- 1Crop Production and Crop Protection, Institute of Biomass Research, University of Applied Sciences Weihenstephan-Triesdorf, Weidenbach, Germany
- 2Department for Crop Production, Horticulture and Viticulture, Kuratorium für Technik und Bauwesen in der Landwirtschaft e.V. (KTBL), Darmstadt, Germany
- 3Molecular Plant Nutrition, Leibniz Institute of Plant Genetics and Crop Plant Research Gatersleben, Stadt Seeland, Germany
- 4Sächsisches Landesamt für Umwelt, Landwirtschaft und Geologie, Abteilung 7 Landwirtschaft, Nossen, Germany
- 5Institute of Soil Science, Leibniz Universität Hannover, Hannover, Germany
- 6Deutsche Saatveredelung AG, Asendorf, Germany
Introduction: Cover crop services depend on biomass production and species composition of the cover crop stand. In this study, we investigated the interactions in shoot biomass of dual cover crop mixtures and their competitiveness to suppress weeds before winter under different growing conditions.
Methods: A field experiment was performed on sandy loam soil in Triesdorf, Germany, for two consecutive years. The cover crop species white mustard (Sinapis alba L.), oil radish (Raphanus sativus var. oleiformis L.), phacelia (Phacelia tanacetifolia J.), Egyptian clover (Trifolium alexandrinum L.), common vetch (Vicia sativa L.), field pea (Pisum sativum L.), and field bean (Vicia faba L.) were grown in pure stands or dual mixtures. Cover crops were sown in August, and shoot biomass was harvested at the end of the vegetation period. Cover crop shoot biomass was dried, and the share of each species and the sown test weed (Brassica napus L.) were determined.
Results: The share of shoot biomass of a cover crop species in dual-species mixtures was closely related to its shoot biomass in its pure stand. In both years, mustard and phacelia showed similar interactions in shoot biomass production when growing with a second species. Regarding competitiveness against weeds, cruciferous species outperformed other cover crop species and could suppress weeds even if they were mixed with a less competitive partner. Weed suppression of mixtures with phacelia depended on the second component in the mixture and was more effective in a dry season. Legume species, especially Egyptian clover and common vetch could not suppress weeds in a pure stand.
Discussion: Our results show that species with high weed suppression potential as single stands retain this ability in dual cover crop mixtures, regardless of the suppression potential of the second species that completes the mixture.
1 Introduction
Increasing food demand, environmental constraints, and societal expectations necessitate sustainable cropping systems. One solution to these needs is to integrate cover crops into crop rotations, established mainly in periods when no main crop is growing (e.g., summer to winter). They are cultivated primarily for their soil and environmental benefits rather than for income generation. Nevertheless, cover crop adoption in Europe has steadily increased due to agricultural policies (Kathage et al., 2022; Klages et al., 2022; Peltonen-Sainio et al., 2023). However, as of 2023, the Common Agricultural Policy (CAP) reduced the general support of widespread cover cropping. While the Ecological Focus Areas program was previously the main driver of the increase in cover crop area, Good Agricultural and Environmental Conditions (especially GAEC 6, 7 & 8) now only account for a small proportion of cover crop area under average farm conditions (European Commission, 2021). Thus, the decision to grow cover crops depends primarily on national legislation and farmers´ knowledge of their agronomic and environmental benefits (Storr et al., 2019).
The provided ecosystem services range from capturing and storing nutrients to preventing nitrate leaching while increasing nutrient efficiency in crop production systems (Ilgen, 1990; Heuermann et al., 2019; Sieling, 2019; Hallama et al., 2021; Gentsch et al., 2022; Heuermann et al., 2022). They improve soil health by protecting bare soil from erosion, increasing macro-aggregate stability (Gentsch et al., 2023), improving water infiltration, and promoting soil microorganisms (Roarty et al., 2017; Restovich et al., 2019; Gentsch et al., 2020; Ruis et al., 2020; Blanco-Canqui et al., 2022; Koudahe et al., 2022). Furthermore, cover crops are a powerful tool for climate change mitigation, as they are a sufficient tool to reduce the carbon footprint in cropping systems (Schön et al., 2024) when adapted to local growing conditions (Poeplau and Don, 2015; Kaye and Quemada, 2017; Abdalla et al., 2019; Seitz et al., 2022). In addition, the establishment of cover crops, especially in simplified crop rotations, promotes the biodiversity of soil, plant, insect, and even avian communities (Axelsen and Kristensen, 2000; Wilcoxen et al., 2018; Schütz et al., 2020; Fiorini et al., 2022). Choosing the right cover crop is an important management tool to regulate pests and soil-borne plant diseases (Wnuk and Wojciechowicz-zytko, 2010; Patkowska and Konopiński, 2014; Hauer et al., 2016; Baysal-Gurel and Liyanapathiranage, 2019; Wright et al., 2019) and they are used for integrated weed management (Haramoto and Gallandt, 2004; Lemessa and Wakjira, 2014; Schappert et al., 2018; Riemens et al., 2022).
Cover crop species vary in their ability to provide ecosystem services under a given set of climatic conditions (Bodner et al., 2010; Ramirez-Garcia et al., 2015; Tribouillois et al., 2015a, 2015b; Wendling et al., 2016; Wagg et al., 2021). Therefore, increasing the number of species and, thus, the diversity of plant traits through cover crop mixtures is more likely to achieve multiple goals (Finney et al., 2017; Ranaldo et al., 2020; Reiss and Drinkwater, 2020). Identifying suitable plant measures for the targeted ecosystem services is essential for validating cover crop mixtures. The production of shoot biomass is an agronomically relevant measure of the establishment and growth of cover crops (Cottney et al., 2022). In addition to the simple on-farm evaluation of this measure, biomass serves as a source of soil organic matter and provides the potential to suppress weeds and volunteer plants (Lavergne et al., 2021; Kümmerer et al., 2023). Nevertheless, several controversial strategies for optimizing shoot biomass production have been discussed. Strategies range from the best-selected pure stand that produces the highest shoot biomass under the given conditions (Florence and McGuire, 2020) to cover crop mixtures that produce high biomass best adapted to specific climatic conditions (Wendling et al., 2019). However, the general assumption that mixtures produce higher shoot biomass than pure stands is highly dependent on the species used (Elhakeem et al., 2021), so the choice of cover crop species is crucial and requires consideration of environmental conditions and agronomic objectives (Hendrickson et al., 2021).
In field experiments, pure stands that were best adapted to the climatic conditions at the time of selection had the best shoot biomass production in the first few weeks of development but not throughout the growing season. By contrast, cover crop mixtures have a higher potential to limit the risk of crop growth failure under changing weather conditions (Heuermann et al., 2022). For this reason, developing resilient mixtures that produce high biomass yields under different climatic conditions is a significant goal. Although the goals of cover crops are well defined in practice, there currently need to be general rules about species in mixtures, how they affect each other, and the services they provide. Selection for a mixture can be based either on the performance of a species in pure stand to predict its share of shoot biomass in a cover crop mixture (Wortman et al., 2012; Wilson et al., 2019) or on the use of species that regularly dominate biomass in multi-species cover crop mixtures (Baraibar et al., 2020). However, due to their single-species performance, some dual cover crop mixtures reached higher shoot biomass than expected (Wendling et al., 2017). At best, mixtures should be designed to adapt to variable weather conditions and reach over-yielding or transgressive over-yielding (Wendling et al., 2017). This is particularly important for weed suppression (MacLaren et al., 2019; Baraibar et al., 2021), fast ground cover (Brust et al., 2014), high leaf area index, and maximum canopy height (Florence et al., 2019; Smith et al., 2020).
The present study systematically quantified the interaction between commonly used cover crop species, sown in August, in dual mixtures to improve cover crop performance in shoot biomass and weed suppression.
2 Materials and methods
2.1 Location
The field experiment was conducted in Triesdorf in southern Germany and repeated for two years, namely 2016 (49°12’33.04”N 10°39’1.25”E) and 2017 (49°12’9.99”N 10°39’33.60”E). The sites, with a mean annual air temperature of 8.7°C and annual precipitation of 674 mm (2005 - 2015), are characterized by a warm, fully humid climate with warm summer (Kottek et al., 2006). The soils are classified as a Stagnic Cambisol (IUSS Working Group WRB, 2015). The soil texture of the fields is sandy loam.
2.2 Experimental design
Seven cover crop species, namely white mustard (Sinapis alba L.), oil radish (Raphanus sativus var. oleiformis L.), phacelia (Phacelia tanacetifolia J.), Egyptian clover (Trifolium alexandrinum L.), common vetch (Vicia sativa L.), field bean (Vicia faba L.) and field pea (Pisum sativum L.) were established either as pure stands with common seed rates (grains m-2) or in dual mixtures, with adjusted seed rates of 50% of its pure stand (Table 1).
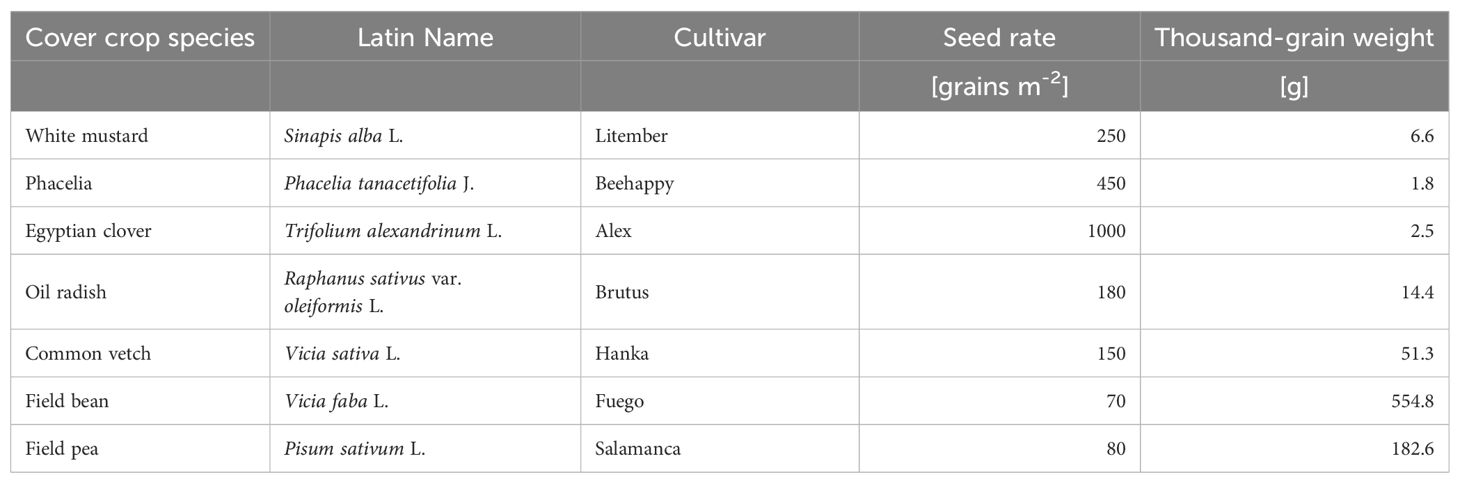
Table 1. Cover crop species, cultivars, seed rates in pure stands, and thousand-grain weight used in the field trials in Triesdorf, Germany.
Field trials were designed in a randomized complete block design with four replicates using 10.5 m × 4.5 m plots. In addition to the cover crop treatments, canola (Brassica napus L.) was used as a test weed (5 seeds m-2) to quantify the competitiveness of the cover crops. The seeds were sown together with the cover crops.
2.3 Growing conditions and crop management
Winter barley (Hordeum vulgare L.) was the preceding crop in the field trials in both years, harvested on July 18, 2016, or July 17, 2017. After fertilizing with biogas manure (80 kg N ha-1 in 2016 and 60 kg N ha-1 in 2017 adjusted to Nmin levels which are the calcium chloride extractable mineral N compounds (NO3- & NH4+) (Amelung et al., 2018), a three-bar tine cultivator was used for a 20 cm deep tillage, followed by seedbed preparation using a PTO harrow, and cover crops were sown on August 19, 2016, and August 17, 2017. All plots were sown with a field trial seed drill (Plotseed S, Wintersteiger, Ried, Austria) with a row spacing of 13.6 cm in a seeding depth of 2.0 cm. For quantification of shoot biomass, cover crops were harvested 89 days after sowing on November 15, 2016, and 85 days after sowing on November 9, 2017, at the end of the vegetation period using a field trial green forage harvester (Hege 212, Hege Saatzuchtmaschinen, Hohebuch, Germany).
Growing conditions for cover crop establishment were very different for the two seasons. The 2016 season was characterized by dry to moderate weather conditions, with total precipitation of 33 mm in July and 38 mm in August. Despite this low total precipitation before the experiment was started, rainfall events were reported in 2016 after sowing in a two-week pattern in September and October (Figure 1A). The monthly average temperature was July 18.7°C, August 17.8°C, September 16.1°C, and October 7.5°C. The 2017 cover crop growing season was characterized by wet weather conditions with total precipitation of 128 mm in July, 66 mm in August, 55 mm in September, and 63 mm in October. The average monthly temperature was 18.1°C in July, 17.9°C in August, 11.7°C in September, and 10.0°C in October (Figure 1B).
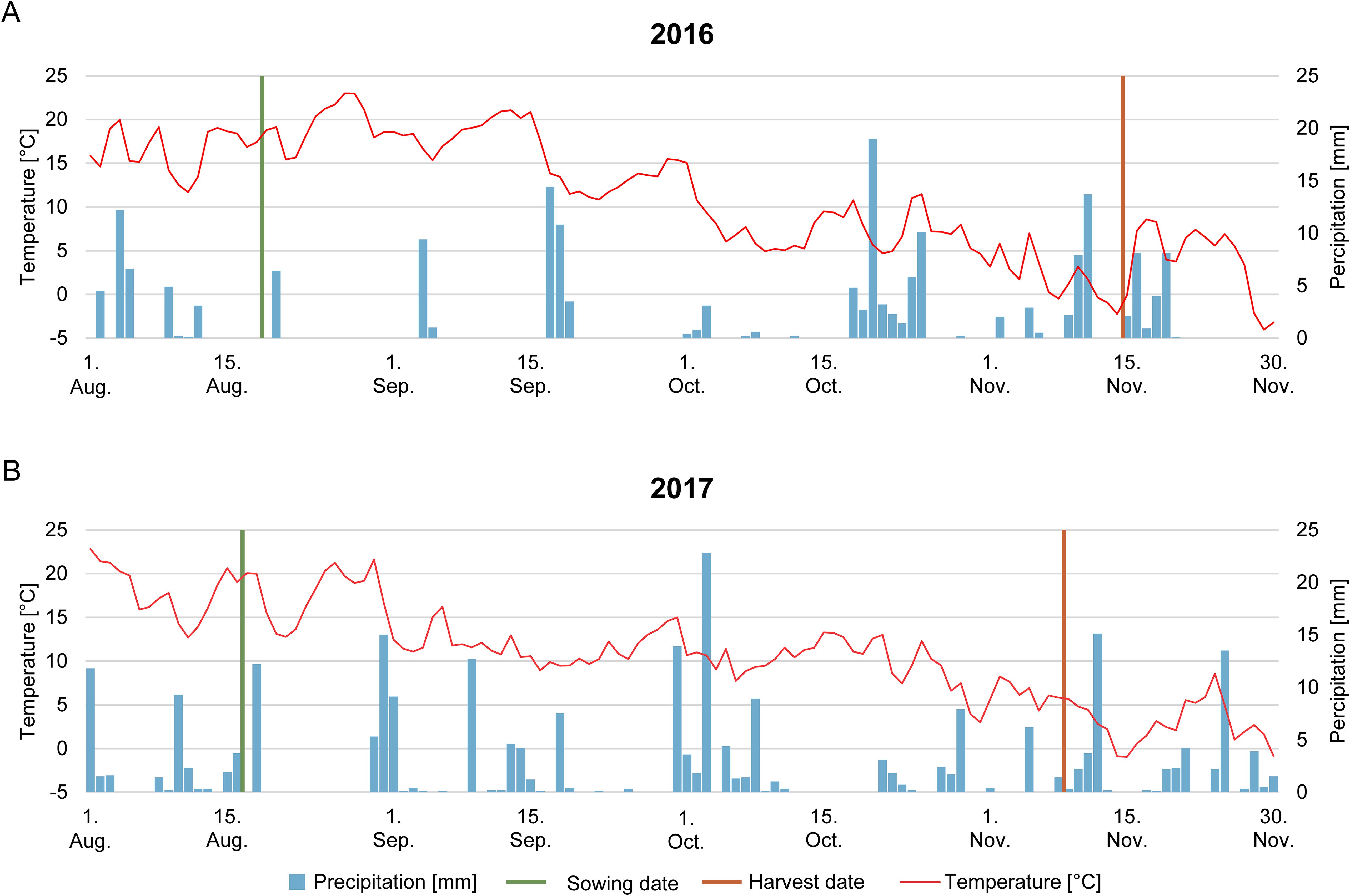
Figure 1. Growing conditions during the cover crop vegetation period [mean daily air temperature [°C] (red line), daily precipitation [mm] (blue bars)]. A green line indicates the time point of sowing, and a red line indicates the harvest in 2016 (A) and 2017 (B).
2.4 Plant sampling
According to the BBCH code, cover crop development was monitored until the end of the vegetation period (Meier, 2018). After field emergence, the stand density of all species was determined by counting and distinguishing the plant species in the four replicates on September 9, 2016, and September 6, 2017. Stand density was counted in each of the four replicates in two seeding rows, each for a 1-meter distance (covering 2,2% of the core plot) and converted into stand density per m² using the row distance of the seeder.
All above-ground biomass (fresh weight) was quantified by harvesting cover crop shoots from 11.25 m-2 in all four repetitions at a cutting height of 5.0 cm from each plot and weighed using a field trial green forage harvester with an integrated weighing system (Hege 212, Hege Saatzuchtmaschinen, Hohebuch, Germany). The harvester continuously collected a sample of 300 g of chopped fresh material from each plot to determine the total dry matter content after drying at 105°C until constant weight to calculate the dry matter yield based on the fresh matter yield. In addition, a second sub-sample of 4000 - 5000 g of above-ground biomass was collected from each plot to fractionate into the different plant species to determine their portion in the fresh matter shoot biomass. Subsequently, up to 300 g per species were dried at 60°C until constant weight to determine species-specific dry matter content, and the material was kept for further analysis. Finally, the dry matter yield of all species was calculated.
2.5 Analysis of interactions and competitiveness between cover crop species
Shoot biomass in mixtures was compared to the shoot biomass yields of pure stands of each species, which were used to analyze interactions in dual mixtures (De Wit, 1960). A graph was created to show the interspecific interactions in shoot biomass to visualize the effect of dual mixtures compared to single species. The measurements of the pure stands are shown as columns on the outer left and right sides of the graph (Figures 2A, B). The measurements of the shoot biomass in the dual mixtures of the two sown species at a seeding rate of 50% of the species´ pure stand are shown in between as two individual columns. While the bar on the middle left side shows the estimated shoot biomass based on half of the shoot biomass yields of the two pure stands, the bar on the middle right side displays the measured shoot biomasses of the dual mixture of the field trial. Two lines have been added to better visualize the differences between estimated and measured above-ground biomass yields. The black-dotted line represents the estimated yield, while the red line displays the measured shoot biomass yield (Wendling et al., 2017; Bernardo, 2020) (Figures 2A, B). When mixtures outperform even the highest-yielding pure stand, the interaction of the two species is described as transgressive over-yielding (De Wit, 1960; Hooper and Dukes, 2004; Hector, 2006; Wendling et al., 2017). If the measured total shoot biomass yield of the mixture is less than the highest shoot biomass of the pure stand but exceeds the expected shoot biomass of the mixture (Figure 2A), the interaction of the two species is called over-yielding (Hooper and Dukes, 2004; Hector, 2006; Wendling et al., 2017; Couëdel et al., 2018b; Elhakeem et al., 2021). Alternatively, the shoot biomass yield of the mixture is equal to the estimated shoot biomass derived from the pure stand yield. In this case, the interaction of the two species is described as an additive effect (Figure 2B). If one plant species impedes another’s growth and the measured shoot biomass is lower than the calculated one, these are antagonistic effects.
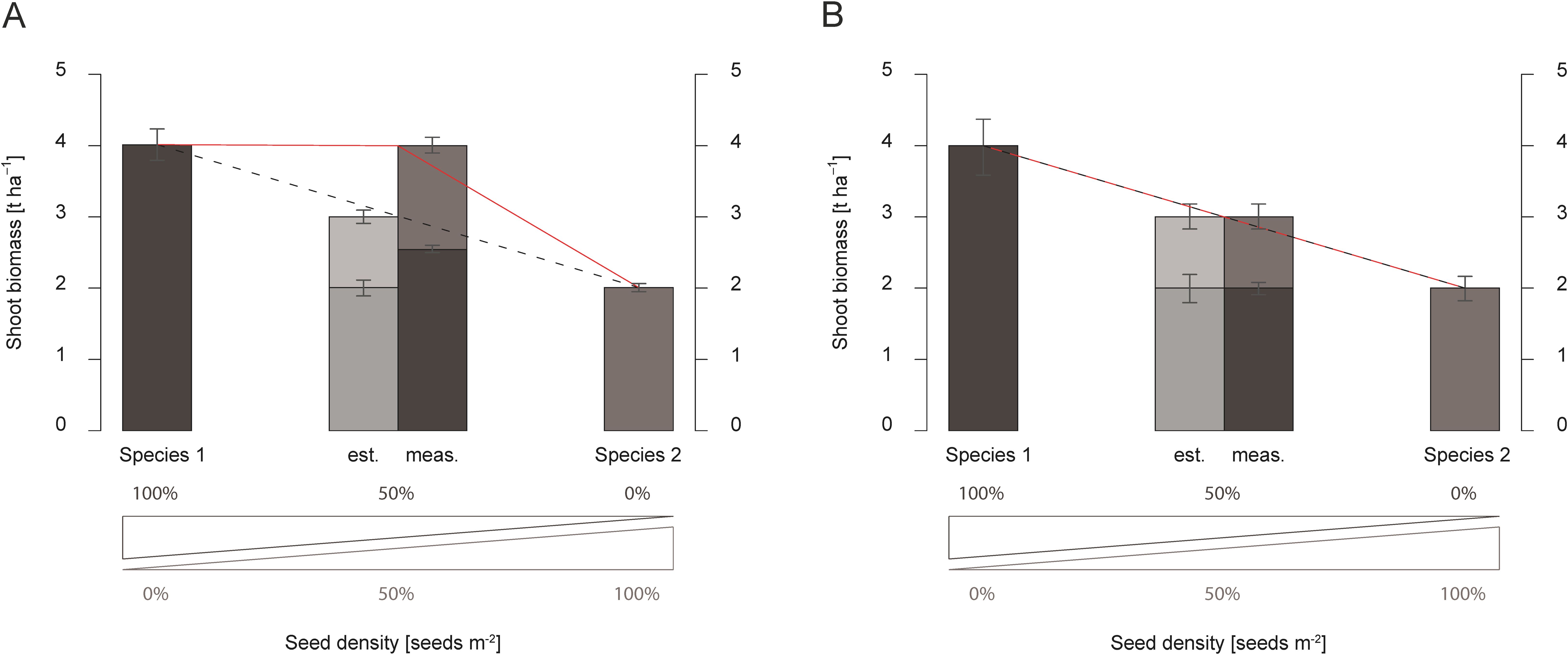
Figure 2. Examples demonstrating over-yielding (A) and additive effects (B) in dual mixtures. The plots show schematically shoot biomass production [t ha-1] of pure stands of the two species (1 & 2) used in the dual mixture. The estimated shoot biomass (est.) based on half of the pure stands´ single species above-ground biomass of all four repetitions is displayed in the middle-left bar. In comparison, the measured shoot biomass (meas.) of the dual mixture at the end of the vegetation period is shown in the middle-right bar. Error bars represent standard deviation.
Oilseed rape was selected as a test weed in the experiment because of its strong competitiveness and its similar use in other studies (Krato and Petersen, 2012; Gregoire et al., 2021). Besides the test weed and cover crop shoot biomass, other weed species were not considered due to their negligible proportion of the shoot biomass. The percentage of test weed shoot biomass refers to its proportion in the total shoot biomass. The competitiveness of cover crop treatments to suppress test weeds is described by the total dry matter shoot biomass (i.e., cover crops and weeds) harvested before winter, minus the percentage of test weed shoot biomass (Di Bella et al., 2021), customized to: Equation 1.
2.6 Statistical analysis
Statistical analysis was conducted using R version 4.3.1 (R Core Team, 2021). A mixed model accounting for block and year effects was fitted using the lmer function from the “lme4” package (Bates et al., 2015) to model the dry matter yield of the cover crops. Since analysis of variance (ANOVA) of this model showed a significant interaction of cover crop species and year, we decided to analysis each year with a separate model accounting for the block effects (treated as random). Residuals of the models were checked for normal distribution and heteroscedasticity with diagnostic plots before performing an ANOVA (Kozak and Piepho, 2018). If needed, observed values were transformed to meet this assumption.
Models were fitted at three different levels for each year: at the total plot level, at the species level, and with total plot values of observed and expected mixtures. Block effects were included as random effects, and at the species level, the interaction of species and cover crop treatment and the effect of the plot (both treated as random) were included. If the ANOVA was significant (p < 0.05), analyses were performed for each species without adjustment of p-values (Fisher´s LSD test) at the plot yield level. Analysis at the species level was also performed separately for each combination of species with Tukey’s adjustment of p-values (Tukey’s HSD test) due to 4 groups (species 1 & 2 in pure stand and their share in the mixture) being compared, using the ‘emmeans package’ (Lenth, 2017). A two-sample t-test was performed for each mixture to compare the actual measured total yield of a mixture with the estimated total yield. Linear regression analysis was performed to test the effect of cover crop shoot biomass on test weed shoot biomass. Prior to analysis, the data were checked for zero inflation using the R package “Performance” (Lüdecke et al., 2021).
3 Results
Multi-species cover crop mixtures are supposed to improve cropping systems and agronomic ecosystem services such as weed suppression. For this reason, the shoot biomass of cover crops in pure stands or dual mixtures was determined under two different conditions. While the 2016 growing season was characterized by moderate precipitation, growing conditions in 2017 were influenced by high precipitation and unlimited water supply.
3.1 Interactions in dual cover crop mixtures on shoot biomass formation
All species were grown as pure stands or in mixtures with adjusted seeding rates (50% of pure stands Table 1) to evaluate the interactions between different species in dual mixtures compared to pure stands. In the first step, mustard-containing cover crops were tested based on mustards’ historical relevance in cover cropping.
3.1.1 Dual mixtures based on mustard
In 2016, mustard produced 4.37 t ha-1 shoot biomass in a pure stand, while the second species tested in a dual mixture, oil radish, had 3.71 t ha-1 when grown in a pure stand (Figure 3A). Consequently, the estimated yield of the mustard and oil radish mixture (half of the shoot biomass yields of both species) reached 4.04 t ha-1 in 2016. The dual mixture achieved above-ground biomass of 4.44 t ha-1, which was not significantly higher than the estimate, thus indicating an additive effect on shoot biomass (Figure 3A). Interestingly, oil radish had the highest proportion of shoot biomass in the mixture, exceeding its estimated yield (Figure 3A), indicating a higher interspecific competitiveness than mustard. This result was reproduced under the non-water limiting conditions in 2017. However, the mixture’s yield increase exceeded mustard’s shoot biomass, although the differences were not statistically significant (Figure 4A).
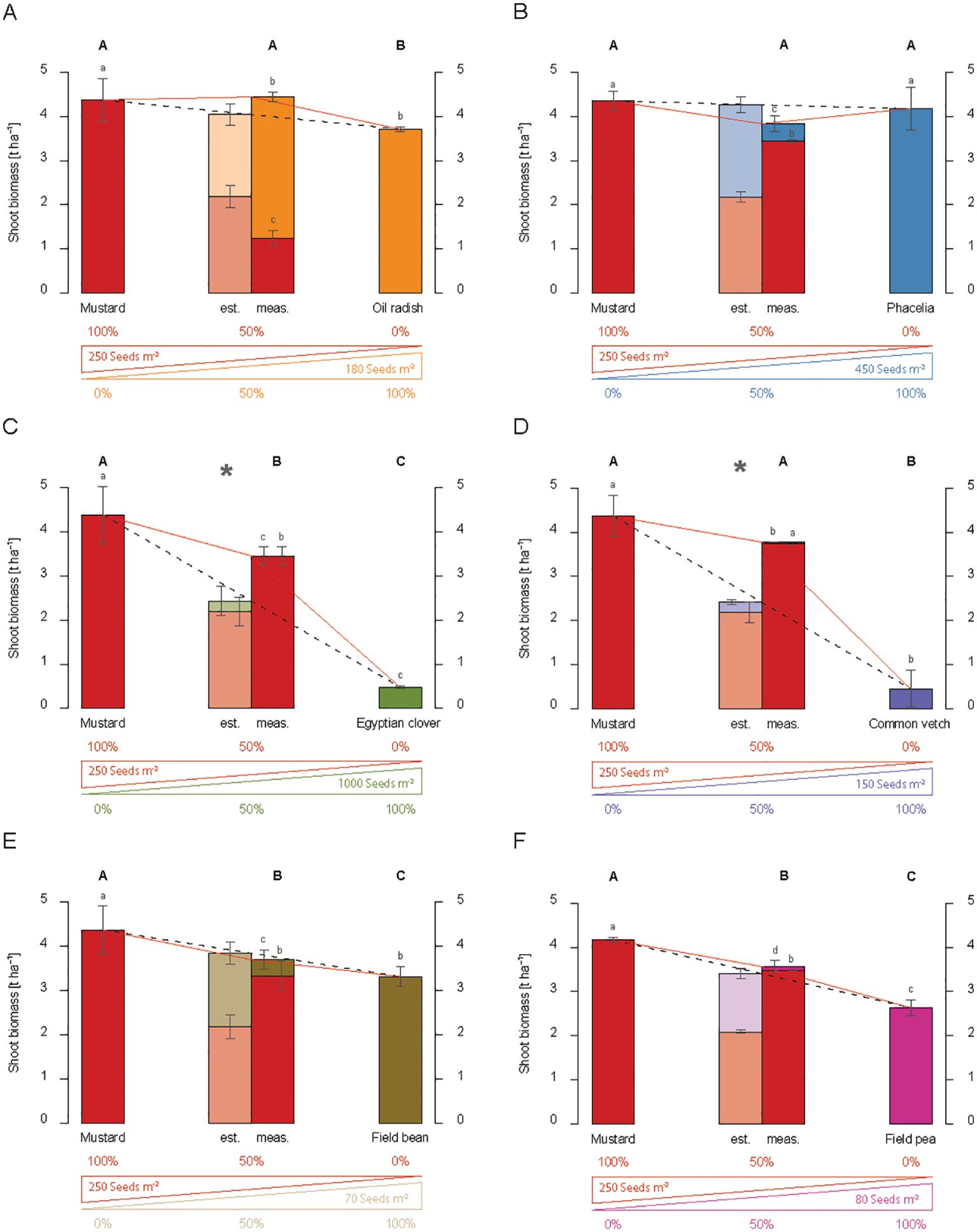
Figure 3. Shoot biomass production (dry matter) [t ha-1] of dual mixtures of cover crops consisting of mustard and a second species, namely oil radish (A), phacelia (B), Egyptian clover (C), common vetch (D), field bean (E), or field pea (F) compared to pure stands in 2016. The plots show results for pure stands of the two species used in the dual mixture, the estimated shoot biomass (est.) based on 50 % of each species in pure stand in relation to the measured shoot biomass (meas.) of the dual mixture at the end of the vegetation period, and the proportion of the individual species in shoot biomass of the mixture. The bars show means with standard deviations, n=4. Different capital letters indicate the significant differences in total biomass yield of treatments at p < 0.05 by Fisher LSD-Test, while different lower-case letters indicate significant differences in the total biomass of different species at p < 0.05 by Tukey’s HSD-Test. Significant differences between estimated and measured shoot biomass of dual mixtures are indicated by an asterisk (*, t-test, p < 0.05).
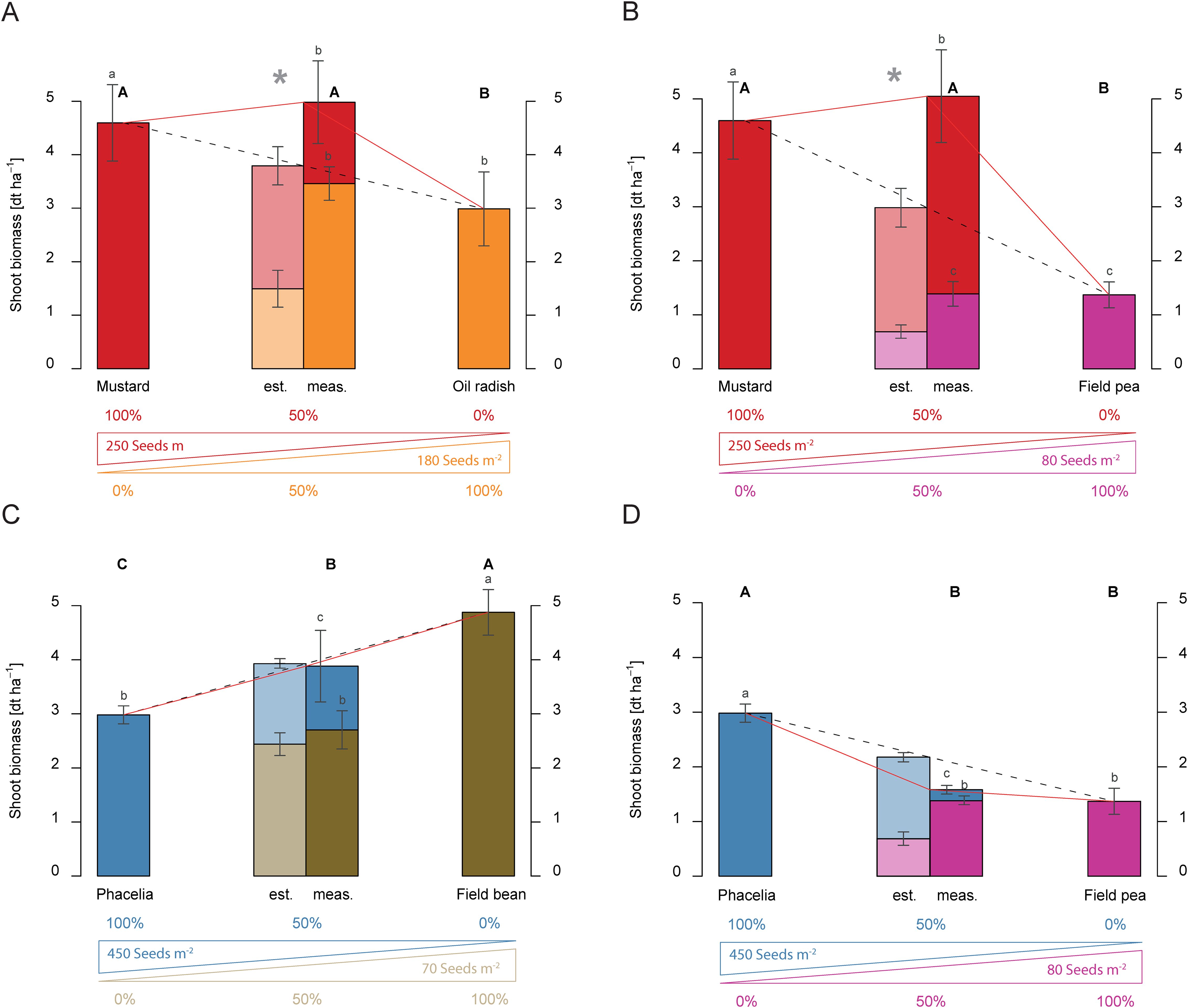
Figure 4. Shoot biomass production (dry matter) [t ha-1] of dual mixtures of cover crops consisting of mustard and a second species, namely oil radish (A), and field pea (B), or phacelia in combination with field bean (C) or field pea (D) on shoot biomass production [t ha-1] compared to pure stands in 2017. The plots show results for pure stands of the two species used in the dual mixture, the estimated shoot biomass (est.) based on 50 % of each species in pure stand in relation to the measured shoot biomass (meas.) of the dual mixture at the end of the vegetation period, and the proportion of the individual species in the shoot biomass of the mixtures. The bars show means with standard deviations, n=4. Different capital letters indicate the significant differences in total biomass yield of treatments at p < 0.05 by Fisher LSD-Test, while different lower-case letters indicate significant differences in the total biomass of different species at p < 0.05 by Tukey’s HSD-Test. Significant differences between estimated and measured shoot biomass of dual mixtures are indicated by an asterisk (*, t-test, p < 0.05).
When mustard was grown with phacelia, the measured above-ground biomass yield was equal to that of the pure stands (Figure 3B). Mustard reached a higher proportion of shoot biomass than estimated based on the performance of the pure stands of both species. However, the measured above-ground biomass of the dual mixture showed no advantage over the pure stands or the estimated mixture. Thus, it resulted in an additive effect, which was also observed in the wet conditions of 2017, even though the proportion of phacelia’s shoot biomass was lower in the dual mixture (Supplementary Figure S1A).
Overyielding was observed in 2016 when mustard was grown in a dual mixture with the legume Egyptian clover and common vetch. Clover alone had a shoot dry matter yield of only 0.48 t ha-1, and the estimated yield of the mixture was 2.43 t ha-1. In the field trial, the measured shoot biomass of the Egyptian clover and mustard mixture exceeded the estimated shoot biomass and significantly overreached it by 1.02 t ha-1 in 2016. However, Egyptian clover contributed only 0.001 t ha-1 to the shoot biomass in this mixture (Figure 3C), and mustard reached 78.8% of the yield level of its pure stand even when half of the seed density was applied. A similar over-yielding effect was observed in the mustard-common vetch mixture. Here, the measured shoot biomass exceeded the estimated shoot biomass significantly by as much as 1.55 t ha-1 (Figure 3D).
These results suggest that combining mustard and legumes leads to an overyielding effect. However, this is in contrast to the fact that a combination of mustard with the legume field bean or field pea only led to additive effects in 2016 (Figures 3E, F), even though the above-ground biomass of field bean (3.31 t ha-1, Figure 3E) and field pea (2.64 t ha-1, Figure 3F) was apparently higher than that of Egyptian clover (0.48 t ha-1, Figure 3C) or common vetch (0.45 t ha-1, Figure 3D). Despite the comparatively high shoot biomass of field beans and field peas, the proportion of these crops in the above-ground biomass of the mixtures was surprisingly low (Figures 3E, F). These observations were stable for field beans over the years or for weather conditions.
Comparable observations were made for the grain legume field pea. The mixture with mustard showed 0.19 t ha-1 above the estimate in 2016, and mustard produced 97.7% of the biomass (Figure 3F). In 2017, the non-limiting water conditions changed the biomass proportions. The above-ground biomass of the mustard–field pea mixtures was 2.10 t ha-1 higher than the estimate. This resulted in total shoot biomass of 5.02 t ha-1 (Figure 4B) and an over-yielding effect. Interestingly, the proportion of field pea shoot biomass in the mixture (1.43 t ha-1) was on the same level as the total shoot biomass of the field peas in the pure stand (1.36 t ha-1).
These results indicate that the interactions between mustard and a second species and its impact on shoot biomass are rather species-specific than plant family-specific. However, the effects on the above-ground biomass yield of mustard-based mixtures tested were highly comparable under different growing conditions.
3.1.2 Dual mixtures based on phacelia
To investigate if the interactions between species tested are species-specific, mustard was replaced by the less competitive cover crop species phacelia in dual mixtures (Figure 5). Compared to the results of mustard-containing mixtures (Figure 3), the legumes showed increased competitiveness against phacelia. The small-seeded legumes Egyptian clover and common vetch contributed a slightly higher proportion of shoot biomass in the mixtures (Figures 5A, B) than in the dual mixtures with mustard, and the share of field beans and field peas in dual mixtures with phacelia was markedly higher (Figures 5C, D).
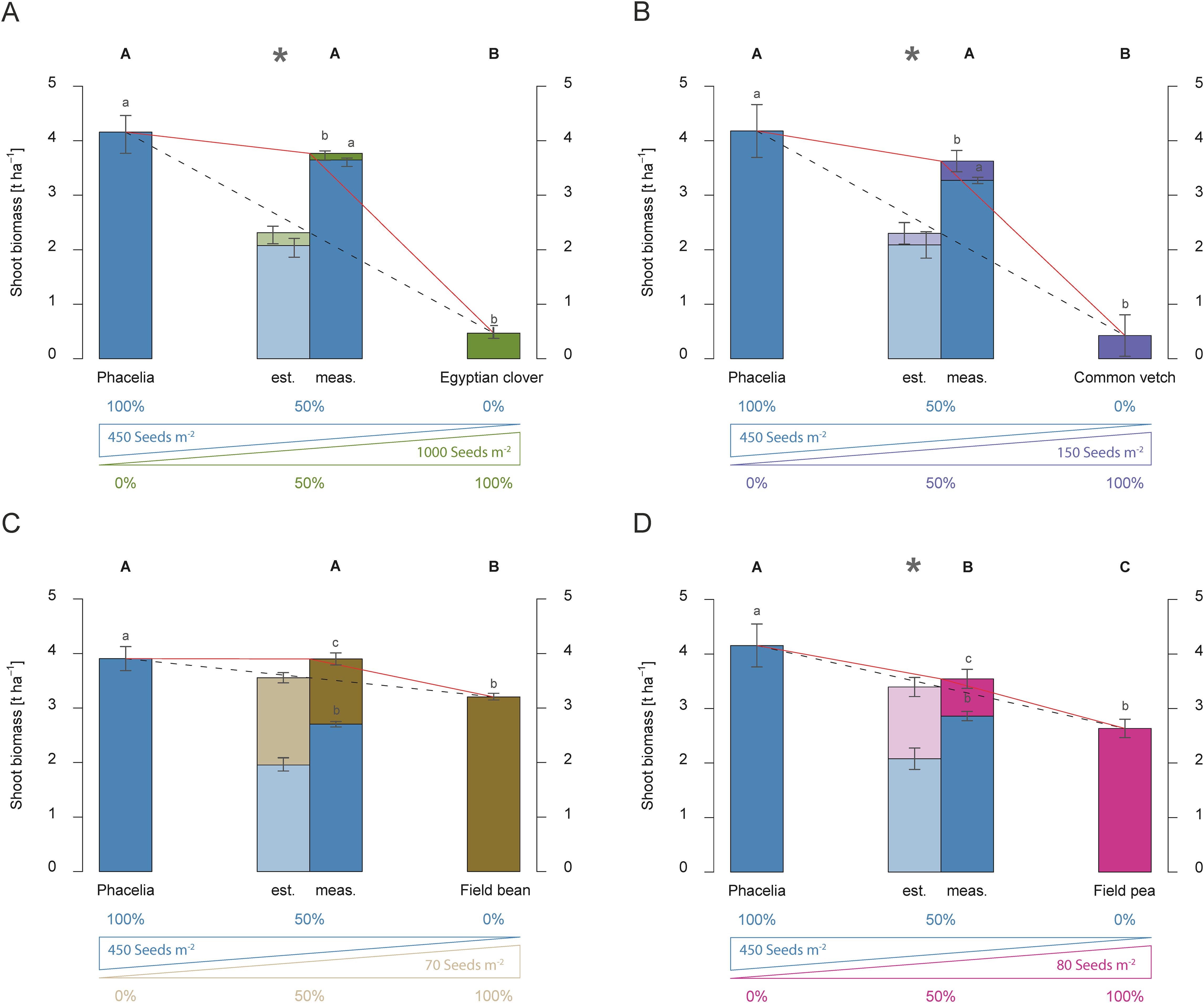
Figure 5. Shoot biomass production (dry matter) [t ha-1] of dual mixtures of cover crops consisting of phacelia and a second species, namely Egyptian clover (A), common vetch (B), field bean (C), and field pea (D) compared to pure stands in 2016. The plots show results for pure stands of the two species used in the dual mixture, the estimated shoot biomass (est.) based on 50 % of each species in pure stand in relation to the measured shoot biomass (meas.) of the dual mixture at the end of the vegetation period, and the proportion of the individual species in shoot biomass of the mixture. The bars show means with standard deviations, n=4. Different capital letters indicate the significant differences in total biomass yield of treatments at p < 0.05 by Fisher LSD-Test, while different lower-case letters indicate significant differences in the total biomass of different species at p < 0.05 by Tukey’s HSD-Test. Significant differences between estimated and measured shoot biomass of dual mixtures are indicated by an asterisk (*, t-test, p < 0.05).
Over-yielding was observed when phacelia was combined with Egyptian clover. The measured shoot biomass exceeded the estimated shoot biomass significantly by 1.45 t ha-1 in 2016 (Figure 5A). A similar effect occurred in the phacelia-common vetch mixture; the measured shoot biomass surpassed the estimate significantly by 1.32 t ha-1 (Figure 5B). In phacelia-field bean mixtures, only an additive effect was observed regardless of the water condition (Figures 5C, 4C). Field beans contributed a larger share of shoot biomass of 1.11 t ha-1 in the mixture with phacelia than 0.30 t ha-1 in a mixture with mustard (Figure 3E). In contrast to 2016 (Figure 5C), field beans contributed 2.85 t ha-1 under non-water-limited conditions (Figure 4C). So, field beans provided the majority of shoot biomass of the 4.00 t ha-1 in 2017.
Combining phacelia and field peas instead of field beans led to slight overyielding effects in shoot biomass formation, with a total yield of 3.54 t ha-1 in 2016 (Figure 5D). Surprisingly, a suppressive effect was observed in the phacelia-field pea mixture under water-sufficient conditions in 2017 (Figure 4D). In contrast to the mustard-field pea mixture (Figure 4B), phacelia contributed only a small portion of the shoot biomass in the phacelia-field pea mixture (Figure 4D), while under the water-limited conditions in 2016, phacelia produced more than 80% of the shoot biomass of the mixture (Figure 5D). Nevertheless, the most striking observation was that dual mixtures of legumes with phacelia showed similar shoot biomass yield response with mustard.
3.1.3 Single plant development in dual mixtures
So far, this work has focused on the total shoot biomass yield (dry matter) of the mixtures and the species involved in pure stands. We then shifted the focus to individual plants to examine the interactions between species in mixtures in more detail. One measure of the intraspecific competition of a species is the average weight of individual plants. Mustard showed significantly higher single-shoot weights (dry matter) when grown with Egyptian clover, common vetch, field bean, field pea or phacelia than with oil radish (Figure 6A). In contrast, phacelia (Figure 6B) significantly decreased single-shoot weights in mixtures with oil radish and mustard compared to those with Egyptian clover, field bean, or field pea. Similar observations were made for single-plant shoot weight of mustard or phacelia under water-sufficient conditions in 2017, except for field pea treatments (Supplementary Figure S2). One explanation may lie in the generally poor phacelia and field pea growth under water-sufficient conditions in 2017. In addition, field emergence was determined to rule out the possibility that plant density differences affected individual plants´ growth conditions (Supplementary Table S1), and no statistically significant differences between treatments were found.
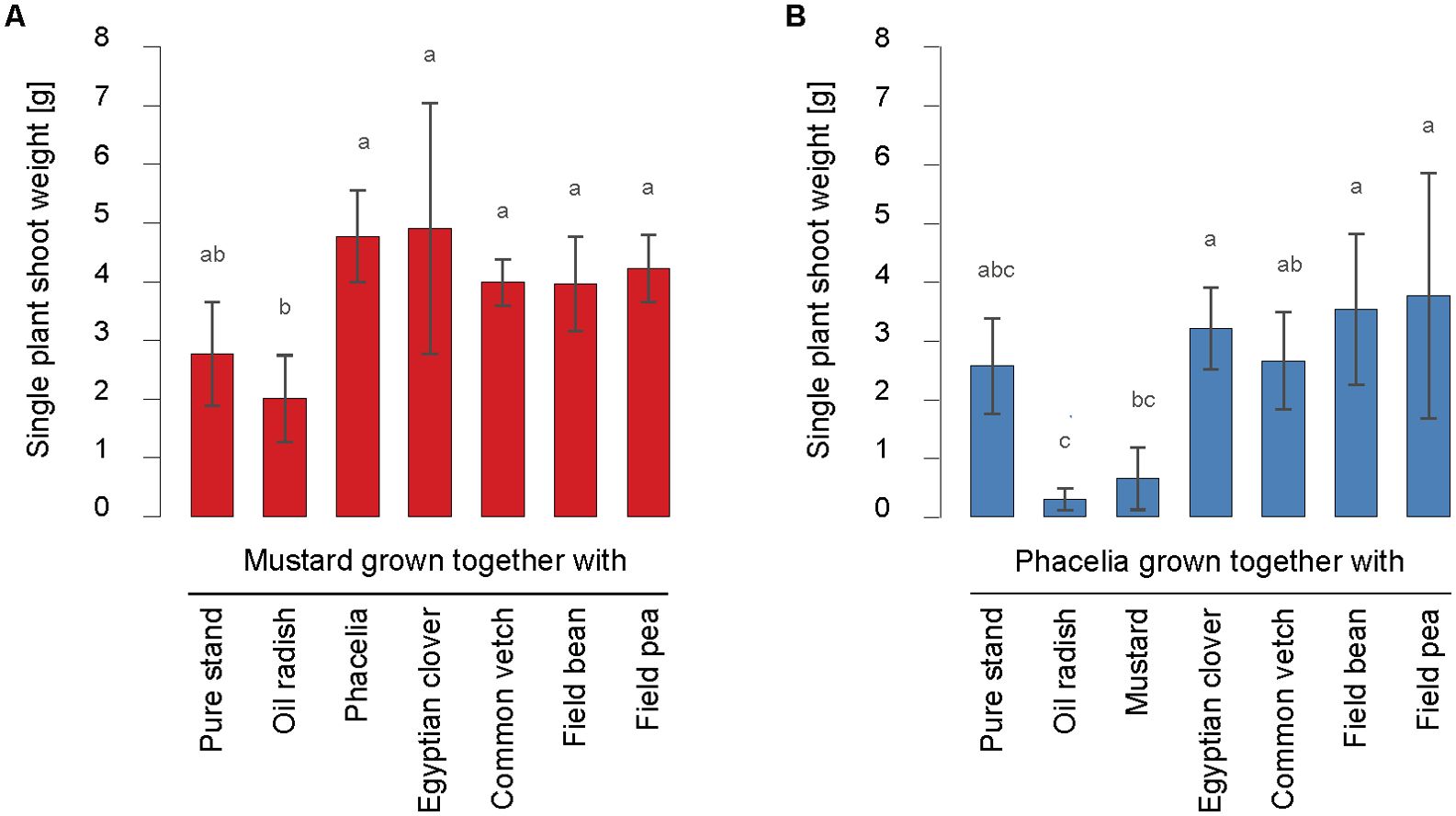
Figure 6. Single plant shoot weight (dry matter) [g] of mustard (A) and phacelia (B) in pure stand and dual mixtures with oil radish, Egyptian clover, common vetch, field bean, and field pea in 2016. Bars show means with standard deviations, n=4; different letters indicate significant differences among treatments at p < 0.05 by Tukey’s HSD-Test.
3.1.4 Interactions in mix containing legumes only
So far, only mixtures between legumes and non-legumes have been investigated. Following the observation that small-seeded legumes, such as Egyptian clover and vetch, caused over-yielding effects in dual mixtures with non-legume species (Figures 3C, D, 5A, B), it was tested whether this observation can also be made in combination containing small-seeded legumes and grain legumes. Significant differences were found between estimated and measured shoot biomass with an overyielding effect of 0.83 t ha-1 when the small-seeded legume common vetch was combined with field bean (Figure 7A). The increased yield of field beans caused the overyielding effect compared to the estimate. However, with 3.16 t ha-1, the above-ground biomass was highest in the pure stand of field beans. A similar result was found for the common vetch-field pea mixture. Here, an overyielding effect was observed with a difference of 0.53 t ha-1 between measured and estimated shoot biomass (Figure 7B). An additive effect was also observed when the field beans and field peas were grown together (Figure 7C). Here, the above-ground biomass yield of the two species and their expected share in the mixture corresponded to the estimate.
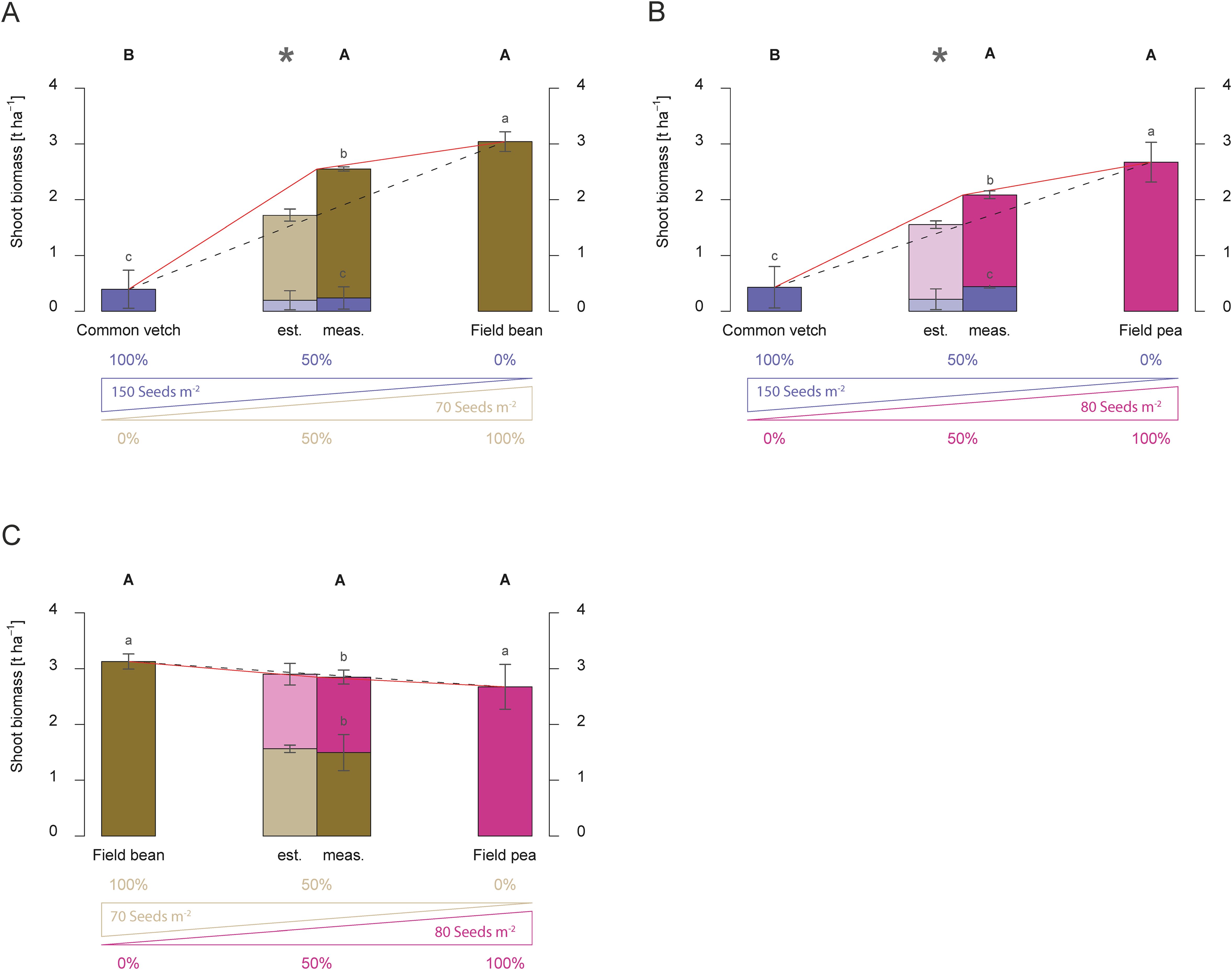
Figure 7. Shoot biomass production (dry matter) [t ha-1] of dual mixtures of cover crops consisting of legumes compared to pure stands in 2016. Mixtures combine common vetch and field bean (A), common vetch and field pea (B), and field bean and field pea (C). The plots show results for pure stands of the two species used in the dual mixture, the estimated shoot biomass (est.) based on 50 % of each species in pure stand in relation to the measured shoot biomass (meas.) of the dual mixture at the end of the vegetation period, and the proportion of the individual species in shoot biomass of the mixture. The bars show means with standard deviations, n=4. Different capital letters indicate the significant differences in total biomass yield of treatments at p < 0.05 by Fisher LSD-Test, while different lower-case letters indicate significant differences in the total biomass of different species at p < 0.05 by Tukey’s HSD-Test. Significant differences between estimated and measured shoot biomass of dual mixtures are indicated by an asterisk (*, t-test, p < 0.05).
Taken together, the interactions among cover crop species mainly resulted in an additive or overyielding effect on above-ground biomass. Interestingly, observations in mixtures showed comparable interaction patterns regardless of whether phacelia or mustard was grown with a second species. Additive effects were observed, especially when grain legumes were added, while overyielding occurred when small-seeded legumes complemented the mixture.
3.2 Competitiveness of cover crops against weeds
Weed suppression is one management service that cover crops provide in crop rotations. We evaluated the competitiveness of cover crop treatments against weeds by determining the shoot biomass of oilseed rape as test weed at cover crop harvest at the end of the vegetation period.
A negative correlation was observed between the above-ground dry matter biomass of cover crops and the weed under water-limited conditions in 2016 (Figure 8A) but not under sufficient water supply in 2017 (Figure 8B). The cover crop shoot biomass in 2016 explained 72% (p < 0.001) of the variance in test weed shoot biomass. A species-specific pattern was evident for pure stands (marked with crosses). The small-seeded legumes Egyptian clover and common vetch did not suppress weeds sufficiently. In contrast, the grain legumes field peas and even more field beans were able to reduce weed shoot biomass. However, the test weed shoot biomass was higher than 0.5 t ha-1. Best results in weed suppression were obtained with phacelia, mustard, or oil radish, where the test weed no longer developed.
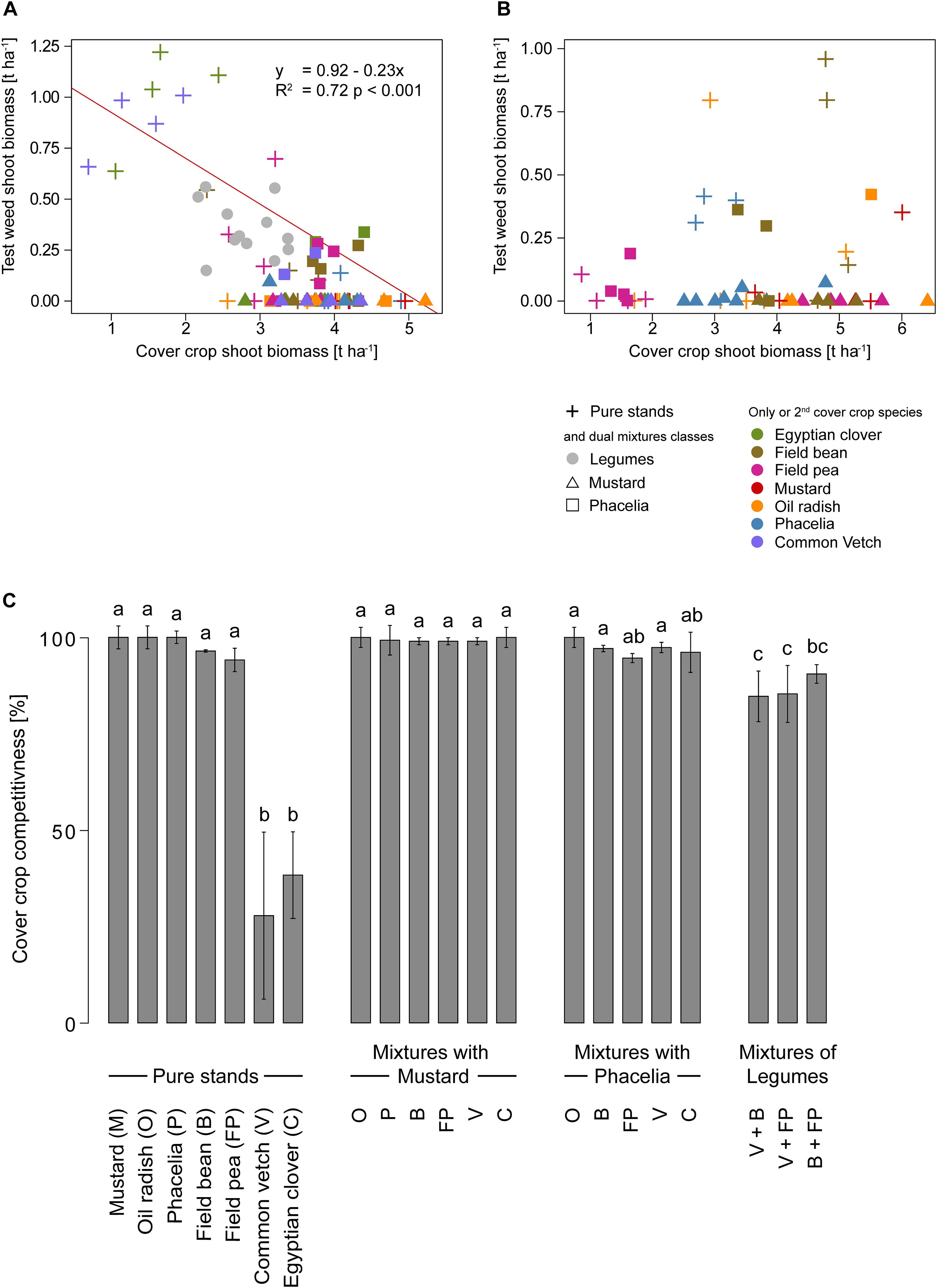
Figure 8. Correlation between cover crop shoot biomass (dry matter) [t ha-1] in pure stand (crosses) or dual mixtures containing legumes only (dots), mustard (triangle), phacelia (square), with a second partner (color code) and test weed shoot biomass (dry matter) [t ha-1] in 2016 (A) and 2017 (B). Color code represents the species of the pure stand or the second partner in the dual mixture and the influence of cover crops pure stands and dual mixtures on competitiveness (C) in 2016. Bars show means with standard deviations, n=4; different letters indicate significant differences among treatments at p < 0.05 by Tukey’s HSD-Test.
As shown earlier, over-yielding effects were observed in some dual mixtures. We therefore tested whether the increased above-ground biomass would also lead to increased weed suppression. Dual mixtures consisting only of legumes (marked with grey dots) showed better weed suppression (Figure 8A), although weed suppression was significantly lower than in other dual mixtures (Figure 8C). Mixtures of phacelia with other cover crops (marked with squares) showed that all legumes tested decreased the competitiveness compared to the pure phacelia stand (Figure 8A). This even held for the grain legumes field bean and field pea, which produced much more biomass than small-grained legumes. Combining phacelia with mustard or oil radish outcompeted the weeds. On the other hand, mustard (marked with triangles) suppressed the weeds, regardless of which other species were grown in the dual mixture.
The competitiveness of cover crop treatments against weeds can also be described by the percentage of cover crop shoot biomass to the whole shoot biomass of the treatment. The cruciferous species oil radish and white mustard and the non-cruciferous species phacelia had a high competitiveness of 99.2 to 100.0% in a pure stand (Figure 8C). Pure stands of field beans and field peas had a lower competitiveness of 92.3% compared to cruciferous species. Generally, pure stands of Egyptian clover (38.5%) and common vetch showed significantly lower competitiveness (28.2%, Figure 8C) than the other cover crops in pure stands.
Combinations with cruciferous species attained a high competitiveness of 99.0% to 100.0% (Figure 8C). In contrast, the competitiveness decreased in dual mixtures of phacelia with Egyptian clover (96.1%), common vetch (97.4%), field bean (96.1%), and field pea (96.0%). Mixtures containing only legumes showed the lowest competitiveness in dual mixtures. Compared to other dual mixtures, combinations of common vetch and field bean (84.8%), common vetch and field pea (85.4%), and field bean and field pea (90.6%) showed significantly lower competitiveness against the test weed, compared to mixtures containing cruciferous species and mixtures of phacelia with field peas or common vetch (Figure 8C).
Under sufficient water conditions in 2017, no correlation was observed between cover crop shoot biomass and test weed shoot biomass. However, competitive species such as oil radish, mustard, and phacelia competed well with the test weed, regardless of whether it was grown in pure stands or mixtures (Figure 8B). A closer look at the competitiveness showed that phacelia had significantly lower competitiveness in the pure stand than mustard or oil radish under the conditions of 2017 (Supplementary Figure S3). Nevertheless, similar patterns were observed in 2016 and 2017.
In summary, the ability of competitive species to compete against the test weed was not hampered when dual mixtures with less competitive species were formed. However, unlike shoot biomass, no synergistic effects in suppression performance were observed between the species used.
4 Discussion
The integration of cover crops into cropping systems offers many benefits in terms of ecosystem services and crop management. These benefits are further enhanced when combined with other techniques, such as reduced tillage (Groß, 1996; Wittwer et al., 2017; Abdalla et al., 2019; Alletto et al., 2022). On the other side, it becomes more challenging to control volunteer plants and weeds. In conventional farming systems, volunteer seed germination is often controlled with non-selective herbicides before planting to maintain cover crop benefits and reduced tillage intensity. However, this strategy has been criticized from several perspectives. A promising strategy is to control volunteer plants and weeds by growing cover crops during the off-season. This approach requires high competitiveness of cover crop mixtures by using species that produce more above-ground biomass or better compete with weeds (Büchi et al., 2018; Grosse and Heß, 2018; Schappert et al., 2018; Schmidt et al., 2019; Bunchek et al., 2020). Therefore, the present study aimed to identify mechanisms in shoot biomass formation in dual cover crop mixtures of different plant species and their ability to suppress weeds.
4.1 Impact of interactions between species in cover crop mixtures on shoot biomass is species-specific
Plants growing in a mixed stand compete for space, light, water, and nutrients. This becomes even more important when combining species with different characteristics, as in cover crop mixtures. Nevertheless, interactions among species can also promote plant growth (Baeumer and De Wit, 1968; Heuermann et al., 2019). One hypothesis as to why mixtures can produce more shoot biomass than pure stands assumes that competitive species benefit from less competitive partners in mixtures. However, in contrast to previous studies (Finney et al., 2017; Wendling et al., 2017), we did not observe significantly higher shoot biomass yields than in pure stands, described as transgressive over-yielding under the water-limited conditions in 2016 (Figures 3, 5 & 7), but in mustard - oil radish and mustard – field pea mixtures under the water-sufficient conditions in 2017 (Figures 4A, B). Nevertheless, an overyielding effect was evident in 2016 when the competitive species mustard was combined in dual mixtures with less competitive species such as Egyptian clover and common vetch (Figures 3C, D). Similar observations were made for phacelia. While phacelia is not as competitive as mustard (Figure 3B), the number of less competitive partners leading to over-yielding effects was even higher and included field beans in addition to Egyptian clover and common vetch (Figures 5A–C). Even when dual mixtures were designed among much less competitive species, such as field bean or field pea, the combinations with even less competitive species, such as common vetch, resulted in an over-yielding effect (Figures 7A, B).
Compared to the estimated shoot biomass based on the pure stand, the shoot biomass of the competitive partner (mustard, phacelia, and field bean) increased significantly in dual mixtures with weaker species, not only in dual mixtures showing over-yielding. For mustard, this observation is consistent with other studies showing its high competitiveness in dual mixtures with legumes (Wortman et al., 2012; Couëdel et al., 2018b). However, our results suggest that this is wider than legumes. Mustard also increased shoot biomass relative to the estimate in mixtures with phacelia (Figure 3B). There is a clear indication that this effect generally works, as shown for phacelia against less competitive species such as Egyptian clover, common vetch, and field bean (Figures 5A–C), or field bean against common vetch (Figure 7A). Moreover, this effect is even reversible when these previously competitive species are combined with an even more competitive species, such as oil radish in the case of mustard (Figure 3A), mustard in the case of phacelia (Figure 3B), or mustard and phacelia in the case of field bean (Figures 3E, 5C). When placed as the weaker partner in another mixture, the former dominant species showed less shoot biomass than the estimate based on pure stand yields.
The mode of action of the increasing above-ground biomass of the competitive species in a mixture is unlikely due to the promotive effect of nitrogen fixation of the less-competitive partner because it was also observed with non-legume partners. Non-legume species increased the growth of more competitive partners, even if they belonged to the same family and shared similar root properties for nutrient acquisition or pest control, such as oil radish and mustard (Figure 3A). The promotive effect was also observed even if only legumes, such as field beans and common vetch, were combined (Figure 7A). This observation is even more significant because similar results were observed under the water sufficient conditions in 2017, and transgressive over-yielding even occurred for the mustard-field pea mixture when mustard was the dominant partner (Figure 4B) and the mustard-oil radish mixture when mustard became the weaker partner of the mixture (Figure 4A).
Another mechanism is explained by a niche differentiation leading to a complementary effect or the dominance of a higher-yielding species, known as a selection effect (Loreau and Hector, 2001). Studies by Elhakeem et al. (2021) and Couëdel et al. (2018b) observed both effects in mixtures containing oil radish. These findings might further indicate that the competitive species mustard, oil radish, and phacelia generated higher shoot biomass through a selection effect. In another experiment, higher crop growth rates and higher crop nitrogen uptake rates of mustard than phacelia and mustard were measured across different environments (Tribouillois et al., 2015a).
Another approach to further investigate the mechanism of increased above-ground biomass of the competitive species by companion crops is to shift the focus from total shoot biomass in the stand to the single plant weight. Both mustard and phacelia decreased shoot weight per plant when combined with more competitive species (Figures 6A, B). Mustard reduced shoot formation by over one-fourth when combined with oil radish (Figure 6A) and phacelia by factor 4 when grown together with mustard (Figure 6B). However, the seeding rate was reduced by half for all species in the dual mixture. The situation was quite different when tested species acted as the competitive partner in a mixture. They showed an apparent increase in the shoot weight per plant regardless of whether the less competitive partner was a legume or not (Figures 6A, B). Based on these observations, the main advantage of the weak partner, besides increasing diversity, appears to be its retarded development or the small plant size, resulting in higher availability of space and resources for the rapidly developing competitive partner. Supposing the advantage is only based on more space and resources per plant, the effect must also be present when reducing seed density. However, a reduction in the seeding rate did not increase the above-ground biomass in the species tested (data not shown). This is also consistent with Wendling et al. (2017), who showed that responses to sowing rate adjustments are species-specific and dependent on the fertilization level.
The stimulatory effect on the single plant weight of a competitive partner in a mixture was observed regardless of whether the combination resulted in over-yielding (Figures 3C, D, 5A–C) or not (Figures 3B, E, F, 5D). There is a clear indication that over-yielding is only possible if the weak partner reduces its proportion of shoot biomass compared to the estimate. However, this conclusion does not align with the transgressive over-yielding effects observed under water-sufficient conditions in 2017 (Figure 4). While the radish-mustard mixture followed the pattern of 2016 and mustard significantly reduced its shoot growth under these conditions (Figure 4A), the mustard-pea mixture showed a significant increase in shoot biomass of both species compared to the estimate (Figure 4B).
One explanation can be found in the favorable starting conditions for field peas in 2017 compared to 2016. Moderate temperatures and sufficient soil moisture provided perfect conditions for germination and seedling development, even for field peas, which have a high water demand during germination (Raveneau et al., 2011; Tribouillois et al., 2016). For this reason, an equal development of mustard and field peas during early development could lead to similar competitiveness. These observations align with other studies that report transgressive overyielding of mustard-field pea mixture under non-water-limited conditions (Wendling et al., 2017). While the non-limited water conditions promoted field peas, they hampered the development of phacelia (Figures 4C, D compared to 5C, D). Thus, the mixture of phacelia and field beans showed only an additive effect in 2017 (Figure 4C) instead of an over-yielding effect in the water-limited conditions in 2016. The retarded performance of phacelia under water-sufficient conditions in 2017 was also obvious in the phacelia-field pea mixture, resulting even in a suppressive effect (Figure 4D). However, besides phacelia-containing mixtures, the weather conditions had no significant effect on the observed patterns of interactions in the mixtures.
Taken together for autumn-grown cover crops in the two tested environments, over-yielding in cover crop mixtures is likely to occur when two characteristics of the combined species are met: (i) Species must differ in competitiveness. The present study allows competitiveness to be ranked from the strongest to the weakest species from oilseed radish > mustard > phacelia > field bean > field pea > common vetch > Egyptian clover. (ii) The shoot biomass production in pure stands of the less competitive partner must be lower by at least a factor of two than that of the competitive species. However, the system has only been tested for dual mixtures. It should also be noted that phacelia significantly reduces biomass and competitiveness, especially in wet years.
4.2 Improved cover crop mixtures can suppress weeds and volunteer plants
A large above-ground biomass is often proposed as the primary property of a cover crop in weed control (Hoffman et al., 1993; Gerhards and Schappert, 2020). In this study, a negative correlation between the shoot biomass of the cover crop and the shoot biomass of the test weed oilseed rape was only observed in one of the two experimental years (Figures 8A, B). While cover crop shoot biomass was important for weed suppression under water-limited conditions in 2016 (Figure 1A), biomass production was not the mode of action under sufficient water supply in 2017 (Figure 1B). This is consistent with previous observations, which showed no correlation between cover crop shoot biomass and weed suppression (Schappert et al., 2019b; Smith et al., 2020). However, in both years, a suppressive effect was observed for some of the cover crop species or mixtures tested.
Pure stands of mustard and phacelia have been well described to reduce weed shoot biomass more effectively than small-seeded legumes under European field conditions (Brant et al., 2009; Brust et al., 2011). In the present study, the cover crops mustard, oil radish, and phacelia showed the best competitiveness against weeds regardless of the weather conditions. However, the suppressive effect was weaker for phacelia and showed a larger variation in the case of mustard and oil radish under wet conditions in 2017 (Figure 8B). In general, these observations were confirmed by other studies, which also found that pure stands of mustard, oil radish, and phacelia were able to suppress weeds effectively (Brust et al., 2014; Schappert et al., 2019a; Cottney et al., 2022; Tadiello et al., 2022), even under similar growing conditions as in southern Germany in 2016 and 2017 (Schappert et al., 2019a).
Other tested cover crop species, such as field bean and field pea, showed lower competitiveness than cruciferous species. At the same time, common vetch and Egyptian clover failed to outcompete weeds (Figure 8A). Previous studies reported the low competitiveness of pure legume stands against weeds compared to phacelia and oil radish (Cottney et al., 2022). An explanation for this is that the conditions of the locations may not fit this species. However, in northern Italy, Egyptian clover and common vetch as pure stands showed similar low biomass production (Tadiello et al., 2022) as in this study (Figures 3C, D, 5A, B), and hence lower competitiveness against weeds than mustard (Figure 8A). Our results are consistent with studies from Canada, which have shown that legumes have a lower competitiveness against weeds compared to crucifer or forb species (Wagg et al., 2021). Thus, neither the climatic conditions nor the daylength effect cause a low level of competitiveness of the small-seeded legume species.
The use of mixtures is often discussed as a strategy to increase the competitiveness of cover crops, as the species then complement each other in their suppressive effect on weeds. In our study, this hypothesis can only be confirmed in some cases: While mustard alone perfectly suppressed the test weed oilseed rape (Figures 8A, B) and showed a competitiveness factor of almost 100% (Figure 8C; Supplementaryray Figure S3), the addition of other species to form dual mixtures neither increased nor decreased the ability of mustard (Figure 8), regardless of whether such dual mixtures lead to an over-yielding effect (Figure 3). Other studies have shown similar results. For instance, the over-yielding of cover crop mixtures compared to the best-performing pure stands did not lead to benefits in weed suppression or other ecosystem services (Smith et al., 2014, 2020). The question then arises whether increasing the mixtures’ biodiversity affects weed suppression. Schappert et al. (2019b) showed that mixtures containing three or more cover crop species have a reduced ability to suppress weeds compared to pure cruciferous crops. Lower soil coverage and shoot biomass best explained the lack of competitiveness.
Regarding weed suppression, the beneficial effects of competitive cover crops like mustard are rapid field emergence and soil coverage (Brust et al., 2014). These benefits are still present in dual mixtures containing mustard. Other mechanisms of action, such as allelopathic effects via released glucosinolates, were found to be 20% lower in dual mixtures than in pure crucifer stands (Couëdel et al., 2018a). For phacelia, the choice of a partner is more important, even though phacelia showed high competitiveness (99.2%, Figure 8C) under dry growing conditions (Brust et al., 2014; Schappert et al., 2018). In dual mixtures with legumes, phacelia could not fully compensate for the low competitiveness of legumes (Figure 8C), which slightly increased the observed test weed shoot biomass (Figure 8A). Whether this was due to the lower competitiveness of phacelia compared to mustard (Figure 3B), the tendentially lower biomass of the phacelia-legume mixtures (Figure 5), the lack of allelopathic effects (Couëdel et al., 2018a), or further characteristics of the mixture partners (Heuermann et al., 2023) could not be determined here. It remains to be noted that the mixtures showing over-yielding effects (Figures 5A–C) did not perform better in weed suppression (Figure 8).
A remaining open question is whether legumes, in general, are less competitive against weeds than non-legumes. Legume-only combinations were designed to investigate this further. All mixtures tested showed weaker competitiveness against test weeds than cruciferous species or phacelia (Figures 8A, C). Small-seeded legumes such as Egyptian clover and common vetch showed weak weed suppression and weak competitiveness even when combined with grain legumes with higher weed suppression, such as field bean or field pea (Figures 8A–C). Explanations for weak weed suppression by mixtures with legumes, especially small-seeded legumes, may be found in lower growth rates of the legumes compared to other species (Wendling et al., 2016) or poor performance under conditions of lower precipitation and temperature (Lavergne et al., 2021). Although the shoot biomass increased when two legumes were grown in mixtures, their competitiveness did not reach the level of the dual mixtures or the pure stands of the crucifer species (Figure 8).
In conclusion, our results indicate that weed suppression by cover crop mixtures is best achieved by the inclusion of a competitive species such as oil radish or mustard that effectively suppresses weeds even in combination with less competitive species such as field bean, field pea, common vetch, or Egyptian clover under different weather conditions. The use of phacelia is rather recommended under dry conditions. Under water-sufficient conditions, a mixture containing grain legumes as competitive partners, such as field beans or field peas, also performs satisfactorily.
5 Conclusion
In agricultural practice, cover crop mixtures are designed mainly through trial and error, consuming field trial resources to find the best-performing combinations. This study systematically evaluated two-component mixtures under two contrasting weather conditions. Our results show that competitive species in cover crop mixtures are essential for biomass production and weed suppression. Biomass formation showed over-yielding effects when competitive species, such as oil radish, mustard, and phacelia, and to a lower extent, field beans were combined in dual mixtures with species that form less above-ground biomass, such as common vetch or Egyptian clover. By contrast, additional species did not significantly enhance competitiveness against the test weed. Generally, the most competitive cover crops were the dominant ones, such as oilseed radish or mustard, while phacelia performed better, especially in dry conditions. For adaptions strategies, this means that cruciferous species such as mustard and oil radish form the basis of cover crop mixtures in crop rotations without oilseed rape. In oilseed rape rotations, the basic component is phacelia. The other species completing the mixtures must either have a high level of weed suppression or produce significantly less biomass than the main species to induce over-yielding effects in the cover crop mixture. Future research must determine which non-legumes are suitable as weak components to create optimized cover crop mixtures in legume-free rotations with high weed suppression potential. Generally, breeding programs to optimize species for cover crop mixtures and the identification of suitable seeding ratios for mixtures will be promising future strategies.
Data availability statement
The raw data supporting the conclusions of this article will be made available by the authors, without undue reservation.
Author contributions
JG: Conceptualization, Data curation, Formal analysis, Methodology, Visualization, Writing – original draft, Writing – review & editing. RK: Data curation, Visualization, Writing – review & editing. DH: Validation, Writing – review & editing. NG: Data curation, Formal analysis, Writing – review & editing. DS: Writing – review & editing. UF: Writing – review & editing. GG: Writing – review & editing. Nv: Writing – review & editing. BB: Conceptualization, Formal analysis, Methodology, Supervision, Validation, Writing – review & editing.
Funding
The author(s) declare financial support was received for the research, authorship, and/or publication of this article. This work was supported by the German Federal Ministry of Education and Research (grant number 031A559 -CATCHY).
Acknowledgments
We are grateful to Silke Bokeloh, Gerald Fiedler, Steffen Schierding, Farruh Ulmasov, Stefan Uhl, and Johannes Brunner for the excellent technical support during soil sampling and lab work and to Alexander Reinl, Johannes Lindner, Jan Walter Stapf, Dominic Hoffmann, Michael Koch, and Vincent Panzer for the biomass analysis.
Conflict of interest
The authors declare that the research was conducted in the absence of any commercial or financial relationships that could be construed as a potential conflict of interest.
Publisher’s note
All claims expressed in this article are solely those of the authors and do not necessarily represent those of their affiliated organizations, or those of the publisher, the editors and the reviewers. Any product that may be evaluated in this article, or claim that may be made by its manufacturer, is not guaranteed or endorsed by the publisher.
Supplementary material
The Supplementary Material for this article can be found online at: https://www.frontiersin.org/articles/10.3389/fagro.2024.1416379/full#supplementary-material
References
Abdalla M., Hastings A., Cheng K., Yue Q., Chadwick D., Espenberg M., et al. (2019). A critical review of the impacts of cover crops on nitrogen leaching, net greenhouse gas balance and crop productivity. Global Change Biol. 25, 2530–2543. doi: 10.1111/gcb.14644
Alletto L., Vandewalle A., Debaeke P. (2022). Crop diversification improves cropping system sustainability: An 8-year on-farm experiment in South-Western France. Agric. Syst. 200, 103433. doi: 10.1016/j.agsy.2022.103433
Amelung W., Blume H.-P., Fleige H., Horn R., Kandeler E., Kögel-Knabner I., et al. (2018). Scheffer/Schachtschabel Lehrbuch der Bodenkunde (Berlin, Heidelberg: Springer Berlin Heidelberg).
Axelsen J. A., Kristensen K. T. (2000). Collembola and mites in plots fertilised with different types of green manure. Pedobiologia 44, 556–566. doi: 10.1078/S0031-4056(04)70071-2
Baeumer K., De Wit C. T. (1968). Competitive interference of plant species in monocultures and mixed stands. NJAS 16, 103–122. doi: 10.18174/njas.v16i2.17415
Baraibar B., Murrell E. G., Bradley B., Barbercheck M. E., Mortensen D. A., Kaye J. P., et al. (2020). Cover crop mixture expression is influenced by nitrogen availability and growing degree days. PloS One 15, e0235868. doi: 10.1371/journal.pone.0235868
Baraibar B., White C. M., Hunter M. C., Finney D. M., Barbercheck M. E., Kaye J. P., et al. (2021). Weeds in cover crops: context and management considerations. Agriculture 11, 193. doi: 10.3390/agriculture11030193
Bates D., Mächler M., Bolker B., Walker S. (2015). Fitting linear mixed-effects models using lme4. J. Stat. Soft 67, 1–48. doi: 10.18637/jss.v067.i01
Baysal-Gurel F., Liyanapathiranage P. (2019). Pathogenicity of Rhizoctonia solani and Phytophthora nicotianae to Brassicaceae cover crops. Arch. Phytopathol. Plant Prot. 52, 288–302. doi: 10.1080/03235408.2019.1617499
Bernardo R. (2020). Reinventing quantitative genetics for plant breeding: something old, something new, something borrowed, something BLUE. Heredity 125, 375–385. doi: 10.1038/s41437-020-0312-1
Blanco-Canqui H., Ruis S. J., Holman J. D., Creech C. F., Obour A. K. (2022). Can cover crops improve soil ecosystem services in water-limited environments? A review. Soil Sci. Soc. Amer J. 86, 1–18. doi: 10.1002/saj2.20335
Bodner G., Himmelbauer M., Loiskandl W., Kaul H.-P. (2010). Improved evaluation of cover crop species by growth and root factors. Agron. Sustain. Dev. 30, 455–464. doi: 10.1051/agro/2009029
Brant V., Neckář K., Pivec J., Duchoslav M., Holec J., Fuksa P., et al. (2009). Competition of some summer catch crops and volunteer cereals in the areas with limited precipitation. Plant Soil Environ. 55, 17–24. doi: 10.17221/378-PSE
Brust J., Claupein W., Gerhards R. (2014). Growth and weed suppression ability of common and new cover crops in Germany. Crop Prot. 63, 1–8. doi: 10.1016/j.cropro.2014.04.022
Brust J., Gerhards R., Karanisa T., Ruff L., Kipp A. (2011). Warum Untersaaten und Zwischenfrüchte wieder Bedeutung zur Unkrautregulierung in Europäischen Ackerbausystemen bekommen. Gesunde Pflanzen 63, 191–198. doi: 10.1007/s10343-011-0263-9
Büchi L., Wendling M., Amossé C., Necpalova M., Charles R. (2018). Importance of cover crops in alleviating negative effects of reduced soil tillage and promoting soil fertility in a winter wheat cropping system. Agriculture Ecosyst. Environ. 256, 92–104. doi: 10.1016/j.agee.2018.01.005
Bunchek J. M., Wallace J. M., Curran W. S., Mortensen D. A., VanGessel M. J., Scott B. A. (2020). Alternative performance targets for integrating cover crops as a proactive herbicide-resistance management tool. Weed Sci. 68, 534–544. doi: 10.1017/wsc.2020.49
Cottney P., Black L., Williams P., White E. (2022). How cover crop sowing date impacts upon their growth, nutrient assimilation and the yield of the subsequent commercial crop. Agronomy 12, 369. doi: 10.3390/agronomy12020369
Couëdel A., Alletto L., Kirkegaard J., Justes É. (2018a). Crucifer glucosinolate production in legume-crucifer cover crop mixtures. Eur. J. Agron. 96, 22–33. doi: 10.1016/j.eja.2018.02.007
Couëdel A., Alletto L., Tribouillois H., Justes É. (2018b). Cover crop crucifer-legume mixtures provide effective nitrate catch crop and nitrogen green manure ecosystem services. Agriculture Ecosyst. Environ. 254, 50–59. doi: 10.1016/j.agee.2017.11.017
Di Bella L., Zahmel M., van Zwieten L., Rose T. J. (2021). Weed suppression, biomass and nitrogen accumulation in mixed-species and single-species cover crops in a tropical sugarcane fallow. Agriculture 11, 640. doi: 10.3390/agriculture11070640
Elhakeem A., Bastiaans L., Houben S., Couwenberg T., Makowski D., van der Werf W. (2021). Do cover crop mixtures give higher and more stable yields than pure stands? Field Crops Res. 270, 108217. doi: 10.1016/j.fcr.2021.108217
European Commission (2021). REGULATION (EU) 2021/2115 OF THE EUROPEAN PARLIAMENT AND OF THE COUNCIL of 2 December 2021 establishing rules on support for strategic plans to be drawn up by Member States under the common agricultural policy (CAP Strategic Plans) and financed by the European Agricultural Guarantee Fund (EAGF) and by the European Agricultural Fund for Rural Development (EAFRD) and repealing Regulations (EU) No 1305/2013 and (EU) No 1307/2013. Off. J. Eur. Union 2021, p. 117–151.
Finney D. M., Murrell E. G., White C. M., Baraibar B., Barbercheck M. E., Bradley B., et al. (2017). Ecosystem services and disservices are bundled in simple and diverse cover cropping systems. Agric. Environ. Lett. 2, 170033. doi: 10.2134/ael2017.09.0033
Fiorini A., Remelli S., Boselli R., Mantovi P., Ardenti F., Trevisan M., et al. (2022). Driving crop yield, soil organic C pools, and soil biodiversity with selected winter cover crops under no-till. Soil Tillage Res. 217, 105283. doi: 10.1016/j.still.2021.105283
Florence A. M., Higley L. G., Drijber R. A., Francis C. A., Lindquist J. L. (2019). Cover crop mixture diversity, biomass productivity, weed suppression, and stability. PloS One 14, e0206195. doi: 10.1371/journal.pone.0206195
Florence A. M., McGuire A. M. (2020). Do diverse cover crop mixtures perform better than monocultures? A systematic review. Agron.j. 112, 3513–3534. doi: 10.1002/agj2.20340
Gentsch N., Boy J., Batalla J. D. K., Heuermann D., Wirén N., Schweneker D., et al. (2020). Catch crop diversity increases rhizosphere carbon input and soil microbial biomass. Biol. Fertil Soils 56, 943–957. doi: 10.1007/s00374-020-01475-8
Gentsch N., Heuermann D., Boy J., Schierding S., Wirén N., Schweneker D., et al. (2022). Soil nitrogen and water management by winter-killed catch crops. SOIL 8, 269–281. doi: 10.5194/soil-8-269-2022
Gentsch N., Riechers F. L., Boy J., Schwenecker D., Feuerstein U., Heuermann D., et al. (2023). Cover crops improve soil structure and change organic carbon distribution in macroaggregate fractions. EGUsphere 10 (1), 1–18. doi: 10.5194/egusphere-2023-1885
Gerhards R., Schappert A. (2020). Advancing cover cropping in temperate integrated weed management. Pest Manage. Sci. 76, 42–46. doi: 10.1002/ps.5639
Gregoire P., Rosset J. D., Gulden R. H. (2021). Volunteer Brassica napus (L.) interference with soybean [Glycine max (L.) Merr.]: management thresholds, plant growth, and seed return. Can. J. Plant Sci. 101 (4), 556–567. doi: 10.1139/cjps-2020-0258
Groß U. (1996). Einfluß unterschiedlicher Bodenbearbeitungssysteme auf Verschlammungsneigung und Aggregatstabilitat verschiedener Boden: Forschungsbericht Agrartechnik des Arbeitskreises Forschung und Lehre der Max-Eyth-Gesellschaft Agrartechnik im VDI. Justus-Liebig Universität Gießen, Gießen, 143. Dissertation.
Grosse M., Heß J. (2018). Sommerzwischenfrüchte für verbessertes Stickstoff- und Beikrautmanagement in ökologischen Anbausystemen mit reduzierter Bodenbearbeitung in den gemäßigten Breiten. Verlag Eugen Ulmer. 70 (6). doi: 10.1399/JFK.2018.06.01
Hallama M., Pekrun C., Pilz S., Jarosch K. A., Frąc M., Uksa M., et al. (2021). Interactions between cover crops and soil microorganisms increase phosphorus availability in conservation agriculture. Plant Soil 463, 307–328. doi: 10.1007/s11104-021-04897-x
Haramoto E. R., Gallandt E. R. (2004). Brassica cover cropping for weed management: A review. Renew. Agric. Food Syst. 19, 187–198. doi: 10.1079/RAFS200490
Hauer M., Koch H.-J., Krüssel S., Mittler S., Märländer B. (2016). Integrated control of Heterodera schachtii Schmidt in Central Europe by trap crop cultivation, sugar beet variety choice and nematicide application. Appl. Soil Ecol. 99, 62–69. doi: 10.1016/j.apsoil.2015.11.017
Hector A. (2006). Overyielding and stable species coexistence. New Phytol. 172, 1–3. doi: 10.1111/j.1469-8137.2006.01865.x
Hendrickson J. R., Liebig M. A., Archer D. W., Schmer M. R., Nichols K. A., Tanaka D. L. (2021). Late-seeded cover crops in a semiarid environment: overyielding, dominance and subsequent crop yield. Renew. Agric. Food Syst. 36, 587–598. doi: 10.1017/S174217052100020X
Heuermann D., Döll S., Schweneker D., Feuerstein U., Gentsch N., von Wirén N. (2023). Distinct metabolite classes in root exudates are indicative for field- or hydroponically-grown cover crops. Front. Plant Sci. 14. doi: 10.3389/fpls.2023.1122285
Heuermann D., Gentsch N., Boy J., Schweneker D., Feuerstein U., Groß J., et al. (2019). Interspecific competition among catch crops modifies vertical root biomass distribution and nitrate scavenging in soils. Sci. Rep. 9, 11531. doi: 10.1038/s41598-019-48060-0
Heuermann D., Gentsch N., Guggenberger G., Reinhold-Hurek B., Schweneker D., Feuerstein U., et al. (2022). Catch crop mixtures have higher potential for nutrient carry-over than pure stands under changing environments. Eur. J. Agron. 136, 126504. doi: 10.1016/j.eja.2022.126504
Hoffman M. L., Regnier E. E., Cardina J. (1993). Weed and Corn (Zea mays) Responses to a Hairy Vetch (Vicia villosa) Cover Crop. Weed Technol. 7, 594–599. doi: 10.1017/S0890037X00037398
Hooper D. U., Dukes J. S. (2004). Overyielding among plant functional groups in a long-term experiment. Ecol. Lett. 7, 95–105. doi: 10.1046/j.1461-0248.2003.00555.x
Ilgen B. (1990). Wachstumsverlauf und N-Aufnahme verschiedener Zwischenfruchtarten in Abhängigkeit vom NO3-N Angebot im Boden. Eidgenössische technische Hochschule Zürich, Zürich. Dissertation.
IUSS Working Group WRB (2015). World reference base for soil resources 2014: International soil classification system for naming soils and creating legends for soil maps (Rome: FAO).
Kathage J., Smit B., Janssens B., Haagsma W., Adrados J. L. (2022). How much is policy driving the adoption of cover crops? Evidence four EU regions. Land Use Policy 116, 106016. doi: 10.1016/j.landusepol.2022.106016
Kaye J. P., Quemada M. (2017). Using cover crops to mitigate and adapt to climate change. A review. Agron. Sustain. Dev. 37. doi: 10.1007/s13593-016-0410-x
Klages S., Aue C., Reiter K., Heidecke C., Osterburg B. (2022). Catch crops in lower saxony—More than 30 years of action against water pollution with nitrates: all in vain? Agriculture 12, 447. doi: 10.3390/agriculture12040447
Kottek M., Grieser J., Beck C., Rudolf B., Rubel F. (2006). World Map of the Köppen-Geiger climate classification updated. metz 15, 259–263. doi: 10.1127/0941-2948/2006/0130
Koudahe K., Allen S. C., Djaman K. (2022). Critical review of the impact of cover crops on soil properties. Int. Soil Water Conserv. Res. 1–12. doi: 10.1016/j.iswcr.2022.03.003
Kozak M., Piepho H.-P. (2018). What’s normal anyway? Residual plots are more telling than significance tests when checking ANOVA assumptions. J. Agron. Crop Sci. 204, 86–98. doi: 10.1111/jac.12220
Krato C., Petersen J. (2012). Competitiveness and yield impact of volunteer oilseed rape (Brassica napus) in winter and spring wheat (Triticum aestivum). J Plant Dis Prot. 119, 74–82. doi: 10.1007/BF03356423
Kümmerer R., Noack P. O., Bauer B. (2023). Using high-resolution UAV imaging to measure canopy height of diverse cover crops and predict biomass. Remote Sens. 15, 1520. doi: 10.3390/rs15061520
Lavergne S., Vanasse A., Thivierge M.-N., Halde C. (2021). Using fall-seeded cover crop mixtures to enhance agroecosystem services: A review. Agrosyst. Geosci. Environ. 4. doi: 10.1002/agg2.20161
Lemessa F., Wakjira M. (2014). Mechanisms of ecological weed management by cover cropping: A review. J. @ Biol. Sci. 14, 452–459. doi: 10.3923/jbs.2014.452.459
Lenth R. V. (2017). emmeans: Estimated Marginal Means, aka Least-Squares Means. doi: 10.32614/CRAN.package.emmeans
Loreau M., Hector A. (2001). Partitioning selection and complementarity in biodiversity experiments. Nature 412, 72–76. doi: 10.1038/35083573
Lüdecke D., Ben-Shachar M., Patil I., Waggoner P., Makowski D. (2021). Performance: an R package for assessment, comparison and testing of statistical models. . JOSS 6, 3139. doi: 10.21105/joss.03139
MacLaren C., Swanepoel P., Bennett J., Wright J., Dehnen-Schmutz K. (2019). Cover crop biomass production is more important than diversity for weed suppression. Crop Sci. 59, 733–748. doi: 10.2135/cropsci2018.05.0329
Meier U. (2018). Entwicklungsstadien mono- und dikotyler Pflanzen: BBCH Monografie. Open Agrar Repositorium. 23–137. doi: 10.5073/20180906-075119
Patkowska E., Konopiński M. (2014). Occurrence of antagonistic fungi in the soil after cover crops cultivation. Plant Soil Environ. 60, 204–209. doi: 10.17221/67/2014-PSE
Peltonen-Sainio P., Jauhiainen L., Känkänen H. (2023). Finnish farmers feel they have succeeded in adopting cover crops but need down-to-earth support from research. Agronomy 13, 2326. doi: 10.3390/agronomy13092326
Poeplau C., Don A. (2015). Carbon sequestration in agricultural soils via cultivation of cover crops – A meta-analysis. Agriculture Ecosyst. Environ. 200, 33–41. doi: 10.1016/j.agee.2014.10.024
Ramirez-Garcia J., Gabriel J. L., Alonso-Ayuso M., Quemada M. (2015). Quantitative characterization of five cover crop species. J. Agric. Sci. 153, 1174–1185. doi: 10.1017/S0021859614000811
Ranaldo M., Carlesi S., Costanzo A., Bàrberi P. (2020). Functional diversity of cover crop mixtures enhances biomass yield and weed suppression in a Mediterranean agroecosystem. Weed Res. 60, 96–108. doi: 10.1111/wre.12388
Raveneau M. P., Coste F., Moreau-Valancogne P., Lejeune-Hénaut I., Durr C. (2011). Pea and bean germination and seedling responses to temperature and water potential. Seed Sci. Res. 21, 205–213. doi: 10.1017/S0960258511000067
R Core Team (2021). R: a language and environment for statistical computing (Vienna: Foundation for Statistical Computing).
Reiss E. R., Drinkwater L. E. (2020). Ecosystem service delivery by cover crop mixtures and monocultures is context dependent. Agron.j. 112, 4249–4263. doi: 10.1002/agj2.20287
Restovich S. B., Andriulo A. E., Armas-Herrera C. M., Beribe M. J., Portela S. I. (2019). Combining cover crops and low nitrogen fertilization improves soil supporting functions. Plant Soil 442, 401–417. doi: 10.1007/s11104-019-04205-8
Riemens M., Sønderskov M., Moonen A.-C., Storkey J., Kudsk P. (2022). An Integrated Weed Management framework: A pan-European perspective. Eur. J. Agron. 133, 126443. doi: 10.1016/j.eja.2021.126443
Roarty S., Hackett R. A., Schmidt O. (2017). Earthworm populations in twelve cover crop and weed management combinations. Appl. Soil Ecol. 114, 142–151. doi: 10.1016/j.apsoil.2017.02.001
Ruis S. J., Blanco-Canqui H., Elmore R. W., Proctor C., Koehler-Cole K., Ferguson R. B., et al. (2020). Impacts of cover crop planting dates on soils after four years. Agron.j. 112, 1649–1665. doi: 10.1002/agj2.20143
Schappert A., Linn A. I., Sturm D. J., Gerhards R. (2019a). Weed suppressive ability of cover crops under water-limited conditions. Plant Soil Environ. 65, 541–548. doi: 10.17221/516/2019-PSE
Schappert A., Messelhäuser M., Saile M., Peteinatos G., Gerhards R. (2018). Weed suppressive ability of cover crop mixtures compared to repeated stubble tillage and glyphosate treatments. Agriculture 8, 144. doi: 10.3390/agriculture8090144
Schappert A., Schumacher M., Gerhards R. (2019b). Weed control ability of single sown cover crops compared to species mixtures. Agronomy 9, 294. doi: 10.3390/agronomy9060294
Schmidt J. H., Junge S., Finckh M. R. (2019). Cover crops and compost prevent weed seed bank buildup in herbicide-free wheat-potato rotations under conservation tillage. Ecol. Evol. 9, 2715–2724. doi: 10.1002/ece3.4942
Schön J., Gentsch N., Breunig P. (2024). Cover crops support the climate change mitigation potential of agroecosystems. PloS One 19, e0302139. doi: 10.1371/journal.pone.0302139
Schütz L., Lettow N., Dachbrodt-Saaydeh S. (2020). Vorbeugende Pflanzenschutzmaßnahmen im Ackerbau und ihre Eignung zur Förderung von Arthropoden. Journal für Kulturpflanzen 72 (12), 545–560. doi: 10.5073/JFK.2020.12.01
Seitz D., Fischer L. M., Dechow R., Wiesmeier M., Don A. (2022). The potential of cover crops to increase soil organic carbon storage in German croplands. Plant Soil. doi: 10.1007/s11104-022-05438-w
Sieling K. (2019). Improved N transfer by growing catch crops – a challenge. Journal für Kulturpflanzen. 71 (6), 145–160. doi: 10.5073/JFK.2019.06.01
Smith R. G., Atwood L. W., Warren N. D. (2014). Increased productivity of a cover crop mixture is not associated with enhanced agroecosystem services. PloS One 9, e97351. doi: 10.1371/journal.pone.0097351
Smith R. G., Warren N. D., Cordeau S. (2020). Are cover crop mixtures better at suppressing weeds than cover crop monocultures? Weed Sci. 68, 186–194. doi: 10.1017/wsc.2020.12
Storr T., Simmons R. W., Hannam J. A. (2019). A UK survey of the use and management of cover crops. Ann. Appl. Biol. 174, 179–189. doi: 10.1111/aab.12488
Tadiello T., Potenza E., Marino P., Perego A., Della Torre D., Michelon L., et al. (2022). Growth, weed control, and nitrogen uptake of winter-killed cover crops, and their effects on maize in conservation agriculture. Agron. Sustain. Dev. 42, 1–15. doi: 10.1007/s13593-021-00747-3
Tribouillois H., Cruz P., Cohan J.-P., Justes É. (2015a). Modelling agroecosystem nitrogen functions provided by cover crop species in bispecific mixtures using functional traits and environmental factors. Agriculture Ecosyst. Environ. 207, 218–228. doi: 10.1016/j.agee.2015.04.016
Tribouillois H., Dürr C., Demilly D., Wagner M.-H., Justes É. (2016). Determination of germination response to temperature and water potential for a wide range of cover crop species and related functional groups. PloS One 11, e0161185. doi: 10.1371/journal.pone.0161185
Tribouillois H., Fort F., Cruz P., Charles R., Flores O., Garnier E., et al. (2015b). A functional characterisation of a wide range of cover crop species: growth and nitrogen acquisition rates, leaf traits and ecological strategies. PloS One 10, e0122156. doi: 10.1371/journal.pone.0122156
Wagg C., van Erk A., Fava E., Comeau L.-P., Mitterboeck T. F., Goyer C., et al. (2021). Full-season cover crops and their traits that promote agroecosystem services. Agriculture 11, 830. doi: 10.3390/agriculture11090830
Wendling M., Büchi L., Amossé C., Jeangros B., Walter A., Charles R. (2017). Specific interactions leading to transgressive overyielding in cover crop mixtures. Agriculture Ecosyst. Environ. 241, 88–99. doi: 10.1016/j.agee.2017.03.003
Wendling M., Büchi L., Amossé C., Sinaj S., Walter A., Charles R. (2016). Influence of root and leaf traits on the uptake of nutrients in cover crops. Plant Soil 409, 419–434. doi: 10.1007/s11104-016-2974-2
Wendling M., Charles R., Herrera J. M., Amossé C., Jeangros B., Walter A., et al. (2019). Effect of species identity and diversity on biomass production and its stability in cover crop mixtures. Agriculture Ecosyst. Environ. 281, 81–91. doi: 10.1016/j.agee.2019.04.032
Wilcoxen C. A., Walk J. W., Ward M. P. (2018). Use of cover crop fields by migratory and resident birds. Agriculture Ecosyst. Environ. 252, 42–50. doi: 10.1016/j.agee.2017.09.039
Wilson R., Culp D., Peterson S., Nicholson K., Geisseler D. (2019). Cover crops prove effective at increasing soil nitrogen for organic potato production. Calif Agr 73, 79–89. doi: 10.3733/ca.2019a0005
Wittwer R. A., Dorn B., Jossi W., van der Heijden M. G. A. (2017). Cover crops support ecological intensification of arable cropping systems. Sci. Rep. 7, 41911. doi: 10.1038/srep41911
Wnuk A., Wojciechowicz-zytko E. (2010). Effect of intercropping of broad bean (Vicia faba L.) with tansy phacelia (Phacelia tanacetifolia Benth.) on the occurrence of Aphis fabae Scop. and predatory Syrphidae. Aphids And Other Hemipterous Insects 13, 211–217. Available at: http://knsos.student.kul.lublin.pl/files/323/24wnukwojciechowicz.pdf.
Wortman S. E., Francis C. A., Lindquist J. L. (2012). Cover crop mixtures for the western corn belt: opportunities for increased productivity and stability. Agron.j. 104, 699–705. doi: 10.2134/agronj2011.0422
Keywords: interspecific interaction, integrated weed management, cruciferous species, legumes, phacelia, sustainable crop production, binary mixtures, catch crops
Citation: Groß J, Kümmerer R, Heuermann D, Gentsch N, Schweneker D, Feuerstein U, Guggenberger G, von Wirén N and Bauer B (2024) Improving dual cover crop mixtures to increase shoot biomass production and weed suppression potential. Front. Agron. 6:1416379. doi: 10.3389/fagro.2024.1416379
Received: 12 April 2024; Accepted: 05 August 2024;
Published: 03 September 2024.
Edited by:
Walter Daniel Carciochi, National University of Mar del Plata, ArgentinaReviewed by:
Daniele Antichi, University of Pisa, ItalyIoannis Roussis, Agricultural University of Athens, Greece
Copyright © 2024 Groß, Kümmerer, Heuermann, Gentsch, Schweneker, Feuerstein, Guggenberger, von Wirén and Bauer. This is an open-access article distributed under the terms of the Creative Commons Attribution License (CC BY). The use, distribution or reproduction in other forums is permitted, provided the original author(s) and the copyright owner(s) are credited and that the original publication in this journal is cited, in accordance with accepted academic practice. No use, distribution or reproduction is permitted which does not comply with these terms.
*Correspondence: Bernhard Bauer, QmVybmhhcmQuYmF1ZXJAaHN3dC5kZQ==; Jonas Groß, ai5ncm9zc0BrdGJsLmRl
†ORCID: Jonas Groß, orcid.org/0000-0001-9628-1609
Robin Kümmerer, orcid.org/0000-0002-9824-5692
Diana Heuermann, orcid.org/0000-0001-8664-0559
Norman Gentsch, orcid.org/0000-0003-1166-8973
Georg Guggenberger, orcid.org/0000-0002-6962-8264
Nicolaus von Wirén, orcid.org/0000-0002-4966-425X
Bernhard Bauer, orcid.org/0009-0000-9277-1512