- Crop Health, Faculty of Agricultural and Environmental Sciences, University of Rostock, Rostock, Germany
Synthetic herbicides are used for perennial weed management, but owing to environmental and health concerns they face increasing regulatory restrictions. Consequently, there is growing interest in ecologically friendly alternatives including bio-herbicides based on natural compounds such as the active ingredient pelargonic acid (PA). PA acts as a broad-spectrum non-selective contact herbicide. However, when used as a contact herbicide, regrowth of the aboveground parts of plants still presents a challenge. The aim of this study was to investigate the control effect of a two-year application of PA on perennial weeds. The study was conducted between spring 2020 and autumn 2021 as a semi-field experiment. The factors were two levels of weed species (Cirsium arvense and Sonchus arvensis), three levels of herbicide treatment (untreated control, PA, and glyphosate), and three levels of initial ramet size (5, 10, and 15 cm). The results showed that a two-year application of PA increased its efficacy on C. arvense and S. arvensis when combined with the smaller initial ramet size (5 cm), but did not prevent regrowth in either species. PA efficacy was greater on C. arvense than on S. arvensis. The plant coverage decreased by 24 % when the initial ramet size was 5 cm for C. arvense, while for S. arvensis with the same initial ramet size it was reduced by just 4 %. For PA-treated C. arvense with an initial ramet size of 5 cm, aboveground biomass and belowground biomass were reduced by 43 % and 22 % respectively. In S. arvensis, the reductions in aboveground and belowground biomass for an initial ramet sizes of 5 cm were 13 % and 12 % respectively. In general, PA efficacy was not as high as glyphosate efficacy for both species. In conclusion, the results revealed that after PA application the regrowth of shoots from the creeping roots in C. arvensis and S. arvensis decreased when the initial ramet size was 5 cm. This reduction suggests that PA efficacy on these plants increases when it is applied repeatedly on the same patches with smaller initial root fragments.
1 Introduction
As perennial weeds, Cirsium arvense (L.) Scop. and Sonchus arvensis L. represent significant threats to agricultural productivity due to their ability to persist and spread over time and compete with crops for resources (Ramesh et al., 2017). C. arvense (creeping thistle) and S. arvensis (sow thistle) are found in various crops and are considered highly problematic in temperate regions (Liew et al., 2013; Tørresen and Gerowitt, 2022; Andert et al., 2023). Both weeds form patches and mainly have rapid vegetative reproduction through their subterranean reproductive organs (horizontal creeping roots) and abundant seed production (Tørresen and Gerowitt, 2022). Their allelopathic effects on some crops, especially the prevention of seed germination or seedling growth have been reported in previous studies (Szabó and Halbritter, 2015; Bashir et al., 2018; Egushova and Anokhina, 2022).
There are various control methods for these weeds, including chemical, mechanical, and cultural methods (Melander et al., 2012). The common chemical control method for perennial weeds is the use of systemic herbicides, e.g. the non-selective active ingredient glyphosate in the intercropping period (Beckie et al., 2020). Furthermore, certain systemic herbicides are registered for application during the cropping period, e.g., the active ingredient metsulfuron-methyl can be used in cereals, effectively controlling a broad spectrum of weeds including C. arvense (Bhullar et al., 2013; Zargar et al., 2019). For decades, synthetic herbicides have been crucial for perennial weed control owing to their practical and financial advantages (Loddo et al., 2023). However, for herbicides such as glyphosate, stricter regulations concerning their registration and usage have been implemented in numerous countries due to concerns about herbicide resistance and their adverse effects on the environment and human health (Antier et al., 2020; Beckie et al., 2020). The ‘Farm to Fork’ strategy is one of the key components of the European Green Deal that reflects the growing interest in sustainable and ecologically friendly weed control solutions, including synthetic herbicide substitutions (European Commission, 2020; Radicetti and Mancinelli, 2021). Alternative approaches include mechanical methods, cultural tools, and alternative herbicides, which are non-chemical, natural, or less toxic (Synowiec et al., 2017; Ibáñez and Blázquez, 2018; Beckie et al., 2020).
Bio-herbicides are products of natural origin for weed control that are either microorganisms or products derived from living organisms, including the natural metabolites produced by these organisms (Cordeau et al., 2016). The herbicidal effects of natural substances, such as plant essential oils and organic acids, have been the subject of many studies in recent years. (Barton et al., 2014; Synowiec et al., 2017; Casella et al., 2023; Kouki et al., 2023). Among tested natural active ingredients, pelargonic acid (PA) is the sole ingredient available on the market (Loddo et al., 2023). PA is a naturally occurring fatty acid found in foods such as vegetables and fruits and has been approved as a safe food agent in numerous countries ( Ciriminna et al., 2019). PA degrades quickly in the environment and does not cause long-term runoff contamination in rainy seasons (EFSA (European Food Safety Authority), 2021). Bio-herbicides that contain the active ingredient PA are known as burndown herbicides and are increasingly used for weed control, e.g. on gardens, lawns, and walkways (Ciriminna et al., 2019).
In light of PA’s potential as a bio-herbicide and its ability to contribute to the reduction of synthetic herbicides, researchers have been exploring its efficacy, and have shown that PA is the most successful bio-herbicide available (Webber et al., 2014a; Carroll et al., 2022; Muñoz et al., 2022; Pannacci et al., 2022). However, its effectiveness varies between weed species (Webber et al., 2014a; Webber et al., 2014b). Monocotyledon weeds such as Alopecurus myosuroides Huds. and Lolium rigidum Gaud are less sensitive to PA, and may display reduced and transient symptoms at higher doses (Travlos et al., 2020; Loddo et al., 2023). Dicotyledon weeds also exhibit considerable differences in sensitivity to PA (Webber et al., 2014a; Webber et al., 2014b; Loddo et al., 2023). Furthermore, there is evidence of regrowth after the application of PA for most weeds (Ciriminna et al., 2019; Muñoz et al., 2020; Travlos et al., 2020; Muñoz et al., 2022; Loddo et al., 2023). Due to the occurrence of regrowth, previous studies have suggested sequential applications of PA with short intervals within a growing season (Barker and Prostak, 2009; Webber et al., 2014a; Webber et al., 2014b). Earlier investigations mainly concentrated on annual weeds, particularly examining the impact of PA with a focus on the aboveground parts of plants (Webber et al., 2014a; Webber et al., 2014b; Ciriminna et al., 2019; Muñoz et al., 2020; Travlos et al., 2020; Muñoz et al., 2022; Pannacci et al., 2022; Loddo et al., 2023).
The presence of creeping roots in C. arvense and S. arvensis negatively affects the success of control methods (Liew et al., 2013). Adventitious buds are formed on the horizontal creeping roots, from which new shoots can emerge (Brandsæter et al., 2010; Liew et al., 2013). The activities of adventitious buds and the emergence of shoots differ from species to species (Brandsæter et al., 2010). Furthermore, the ability of creeping roots to sprout varies significantly during the season (Brandsæter et al., 2010; Liew et al., 2013). For S. arvensis, the sprouting capacity appears to decrease in late summer to early autumn (Håkansson, 1969; Håkansson and Wallgren, 1972; Brandsæter et al., 2010), while sprouting for C. arvense does not decrease as long as environmental conditions allow it (Brandsæter et al., 2010). The root fragment of the creeping perennial is called a ramet, which is genetically identical to the mother plant (Tørresen and Gerowitt, 2022), and it is often induced by mechanical soil disturbance (Håkansson, 2003). Large ramets can rapidly produce new C. arvense plants, while smaller ramets often do not survive to produce vegetative offspring due to their low carbohydrate reserves (Hamdoun, 1972). Similar to C. arvense, the emergence and number of sprouts in S. arvensis depend on the dry matter content of the roots (Lemna and Messersmith, 1990; Vanhala et al., 2006). When the creeping roots are fragmented by tillage, the resulting smaller ramets are less viable and have less dry matter, making them more likely to die (Vanhala et al., 2006). Furthermore, there is a phenological stage at which belowground biomass reaches its minimum dry weight before it begins to increase again (Tavaziva, 2012). In both species, this stage occurs when they have between four and seven leaves (Håkansson, 2003). Depleting the belowground carbohydrate reserves through fragmentation of the regenerative structures and applying treatments to the lowest belowground biomass have been suggested by previous research as offering better control (Håkansson, 1969; Gustavsson, 1997; Brandsæter et al., 2010). To achieve successful control, it is essential to apply control methods to these perennial weeds when they are most sensitive to disturbance (Verwijst et al., 2018).
An earlier study investigated the effects of plant growth stage, application volume and the addition of adjuvant on PA efficacy to control C. arvense. The results demonstrated that PA efficacy is greater when applied on a 4-8 leaf-stage plant using an increased application volume, and also by adding an adjuvant (Ganji et al., 2022). The present study investigated the feasibility of repeated PA application over two consecutive years on the same spot (patch of C. arvense and S. arvensis) considering their initial ramet size. Its aim was to determine the PA herbicidal impact on the entire life cycle of the perennial weed species and their regrowth patterns that might not be identified in shorter-term studies. Moreover, the efficacy of PA as a potential herbicidal treatment was compared with that of the commonly used active ingredient glyphosate (GLY). This design facilitated a comprehensive comparison of the effects of PA treatment and two reference conditions: the untreated control (UC), representing the baseline or natural state, and GLY treatment, which serves as a standard for effective perennial weed control (Hudek et al., 2021; Kanatas et al., 2021).
The present study focused on perennial weeds with creeping roots and examined the influence of initial ramet size on PA efficacy after two applications within two consecutive years on the same spot (patch of C. arvense and S. arvensis), with the aim of improving knowledge about the control of perennial weeds with creeping roots using PA. Considering the regenerative capacity of the creeping roots, the objectives of this study were to determine (i) whether the initial ramet size influences PA efficacy and regrowth of C. arvense and S. arvensis after PA application and (ii) whether there are any differences between these perennial weeds in terms of PA efficacy and regrowth patterns after repeated application.
It was hypothesized that repeated application of PA over two consecutive years reduces growth parameters, aboveground and belowground biomass, and flower numbers in both species. It was expected that S. arvensis would be more susceptible to PA than C. arvense due to seasonal variations that impact the sprouting abilities of S. arvensis by demonstraiting reduced capacity in late summer to early autumn (Håkansson, 1969; Håkansson and Wallgren, 1972; Brandsæter et al., 2010). For this reason, it was assumed that regrowth after herbicide application would differ between these species. Finally, it was also assumed that smaller C. arvense and S. arvensis ramets have a lower regenerative capacity, which reduces regrowth and increases PA efficacy.
2 Materials and methods
A two-year pot experiment (from spring 2020 to autumn 2021) under semi-field conditions was conducted in the experimental field of Rostock University in northeast Germany (location Rostock: 54° 4' 6.726'' N, 12° 4' 54.0876'' E).
Figure 1 provides a concise overview of the experimental workflow used in this semi-field experiment. The process included (1) the preparation of the semi-field experiment by planting ramets in pots, (2) the application of the herbicide treatments in each experimental year, and (3) visual assessments to measure the efficacy of the herbicides compared with the untreated control.
The experiment was a factorial, completely randomized block design with four replications. The factors were plant species with two levels, treatments with three levels, and ramets with three levels of initial size: 5, 10, and 15 cm (Table 1).
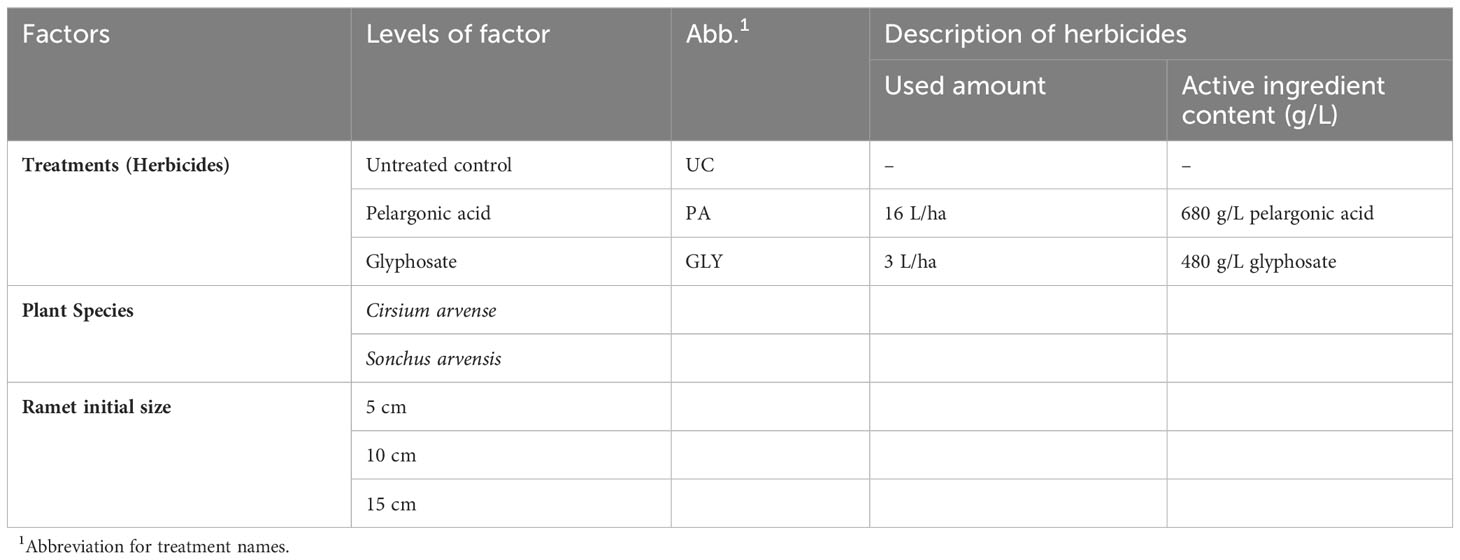
Table 1 Overview of factorial experiment design with plant species, herbicide treatments, and initial ramet size as factors.
For this research, Beloukha® (680 g/L pelargonic acid) and Roundup Powerflex® (480 g/L glyphosate and 393.6 g/L acid equivalent) were used as the PA and GLY treatments, respectively.
2.1 Ramet planting in pots
In December 2019, creeping roots of C. arvense were collected from the University of Rostock’s experimental field and those of S. arvensis from a field near Güstrow (53°46’14.6"N, 12°09’59.3"E). The collected roots were stored by propagation in pots in the greenhouse. In February 2020, the pots were buried in the soil and filled with a mixture of ½ field soil, ¼ potting soil, and ¼ compost. The pot size was 200 L with a diameter of 80 cm (pot surface area 0.503 m2). The distance between pots was one meter. The creeping roots obtained from propagated greenhouse pots (cleaned and washed to eliminate the remaining soil) were used for the semi-field experiment. Roots that were more than 3 mm in diameter were fragmented into ramets 5, 10, and 15 cm in length, and weighed. The term ramet is used in the text instead of root fragment because the root fragments used in this experiment were derived from one progenitor of each plant species, and shared the same genotypes as the parent plants (Tørresen and Gerowitt, 2022). Finally, one single ramet was planted directly at a 5 cm soil depth in each pot. The pots were irrigated immediately after the ramets were planted and again when there was a need for irrigation on warm days during the growing seasons in both experimental years. After the establishment of the pots in the soil and until the end of the experiment in the second year, other weeds were removed from the pots by hand. Before herbicide application in both experimental years, fertilization was undertaken to achieve nutrient conditions comparable with spring cereal fields. Hakaphos Blau® as an NPK fertilizer (15 % N, 10 % P, and 15 % K) was applied to the soil at the amount of 16.65 g per pot as a balanced nutrient solution. These rates correspond to 50 kg of nitrogen per hectare, which is less fertilization than regular spring cereal fields as there was no crop in the pots to compete for the nutrients.
2.2 Application of herbicide treatments
At the end of May 2020, herbicide treatments were applied to the S. arvensis pots. Owing to the late emergence of C. arvense sprouts, the herbicide application for this species was performed two weeks later. The plant growth stage at the time of herbicide applications for both plant species was the four-to-eight-leaf-stage considering the compensation point according to Håkansson (2003) and BBCH 14-18 according to Meier (2018), and each pot had one or two plants (shoots from the same ramet).
At the beginning of May 2021, the herbicide treatments were applied again. Each pot was treated with the same herbicide treatment as the previous year. The plant growth stage at herbicide application time was four-to-eight-leaf, but each pot had many shoots emerged from the same ramet which was recorded. In both years, a plot-spraying device with a pressure of 2.1 bar and a speed of 4 kilometers per hour was utilized for the herbicide applications. The application volume for the treatments was 200 L/ha. The operated flat jet nozzle was size 02.
2.3 Assessments
The herbicide treatments were assessed by visually estimating the percentage of necrotization. The assessments were conducted at 1, 7, 14, and 21 days after treatment (DAT) of the herbicides. A value of 0 % necrotization was equivalent to completely vital vegetation, while a value of 100 % represented completely dead vegetation. The level of necrotization was interpreted as herbicide efficacy. The pots were evaluated for regrowth from the ramets starting 28 days after herbicide treatment (28th_DAT). To be able to monitor the regrowth pattern and effect of the treatments over the long-term experimental period and identify the possible variability in regrowth pattern, data on plant height, shoot density per m2, and BBCH stage (Meier, 2018) were collected monthly until the end of the growing season. The monthly evaluations were performed from July to October 2020 and from April to September 2021. To obtain information on how the weed species population was established and regrew after herbicide application during the growing season, in August of each year before the withering of the plants, the shoot density per m2, the percentage coverage of the soil surface by the plant, and the number of flowers were assessed. All emerged shoots were counted and then shoot density per m2 was calculated according to a pot surface area of 0.503 m2. Counting of the flowers indicates the reproductive capacity of the weed species, and helps to understand the potential for seed production after application of the treatments. By undertaking this assessment in August, the weed’s reproductive success was estimated, before it completed its life cycle. Aboveground and belowground biomass was measured per pot using the destructive method at the end of the experiment in 2021, which determined the effectiveness of the herbicide treatment at controlling weed growth. For this, the collected plant materials were placed in an oven at 60 °C for at least 24 hours, and the dried biomass was then measured. As mentioned in the experimental setup, ramets were weighed before being planted in the pots, and this was used as a covariate in the statistical analysis. Figure 1 gives an overview of the above-mentioned experimental setup and assessments.
2.4 Weather conditions
Figure 2 shows the weather conditions for the entire experimental period. The average air temperature for the growing season (March to September) was 13.2 °C in 2020 and 13.1 °C in 2021. In the 2020 growing season, the minimum average soil temperatures were 5.9 °C and 5.6 °C respectively at 5 cm and 20 cm belowground level in March. In August, the maximum average temperatures at 5 cm and 20 cm belowground level were 19.6 °C and 18.8 °C respectively. In 2021, the minimum soil temperature at these belowground levels averaged 2.3 °C and 2.1 °C, respectively, and the maximum was 20.7 °C in 5 cm and 19.8 °C in 20 cm soil depth in July. The total amount of precipitation in 2020 was 428 mm. Of this amount, 321 mm occurred in the growing season. The precipitation amount from January until the end of September 2021 was 500 mm, of which 376 mm occured in the growing season (Figure 2).
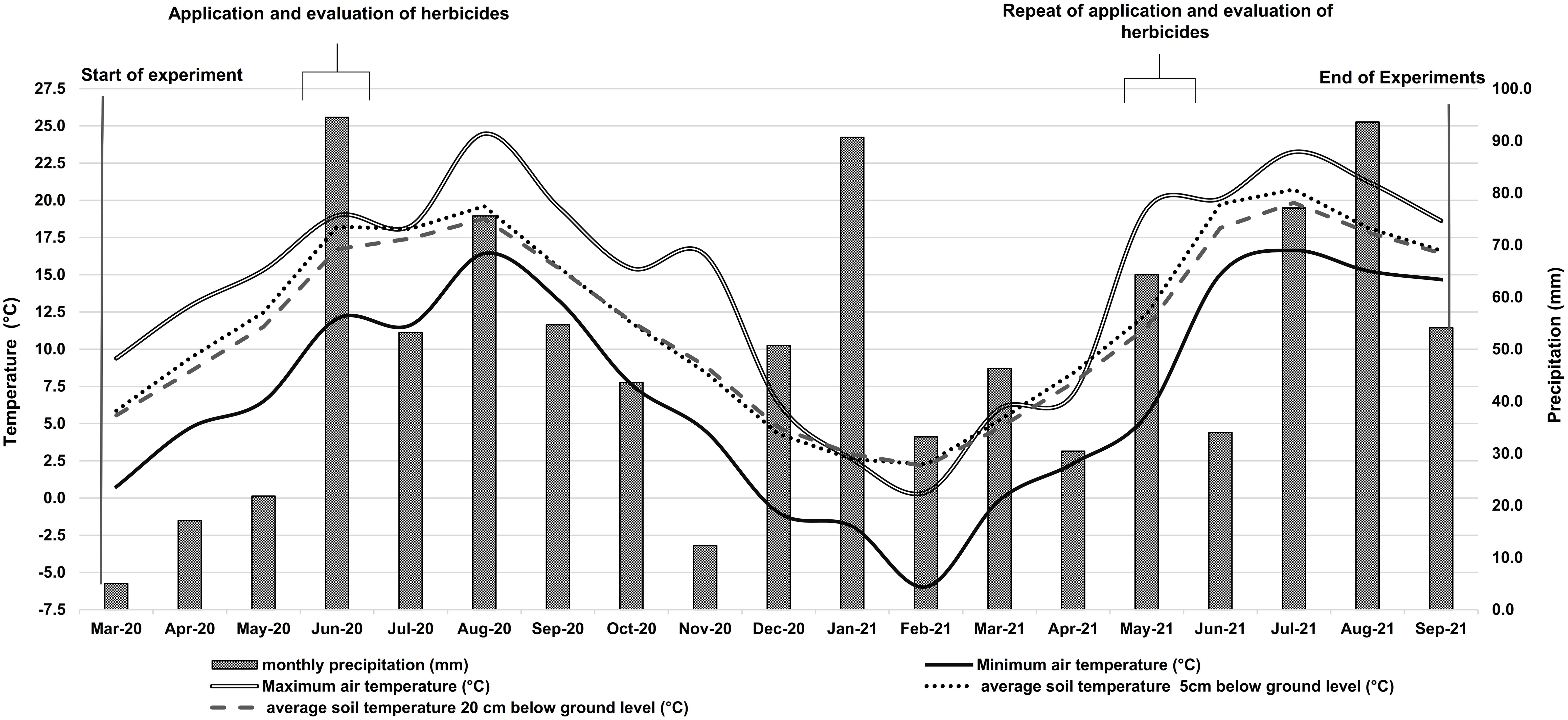
Figure 2 Air temperature (°C), soil temperatures at 5 cm and 20 cm below-ground level, and precipitation (mm) during the experimental period in 2020 and 2021. Information for both years was obtained from the research weather station of the University of Rostock’s Department of Hydrology and Applied Meteorology.
2.5 Statistical analysis
Data on herbicide efficacy (necrotization percentage) were analyzed using linear mixed model analysis (LMM), with weed species, herbicide treatments, initial ramet size, DATs and their interaction as fixed effects, and replicates as random effects. In this analysis, the following model (Equation 1) was fitted for two consecutive years, 2020 and 2021. For each year, the same model structure with shared fixed effects was used, but allowed for separate random intercepts (bm) to capture year-specific variability:
where μ is the overall mean; Si, Hj, RSk and Dl represent the fixed effects for “weed species”, “herbicide treatment”, “initial ramet size”, and “DATs” respectively; bm represents the random intercepts for the grouping variable “block”, and ϵijkl is the error term that accounts for unexplained variability in the model.
The long-term effectiveness of PA was revealed through a separate analysis at 21st_DAT for each experimental year, providing information to understand the sustained impact of weed control over the course of the experiment. The same model structure (Equation 2) with shared fixed effects was utilized to analyze the herbicide efficacy in the final assessment at 21st_DAT as follows:
where μ is the overall mean; Si, Hj and RSk are the fixed effects for “weed species”, “herbicide treatment”, “initial ramet size”, and “DATs” respectively; bl represents the random intercepts for the grouping variable “block”, and ϵijkl is the error term.
As the experimental years were not independent of each other, in order to examine the effect of factors on the measured variables after regrowth, which were shoot density per m2, the percentage coverage of the soil surface by the plant, and the number of flowers, the data from the measurements at the end of the experiment were utilized for statistical analysis using LMM (Equations 3–7). Three factors (weed species, herbicide treatment, and initial ramet size) and their interaction were fixed effects in this analysis. The random effects were replicates (blocks) and the initial weight of ramets (as covariates). It was assumed that the association between the initial weight of ramets and measured variables depended on the magnitude of weight, thus the heterogeneity of weight was modeled as a random effect in the data analysis of this research. The model equations are as follows:
where µ is the overall mean; Si is the effect of species; Hj is the effect of herbicide treatment; RSij is the effect of ramet size; bk represents the random intercept for block and initial weight of ramets; ϵijk is the random error term.
For the analysis of the monthly evaluation of plant height, in addition to the fixed effects of weed species, herbicide treatments, initial ramet size, and their interactions, the fixed effect of the experimental year and its interaction with the fixed effects of these three factors were included in the model (Equation 8). To account for variability within different levels of block and month, random intercepts were included. This allowed the model to capture random variations in plant height within these nested grouping variables. In addition, the model assumed a first-order autoregressive correlation structure (AR1) within each combination of block and month. This choice of correlation structure accounted for potential temporal autocorrelation in plant height measurements within the same block and month combinations. The model equation is as follows:
where µ is the overall mean; Si is the effect of species; Hj is the effect of herbicide treatment; RSk is the effect of ramet size; Yl is the effect of year; bm represents the random intercept for block and month in which the subscript m represents different levels of the month nested within block, and εijkl is the random error term.
All the models were fitted using the restricted maximum likelihood (REML) method. To evaluate the goodness of fit of the linear mixed models (LMMs), the variance decomposition method was employed to calculate conditional R-squared values for all models. The variance decomposition method was chosen because it has the ability to dissect the total variance in the response variable into components related to fixed effects, random effects, and residual error. The same method was used to calculate marginal R-squared values for all models except the model for plant height. The log-likelihood method was utilized due to the unique characteristics of this particular model, which required a different approach for evaluating goodness of fit. The log-likelihood method was more appropriate in this specific case, as it was able to capture the subtle details of the data better, resulting in a more precise evaluation of the model’s performance.
For all the data, pairwise comparisons were conducted using Tukey’s HSD tests on the results of LMMs to identify significant differences between treatments by including all the interactions of fixed effects. R version 4.3.0 (R Core Team, 2023) was used to conduct all statistical analyses, and the packages “nlme” (Pinheiro et al., 2023), “lme4” (Bates et al., 2015), “lmerTest” (Kuznetsova et al., 2017), and “emmeans” (Lenth et al., 2018) were used for the LMMs and pairwise comparisons.
3 Results
3.1 Herbicide efficacy
The results of herbicide efficacy at all DATs showed that the main effect of herbicide treatment on herbicide efficacy was statistically significant, which means that the relationship between herbicide treatment and herbicide efficacy varied at different DATs. The results did not express a significant difference between initial ramet sizes or between the weed species C. arvense and S. arvensis (Supplementary Table 1). However, ramet sizes and species will be presented separately in the text due to biological concerns.
Herbicide efficacy across multiple assessment days in both years showed that the PA treatment had highest efficacy on both weed species at 1st_DAT in 2020, which decreased over the experimental period. PA treatment efficacy for both species was significantly higher than the GLY treatment and UC for all ramet sizes at 1st and 7th_DAT in 2020. The PA treatment showed a statistically significantly higher efficacy on the 5 cm ramet size in C. arvense compared with all UC treatments until 2lst_DAT in 2020 (Supplementary Table 1). The PA treatment efficacy between various ramet sizes was not significant. For both weed species in 2020, the differences between 1st_DAT and 14th/21st_DAT were significant for PA treatment efficacy on all ramet sizes. PA treatment efficacy at 7th_DAT was significantly different from 21st_DAT for C. arvense ramet sizes 10 cm and 15 cm and for all ramet sizes of S. arvensis (Supplementary Table 1 and Figure 3).
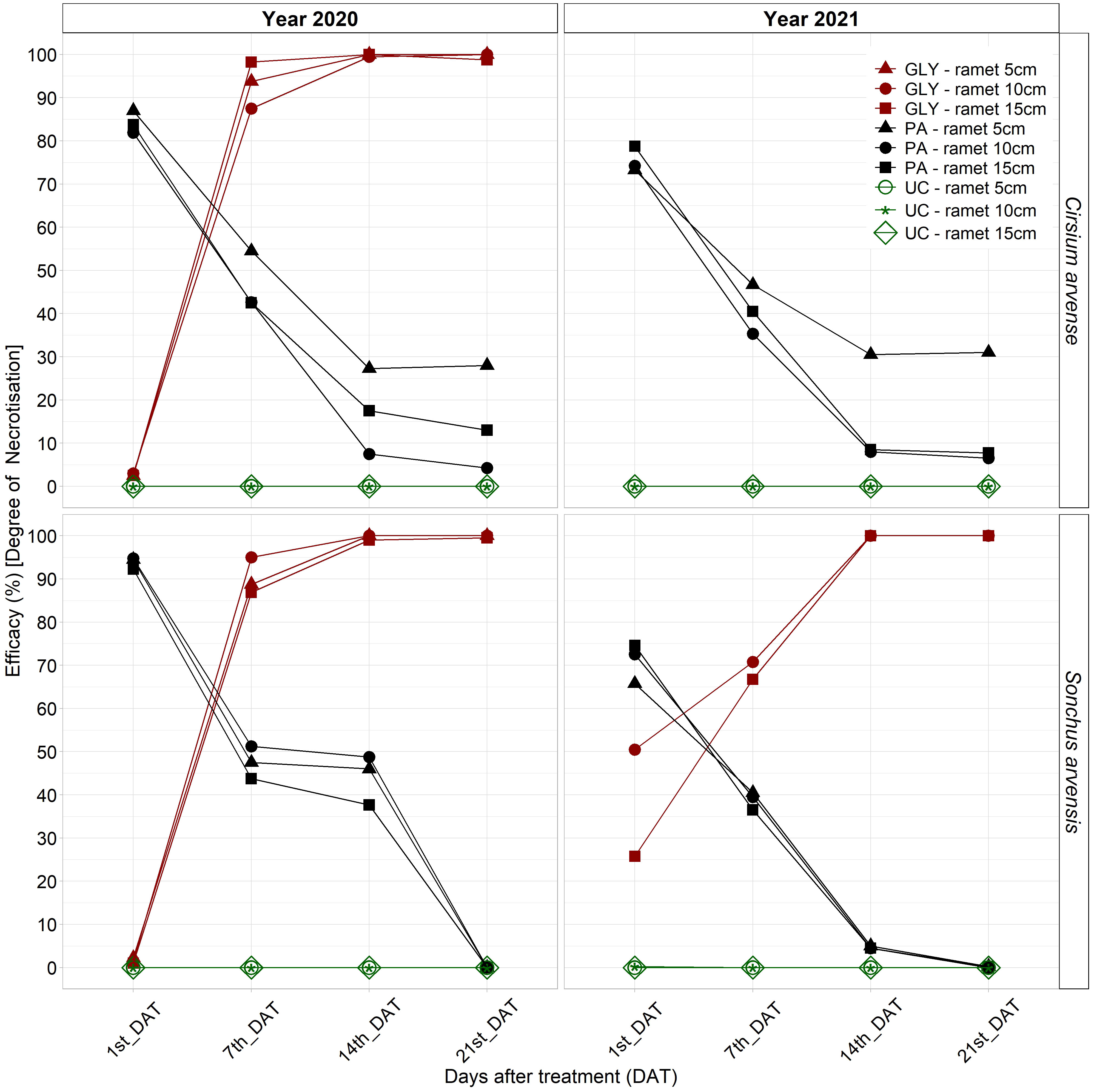
Figure 3 PA treatment efficacy (%) [degree of necrotization] compared with the untreated control and GLY treatment at 1st, 7th, 14th, and 21st_DAT after application on C. arvense and S. arvensis in the first year and repeated application in the second year of experiment. PA, Pelargonic acid; GLY, glyphosate; UC, untreated control.
After repeated application in 2021, the effect of the PA treatment showed a similar trend and efficacy on both weed species. The GLY treatment in this experiment showed 90 -100 % efficacy for all ramet sizes in both species starting at 7th_DAT in 2020 and on a day between 7th and 14th_DAT in 2021. The plants with the smallest ramet size treated with GLY in 2020 did not regrow in 2021. The C. arvense plants with initial ramet sizes of 10 cm and 15 cm treated with GLY did not regrow in the second year of the experiment either. Therefore, GLY treatment efficacies for all ramet sizes of C. arvense and the 5 cm ramet size in S. arvensis are not displayed on the graph in the second year (Figure 3).
For the evaluation of herbicide efficacy at 21st_DAT in both 2020 and 2021, individual effects of weed species and ramet size were found to be non-significant (Supplementary Figure 1). At 21st_DAT in both years, the PA treatment exhibited an effect that was not statistically significant, whereas the GLY treatment demonstrated a statistically significant impact. In 2020, the interaction effects between S. arvense and the PA treatment as well as between the 5 cm ramet size and the PA treatment did not exhibit a statistical significance. In 2021, the interaction effects between the GLY treatment and the initial ramet size of 10 cm showed a significant positive influence. There was a statistically significant positive association between the 5 cm ramet size and herbicide efficacy when the PA treatment was applied (Supplementary Figure 1 and Figure 3).
3.2 Regrowth after herbicide application
3.2.1 Nondestructive regrowth evaluation
For shoot density per m2, the main effects of weed species and the interaction between weed species with the GLY treatment were significant, while other fixed effects and their interactions did not have a significant effect (Figure 4). According to these results, the difference between species was statistically significant. With the untreated control and considering the same initial ramet size, the shoot density of S. arvensis was on average 249.8 shoots per m2 greater than C. arvense. With the PA treatment, after regrowth the shoot density of C. arvense for all initial ramet sizes was significantly lower than for S. arvensis. There was no statistically significant difference between the PA and UC treatments in all initial ramet sizes for both weed species. However, when not accounting for initial ramet size, application of PA compared with untreated control led to a maximum 9 % reduction in shoot density of C. arvense per m2. Shoot density in the GLY treatments was significantly lower than in all the other treatments (Figure 4).
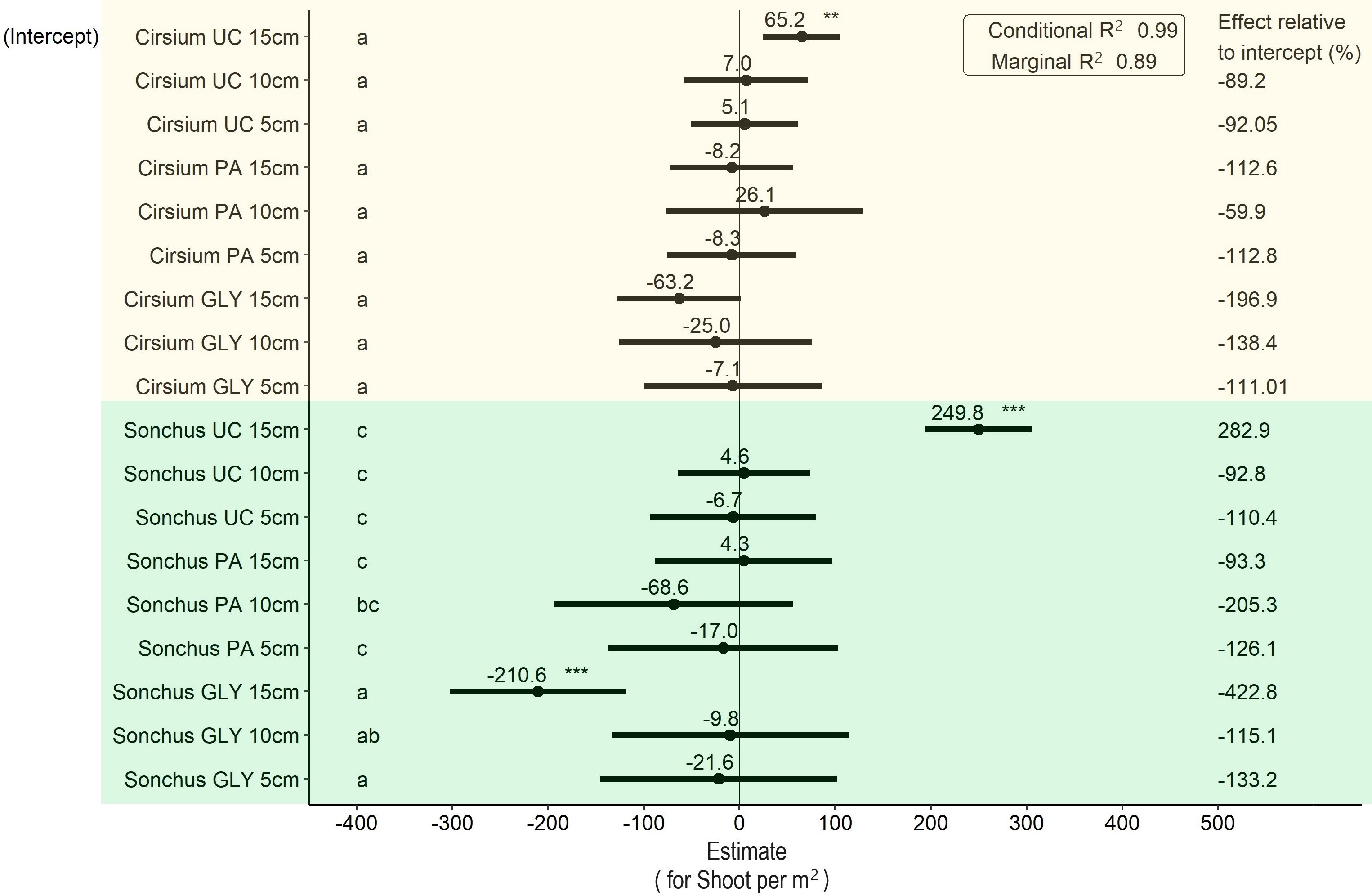
Figure 4 Results of the LMM analysis followed by Tukey’s HSD on shoot density per m2 at the end of the experiment. Significance codes obtained from the LMM analysis for main effects shown in this graph are as follows: * p< 0.05, ** p< 0.01, *** p< 0.001. Groups with different letters are significantly different at p< 0.05 level based on Tukey’s HSD test. PA, Pelargonic acid; GLY, glyphosate; UC, untreated control; Cirsium, Cirsium arvense; Sonchus, Sonchus arvensis. 5 cm, 10 cm and 15 cm represent the initial size of ramets.
The results showed significant differences in soil coverage between the two plant species and between the three levels of herbicide (Figure 5). The average soil coverage of S. arvensis was 27.8% higher than C. arvense when S. arvensis with the same initial ramet size was exposed to the UC treatment. The average soil coverage by plants treated with GLY was 65.4 % lower than that of UC plants in the same weed species and with the same initial ramet size. This difference was also statistically significant. Although PA-treated C. arvense plants covered an average lower percentage of the soil surface on average than PA-treated S. arvensis plants, the difference between the two species was not significant for any ramet size. In C. arvense, the initial ramet size of 5 cm treated with PA covered around 50% of the soil surface, while for the same ramet size under UC around 70 % of the soil surface was covered. S. arvensis with an initial ramet size of 10 cm also showed the lowest soil coverage compared with UC with the same initial ramet size. However, the differences in both cases were not statistically significant (Figure 5).
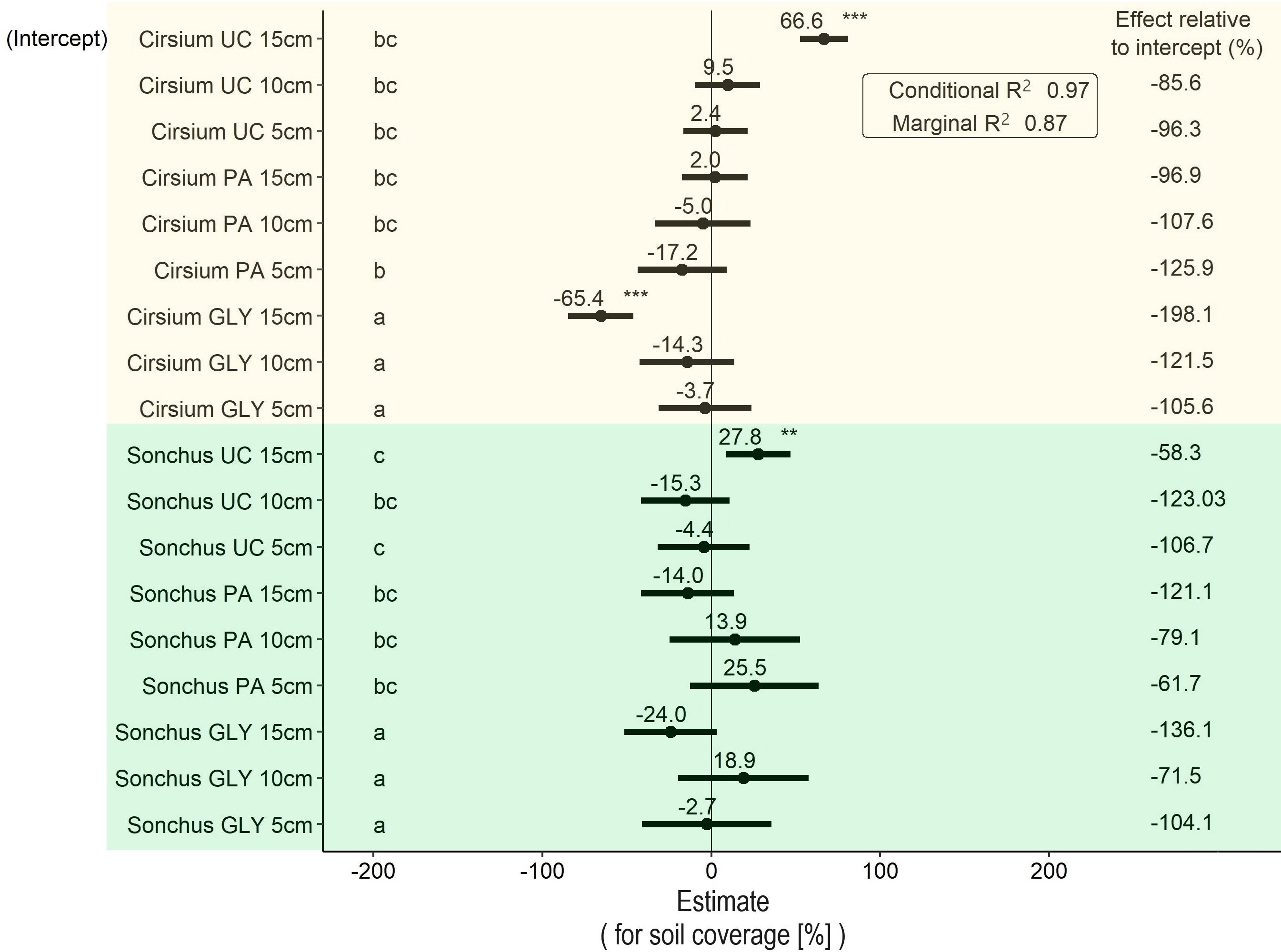
Figure 5 Results of the LMM analysis followed by Tukey’s HSD on soil surface coverage (%) at the end of the experiment. Significance codes obtained from the LMM analysis for main effects shown in this graph are as follows: * p< 0.05, ** p< 0.01, *** p< 0.001. Groups with different letters are significantly different at p< 0.05 level based on Tukey’s HSD test. PA, Pelargonic acid; GLY, glyphosate; UC, untreated control; Cirsium, Cirsium arvense; Sonchus, Sonchus arvensis. 5 cm, 10 cm and 15 cm represent the initial size of ramets.
The main effect of herbicide treatments on the number of flowers was significant (Figure 6). When comparing untreated plants with the same initial ramet size, S. arvensis produced approximately 10 flowers more than C. arvense on average. PA-treated plants of S. arvensis in 5 cm and 15 cm ramet sizes produced a smaller number of flowers compared with UC of the same ramet sizes, while a smaller number of flowers were produced by C. arvense with initial ramet sizes of 5 cm and 10 cm, but these differences were not statistically significant (Figure 6).
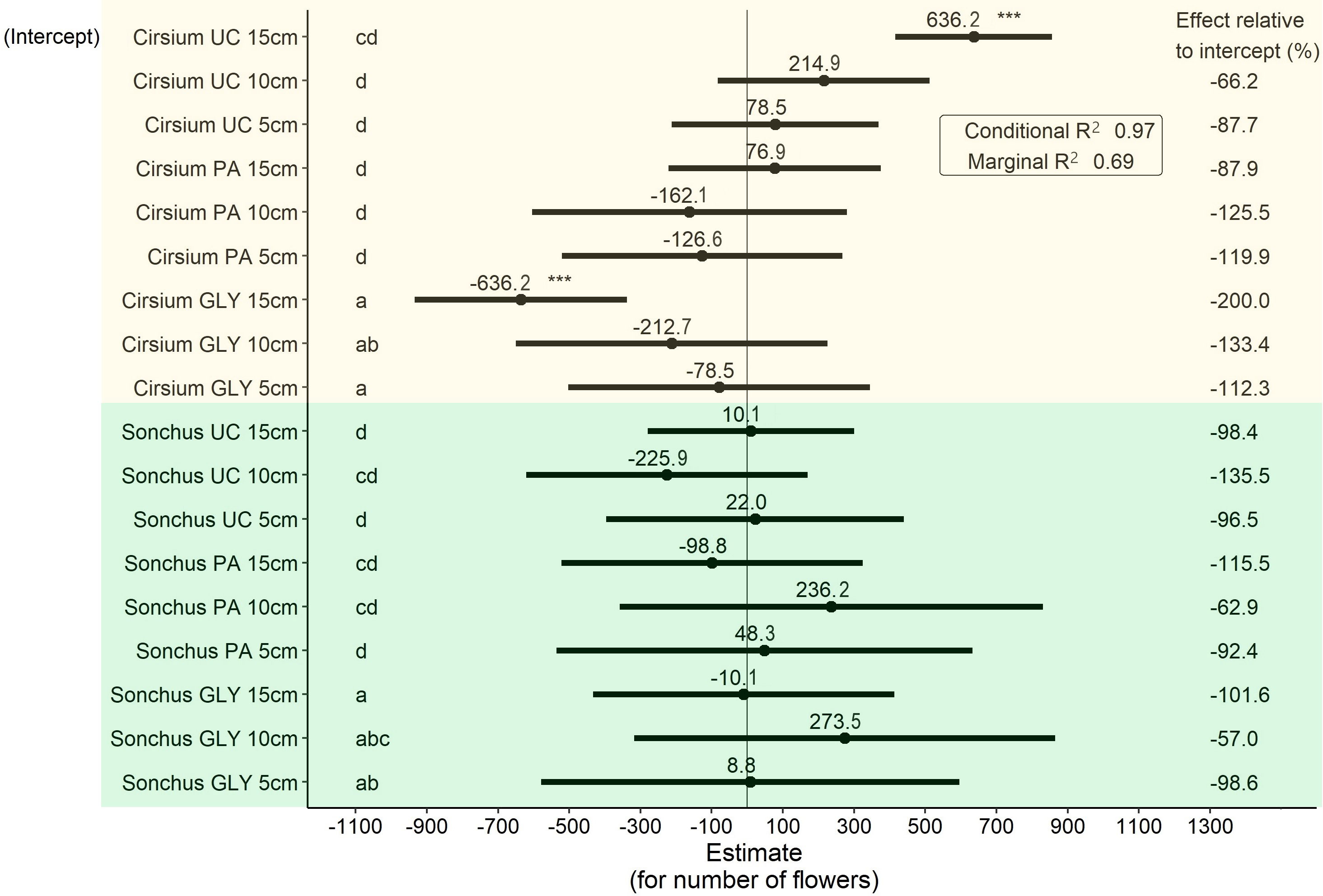
Figure 6 Results of the LMM analysis followed by Tukey’s HSD on the number of produced flowers at the end of the experiment. Significance codes obtained from the LMM analysis for main effects shown in this graph are as follows: * p< 0.05, ** p< 0.01, *** p< 0.001. Groups with different letters are significantly different at p< 0.05 level based on Tukey’s HSD test. PA, Pelargonic acid; GLY, glyphosate; UC, untreated control; Cirsium, Cirsium arvense; Sonchus, Sonchus arvensis. 5 cm, 10 cm and 15 cm represent the initial size of ramets.
3.2.2 Destructive evaluation of biomass
The results for aboveground and belowground biomass demonstrated no evidence of differences between the two weed species after regrowth (Figure 7; Supplementary Tables 2, 3). The fixed effect of herbicide treatments was significant. No significant difference was found in aboveground or belowground biomass between the PA treatments and UC treatments. The effect of initial ramet size on both biomass categories was not significant either. However, the results showed a significant interaction effect between PA treatment and ramet size of 5 cm in the case of aboveground biomass. The results also suggested that there might be an interaction effect on aboveground biomass between species, PA treatment, and ramet size of 5 cm, but this was not strong enough to be considered statistically significant. In the case of belowground biomass, there was a statistically significant interaction effect between species and ramet size of 10 cm (Supplementary Tables 2, 3).
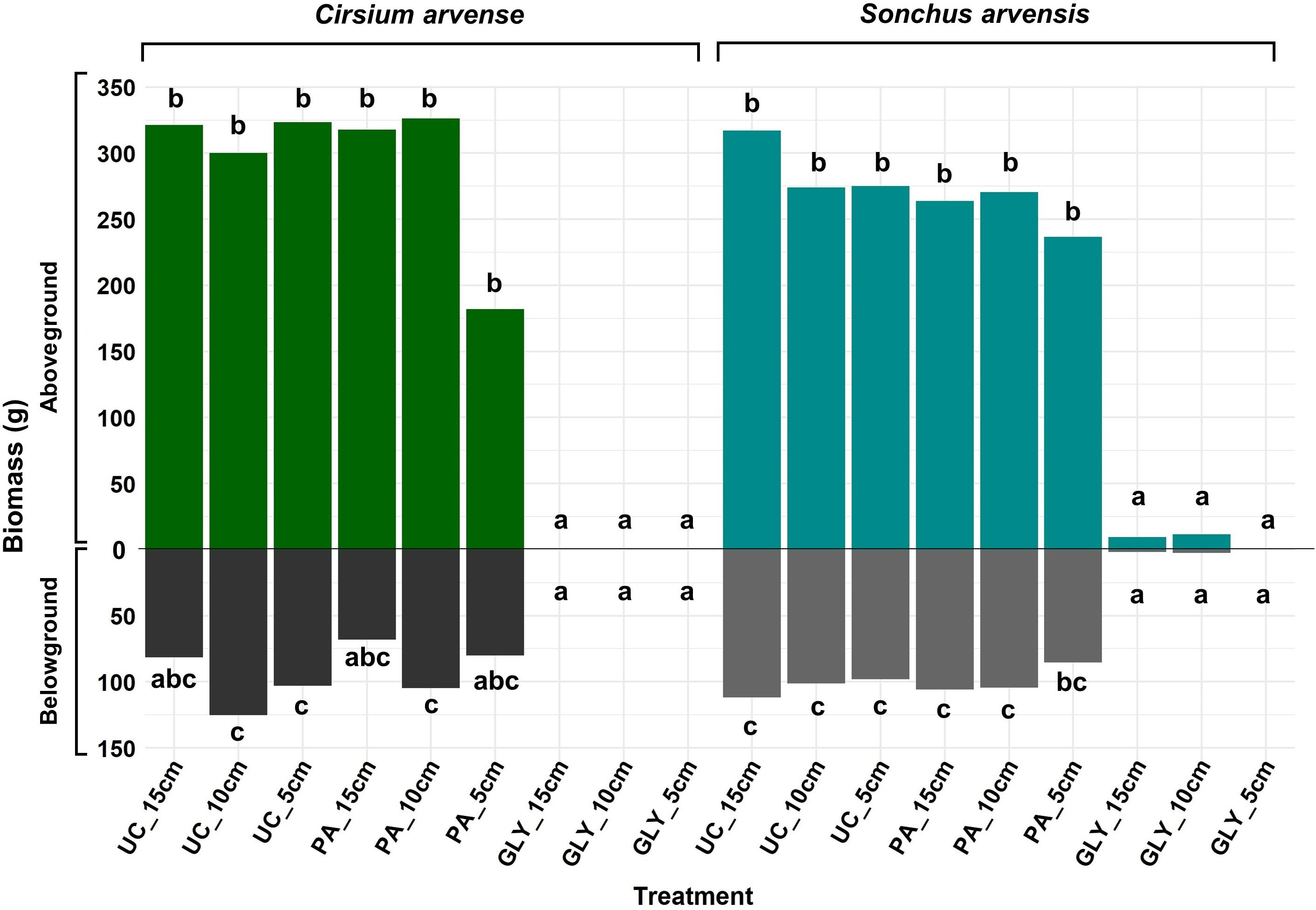
Figure 7 Aboveground and belowground biomass of C. arvense and S. arvensis after regrowth. Different letters show significant differences between treatments at p< 0.05 using Tukey’s HSD test separately for aboveground biomass and belowground biomass. PA, Pelargonic acid; GLY, glyphosate; UC, untreated control; 5 cm, 10 cm and 15 cm represent the initial size of ramets.
The results showed no significant differences in aboveground and belowground biomass between PA-treated plants and UC treatments for any ramet size (Figure 7). Nevertheless, when comparing the UC treatments with PA treatments in S. arvensis, the lowest aboveground biomass of 236.7 g and belowground biomass of 85.4 g belonged to PA-treated plants with an initial ramet size of 5 cm, which corresponds to reduction of 13 % and 12 % in aboveground and belowground biomass respectively. The lowest aboveground biomass for C. arvense was 181.9 g obtained from PA-treated plants with an initial ramet size of 5 cm, but the lowest belowground dry biomass was 68.2 g obtained from an initial ramet size of 15 cm treated with PA. When comparing untreated control and PA-treated plants in C. arvense, the application of PA decreased the belowground biomass of C. arvense by 22 % with a 5 cm initial ramet size and by 16 % when the ramet sizes were larger. The aboveground biomass of C. arvense with a 5 cm initial ramet size was reduced by 43 %, while for the larger ramet sizes, it was just 2 % (Figure 7).
3.3 Plant height during the experimental period
The main effects of experimental year, weed species, and herbicide treatments on plant height were statistically significant (Figure 8; Supplementary Table 4). Other significant interaction effects on plant height were found between species and the year 2021, and between herbicide treatments and the year 2021. Specifically, S. arvensis had almost the same height in 2021 compared with 2020, while C. arvense was taller in 2021 than in 2020. Generally, C. arvense tended to be significantly taller than S. arvensis. In the case of herbicide treatments, the PA treatment had a more negative effect on plant height in 2020 than in 2021. The application of the PA and GLY treatments resulted in significantly shorter plants compared with the UC, while initial ramet size did not have a significant effect on height (Supplementary Table 4). In 2021, PA-treated plants of C. arvense with an initial ramet size of 10 cm were significantly shorter than C. arvense plants with the same initial ramet size in the UC treatment. For S. arvensis, there were no significant differences between the PA and UC treatments (Figure 8; Supplementary Table 4).
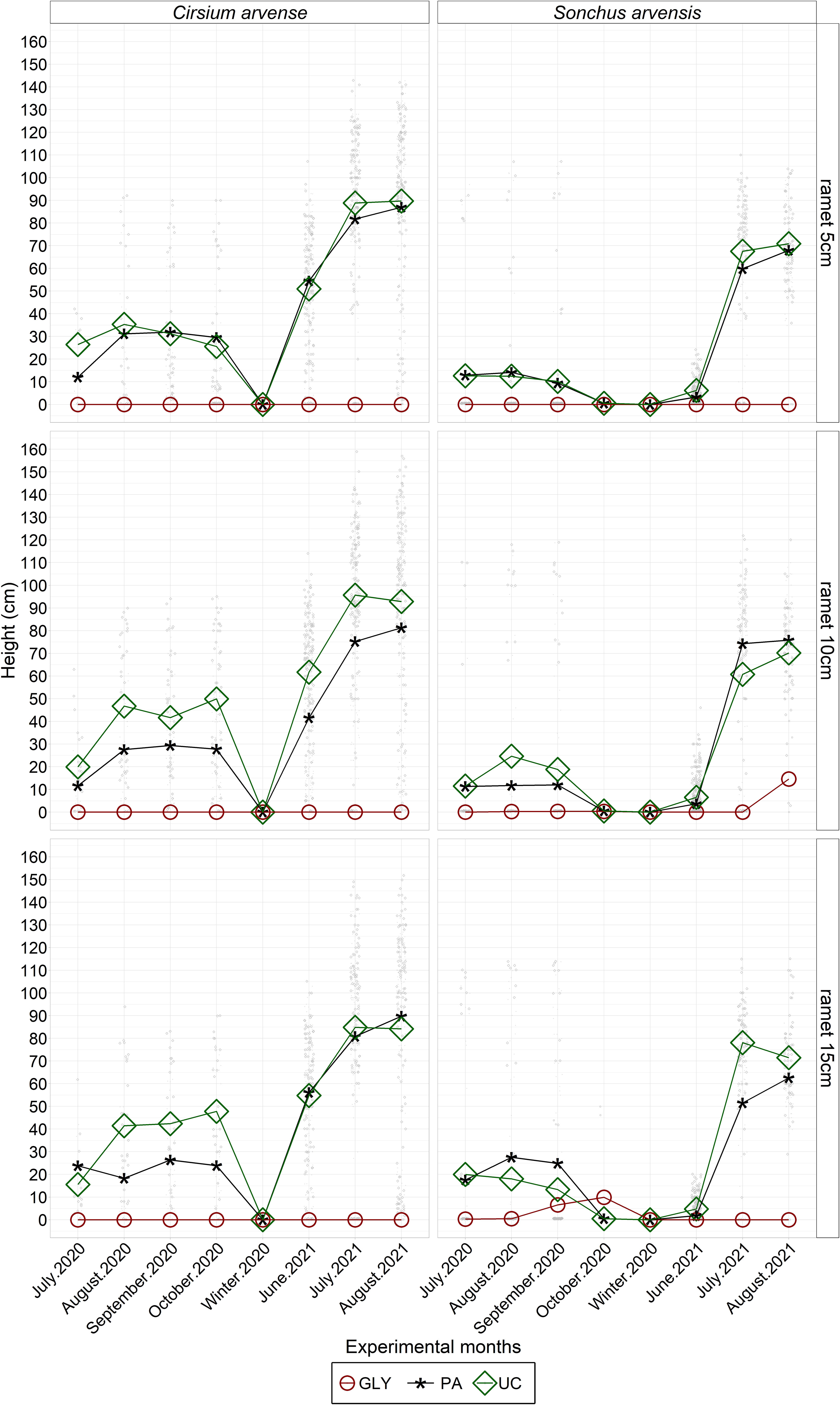
Figure 8 Monthly measured plant heights (cm) of regrown C. arvense and S. arvensis after herbicide applications during the experimental period. PA, Pelargonic acid; GLY, glyphosate; UC, untreated control.
4 Discussion
The aim of this study was to investigate the effects of twice application of PA on C. arvensis and S. arvensis in two consecutive growing seasons. Their regrowth after PA applications was evaluated by combining two approaches: targeting the aboveground parts of weed species with twice application of PA, and addressing the belowground root systems using three different initial ramet sizes. By adopting different initial ramet sizes, the objective was to obtain insights into the effects of fragmenting the creeping root system of perennials as it would be affected by mechanical disturbance (e.g. tillage practices) in real on-farm situations. However, the use of initial ramet sizes and their effect on PA efficacy did not represent mechanical control, rather, offered a valuable perspective and understanding of the fragmentation effects of creeping roots.
This study provided evidence that PA application reduces the aboveground and belowground biomass, as well as flower production in C. arvensis and S. arvensis. This was remarkable when the initial ramet size was smaller, suggesting that PA exhibits an enhanced efficacy on these plants when applied on the same patches repeatedly in combination with smaller initial root fragments. However, the quantity and persistence of these effects were lower than those produced by the GLY treatment. After application of GLY in the first experimental year, C. arvense did not regrow regardless of ramet size, and S. arvense demonstrated regrowth only for ramet sizes of 10 cm and 15 cm. In November 2023, the European Commission announced the extension of the glyphosate license for another decade after months of debate. However, this renewed license would be accompanied by new limitations and rules. The statement also highlighted that governments retained the authority to restrict the use of glyphosate in their own countries if they deemed the risks too high (Casassus, 2023), particularly concerning the preservation of biodiversity (Sullivan and Sullivan, 2003; Andert et al., 2022; El Jaouhari et al., 2023). Given the strong criticism regarding the use of glyphosate, as well as the new limitations and rules imposed by the commission, it is still crucial to find sustainable alternatives for it (Antier et al., 2020; Beckie et al., 2020; Casassus, 2023). Glyphosate is a non-selective systemic herbicide. Its effect is not visible in the early days after application because the plant takes some days to absorb and distribute glyphosate inside its tissues, which varies depending on the plant type (annual, perennial), growth stage, and environmental conditions (Sprankle et al., 1975; Satchivi et al., 2000; Fadin et al., 2018). PA causes necrotic lesions on plant aerial parts by attacking and destroying cell membranes of the plant epidermis, and causing rapid tissue dehydration (Ciriminna et al., 2019; Campos et al., 2022b). The PA mode of action implies that as a burndown herbicide, it could be a fast but temporary solution for controlling weeds, especially when there are work bottlenecks such as weather conditions or time constraints (Webber et al., 2014c; Campos et al., 2022a; Pannacci et al., 2022). Although PA may offer various advantages, it is occasionally misinterpreted as being similar to glyphosate and other pre-emergence herbicides, creating misleading expectations about its effectiveness and thus improper use (Campos et al., 2022b). A single application of PA does not provide lasting weed control (Patton et al., 2019; Loddo et al., 2023). Nevertheless, the present results show that it has the potential to be used in combination with other approaches, and thus offers an alternative to glyphosate against perennial weeds. Its combination with the smallest ramet size reduced growth parameters compared with the untreated control, even though there was no second fragmentation or soil mechanical treatments during the two-year experimental period. This finding is similar to the findings previously reported by Kanatas et al. (2020) that the use of a stale seedbed method integrated with the application of PA decreases perennial weeds. Other studies using PA as a weed control tool suggest the use of PA as a valuable tool for weed management approaches that use multiple tactics (Kanatas et al., 2022; Pannacci et al., 2022; Loddo et al., 2023). Due to its rapid burn-down effect, PA has a wide range of practical applications in weed management (Crmaric et al., 2018; Krauss et al., 2020), such as precision spot weeding (Webber and Webber, 2011; EFSA (European Food Safety Authority), 2021), crop desiccation, and sucker control in plants (Coleman and Penner, 2008; Short et al., 2020). Given its effectiveness on many annual herbaceous weeds, it could potentially be used to manage weed growth in stubble and for pre-sowing herbicide applications (Andert and Gerowitt, 2020). The use of soil cultivation tools on a farm infested with perennial weeds such as S. arvensis leads to root fragmentation and buries the fragmented roots deep in the soil (Brandsæter et al., 2017), or brings them to the soil surface and enhances the decay of root fragments (Vanhala et al., 2006). It is likely that small perennial weed plants remain in the field after harvest, and if stubble cultivation does not control these small plants, they will accumulate nutrient reserves in their creeping roots for the next growing season (Håkansson, 2003; Vanhala et al., 2006). During this period, PA application might help achieve successful perennial weed control because PA can be applied on the 4 8-leaf-stage plants (Ganji et al., 2022) that are not only at their sensitive aboveground stage (Håkansson, 2003; Tavaziva, 2012), but also face a lack of nutrient reserves due to fragmentation by soil cultivation tools (Brandsæter et al., 2010). In this study, enhancement in PA efficacy was observed when applied on plants at the 4-8-leaf-stage, that grew from initial smaller ramet sizes, proving the sensitivity of plants because of their smaller amount of nutrient reserves according to the abovementioned research findings.
It was anticipated that S. arvensis would probably be more susceptible to PA than C. arvense due to seasonal variations affecting its sprouting ability. As anticipated, the regrowth of weed species after herbicide did differ, but contrary to expectations, C. arvense seemed to be more susceptible to PA than S. arvensis (Figures 7, 8). The assessments of plant growth parameters after regrowth suggested that shoot density per m2 and soil coverage varied based on the weed species (Figures 4, 5). When comparing weed species considering the same initial ramet size, S. arvensis has a higher shoot density per m2. When comparing the effects of PA treatment on shoot density between the two species, C. arvense has a lower shoot density than S. arvensis. According to the investigation carried out by Liew et al. (2013), the higher shoot density in S. arvensis compared with C. arvense is due to the presence of a higher bud density on adventitious roots of S. arvensis. In S. arvensis, there was an observable effect of 5 cm ramet size on PA efficacy. There was a lower shoot density per m2 in PA-treated S. arvensis with 5 cm initial ramet compared with the untreated control. Previous studies have confirmed that a longer root fragment of S. arvensis produces more shoots than a shorter one (Anbari et al., 2011). In the untreated conditions of the current study, S. arvensis generally exhibited greater soil coverage than C. arvense. PA application resulted in a lower percentage of coverage for both species, although it was lower for C. arvense plants, particularly with smaller initial ramets, than for S. arvensis plants. These results are in agreement with the findings of Ward and Mervosh (2012) whose application of a PA treatment for two consecutive years on the same plot effectively reduced the coverage of Microstegium vimineum.
In general, PA-treated plants produced a smaller number of flowers than untreated control plants (Figure 6). Among PA-treated plants in both species, the smaller ramet size exhibited a smaller number of flowers. In a semi-field experiment using boxes, Anbari et al. (2011) tested the sprouting and shoot development of S. arvensis in relation to initial ramet size. They reported a positive correlation between the flower number and ramet length in S. arvensis and proved that the fragmentation of creeping roots delayed growth and reduced flower production (Anbari et al., 2011). These results are in agreement with the results of the present study.
The 5 cm initial ramet size enhanced PA efficacy, and a reduction in the aboveground and belowground biomass of both species under the mentioned treatments was observed, although it was not statistically significant (Figure 7). In previous studies conducted by Gustavsson (1997) on C. arvense and by Anbari et al. (2011) on S. arvensis, a smaller aboveground biomass was produced by a shorter ramet size than by a longer ramet size. The results of the present study in relation to aboveground biomass are in agreement with these findings. For C. arvense, the lowest belowground biomass was found for the 15 cm initial ramet size when comparing PA treatments with each other. Additionally, among untreated C. arvense plants, the biomass for the initial ramet size of 15 cm was the lowest. One reason for this could be environmental conditions. C. arvense biomass increases when more water is available (Sciegienka et al., 2011). When the temperature is lower and the photoperiod is shorter, then the root biomass of C. arvense is higher than shoot biomass. With an increase in temperature, the shoot growth increases and results in taller and more robust plants, which rapidly form flower heads (Hunter and Smith, 1972). In the present study, the aboveground biomass produced by plants with the initial ramet size of 15 cm was high due to favorable temperatures and high precipitation in both experimental years (particularly the high precipitation one month before biomass measurements). Moreover, PA-treated C. arvense with an initial ramet size of 15 cm produced a larger number of flowers than untreated C. arvense with the same initial ramet size. Furthermore, the produced aboveground biomass was almost similar between these two treatments, while the belowground biomass in PA-treated C. arvense was smaller than that of the untreated plants. It can thus be inferred that PA-treated C. arvense with the initial ramet size of 15 cm attempted to ensure its survival by producing more flowers, which leads to more aboveground biomass production and more belowground depletion. Additionally, it should be considered that there can be an effect of root longevity, and the creeping roots of C. arvense cannot live longer than one to two years (Moore, 1975; Bourdôt et al., 2000; Leathwick and Bourdôt, 2012).
Plant height is a direct indicator of herbicide impact, providing detailed information on how the herbicide influences the physical structure of plants. Therefore, the dynamic changes in monthly plant height after herbicide application provided insights into the regrowth patterns of both weed species in both experimental years (Figure 8). The average height of S. arvensis was similar in both years, while the height of C. arvense was greater in 2021. PA application reduced plant height compared with untreated plants in both years. However, this plant height reduction in 2020 was greater than in 2021. The results showed that the effects of species and herbicides varied depending on the year. This could be due to the variations in weather conditions between 2020 and 2021, and unexpected environmental effects, as discussed by Hunter and Smith (1972) and Sciegienka et al. (2011). The effect of PA on the height of weed species in the present study is in line with earlier studies on Lolium rigidum Gaud and Avena sterilis L., which reported a lower height in PA-treated plants compared with untreated plants (Travlos et al., 2020). Overall, the ramet size of 5 cm produced shorter plants in all treatments. This supports the findings of Sciegienka et al. (2011) on C. arvense that a smaller root fragment size produces shorter plants.
The herbicide efficacy analysis determined the high efficacy of PA compared with the untreated control and revealed the negative relationship of PA efficacy with days after applications in both years (Figure 3). Due to its rapid effect, PA efficacy was higher compared with other treatments at the beginning and until 7th day after application, but then declined over time due to the occurrence of regrowth. The findings of previous research on both annual and perennial species have demonstrated that PA reaches its maximum efficacy within several hours of application and remains effective for up to one week, although the plant regrowth subsequently reduces its efficacy (Muñoz et al., 2020; Travlos et al., 2020; Ganji et al., 2022; Muñoz et al., 2022; Pannacci et al., 2022; Ganji et al., 2023; Loddo et al., 2023).
5 Conclusions
It is concluded that a two-year application of PA on the same specific spot in combination with a smaller ramet size facilitates the development of integrated weed management (IWM) strategies. To reduce the infestation level of perennial weeds, PA application could be combined with mechanical fragmentation of creeping roots in the intercropping period. From today’s perspective, however, PA is registered on the European market for use as a plant desiccant in potatoes and to kill suckers in perennial crops, such as hops and grapevine. It is currently not registered for other applications in arable crops. Since current policies towards restricting the use of synthetic herbicides in arable farming enforce the use of alternatives such as bio-herbicides, efforts at economic and political levels are required. To ensure the proper use of this active substance, it is crucial to educate farmers about integrated weed management, conduct field applications at recommended times, and adhere to label instructions. This would help a suitable niche market for this active ingredient to be established.
On the market, bio-herbicides based on PA are costly, and their application rate per hectare is higher compared with synthetic herbicides, and may not be cost-effective for large-scale applications in arable farming. Therefore, expanding the application time from multiple repeated applications in one year to a two-year repeated applications on the same spot might assist with financially balancing PA application costs and achieving acceptable weed control. Further research studies should be undertaken to perform financial comparisons of PA applications and synthetic herbicides. Additional studies regarding enhancements in the technical aspects of PA application, such as the incorporation of adjuvants or the adjustment of water volume to achieve more comprehensive plant coverage, are essential for more successful weed control.
Data availability statement
The data presented in this study are available upon reasonable request from the corresponding author. The data are not publicly available due to project funding policy. Requests to access the datasets should be directed to Eliyeh Ganji, ZWxpeWVoLmdhbmppQHVuaS1yb3N0b2NrLmRl.
Author contributions
EG: Conceptualization, Data curation, Formal analysis, Investigation, Methodology, Validation, Visualization, Writing – original draft, Writing – review & editing. SA: Conceptualization, Supervision, Validation, Writing – review & editing.
Funding
The author(s) declare financial support was received for the research, authorship, and/or publication of this article. This research was part of the project “AC/DC-weeds-Applying and Combining Disturbance and Competition for an agro-ecological management of creeping perennial weeds” funded by ERA-Net Cofund SusCrop/EU Horizon 2020, Grant no. 771134. The German part is funded by DFG (Deutsche Forschungsgemeinschaft), GE 558/3-1.
Acknowledgments
We sincerely thank our colleagues in the Crop Health group at the University of Rostock for their technical assistance. We also would like to acknowledge the support of the Department of Hydrology and Applied Meteorology at the University of Rostock for providing weather data.
Conflict of interest
The authors declare that the research was conducted in the absence of any commercial or financial relationships that could be construed as a potential conflict of interest.
Publisher’s note
All claims expressed in this article are solely those of the authors and do not necessarily represent those of their affiliated organizations, or those of the publisher, the editors and the reviewers. Any product that may be evaluated in this article, or claim that may be made by its manufacturer, is not guaranteed or endorsed by the publisher.
Supplementary material
The Supplementary Material for this article can be found online at: https://www.frontiersin.org/articles/10.3389/fagro.2024.1330199/full#supplementary-material
References
Anbari S., Lundkvist A., Verwijst T. (2011). Sprouting and shoot development of Sonchus arvensis in relation to initial root size. Weed Res. 51 (2), 142–150. doi: 10.1111/j.1365-3180.2010.00837.x
Andert S., Gerowitt B. (2020). Controlling arable weeds with natural substances as bio-based herbicides. Tagungsband: 29. Deutsche Arbeitsbesprechung über Fragen der Unkrautbiologie und - bekämpfung; 3. - 5. März 2020, Braunschweig: Julius Kühn-Institut. 464, 407–414. doi: 10.5073/jka.2020.464.061
Andert S., de Mol F., Koning L., Gerowitt B. (2022). Weed response in winter wheat fields on a gradient of glyphosate use in the recent past. Agric. Ecosyst. Environ. 333, 107977. doi: 10.1016/j.agee.2022.107977
Andert S., Guguin J., Hamacher M., Valantin-Morison M., Gerowitt B. (2023). How farmers perceive perennial weeds in Northern France and Eastern Germany. Front. Agron. 5. doi: 10.3389/fagro.2023.1247277
Antier C., Kudsk P., Reboud X., Ulber L., Baret P. V., Messéan A. (2020). Glyphosate use in the European agricultural sector and a framework for its further monitoring. Sustainability 12 (14), 5682. doi: 10.3390/su12145682
Barker A. V., Prostak R. G. (2009). Alternative management of roadside vegetation. HortTechnology. 19 (2), 346–352. doi: 10.21273/HORTTECH.19.2.346
Barton A. F., Clarke B. R., Dell B., Knight A. R. (2014). Post-emergent herbicidal activity of cineole derivatives. J. Pest. Sci. 87, 531–541. doi: 10.1007/s10340-014-0566-6
Bashir T., Anum W., Ali I., Ghaffar A., Ali L., Raza M. U., et al. (2018). Allelopathic effects of perennial sow thistle (Sonchus arvensis L.) on germination and seedling growth of maize (Zea mays L.). Allelopathy J. 43 (1), 105–116. doi: 10.26651/allelo.j/2018-43-1-1134
Bates D., Mächler M., Bolker B., Walker S. (2015). Fitting linear mixed-effects models using lme4. J. Stat. Software 67 (1), 1–48. doi: 10.48550/arXiv.1406.5823
Beckie H. J., Flower K. C., Ashworth M. B. (2020). Farming without glyphosate? Plants. 9 (1), 96. doi: 10.3390/plants9010096
Bhullar M. S., Kaur S., Kaur T., Singh T., Singh M., Jhala A. J. (2013). Control of broadleaf weeds with post-emergence herbicides in four barley (Hordeum spp.) cultivars. Crop Prot. 43, 216–222. doi: 10.1016/j.cropro.2012.10.005
Bourdôt G. W., Leathwick D. M., Hurrell G. A. (2000). Longevity of Californian thistle roots. N. Z. Plant Prot. 53, 258–261. doi: 10.30843/nzpp.2000.53.3704
Brandsæter L. O., Fogelfors H., Fykse H., Graglia E., Jensen R. K., Melander B., et al. (2010). Seasonal restrictions of bud growth on roots of Cirsium arvense and Sonchus arvensis and rhizomes of Elymus repens. Weed Res. 50 (2), 102–109. doi: 10.1111/j.1365-3180.2009.00756.x
Brandsæter L. O., Mangerud K., Helgheim M., Berge T. W. (2017). Control of perennial weeds in spring cereals through stubble cultivation and mouldboard ploughing during autumn or spring. Crop Prot. 98, 16–23. doi: 10.1016/j.cropro.2017.03.006
Campos J., Bodelon L., Verdeguer M., Baur P. (2022a). Mechanistic aspects and effects of selected tank-mix partners on herbicidal activity of a novel fatty acid ester. Plants 11 (3), 279. doi: 10.3390/plants11030279
Campos J., Mansour P., Verdeguer M., Baur P. (2022b). Contact herbicidal activity optimization of methyl capped polyethylene glycol ester of pelargonic acid. J. Plant Dis. Prot. 130 (1), 93–103. doi: 10.1007/s41348-022-00661-0
Carroll D. E., Kaminski J. E., Borger J. A. (2022). Efficacy of natural herbicides on dandelion (Taraxacum officinale GH Weber ex Wiggers) and white clover (Trifolium repens L.) populations. ITSRJ 14 (1), 759–769. doi: 10.1002/its2.8
Casassus B. (2023). EU allows use of controversial weedkiller glyphosate for 10 more years. Nature. doi: 10.1038/d41586-023-03589-z
Casella F., Vurro M., Valerio F., Perrino E. V., Mezzapesa G. N., Boari A. (2023). Phytotoxic effects of essential oils from six lamiaceae species. Agronomy 13 (1), 257. doi: 10.3390/agronomy13010257
Ciriminna R., Fidalgo A., Ilharco L. M., Pagliaro M. (2019). Herbicides based on pelargonic acid: Herbicides of the bioeconomy. Biofpr. 13 (6), 1476–1482. doi: 10.1002/bbb.2046
Coleman R., Penner D. (2008). Organic acid enhancement of pelargonic acid. Weed Tech. 22 (1), 38–41. doi: 10.1614/WT-06-195.1
Cordeau S., Triolet M., Wayman S., Steinberg C., Guillemin J. P. (2016). Bioherbicides: Dead in the water? A review of the existing products for integrated weed management. J. Crop Prot. 87, 44–49. doi: 10.1016/j.cropro.2016.04.016
Crmaric I., Keller M., Krauss J., Delabays N. (2018). “Efficacy of natural fatty acid based herbicides on mixed weed stands,” in Tagungsband 28. Deutsche Arbeitsbesprechung über Fragen der Unkrautbiologie und – bekämpfung : 27. Februar - 1. Eds. Nordmeyer H., Ulber L.. Julius Kühn-Institut. 458, 328–333 doi: 10.5073/jka.2018.458.048
EFSA (European Food Safety Authority), Alvarez F., Arena M., Auteri D., Borroto J., Brancato A., et al. (2021). Conclusion on the peer review of the pesticide risk assessment of the active substance pelargonic acid (nonanoic acid). EFSA J. 19 (8), 6813. doi: 10.2903/j.efsa.2021.6813
Egushova E. A., Anokhina O. V. (2022). Allelopathic effect of weed extracts on vegetable seeds. IOP Conf. Ser. Earth Environ. Sci., 1010. doi: 10.1088/1755-1315/1010/1/012104
El Jaouhari M., Damour G., Tixier P., Coulis M. (2023). Glyphosate reduces the biodiversity of soil macrofauna and benefits exotic over native species in a tropical agroescosystem.Basic Appl. Ecol. 73, 18–26. doi: 10.1016/j.baae.2023.10.001
European Commission (2020). Farm to fork strategy: for a fair, healthy and environmentally-friendly food system. Available at: https://food.ec.europa.eu/horizontal-topics/farm-fork-strategy_en#Publications (Accessed December 03, 2023).
Fadin D. A., Tornisielo V. L., Barroso A. A. M., Ramos S., Dos Reis F. C., Monquero P. A. (2018). Absorption and translocation of glyphosate in Spermacoce verticillata and alternative herbicide control. Weed Res. 58 (5), 389–396. doi: 10.1111/wre.12329
Ganji E., Andert S., Gerowitt B. (2022). “The herbicidal potential of Pelargonic Acid to control Cirsium arvense (L.) Scop. in relation to the timing of application and the application volume,” in Tagungsband: 30. Deutsche Arbeitsbesprechung über Fragen der Unkrautbiologie und -bekämpfung. Eds. L. Ulber and D. Rissel. Julius Kühn-Institut. 468, 86–93. doi: 10.5073/20220117-074121
Ganji E., Grenzdörffer G., Andert S. (2023). Estimating the reduction in cover crop vitality followed by pelargonic acid application using drone imagery. Agronomy 13 (2), 354. doi: 10.3390/agronomy13020354
Gustavsson A. M. D. (1997). Growth and regenerative capacity of plants of Cirsium arvense. Weed Res. 37 (4), 229–236. doi: 10.1046/j.1365-3180.1997.d01-37.x
Håkansson S. (1969). Experiments with Sonchus arvensis L. I. Development and growth, and the response to burial and defoliation in different developmental stages. Lantbrukshögskolans Annaler 35, 989–1030.
Håkansson S. (2003). Weeds and weed management on arable land: An ecological approach (Wallingford, Oxon, UK, Cambridge: MA: CABI Pub). doi: 10.1079/9780851996516.0000
Håkansson S., Wallgren B. (1972). Experiments with Sonchus arvensis L. II. Reproduction, plant development and response to mechanical disturbance. Swed. J. Agric. Res. 2, 3–14.
Hamdoun A. M. (1972). Regenerative capacity of root fragments of Cirsium arvense (L.) Scop. Weed Res. 12 (2), 128–136. doi: 10.1111/j.1365-3180.1972.tb01196.x
Hudek L., Enez A., Bräu L. (2021). Comparative analyses of glyphosate alternative weed management strategies on plant coverage, soil and soil biota. Sustain. 13 (20), 11454. doi: 10.3390/su132011454
Hunter J. H., Smith L. W. (1972). Environment and herbicide effects on Canada thistle ecotypes. Weed Sci. 20 (2), 163–167. doi: 10.1017/S0043174500035268
Ibáñez M. D., Blázquez M. A. (2018). Phytotoxicity of essential oils on selected weeds: Potential hazard on food crops. Plants 7 (4), 79. doi: 10.3390/plants7040079
Kanatas P., Antonopoulos N., Gazoulis I., Travlos I. S. (2021). Screening glyphosate-alternative weed control options in important perennial crops. Weed Sci. 69 (6), 704–718. doi: 10.1017/wsc.2021.55
Kanatas P., Travlos I., Papastylianou P., Gazoulis I., Kakabouki I., Tsekoura A. (2020). Yield, quality and weed control in soybean crop as affected by several cultural and weed management practices. Not. Bot. Horti Agrobot. 48 (1), 329–341. doi: 10.15835/nbha48111823
Kanatas P., Zavra S. M., Tataridas A., Gazoulis I., Antonopoulos N., Synowiec A., et al. (2022). Pelargonic acid and caraway essential oil efficacy on barnyardgrass (Echinochloa crus-galli (L.) P. Beauv.) and johnsongrass (Sorghum halepense (L.) Pers.). Agronomy 12 (8), 1755. doi: 10.3390/agronomy12081755
Kouki H., Amri I., Souihi M., Pieracci Y., Trabelsi I., Hamrouni L., et al. (2023). Chemical composition, antioxidant, herbicidal and antifungal activities of leaf essential oils from three Tunisian Eucalyptus species. J. Plant. Dis. Prot. 130, 1411–1422. doi: 10.1007/s41348-023-00772-2
Krauss J., Eigenmann M., Keller M. (2020). “Pelargonic acid for weed control in onions: factors affecting selectivity,” in Tagungsband : 29. Deutsche Arbeitsbesprechung über Fragen der Unkrautbiologie und - bekämpfung. Eds. Nordmeyer H., Ulber L.. Braunschweig: Julius Kühn-Institut. 464, 415–419. doi: 10.5073/jka.2020.464.062
Kuznetsova A., Brockhoff P. B., Christensen R. H. B. (2017). lmerTest package: tests in linear mixed effects models. J. Stat. Software 82 (13), 1–26. doi: 10.18637/jss.v082.i13
Leathwick D. M., Bourdôt G. W. (2012). A conceptual model for the population dynamics of Cirsium arvense in a New Zealand pasture. N. Z. J. Agric. Res. 55 (4), 371–384. doi: 10.1080/00288233.2012.728532
Lemna W. K., Messersmith C. G. (1990). The biology of Canadian weeds. 94. Sonchus arvensis L. Can. J. Plant Sci. 70 (2), 509–532. doi: 10.4141/cjps90-060
Lenth R., Singmann H., Love J., Buerkner P., Herve M. (2018). Package “Emmeans”. R package version 4.0-3. Available at: http://cran.r-project.org/package=emmeans.
Liew J., Andersson L., Boström U., Forkman J., Hakman I., Magnuski E. (2013). Regeneration capacity from buds on roots and rhizomes in five herbaceous perennials as affected by time of fragmentation. Plant Ecol. 214, 1199–1209. doi: 10.1007/s11258-013-0244-4
Loddo D., Jagarapu K. K., Strati E., Trespidi G., Nikolić N., Masin R., et al. (2023). Assessing herbicide efficacy of pelargonic acid on several weed species. Agronomy 13 (6), 1511. doi: 10.3390/agronomy13061511
Meier U. (2018). Growth stages of mono- and dicotyledonous plants: BBCH Monograph (Quedlinburg: Open Agrar Repositorium). doi: 10.5073/20180906-074619
Melander B., Holst N., Rasmussen I. A., Hansen P. K. (2012). Direct control of perennial weeds between crops–Implications for organic farming. Crop Prot. 40, 36–42. doi: 10.1016/j.cropro.2012.04.029
Moore R. J. (1975). The biology of Canadian weeds.: 13. Cirsium arvense (L.) Scop. Can. J. Plant Sci. 55 (4), 1033–1048. doi: 10.4141/cjps75-163
Muñoz M., Torres-Pagán N., Jouini A., Araniti F., Sánchez-Moreiras A. M., Verdeguer M. (2022). Control of problematic weeds in Mediterranean vineyards with the bioherbicide pelargonic acid. Agronomy 12 (10), 2476. doi: 10.3390/agronomy12102476
Muñoz M., Torres-Pagán N., Peiró R., Guijarro R., Sánchez-Moreiras A. M., Verdeguer M. (2020). Phytotoxic effects of three natural compounds: Pelargonic acid, carvacrol, and cinnamic aldehyde, against problematic weeds in Mediterranean crops. Agronomy 10 (6), 791. doi: 10.3390/agronomy10060791
Pannacci E., Ottavini D., Onofri A., Tei F. (2022). Dose–response curves of pelargonic acid against summer and winter weeds in Central Italy. Agronomy 12 (12), 3229. doi: 10.3390/agronomy12123229
Patton A. J., Braun R. C., Weisenberger D. V. (2019). Single applications of natural postemergence weed control options do not provide effective ground ivy control. CFTM 5 (1), 1–7. doi: 10.2134/cftm2018.12.0101
Pinheiro J., Bates D., R Core Team (2023). nlme: Linear and Nonlinear Mixed Effects Models. R package version 3.1-163. Available at: https://CRAN.R-project.org/package=nlme.
Radicetti E., Mancinelli R. (2021). Sustainable weed control in the agro-ecosystems. Sustainability 13 (15), 8639. doi: 10.3390/su13158639
Ramesh K., Matloob A., Aslam F., Florentine S. K., Chauhan B. S. (2017). Weeds in a changing climate: vulnerabilities, consequences, and implications for future weed management. Front. Plant Sci. 8. doi: 10.3389/fpls.2017.00095
R Core Team. (2023). R: A language and environment for statistical computing (Vienna, Austria: R Foundation for Statistical Computing). Available at: https://www.r-project.org.
Satchivi N. M., Wax L. M., Stoller E. W., Briskin D. P. (2000). Absorption and translocation of glyphosate isopropylamine and trimethylsulfonium salts in Abutilon theophrasti and Setaria faberi. Weed Sci. 48 (6), 675–679. doi: 10.1614/0043-1745(2000)048[0675:AATOGI]2.0.CO;2
Sciegienka J., Keren E., Menalled F. (2011). Impact of root fragment dimension, weight, burial depth, and water regime on Cirsium arvense emergence and growth. Can. J. Plant Sci. 91 (6), 1027–1036. doi: 10.4141/cjps2011-059
Short M. M., Vann M. C., Suchoff D. H. (2020). Organic sucker control: screening different active ingredients for commercial application. Tob. Sci. 57 (1), 17–20. doi: 10.3381/TOBSCI-D-20-00008
Sprankle P., Meggitt W. F., Penner D. (1975). Absorption, action, and translocation of glyphosate. Weed Sci. 23 (3), 235–240. doi: 10.1017/S0043174500052930
Sullivan T. P., Sullivan D. S. (2003). Vegetation management and ecosystem disturbance: impact of glyphosate herbicide on plant and animal diversity in terrestrial systems. Environ. Rev. 11 (1), 37–59. doi: 10.1139/a03-005
Synowiec A., Kalemba D., Drozdek E., Bocianowski J. (2017). Phytotoxic potential of essential oils from temperate climate plants against the germination of selected weeds and crops. J. Pest. Sci. 90, 407–419. doi: 10.1007/s10340-016-0759-2
Szabó K., Halbritter P. S. R. (2015). Allelopathic effects of Cirsium arvense (L.) Scop. in Hun-gary. Bulg. J. Agric. Sci. 21 (5), 1012–1021.
Tavaziva V. J. (2012). Effects of competition on compensation point and phenological development in Sonchus arvensis L. [Master thesis] (Uppsala, Sweden: Swedish University of Agricultural Sciences). Available at: https://stud.epsilon.slu.se/4572/.
Tørresen K. S., Gerowitt B. (2022). Late-autumn ramet sprouting of three arable creeping perennial weed species. Agronomy 12 (9), 2175. doi: 10.3390/agronomy12092175
Travlos I., Rapti E., Gazoulis I., Kanatas P., Tataridas A., Kakabouki I., et al. (2020). The herbicidal potential of different pelargonic acid products and essential oils against several important weed species. Agronomy 10 (11), 1687. doi: 10.3390/agronomy10111687
Vanhala P., Lötjönen T., Hurme T. (2006). Managing Sonchus arvensis using mechanical and cultural methods. AFSci. 15 (4), 444–458. doi: 10.2137/145960606780061498
Verwijst T., Tavaziva V. J., Lundkvist A. (2018). Assessment of the compensation point of Cirsium arvense and effects of competition, root weight and burial depth on below-ground dry weight–leaf stage trajectories. Weed Res. 58 (4), 292–303. doi: 10.1111/wre.12312
Ward J. S., Mervosh T. L. (2012). Nonchemical and herbicide treatments for management of Japanese stiltgrass (Microstegium vimineum). Invasive Plant Sci. Manage. 5 (1), 9–19. doi: 10.1614/IPSM-11-00018.1
Webber C. L., Shrefler J. W., Taylor M. J. (2014c). Adjuvants affect duckweed (Lemna minor) control with pelargonic acid. J. Agric. Sci. 6 (12), 1. doi: 10.5539/jas.v6n12p1
Webber C. L., Taylor M. J., Shrefler J. W. (2014a). Weed control in yellow squash using sequential post directed applications of pelargonic acid. HortTechnology. 24 (1), 25–29. doi: 10.21273/HORTTECH.24.1.25
Webber C. L., Taylor M. J., Shrefler J. W. (2014b). Weed control in sweet bell pepper using sequential post directed applications of pelargonic acid. HortTechnology. 24 (6), 663–667. doi: 10.21273/HORTTECH.24.6.663
Webber C. L., Webber D. M. (2011). Duckweed control with over-the-top application of pelargonic acid. J. Environ. Monitor. Restor. 7, 78–86. doi: 10.4029/2011jemrest7no008
Keywords: perennial weeds, bio-herbicide, ramet, creeping thistle, sow-thistle
Citation: Ganji E and Andert S (2024) The effect of two-year application of pelargonic acid on the growth of Cirsium arvense (L.) Scop. and Sonchus arvensis L. Front. Agron. 6:1330199. doi: 10.3389/fagro.2024.1330199
Received: 30 October 2023; Accepted: 11 January 2024;
Published: 31 January 2024.
Edited by:
Rick Llewellyn, Commonwealth Scientific and Industrial Research Organisation (CSIRO), AustraliaReviewed by:
Agnieszka Synowiec, University of Agriculture in Krakow, PolandAhmet Uludag, Çanakkale Onsekiz Mart University, Türkiye
Copyright © 2024 Ganji and Andert. This is an open-access article distributed under the terms of the Creative Commons Attribution License (CC BY). The use, distribution or reproduction in other forums is permitted, provided the original author(s) and the copyright owner(s) are credited and that the original publication in this journal is cited, in accordance with accepted academic practice. No use, distribution or reproduction is permitted which does not comply with these terms.
*Correspondence: Eliyeh Ganji, ZWxpeWVoLmdhbmppQHVuaS1yb3N0b2NrLmRl
†Present address: Sabine Andert, Institute for Plant Protection in Field Crops and Grassland, Julius Kühn-Institut (JKI), Braunschweig, Germany