- 1Dohne Agricultural Development Institute, Stutterheim, South Africa
- 2Department of Animal and Wildlife Sciences, University of Pretoria, Pretoria, South Africa
- 3Department of Plant and Soil Sciences, University of Pretoria, Pretoria, South Africa
The ecosystems across the world are affected by diverse impacts of climate change. Climate change will also affect soil seed bank as the seed bank is determined by the plants that are above the ground. This study was conducted to determine whether rainfall interception and resting period will affect the soil seed bank or not. Rainfall interception was employed as main plot treatment at four different levels—namely, 15%, 30%, 60%, and 0%—and the main plot treatments were each replicated five times using the rainout shelters arranged as main plot treatments in a split-plot experimental design. The main plots are 49 m2 (7 m × 7 m) in size and were covered by metal frame structures with V-shaped clear acrylic bands on top without ultraviolet filter, and these plots were sub-divided into two sub-plots to determine the effect of resting periods (70 and 90 days). The soil samples were 8 cm in diameter and 20 cm in depth (1,005 cm3). We used seed emergence method rather than seed separation method to determine the soil seed bank due to the very small size of some seeds for some species. The 60% rainfall interception resulted in significantly high forb densities at both resting periods. The forb densities decreased by 32 and 35% at 15% and 30%, while they increased by 195% at 60% rainfall interception compared to 0% rainfall interception at 70-day resting period. Resting period significantly affected grass densities at 60% rainfall as the densities were higher at 70- than 90-day resting period. At 90-day resting period, grass densities were significantly higher at 0% rainfall interception than the rest of the treatments, while the grass densities were significantly lower at 60% rainfall interception. The 60% rainfall interception resulted in significantly (p< 0.05) higher total densities (forbs and grass) in both resting periods. Total density increased by 2.9% and 143.48% at 15% and 60% rainfall interception, respectively. Our results show that drought has a negative effect on grass soil seed bank, while it improves the forbs soil seed bank. Furthermore, the resting period has no significant effects on soil seed bank in grasslands.
1 Introduction
The ecosystems across the world are affected by diverse impacts of climate change. Climate change will also affect soil seed bank as it is determined by the plants that are above the ground. Soil seed bank is the repository for the next generation of plants; these are the seeds on the soil surface and within the soil (Hu et al., 2019). Soil seed bank is very important in grassland productivity as it is the basis for survival, population settlement, expansion, and reproduction (Milberg and Hansson, 1993). History and the current situation of a community is reflected in the soil seedbank (Thompson, 2000; Kalamees et al., 2012). Any factors contributing to vegetation productivity or reduction in biodiversity and grassland degradation can affect the size and the composition of the soil seed bank (Dreber and Esler, 2011; Báldi et al., 2013). The distribution of rainfall also affects seed production and release (Gutterman, 2012). Disturbance related to environmental changes influence the overall heterogeneity in the soil seed bank (Solomon et al., 2006; Kassahun et al., 2009).
By allowing a part of their offspring into a dormancy and therefore forming seed bank, plants commonly delay their reproduction (Baskin and Baskin, 1998). A reduced mean annual number of offspring is because of delayed reproduction which is an apparent maladministration (Aikio et al., 2002). Soil seed bank is an important aspect of the grassland as it determines its current and future condition. Seed recruitment from the soil seed bank is restricted to soil parameters and periods with favorable conditions for seed germination (Solomon et al., 2006). Soil water is one of the important variables in this regard (Snyman, 2004), along with soil pH (Henig-Server et al., 1996), light (Trabaud and Renard, 1999), and heat exposure (Snyman, 2005).
According to del Cacho et al. (2012), simulated climate experiments provide the best approach to study the processes that determine the community changes at stand level. Most farmers rely on the natural rangeland for all-year-round grazing of their livestock, and therefore the status of the soil seed bank is important to understand how climate change will affect the soil seed bank to prepare for the future. This will also assist farmers to understand the measures that can be undertaken to restore the rangeland. Understanding the deterioration of the rangelands will also assist on how they can be restored (Solomon et al., 2006). Climate manipulation may affect soil seed bank when the seed input is not depleted by the germination due to unfavorable conditions imposed by climate modification (del Cacho et al., 2012). Grassland researchers and scientists predict a reduction in cover and productivity due to summer droughts (Hufkens et al., 2016; Basto et al., 2018). However, some studies on climate manipulations show that these responses will defer from community to community and soil properties (Fridley et al., 2011). Furthermore, some components such as soil seed bank have not been incorporated enough in studies that evaluate the impact of climate change on rangeland productivity. Most rangeland studies in Africa focus only on the aboveground vegetation community and ignore the soil seed bank which also requires attention. Therefore, this study was conducted to determine whether the rainfall interception and resting period have a significant effect on the soil seed bank or not. We examined the forbs and grass composition and density in four different rainfall interceptions (0%, 15%, 30%, and 60%) and two resting periods (70 and 90 days). Resting period was employed to determine the period required to allow seed dispersal when the veld is rested.
2 Materials and methods
2.1 Site
The study was conducted at the University of Pretoria, Hatfield Experimental Farm. The farm is situated at an altitude of 1,372 m above sea level. The coordinates of the study site are 25° 45′ S and 28° 16′ E. The farm is situated in a summer rainfall area with a long-term mean annual precipitation of 674 mm with hot summers and occasional rainstorms. The soil on the farm is categorized as non-calcareous with a homogenous red color, with a weak structure of Hutton form, and is sandy loam with 20%–35% clay (Soil Classification Working Group, 1991) and a pH of 6 in a 2.5:1 soil–water ratio. The study site has not been grazed for more than 65 years. The research was conducted by using rainout shelters constructed on natural semi-arid grassland vegetation as described by Mucina and Rutherford (2006).
2.2 Rainout shelters and resting periods
Rainout shelters were established as described by Yahdjian and Sala (2002) and modified by Talore (2015). Rainfall interception was employed as main plot treatment at four different levels—namely, 15%, 30%, 60% and 0% (control/ambient)—and the main plot treatments were each replicated five times using the rainout shelters arranged as main plot treatments in a split-plot experimental design (Figure 1). These rainfall reduction treatments are based on the projected precipitation reductions of 20% between 2071 and 2099 in the Southern African region (Jury, 2013). The main plots are 49 m2 (7 m × 7 m) in size and are covered by metal frame structures with V-shaped clear acrylic bands on top without ultraviolet filter, and these plots are sub-divided into two sub-plots to determine the effect of resting periods (70 and 90 days). The resting period was determined by cutting grass at intervals of 70 and 90 days. A 25-cm ditch was excavated along the boundary of the experimental site to avoid water runoff into the plots. The bands were constructed with a longitudinal plate of 120°, and a mean height of 1 m at the lowest side of the shelter was maintained. Water was collected from the acrylic material and channeled through the gutters away from the plots. Ambient rainfall was measured after each rainfall event using five rain gauges that were scattered over the experimental site.
2.3 Soil and soil seed bank sampling
Sagar and Mortimer (1976) defined soil seed bank as seeds that are on top or beneath the soil surface that are capable to germinate. The soil samples were collected in August 2018 and August 2019. This sampling period was after the seed production event but before seed germination. According to Solomon et al. (2006), this can justify that viable seeds did not germinate in the field over the season as the rains start in September. Four soil samples were taken per sub-plot (70- and 90-day resting periods). The four soil samples per sub-plot were bulked together to get five replications per sub-plot treatment. The soil samples were 8 cm in diameter and 20 cm in depth (1,005 cm3). The samples were placed in plastic bags. The soil was passed through a 5-mm sieve to remove any stones and roots that could have been in the soil and was kept in a cold room while preparing the space at the glass house. We used seed emergence method other than seed separation method to determine the soil seed bank due to the very small size of some seeds of some species such as Eragrostis curvula, although the method could result in underestimation of the seed bank (Leck et al., 1989; Baskin and Baskin, 1998; del Cacho et al., 2012).
Before placing soil seed bank in the trays, we placed sterile filter sand in the germination trays. The filter sand was irrigated for a period of 3 weeks to ensure that no other plants would grow except the ones that were in the soil seed bank. The soil samples were spread in thin layers of about 2 cm in depth over the germination trays. The soil was kept in the trays for a period of 3 months, i.e., September, October, and November 2018 and 2019. Germination trays were randomly placed in a glass house and were irrigated daily to maintain soil moisture. Seedling emergence was recorded every second day from September 1, 2018 to November 28, 2019, after which there was no emergence. Grasses were identified using van Oudtshoorn (2012). Forb species were identified at the South African National Biodiversity Institute (SANBI) in Forb species were identified at the South African National Biodiversity Institute (SANBI) 2018 and were identified at the University of Pretoria Herbarium in 2019.
3 Data analysis
Data for seedling emergence was analyzed using the general linear model procedure (Proc GLM) of SAS (2008). The rainfall interceptions and the resting periods were the fixed effects, while the blocks (plots arranged in rows) were the random effects. Seedling emergence was the number of seedlings that emerged in each tray. The model was designed to determine whether rainfall interception and resting period have a significant effect on soil seed bank or not. The seedlings were separated into non-grasses (forbs) and grasses. Grasses and forbs were response variables, while rainfall interception and resting period were response variables while rainfall interception and resting period were the studied factors.. The statistical were conducted separate for each group. Densities per tray and per square meter were analyzed for forbs, grasses, and total number of seedlings. Raw data was tested for normality and where it fails log transformed data were statistically anlaysed. However, the actual means were presented in Tables and Figures.
Species diversity was calculated using Simpson’s index (Yadav and Misshra, 2013):
where D = diversity, ni=total number of plant species of each individual species, and N = the total number of all plant species
Species richness was calculated using Margalef’s index (Margalef, 1958):
where S = total number of species, N = total number of individuals in the sample, and ln = natural logarithm
4 Results
4.1 Species composition
A total of seven grass species emerged from the soil seed bank, and all the grasses were perennial species in 2018. These grasses were identified using van Oudtshoorn (2012). Forb species were identified at the South African National Biodiversity Institute (SANBI) in 2018 (Table 1), while they were identified at the University of Pretoria Herbarium in 2019 (Table 2). A total of 26 plant species were identified in 2018. Out of the 26 plant species, 19 were forbs, while seven were grasses. In 2019, a total of 25 plant species were identified. Out of the 25 plant species, seven were grasses, 17 were forbs, and one was sedge. In 2018, plants were identified into species level without taking into consideration the effect of resting period treatment as some of the plants died while the forbs were being identified at SANBI.
4.2 Grass abundance per tray
Grasses were identified at the functional group level during emergence as it is difficult to identify at the species level during seedling stage. Rainfall interception and resting period interaction had no significant effect on grass seedling emergence in both years (Table 2). However, rainfall interception had a significant effect on grass species abundance in both years. On the other hand, resting period had no significant effect (p > 0.05) on grass soil seed bank abundance in 2018, while it had a significant effect on the grass soil seed bank in 2019. Grass soil seed bank was significantly (p< 0.05) lower at 60% rainfall interception in both years. Moreover, resting period had no significant effect on grass soil seed bank abundance in 2018, while 90-day resting period had a significantly high grass soil seed bank abundance than 70-day resting period compared to 0% rainfall interception in 2019. The grass soil seed bank abundance decreased by 49.5% at 60% rainfall interception in 2018 while it decreased by 39.4% at 60% rainfall interception compared to 0% rainfall interception in 2019.
4.3 Forbs abundance per tray
Rainfall interception had a significant (p< 0.05) effect on forbs abundance in both years (Table 3). The forbs abundance was significantly (p< 0.05) higher at 60% rainfall interception compared to 0% rainfall interception in both years. The forbs abundance increased by 64% and 46% at 60% rainfall interception compared to 0% rainfall interception in 2018 and 2019, respectively. On the other hand, 70-day resting period resulted in significantly high forbs abundance compared to 90-day resting period in 2019. On the other hand, resting period hadno significant effect on forbs abundance in 2018.
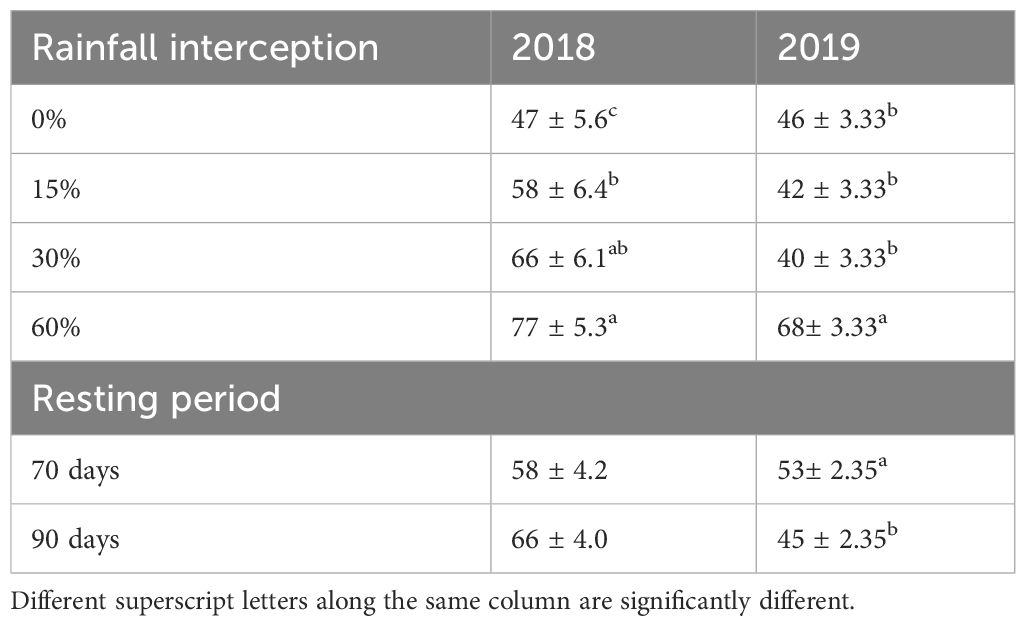
Table 3 Effect of rainfall interception and resting period on forbs soil seed bank abundance (%) in 2018 and 2019.
4.4 Forbs densities
The resting period and rainfall interception had no significant effect on forbs soil seed bank densities in both years after data was log-transformed (Figures 2A, B), the actual values were displayed. Resting period had no significant effect on forbs soil seed bank densities per square meter, while rainfall interception had a significant effect in both years. Forbs soil seed bank densities increased with the increase in rainfall interception. The soil seed bank densities of the forbs increased by 176% and 26% at 60% rainfall interception in 2018 and 2019, respectively.
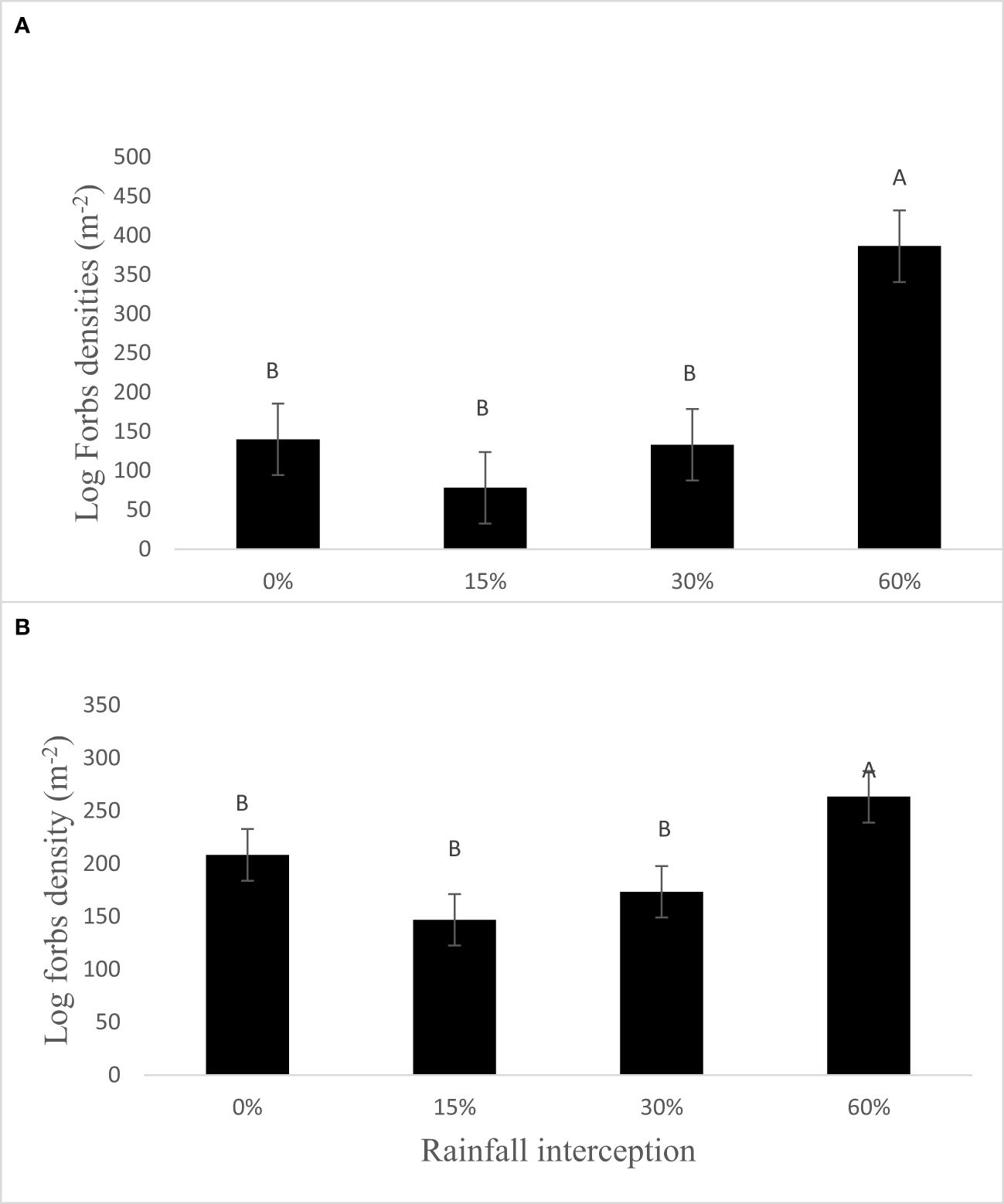
Figure 2 Effect of rainfall interception and resting period on forbs seed densities per square meter in (A) 2018 and (B) 2019. Bars indicate standard error values, while different letters indicate values that are significantly different.
4.5 Grass densities
Rainfall interception and resting period interaction had no significant effect on grass soil seed bank densities in both years (Table 4). In 2018 resting period, rainfall interception and their interaction had no significant effect on grass soil seed bank densities. However, in 2019, both resting period and rainfall interception had a significant effect on grass soil seed bank densities, while their interaction had no significant effect. Although rainfall interception had no significant effect on grass soil seed bank density in 2018, it was reduced by 21% at 60% rainfall interception compared to 0% rainfall interception. Moreover, in 2019, rainfall interception significantly reduced grass soil seed bank density by 52% at 60% rainfall interception compared to 0% rainfall interception, while 90-day resting period resulted in significantly high grass soil seed bank density compared to 70-day resting period.
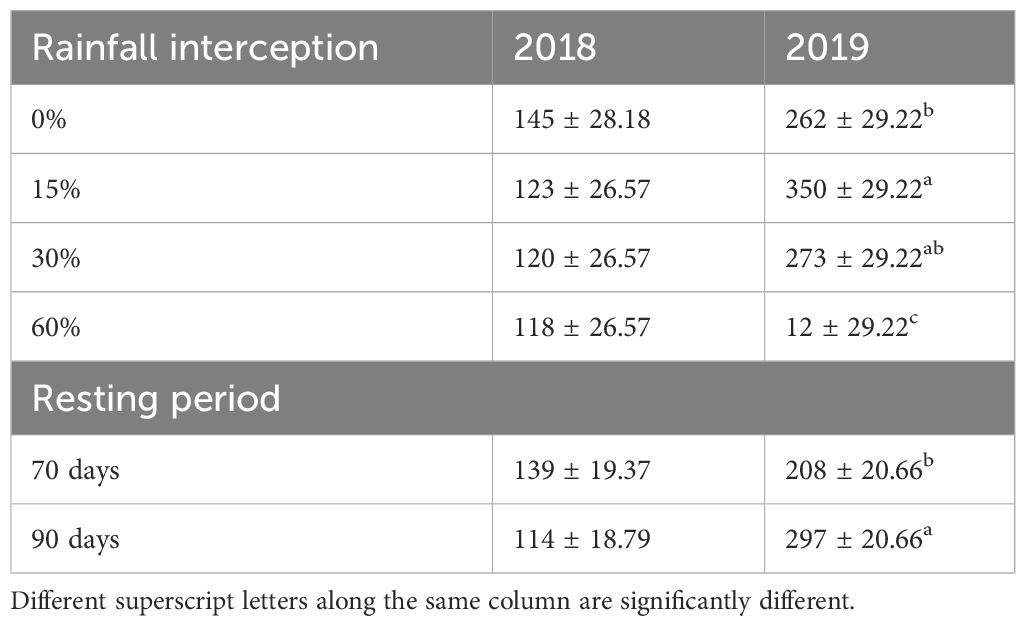
Table 4 Effect of rainfall interception and resting period on grass seed densities per square meter in 2018 and 2019.
4.6 Total densities per square meter
Rainfall interception and resting period interaction had no significant effect (p = 0.456 in 2018 and p = 0.6948 in 2019) on total soil seed bank densities (Figure 3). Resting period also had no significant effect on total soil seed bank densities in both years. However, rainfall interception had a significant effect on total soil seed bank density in both years. Total soil seed bank densities were higher at 60% rainfall interception in 2018, while they were higher at 15% rainfall interception in 2019 compared to 0% rainfall interception. In 2018, the total soil seed bank density was increased by 78% at 60% rainfall interception, while it increased by 27% at 15% rainfall interception in 2019 compared to 0% rainfall interception.
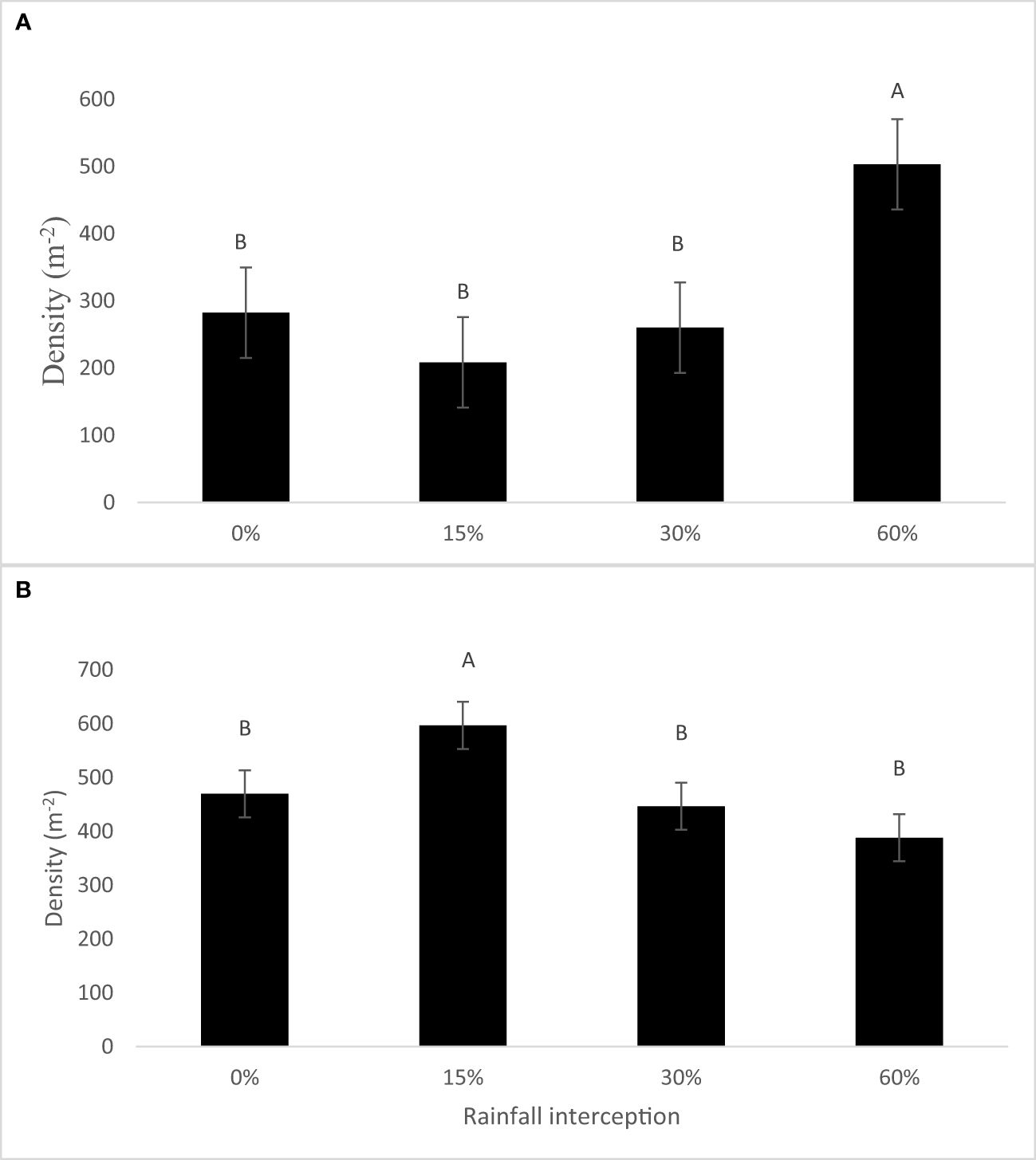
Figure 3 Total soil seed bank densities (m−2) as determined by rainfall interception in (A) 2018 and (B) 2019. Bars indicate standard error values, while different letters indicate values that are significantly different. .
4.7 Species richness as determined by rainfall interception in 2019
Rainfall interception and resting period had no significant interaction effect on soil seed bank species richness in 2019 (Figure 4). Moreover, resting period had no significant effect on soil seed bank species richness. On the other hand, rainfall interception had a significant effect on soil seed bank species richness. Rainfall interception resulted in reduced species richness as the 60% rainfall interception resulted in lower soil seed bank species richness compared to 15% rainfall interception, while there was no significant difference between 0%, 30%, and 60% rainfall interception.
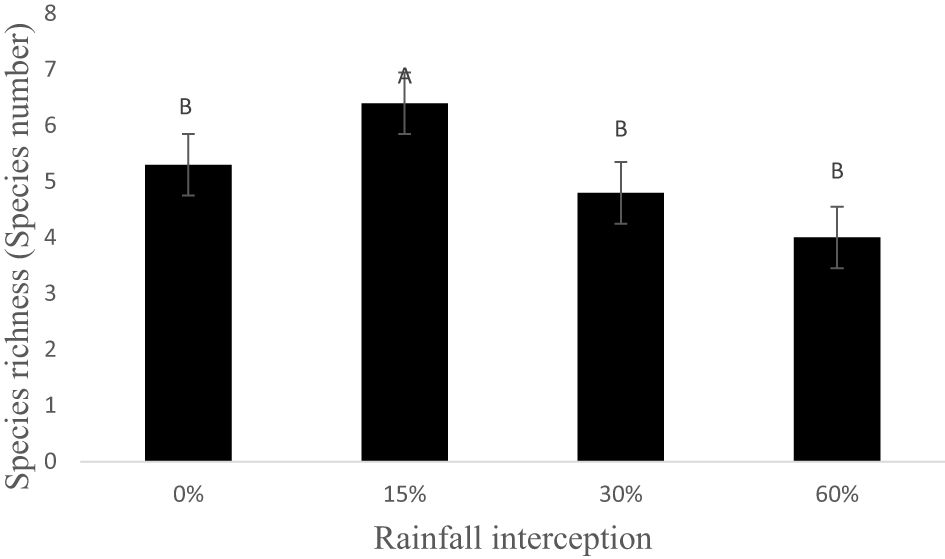
Figure 4 Mean soil seed bank species richness as determined by rainfall interception in 2019. Bars indicate standard error values, while different letters indicate values that are significantly different.
4.8 Species diversity as determined by rainfall interception in 2019
Rainfall interception and resting period interaction had no significant effect on species diversity (Figure 5). Moreover, resting period resulted in no significant effect on species diversity. However, rainfall interception had a significant effect on soil seed bank species diversity. The 30% rainfall interception resulted in a high species diversity compared to 0% rainfall interception.
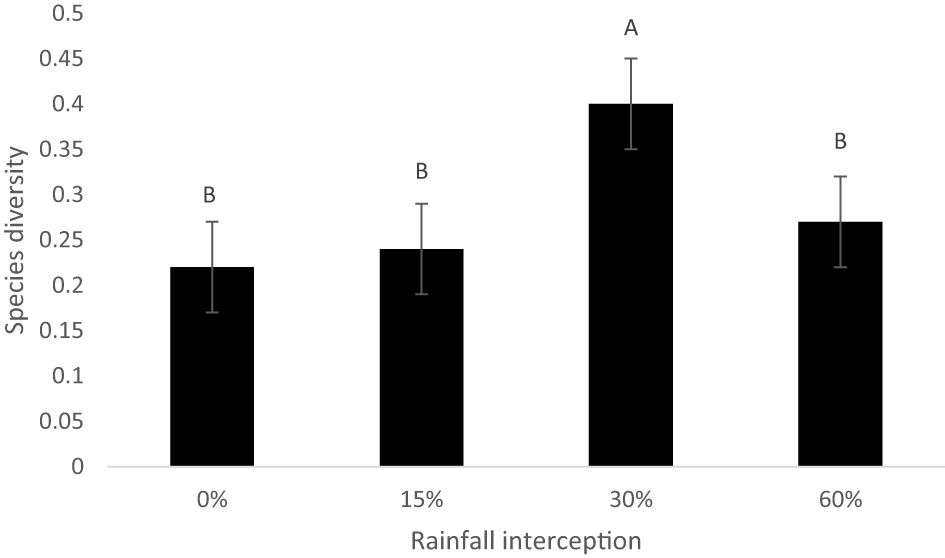
Figure 5 Mean soil seed bank species diversity as determined by rainfall interception in 2019. Bars indicate standard error values, while different letters indicate values that are significantly different.
5 Discussion
A total of 26 plant species, of which seven were grass species and 19 were forbs, were recorded in the soil seedbank in 2018, while there were 25 plant species in 2019 with six grass species, 18 forbs, and one sedge (Tables 1, 2), respectively. This means that grasses were mainly affected by rainfall interception than forbs. Emergence in terms of functional groups (grasses and forbs) differed from treatment to treatment, with 60% rainfall interception treatment having the lowest grass seed abundance in both years (Table 3),while forbs seed abundance was highest in both years (Table 4). On the other hand, 90-day resting period had higher grass abundance than 70-day resting period in 2019, while the resting period had no significant effect on grass soil seed bank abundance in 2018 (Table 3). This could be due to the fact that 70 days’ harvest took place before the grasses dropped their seeds, while 90 days’ harvest took place after they dropped their seeds. This resulted in 70-day resting period having less grass soil seed bank than 90-day resting period, while resting period had no significant effect on grass soil seed bank in 2018. These results concur with those reported by Beier et al. (2004) when it comes to grasses, as they reported that soil seed bank fell at drought treatments compared to control treatments due to the probability of indirect water deficit. The site has not been grazed for over 60 years, and the plots have been subjected to rainfall interception and cutting for 5 years. These treatments have had an impact on the above-mentioned vegetation, which has led to a decrease in grass productivity on high rainfall interception treatment (60%), while that of forbs increased with the increase in rainfall interception (Table 4). This is due to the fact that most forbs have a deep root system than grasses. These forbs dropped their seeds which, in turn, contributed largely to the soil seed bank. According to Akinola et al. (1998a, 1998b), rainfall manipulation scarcely modifies the abundance soil seed bank.
Water availability limits plant growth in the ecosystem (Larcher, 2000), difficulties in plant growth and survival could be exacerbated by drought (Llorens et al., 2004; Prieto et al., 2009), and ultimately seed production will be affected (del Cacho et al., 2012). However, our rainfall interception treatments did not completely exclude soil moisture but rather reduced its availability. As a result, seedlings were able to emerge after rainfall events, and it could affect the full plant reproductive cycle of producing seeds (del Cacho et al., 2012). The experiment may result in cumulative effects if continued over several years, resulting in a significant decrease in soil seed bank of grasses and more forbs at high rainfall interception compared to control treatments. This also concurs with the results that were reported by Akinola et al. (1998a), who argued that annual species, which are mostly forbs, respond faster to climate manipulation.
Many previous studies have reported large persistent seed banks on rangelands (Milberg and Hansson, 1993; Edwards and Crawley, 1999), where in most cases below-ground species composition differs from that aboveground (Snyman, 2004). In our study, our results showed lower densities of soil seed bank especially at the 0% and 15% rainfall interception treatments (Figure 3A). The high seedling density of forbs at 60% rainfall interception in both years correlated with high rainfall interception. This is also in agreement with what was reported by Snyman (2004) who reported a slightly larger soil seed bank production at the degraded rangeland. Few decreaser grass species such as D. eriantha and T. triandra were recorded at low rainfall interception than high rainfall interception treatments. The absolute soil seed bank composition can be important in determining the future condition of the rangeland and dictating which species will play part in successional processes (Kinucan and Smeins, 1992; Snyman, 2004). Rangeland degradation has a potential to reduce the soil seed bank and therefore change its species composition (O’Connor et al., 2001; Ingram, 2003); however, opposing trends have been observed (O’Connor and Bredenkamp, 1997; Snayman, 2004), and this is what has happened in our study as the total densities per square meter have increased at 60% rainfall interception in 2018 and at 15% rainfall interception in 2019.
In other studies where there were no grazers involved such as this one, it was found that perennial grasses increase, and this results in a rapid decline in species diversity (Sykora et al., 1990; Hill et al., 1992; Snyman, 2004). It is important to take different methods used in data collection when comparing soil seed bank studies such as this one, including depth, sample size, number of germination cycles, seasons, and whether there are grazing animals involved. Soil seed bank densities from our treatments (282.74, 208.60, 260.32, and 503.41 total seedlings per square meter for 0%, 15%, 30%, and 60% rainfall interception, respectively) and these values were lower than the previous reported results reported outside South Africa, but within the South African results. In a study that was conducted by Ruyle et al. (1988), they recorded 800 m−2 in southern Arizona and 2,252 to 4,409 seeds per square meter in Texas (Kinucan and Smeins, 1992). On the other hand, our results are within the South African reported results where Adams (1996) recorded 200 seeds per square meter in KwaZulu-Natal and O’Connor (1997) who recorded 350 seeds per square meter of Themeda triandra in the soil seed bank.
The 60% rainfall interception treatment had more bare patches than the rest of the treatment plots compared to other rainfall interception treatments. Seed predators such as ants also play a pivotal role in predation and seed dispersal; on the other hand, the vegetation determines the composition of ant communities and structure (Retana and Cerdá, 2000; Arnan et al., 2006). These ants were more abundant at the 60% than 0%, 15%, and 30% rainfall interceptions. This is in agreement with the results that were reported by Arnan et al. (2006) and Rodrigo and Retana (2006) who reported that ants were more abundant under low vegetation cover as well as Wolff and Debussche (1999) who reported that ants disappear with the increase in vegetation cover. According to del Cacho et al. (2012), ants’ activities may increase in plots with larger bare patches due to water deficit that hampers the regeneration of vegetation, leading to increased seed removal. However, it is also possible that the ants may also bring the seeds from outside the plots, but this would be minimal as there were no visual observations of high densities of ants outside the 60% rainfall interception treatments. However, these bare patches reduced the densities of grass seedlings and increased those of forbs. There might be reasons for these termites to prefer grass seeds over those of forb plants, e.g., size.
Basto et al. (2018) reported that grass seeds in calcareous grassland soils are not resistant to long-term summer drought. This may also justify why there were no major differences between the aboveground and the soil seedbank as the grass seeds might have died as the experiment has been in existence for more than 5 years. The decline in grass soil seed bank varies with soil water content and grass species, while other species increase germination as the soil moisture decreases (Gazanchian et al., 2006; Rohollahi et al., 2015). Reduced soil moisture also influences the availability of soil nutrients that are required by plants (Köhler et al., 2001)—for example, some species growing in dry grassland soils have smaller nitrogen uptake (Durand et al., 2010), and the nitrogen may accumulate in soil and have possible effects on soil seed bank (Basto et al., 2015a) and soil pH (Carroll et al., 2003), which may also result in seeds being persistent in grassland soils (Basto et al., 2015b). Even 1-year drought events may have long-term effects that are unpredictable on grasslands (Haddad et al., 2002).
6 Conclusion
Our results show that rainfall reduction has a negative effect on grass soil seed bank, while it improves the forbs soil seed bank. These results also show that rainfall reduction may eventually affect seed storage in the soil, which is the one that plays a pivotal role in grassland plant dynamics. The results from this study paint a picture of how the rangelands will look like during and after the events of reduced precipitation. The most palatable species such as T. triandra and D. eriantha were fewer in the soil seed bank from the highest rainfall interception. The reason for this could be that these grasses did not produce seeds at this treatment as it was visually observed during data collection. Future rainfall reductions may lead to grasslands being dominated by forbs over grasses with larger bare patches, which will lead to soil erosion and land degradation. These forbs are probably suppressed by the grasses at other rainfall interception treatments and take advantage as there are less grasses at 60% rainfall interception. According to Snyman (2004), most researchers recommend 80-mm depth when collecting soil seed bank; however, we collected up to 20 mm as the density of the seed bank declines with depth (Fenner and Thompson, 2005), with the majority of the seeds being found in the first 5 cm of the topsoil (Valbuena and Trabaud, 2001). However, we would encourage more studies of this nature to be conducted taking into consideration the effect of time lags between soil seed bank collections. This calls for alternative interventions that can be taken into consideration should the droughts be more persistent—for example, reseeding of rangelands with naturally occurring grasses in a specific area.
Data availability statement
The original contributions presented in the study are included in the article/supplementary material. Further inquiries can be directed to the corresponding author.
Ethics statement
The manuscript presents research on plants and does not require ethical approval for their study.
Author contributions
TM: Writing – original draft, Funding acquisition. AH: Supervision, Conceptualization, Project administration, Writing – review & editing. ET: Supervision, Writing – review & editing.
Funding
The author(s) declare financial support was received for the research, authorship, and/or publication of this article. The research was funded by the Agricultural Research Council Centre for Climate Change Collaboration (ARC-CCCC). The first author benefited from the PhD bursary that was available from National Research Foundation (NRF).
Acknowledgments
The authors would like to thank the National Research Foundation, University of Pretoria Adhoc Bursary and Agricultural Research Council Climate Change Collaboration for financial support. We further thank Mr. Mthunzi Mndela for his assistance on soil sampling and plant identification.
Conflict of interest
The authors declare that the research was conducted in the absence of any commercial or financial relationships that could be construed as a potential conflict of interest.
Publisher’s note
All claims expressed in this article are solely those of the authors and do not necessarily represent those of their affiliated organizations, or those of the publisher, the editors and the reviewers. Any product that may be evaluated in this article, or claim that may be made by its manufacturer, is not guaranteed or endorsed by the publisher.
References
Adams K. M. (1996). Influence of sward defoliation and soil disturbance on seedling emergence and survival in the Southern Tall Grassveld. Afr J. Range For Sci. 13, 131–136. doi: 10.1080/10220119.1996.9647909
Aikio S., Ranta E., Kaitala V., Lundberg P. (2002). Seed bank in annuals: competition between banker and non-banker morphs. J. Theor. Biol. 217, 314–349. doi: 10.1006/jtbi.2002.3034
Akinola M., Thompson K., Buckland S. M. (1998a). Soil seed bank of an upland calcareous grassland after 6 years of climate and management manipulations. J. Appl. Ecol. 35, 544–552. doi: 10.1046/j.1365-2664.1998.3540544.x
Akinola M., Thompson K., Hillier S. (1998b). Development of soil seed banks beneath synthesized meadow communities after seven years of climate manipulations. Seed Sci. Res. 8, 493–500. doi: 10.1017/S0960258500004463
Arnan X., Rodrigo A., Retana J. (2006). Post-fire recovery of Mediterranean ground communities follow vegetation and dryness gradients. J. Biogeogr 33, 1246–1258. doi: 10.1111/j.1365-2699.2006.01506.x
Báldi A., Batáry P., Kleijn D. (2013). Effects of grazing and biogeographic regions on grassland biodiversity in Hungary–analysing assemblages of 1200 species. Agr Ecosyst. Environ. 166, 28–34. doi: 10.1016/j.agee.2012.03.005
Baskin C. C., Baskin J. M. (1998). Seed-ecology, biogeography and evolution of dormancy and germination (London: Academic Press).
Basto S., Thompson K., Grime J. P., Fridley J. D., Calhim S., Askew A. P., et al. (2018). Severe effects of long term drought on calcareous grassland seed banks. Clim. Atmos. Sci. 1, 1. doi: 10.1038/s41612-017-0007-3
Basto S., Thompson K., Phoenix G., Sloan V., Leake J., Rees M. (2015a). Long-term nitrogen deposition depletes grassland seed banks. Nat. Commun. 6, 6185. doi: 10.1038/ncomms7185
Basto S., Thompson K., Rees M. (2015b). The effect of soil pH on persistence of seeds of grassland species in soil. Plant Ecol. 216, 1163–1175. doi: 10.1007/s11258-015-0499-z
Beier C., Emmett B., Gundersen P., Tietema A., Penuelas J., Estiarte M., et al. (2004). Novel approaches to study climate change effects on terrestrial ecosystems in the field: drought and passive nighttime warming. Ecosystems 7, 583–597. doi: 10.1007/s10021-004-0178-8
Carroll J. A., Caporn S. J., Johnson D., Morecroft M. D., Lee J. A. (2003). The interactions between plant growth, vegetation structure and soil processes in seminatural acidic and calcareous grasslands receiving long-term inputs of simulated pollutant nitrogen deposition. Environ. pollut. 121, 363–376. doi: 10.1016/S0269-7491(02)00241-5
del Cacho M., Saura-Mas S., Estiarte M., Josep Peñuelas’ J., Lloret F. (2012). Effect of experimentally induced climate change on the seed bank of a Mediterranean shrubland. J. Veg Sci. 23, 280–291. doi: 10.1111/j.1654-1103.2011.01345.x
Dreber N., Esler K. J. (2011). Spatio-temporal variation in soil seed banks under contrasting grazing regimes following low and high seasonal rainfall in arid Namibia. J. Arid Environ. 75, 174–184. doi: 10.1016/j.jaridenv.2010.09.007
Durand J. L., Gonzalez-Dugo V., Gastal F. (2010). How much do water deficits alter the nitrogen nutrition status of forage crops? Nutr. Cycl. Agroecosyst. 88, 231–243. doi: 10.1007/s10705-009-9330-3
Edwards G. R., Crawley M. J. (1999). Herbivores, seed banks and seedling recruitment in mesic grassland. J. Ecol. 87, 423–435. doi: 10.1046/j.1365-2745.1999.00363.x
Fenner M., Thompson K. (2005). The ecology of seeds (Cambridge, MA, US: Cambridge University Press). doi: 10.1017/CBO9780511614101
Fridley J. D., Grime J. P., Askew A. P., Moser B., Stevens C. J. (2011). Soil heterogeneity buffers community response to climate change in species-rich grassland. Global Change Biol. 17, 2002–2011. doi: 10.1111/j.1365-2486.2010.02347.x
Gazanchian A., Sima K. K., Nayer A., Malboobi M. A., Heravan E. M. (2006). Relationships between emergence and soil water content for perennial cool-season grasses native to Iran. Crop Sci. 46, 544–553. doi: 10.2135/cropsci2005.04-0357
Gutterman Y. (2012). ‘Seed Germination in Desert Plants.’ (Berlin: Springer Science & Business Media).
Haddad N. M., Tilman D., Knops J. M. (2002). Long-term oscillations in grassland productivity induced by drought. Ecol. Lett. 5, 110–120. doi: 10.1046/j.1461-0248.2002.00293.x
Henig-Server N., Eshel A., Ne’eman G. (1996). pH and osmotic potential of pine ash as post-fire germination inhibitors. Physiol. Plantarum 96, 71–76.
Hill M. O., Evans D. F., Bell S. A. (1992). Long-term effects of excluding sheep from hill pastures in Wales. J. Ecol. 80, 1–14. doi: 10.2307/2261058
Hu A., Zhang J., Chen X. J., Millner J. P., Chang S. H., Bowatte S., et al. (2019). The composition, richness, and evenness of seedlings from the soil seed bank of a semi-arid steppe in northern China are affected by long-term stocking rates of sheep and rainfall variation. Rangel. J. 41, 23–32. doi: 10.1071/RJ18025
Hufkens K., Keenan T. F., Flanagan L. B., Scott R. L., Bernacchi C. J., Joo E., et al. (2016). Productivity of North American grasslands is increased under future climate scenarios despite rising aridity. Nat. Clim Change 6, 710–714. doi: 10.1038/nclimate2942
Ingram L. J. (2003). Growth, Nutrient Cycling and Grazing of Three Perennial Tussock Grasses of the Pilbara Region of NW AUSTRALIA. Department of Botany, University of Western Australia. 279pp.
Jury M. R. (2013). Climate trends in southern Africa. S Afr J. Sci. 109, 1–11. doi: 10.1590/sajs.2013/980E
Kalamees R., Püssa K., Zobel K., Zobel M. (2012). Restoration potential of the persistent soil seed bank in successional calcareous (alvar) grasslands in Estonia. Appl. Veg Sci. 15, 208–218. doi: 10.1111/j.1654-109X.2011.01169.x
Kassahun A., Snyman H. A., Smit G. N. (2009). Soil seed bank evaluation along a degradation gradient in arid rangelands of the Somali region, eastern Ethiopia. Agr Ecosyst. Environ. 129, 428–436. doi: 10.1016/j.agee.2008.10.016
Kinucan R. J., Smeins F. E. (1992). Soil seed bank of a semi-arid Texas Grassland under three long-standing (36-years) grazing regimes. Am. Midl. Nat. 128, 11–21. doi: 10.2307/2426408
Köhler B., Ryser P., Güsewell S., Gigon A. (2001). Nutrient availability and limitation in traditionally mown and in abandoned limestone grasslands: a bioassay experiment. Plant Soil 230, 323–332.
Larcher W. (2000). Temperature stress and survival ability of Mediterranean screlophyllous plants. Plant Biosyst. 134, 279–295.
Leck M. A., Parker V. T., Simpson R. L. (1989). Ecology of soil seed banks (San Diego, CA, US: Academic Press).
Llorens L., Peñuelas J., Estiarte M., Bruna P. (2004). Contrasting growth changes in two dominant species of a mediterranean shrubland submitted to experimental drought and warming. Ann. Bot-London 94, 843–853. doi: 10.1093/aob/mch211
Margalef R. (1958). “Temporal succession and spatial heterogeneity in phytoplankton,” in Perspectives in Marine biology. Ed. Buzzati-Traverso (Univ. Calif. Press, Berkeley), 323–347.
Milberg P., Hansson M. L. (1993). Soil seed bank and species turnover in a limestone grassland. J. Veg. Sci. 4, 35–42. doi: 10.2307/3235635
Mucina L., Rutherford M. C. (2006). The vegetation of South Africa, Lesotho and Swaziland. Strelitzia 19 (Pretoria, South Africa: South African National Biodiversity Institute).
O’Connor T. G. (1997). Microsite influence on seed longevity and seedling emergence of a bunch-grass (Themeda triandra) in a semi-arid savanna. Afr J. Range For Sci. 14, 7–11.
O’Connor T. G., Bredenkamp G. J. (1997). “Grassland,” in Vegetation of South Africa. Eds. Cowling R. M., Richardson D. M., Pierce S. M. (Cambridge, United Kingdom: Cambridge University Press).
O’Connor T. G., Haines L. M., Snyman H. A. (2001). Influence of precipitation and species composition on phytomass of a semi-arid African grassland. J. Ecol. 89, 850–860.
Prieto P., Peñuelas J., Llusiá J., Asensio D., Estiarte M. (2009). Effects of experimental warming and drought on biomass accumulation in a Mediterranean shrubland. Plant Ecol. 205, 179–191. doi: 10.1007/s11258-009-9608-1
Retana J., Cerdá X. (2000). Patterns of diversity and composition of Mediterranean ground ant communities tracking spatial and temporal variability in the thermal environment. Oecologia 123, 436–444. doi: 10.1007/s004420051031
Rodrigo A., Retana J. (2006). Post-fire recovery of ant communities in sub-Mediterranean Pinus nigra forests. Ecography 29, 231–239. doi: 10.1111/j.2006.0906-7590.04272.x
Rohollahi I., Khoshkholghsima N. A., Yamada T., Kafi M., Hoshino Y., Liaghat A., et al. (2015). Evaluation of seedling emergence and relative DNA content under dry soil conditions of wild Festuca arundinacea populations collected in Iran. Grassl Sci. 61, 6–14. doi: 10.1111/grs.12074
Ruyle G. B., Roundy B. A., Cox J. R. (1988). Effects of burning on germinability of Lehmann lovegrass. J. Range Manage 41, 404–406. doi: 10.2307/3899577
Sagar G. R., Mortimer A. M. (1976). An approach to the study of the population dynamics of plants. Appl. Biol. 1, 1–49.
SAS (Statistical Analysis System). (2008). User’s guide; SAS/STAT ®9.2 (Cary, NC, United States: SAS Institute Inc.).
Snyman H. A. (2004). Soil seed bank evaluation and seedling establishment along a degradation gradient in a semi-arid rangeland. Afr. J. Range Forage Sci. 21, 37–47. doi: 10.2989/10220110409485832
Snyman H. A. (2005). The effect of fire on the soil seed bank of a semiarid grassland in South Africa. Afr J. Range For Sci. 71, 53–60.
Soil Classification Working Group. (1991). Soil classification. A taxonomic system for South Africa (Pretoria: Soil Classification Working Group, Department of Agricultural Development).
Solomon T. B., Snyman H. A., Smit G. N. (2006). Soil seed bank characteristics in relation to land use systems and distance from water in a semi-arid rangeland of southern Ethiopia. S Afr J. Bot. 72, 263–271. doi: 10.1016/j.sajb.2005.09.003
Sykora K. V., van der Krogt G., Rademakers J. (1990). Vegetation change in the south-western part of the Netherlands under the influence of different management practices. Biol. Conserv. 52, 49–81.
Talore D. G. (2015). Quantintative and qualitative herbage yield, and carbon sequestration in subtropical grasslands subjected to different precipitation, grazing management and land use type.
Thompson K. (2000). “The functional ecology of soil seed banks,” in ‘Seeds: the Ecology of Regeneration in Plant Communities’, 2nd edn. Ed. Fenner M. (CABI Publishing, Wallingford, UK), 215–235.
Trabaud L., Renard P. (1999). Do light and litter influence the recruitment of Cistus spp. stands? Israel J. Plant Sci. 47, 1–9. doi: 10.1080/07929978.1999.10676745
Valbuena L., Trabaud L. (2001). Contribution of the soil seed bank to post-fire recovery of a heathland. Plant Ecol. 152, 175–183. doi: 10.1023/A:1011419721307
van Oudtshoorn F. (2012). Guide to grasses of southern Africa (Pretoria, South Africa: Briza publications), ISBN: 9781920217358.
Wolff A., Debussche M. (1999). Ants as seed dispersers in a Mediterranean old-field succession. Oikos 84, 443–452. doi: 10.2307/3546423
Yadav S. K., Mishra G. C. (2013). Biodiversity measurement determines stability of ecosystems. IJESDM 4, 2231–1289.
Keywords: climate change, densities, ecosystems, grass, forbs
Citation: Magandana TP, Hassen A and Tesfamariam E (2024) Effect of rainfall interception and resting period on the soil seed bank. Front. Agron. 6:1342138. doi: 10.3389/fagro.2024.1342138
Received: 21 November 2023; Accepted: 18 April 2024;
Published: 03 July 2024.
Edited by:
Victor Rolo, University of Extremadura, SpainReviewed by:
Dagnija Lazdiņa, Latvian State Forest Research Institute Silava (LSFRI), LatviaLuciana Ghermandi, National Scientific and Technical Research Council (CONICET), Argentina
Copyright © 2024 Magandana, Hassen and Tesfamariam. This is an open-access article distributed under the terms of the Creative Commons Attribution License (CC BY). The use, distribution or reproduction in other forums is permitted, provided the original author(s) and the copyright owner(s) are credited and that the original publication in this journal is cited, in accordance with accepted academic practice. No use, distribution or reproduction is permitted which does not comply with these terms.
*Correspondence: Thabo Patrick Magandana, dG1hZ2FuZGFuYUBnbWFpbC5jb20=