- 1Department of Plant Sciences, North Dakota State University, Fargo, ND, United States
- 2Department of Horticulture, Washington State University Northwestern Washington Research and Extension Center, Mount Vernon, WA, United States
- 3Mechanical & Industrial Engineering Department, Montana State University, Bozeman, MT, United States
- 4The United States Department of Agriculture, USDA-Agricultural Research Services (ARS) North Central Soil Conservation Research Laboratory, Morris, MN, United States
- 5Agriculture and Natural Resources, Washington State University (WSU) Extension, Washington State University, Pullman, WA, United States
Polyethylene (PE) mulches are widely used in strawberry (Fragaria × ananassa Duch) production for weed suppression and crop growth optimization. However, PE mulches are not biodegradable and contribute to plastic pollution. Our objective was to develop and test biodegradable liquid-applied ‘hydromulches’ (HMs) as a sustainable alternative to PE mulch. HM weed suppression efficacy, strawberry plant growth, and yield were evaluated. HM formulations consisted of shredded newsprint paper (NP), water, and a tackifier, either guar gum (GG) or psyllium husk (PH) added at 2 or 6%. Experiments were conducted at two environmentally distinct locations: northwest Washington (WA) and eastern North Dakota (ND). Five HM formulations were compared to black PE mulch within a randomized complete block design with four replications. PE mulch suppressed weeds completely at peak weed emergence and peak weed vegetative growth at both locations. Formulations of HM containing GG provided superior weed suppression compared to other HM formulations at peak weed emergence (4–6 vs. 18–22 plants m-2, respectively). At peak vegetative growth, HM formulations containing GG had the lowest weed density compared to other HMs in ND (1 vs. 9–12 plants m-2), whereas these differences were not observed in WA. Total weed biomass did not differ among HMs across both locations. GG HM formulations deteriorated similarly to PE mulch (3–5% vs. 2%, respectively) in ND, whereas other HMs deteriorated more substantially. In WA, all HMs deteriorated more than PE mulch (6–12% vs. 1%, respectively). Fruit yield did not differ among treatments in weedy and weed-free subplots (194–254 g plant-1) in WA. In ND, yield was greater in all HM treatments compared to PE mulch in both weedy and weed-free subplots. Across both locations, strawberry canopy cover was greater in PE mulch (56.1% canopy cover) compared to 2%GG and NP (42.4 and 39.8% canopy cover). Strawberry plant biomass was similar among mulch treatments. However, strawberry leaf and crown biomass were slightly lower in 2%PH compared to other mulch treatments. Results demonstrate HMs with GG tackifier are a promising alternative to PE mulch in organic strawberry systems based on ability to suppress weeds, enhance strawberry growth, and maintain yield.
1 Introduction
Strawberry (Fragaria × ananassa Duch) is an economically significant crop across the globe prized for its flavorful and health-promoting fruits (Giampieri et al., 2015; Simpson, 2018). The area and volume of organically grown strawberries is expanding with increased consumer interest for organically grown food, yet best practices to sustainability manage weeds within organic systems is challenging (Granatstein et al., 2013; Fortune Business Insights, 2020). Strawberry plants have a low, creeping growth habit and a shallow rooting system, making them susceptible to weed competition, potentially leading to yield losses (Johnson and Fennimore, 2005). Early-season weed competition can result in yield loss of up to 65% in strawberries if weed competition is not managed. The first two months after planting are critical for establishing the plants and managing weeds (Pritts and Kelly, 2001). If unmanaged, weeds compete with the plants for water, mineral nutrients, and light while also reducing harvest efficiency and increasing the risk of pest and disease outbreaks (Salceanu and Olaru, 2017).
Although organic strawberry production can provide premium prices, the prohibition of synthetic pesticides poses a challenge for weed control (NCAT-ATTRA, 2007). To overcome this limitation, installation of polyethylene (PE) mulches is a standard practice to control weeds and promote crop growth and yield through beneficial effects on the soil and canopy microclimate (Lamont, 2004; Kasirajan and Ngouajio, 2012; DeVetter et al., 2017; Zhang et al., 2021). PE mulches provide weed control ranging from 64% to 98% during the growing season (Kasirajan and Ngouajio, 2012). Early ripening and improved crop quality are some additional attributes associated with PE mulches. Certain reflective mulches also repel insects and can provide a barrier to soil pathogen infestation/infection (Sarkar et al., 2018). However, PE mulches are non-biodegradable and are only permitted for organic production if they are completely removed at the end of the growing season [7 CFR 205.601] (Miles et al., 2017). Furthermore, annual removal and disposal of PE mulch is laborious and expensive with landfilling, stockpiling, on-farm burial, burning, or illegal dumping used by farmers to manage mulch waste (Goldberger et al., 2019). Plastic pollution is a major concern associated with plastic mulch waste due to the ecological implications of plastic contaminants on marine and terrestrial ecosystem health (Ng et al., 2018). Plastic particles can accumulate in food chains but research to understand human health impacts is lacking (Yuan et al., 2022). Approximately 6.3 billion metric tons of plastic waste has been generated since 2005 globally and only 9% was recycled, 12% incinerated, and 79% accumulated in landfills (Geyer et al., 2017). If current production trends continue, approximately 12 billion metric tons of plastic mulch waste will accumulate in landfills by 2050 (Geyer et al., 2017). As mentioned, removal of plastic mulches from agricultural fields is a costly and labor-intensive process (Liu et al., 2014). Plastic removal and disposal required an estimated 42 h hectare-1 (Velandia et al., 2019). Warnick et al. (2006a) estimated removal cost at approximately $250 hectare -1 and this expense has risen in many states with increases in labor wages. Disposing plastic mulches into landfills is a common practice in the United States (Goldberger et al., 2019), but is costly with average tipping fees in the US at $54.17 per metric ton. Costs vary widely depending on state and range from $192 metric ton-1 in Alaska to $29.82 metric ton-1 in Kentucky (EREF, 2022). However, access to landfills at the local level will soon become a serious issue in the United States as the number of active landfills has fallen from 7924 to 1954 during 1988–2006 (EPC, 2006).
Soil-biodegradable plastic mulches (BDMs) were created in the 1990s as an alternative to PE mulches (Brodhagen et al., 2017). Feedstocks used to make BDMs are compostable or in-soil biodegradable and can be directly incorporated into the soil where they are biodegraded by native soil microorganisms into carbon dioxide, methane, water, and microbial biomass (Kasirajan and Ngouajio, 2012; Serrano-Ruiz et al., 2021). Commercial BDMs are made using a blend of synthetic and biobased feedstocks, which limits their use in organic agriculture in the US given the requirement for 100% biobased content (Miles et al., 2023). Currently, no commercially available BDM contains 100% biobased content, so these alternatives are not permitted in US organic agriculture (Miles et al., 2023).
To meet organic requirements set forth by the National Organic Program (NOP), biodegradable mulch formulations must meet composability specifications: demonstrate at least 90% biodegradation in soil according to specific test methods, be produced without organisms or feedstocks derived from excluded methods, and be fully biobased (Miles et al., 2023). Hydromulches (HMs) can be formulated to meet these requirements and are defined as a mixture of water and plant-based cellulose fibers with additional binding agents called tackifiers. The resultant liquid slurry is sprayed onto soil surfaces, and then dries to form a mulch sheet (Cline et al., 2011). Hydromulches (HMs) are frequently used to control soil erosion but the application of HMs in horticultural production systems is not fully developed (Faucette et al., 2006). Application rates for HMs vary between 2,243–5,059 kg of dry matter ha-1 (Vaughn et al., 2013; Ricks et al., 2020). Materials previously evaluated for HM feedstock include straw, wood fiber, cotton (Gossypium arboreum L.), and wheat (Triticum aestivum L.) fibers (Ricks et al., 2020); barley (Hordeum vulgare L.) straw, rice (Oryza sativa L.) husk, rests from mushroom production, and wood chips (Moreno et al., 2022); corn (Zea mays L.) starch, glycerol, keratin hydrolysate, corn gluten meal, corn zein, egg shells, and isolated soy (Gloeb et al., 2023); and recycled paper, and cardboard (López-Marín et al., 2021).
Hydromulch can be an effective weed control method for established perennial fruit trees as well as horticultural crops and should be easy to apply (Valencia et al., 2021). Puka-Beals and Gramig (2021) found an 88% reduction of weeds associated with hydromulch treatments made using shredded newsprint paper compared to soil with no mulch. Hydromulch formulations of paper pulp with wheat straw and rice husk provided weed control ranging from 64.6% to 85.7% (Claramunt et al., 2020). HMs are capable of absorbing water, but when they become wet, they can lose their durability and puncture resistance against weed seedlings. Consequently, developing hydromulch formulations that can maintain their durability and puncture resistance is important in developing the technology (Moreno et al., 2022). Furthermore, developing rapid and effective application technologies for large-scale deployment of HM is a necessity. Previous research findings also suggest a need to focus on improving the profitability of hydromulches in horticulture production (López-Marín et al., 2021).
The aim of this research was to develop and test multiple hydromulch formulations against standard PE mulch within organically-managed day-neutral strawberry grown in two distinctive environments. This study evaluated various formulations of cellulose-based biodegradable hydromulches on weed suppression, crop growth, and yield. Application modifications to achieve an effective and convenient hydromulch application was also an important focus of this research. We hypothesized that hydromulch formulations with tackifiers would enhance weed suppression efficacy and increase strawberry total yield compared to paper-only hydromulch. Similarly, we hypothesized that formulations with tackifiers would result in weed suppression and strawberry total yield similar to industry-standard PE mulch.
2 Materials and methods
2.1 Experimental locations
A field study was conducted during summer of 2022 at the Dale E. Herman Research Arboretum near Absaraka, ND (46° 59’ 17.1” N, 97° 21’ 5.8” W, with an altitude of 291 m above sea level) and at the Washington State University (WSU) Northwestern Washington Research and Extension Center (NWREC) in Mount Vernon, WA (48° 26’ 28.3” N, 122° 23’ 43.4” W, with an altitude of 5 m above sea level), two highly contrasting production environments. The soil at ND experimental location consists of Warsing sandy loam, loamy substratum with zero to two percent slopes, and are classified as fine-loamy over sandy or sandy-skeletal, mixed, superactive, frigid oxyaquic hapludolls in terms of soil taxonomy. This soil type possesses a moderate productivity potential, as indicated by a crop productivity index of 48 (Soil Survey Staff, 2000). However, the soil at WA field location consists of Field silt loam, protected with zero to three percent slope. This soil is categorized as medial over sandy or sandy-skeletal, mixed, nonacid, mesic aquic xerofluvents in taxonomic classification (Soil Survey Staff, 1988). The ND field location is certified organic while the WA field location has been organically managed according to the U.S. National Organic Program (NOP) standards. The field location near Absaraka was managed under organic practices for several years with an alfalfa (Medicago sativa L.) cover crop as an organic weed control method. Weekly average air temperature and weekly cumulative precipitation during the 2022 strawberry production season are presented (Figure 1) for both experimental locations.
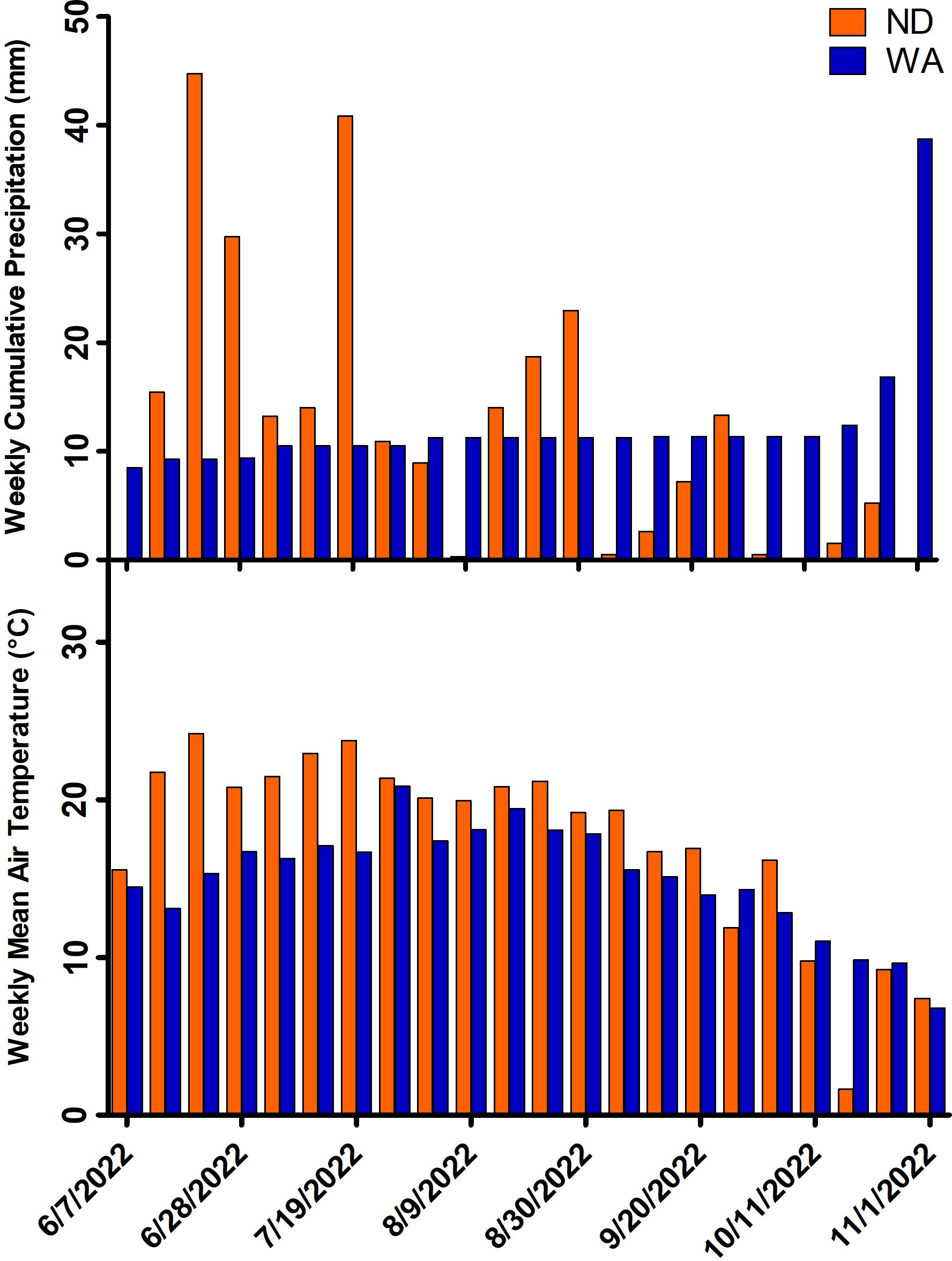
Figure 1 Weekly mean air temperature (°C) and weekly cumulative precipitation (mm) at Absaraka, ND, and Mount Vernon, WA, during the 2022 strawberry production season as reported by North Dakota Agricultural Weather Network (at ND) and WSU-AGWeatherNet (at WA).
2.2 Experimental design, treatments, and field operations
A randomized complete block design (RCBD) was used to arrange six treatments within four blocks at both locations. Field preparation in ND was accomplished using a field cultivator and rotary tiller (John Deere 655 rotary hoe) as soon as field soil conditions allowed (May 2022). To inform pre-plant organic fertilization, soil nutrient analysis was performed to quantify baseline soil macro- and micro-nutrients including NO3, phosphorous, potassium, calcium, magnesium, sulfur, boron, zinc, manganese, copper, iron, soil pH, and soil organic matter percentage (Table 1). At ND, soil samples were collected at two depths (0–0.15 m and 0.15–0.30 m) using a standard soil probe (0.02 m diameter) in a zigzag pattern and 12 soil cores per replication were sampled. Soil samples were bulked for each replication to get a composite sample for each soil depth. Soil samples were then sent to the soil testing laboratory (Agvise Laboratories Inc., Northwood, ND). In WA, baseline soil sampling entailed collecting 15–20 cores to a depth of 0.30 m per sampling area/location using a standard soil probe (0.02 m diameter cores). Soil was then mixed in a bucket and a composite sample was sent to a soil testing lab (Simply Soil Testing, Burlington, WA). Feather meal [(11–0-0), 15598 McLean Rd, Skagit Farm, Mount Vernon, WA)] was applied in Washington as a preplant fertilizer, using a 6ft wide drop spreader (6-ft. Drop Spreader with Tow Hitch | Gandy) at an application rate of 67.22 kg N hectare-1. At ND, aerobically composted chicken manure (ChickNPoo 2–4-3, Pearl Valley Farms, Inc., 968 S. Kent Road Pearl City, IL) was applied using broadcast method by hand to achieve the same preplant fertilizer target (OSU Extension Service, 2019). A rotary tiller (John Deere 655 rotary hoe) was used to incorporate granular fertilizers at both locations. Throughout the growing season from planting to harvest, soil moisture percentage and soil temperature data was recorded with ‘Teros 11’ soil moisture content and temperature sensors (Meter Group, Inc., 2365 NE Hopkins Court, Pullman, WA) at one-hour intervals in one experimental block at both locations. At both locations, an auger was used to remove soil to 0.25 m and sensors were installed at least 0.05 m away from the drip tape at 0.15 m depth. Sensor prongs were installed horizontally in undisturbed soil to achieve maximum accuracy and the removed soil was used to backfill the auger hole.

Table 1 Baseline soil macro- and micro-nutrients at Absaraka, ND and Mount Vernon, WA: nitrate (N), sulfur (S), phosphorus (P), potassium (K), calcium (Ca), magnesium (Mg), boron (B), zinc (Z), iron (Fe), manganese (Mn), copper (Cu), organic matter (OM), and pH.
Raised beds were constructed using a tractor-pulled bed shaping implement (MRB-448 model from Berry Hill Irrigation in ND and Rain-Flo 2600 model from Rain-Flo Irrigation LLC. in WA). Drip irrigation was installed at a soil depth of 0.0635m in ND (Drip tape, SKU TDE804100, 2.03e-4 m, 0.1016 m emitter spacing, 6.309e-5 m3s-1 per 30.48 m at 8 PSI flow rate, DripWorks, Inc., 190 Sanhedrin Circle, Willits, CA). Conversely, in WA, the drip tape was placed on top of the bed surface (T-Tape, Model 508–08-340, 0.0002 m, 0.2 m emitter spacing, 7.05e-5 m3s-1 per 100 m flow rate, San Diego, CA). Raised beds, measuring 4.6 × 0.9 m, served as an experimental unit. In each experimental unit, a 3m length was dedicated for data collection with 0.76 m buffers on each end to separate treatments within a block. This arrangement resulted in a total of 24 experimental units at each location. Experimental blocks were separated by alleys measuring 2.13 m wide with a 3.1 m border around all four sides of the experiment. Alleys and borders were maintained with periodic tillage with a BCS power harrow (Model 732, BCS, 14151 Fir Street, Oregon City, OR) to manage weeds and limit edge effects from encroaching weeds.
Based on the results of puncture resistance and tensile strength testing of hydromulches in a controlled laboratory setting at Montana State University, a preliminary greenhouse experiment was designed to evaluate the weed suppression of hydromulches for one small-seeded species [redroot pigweed (Amaranthus retroflexus L.)], one large seeded species [velvetleaf (Abutilon theophrasti Medik.)], and one grass species [yellow foxtail, Setaria pumila (Poir.) Roem. & Schult]. This greenhouse study was focused to determine five most promising hydromulch formulations and the lowest effective target application rate for field testing. Guar gum and psyllium husk hydromulch formulations were tested at 2% and 6% rate of total dry matter ha-1. For application rate, Puka-Beals and Gramig (2021) used an application rate of 3,430 kg of dry matter ha-1. Their recommended recipe for the preparation of hydromulch involved using 1 kg dry matter per 0.035 m3 of water. However, Gloeb et al. (2023) found that increasing the viscosity of hydromulch was correlated with improved weed suppression. Our project team collaborators from Montana State University suggested an application rate of 4,483 kg dry matter ha-1. To increase the viscosity of the hydromulch, the recipe for the preparation of hydromulch formulations was adjusted to use 1.25kg dry matter per 0.035 m3 of water, and a 0.01905 m (¾”) flat fan brass nozzle was used to achieve a fine and even spray pattern. Application rates of 3,076 kg dry matter ha-1 (1×) and 6,151 kg dry matter ha-1 (2×) were compared for the preliminary greenhouse experiment. Based on outcomes, hydromulch treatments and application rate were adjusted for the field experiment.
Five hydromulch (HM) treatments were applied at an application rate of 4,535 kg dry matter ha-1. These hydromulch treatments were composed of shredded newsprint as a source of cellulosic fiber, blended with one of two binding agents (i.e., tackifiers) and water to form a smooth slurry. The two tackifiers were certified organic psyllium husk powder and certified organic guar gum powder (Mountain Rose Herbs, 4020 Stewart Rd, Eugene, OR). The five hydromulch treatments were 2% guar gum (2%GG), 6% guar gum (6%GG), 2% psyllium husk (2%PH), 6% psyllium husk (6%PH), and shredded newsprint paper with no tackifier (NP) (Figure 2). Application equipment, which was slightly different in construction at each location (see description below) was used to apply hydromulch slurry at each location (ND in two and WA in three passes) to achieve the target application rate with even mulch surface coverage. Black plastic mulch was installed manually at both locations. Bare-root day-neutral strawberry plants “Albion” cultivar, acquired from Indiana Berry & Plant Co. in Plymouth, IN (ND), and Norcal Nursery (Planasa) in Washington, were transplanted through all mulches on June 8th (Figure 2F) in WA and June 29th (Figure 2C) in ND. Strawberry plants were transplanted in a double-row staggered pattern with a planting distance of 0.31 m between adjacent plants within a row (plant-to-plant distance), 0.41 m between adjacent rows (row-to-row distance), and a total 21 plants were planted in each experimental unit. Additionally, the bare-root day-neutral strawberry plants in each row were planted 0.20 m away from bed corners. Plants were transplanted by using a hand-held metal bar with a notch on one end to help insert roots in the soil with minimal mulch disturbance. Liquid fertilizer (TRUE 4–2-2 fish emulsion; TRUE Organic Products, Inc., 20225 W Kamm Ave, Helm, CA) was applied with the drip irrigation system during the growing season at an application rate of 22.42 kg N ha -1 per month at both locations. EZ-FLO fertilizer injector (DripWorks, Inc., 190 Sanhedrin Circle, Willits, CA) was attached with a drip irrigation system for liquid fertilizer application. EZ-FLO fertilizer injector can handle 5 to 80 psi pressure and provide a flow rate of 1.2618e-4 m3s-1.
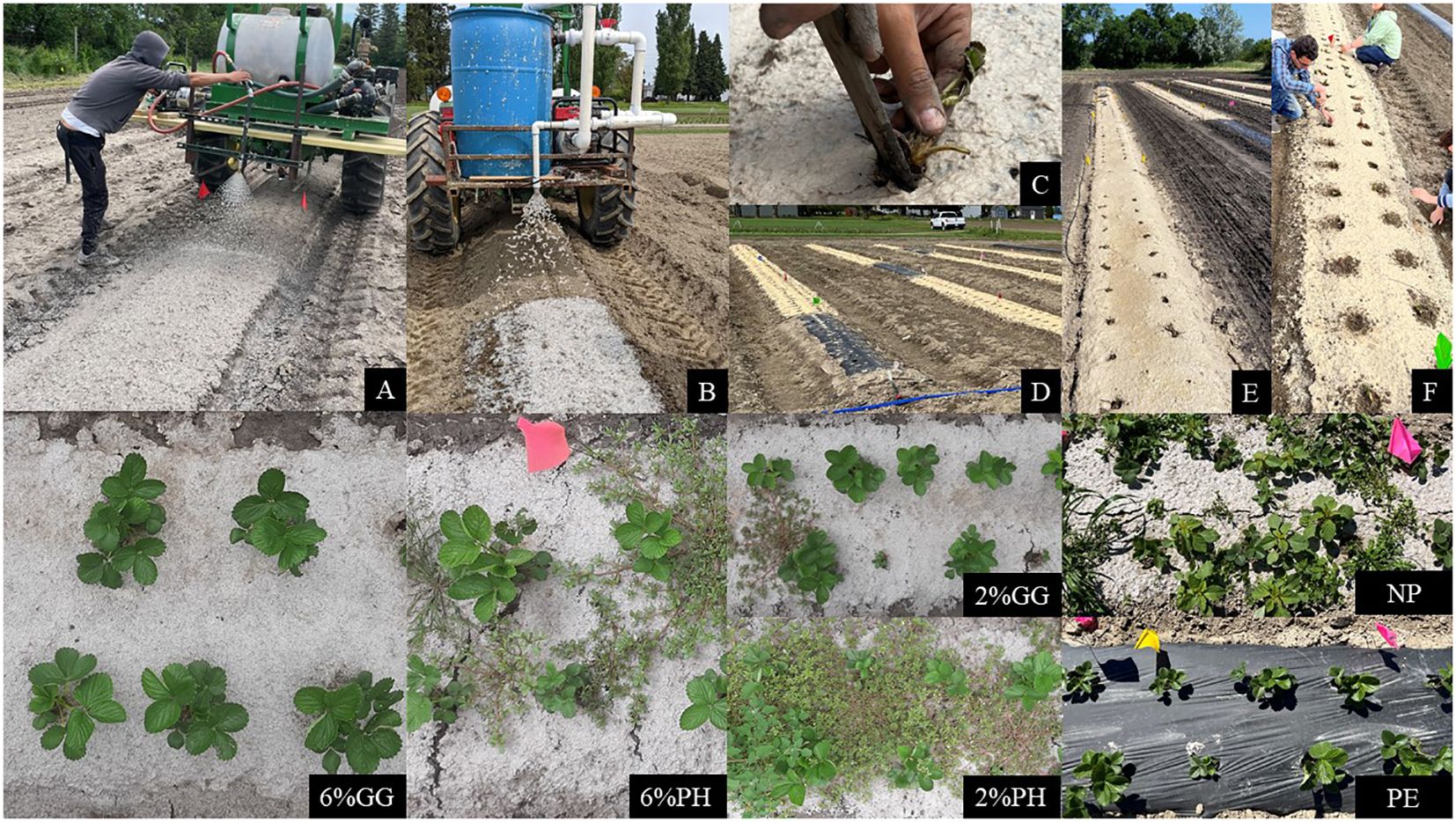
Figure 2 Hydromulch application at ND (A) and WA (B). Experimental locations after planting at ND (E) and WA (D). Strawberry planting at ND (C) and WA (F). Mulch treatments; six percent guar gum, 6%GG; six percent psyllium husk, 6%PH; two percent guar gum, 2%GG; two percent psyllium husk, 2%PH; newsprint paper with no tackifier, NP; and polyethylene mulch, PE.
2.3 Application technology and hydromulch preparation
In North Dakota, a hydromulch applicator (Figure 2A) was constructed using a commercial hydroseeder (Model: HS-50, Turbo Technologies, Inc.,1500 First Ave., Beaver Falls, PA 15010), a 0.01905 m (¾”) brass flat fan nozzle, an Ironton clear water pump (Northern Tool + Equipment, 2800 Southcross Drive West, Burnsville, Minnesota), and a wooden platform (built at NDSU Service Center, 3317 19th Ave N, Fargo, ND) capable of being attached to any tractor. The commercial hydroseeder was used for the purpose of continuously and forcefully mixing dry matter (shredded newsprint and tackifiers) with water to produce a homogeneous slurry of hydromulch. The hydroseeder machine was equipped with a 0.0508 × 0.0508 m (2” × 2”) centrifugal pump powered by a 4,847 Watts (W) Briggs & Stratton recoil start engine and a 0.21 m3 plastic tank. The hydroseeder pump discharges the hydromulch slurry too forcefully, creating unwanted mixing with surface soil. To overcome this issue, a modification of the commercial hydroseeder was made by attaching a 0.0254 m (1”) diameter hose to the discharge outlet at the bottom of the hydroseeder tank and connecting it to the inlet of an Ironton clear water pump (Model 60729, Ironton). The Ironton pump, with a 7.9e-5 m3 engine-driven centrifugal pump and 0.0254 m (1”) suction and discharge ports, was used as the hydromulch application pump. A 0.01905 m (¾”) nozzle (Model 80400, 0.0025 m3s-1, 80-degree flat spray) was attached as a discharge outlet to achieve the desired application rates. This hydromulch application system was mounted to a tractor for field application.
The hydromulch applicator in WA (Figure 2B) was fabricated using a Predator® 2.12e-4 m3 gasoline-powered semi-trash water pump (Harbor Freight Tools®; Calabasas, CA, USA). The pump and a 0.21 m3 plastic barrel were systematically attached with 0.051 m PVC pipes for by-pass flow (agitation) and a 0.051 m PVC pipe stepped down to 0.025 m pipe linked with a single 0.025 m discharge nozzle (0.0037 m3s-1, 80-degree flat fan spray). The metal frame that housed the hydromulch applicator was equipped with a 3-point hitch that attached to a tractor for application. In WA, a single pump was used to mix and apply hydromulch.
In ND, hydromulches were prepared by placing 0.035 m3 of municipal tap water into the 0.21 m3 tank. Subsequently, the hydroseeder pump was activated and shredded newsprint was gradually added to meet the specified quantity of dry matter while ensuring continuous mixing. For each treatment, an appropriate tackifier, proportionate to the total dry matter, was initially mixed in a 0.0189 m3 bucket using a drill to ensure thorough blending and prevent clumping. This tackifier mixture was then introduced into the 0.21 m3 tank and hydroseeder pump was operated at full power for 5–10 minutes to achieve proper blending of tackifier and paper fibers, resulting in a homogeneous slurry of hydromulch. Tractor speed was then calibrated in alignment with the discharge pump to attain the required application rate.
In WA, hydromulches were prepared by adding 6.75 kg of crumpled newsprint sheets to 0.19 m3 trash cans. The newsprint paper source was the same as ND. Cans were then topped off with water and agitated vigorously using a cabbage splitter, forks, and shovels. This mixture was then allowed to sit for 24 hours in the field, agitated again to create a thick slurry, and siphoned into the hydromulcher. The slurry was recirculated in the hydromulcher until a pulpy, viscous consistency was obtained. Tackifier was then added and the system recirculated for an additional 5–10 minutes until the tackifier was fully incorporated into the slurry. The tractor speed, discharge pressure, and discharge rate were then calibrated and the mulch applied onto preformed raised beds.
2.4 Strawberry management
Strawberry blossoms were removed for the first six weeks of bloom onset to encourage good root and canopy establishment. This was done by pinching or clipping off blossoms or visible trusses on a weekly basis. Subsequently, strawberry blossoms were allowed to develop into fruits. The first strawberry blossoms were observed on June 20th in WA (12 days after planting [DAP]) and on July 6th (7 DAP) in ND. Strawberry runners were also removed weekly to encourage root and canopy development. Post-plant fertigation was started on June 27th (19 DAP) in WA and on July 12th (13 DAP) in ND in two split applications to achieve 22.42 kg N ha -1 per month at both locations. Irrigation was initiated when the soil moisture tension dropped below 25% (0.25 cm3/cm3). A weekly 0.0254 m3 water supply was provided (rain or drip irrigation) based on strawberry moisture requirements. Insect pest and disease scouting was conducted weekly. In WA, OMRI listed neem oil (Pyganic 5.0 EC, Valent U.S.A. LLC, San Ramon, CA) was used to manage lygus bug infestation to prevent fruit damage.
2.5 Data collection
Weed presence was quantified by counting weeds emerging through the mulches at peak weed emergence (PWE), defined as the point when the first flush of annual weeds reached approximately 4 to 6 true leaves (July 22nd [23 DAP] in ND, July 5th [27 DAP] in WA). Weed counts were conducted within two randomly placed 0.25 m2 quadrats in each plot data collection area. Weeds were separated according to species for broadleaves, but according to family for grasses and sedges. A second set of weed measurements was conducted at peak weed vegetative growth (PWVG), defined as the period when weeds were entering reproductive phase (August 18th [50 DAP] in ND and July 27th [49 DAP] in WA). For these assessments, weed density and biomass were quantified within two quadrats, placed as described above. Weeds were clipped at the soil surface, separated by species, counted, and placed in paper sample bags. These samples were dried at 60°C to a constant weight to determine weed dry biomass. Weeds growing in planting holes were removed on a weekly basis to exclude the effect of these weeds.
Two one-meter-long subplots were established for season-long assessment of mulch deterioration and crop canopy development. Once a month, mulch deterioration was measured using visual estimates to determine percentage soil exposure (PSE). For this measurement, 0% represented completely intact mulch and 100% represented fully deteriorated mulch with the soil surface completely exposed. These assessments included just the surface top of the raised bed, not the edges/walls of the raised bed (where mulch application can be thinner), and were quantified in 1% increments until 20% PSE, and in 5% increments thereafter. Weeds were removed once a month from a 1 m subplot of the crop canopy area before capturing photos to isolate the crop canopy from weeds. These photos were processed using the Canopeo app to facilitate digital plant cover estimates (Patrignani and Ochsner, 2015). Strawberry dry biomass was measured at the end of the growing season (107 DAP at ND and 134 DAP at WA). Two plants were randomly selected from the subplots of the crop canopy assessment area and separated by roots, leaves and crown. Plant materials were then dried at 60°C until constant weight was achieved. Strawberry fruits were harvested separately for the canopy subplot (weed-free area) and the remainder of the data collection area (weedy area) 1–2 times a week and total yield of strawberry fruit was quantified.
2.6 Statistical analyses
Analysis of variance (ANOVA) was used to test mulch treatment and location effects on measured response variables via SAS Software (SAS release 9.2, SAS Institute Inc., Cary, N.C.) using PROC GLIMMIX. Response variables included PWE weed density, PWVG weed density, PWVG weed biomass, percentage mulch deterioration, percentage canopy cover, weed-free fruit yield, weedy fruit yield, strawberry plant total biomass, strawberry plant leaf biomass, strawberry plant crown biomass, and strawberry plant root biomass. Statistical significance was tested at α = 0.05 and post-hoc treatment means comparisons were conducted using Tukey’s HSD test. Replication was considered a random effect whereas mulch treatment and location, and their interaction, were considered fixed effects. If ANOVAs revealed mulch treatment × location interactions, the slice statement was used for means comparisons (also adjusted via Tukey’s HSD) within each mulch treatment and location. Model fit statistics were used to determine which distribution best fit the data. For count data, a negative binomial distribution was used. For biomass data, either a lognormal or gamma distribution was used. For data analyzed using these distributions, a ‘one’ was added to each data point if the dataset contained zeros. For percentage data, a beta distribution was used. For many weed response variables (i.e., weed density and biomass), the PE mulch treatment often contained all zero values, in which case 95% confidence limits were used to test whether the other HM treatments differed from this treatment, because these data could not be included in ANOVA due to lack of variance components.
3 Results
3.1 Weed density
At peak weed emergence, weeds were absent with PE mulch and no confidence intervals associated with hydromulch treatments included zero (Figure 3A). Both 2% and 6%GG formulations were more effective at suppressing weeds than NP and PH treatments (Figure 3A). Weed densities were similar among 2% and 6%PH formulations (both) and NP hydromulch. The WA location displayed greater average weed density (48 plants m-2) compared to the ND location (3 plants m-2) (data not shown). Hence, total weed count results at peak emergence highlight the guar gum tackifier for enhancing the weed suppression efficacy of hydromulches.
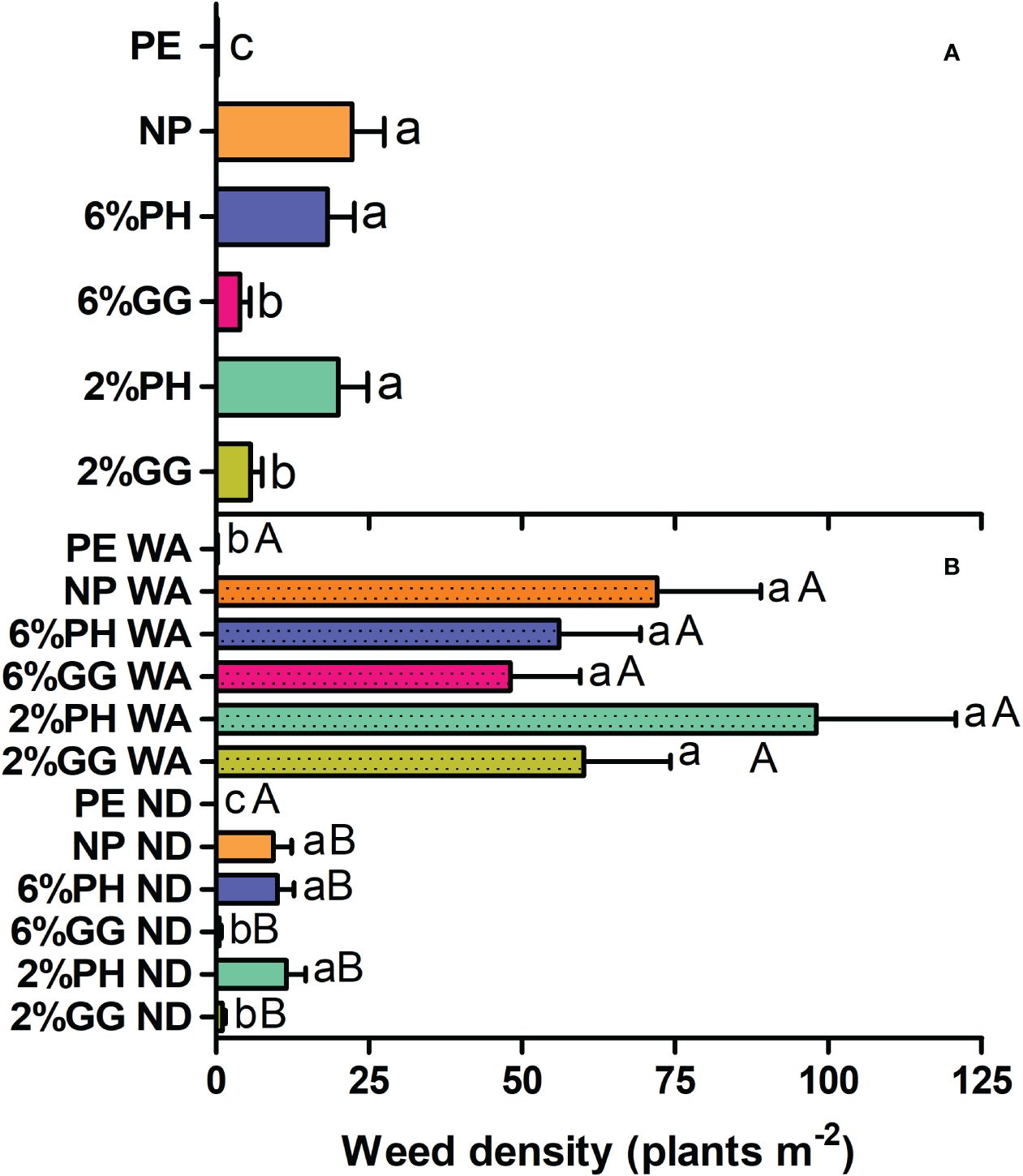
Figure 3 Mean + standard error total weed density (plants m-2) measured at (A) peak weed emergence (23 DAP at ND and 27 DAP at WA) and (B) peak weed vegetative growth (50 DAP at ND and 49 DAP at WA) for (i) polyethylene mulch, PE; (ii) newsprint paper with no tackifier, NP; (iii) six percent psyllium husk, 6%PH; (iv) six percent guar gum, 6%GG; (v) two percent psyllium husk, 2%PH; and (vi) two percent guar gum, 2%GG. Means labeled with differing uppercase letters represent location and lowercase letters represent treatment differences at α = 0.05. Results from data collected at PWVG are presented separately by location (ND, WA) and means comparisons are made within location.
At peak weed vegetative growth, the PE film mulch had zero weeds at both locations, and none of the confidence intervals associated with the hydromulch treatments included zero (Figure 3B). Due to a location × mulch treatment interaction (P = 0.003) results are presented separately for each location. At WA, weed density did not differ among hydromulch treatments (P = 0.273). Conversely, at ND, weed density of 2% and 6%GG formulations (4 and 2 plants m-2, respectively) were significantly lower than NP and 6% and 2%PH HMs (9, 10, 12 plants m-2, respectively).
At peak weed emergence and peak weed vegetative growth, all weeds at the ND location were broadleaf species, with minimal grass emergence through HMs (data not shown). In contrast, both broadleaf and grass weed species occurred at WA. At peak weed emergence and peak weed vegetative growth, the hydromulch formulations did not differ with respect to broadleaf weed suppression at WA (P = 0.185 and P = 0.344, respectively; Figure 4). However, at WA for peak weed emergence, hydromulch treatments differed with respect to grass suppression, with the 2%GG treatment suppressing weeds the most (2 plants m-2). The 6%GG and 6%PH treatments showed an intermediate response (3 and 9.5 plants m-2, respectively), followed by 2%PH and newsprint paper with no tackifier, the least effective hydromulch treatments (11.5 and 12.5 plants m-2, respectively, Figure 4A). At peak weed vegetative growth, hydromulch treatments did not differ with respect to grass suppression [Figure 4B, (P = 0.448)]. These results suggest potential for guar gum tackifiers to improve early season grass weed suppression.
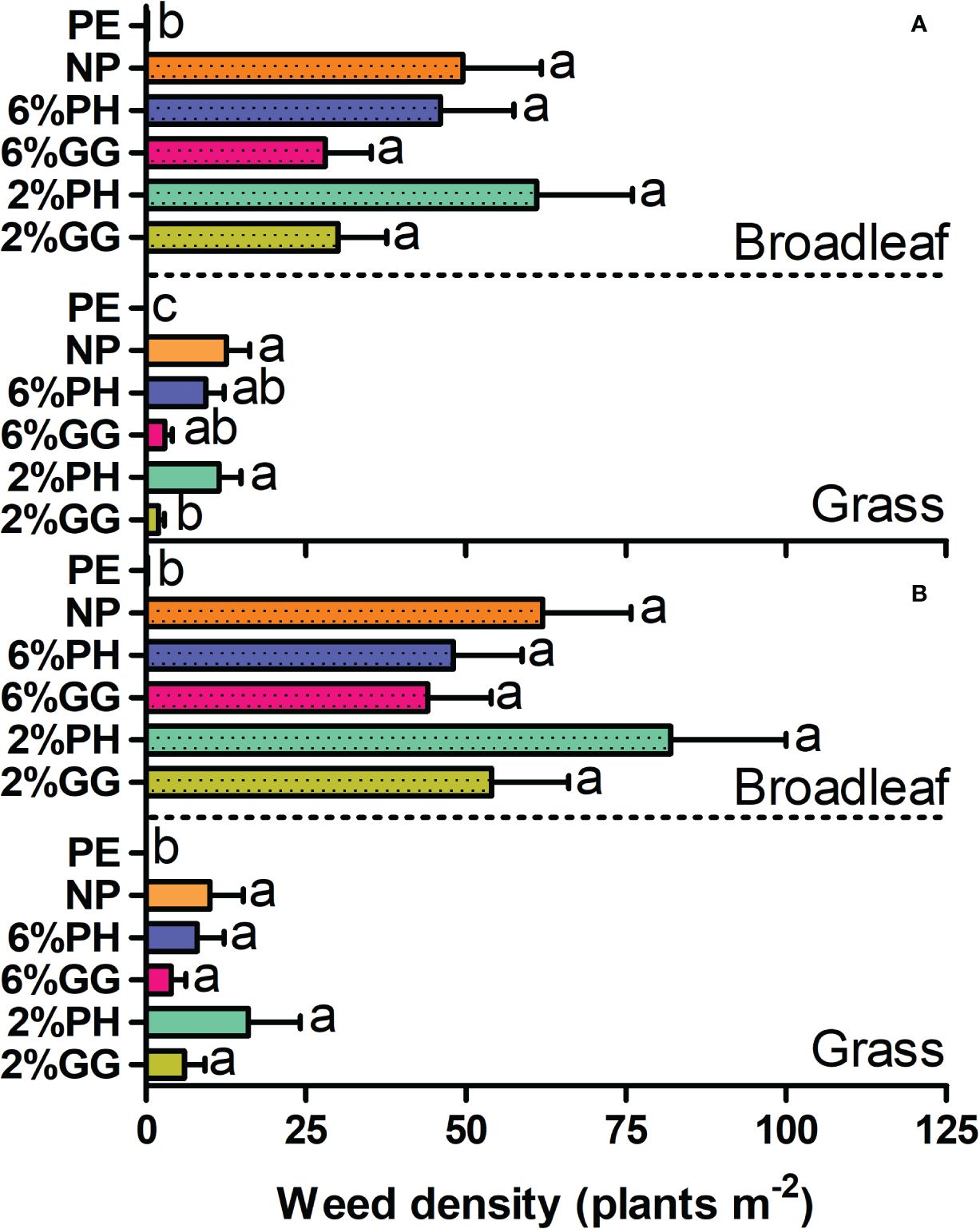
Figure 4 Mean + standard error total broadleaf and grass weed density (plants m-2) in WA measured at (A) peak weed emergence and (B) peak weed vegetative growth for (i) polyethylene mulch, PE; (ii) newsprint paper with no tackifier, NP; (iii) six percent psyllium husk, 6%PH; (iv) six percent guar gum, 6%GG; (v) two percent psyllium husk, 2%PH; and (vi) two percent guar gum, 2%GG. Means labeled with different lowercase letters differed at α = 0.05. Comparisons are made separately within each sampling period/plant functional group. Grasses were mostly found only at WA.
3.2 Weed biomass at peak vegetative growth
Total weed dry biomass measured at peak weed vegetative growth was influenced by a mulch treatment × location interaction (P = 0.02). At the WA location, weed dry biomass did not differ among HM treatments, however means were marked by high variability, limiting the ability to show treatment differences (Figure 5). At ND, the 6%GG HM contained little weed dry biomass and did not differ from PE mulch (6.5 vs. 0 g biomass m-2, respectively). All other HM treatments contained more weed biomass than the 6%GG and PE control (Figure 5).
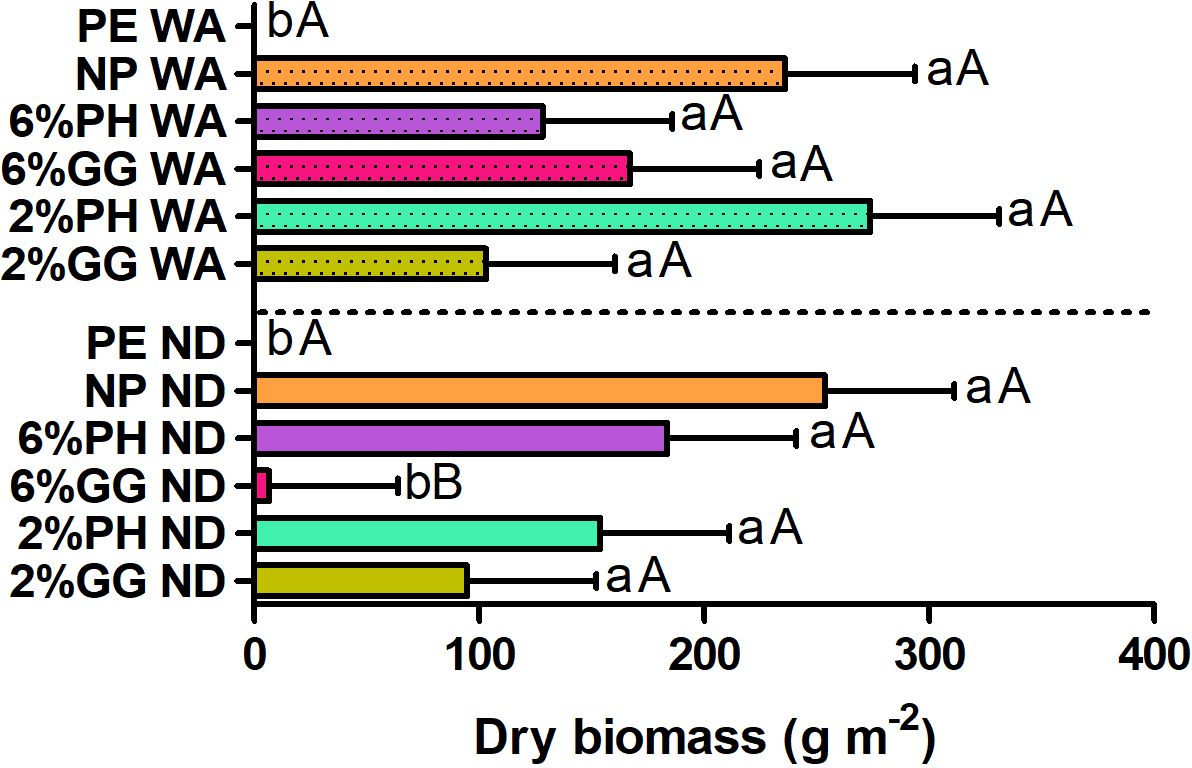
Figure 5 Mean + standard error total weed dry biomass (g m-2) measured at peak weed vegetative growth (50 DAP at ND and 49 DAP at WA) for (i) polyethylene mulch, PE; (ii) newsprint paper with no tackifier, NP; (iii) six percent psyllium husk, 6%PH; (iv) six percent guar gum, 6%GG; (v) two percent psyllium husk, 2%PH; and (vi) two percent guar gum, 2%GG. Means labeled with differing uppercase letters represent location and lowercase letters represent treatment differences at α = 0.05. Results are presented separately by location and means comparisons are made within each location.
3.3 Mulch deterioration
The effect of location and mulch treatment on mulch deterioration, characterized as percentage soil exposure (PSE), was influenced by a location × mulch treatment interaction (P = 0.017), therefore data is shown separately for each location (Figure 6). In general, PE mulch emerged as the most resistant to deterioration at both locations during the growing season. Specifically, in WA, mulch deterioration was similar among PE mulch, 2%PH and 6%GG mulches (0.5, 6.0 and 5.7 PSE, respectively; Figure 6), whereas 6%PH, 2%GG, and NP (7.2, 6.0, and 12.2 PSE, respectively) deteriorated more than PE mulch. At ND, mulch deterioration was similar among PE mulch, and 6% and 2%GG HMs (1.7, 3.2 and 5.4 PSE, respectively). Other hydromulch formulations (NP, 6%PH, and 2%PH) had greater mulch deterioration (14.9, 15.1, and 14.2 PSE, respectively) compared to both PE and GG formulations. These findings indicate the efficacy of GG formulations for increasing mulch resistance to deterioration.
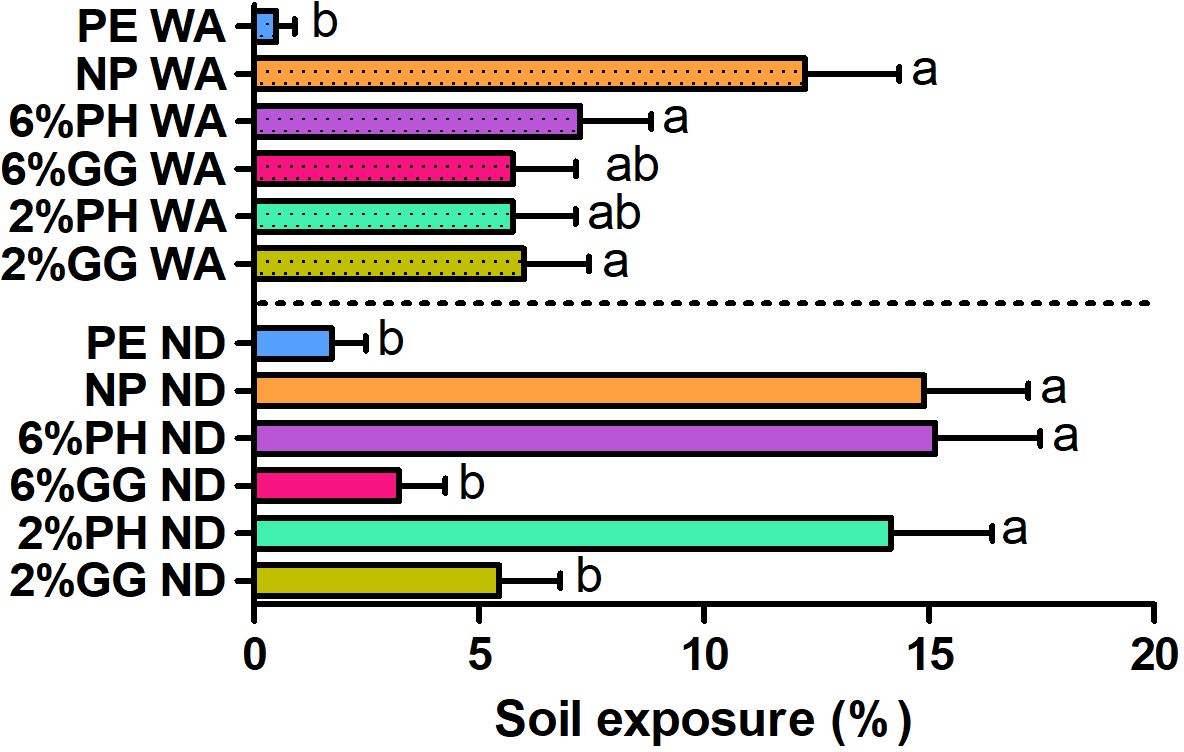
Figure 6 Mean + standard error percentage soil exposure (107 DAP at ND and 99 DAP at WA) for (i) polyethylene mulch, PE; (ii) newsprint paper with no tackifier, NP; (iii) six percent psyllium husk, 6%PH; (iv) six percent guar gum, 6%GG; (v) two percent psyllium husk, 2%PH; and (vi) two percent guar gum, 2%GG, as measured at Washington (WA) and North Dakota (ND) locations. Means labeled with different lowercase letters differed at α = 0.05. Means comparisons made within each location separately.
3.4 Strawberry fruit yield-weedy and weed-free
Total cumulative strawberry fruit yield (g plant-1), measured within the weedy area, was impacted by a mulch treatment × location interaction (P = <.0001), therefore results are presented separately by location (Figure 7A). At the WA location, mulch type did not affect total fruit yield (Figure 7A). However, in ND, PE mulch treatment had the lowest yield (5 g plant-1) and statistically differed from all the hydromulches. Remarkably, the total strawberry yield in the weedy area under PE mulch was 15 times less than the highest yielding HM formulation (6%GG, at 74 g plant-1). In ND, in the weedy area, total strawberry yield did not differ among the hydromulches (Figure 7A). Slicing the interaction revealed that within each mulch treatment, fruit yield at WA was greater than fruit yield at ND. Comparatively, the total yield of strawberries in the weedy area at WA surpassed the ND location by approximately fivefold, highlighting the substantial impact of environmental conditions on strawberry production.
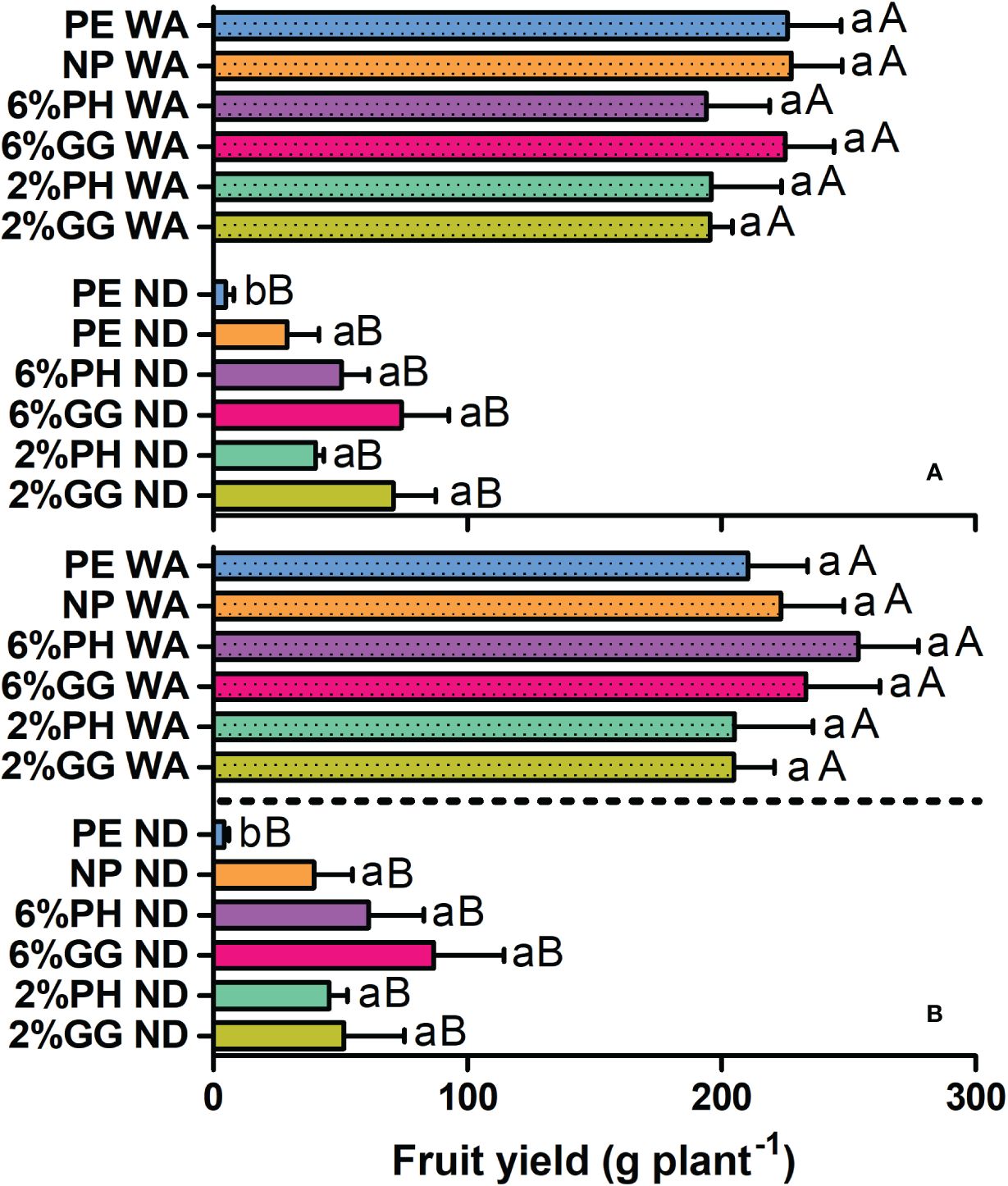
Figure 7 Mean + standard error total berry yield (g plant-1) measured in weedy (A) and weed-free (B) areas for (i) polyethylene mulch, PE; (ii) newsprint paper with no tackifier, NP; (iii) six percent psyllium husk, 6%PH; (iv) six percent guar gum, 6%GG; (v) two percent psyllium husk, 2%PH; and (vi) two percent guar gum, 2%GG, as measured at Washington (WA) and North Dakota (ND) locations. Means labeled with differing uppercase letters represent location and lowercase letters represent treatment differences at α = 0.05. Means comparisons made within each weed condition/location separately.
Total cumulative strawberry fruit yield (g plant-1) measured within the weed-free area was also impacted by a mulch treatment × location interaction (P = 0.0006), therefore results are presented separately by location (Figure 7B). In WA, mulches had no significant impact on total fruit yield. In ND, the total strawberry yield in the weed-free area was similar among hydromulch treatments, and all yielded significantly more than the PE mulch (Figure 7B). Slicing the interaction by mulch treatment revealed that within each mulch treatment, weed-free total fruit yield was greater at WA than ND. The average total strawberry yield in the weed-free area in WA was 222 g plant-1 compared to 48 g plant-1 in ND, suggesting regional environmental influences most notably a longer growing season in WA.
3.5 Strawberry canopy development and plant biomass
Crop canopy development was influenced by both mulch treatment (P = 0.0008) and location (P = 0.0001). Percentage canopy cover at WA was greater than at ND (52.1 vs. 42.7%). Pooled across locations, PE mulch was associated with greater canopy cover compared to NP with no tackifier and 2%GG (56.1 vs. 39.8 and 42.4%, respectively). The other HMs were associated with intermediate percentage canopy cover (Table 2).
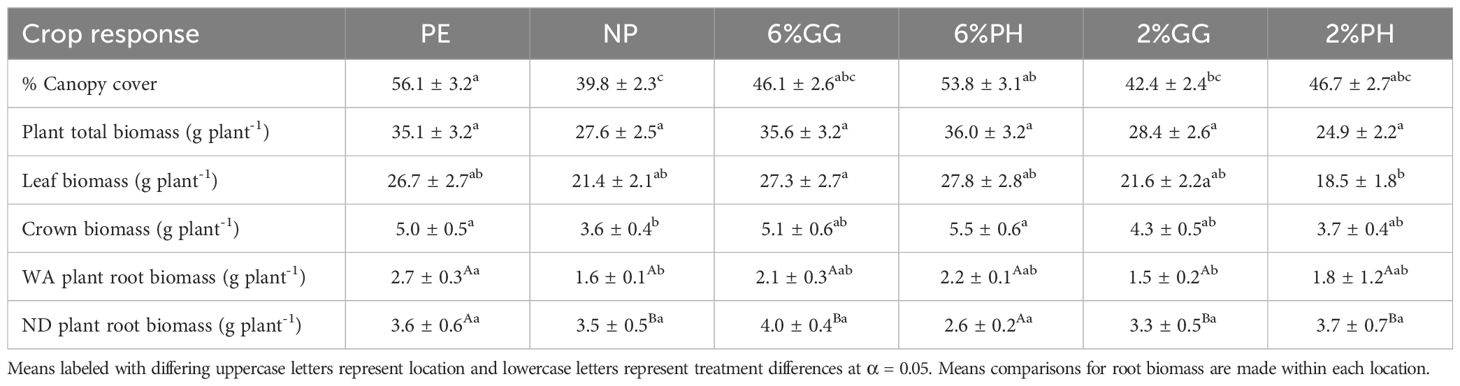
Table 2 Mean ± standard error for crop growth and development responses (107 DAP at ND and 134 DAP at WA): percentage strawberry canopy cover, strawberry plant total biomass, strawberry leaf biomass, strawberry plant crown biomass, strawberry root biomass at Washington and North Dakota for (i) polyethylene mulch, PE; (ii) newsprint paper with no tackifier, NP; (iii) six percent guar gum, 6%GG; (iv) six percent psyllium husk, 6%PH; (v) two percent guar gum, 2%GG; and (vi) two percent psyllium husk, 2%PH.
Location (P = 0.0007) and mulch treatment (P = 0.0144) both had significant impacts on strawberry plant total dry biomass. Total strawberry plant dry biomass was greater at the WA location (41 g plant-1) compared to the ND location (23 g plant-1). As shown in Table 2, 2%PH formulation was associated with the lowest strawberry plant total dry biomass (25 g plant-1) and was statistically similar to 6%PH and 6%GG (both 36 g plant-1) HMs. HMs showed statistical similarity with PE mulch (35 g plant-1) in their impact on strawberry plant total dry biomass, indicating similar outcomes to PE mulch on plant growth and development.
Strawberry plant leaf biomass differed among mulches (P = 0.0227) and location (P = 0.0033). Strawberry leaf biomass was significantly lower in 2%PH than in 6%GG (Table 2). Leaf biomass associated with PE mulch was similar to leaf biomass associated with HMs. The average plant leaf biomass across all mulch treatments was lower in ND (19 g plant-1) compared to WA (30 g plant-1). Strawberry plant crown dry biomass differed among mulches (P = 0.0155) and location (P = 0.0001). PE and 6%PH had the greatest crown biomass compared to NP (5.0 and 5.5 vs. 3.6 g plant-1, respectively). Average strawberry plant crown biomass at the WA location was three times higher compared to the ND location (2.6 vs. 7.7 g plant-1).
A significant interaction between location and treatment (P = 0.0409) was observed for strawberry plant root biomass. Mulch treatments (P = 0.0645) had no impact on root biomass at either location (Table 2), but strawberry plant root biomass at WA location was twice as high as observed in ND (data not shown). Thus, overall growth and development of strawberry plants in WA outperformed that of ND, leading to a significantly higher strawberry yield at the WA location compared to ND.
4 Discussion
4.1 Weed suppression efficacy
At both locations, black PE mulch was associated with zero weed presence at both the peak weed emergence and peak weed vegetative growth stages. Similarly, Johnson and Fennimore (2005) showed that black PE mulch was associated with 100% weed suppression efficacy compared to a no-mulch treatment. Furthermore, black PE mulch suppressed broadleaf weeds 100% during peak weed emergence but 74% efficacy was observed for grass weed suppression, indicating the ability of grasses [mostly foxtail species (Setaria spp.) and large crabgrass [Digitaria sanguinalis (L.)] to penetrate through the PE mulch. At peak vegetative growth stage, 2% reduction in broadleaf weed control in black PE mulch was attributed to the loss of mulch strength and weed emergence throughout the growing season (Masiunas et al., 2003). For the current study, grass weed density in ND was minimal and grass density in WA was approximately six times lower compared to broadleaf weed species. This lower grass weed density contributed to the complete absence of weed emergence associated with PE mulch.
At both WA and ND locations, NP hydromulch was found to be the least effective for controlling weeds during both peak emergence and peak vegetative growth stages. Using guar gum powder as a tackifier improved weed suppression by HMs at both locations, making these the most effective HM formulations for weed control. Psyllium tackifier demonstrated intermediate performance in hydromulches for weed suppression. Previous research in buffalo grass [Bouteloua dactyloides (Nutt.)] turfgrass establishment in hydromulch reduced weed vegetative cover at four weeks after planting compared to no mulch, gin trash, and wheat straw mulch (20% vs. 32%, 47%, and 60% cover, respectively) (Henry and Hoyle, 2021). Additionally, the hydromulch treatment (12 weeks after planting) maintained the lowest weed cover (22%) compared to no mulch (36%), gin trash (52%), and wheat straw (72%) treatments (Henry and Hoyle, 2021). The results from the present study were also consistent with Mas et al. (2021), where hydromulch treatments (rice husk and used mushroom substrate) suppressed weed rhizome sprouting (55% and 56%, respectively) compared to a non-mulch treatment (72% sprouting rate of weed rhizomes).
For the current study, the composition of hydromulch had a substantial impact on weed control, with GG hydromulch formulations proving to be the most effective and PH formulations performing at an intermediate level for weed suppression. In a separate study, ‘hydromulch A’ (paper pulp + non-crop-products + wheat straw) resulted in greater weed suppression, ranging from 93% to 86%, compared to ‘hydromulch B’ (paper pulp + non-crop-products + rice husk), ranging from 86% to 65% (Claramunt et al., 2020). These results reinforce that addition of specific functional components in hydromulch formulations can improve HM weed suppressive ability, similar to the significant outcomes observed with guar gum HM formulations in the present study. Previous research showed that black hydromulch performed similarly in suppressing weeds compared to black PE mulch during mid-season (1 vs. 2 plants m-2, respectively) and late-season (2 vs. 1 plants m-2, respectively) (Masiunas et al., 2003). These findings suggest that hydromulches can serve as an alternative to PE mulches for effective weed control in horticultural production. Overall, weed density in WA was greater compared to the ND location. Similar trends were observed at two different locations in FL (Gainesville with 329 plants m-2 and Loxahatchee with 50 plants m-2) during peak vegetative stage in a weedy control treatment (Warnick et al., 2006b).
Guar gum HM formulations were associated with minimal weed biomass at both locations compared to NP. Similar outcomes were noted when application of hydromulch ‘BSM1’ (Biobased sprayable mulch consisting of corn starch, corn gluten meal, isolated soy protein, glycerol, corn zein, keratin hydrolysate, eggshell powder, and water) resulted in significantly reduced weed biomass (0.046 g pot-1) compared to BSM2 (corn starch, corn gluten meal, isolated soy protein, glycerol, and water) and no mulch treatments (0.075 and 0.21 g pot-1, respectively) (Gloeb et al., 2023). Despite greater weed density in WA compared to the ND location, weed dry biomass remained similar at both locations.
We speculated that the guar gum tackifier, particularly at 6% concentration, has greater ability to enhance fiber particle bonding in newsprint paper compared to psyllium husk and newsprint paper without tackifier. This enhanced bonding improves resilience in the hydromulch sheet, preventing weed emergence, as evidenced by zero weeds in three replicates of 6%GG formulation in ND during peak weed emergence and vegetative growth stage. However, maximum puncture resistance of a sheet through which a weed can emerge is 0.5 MPa (Verdú et al., 2020). Durado (2023) confirmed the puncture resistance of 6%GG formulation as 1.43 MPa compared to 6%PH, 2%PH, and 2%GG (1.32, 1.00, and 0.87 MPa, respectively). Although these findings are based on laboratory testing, it is important to consider variations in field conditions such as irrigation intervals and rainfall that may compromise the strength of hydromulch, potentially leading to weed emergence. In contrast, PE mulches are water repellent and maintain their shape regardless of irrigation or rainfall.
4.2 Mulch stability
At ND, PE mulch, 2%GG, and 6%GG experienced the least deterioration compared to other HM formulations; whereas at WA PE, 2%PH, and 6%GG experienced the least deterioration compared to other HMs. These results suggest that HMs formulated with certain tackifiers can match PE mulch strength and resilience against mulch deterioration. Notably, at ND, NP hydromulch and psyllium formulation exhibited greater mulch deterioration compared to the GG HM formulations. Few previous hydromulch studies have included mulch deterioration assessments, but literature related to commercial biodegradable paper mulches and PE mulches suggests that HMs in the current study offer comparable mulch stability. Percent soil exposure in a commercial biodegradable plastic mulch (Clear Organix AG) reached 45% (Ghimire et al., 2020), approximately three times greater than NP hydromulch, which displayed maximum mulch deterioration in present study. All other mulches (Bio360, Exp. PLA/PHA, Naturecycle, Organix AG, and Polyethylene) demonstrated percent soil exposure ranging from 0 to 5% (Ghimire et al., 2020), similar to the range observed in the present study for the guar gum hydromulch formulations.
The findings indicate potential for guar gum hydromulch formulations to replace both PE and biodegradable plastic mulches, providing maximum mulch strength throughout the growing season. By comparison, brown-colored paper sheet mulch (WeedGuardPlus) was associated with a soil exposure of 15% (Zhang et al., 2020), three times higher on average compared to guar gum HMs tested in present study. Paper films, unlike liquid applied hydromulches, are placed on the soil surface without being firmly fixed to the soil. Due to this, paper mulches are prone to being dislodged by heavy wind (Harrington and Bedford, 2004). Additionally, paper mulches lack necessary flexibility and strength to withstand harsh weather conditions such as heavy rainfall and high winds (Haapala et al., 2014; Marí et al., 2019). Naturecycle and Weed Guard Plus mulch experienced 100% mulch deterioration, while BioAgri mulch was associated with 36% soil exposure at the end of the 2015 growing season in Knoxville, TN (Ghimire et al., 2018). In 2016, percent soil exposure for biodegradable mulches (BioAgri, Exp. PLA/PHA, Naturecycle, Organix, and WeedGuardPlus) ranged from 14.3% to 25% (Ghimire et al., 2018), surpassing the least stable hydromulch (NP) in the present study.
4.3 Strawberry growth and yield protection
Strawberry total fruit yield did not vary among any of the mulch treatments in WA. In ND, PE mulch resulted in strawberry fruit yield reduction, while all hydromulches impacted strawberry yield similarly. The average yield of strawberries in biodegradable plastic mulches was 375 g plant-1 (Andrade et al., 2014) in the Ribatejo Region, Portugal, compared to hydromulches in the present study (216 g plant-1). Notably, Andrade et al. (2014) highlighted 26% mean loss in strawberry crop yield with biodegradable mulches compared to PE mulch. In contrast, our study indicates that hydromulches did not show any substantial yield loss relative to PE mulch despite greater weed densities. This indicates that the presence of weeds did not have a substantial impact on strawberry yield. These results suggest that the hydromulch formulations in the present study provide similar, if not better, strawberry yield when compared to PE and biodegradable mulches. Hydromulches have not been tested previously in strawberry production, but a previous study with artichokes (Cynara cardunculus) demonstrated that yield in hydromulch treatments was similar to yield in PE mulch, indicating that HMs could be used as an alternative mulch without substantial yield loss (López-Marín et al., 2021).
Notably, total strawberry fruit yield in WA was greater compared to ND. The observed yield loss shown in PE mulch in ND could not be linked to weed competition, as no weeds were present. In ND, strawberry plants were transplanted three weeks later compared to WA due to wet soil conditions. Late strawberry planting led to a reduced period in early growth and vigorous plant establishment in ND, potentially leading to lower yield. A previous study noted that planting strawberries one week earlier in Morris, MN than in St. Paul MN was associated with 400 g vs. 340 g plant-1 strawberry fruit (Petran et al., 2017). Additionally, a yield reduction under black plastic mulch was observed in Albion day-neutral strawberries in Mount Vernon, WA, in 2015 (200 g plot-1) when plants were planted one-month late compared to 2014 [295 g plot-1] (DeVetter et al., 2017).
The selection of mulch color for strawberry production is critical, particularly considering the sensitivity of strawberry plants to mulch color (Johnson and Fennimore, 2005). In Salinas, CA, strawberry plants grown under black plastic mulch resulted in a similar yield to no-mulch treatment (900 g m-2), but strawberry fruit yield under clear plastic mulch (1200 g m-2) was comparatively greater (Johnson and Fennimore, 2005). In the Midwest region, greater strawberry yield was observed under white-on-black PE mulch compared to black PE mulch (Petran et al., 2017), indicating white-on-black plastic mulch as an optimal mulch option in ND.
Day-neutral strawberries grow best in temperatures between 7.2°C and 29.4°C, with growth stagnating outside this range. Cooling measures in such conditions can enhance flower initiation and fruit development (University of Minnesota Extension, 2023). In the present study at ND, the average daily maximum temperature under the black PE mulch exceeded 29.4°C multiple times during July 2022 (unpublished data). Surface mulch air temperature was not quantified, but during periods when the temperature below the plastic mulch exceeded 29.4°C, the surface temperature would probably have also been increased. This environmental stress, particularly during the first two weeks of bare root strawberry plant establishment, likely adversely affected the plants. Throughout the growing season, the temperature below the black plastic mulch was also greater compared to hydromulches (unpublished data). This elevated temperature may have negatively impacted flower initiation and fruit development in strawberries under black PE mulch, resulting in substantial reduced total yield. Additionally, strawberry plants under black PE mulch exhibited a notably low flowering rate, and the development of these flowers into fruits was also lower compared to hydromulches at ND. This observed phenomenon confirms the influence of higher temperatures on strawberry plants under the black plastic mulch. Previous research indicated that the temperature above 29.4°C results in reduced number of inflorescences, number of flowers, strawberry fruit set and fruit size (Ledesma et al., 2008). Black plastic mulches raise the soil temperature up to 6.1°C while white-on-black plastic mulches maintain soil temperature up to 2.2°C higher compared to bare soil (Heißner et al., 2005). Use of white-on-black plastic mulch as a standard control would have resulted in optimum strawberry yield vs. black PE mulch at ND. However, we wanted to have consistent treatments at ND and WA, which is one reason why black PE mulch was chosen.
The strawberry plant canopy percentage in PE mulch was statistically different from NP and 2%GG formulations. Strawberry plant canopy percentage was at peak in PE mulch, followed by 6%PH, 2PH, 6%GG, 2%GG, and NP hydromulch. These results align with the prior study by Wang et al. (2022), which indicated that PE mulch provided optimal strawberry crop canopy percentage compared to biodegradable plastic mulch and paper mulch. One might anticipate a similar pattern for strawberry crop canopy and leaf dry biomass across mulch treatments, but the present study revealed different outcomes from expectations. Strawberry leaf dry biomass showed statistical similarities for PE mulch vs. all hydromulch formulations. Observations made in previous study supported these results which indicated similar basil (Ocimum basilicum L.) shoot biomass among hydromulches and plastic mulch (Masiunas et al., 2003). However, strawberry leaf dry biomass was greater in 6%PH hydromulch, followed by 6%GG, PE mulch, 2%GG, NP, and 2%PH formulations.
In contrast, strawberry plant total dry biomass, composed of strawberry leaf, crown, and root dry biomass, did not differ among mulch treatments. Similar results were noticed in a previous study by DeVetter et al. (2017), where no significant differences were found in strawberry plant total dry biomass under black PE and biodegradable mulches. The influence of PE and biodegradable mulch on sweet corn (Zea mays conva. Saccharata var. rugosa) growth also showed equivalence, indicating that plant growth was not influenced by different mulch materials (Ghimire et al., 2020). The pattern of strawberry total dry biomass, as well as strawberry leaf and crown dry biomass, remained consistent among mulch treatments in this study. This alignment is anticipated, given that the leaf and crown biomass of strawberry plants contribute significantly to the overall biomass measurement of strawberries.
Interestingly, all hydromulch formulations were associated with similar strawberry root biomass at ND. On the other hand, PE mulch was associated with higher root dry biomass, compared to 2%GG and NP hydromulch at WA. These findings contrast with a previous study, where strawberry root biomass was not influenced by mulch treatments (DeVetter et al., 2017). However, DeVetter et al. (2017) used various non-biodegradable and soil-biodegradable black plastic mulches and the effects of black PE mulches on soil and canopy conditions is likely different compared to hydromulches due to color and material properties. Furthermore, we speculate that the variation in the pattern of strawberry crop canopy and root dry biomass may be attributed to differences in soil moisture and temperatures under mulches. Soil temperature and moisture beyond optimums for the plant are known to impact growth and development (Kadir et al., 2006). In a previous study, the rise in root zone temperature (30°C) reduced root oxygen consumption and resulted in a significant root cell damage in strawberry plants compared to low root zone temperature exposure (10°C and 20°C) (Sakamoto et al., 2016). Similarly, strawberry root dry weight was significantly reduced at 23°C than at 18°C and 13°C, while strawberry leaf and crown biomass remained similar across all three temperature treatments (Udagawa et al., 1989). This could explain the consistent patterns seen in strawberry leaf and crown biomass across mulches, in contrast to the varying outcomes observed in root biomass among mulch treatments. Strawberry plants showed insufficient growth under NP. Notably, the NP formulation also displayed maximum percent soil exposure. This suggests that NP hydromulch may not be effective in providing soil microclimatic modifications to enhance strawberry crop growth compared to HMs with tackifier and PE mulch. Further research is needed to explore the primary factors contributing to these variations in growth parameters under different mulch materials.
In the present study, strawberry plant total dry biomass, leaf dry biomass, crown, and root dry biomass at WA were significantly greater than at ND. This suggests that the growth conditions at WA were more favorable such as milder temperatures, longer growing season, and absence of late spring or early fall frost for strawberry plant growth and establishment compared to ND. The higher production of strawberry plant biomass at WA is consistent with the observed greater strawberry yield in that region. Conversely, due to lower plant strawberry biomass and suboptimal plant establishment at ND, an overall loss in strawberry plant yield was observed.
5 Conclusion
The frequent use of plastic mulches in strawberry production has raised concerns about environmental pollution and potential associated toxicity. Currently, none of the commercially available biodegradable plastic mulches are approved within the organic production system in the USA. Furthermore, using herbicides for weed control in organic production is not a viable option. In response to these challenges, hydromulch technology emerges as a promising alternative for weed suppression and enhanced strawberry yield in organic production systems. The results from this study emphasize that hydromulch formulations incorporating guar gum as a tackifier may be a promising substitute for PE mulches for weed suppression and yield enhancement in organic strawberry production under the conditions of our study. Moreover, it is evident that guar gum hydromulch provided season-long mulch stability and resilience against weed emergence in the respective areas of study and was comparable to PE mulch. For future directions, information on hydromulches durability in a multi-year strawberry trial is needed, as strawberries are often grown as a perennial crop (Hernández-Martínez et al., 2023).
Furthermore, hydromulch technology requires substantial improvements to make it suitable for certified organic commercial-scale production. Another important aspect that requires attention is the precision of the hydromulch applicator. Currently, the applicator sprays hydromulch with force, leading to undesired soil disturbance and potential mixing of weed seeds with the hydromulch slurry. An expert specializing in farm mechanization is required to enhance the hydromulch applicator precision, allowing the smooth application of hydromulch onto the soil surface without disturbance. In addition, food-grade tackifiers used in this study may prove too expensive for farmers engaged in profitable organic strawberry production. There is a need to explore cost-effective alternatives or test different tackifiers, particularly those derived as waste products. The current study involved the time-consuming process of shredding paper sheets or soaking them overnight in big plastic containers to achieve a homogeneous form of hydromulch slurry. However, for commercial organic production, adaptation to commercially available larger bales of shredded newsprint are essential. Such commercially available products will be more practical for farmers who lack the time to individually shred paper sheets. In summary, hydromulch technology needs further attention to reach its full potential for commercial organic horticulture production.
Data availability statement
The datasets presented in this study can be found in online repositories. The names of the repository/repositories and accession number(s) can be found below: https://hdl.handle.net/10365/33038.
Author contributions
WA: Writing – review & editing, Writing – original draft, Methodology, Investigation. LD: Writing – original draft, Writing – review & editing, Methodology, Investigation, Conceptualization. DM: Writing – review & editing, Investigation. BM: Writing – review & editing, Methodology, Investigation. DB: Writing – review & editing, Methodology, Conceptualization. AD: Writing – review & editing, Methodology. SW: Writing – original draft, Writing – review & editing, Conceptualization. SG: Writing – review & editing, Conceptualization. BW: Writing – review & editing. GG: Formal analysis, Writing – review & editing, Writing – original draft, Supervision, Project administration, Methodology, Investigation, Funding acquisition, Data curation, Conceptualization.
Funding
The author(s) declare financial support was received for the research, authorship, and/or publication of this article. Funded by USDA-NIFA Organic Research and Extension Initiative program, project number 2021-51300-34909.
Acknowledgments
USDA is an equal opportunity provider and employer. We sincerely thank Keith Biggers and Peter Gregoire for providing technical support. We also thank Andres Torres (graduate student) for his help conducing this experiment.
Conflict of interest
The authors declare that the research was conducted in the absence of any commercial or financial relationships that could be construed as a potential conflict of interest.
Publisher’s note
All claims expressed in this article are solely those of the authors and do not necessarily represent those of their affiliated organizations, or those of the publisher, the editors and the reviewers. Any product that may be evaluated in this article, or claim that may be made by its manufacturer, is not guaranteed or endorsed by the publisher.
References
Andrade C. S., Palha M. D. G., Duarte E. (2014). Biodegradable mulch films performance for autumn-winter strawberry production. J. Berry Res. 4, 193–202. doi: 10.3233/JBR-140080
Brodhagen M., Goldberger J. R., Hayes D. G., Inglis D. A., Marsh T. L., Miles C. (2017). Policy considerations for limiting unintended residual plastic in agricultural soils. Environ. Sci. Policy. 69, 81–84. doi: 10.1016/j.envsci.2016.12.014
Claramunt J., Mas M. T., Pardo G., Cirujeda A., Verdú A. M. (2020). Mechanical characterization of blends containing recycled paper pulp and other lignocellulosic materials to develop hydromulches for weed control. Biosyst. Engineering. 191, 35–47. doi: 10.1016/j.biosystemseng.2019.12.012
Cline J., Neilsen G., Hogue E., Kuchta S., Neilsen D. (2011). Spray-on-mulch technology for intensively grown irrigated apple orchards: Influence on tree establishment, early yields, and soil physical properties. HortTechnology 21, 398–411. doi: 10.21273/HORTTECH.21.4.398
DeVetter L. W., Zhang H., Ghimire S., Watkinson S., Miles C. A. (2017). Plastic biodegradable mulches reduce weeds and promote crop growth in day-neutral strawberry in western Washington. HortScience 52, 1700–1706. doi: 10.21273/HORTSCI12422-17
Durado A. D. (2023). Biodegradable composite hydromulches for sustainable organic horticulture. Montana State University, Bozeman (MT.
Environmental Planning Consultant (2006) Municipal solid waste generation, recycling and disposal in the United States: 2006 facts and figures. Available at: https://archive.epa.gov/epawaste/nonhaz/municipal/web/pdf/msw06.pdf (Accessed November 4, 2022).
Environmental Research and Education Foundation (2022) Analysis of MSW landfill tipping fees-2022. Available at: https://erefdn.org/2022-msw-landfill-tipping-fees/ (Accessed January 22, 2024).
Faucette L. B., Risse L. M., Jordan C. F., Cabrera M. L., Coleman D. C., West L. T. (2006). Vegetation and soil quality effects from hydroseed and compost blankets used for erosion control in construction activities. J. Soil Water Conserv. 61, 355–362.
Fortune Business Insights (2020) Organic barriers market size, share & covid-19 impact analysis, by type (strawberries, raspberries, cranberries, gooseberries, blueberries, and others), application (food and beverage, pharmaceuticals, cosmetics, and personal care, and household), processing type (processed and fresh), and regional forecast 2020–2027. Available at: https://www.fortunebusinessinsights.com/organic-berries-market-103191 (Accessed January 10, 2024).
Geyer R., Jambeck J. R., Law K. L. (2017). Production, use, and fate of all plastics ever made. Sci. Advances. 3, e1700782. doi: 10.1126/sciadv.1700782
Ghimire S., Scheenstra E., Miles C. A. (2020). Soil-biodegradable mulches for growth, yield, and quality of sweet corn in a Mediterranean-type climate. HortScience 55, 317–325. doi: 10.21273/HORTSCI14667-19
Ghimire S., Wszelaki A. L., Moore J. C., Inglis D. A., Miles C. (2018). The use of biodegradable mulches in pie pumpkin crop production in two diverse climates. HortScience 53, 288–294. doi: 10.21273/HORTSCI12630-17
Giampieri F., Forbes-Hernandez T. Y., Gasparrini M., Alvarez-Suarez J. M., Afrin S., Bompadre S., et al. (2015). Strawberry as a health promoter: an evidence based review. Food Funct. 6, 1386–1398. doi: 10.1039/C5FO00147A
Gloeb E., Irmak S., Isom L., Lindquist J. L., Wortman S. E. (2023). Biobased sprayable mulch films suppressed annual weeds in vegetable crops. HortTechnology 33, 27–35. doi: 10.21273/HORTTECH05112-22
Goldberger J. R., DeVetter L. W., Dentzman K. E. (2019). Polyethylene and biodegradable plastic mulches for strawberry production in the United States: Experiences and opinions of growers in three regions. HortTechnology 29, 619–628. doi: 10.21273/HORTTECH04393-19
Granatstein D., Kirby E., Willer H. (2013). Global area and trends of organic fruit production. Acta Horticulturae. 1001, 383–394. doi: 10.17660/ActaHortic.2013.1001.45
Haapala T., Palonen P., Korpela A., Ahokas J. (2014). Feasibility of paper mulches in crop production—a review. Agric. Food Science. 23, 60–79. doi: 10.23986/afsci.8542
Harrington K. C., Bedford T. A. (2004). Control of weeds by paper mulch in vegetables and trees. New Z. Plant Protection. 57, 37–40. doi: 10.30843/nzpp.2004.57.6938
Heißner A., Schmidt S., von Elsner B. (2005). Comparison of plastic films with different optical properties for soil covering in horticulture: test under simulated environmental conditions. J. Sci. Food Agriculture. 85, 539–548. doi: 10.1002/jsfa.1862
Henry G., Hoyle J. (2021). Weed pressure and turfgrass establishment in response to mulching media. Agron. J. 113, 3764–3770. doi: 10.1002/agj2.20464
Hernández-Martínez N., Blanchard C., Wells D., Salazar-Gutiérrez M. (2023). Current state and future perspectives of commercial strawberry production: A review. Scientia Horticulturae. 312, 111893. doi: 10.1016/j.scienta.2023.111893
Johnson M. S., Fennimore S. A. (2005). Weed and crop response to colored plastic mulches in strawberry production. HortScience 40, 1371–1375. doi: 10.21273/HORTSCI.40.5.1371
Kadir S., Sidhu G., Al-Khatib K. (2006). Strawberry (Fragaria× ananassa Duch.) growth and productivity as affected by temperature. HortScience 41, 1423–1430. doi: 10.21273/HORTSCI.41.6.1423
Kasirajan S., Ngouajio M. (2012). Polyethylene and biodegradable mulches for agricultural applications: a review. Agron. Sustain. Dev. 32, 501–529. doi: 10.1007/s13593-011-0068-3
Lamont W. (2004). “Plastic mulches,” in Production of Vegetables, Strawberries, and Cut Flowers Using Plasticulture. Ed. Lamont W. (Natural Resource, Agriculture, and Engineering Service, Ithaca, NY), 9–12. Available at: https://hdl.handle.net/1813/69448.
Ledesma N. A., Nakata M., Sugiyama N. (2008). Effect of high temperature stress on the reproductive growth of strawberry cvs.’Nyoho’and ‘Toyonoka’. Scientia Horticulturae. 116, 186–193. doi: 10.1016/j.scienta.2007.12.010
Liu E. K., He W. Q., Yan C. R. (2014). ‘White revolution’to ‘white pollution’—agricultural plastic film mulch in China. Environ. Res. Letters. 9, 91001. doi: 10.1088/1748–9326/9/9/091001
López-Marín J., Romero M., Gálvez A., del Amor F. M., Piñero M. C., Brotons-Martínez J. M. (2021). The use of hydromulching as an alternative to plastic films in an artichoke (Cynara cardunculus cv. Symphony) Crop: A study of the economic viability. Sustainability 13, 5313. doi: 10.3390/su13095313
Marí A. I., Pardo G., Cirujeda A., Martínez Y. (2019). Economic evaluation of biodegradable plastic films and paper mulches used in open-air grown pepper (Capsicum annum L.) crop. Agronomy 9, 36. doi: 10.3390/agronomy9010036
Mas M. T., Pardo G., Pueyo J., Verdú A. M., Cirujeda A. (2021). Can hydromulch reduce the emergence of perennial weeds? Agronomy 11, 393. doi: 10.3390/agronomy11020393
Masiunas J., Wahle E., Barmore L., Morgan A. (2003). A foam mulching system to control weeds in tomatoes and sweet basil. HortTechnology 13, 324–328. doi: 10.21273/HORTTECH.13.2.0324
Miles C., DeVetter L., Ghimire S., Hayes D. G. (2017). Suitability of biodegradable plastic mulches for organic and sustainable agricultural production systems. HortScience 52, 10–15. doi: 10.21273/HORTSCI11249-16
Miles C. A., Madrid B., DeVetter L., Weiss B. (2023) Soil-biodegradable plastic mulch for organic production systems. Available at: https://s3.wp.wsu.edu/uploads/sites/2181/2023/04/BDM-Organic.pdf.
Moreno M. M., Villena J., González Mora S., Morales P. A., Atance C., Ortega C., et al. (2022). Evolution and behaviour of hydromulches in organic woody crops under open field and container conditions (EGU General Assembly 2022, EGU22–11013). Abstract retrieved from Abstracts in Astrophysics Data System. (Session SSS9.4). doi: 10.5194/egusphere-egu22–11013
NCAT-ATTRA Sustainable Agriculture (2007) Strawberries: organic production. Available at: https://attra.ncat.org/publication/strawberries-organic-production/ (Accessed November 08, 2023).
Ng E. L., Lwanga E. H., Eldridge S. M., Johnston P., Hu H. W., Geissen V., et al. (2018). An overview of microplastic and nanoplastic pollution in agroecosystems. Sci. Total Environment. 627, 1377–1388. doi: 10.1016/j.scitotenv.2018.01.341
OSU Extension Service (2019) Strawberry Nutrient Management Guide for Oregon and Washington. Available at: https://catalog.extension.oregonstate.edu/em9234/html (Accessed February 22, 2023).
Patrignani A., Ochsner T. E. (2015). Canopeo: A powerful new tool for measuring fractional green canopy cover. Agron. J. 107, 2312–2320. doi: 10.2134/agronj15.0150
Petran A., Hoover E., Hayes L., Poppe S. (2017). Yield and quality characteristics of day-neutral strawberry in the United States Upper Midwest using organic practices. Biol. Agric. Horticulture. 33, 73–88. doi: 10.1080/01448765.2016.1188152
Pritts M. P., Kelly M. J. (2001). Early season weed competition reduces yield of newly planted matted row strawberries. HortScience 36, 729–731. doi: 10.21273/HORTSCI.36.4.729
Puka-Beals J., Gramig G. (2021). Weed suppression potential of living mulches, newspaper hydromulches, and compost blankets in organically managed carrot production. HortTechnology 31, 89–96. doi: 10.21273/HORTTECH04745-20
Ricks M. D., Wilson W. T., Zech W. C., Fang X., Donald W. N. (2020). Evaluation of hydromulches as an erosion control measure using laboratory-scale experiments. Water 12, 515. doi: 10.3390/w12020515
Sakamoto M., Uenishi M., Miyamoto K., Suzuki T. (2016). Effect of root-zone temperature on the growth and fruit quality of hydroponically grown strawberry plants. J. Agric. Science. 8, 122–131. doi: 10.5539/jas.v8n5p122
Salceanu C., Olaru L. (2017). Researches on weed control on strawberries. Ann. Univ. Craiova-Agriculture Montanology Cadastre Series. 46, 271–275.
Sarkar D. J., Barman M., Bera T., De M., Chatterjee D. (2018). “Agriculture: Polymers in crop production mulch and fertilizer,” in Encyclopedia of polymer applications, 28–47 Boca Raton, FL: CRC Press Taylor & Francis Group.
Serrano-Ruiz H., Martin-Closas L., Pelacho A. M. (2021). Biodegradable plastic mulches: Impact on the agricultural biotic environment. Sci. Total Environment. 750, 141228. doi: 10.1016/j.scitotenv.2020.141228
Simpson D. (2018). “The economic importance of strawberry crops,” in The Genomes of Rosaceous Berries and Their Wild Relatives. Eds. Hytönen T., Graham J., Harrison R. (Springer International Publishing AG, Cham, Switzerland), 1–7. doi: 10.1007/978–3-319–76020-9
Soil Survey Staff (1988) fficial Soil Series Description- Field Series USDA-NRCS. Available at: https://soilseries.sc.egov.usda.gov/OSD_Docs/F/FIELD.html (Accessed November 14, 2023).
Soil Survey Staff (2000) Official Soil Series Description- Warsing Series USDA-NRCS. Available at: https://soilseries.sc.egov.usda.gov/OSD_Docs/W/WARSING.html (Accessed November 14, 2023).
Udagawa Y., Ito T., Gomi K. (1989). Effects of root temperature on some physiological and ecological characteristics of strawberry plants′ reiko′ grown in nutrient solution. J. Japanese Soc. Hortic. Science. 58, 627–633. doi: 10.2503/jjshs.58.627
University of Minnesota Extension (2023) Day-neutral strawberries. Available at: https://extension.umn.edu/strawberry-farming/day-neutral-strawberries#:~:text=Strawberries%20have%20shallow%20root%20systems,performs%20well%20for%20this%20purpose (Accessed December 20, 2023).
Valencia M. M. M., Villena J., González-Mora S., Atance C., Ortega C., Ruiz-Orejón A., et al. (2021). Hydromulches as a possible alternative to herbicides in organic woody crops (EGU General Assembly 2021, EGU21–13141). Abstract retrieved from Abstracts in Astrophysics Data System. (Session SSS9.7). doi: 10.5194/egusphere-egu21–13141
Vaughn S. F., Kenar J. A., Felker F. C., Berhow M. A., Cermak S. C., Evangelista R. L., et al. (2013). Evaluation of alternatives to guar gum as tackifiers for hydromulch and as clumping agents for biodegradable cat litter. Ind. Crops Products. 43, 798–801. doi: 10.1016/j.indcrop.2012.09.002
Velandia M., Smith A., Wszelaki A., Galinato S. (2019). The economic feasibility of adopting plastic biodegradable mulches in pumpkin production (EconPapers), 302733. Available at: https://econpapers.repec.org/scripts/redir.pf?u=https%3A%2F%2Fdoi.org%2F10.22004%252Fag.econ.302733;h=repec:ags:utaeer:302733.
Verdú A. M., Mas M. T., Josa R., Ginovart M. (2020). The effect of a prototype hydromulch on soil water evaporation under controlled laboratory conditions. J. Hydrology Hydromechanics. 68, 404–410. doi: 10.2478/johh-2020-0018
Wang X., Shrestha S., Tymon L., Zhang H., Miles C., DeVetter L. (2022). Soil-biodegradable mulch is an alternative to non-biodegradable plastic mulches in a strawberry-lettuce double-cropping system. Front. Sustain. Food Systems. 6. doi: 10.3389/fsufs.2022.942645
Warnick J. P., Chase C. A., Rosskopf E. N., Scholberg J. M., Simonne E. H., Koenig R. L. (2006a). Hydramulch for muskmelon and bell pepper crop production systems. J. Vegetable Science. 12, 39–55. doi: 10.1300/J484v12n02_05
Warnick J. P., Chase C. A., Rosskopf E. N., Simonne E. H., Scholberg J. M., Koenig R. L., et al. (2006b). Weed suppression with hydramulch, a biodegradable liquid paper mulch in development. Renewable Agric. Food Systems. 21, 216–223. doi: 10.1079/RAF2006154
Yuan Z., Nag R., Cummins E. (2022). Human health concerns regarding microplastics in the aquatic environment-From marine to food systems. Sci. Total Environment. 823, 153730. doi: 10.1016/j.scitotenv.2022.153730
Zhang H., DeVetter L. W., Scheenstra E., Miles C. (2020). Weed pressure, yield, and adhesion of soil-biodegradable mulches with pie pumpkin (Cucurbita pepo). HortScience 55, 1014–1021. doi: 10.21273/HORTSCI15017-20
Keywords: biodegradable mulches, sustainable agriculture, plastic mulch, weed management, sprayable mulch
Citation: Ahmad W, DeVetter LW, McFadden D, Maupin B, Bajwa DS, Durado A, Weyers S, Galinato SP, Weiss B and Gramig G (2024) Hydromulches suppress weeds and maintain fruit production in organically managed strawberry systems. Front. Agron. 6:1375505. doi: 10.3389/fagro.2024.1375505
Received: 23 January 2024; Accepted: 23 April 2024;
Published: 31 May 2024.
Edited by:
Stéphane Cordeau, UMR Agroécologie, FranceReviewed by:
Ioannis Roussis, Agricultural University of Athens, GreeceStephen Christopher Marble, University of Florida, United States
Copyright © 2024 Ahmad, DeVetter, McFadden, Maupin, Bajwa, Durado, Weyers, Galinato, Weiss and Gramig. This is an open-access article distributed under the terms of the Creative Commons Attribution License (CC BY). The use, distribution or reproduction in other forums is permitted, provided the original author(s) and the copyright owner(s) are credited and that the original publication in this journal is cited, in accordance with accepted academic practice. No use, distribution or reproduction is permitted which does not comply with these terms.
*Correspondence: Greta Gramig, Z3JldGEuZ3JhbWlnQG5kc3UuZWR1