 2014). Beest et al. (2015) found that the invasive species Chromolaena odorata has a higher specific leaf area and leaf area index than native species. As a result, C. odorata grows faster, resulting in more biomass, and higher competitive abilities. This is also the case for Lespedeza cuneate as documented by Allred et al. (2010).
The invasion rate of a species to new environments is likely enhanced when both reproductive systems – seeds and rhizomes – are capable of producing viable propagules. This dual capacity for propagation increases the likelihood of successful establishment and spread under various environmental conditions. Seeds are used for long-distance distribution while rhizomes may extend dispersal within the introduction site. However, in cases where interference occurs, rhizomes may also serve as long-distribution agents. In agricultural habitats, human activities such as mowing, digging, cultivation, and more, may serve as a vector for long-distance distribution of invasive species. Rhizomes may be transferred via agricultural machinery between fields in the same region, and even to distant areas. After three years of research, we have learned through our observations in the Arava region, that the propagation of date tillers and using an excavator for niche irrigation in the orchards, may have played a key role in the distribution of C. acutum in the region. New patches have appeared in non-infested orchards around specific date trees only after excavation activities were performed to maintain the irrigation channel structure.
Cynanchum acutum is considered a common pest across the Mediterranean region and in the Northern African countries (Gilbert et al., 1995; Ollerton and Liede, 1997), however, studies exploring management practices for this weed are scarce. In our research, we tested both indaziflam and pyroxasulfone applied as pre-emergence herbicide treatment. We found that both herbicides inhibited root formation, shoot emergence, and impaired the development of rhizome-originated plants (Figure 5). A study that was conducted using another Cynanchum species, C. leave, suggested that pre-emergence application of either clomazone or trifluralin were both efficient for the control of this weed (Masabni et al., 2013). The use of pre-emergence herbicide application in orchards is not common; however, this may be developed in the future as part of an integrated weed management approach.
Post-emergence application of herbicides such as glyphosate and auxin inhibitors (fluroxypyr and aminopyralid) were reported as highly effective for the control of Cynanchum species in several studies (DiTommaso et al., 2013; Meighani et al., 2021). In our study, combinations of herbicides have been shown to be highly effective in preventing the regeneration of C. acutum plants (Figure 6). Treatments including the herbicide fluroxypyr, as well as the treatment of glyphosate + saflufenacil + surfactant were the most effective in controlling C. acutum plants. For the glyphosate + saflufenacil + surfactant and fluroxypyr + flumioxazin treatment, higher efficacy was emphasized as less regenerative abilities were achieved when plants were sprayed at the mature growth stage of 10-12 leaves. In most herbicide efficacy studies and on herbicide product labels, farmers are advised to apply herbicides at the early growth stage, as weeds are more herbicide-sensitive at this growth stage (Lee and Oliver, 1982; Chauhan and Abugho, 2012). However, herbicide application in a later growth stage was found to be more effective for the control of perennial weed species such as Solanum elaeagnifolium and Ambrosia grayi (Currie et al., 2000; Wu et al., 2016). Other reasons for low herbicide efficacy (Lee and Oliver, 1982) may be related to reduced herbicide penetration or decreased translocation through the plant to the active site. Differences in herbicide penetration may be induced by foliar surface features, such as trichomes, cuticle, and stomata wax coating (Kraehmer et al., 2014; Abu-Nassar et al., 2022), thus reducing general herbicide efficacy. Herbicide translocation is governed by a source-to-sink pattern, especially for phloem-mobile herbicides such as glyphosate and fluroxypyr (Shaner, 2009). Differences in leaf surface features or source-to-sink patterns may be the reason for the observed differences in herbicide efficacy among growth stages; however, further research is needed to clarify these issues.
In conclusion, the biological insights gained in this study, in terms of C. acutum sprouting and flowering dynamics, can facilitate optimal decision-making regarding control activities. Future research should aim at a deeper understanding of the weed’s ecology, especially targeting the spread through seeds vs. the spread through rhizomes and the impact of agricultural practice on these processes. Revealing flowering and sprouting dynamics enables control activity to be targeted to the time when most of the population has sprouted and prevent early or late herbicide applications. Further knowledge about the ecology of the weed will improve this targeted control and help to create integrated weed management protocols. Non-chemical means, such as mowing and hand-weeding, can be integrated into such protocols, target pre-flowering timings, and reduce the seed-based dispersal of this species into new areas.
Data availability statement
The raw data supporting the conclusions of this article will be made available by the authors, without undue reservation.
Author contributions
MM: Conceptualization, Funding acquisition, Methodology, Project administration, Supervision, Writing – original draft, Writing – review & editing. OB: Data curation, Formal analysis, Investigation, Methodology, Visualization, Writing – original draft, Writing – review & editing. RL: Funding acquisition, Investigation, Methodology, Supervision, Writing – original draft, Writing – review & editing. JS: Conceptualization, Data curation, Funding acquisition, Investigation, Methodology, Project administration, Supervision, Writing – original draft, Writing – review & editing. OK: Formal analysis, Visualization, Writing – review & editing. MS: Conceptualization, Funding acquisition, Methodology, Writing – review & editing. AG: Investigation, Supervision, Writing – review & editing.
Funding
The author(s) declare financial support was received for the research, authorship, and/or publication of this article. The authors wish to thank The Ministry of Agriculture and Keren Kayemeth L’Israel (KKL) for funding this project (Project number 94-008-20).
Acknowledgments
The authors thank Jackline Abu-Nassar and Guy Achdari for their valuable contribution. Thank you to the date farmers and kibbutzim of the southern Arava for cooperating with data collection and information sharing. Special thanks to Kibbutz Ketura for devoting part of their plantations to our field experiments.
Conflict of interest
The authors declare that the research was conducted in the absence of any commercial or financial relationships that could be construed as a potential conflict of interest.
Publisher’s note
All claims expressed in this article are solely those of the authors and do not necessarily represent those of their affiliated organizations, or those of the publisher, the editors and the reviewers. Any product that may be evaluated in this article, or claim that may be made by its manufacturer, is not guaranteed or endorsed by the publisher.
Supplementary material
The Supplementary Material for this article can be found online at: https://www.frontiersin.org/articles/10.3389/fagro.2024.1448556/full#supplementary-material
References
Abu-Nassar J., Gal S., Shtein I., Distelfeld A., Matzrafi M. (2022). Functional leaf anatomy of the invasive weed Solanum rostratum Dunal. Weed. Res. 62, 172–180. doi: 10.1111/wre.12527
Allred B. W., Fuhlendorf S. D., Monaco T. A., Will R. E. (2010). Morphological and physiological traits in the success of the invasive plant Lespedeza cuneata. Biol. Invasions. 12, 739–749. doi: 10.1007/s10530-009-9476-6
Bajwa A. A., Zhu X., Chauhan B. S., Adkins S.W., Weston L.A. (2015). “Screening of gene regions for genetic diversity in global parthenium weed (Parthenium hysterophorus L.) populations,” in Proceedings of the 21st Australasian Weeds Conference, Sydney, NSW, Australia. 318–321.
Beest M., Esler K. J., Richardson D. M. (2015). Linking functional traits to impacts of invasive plant species: a case study. Plant Ecol. 216, 293–305. doi: 10.1007/s11258-014-0437-5
Bossdorf O., Auge H., Lafuma L., Rogers W. E., Siemann E., Prati D. (2005). Phenotypic and genetic differentiation between native and introduced plant populations. Oecologia 144, 1–11. doi: 10.1007/s00442-005-0070-z
Brooks M. E., Kristensen K., Van Bethem K. J., Magnusson A., Berg C. W., Nielsen A., et al. (2017). glmmTMB balances speed and flexibility among packages for zero-inflated generalized linear mixed modeling. R. J. 9, 378–400. doi: 10.32614/RJ-2017-066
Brown R. F., Mayer D. G. (1988). Representing cumulative germination. 2. The use of the Weibull function and other empirically derived curves. Ann. Bot. 61, 127–138. doi: 10.1093/oxfordjournals.aob.a087535
Caño L., Escarré J., Fleck I., Blanco-Moreno J. M., Sans F. X. (2008). Increased fitness and plasticity of an invasive species in its introduced range: A study using Senecio pterophorus. J. Ecol. 96, 468–476. doi: 10.1111/j.1365-2745.2008.01363.x
Chauhan B. S., Abugho S. B. (2012). Effect of growth stage on the efficacy of postemergence herbicides on four weed species of direct-seeded rice. Sci. World J. 2012, 123071. doi: 10.1100/2012/123071
Cohen S., Kern J., Harpaz I., Ben-Joseph R. (1988). Epidemiological studies of the tomato yellow leaf curl virus (TYLCV) in the Jordan Valley, Israel. Phytoparasitica 16, 259. doi: 10.1007/BF02979527
Currie R. S., Thompson C. R., Technology S. W., Mar J., Mar N. J. (2000). Effects of herbicides and application timing on woollyleaf bursage (Ambrosia grayi). Weed. Sci. 14, 188–190. doi: 10.1614/0890-037X(2000)014[0188:EOHAAT]2.0.CO;2
Daehler C. C. (2003). Performance comparisons of co-occurring native and alien invasive plants: implications for conservation and restoration. Annu. Rev. Ecol. Evol. Syst. 34, 183–211.
DiTommaso A., Lawlor F. M., Darbyshire S. J. (2005). The biology of invasive alien plants in Canada. 2. Cynanchum rossicum (Kleopow) borhidi [= Vincetoxicum rossicum (Kleopow) barbar.] and Cynanchum louiseae (L.) Kartesz & Gandhi [= Vincetoxicum nigrum (L.) Moench. Can. J. Plant Sci. 85, 243–263. doi: 10.4141/P03-056
DiTommaso A., Milbrath L. R., Bittner T., Wesley F. R. (2013). Pale swallowwort (Vincetoxicum rossicum) response to cutting and herbicides. Invasive. Plant Sci. Manage. 6, 381–390. doi: 10.1614/IPSM-D-12-00078.1
El-Katony T. M., Khedr A. H. A. F., Mergeb S. O. (2018). Drought stress affects gas exchange and uptake and partitioning of minerals in swallowwort (Cynanchum acutum L.). Rendiconti. Lincei. Sci. FISICHE. E. NATURALI. 29, 23–34. doi: 10.1007/s12210-017-0654-7
Ellmouni F. (2019). Geometric morphometrics of leaves of Cynanchum acutum L. (Apocynaceae) from Egypt. Taeckholmia 39, 86–102. doi: 10.21608/taec.2019.19141.1009
Flores-Moreno H., García-Treviño E. S., Letten A. D., Moles A. T. (2015). In the beginning: phenotypic change in three invasive species through their first two centuries since introduction. Biol. Invasions. 17, 1215–1225. doi: 10.1007/s10530-014-0789-8
Gilbert M. G., Stevens W. D., Ping-tao L. (1995). Notes on the asclepiadaceae of China. Novon 5, 1–16. doi: 10.2307/3391820
Golzardi F., Vaziritabar Y., Vaziritabar Y., Falah Tafti S., Sadat Asilan K., Jafari Sayadi M. H., et al. (2014). Investigation of drying effect on developing growth of two Cynanchum acutum L. populations. Int. J. Adv. Life Sci. 7, 680–685.
Golzardi F., Vaziritabar Y., Vaziritabar Y., Falah Tafti S., Sarvaramini S. (2015). Freezing effect on developing growth of two Cynanchum acutum L. populations. J. Biodivers. Environ. Sci. 6, 172–178.
Grundy A. C., Peters N. C. B., Rasmussen I. A., Sattin M., Andersson L., Mead A., et al. (2003). Emergence of Chenopodium album and Stellaria media of different origins under different climatic conditions. Weed. Res. 43, 163–176. doi: 10.1046/j.1365-3180.2003.00330.x
Kraehmer H., Laber B., Rosinger C., Schulz A. (2014). Herbicides as weed control agents: state of the art: I. weed control research and safener technology: the path to modern agriculture. Plant Physiol. 166, 1119–1131. doi: 10.1104/pp.114.241901
Lee S. D., Oliver L. R. (1982). Efficacy of acifluorfen on broadleaf weeds times and methods for application. Weed. Sci. 30, 520–526. doi: 10.1017/S0043174500041096
Leger E. A., Rice K. J. (2003). Invasive California poppies (Eschscholzia californica Cham.) grow larger than native individuals under reduced competition. Ecol. Lett. 6, 257–264. doi: 10.1046/j.1461-0248.2003.00423.x
Leishman M. R., Cooke J., Richardson D. M. (2014). Evidence for shifts to faster growth strategies in the new ranges of invasive alien plants. J. Ecol. 102, 1451–1461. doi: 10.1111/1365-2745.12318
Lenth R. (2020). emmeans: estimated marginal means, aka least-square means. R package version 1.5.4. Available online at: https://CRAN.R-project.org/package=emmeans (Accessed Aug 5, 2024).
Malka S., Eizenberg H., Matzrafi M. (2023). Variation in seed properties and germination capabilities among populations of the invasive weed Parthenium hysterophorus L. (Asteraceae). Front. Plant Sci. 27, 1222366. doi: 10.3389/fpls.2023.1222366
Masabni J. G., Walters S. A., Young B. G., Coolong T. (2013). Honeyvine milkweed (Cynanchum laeve) control in plasticulture bell pepper production. Weed. Technol. 27, 671–674. doi: 10.1614/WT-D-12-00163.1
Meighani F., Karaminejad M. R., Farrokhi Z. (2021). Invasive weed swallow-wort (Cynanchum acutum L.) response to chemical and mechanical practices. Weed. Biol. Manage. 21, 124–132. doi: 10.1111/wbm.12231
Mesgaran M. B., Matzrafi M., Ohadi S. (2021). Sex dimorphism in dioecious Palmer amaranth (Amaranthus palmeri) in response to water stress. Planta 254, 17. doi: 10.1007/s00425-021-03664-7
Ollerton J., Liede S. (1997). Pollination systems in the Asclepiadaceae: A survey and preliminary analysis. Biol. J. Linn. Soc. 62, 593–610. doi: 10.1111/bij.1997.62.issue-4
Pahlevani A. H., Rashed M. H., Ghorbani R. (2008). Effects of environmental factors on germination and emergence of swallowwort. Weed. Technol. 22, 303–308. doi: 10.1614/WT-07-055.1
Preston J. C., Fjellheim S. (2022). Flowering time runs hot and cold. Plant Physiol. 190, 5–18. doi: 10.1093/plphys/kiac111
R Core Team (2023). R: A language and environment for statistical computing (Vienna, Austria: R Foundation for Statistical Computing). Available online at: https://www.R-project.org/ (Accessed Aug 5, 2024).
Shaner D. L. (2009). Role of translocation as a mechanism of resistance to glyphosate. Weed. Sci. 57, 118–123. doi: 10.1614/WS-08-050.1
Keywords: adaptation, invasive plant species, rhizome, weed biology, weed management
Citation: Bar O, Lati RN, Schäckermann J, Kapiluto O, Spodek M, Gamliel A and Matzrafi M (2024) Biology and chemical weed management of Cynanchum acutum L.. Front. Agron. 6:1448556. doi: 10.3389/fagro.2024.1448556
Received: 13 June 2024; Accepted: 20 August 2024;
Published: 06 September 2024.
Edited by:
Simerjeet Kaur, Punjab Agricultural University, IndiaReviewed by:
Shane Douglas Campbell, The University of Queensland, AustraliaAnnu Kumari, FMC Agricultural Solutions, United States
Copyright © 2024 Bar, Lati, Schäckermann, Kapiluto, Spodek, Gamliel and Matzrafi. This is an open-access article distributed under the terms of the Creative Commons Attribution License (CC BY). The use, distribution or reproduction in other forums is permitted, provided the original author(s) and the copyright owner(s) are credited and that the original publication in this journal is cited, in accordance with accepted academic practice. No use, distribution or reproduction is permitted which does not comply with these terms.
*Correspondence: Maor Matzrafi, bWFvcm1Adm9sY2FuaS5hZ3JpLmdvdi5pbA==