- Escuela Profesional de Ingeniería Agrónoma, Universidad Nacional Autónoma de Alto Amazonas (UNAAA), Yurimaguas, Peru
Cowpea production in the Peruvian Amazon is increasing due to its ability to adapt to diverse environments, its contribution to soil conservation, and its versatility of uses. This crop is particularly valuable in human nutrition due to its high nutritional quality and nutraceutical properties of its bioactive compounds. The aim of the study was to evaluate the physiology of cowpea plants in accessions PER1005854, PER1005851, and PER12645, under the influence of rice husk mulch, screw tree litter, and oil palm fiber, as well as to determine the bioactive compounds present in the grains. This study aims to promote sustainable cowpea production, expose the nutritional aspects of the grains, and foster their consumption both locally and nationally. The experiment was conducted from November 2022 to March 2023, in Yurimaguas, Loreto, Peru. The application of organic mulches demonstrates a significantly positive impact on the growth of cowpea accessions during their vegetative phase. This effect is possibly attributed to increased water availability, as the mulch reduces soil evaporation. In particular, cowpea accessions PER12645 and PER1005851 exhibit desirable physiological characteristics for grain production, with short cycles of 71 days and higher yields of 1141 and 1125 kg/ha respectively. In contrast, accession PER1005854 is distinguished by its higher biomass production, reaching a value of 14497 kg/ha, a relevant trait for foliage production and its contribution to soil conservation. The bioactive compounds, such as proteins, lipids, and carbohydrates, present in the accessions PER1005854, PER1005851, and PER12645, are similar to those found in other legumes. However, the antioxidant activity of dark tegument cowpeas proved to be superior, particularly highlighted in accession PER12645 (black tegument) with 26.3 μmolTG/g, and in accession PER1005854 (dark red tegument) with 19.5 μmolTG/g. This characteristic is particularly important for consumption, as it is related to the ability to combat oxidative stress in the human body.
1 Introduction
Grain legumes are the most important cultivated species globally after cereals (Kebede and Bekeko, 2020). In Peru, grain legumes are a food source in over 8 million households at least once weekly (INEI, 2017). Peru boasts a wide diversity of agroclimatic zones along its coast, highlands, and Amazon, enabling the production of various crops, especially grain legumes, which are essential for the subsistence of small-scale Peruvian farmers.
In the Peruvian Amazon, Vigna unguiculata (cowpea) is the most cultivated grain legume, with over 100 accessions of different colours, sizes, and shapes, yielding between 1.2 to 2.5 t/ha (INIA, 2022). Cowpea provides ground cover, fixes nitrogen, and controls weeds; therefore, it is a viable long-term productive option for soil fertility enhancement (Kyei-Boahen et al., 2017; Beshir et al., 2019; Murga-Orrillo et al., 2023). In other words, cowpea contributes to the restoration of degraded soils; its grains and foliage offer bioactive compounds with nutritional and nutraceutical benefits for human and livestock nutrition.
The bioactive compounds in cowpea grain include phenolic compounds such as catechin, epicatechin, gallic acid, and ferulic acid, which exhibit antioxidant properties (Moreira-Araújo et al., 2018); these compounds are found in higher concentrations in pigmented cowpea varieties, suggesting potential health benefits (Sombié et al., 2018); also considered bioactive compounds are proteins, carbohydrates, lipids, dietary fibres, vitamins, and minerals (Boukar et al., 2019; Abebe and Alemayehu, 2022).
Cowpea is the most important source of macro and micronutrients in the human diet in many world regions, primarily tropical countries (Bialostosky et al., 2002). However, cowpea exhibits extensive genetic variability, and reports documenting differences in its bioactive compounds among accessions are limited.
Reports on the use of organic mulches in cowpea production are also scarce. These mulches act as a physical barrier that retains moisture in the soil, thereby reducing crop evapotranspiration (Jun et al., 2014; Murga-Orrillo et al., 2016). It decreases water and thermal stress on the plants by maintaining soil moisture and regulating soil temperature (Knapp and Smith, 1989). It is particularly crucial in soils exposed to high temperatures, such as those in the Peruvian Amazon, where various cowpea varieties are cultivated. A report on the use of organic mulches in cowpea production found that it significantly increases root density, shoot elongation, and grain yield (Maurya and Lal, 1981), as they influence soil mycorrhizal colonization (Zambonelli et al., 2005). Furthermore, organic mulches increase soil organic matter content (Lal et al., 2018), and their slow decomposition means that nutrients are available for a longer duration to the crop (Murga-Orrillo et al., 2019). However, organic mulches’ decomposition and nutrient availability can vary depending on their plant origin, which, in turn, can affect cowpea growth, production, and the bioactive compounds of the grains.
The hypothesis is posed that organic mulches significantly influence the physiology of three cowpea accessions. It is postulated that during their mineralization process, these mulches may enhance soil structure, increase nutrient availability, and augment water availability for cowpeas. These conditions would favour a healthier growth environment for cowpeas. Furthermore, intrinsic differences between cowpea accessions are expected to lead to phenological variations in plants and the contents of bioactive compounds in the grains. Therefore, the aim is to evaluate physiological aspects of the plant and bioactive compounds of the grain of cowpea accessions PER1005854, PER1005851, and PER12645, and analyse how different types of organic mulches influence the physiology of these accessions.
2 Materials and methods
2.1 Experimental site location
The experiment was conducted at the “Munichis” University Campus of the School of Agronomy, National Autonomous University of Alto Amazonas (UNAAA) from November 12, 2022, to March 3, 2023. The experimental area is geolocated at a longitude of 76.2308W, a latitude of 5.8925S, and an elevation of 140.3 meters (Figure 1).
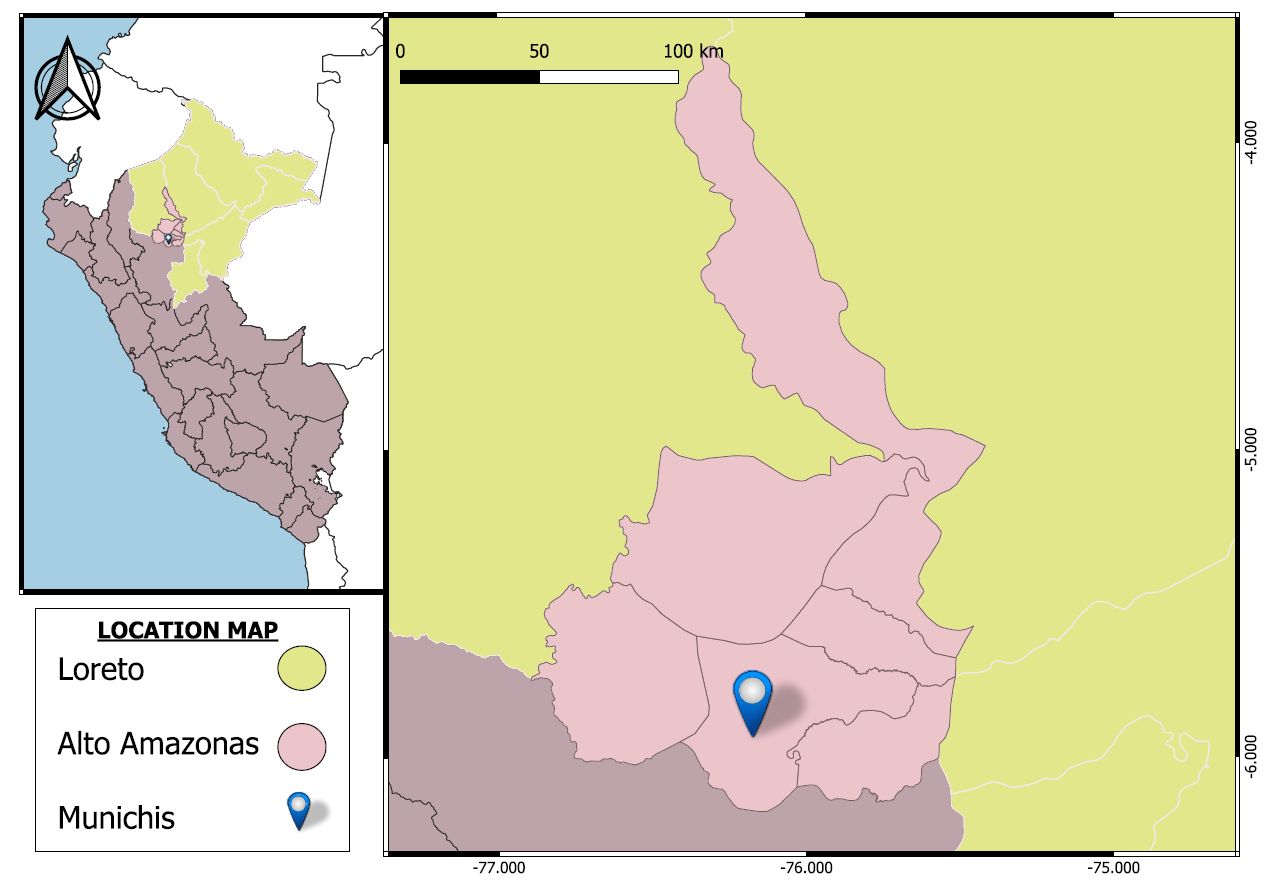
Figure 1 Location map of the experiment for cowpea accessions PER1005854 (red), PER1005851 (white with black eye), and PER12645 (black).
Climatic conditions during the study period are depicted in Figure 2A, based on climatic data from SENAMHI (2023).
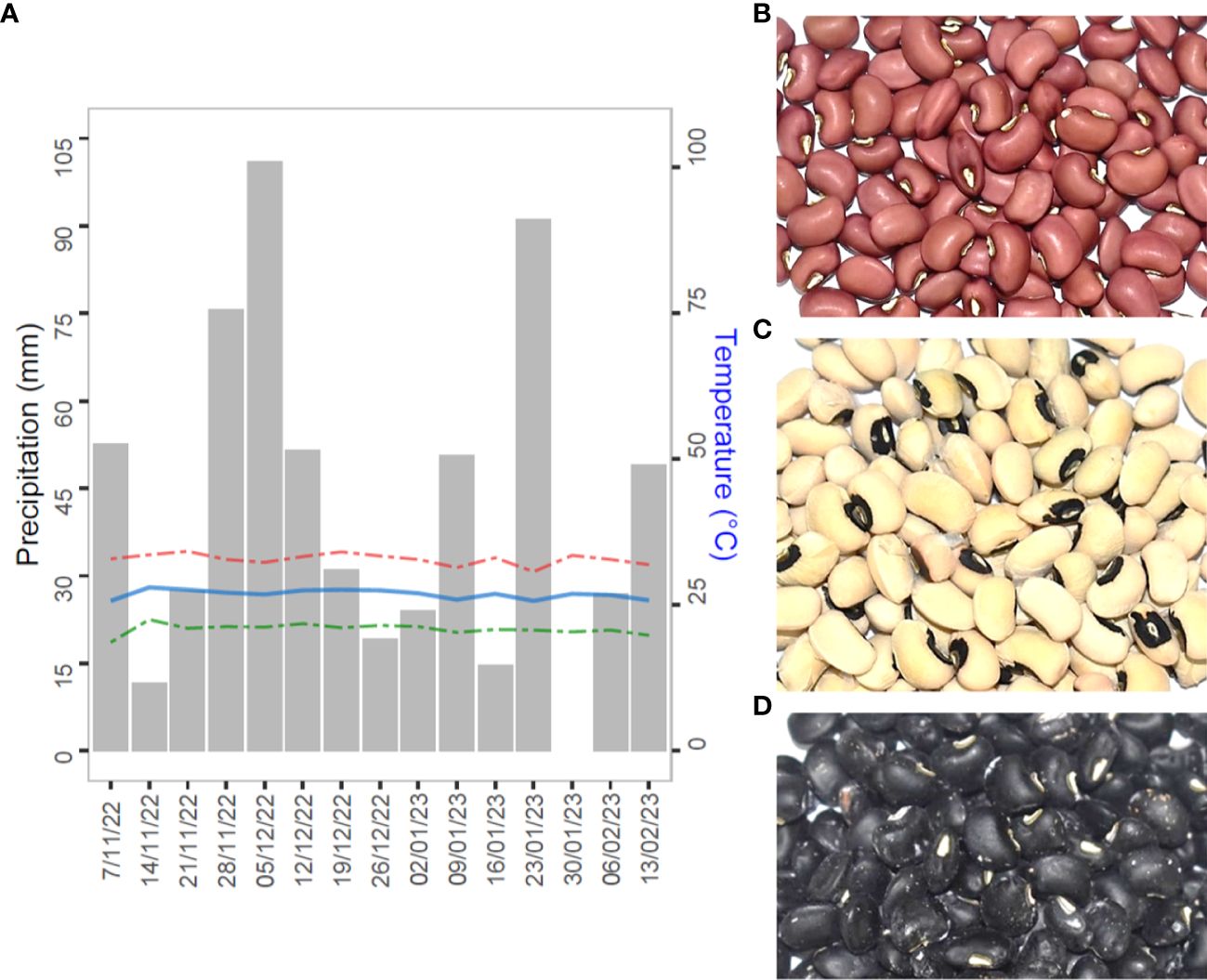
Figure 2 Weekly climate conditions for the study period. (A) Rainfall (bars), maximum temperature (red line), average temperature (blue line), and minimum temperature (green line), and cowpea accessions: (B) PER1005854 (red), (C) PER1005851 (white with black eye), and (D) PER12645 (black).
The cowpea seeds were obtained from the Germplasm Bank of the National Institute of Agricultural Innovation (INIA), Ministry of Agricultural Development and Irrigation, Peru. Accessions PER1005854 (red cowpea) (Figure 2B), PER1005851 (white cowpea with black eye) (Figure 2C), and PER12645 (black cowpea) (Figure 2C) were selected from 100 accessions for their resistance to stress factors, seed coat colour, and higher yield potential.
The total experimental area was 290 m2, each measuring 8 m2, resulting in 36 experimental units. On November 12, 2022, accession PER1005854 was sown, along with PER1005851 and PER12645, at a planting density of 200,000 plants/ha. Plants were spaced at 10x50 cm intervals, with each plot having a border area of 5 m2 and an evaluation area of 3 m2, totalling 60 plants evaluated per plot and 160 plants per experimental unit.
2.2 Soil analysis
The physical and chemical analysis of the soil was performed on a composite sample that was collected using an auger at a depth of 0 to 20 cm. Subsequently, this sample underwent air drying, followed by grinding and sieving through a 2 mm mesh. The determination of organic matter was performed following the Walkley and Black method (1934), while phosphorus (P) was analysed using the method Olsen et al. (1954).
The quantification of exchangeable cations, including magnesium (Mg2+), potassium (K+), calcium (Ca2+), and sodium (Na+), extractions were performed using a 1N potassium chloride (KCl) solution, and the results were determined by atomic absorption, following the protocol described by Hunter (1986). Nitrogen (N) content was calculated using a factor derived from the amount of organic matter in the sample.
2.3 Soil preparation
At the beginning of the soil preparation process, 7.5 t/ha of lime (CaO) was applied to counteract its acidity. This lime was carefully incorporated into the soil to a depth of 30 cm using a disc plow, which was attached to a 120-horsepower agricultural tractor (Kubota, Model: M108S, Japan). Subsequently, the experimental plots were delineated, followed by the planting of cowpea accessions. Finally, organic mulches were applied, averaging 29 t/ha.
2.4 Experimental design and arrangement
A randomized complete block design with a 3x4 factorial arrangement was employed. The first factor consisted of the cowpea accessions: PER1005854 (V1), PER1005851 (V2), and PER12645 (V3). The second factor corresponded to the types of organic mulch: M0 - control group, M1 - rice husk (Oryza sativa), M2 - screw pine litter (Cedrelinga cateneaformis), and M3 - oil palm fruit fibre (Elaeis guineensis). The treatments were distributed into three replicates, resulting in 36 experimental units.
2.5 Determination of physiological variables
A periodic assessment of plant height was performed at a frequency of 15 days after planting (DAP). The final assessment was conducted when the plants reached 50% flowering at 45 DAP for accessions PER1005851 and PER12645. However, in the case of accession PER1005854, the last assessment was done at 53 DAP, as it reached 50% flowering on that date. Physiologically mature pod harvesting occurred on January 22, 2023, for accessions PER1005851 and PER12645. As for accession PER1005854, harvesting was performed on February 9, 2023. Data such as the number of pods per plant, pod length, number of grains per pod, and grain yield were recorded during pod collection. Additionally, stubble biomass was evaluated. Aboveground plant biomass was collected and weighed using a 15 kg spring balance (Kambor brand).
2.6 Bioactive compounds
The chemical composition of the cowpea accession samples was determined following FAO standardized procedures (FAO, 1986). The determination of moisture, ash, proteins, and lipids was performed t as follows: Ash content was calculated from the remaining weight after heating the sample to 550°C. Lipid content was determined using the Soxhlet extraction method. Protein quantification was performed using the Kjeldahl method, applying a nitrogen-to-protein conversion factor of 5.40. Carbohydrate content was determined by difference, subtracting total solids from the contents of proteins, lipids, ash, and dietary fibre. Carbohydrate quantification was measured through polarimetry, following official procedures (Official, 1993). Total phenols measurement was conducted following the protocol of Xu and Chang (2009), and analyses were performed using high-performance liquid chromatography (HPLC). An ethanol extract and a 0.1% DPPH solution in methanol were used to determine antioxidant activity. The quantification was performed using a UV-Vis spectrophotometer, following the methodology of Benzie and Strain (1999).
2.7 Statistical data analysis
Data were assessed for normal distribution and variance homogeneity before conducting variance analysis (ANOVA) using the F-test. Subsequently, mean comparison tests were performed using the Tukey method. These assessments were conducted with a significance level of p<5%. In cases where data did not meet the criteria for normal distribution, a multivariate statistical approach, specifically Principal Component Analysis (PCA), was applied. Statistical differences were determined using confidence intervals (CI: 95%) for variables explaining the most variability in PCA. All these statistical analyses were determined using the R studio statistical software (R Core Team, 2023).
3 Results
The cowpea accession PER1005854 (Figure 2B) exhibited an indeterminate growth habit and was characterized by a 4-day germination period, 49 days in the vegetative phase, and 36 days in the reproductive phase. It resulted in a complete crop cycle spanning a total of 89 days. In contrast, cowpea accessions PER1005851 and PER12645 (Figures 2C, D) displayed a determinate growth habit, with a 4-day germination period, 41 days in the vegetative phase, and 26 days in the reproductive phase. Collectively, the complete cycles of these accessions extended over a total of 71 days.
Table 1 presents the analysis results of soil physical and chemical properties. This soil is characterized by limited fertility, with high acidity (pH 4.9) due to the presence of Al³+ (5.9 cmolc/kg). The soil belongs to the loamy-sandy texture class, with a sand content of 65.2%, silt content of 12.8%, and clay content of 21.0%.
The mean squares obtained through the ANOVA for the evaluated variables are detailed in Table 2. This table shows significant differences (p<0.05) in plant height during the vegetative phase concerning the types of organic mulches. Significant differences were also recorded during the flowering stage for the cowpea accessions. Likewise, significant differences were identified in the number of pods, pod length, number of grains per pod, yield, and stubble biomass.
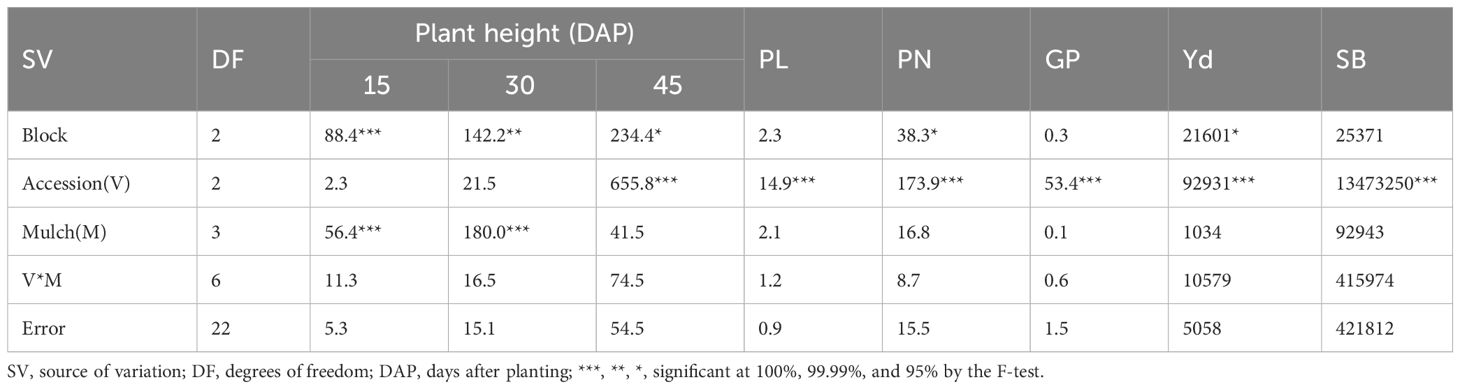
Table 2 Analysis of variance (ANOVA) mean squares for plant height, pod length (PL), pod number (PN), grains per pod (GP), yield (Yd), and straw biomass (SB) of cowpea accessions PER1005854 (red), PER1005851 (white with black eye), and PER12645 (black).
In the mean comparison test conducted using Tukey (p<0.05), significant differences were observed in various aspects. Significant differences were recorded during the vegetative phase (Figures 3A, B) for organic mulches, which exhibited higher plant height values than the control group. However, in the flowering stage (Figure 3C), accessions PER1005854 and PER1005851 had significantly taller plant heights than accession PER12645. On the other hand, accessions PER1005851 and PER12645 displayed a higher number of pods per plant and higher grain yield compared to accession PER1005854 (Figures 3D, G). In contrast, accession PER1005854 showed longer pod lengths and higher stubble biomass than accessions PER1005851 and PER12645 (Figures 3E, H). Regarding the number of grains per pod, accession PER12645 had the highest figure, followed by accession PER1005854 and accession PER1005851.
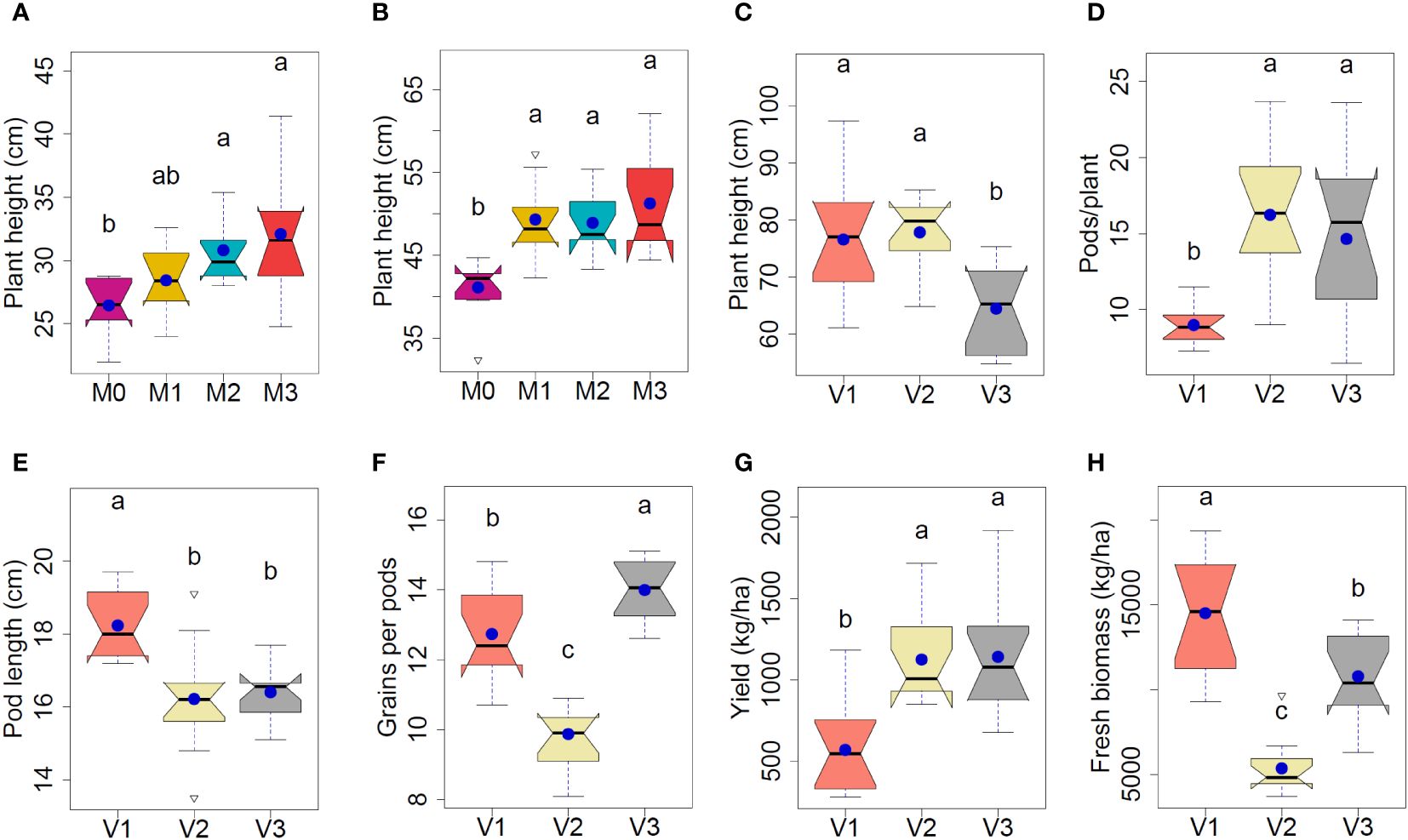
Figure 3 Plant height at 15 (A), 30 (B), and 45 (C) days after planting, pods per plant (D), pod length (E), grains per pod (F), yield (G), and fresh straw biomass (H). For mulch: M0- control, M1- rice husk, M2- screw leaf litter, M3- oil palm fruit fibre; and cowpea accessions: V1- PER1005854 (red), V2- PER1005851 (white with black eye), and V3- PER12645 (black). Lowercase letters above the boxplots indicate no significant differences between them according to the Tukey test (p<0.05).
In the PCA conducted on the measurements of seeds from different cowpea accessions (Figure 4A), in dimension 1 of the PCA explaining 54.5% of the variability, a clear differentiation in seed size of accession PER12645 is evident, with smaller dimensions in terms of length and width compared to accessions PER1005854 and PER1005851. This difference is confirmed when observing the analysis with 95% confidence intervals (CI) specifically for seed length (Figure 4B), where accessions PER1005851 and PER1005854 exhibit larger seeds than accession PER12645. However, regarding seed width and thickness, no significant differences were detected among the three evaluated accessions (Figures 4C, D). It is important to note that the smaller size of seeds in accession PER12645 (Figures 4A, B) is compensated by a higher number of grains per pod (Figure 3F) and superior yield, similar to accession PER1005851 (Figure 3G).
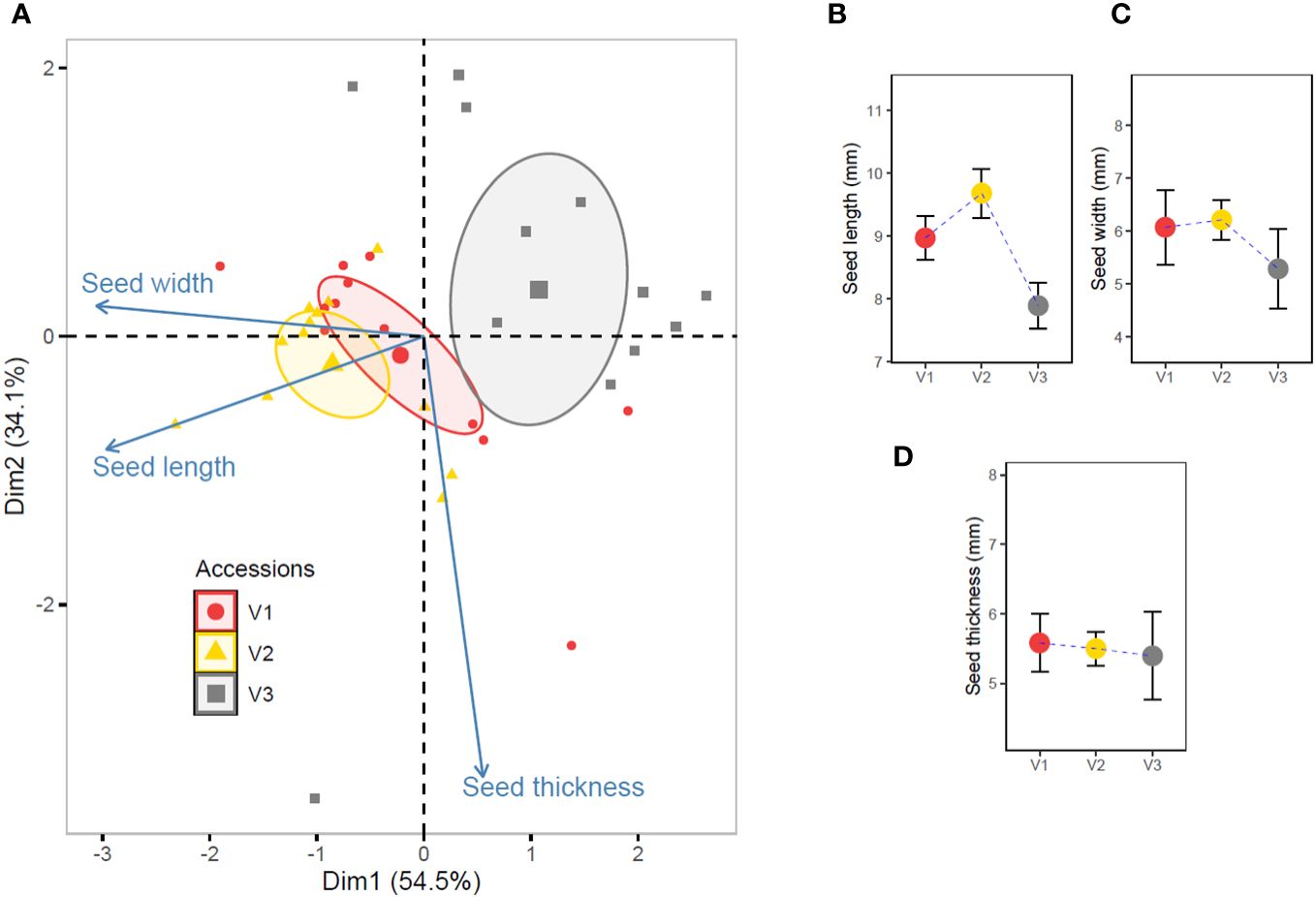
Figure 4 Principal component analysis (PCA) (A) and 95% confidence intervals (CI: 95%) for seed length (B), width (C), and thickness (D) of the accessions: cowpea accessions V1- PER1005854 (red), V2- PER1005851 (white with black eye), and V3- PER12645 (black).
The variations in bioactive compounds present in the grains of different cowpea accessions analysed through PCA are depicted in Figure 5A. In this figure, in dimension 1 of the PCA explaining 41.9% of the variability, a clear differentiation in energy and carbohydrate content is observed, with higher levels in accession PER1005851, in contrast to the levels of antioxidant activity, which are higher in accessions PER1005854 and PER12645. Upon conducting a more detailed analysis through 95% confidence intervals (CI), it was determined that accession PER12645 exhibits higher antioxidant activity, followed by accession PER1005854 and, finally, accession PER1005851 (Figures 5I, J). However, no significant differences were observed regarding moisture, ash, carbohydrates, proteins, lipids, energy, and total phenols content for the three accessions, as illustrated in Figures 5B, 5D–H.
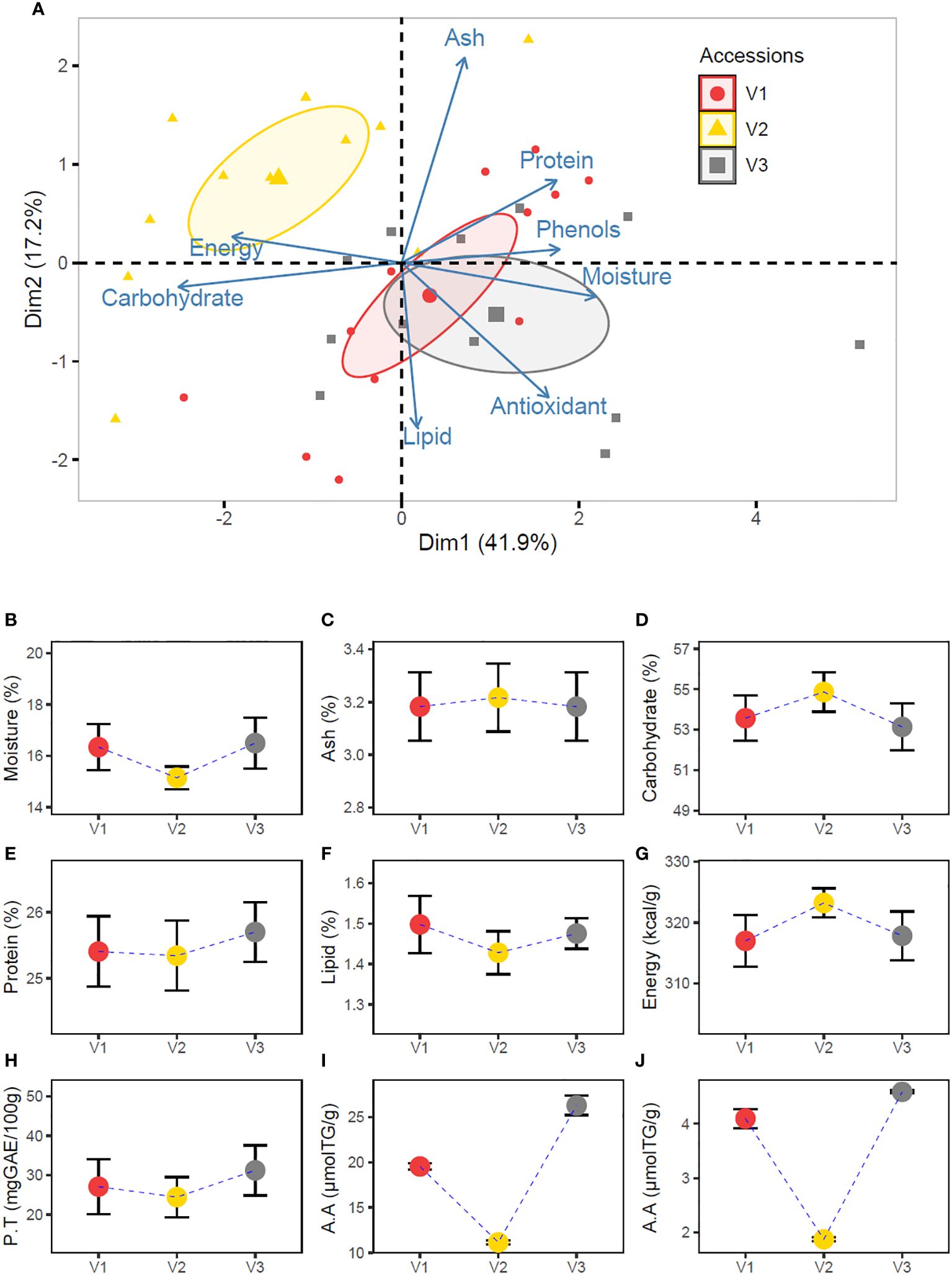
Figure 5 Principal component analysis (PCA) (A) and 95% confidence intervals (CI: 95%) for moisture (B), ash (C), carbohydrates (D), proteins (E), lipids (F), energy (G), total phenols (H), A.A-ABTS antioxidant activity (I), and A.A-DPPH antioxidant activity (J) of the grains of cowpea accessions V1- PER1005854 (red), V2- PER1005851 (white with black eye), and V3- PER12645 (black).
4 Discussion
Understanding the edaphoclimatic conditions of cowpea accessions enables the anticipation of optimizing growth and development, yield, and the quality of both foliage and grain, the selection of suitable accessions, phytosanitary prediction and control, resource use efficiency, and adaptation to climate change. With climatic conditions characterized by maximum average temperatures of 32.9°C, a minimum of 20.9°C, and a mean of 26.8°C (Figure 2A), along with a study period rainfall of 626.9 mm, and acidic, low-fertility soil (Table 1), crop cycles of 89 days were achieved for accession PER1005854, and 71 days for accessions PER1005851 and PER12645. Toudou-Daouda et al. (2018) reported crop cycles of 90 days for cowpea accessions ISV128, IT93K-503-1, IT96D-610, Suvita2, and Tiligré. Favourable cowpea climatic conditions typically include mean temperatures ranging from 25 to 35°C, annual rainfall between 750 to 1100 mm, and diverse soils (Drahansky et al., 2016).
According to the F-test, no significant effects on cowpea accession yields were observed in the organic mulches (Table 2). In a previous study, seven different mulch doses were evaluated, and no significant influence on cowpea yield was found either (Mupangwa et al., 2012), suggesting that cowpea production remains consistent under diverse conditions. However, the mulches demonstrated a significant impact (p<0.05) on the growth of cowpea accessions during the vegetative phase (Figures 3A, B). It can be inferred that the mulches contributed to maintaining higher soil moisture, providing greater water availability for cowpea. This was reflected in significant differences in plant height when compared to the control group (Figures 3A, B). It is important to note that cowpea experienced low water availability due to minimal rainfall until 21 days (Figure 2A). Additionally, the tropical climate and soil with a 65.2% sand content will likely increase water evaporation and infiltration in the control group, leading to water limitation for the crop and resulting in shorter plants. Murga-Orrillo et al. (2016) observed that cowpea (cv. Novaera) in the initial growth phase had an evapotranspiration rate of 1.0 mm/day when cultivated with mulch, compared to 1.7 mm/day when cultivated without mulch. It translates to a 70% increase in water availability for cowpeas when cultivated with mulch.
Understanding the agronomic characteristics of cowpea accessions PER1005854, PER1005851, and PER12645 is essential to support producer decisions, agricultural activity planning, yield projections, and production goals. We highlight the agronomic characteristics relevant to grain production in accessions PER12645 and PER1005851, which are characterized by their determinate growth habit, a 71-day cycle, small pods (Figure 3E), higher yield (Figure 3G), and lower straw biomass production (Figure 3H). Additionally, accession PER12645 is distinguished by having smaller seeds (Figures 4A, B). In contrast, accession PER1005854 exhibits an indeterminate growth habit with lower yields but higher straw biomass production (Figures 3G, H). These differences are fundamental as the cowpea’s growth habit influences crop yield and conditions, tillage techniques, and other agricultural practices (Gumede et al., 2022). Furthermore, qualitative traits of cowpea, such as growth habits, seed size, shape, and seed coat colour, are also important because of their implications for farmer and consumer preferences (Nkhata et al., 2020).
The bioactive compounds in cowpea grains are relevant regarding nutritional quality for human health and sustainable agricultural production. When analysing the data represented in Figures 5B-H and Table 3, it can be observed that most bioactive compounds in cowpea accessions exhibit comparable values. Regarding protein content, cowpea accessions show higher levels compared to those found in lentils (Lens culinaris) and common beans (Phaseolus vulgaris), being similar to values recorded in broad beans (Vicia faba) but lower than those observed in soybeans (Glycine max). A similar pattern is observed regarding lipid content, where values resemble those of lentils, broad beans, and common beans but are lower than soybeans. Lastly, regarding carbohydrate content, cowpea accessions have higher levels than soybeans, similar to those obtained for cowpea beans but lower than those found in lentils and broad beans.
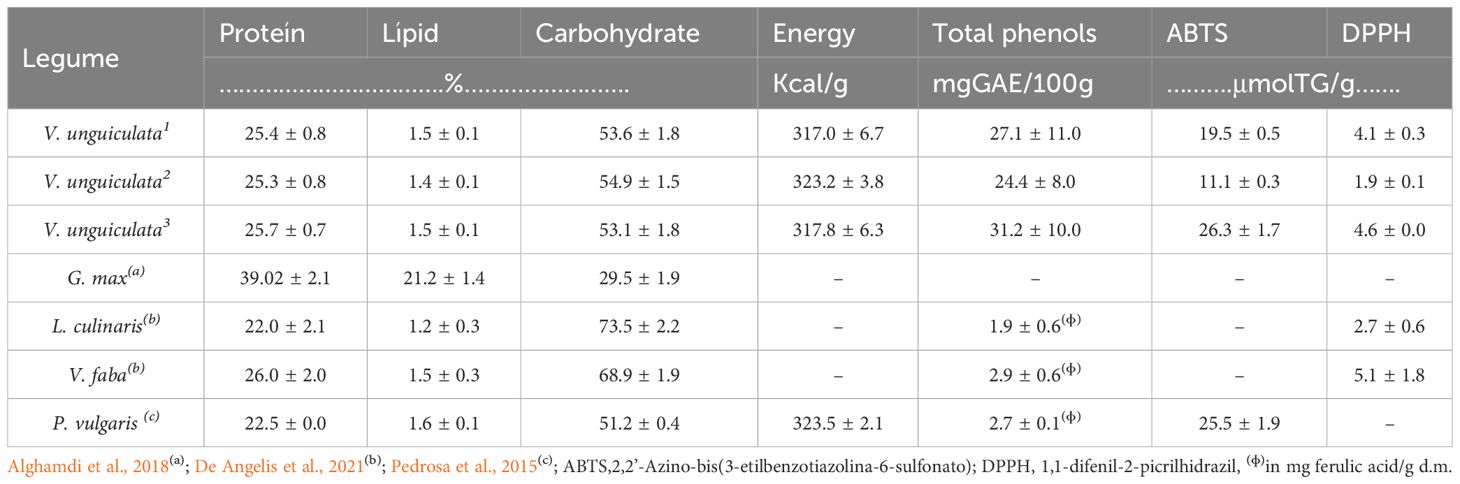
Table 3 Bioactive compounds of V. unguiculata, accessions PER1005854 (red)1, PER1005851 (white with black eye)2, and PER12645 (black)3, in comparison with bioactive compounds in soybean, lentil, broad bean, and common bean.
The antioxidant activity of cowpea accession PER12645 is higher in relation to the other accessions, as evidenced in Figures 5I, J. This phenomenon could be related to black-coloured grain coats (Figure 2D). It has been observed that cowpea accessions with darker-toned grain coats tend to exhibit higher antioxidant potential, even though their protein content may be lower, as documented by Tzanova et al. (2023). Interestingly, in this study, cowpea accession PER12645 slightly had higher protein levels than accessions PER1005854 and PER1005851, which have lighter-toned grain coats (Table 3, Figures 2B, C). According to the research of Kumar et al. (2013), epidemiological studies have revealed that consuming foods with higher antioxidant activity can contribute to protection against human diseases associated with oxidative stress. In this context, cowpea seeds are emerging as a significant source of antioxidants (Adeyemi and Olorunsanya, 2012). It is essential to highlight that the ability of cowpea accessions to adapt to stressful abiotic conditions is related to the production of phenolic acids and higher antioxidant capacity (Makaure et al., 2023).
5 Conclusions
The application of organic mulches demonstrates a significantly positive impact on the growth of cowpea accessions during their vegetative phase. This effect is possibly attributed to increased water availability, as the mulch reduces soil evaporation.
In particular, cowpea accessions PER12645 and PER1005851 exhibit desirable physiological characteristics for grain production, with short cycles of 71 days and higher yields of 1141 and 1125 kg/ha respectively. In contrast, accession PER1005854 is distinguished by its higher biomass production, reaching a value of 14497 kg/ha, a relevant trait for foliage production and its contribution to soil conservation.
The grains of accession PER12645 exhibit superior antioxidant activity of 26.3 μmolTG/g, which is associated with the black colour of its seed coat. This characteristic is essential for human health if this accession is consumed.
Data availability statement
The original contributions presented in the study are included in the article/supplementary material. Further inquiries can be directed to the corresponding author.
Author contributions
HM: Conceptualization, Data curation, Formal analysis, Investigation, Methodology, Supervision, Visualization, Writing – original draft, Writing – review & editing. JC: Conceptualization, Data curation, Writing – original draft. LA: Funding acquisition, Project administration, Visualization, Writing – original draft, Writing – review & editing.
Funding
The author(s) declare financial support was received for the research, authorship, and/or publication of this article. The authors are deeply grateful the Research Institute of the UNAAA for financing the research.
Acknowledgments
We appreciate the support and collaboration with the genetic material to the National Institute of Agrarian Innovation (INIA) through the San Ramon Agrarian Experimental Station and the Investment Project “Improvement of Research Services in the Characterization of Genetic Resources of Agrobiodiversity in 17 Departments of Peru - ProAgrobio with CUI 2480490.
Conflict of interest
The authors declare that the research was conducted in the absence of any commercial or financial relationships that could be construed as a potential conflict of interest.
Publisher’s note
All claims expressed in this article are solely those of the authors and do not necessarily represent those of their affiliated organizations, or those of the publisher, the editors and the reviewers. Any product that may be evaluated in this article, or claim that may be made by its manufacturer, is not guaranteed or endorsed by the publisher.
References
Abebe B. K., Alemayehu M. T. (2022). A review of the nutritional use of cowpea (Vigna unguiculata L. Walp) for human and animal diets. J. Agric. Food Res. 10, 100383. doi: 10.1016/j.jafr.2022.100383
Adeyemi K. D., Olorunsanya A. O. (2012). Comparative analysis of phenolic composition and antioxidant effect of seed coat extracts of four cowpea (Vigna unguiculata) varieties on broiler meat. Iranian J. Appl. Anim. Sci. 2, 343–349.
Alghamdi S. S., Khan M. A., El-Harty E. H., Ammar M. H., Farooq M., Migdadi H. M. (2018). Comparative phytochemical profiling of different soybean (Glycine max (L.) Merr) genotypes using GC–MS. Saudi J. Biol. Sci. 25, 15–21. doi: 10.1016/j.sjbs.2017.10.014
Benzie I. F. F., Strain J. J. (1999). The ferric reducing ability of plasma (FRAP) as a measure of "Antioxidant power": the FRAP assay. Analytical Biochem. 239, 70–76. doi: 10.1006/abio.1996.0292
Beshir B., Amsalu B., Dagmawit T., Selamawit K., Teamir M., Bezawit Y. (2019) Cowpea production, marketing and utilization in Ethiopia. Available online at: http://www.eiar.gov.et.
Bialostosky K., Wright J. D., Kennedy-Stephenson J., McDowell M., Johnson C. L. (2002) Dietary intake of macronutrients, micronutrients, and other dietary constituents: United States 1988-94: data from the National Health Examination Survey, the National Health and Nutrition Examination Surveys, and the Hispanic Health and Nutrition Examination Survey. Available online at: https://stacks.cdc.gov/view/cdc/6454.
Boukar O., Belko N., Chamarthi S., Togola A., Batieno J., Owusu E., et al. (2019). Cowpea (Vigna unguiculata): Genetics, genomics and breeding. Plant Breed. 138, 415–424. doi: 10.1111/pbr.12589
De Angelis D., Pasqualone A., Costantini M., Ricciardi L., Lotti C., Pavan S., et al. (2021). Data on the proximate composition, bioactive compounds, physicochemical and functional properties of a collection of faba beans (Vicia faba L.) and lentils (Lens culinaris Medik.). Data Brief 34, 106660. doi: 10.1016/j.dib.2020.106660
Drahansky M., Paridah M., Moradbak A., Mohamed A., Owolabi F. A. T., Asniza M., et al. (2016). Cowpea: a strategic legume species for food security and health. Legume Seed Nutraceutical Res. Dietary, 47–65. doi: 10.5772/intechopen.79006
FAO (1986). Manuals of food quality control. 7. Food analysis: general techniques, additives, contaminants and composition (Rome, Italy: FAO Food and Nutrition Paper 14/7). Available at: https://www.fao.org/3/AM808E/AM808E.pdf.
Gumede M. T., Gerrano A. S., Amelework A. B., Modi A. T. (2022). Analysis of genetic diversity and population structure of cowpea (Vigna unguiculata (L.) Walp) genotypes using single nucleotide polymorphism markers. Plants 11, 3480. doi: 10.3390/plants11243480
Hunter H.A. (1986). Soil analysis methods for P, K, Cu, Fe, Mn, and Zn, using ASI extracting solution. Technical notes. (Florida: Agro Services International. INC), 70.
INEI (2017) Capítulo 2: Tipos y Ciclos de los Hogares. Available online at: https://www.inei.gob.pe/media/MenuRecursivo/publicaciones_digitales/Est/Lib1711/cap02.pdf.
INIA- Instituto Nacional de Innovación Agraria (2022) Manual técnico de buenas prácticas agrícolas para la producción de frijol caupí en áreas de tierra firme. Available online at: https://repositorio.inia.gob.pe/.
Jun F., Yu G., Quanjiu W., Malhi S. S., Yangyang L. (2014). Mulching effects on water storage in soil and its depletion by alfalfa in the Loess Plateau of northwestern China. Agric. Water Manage. 138, 10–16. doi: 10.1016/j.agwat.2014.02.018
Kebede E., Bekeko Z. (2020). Expounding the production and importance of cowpea (Vigna unguiculata (L.) Walp.) in Ethiopia. Cogent Food Agric. 6, 1769805. doi: 10.1080/23311932.2020.1769805
Knapp A. K., Smith W. K. (1989). Influence of growth from on ecophysiological responses to variable sunlight in subalpine plants. Ecology 70 (4), 1069–1082. doi: 10.2307/1941376
Kumar P., Kumar S., Tripathi M. K., Mehta N., Ranjan R., Bhat Z. F., et al. (2013). Flavonoids in the development of functional meat products: A review. Vet. World 6 (8), 573–578. doi: 10.5455/vetworld.
Kyei-Boahen S., Savala C. E., Chikoye D., Abaidoo R. (2017). Growth and yield responses of cowpea to inoculation and phosphorus fertilization in different environments. Front. Plant Sci. 8. doi: 10.3389/fpls.2017.00646
Lal R., Smith P., Jungkunst H. F., Mitsch W. J., Lehmann J., Nair P. R., et al (2018). The carbon sequestration potential of terrestrial ecosystems. J Soil Water Conserv. 73(6), 145A–152A. doi: 10.2489/jswc.73.6.145A
Makaure B. T., Aremu A. O., Gruz J., Magadlela A. (2023). Phenolic acids and plant antioxidant capacity enhance growth, nutrition, and plant–microbe interaction of Vigna unguiculata L.(Walp) grown in acidic and nutrient-deficient grassland and savanna soils. J. Soil Sci. Plant Nutr. 23, 190–203. doi: 10.1007/s42729-022-00967-w
Maurya P. R., Lal R. (1981). Effects of different mulch materials on soil properties and on the root growth and yield of maize (Zea mays) and cowpea (Vigna unguiculata). Field Crops Res. 4, 33–45. doi: 10.1016/0378-4290(81)90052-6
Moreira-Araújo R. S. D. R., Sampaio G. R., Soares R. A., Silva C. P. D., Araújo M. A. D. M., Arêas J. A. G. (2018). Identification and quantification of phenolic compounds and antioxidant activity in cowpeas of brs xiquexique cultivar. Rev. Caatinga 31, 209–216. doi: 10.1590/1983-21252018v31n124rc
Mupangwa W., Twomlow S., Walker S. (2012). Reduced tillage, mulching and rotational effects on maize (Zea mays L.), cowpea (Vigna unguiculata (Walp) L.) and sorghum (Sorghum bicolor L.(Moench)) yields under semi-arid conditions. Field Crops Res. 132, 139–148. doi: 10.1016/j.fcr.2012.02.020
Murga-Orrillo H., AraújoW. F., Ribeiro Rocha P. R., Sakazaki R. T., Silva Dionisio L. F., Polo-Vargas A. R. (2016). Evapotranspiração e coeficiente de cultivo do feijão-caupi cultivado em solo do cerrado submetido à cobertura morta. Irriga 21, 172. doi: 10.15809/irriga.2016v21n1p172-187
Murga-Orrillo H., Chuquimez G. J. K., Pashanasi A. ,. B., Arevalo L. L. A. (2023). Vigna unguiculata: a productive option in the face of climate change? Front. Agron. 5. doi: 10.3389/fagro.2023.1284173
Murga-Orrillo H., Irigoin-Aguilar J. M., Hilares-Vargas S., Bardales-Lozano R. M., de A. Lobo F. (2019). Adubos e coberturas orgânicas, fontes de nutrientes de liberação lenta na produção de acelga de colheitas múltiplas. Rev. Ciências Agroveterinárias Lages 18, 380–384. doi: 10.5965/223811711832019380
Nkhata W., Shimelis H., Melis R., Chirwa R., Mzengeza T., Mathew I., et al. (2020). Population structure and genetic diversity analyses of common bean germplasm collections of East and Southern Africa using morphological traits and high-density SNP markers. PloS One 15, e0243238. doi: 10.1371/journal.pone.0243238
Official M. O. A (1993). “Official methods of analysis of feed and their raw materials. 34 (a). Starch (Polarimetric method),” in S. M. O. Agriculture, vol. 1. (Madrid).
Olsen R. S., Cole V. C., Watanabe F. S., Dean L. A. (1954). Estimation of available phosphorus in soils by extraction with sodium bicarbonate (Washington, D.C: USDA). Circular 939.
Pedrosa M. M., Cuadrado C., Burbano C., Muzquiz M., Cabellos B., Olmedilla-Alonso B., et al. (2015). Effects of industrial canning on the proximate composition, bioactive compounds contents and nutritional profile of two Spanish common dry beans (Phaseolus vulgaris L.). Food Chem. 166, 68–75. doi: 10.1016/j.foodchem.2014.05.158
R Core Team (2023) A Language and Environment for Statistical Computing (Vienna, Austria: R Foundation for Statistical Computing) 2021. Available online at: https://www.R-project.org/ (Accessed August 15 2023).
SENAMHI (2023) Servicio Nacional de Meteorología e Hidrología del Perú. Available online at: https://www.senamhi.gob.pe/?&p=estaciones.
Sombié P. A. E. D., Compaoré M., Coulibaly A. Y., Ouédraogo J. T., Tignégré J. B. D. L. S., Kiendrébéogo M. (2018). Antioxidant and phytochemical studies of 31 cowpeas (Vigna unguiculata (L. Walp.)) genotypes from Burkina Faso. Foods 7, 143. doi: 10.3390/foods7090143
Toudou-Daouda A. K., Atta S., Inoussa M. M., Hamidou F., Bakasso Y. (2018). Effect of water deficit at different stages of development on the yield components of cowpea (Vigna unguiculata L. Walp) genotypes. Afr. J. Biotechnol. 17, 279–287. doi: 10.5897/AJB2017.16347
Tzanova M. T., Stoilova T. D., Todorova M. H., Memdueva N. Y., Gerdzhikova M. A., Grozeva N. H. (2023). Antioxidant potentials of different genotypes of cowpea (Vigna unguiculata L. Walp.) cultivated in Bulgaria, southern Europe. Agronomy 13, 1684. doi: 10.3390/agronomy13071684
Xu B., Chang S. K. (2009). Total phenolic, phenolic acid, anthocyanin, flavan-3-ol, and flavonol profiles and antioxidant properties of pinto and black beans (Phaseolus vulgaris L.) as affected by thermal processing. J. Agric. Food Chem. 57, 4754–4764. doi: 10.1021/jf900695s
Keywords: organic mulch, legume, proteins, antioxidant activity, biomass, grain
Citation: Murga-Orrillo H, Chuquímez Gonzales JK and Arévalo López LA (2024) Physiological characterization and bioactive compounds of promising accessions of cowpea (Vigna unguiculata L. Walp) in the Peruvian Amazon. Front. Agron. 6:1392068. doi: 10.3389/fagro.2024.1392068
Received: 26 February 2024; Accepted: 15 April 2024;
Published: 07 May 2024.
Edited by:
Fabio Gresta, University of Messina, ItalyReviewed by:
Jiban Shrestha, Nepal Agricultural Research Council, NepalRashmi Yadav, Indian Council of Agricultural Research (ICAR), India
Lydia Ndinelao Horn, University of Namibia, Namibia
Copyright © 2024 Murga-Orrillo, Chuquímez Gonzales and Arévalo López. This is an open-access article distributed under the terms of the Creative Commons Attribution License (CC BY). The use, distribution or reproduction in other forums is permitted, provided the original author(s) and the copyright owner(s) are credited and that the original publication in this journal is cited, in accordance with accepted academic practice. No use, distribution or reproduction is permitted which does not comply with these terms.
*Correspondence: Hipolito Murga-Orrillo, aG11cmdhQHVuYWFhLmVkdS5wZQ==
†ORCID: Hipolito Murga-Orrillo, orcid.org/0000-0001-5039-5838
Luis A. Arévalo López, orcid.org/0000-0002-6417-8161