- 1Queensland Alliance for Agriculture and Food Innovation (QAAFI), The University of Queensland, Gatton, QLD, Australia
- 2Department of Agronomy, Punjab Agricultural University, Ludhiana, India
- 3Queensland Alliance for Agriculture and Food Innovation (QAAFI) and School of Agriculture and Food Sustainability (AGFS), The University of Queensland, Gatton, QLD, Australia
Annual ryegrass (Lolium rigidum Gaudin) is a problematic weed in most of the cropping regions in Australia. Evolved herbicide resistance to multiple modes of action groups, the lack of new herbicidal molecules, and the emergence of late cohorts in winter season crops necessitate the use of existing herbicides in an innovative way to control L. rigidum. Pendimethalin as pre-emergence (PRE) is used for L. rigidum control; however, it is unable to control late cohorts of L. rigidum in winter season crops. Pot studies were conducted from 2019 to 2021 to evaluate the effect of a post-emergence (POST) application of pendimethalin with six doses (0, 220, 440, 880, 1,760, and 3,520 g a.i. ha-1) on L. rigidum control in addition to crop safety in wheat, barley, and sorghum. POST application of pendimethalin was found to be safe for wheat and barley, and the biomass reduction in both crops at varied rates (220 to 3,520 g ha−1) of pendimethalin ranged from 10% to 20%. A dose–response study revealed that the pendimethalin dose required for 50% mortality and growth reduction of L. rigidum was 179 and 144 g ai ha−1, respectively. Pendimethalin at 880 g ai ha−1 completely killed late cohorts of L. rigidum. These results suggest that the POST application of pendimethalin can be used for the effective control of late cohorts of L. rigidum in wheat and barley crops. POST application of pendimethalin suppressed the biomass of sorghum plants compared with the non-treated control treatment, and biomass reduction in sorghum at different pendimethalin doses ranged from 32% to 40%. Results implied that POST applications of pendimethalin in wheat and barley can diversify herbicide programs in managing L. rigidum and may help in delaying the evolution of resistance in this weed.
1 Introduction
Annual ryegrass (Lolium rigidum Gaud.) is the most prevalent weed across the southern cropping region of Australia (Gill, 1996; Peterson et al., 2018). This weed infests a large area (~8 m ha) in Australia and is responsible for a revenue loss of approximately AU$ 93 million each year to Australian grain growers (Llewellyn et al., 2016). Lolium rigidum has evolved resistance to herbicides in multiple modes of action groups in Australia, and the additional cost involved in resistance management of L. rigidum was estimated to be AU$ 103 million annually (Llewellyn et al., 2016).
The adoption of no-till production systems has favored the infestation of L. rigidum throughout Australia (Goggin et al., 2012). Tillage and residue-burning practices for weed control were mostly eliminated in no-till production systems, and the frequent use of herbicides for weed control in these systems has led to herbicide resistance in this weed (Pearce and Holmes, 1976; Walsh et al., 2019). Currently, this weed has evolved resistance to 11 different herbicide modes of action groups, including acetyl coenzyme-A carboxylase (ACCase) inhibitors, acetolactase synthase (ALS) inhibitors, and the 5-enolpyruvylshikimate-3-phosphate (EPSP) synthase inhibitor glyphosate, and the first unique case of herbicide resistance in L. rigidum was reported from Australia (Heap, 2021). This suggests that it is the most predominant herbicide-resistant weed in Australia.
The seed production potential of L. rigidum is very high, and it has been reported that in a wheat (Triticum aestivum L.) crop, a single plant of this weed can produce 45,000 seeds m−2 (Rerkasem et al., 1980). Multiple cohorts of L. rigidum in paddocks make the control of this weed difficult, resulting in plants not being exposed to either pre-emergence (PRE) or post-emergence (POST) herbicide control in the winter season (Chauhan et al., 2006; Owen et al., 2011). Lolium rigidum seeds have innate dormancy, which prevents the complete germination of L. rigidum in the summer season (Gramshaw and Stern, 1977; Steadman et al., 2003). A reduction in wheat yield by 50% was reported with a heavy infestation (200 plants m−2) of L. rigidum compared with weed-free conditions (Wu et al., 1998). Similarly, a 40% reduction in barley (Hordeum vulgare L.) yield was reported with L. rigidum infestation of 300 plants m−2 (Lemerle et al., 1995).
With the onset of a favorable environment (autumn and start of winter seasons) for germination, 40%–60% of seeds germinate and residual viable seeds increase their infestation in succeeding winter crops by producing multiple cohorts. Australian farmers, in general, sow winter crops early to utilize the residual soil moisture, and in that scenario, late cohorts of L. rigidum after crop emergence make its management difficult as they escape from herbicide application (Gill, 1996; Chauhan et al., 2006).
Recently, it has been observed that this weed is emerging in summer seasons due to changing seasonality (Thompson et al., 2021). The occurrence of this has been noticed in the cotton (Gossypium hirsutum L.) crop of eastern Australia (Thompson and Chauhan, 2022). The introduction of glyphosate-tolerant cotton in Australia and the heavy reliance on glyphosate for weed control in fallows have resulted in the occurrence of glyphosate resistance in this weed (Pratley et al., 1996; Powles et al., 1998). In winter crops, POST herbicides (most commonly used groups 1 and 2) are not able to provide effective control of L. rigidum (Boutsalis et al., 2012). The literature suggests that PRE herbicides (e.g., dinitroaniline) are relatively more effective for L. rigidum control compared with POST herbicides (Brunton et al., 2018). However, PRE herbicides exhibit varying residual activity, consequently offering different durations of control, some of which may encompass the period of L. rigidum emergence. It was hypothesized that if PRE herbicides are to be applied as POST to crops (e.g., at the 5–6-leaf stage of crops), they may provide effective control of late emerging L. rigidum. However, information is lacking regarding the phytotoxicity in crops with the use of PRE herbicides if applied POST to crops.
Pendimethalin, a dinitroaniline herbicide, is widely used for the control of grass and broadleaf weeds in cereal crops (Vighi et al., 2017). It is mainly applied as a PRE but is sometimes also used as a POST (Vighi et al., 2017). There is no information available on the control of late emerging L. rigidum by pendimethalin if used as POST in crops and the application rates that are safe to crops, such as wheat, barley, and sorghum [Sorghum bicolor (L.) Moench]. Wheat is the most dominant winter cereal and sorghum is the most dominant summer grain crop grown in Australia. This study investigated the novel use pattern of pendimethalin and its phytotoxicity to wheat, barley, and sorghum. This study also evaluated the effect of the rate of application of pendimethalin on L. rigidum.
2 Materials and methods
The studies were conducted at the research facilities of the University of Queensland, Gatton, Australia, from 2018 to 2020. The seeds of L. rigidum used in this study were collected from Griffith, New South Wales. Appropriate permissions for the collection of weed seeds were obtained. After collection, seeds were dried in sunlight for 3 days and then stored at room temperature (25°C ± 2°C) in the Weed Science Laboratory of the Queensland Alliance for Agriculture and Food Innovation (QAAFI), The University of Queensland, Australia, until used for experimental purpose.
The first experiment on wheat (variety: Spitfire), barley (variety: Commander), and L. rigidum was conducted in April 2019, and the second experiment was conducted in April 2021. The first experiment on sorghum (variety: Sentinel IG) was conducted in March 2019, and the second experiment was conducted in August 2019. All pendimethalin treatments in each species (wheat, barley, sorghum, and L. rigidum) were evaluated in a randomized complete block design. In each experiment, there were three replications in the first experiment and four replications in the second experiment. The studies were conducted in pots (20 cm diameter and 20 cm height) filled with potting mix containing biological organic-based products and natural rock substrates (Centenary Landscape, Australia). The potting mix had a pH of 6.3 and an electrical conductivity of 1.42 dS m−1. No fertilizer was added to the plants. The pots were kept on benches and maintained in an open environment and regularly watered with an automatic sprinkler system of irrigation (two times per day). Wheat and barley were sown with 15 seeds per pot. Sorghum was planted with six seeds per pot. Lolium rigidum was sown with 20 seeds per pot, and these pots were sown 3 weeks after wheat and barley planting to simulate the emergence of late cohorts.
The wheat, barley, and sorghum were sprayed with different pendimethalin rates (0, 220, 440, 880, 1,760, and 3,520 g ai ha−1) 3 weeks after planting (5–6-leaf stage). Pots of L. rigidum were also sprayed (as PRE) during that time. As there is no post-emergent recommendation for this herbicide, the rates were chosen based on the pre-plant recommended rate (440 g ai ha−1) of pendimethalin for L. rigidum in wheat and barley. Herbicide spray was done with a research track sprayer using Teejet XR 110015 flat fan nozzles and a spray volume of 108 L ha−1 was used at 200 kPa. Pots were kept without watering for 24 h after herbicide application and thereafter regularly watered.
The mortality percentage for pendimethalin-treated plants was assessed based on a comparison with non-treated control plants. Plants were considered dead if no new growth or green tissue appeared after herbicide application. For aboveground biomass measurements, plants in each pot were cut close to the base at 28 days after treatment, oven-dried at 70°C for 72 h, and weighed by individual pots. The biomass data were converted into percent biomass reduction compared with the non-treated control (see Equation 1).
where C is the mean biomass of the non-treated control pot and D is the mean biomass of the pendimethalin-treated pot.
Data were subjected to analysis of variance (ANOVA) using Genstat (16th Edition; VSN International, Hemel Hempstead, UK). There was no interaction between the two experiments and treatments; therefore, data were pooled for the two experiments. The biomass reduction of wheat, barley, sorghum, and L. rigidum was assessed at P ≤0.05 using Fisher’s protected least significant difference (LSD) test.
Mortality and biomass reduction of L. rigidum (as a percentage compared with the non-treated control) data were regressed over herbicide treatments using a non-linear regression of a four-parameter log-logistic model (see Equation 2) using SigmaPlot 14.0 (Systat Software, San Jose, CA, USA).
Where y = mortality (%) or biomass reduction (%), y0 = bottom of the curve, a = difference between the top and bottom of the curve, x50 = dose required to kill 50% plants (LD50) or plant growth (GR50), b = slope of the curve, and x = pendimethalin dose.
3 Results and discussion
Pendimethalin effectively controlled L. rigidum. The mortality of L. rigidum at pendimethalin 220 g ai ha−1 was 62%, which increased to 88% at 440 g ai ha−1 and 100% at 880 g ha−1 (Figure 1A). A similar response was found for biomass reduction of L. rigidum with pendimethalin application. The lethal dose of pendimethalin for 50% control of L. rigidum was 179 g ai ha−1 (Figure 1A). Similarly, the pendimethalin dose required for 50% biomass reduction of L. rigidum was 144 g ai ha−1 (Figure 1B). These observations suggest that pendimethalin effectively controlled L. rigidum. No mortality in wheat, barley, and sorghum crops was observed when pendimethalin was applied at different rates as POST (21 days after planting).
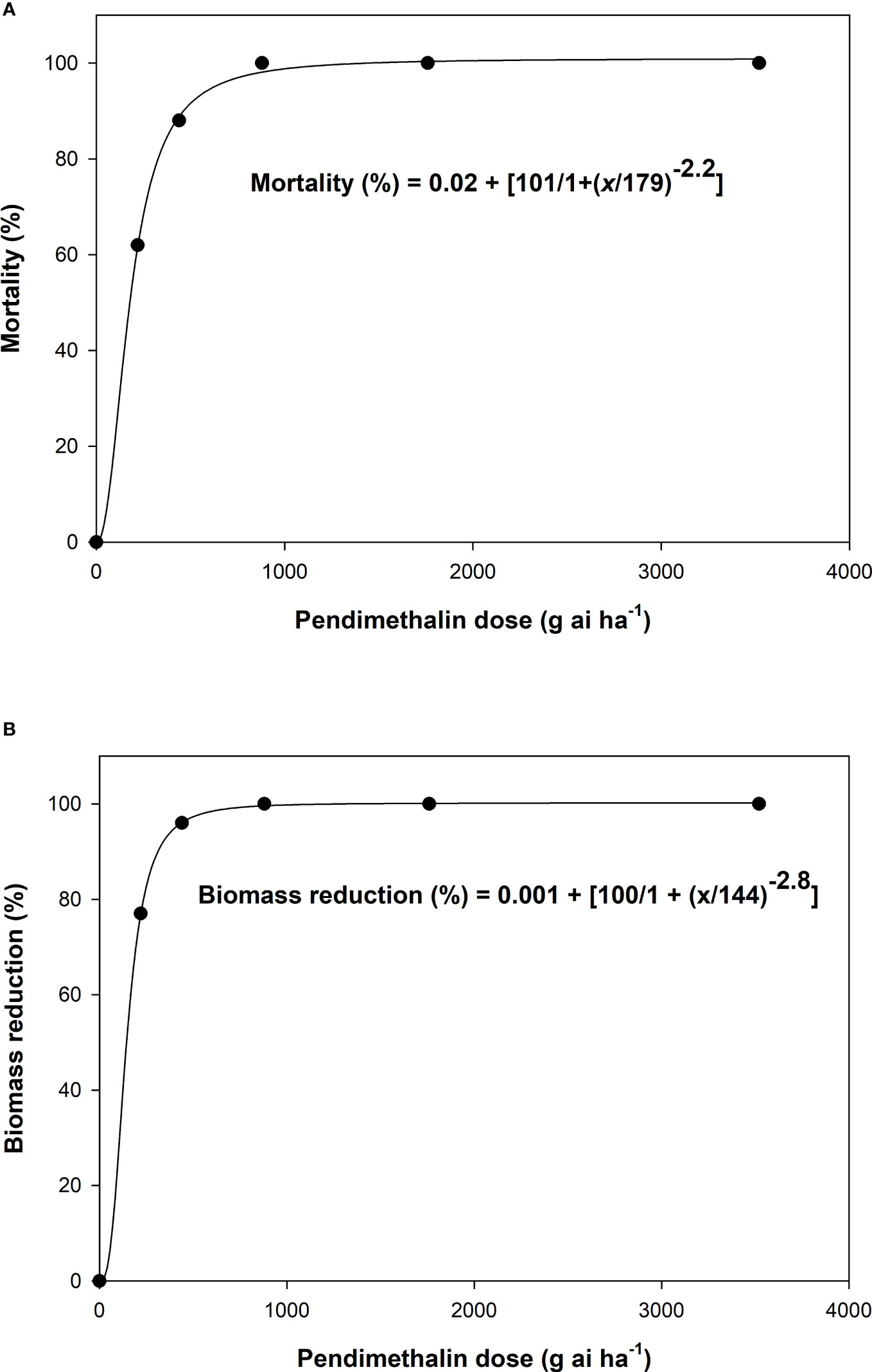
Figure 1 The dose–response curve of Lolium rigidum for (A) mortality percentage and (B) biomass reduction percentage. The curve is a log-logistic regression model fitted to the data.
In wheat and barley crops, the biomass reduction with pendimethalin doses varied from 10% to 20% (Figures 2A, B). Pendimethalin at 880 g ha−1 caused a 16% biomass reduction in both crops; at this dose, 100% control of L. rigidum was achieved. In sorghum crops, phytotoxicity of pendimethalin was observed in terms of biomass reduction (Figure 2C). Pendimethalin caused a biomass reduction of 38%–44% in sorghum and was the highest at 3,520 g ha−1
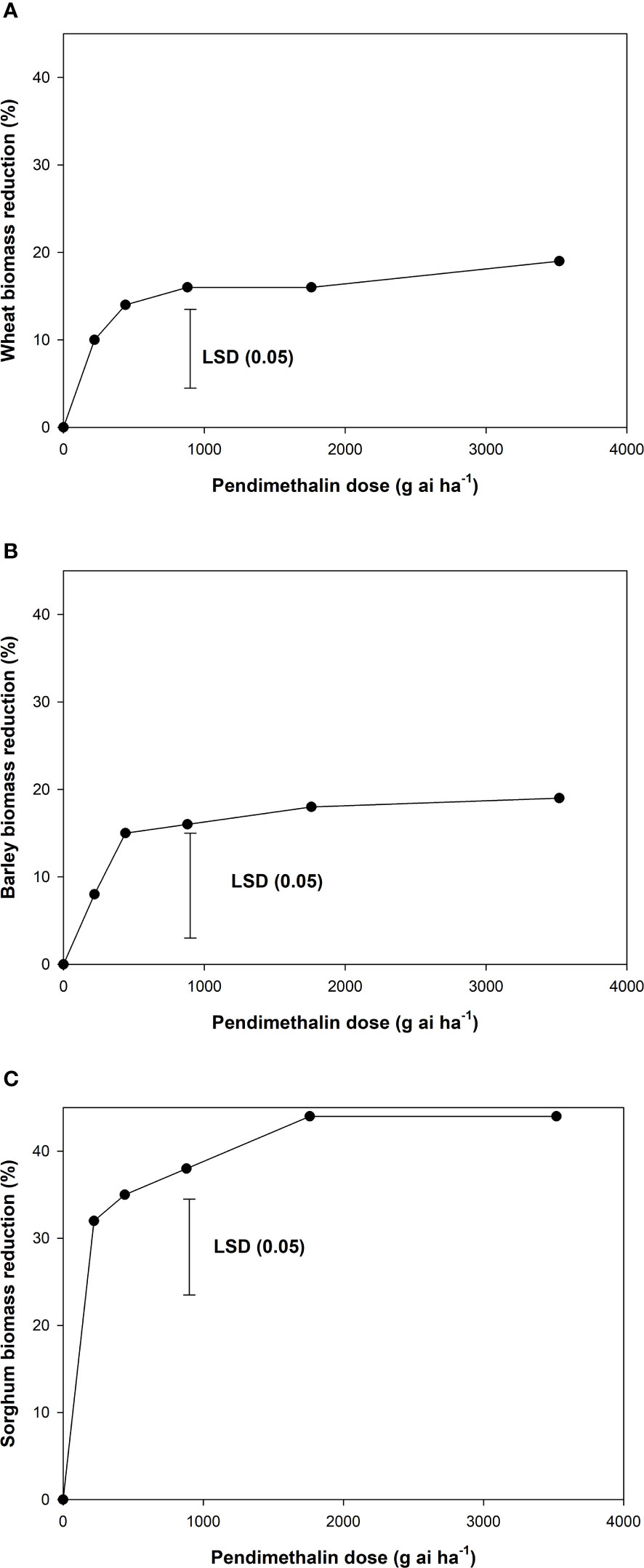
Figure 2 Biomass reduction in (A) wheat, (B) barley, and (C) sorghum crops in response to pendimethalin doses. The error bars represent the least significant difference (LSD) at a 5% level of significance.
Pendimethalin kills susceptible plants by inhibiting mitotic cell division, and tolerant species grow through by having a root system below the treated zone (Vencill, 2002). A previous study reported that a POST application of pendimethalin 1,000 g ai ha−1 at the 2-leaf stage of barley was safe for the crop and effectively controlled Italian ryegrass (Lolium multiflorum Lam.) (Alshallash, 2015).
POST application of pendimethalin is not recommended for wheat, barley, and sorghum crops in Australia. As pendimethalin POST caused major reductions in sorghum biomass, it is unlikely to be recommended in this crop. However, these results confirm that the POST application of pendimethalin is safe for wheat and barley and can provide effective control of late cohorts of L. rigidum. There was no visual injury at any rate of pendimethalin to wheat and barley. The biomass reduction in both crops was 16% at pendimethalin 880 g ai ha−1. As the crop biomass was recorded 4 weeks after pendimethalin application, little suppression was expected. This minor level of suppression is expected to recover at a late stage of the crop; however, this needs verification under field conditions. The minor suppression also needs to be tested under field conditions for a trade-off relationship between grain yield and L. rigidum control.
Glyphosate and paraquat are widely used herbicides for L. rigidum control in fallows or as pre-plant control in crops. Under crop situations, ACCase- and ALS-inhibiting herbicides are mostly used for the control of L. rigidum (Owen et al., 2011; Loureiro et al., 2017). However, widespread resistance of L. rigidum to these herbicides in the no-till system has shifted the herbicides’ pattern toward PRE herbicides (Boutsalis et al., 2014). During the last decade, farmers have diverted their attention to sowing winter crops early to avail the opportunity of residual soil moisture (Anderson et al., 2005). In that situation, late germinating L. rigidum plants resistant to ACCase- and ALS-inhibiting herbicides make weed management complicated. PRE herbicides can be used in that situation; however, limited residual activity for a certain period of these herbicides is a crucial factor to provide season-long weed control. It has been reported that the surface-applied half-life of pendimethalin may occur in 4–6 days due to photochemical, volatilization, and other degradation processes (Savage and Jordan, 1980; Vencill, 2002). Under these circumstances, a POST application of pendimethalin could be another strategy for season-long L. rigidum control; therefore, registration of pendimethalin as POST in these crops needs to be considered. The risk of resistance increases if pendimethalin is allowed to be used twice in one season or as a POST with no follow-up control of survivors.
Overreliance on ACCase and ALS inhibitor herbicides has led to resistant populations as these herbicides create a very high selection pressure (Powles et al., 1998; Saini et al., 2016). Moreover, the continuous use of a herbicide with a single mode of action could lead to the evolution of resistance to that specific herbicide, necessitating the use of a herbicide with another mode of action in the herbicide rotation program (Jasieniuk et al., 1996). Mixing and rotating herbicides with different modes of action is a useful strategy to slow down the evolution of resistance (Vogwill et al., 2012; Lagator et al., 2013). Hence, diversifying weed control options with the use of pendimethalin as a POST could provide sustainable control of L. rigidum.
This research was conducted in pots; therefore, further testing of pendimethalin POST for crop safety is required under field conditions. It is important to assess the trade-off relationship between crop suppression due to the POST application of pendimethalin and grain yield under field situations and various soil and climatic conditions for robust recommendations. There is also a need to assess the yield losses in different crops with late cohorts of L. rigidum. We exclusively utilized one variety per crop species. Hence, future studies should encompass multiple varieties to ascertain whether there exists a variety-specific response to the POST application of pendimethalin.
In conclusion, the POST application of pendimethalin (880 g ai ha−1) can be successfully used in wheat and barley and could provide effective control of late cohorts of L. rigidum. Further information is required regarding the environmental impact of POST-application pendimethalin, its potential for soil pollution reduction, the absence of herbicide residues in grains, and its impact on beneficial soil microorganisms. Additionally, investigation is needed to determine the viability of tank mixing pendimethalin with group 1 or 2 herbicides for season-long, broad-spectrum control of L. rigidum in wheat and barley. Cost-effectiveness assessments are necessary to evaluate if these combinations can control emerged weeds with POST herbicides while utilizing pendimethalin for residual activity against late-germinating L. rigidum. Moreover, testing the effectiveness of POST-application pendimethalin in combination with non-selective herbicides like glufosinate, glyphosate, and paraquat for the season-long control of L. rigidum in fallow areas and along fence lines is also imperative.
Data availability statement
The original contributions presented in the study are included in the article. Further inquiries can be directed to the corresponding author.
Author contributions
GM: Formal analysis, Investigation, Methodology, Writing – original draft. BC: Conceptualization, Funding acquisition, Investigation, Methodology, Project administration, Writing – review & editing.
Funding
The author(s) declare that no financial support was received for the research, authorship, and/or publication of this article.
Conflict of interest
The authors declare that the research was conducted in the absence of any commercial or financial relationships that could be construed as a potential conflict of interest.
The author(s) declared that they were an editorial board member of Frontiers, at the time of submission. This had no impact on the peer review process and the final decision.
Publisher’s note
All claims expressed in this article are solely those of the authors and do not necessarily represent those of their affiliated organizations, or those of the publisher, the editors and the reviewers. Any product that may be evaluated in this article, or claim that may be made by its manufacturer, is not guaranteed or endorsed by the publisher.
References
Alshallash K. S. (2015). Assessment of pre emergence herbicides (chlorotoluron, isoproturon and methabenzthiazuron) and post emergence herbicides (diclofop-methyl and pendimethan) in controlling Italian rye-grass (Lolium multiflorum) growing with barley (Hordeum vulgare). J.Appl Sci. Res. 11, 1–5. Available at: https://www.researchgate.net/publication/342693576
Anderson W. K., Hamza M. A., Sharma D. L., D’Antuono M. F., Hoyle F. C., Hill N., et al. (2005). The role of management in yield improvement of the wheat crop a review with special emphasis on Western Australia. Aust. J. Agric. Res. 56, 1137–1149. doi: 10.1071/AR05077
Boutsalis P., Gill G. S., Preston C. (2012). Incidence of herbicide resistance in rigid ryegrass (Lolium rigidum) across southeastern Australia. Weed Technol. 26, 391–398. doi: 10.1614/WT-D-11-00150.1
Boutsalis P., Gill G. S., Preston C. (2014). Control of rigid ryegrass in Australian wheat production with pyroxasulfone. Weed Technol. 28, 332–339. doi: 10.1614/WT-D-13-00094.1
Brunton D. J., Boutsalis P., Gill G., Preston C. (2018). Resistance to multiple PRE herbicides in a field-evolved rigid ryegrass (Lolium rigidum) population. Weed Sci. 66, 581–585. doi: 10.1017/wsc.2018.31
Chauhan B. S., Preston C., Gill G. (2006). Influence of tillage systems on vertical distribution, seeding recruitment and persistence of rigid ryegrass (Lolium rigidum) seed bank. Weed Sci. 54, 669–676. doi: 10.1614/WS-05-184R.1
Gill G. S. (1996). Why annual ryegrass is a problem in Australian agriculture. Plant Prot. Q. 11, 193–195. Available at: https://caws.org.nz/PPQ1112/PPQ%2011-S1%20pp193-195%20Gill.pdf
Goggin D. E., Powles S. B., Steadman K. J. (2012). Understanding Lolium rigidum seeds: The key to managing a problem weed? Agron 2, 222–239. doi: 10.3390/agronomy2030222
Gramshaw D., Stern W. R. (1977). Survival of annual ryegrass (Lolium rigidum Gaud.) in a Mediterranean type environment. I. Effect of summer grazing by sheep on seed numbers and seed germination in autumn. Aust. J. Agr Res. 28, 81–91. doi: 10.1071/AR9770081
Heap I. (2021) The International Herbicide-Resistant Weed Database. Available at: http://www.weedscience.org (Accessed 13 August 2021).
Jasieniuk M., Brule-Babel A. L., Morrison I. N. (1996). The evolution and genetics of herbicide resistance in weeds. Weed Sci. 44, 176–193. doi: 10.1017/S0043174500093747
Lagator M., Vogwill T., Mead A., Colegrave. N., Neve P. (2013). Herbicide mixtures at high doses slow the evolution of resistance in experimentally evolving populations of Chlamydomonas Reinhardtii. New Phytol. 198, 938–945. doi: 10.1111/nph.12195
Lemerle D., Verbeek B., Coombes N. (1995). Losses in grain yield of winter crops from Lolium rigidum competition depend on crop species, cultivar and season. Weed Res. 35, 503–509. doi: 10.1111/j.1365-3180.1995.tb01648.x
Llewellyn R. S., Ronning D., Ouzman J., Walker S., Mayfield A., Clarke M. (2016). Impact of Weeds on Australian Grain Production: the cost of weeds to Australian grain growers and the adoption of weed management and tillage practices Report for GRDC (Canberra, Australia: CSIRO).
Loureiro I., Escorial M. C., Hernández-Plaza E., González-Andújar J. L., Chueca M. C. (2017). Current status in herbicide resistance in Lolium rigidum in winter cereal fields in Spain: Evolution of resistance 12 years after. Crop Prot. 102, 10–18. doi: 10.1016/j.cropro.2017.08.001
Owen M. J., Michael P. J., Renton M., Steadman K. J., Powles S. B. (2011). Towards large-scale prediction of Lolium rigidum emergence. I. Can climate be used to predict dormancy parameters? Weed Res. 51, 123–132. doi: 10.1111/j.1365-3180.2010.00832.x
Pearce G. A., Holmes J. (1976). The control of annual ryegrass. J. Dep. Agric. West Aust. Ser. 17, 77–81. Available at: https://www.cabdirect.org/cabdirect/abstract/19770763428
Peterson M. A., Collavo A., Ovejero R., Shivrain V., Walsh M. J. (2018). The challenge of herbicide resistance around the world: A current summary. Pest Manage. Sci. 74, 2246–2259. doi: 10.1002/ps.4821
Powles S. B., Lorraine-Colwill D. F., Dellow J. J., Preston C. (1998). Evolved resistance to glyphosate in rigid ryegrass (Lolium rigidum) in Australia. Weed Sci. 46, 604–607. doi: 10.1017/S0043174500091165
Pratley J., Baines P., Eberbach P., Incerti M., Broster J. C. (1996). “Glyphosate resistance in annual ryegrass,” in Proceedings of the 11th Annual Conference of the Grassland Society of New South Wales, (Wagga Wagga, Australia: The Grassland Society of NSW), 10–11 July 1996. 122.
Rerkasem K., Stern W. R., Goodchild N. A. (1980). Associated growth of wheat and annual ryegrass. I. Effect of varying total density and proportion in mixtures of wheat and annual ryegrass. Aus J. Agric. Res. 31, 649–658. doi: 10.1071/AR9800649
Saini R. K., Kleemann S. G., Preston C., Gill G. S. (2016). Alternative herbicides for the control of clethodim-resistant rigid ryegrass (Lolium rigidum) in Clearfield canola in Southern Australia. Weed Technol. 30, 423–430. doi: 10.1614/WT-D-15-00078.1
Savage K. E., Jordan T. N. (1980). Persistence of three dinitroaniline herbicides on the soil surface. Weed Sci. 28, 105–110. doi: 10.1017/S0043174500027879
Steadman K. J., Bignell G. P., Ellery A. J. (2003). Field assessment of thermal after-ripening time for dormancy release prediction in Lolium rigidum seeds. Weed Res. 43, 458–465. doi: 10.1046/j.0043-1737.2003.00363.x
Thompson M., Chauhan B. S. (2022). Changing seasonality of Lolium rigidum (annual ryegrass) in southeastern Australia. Front. Agron. 4. doi: 10.3389/fagro.2022.897361
Thompson M., Mahajan G., Chauhan B. S. (2021). Seed germination ecology of southeastern Australian rigid ryegrass (Lolium rigidum) populations. Weed Sci. 69, 454–460. doi: 10.1017/wsc.2021.36
Vencill W. K. (2002). Herbicide handbook (No. Ed. 8). Weed Science Society of America Herbicide Handbook. (Lawrence: Weed Science Society of America)
Vighi M., Matthies M., Solomon K. R. (2017). Critical assessment of pendimethalin in terms of persistence, bioaccumulation, toxicity and potential for long-range transport. J. Toxicol. Environ. Health Sci. Part B. 20, 1–21. doi: 10.1080/10937404.2016.1222320
Vogwill T., Lagator M., Colegrave N., Neve P. (2012). The experimental evolution of herbicide resistance in Chlamydomonas reinhardtii results in a positive correlation between fitness in the presence and absence of herbicides. J. Evol. Biol. 25, 1955–1964. doi: 10.1111/j.1420-9101.2012.02558.x
Walsh M. J., Broster J., Chauhan B., Rebetzke G. J., Pratley J. (2019). “Weed control in cropping systems–past lessons and future opportunities,” in Australian agriculture in 2020: From Conservation to Automation (Wagga Wagga, Australia: Agronomy Australia and Charles Sturt University), 153–172.
Wu H., Pratley J., Lemerle D., Haig T., Verbeek B. (1998). Differential allelopathic potential among wheat accessions to annual ryegrass, Proceedings of the 9th Australian Agronomy Conference, Wagga Wagga, Australia. Aust. Soc. Agron., 567–571. Available at: http://www.regional.org.au/au/asa/1998/6/139wu.htm
Keywords: barley, crop suppression, GR50, LD50, mortality, wheat, sorghum
Citation: Mahajan G and Chauhan BS (2024) Novel use pattern of pendimethalin for annual ryegrass (Lolium rigidum Gaudin) control in wheat and barley. Front. Agron. 5:1341880. doi: 10.3389/fagro.2023.1341880
Received: 21 November 2023; Accepted: 19 December 2023;
Published: 11 January 2024.
Edited by:
Baruch Rubin, Hebrew University of Jerusalem, IsraelReviewed by:
Candelario Palma-Bautista, University of Cordoba, SpainStephen Christopher Marble, University of Florida, United States
John Broster, Charles Sturt University, Australia
Copyright © 2024 Mahajan and Chauhan. This is an open-access article distributed under the terms of the Creative Commons Attribution License (CC BY). The use, distribution or reproduction in other forums is permitted, provided the original author(s) and the copyright owner(s) are credited and that the original publication in this journal is cited, in accordance with accepted academic practice. No use, distribution or reproduction is permitted which does not comply with these terms.
*Correspondence: Bhagirath S. Chauhan, b.chauhan@uq.edu.au