- 1Chacra Experimental Integrada Barrow, Ministerio de Desarrollo Agrario-Instituto Nacional de Tecnología Agropecuaria (MDA-INTA), Tres Arroyos, Buenos Aires, Argentina
- 2Departamento de Agronomía, Universidad Nacional del Sur, Bahía Blanca, Buenos Aires, Argentina
- 3Centro de Recursos Naturales Renovables de la Zona Semiárida (CERZOS), Consejo Nacional de Investigaciones Científicas y Técnicas (CONICET), Bahía Blanca, Buenos Aires, Argentina
- 4Laboratory of Biotechnology and Plant Genetics, Consejo Nacional de Investigaciones Científicas y Técnicas (CONICET), Tres Arroyos, Buenos Aires, Argentina
- 5Facultad de Agronomía, Universidad Nacional de La Pampa, Santa Rosa, Argentina
Cover crops (CC) are increasingly used worldwide within integrated weed management scenarios. They are usually established between two commercial crops and are not harvested, grazed, or incorporated into the soil, but remain on the surface during their growth cycle. The aim of this work was to determine the performance of different CC mixtures and their effects on weed suppression in the south-central region of Buenos Aires province, Argentina. Field experiments were carried out in 2019 and 2020 at the CEI Barrow (MDA-INTA, Tres Arroyos), where both binary and ternary CC mixtures were evaluated. Binary mixtures consisted of winter cereals (Avena sativa, Secale cereale) and legumes (Vicia villosa, Vicia sativa) while ternary mixtures were obtained by combining binary mixtures with canola (Brassica napus). Weed emergence counting was performed on a 14- day basis to determine weed total density. Vegetation cover of the CC mixtures as well as biomass production from crops and weeds were estimated. The specific composition of the mixtures showed a greater influence on vegetation cover than on biomass production, which would depend mainly on the prevailing environmental conditions. The type of vetch used, the addition of canola, as well as, the proportion of cereals in the mixture determined the CC effect on weeds. CC were consistently more effective in suppressing weed biomass than seedling emergence density. However, weed seedling suppression by CC was similar to or even greater than the chemical-based control (p<0.0001). The average biomass of weeds in all CC (pooled data) was highly reduced when compared to the weedy control (12 vs 259 g m-2, p<0.001), and similar responses were obtained when compared to the chemical fallow (8 g m-2). The mixture S. cereal+ V. villosa+ canola stood out for presenting the highest values of productivity and vegetation cover and high weed suppression. These results provide support for the choice of CC mixtures in a dry sub-humid area of Argentina, aimed at maximizing interference with weeds and also to demonstrate their benefits in short- and long-term management. Therefore, CC implementation within crop sequences should be considered as a complementary tool contributing to the development of more sustainable management strategies.
1 Introduction
Given the need to comprehensively address the problem of weeds in agricultural systems, cover crops (CC) are a useful tool that could be included within integrated weed management (IWM) program. CC are usually established between two commercial crops (Reeves, 1994) and non-harvested, grazed, or incorporated into the soil, but remain on the surface during their growth cycle to improve soil fertility and enhance yields (Ruffo and Parsons, 2004; Scavo et al., 2022). There is a growing interest by farmers and researchers in the adoption of diverse CC mixtures (Groff, 2008; Wortman et al., 2013) considering their ability to offer multiple ecosystem services within cropping systems (Brainard et al., 2011). CC diversification has potential to improve weed management (MacLaren et al., 2019), resulting in a key mechanism to facilitate the transition to the “Agroecological Crop Protection” approach, which promotes the reduction of pest impacts through crop management practices compatible with healthy agricultural and food systems, agroecological principles, and the “one health” concept (Deguine et al., 2023). However, their potential suppressive effect on weeds depends both on the number of species as well as their combination within the mixture (Finney and Kaye, 2017; Suter et al., 2017; Baraibar et al., 2018). So far the use of diverse CC mixtures is relatively incipient and the experimental results are both scarce and inconsistent (Davis et al., 2016; Holmes et al., 2017). Therefore, it is necessary to develop empirical evidence to understand how CC interferes with weeds (Florence et al., 2019). Binary mixtures (BM) (i.e., those formed by two botanical families mainly legumes and grasses) are commonly used as CC due to their high resource efficiency compared to other species or functional groups combinations (Dhima et al., 2007; Hayden et al., 2014). Their suppressive effect on weeds was reported by Akemo et al. (2000) and Hayden et al. (2012). Ternary mixtures (TM) (i.e., formed by three botanical families) may confer additional benefits associated with each component, where the most common include grasses, legumes, and cruciferous. The latter has been less explored concerning weed control (Haramoto and Gallandt, 2004; Björkman et al., 2015; Lorin et al., 2015). Holmes et al. (2017) determined that the exclusion of cruciferous in TM generated an increase in weed biomass, given mainly by their high productivity under the prevailing conditions of interspecific competition. Conversely, Mesbah et al. (2019) observed no differences in weed biomass between both types of mixtures. Therefore, benefits offered by multi-species conjugation versus BM are often considered inconsistent or eventually site-specific regarding weed suppression (Schonbeck et al., 2017).
CC suppress weeds by competition (Ngouajio and Mennan, 2005; Holmes et al., 2017), selective allelopathic activity (Weston, 1996), and physical interference (den Hollander et al., 2007). The allelopathic effect can be species-specific (Norsworthy et al., 2007) and have been reported mainly in grasses such as rye and oats (Kato-Noguchi et al., 1994; Schulz et al., 2013). Therefore, a combination of allelopathic CC might be more effective for a wide range of weeds (Creamer and Stinner, 1997; Wortman et al., 2013). Moreover, since the suppressive effect exerted by CC involves a combination of mechanisms, their suppressive effect on weed would likely depend on the CC specific composition, as well as on the site-specific environmental conditions, cultural practices, and the weed community present (Liebman and Dyck, 1993; Hayden et al., 2012; Baraibar et al., 2018). Therefore, species mixtures are expected to exhibit diverse and complementary suppression mechanisms (physical and chemical) (Baraibar et al., 2017; Schappert et al., 2019), which would increase the suppression capacity (Brainard et al., 2011; Schipanski et al., 2014; Finney et al., 2016).
CC biomass production is often used as an indicator of the capacity to suppress weeds (Brennan and Smith, 2005; Wayman et al., 2015) due to competition for resources (Finney et al., 2016). Also, successful and highly productive CC need a fast initial growth to reach the highest leaf area index to maximize solar radiation interception (Elhakeem et al., 2021). This is why the vegetation cover is used as a parameter to evaluate weed suppression as it correlates negatively with the dry weight of the weeds (Kruidhof et al., 2008; Uchino et al., 2011). The information about the quantity of biomass produced by a species mixture, specifically about to the contribution of each species to the total biomass is scarce (Davis et al., 2016), since many species have been evaluated in monocultures (Holmes et al., 2017). This is why the development of mixtures that favor beneficial interactions for the control of spontaneous vegetation is of great relevance for the design and reproduction of sustainable cropping systems (Brooker et al., 2021). It is also unclear whether the high CC productivity of grasses and legumes can be further improved by including additional functional groups based on eco-physiological traits that show another pattern of productivity in time and space, such as cruciferous (Cong et al., 2018). In addition, studies on species interactions can be useful for a better understanding of the process and consequently improve biomass production (Wendling et al., 2017). In CC mixtures it would be expected that the diversity in the form of growth would allow to creation of a more complete canopy cover to restrict the availability of light for weeds: grasses and cruciferous grow upright, while legumes make them prostrate or extended (MacLaren et al., 2019). The spatial and temporal complementarity of biomass production between CC components can be a useful tool to increase efficiency in the capture of resources to the detriment of weeds (Döring et al., 2012; Finn et al., 2013). Grasses and cruciferous tend to suppress effectively through rapid growth and high biomass production (Brennan and Smith, 2005; Brainard et al., 2011; Hayden et al., 2012; Dorn et al., 2015; Finney et al., 2016), while legumes grow more slowly and are less competitive (Hayden et al., 2012; Lawson et al., 2015).
In Argentina, the lines of research related to the effects of CC on the physicochemical properties of the soil focused mainly in the availability of nitrogen and/or water (Capurro et al., 2012; Restovich et al., 2012; Cazorla et al., 2012; Vanzolini et al., 2013). Whereas, its effect as part of the IWM has been addressed more recently and to a lesser extent, addressing weed communities present at a specific time of the cycle or in the residues mainly during the onset of commercial crops (Baigorria et al., 2013; Miranda et al., 2014; Acciaresi et al., 2016; Kahl et al., 2016; Lobos et al., 2019). Few approaches characterize weed emergence dynamics throughout the CC cycle to evaluate the suppressive effect in different stages (Buratovich and Acciaresi, 2019). It is essential to determine the dynamics of weeding through the diversity of species and their abundance for a better understanding of the processes that regulate crop-weed interactions, thus facilitating the incorporation of IWM-based practices (Buratovich and Acciaresi, 2017). Likewise, the latest ReTTA (ReTTA Relevamiento de Tecnología Aplicada, 2021) determined that the use of CC in Argentina quintupled in the last 5 years and, that this greater implementation was mainly based on the search for a solution against weeds that are difficult to control.
Based on the previous statements, it is necessary to develop further studies to understand how CC influences weed suppression. Novel information is required to support the choice of the best CC species for weed management and to increase knowledge of the behavior of mixtures under variable environmental conditions.
The objective of this contribution was to study the performance of different CC mixtures (productivity and vegetation cover) on weed suppression during two successive periods in the south-central region of Buenos Aires, Argentina. The hypothesis were that (1) the specific composition of the CC mixtures affects the productivity and vegetation cover; (2) binary and ternary mixtures studied as CC interfere with both seedling emergence dynamics and growth of autumn-winter-spring (A-W-S) weeds; (3) the level of weed suppression by CC mixtures is influenced by the level of biomass production and plant cover generated.
2 Materials and methods
2.1 Experimental design
Field experiments were carried out during 2019 and 2020 at the Chacra Experimental Integrada (CEI) Barrow (Tres Arroyos, Buenos Aires, Argentina; 38° 20” S; 60° 13” W).
CC binary mixtures (BM) consisted of winter cereals (Avena sativa or Secale cereale) with legumes (Vicia villosa or Vicia sativa), while ternary mixtures (TM) combined BM with canola (Brassica napus) (Table 1). Sowing rates were determined based on previous works (see Hayden et al., 2014; Baraibar et al., 2017; Finney and Kaye, 2017; Holmes et al., 2017), along with the contributions and recommendations of seed suppliers, producers, and researchers of the region. Since early sowing is pointed out by other authors (Baigorria et al., 2011; Baraibar et al., 2018) as the most recommended practice to maximize biomass production, the CC were seeded on March 21th (2019) and March 16th (2020). Before planting, all legumes were treated with Rhizobium leguminosarum biovar viciae at a dose of 200 cm3 50 kg-1. Two control treatments were used as reference: weedy plots (W, without control) and chemical fallow (CF, with non-selective herbicide). In the latter, two glyphosate LS 60% (1.8 L ha-1) applications were performed at different times depending on the composition of the weed community and the relative abundance of each species.
In the entire trial area for the two study years, the predecessor crop was wheat (Triticum aestivum), which was harvested in December to simulate a sequence of crops typical of the region under study. In 2019, crop sowing was carried out under conventional tillage using a disc harrow and a field cultivator. In 2020 a non-tillage system was applied and fallow consisted of an application of glyphosate LS 60% (1.8 L ha-1) days before the sowing. The sowing depth was calibrated to 1-2 cm, as it is a recommended value for both large and small seeds (Murrell et al., 2017). The distance between furrows was 20 cm and the planting density was variable depending on the type of mixtures (Table 1). A completely randomized block design with four replicates was used. The experimental units (EU) consisted of 3 m wide by 6 m long plots (18 m-2). The evaluated treatments consisted of eight CC mixtures and two controls. Conforming a total of 40 with a 720 m-2 net experimental area (Figure 1).
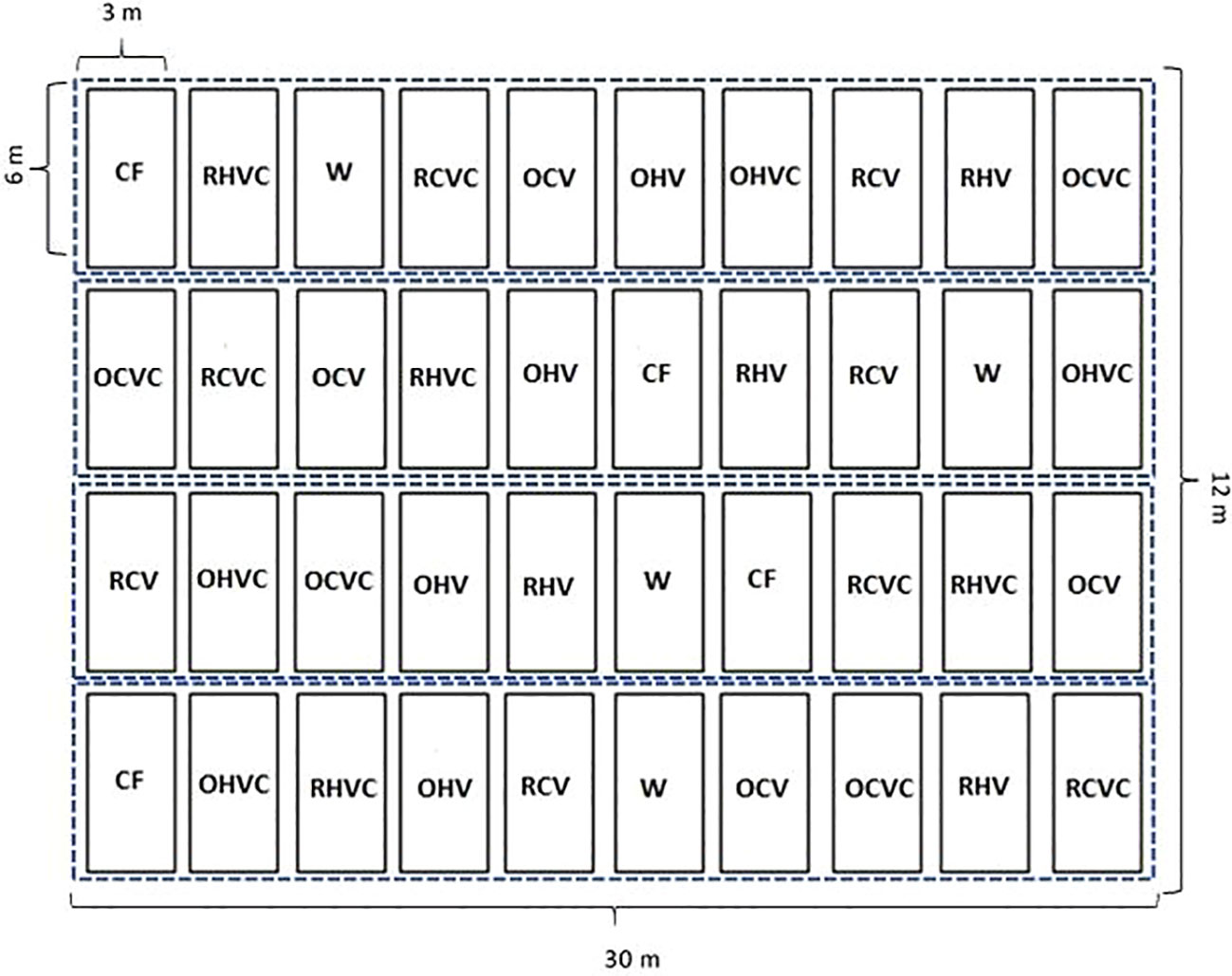
Figure 1 Experimental design in complete randomized blocks with four replicates (indicated by the blue dotted line) with the measurements of each experimental units and approximate measurements of the entire test (not counting borders and paths). The experimental units are represented and the acronyms indicate randomly assigned treatments in each block: CF (Chemical fallow), W (Weedy fallow), OHV (Oats+ Hairy vetch), OCV (Oats+ Common vetch), RHV (Rye+ Hairy vetch), RCV (Rye+ Common vetch), OHVC (Oats+ Hairy vetch+ Canola), OCVC (Oats+ Common vetch+ Canola), RHVC (Rye+ Hairy vetch+ Canola), RCVC (Rye+ Common vetch+ Canola).
According to Soil Taxonomy (USDA, 1975), soil belongs to a “Tres Arroyos” series, with original material based on loess sediments and classified as Paleudol petrocalcic. These soils are characterized by having a horizon profile: Ap/A (0-22cm) and BA (22-32 cm) loam-clay-sandy with subangular block structure, Btn (32-75 cm) with a clayey texture and coarse prism structure. At 75 cm is the petrocalcic horizon (INTA, 2014). Chemical soil analyses at a depth of 0-20 cm, for both years at the study site before sowing, indicated adequate conditions for the correct development of the crops: acidic pH, medium to high values of organic matter and phosphorus, although with a low nitrate content (Table 2).
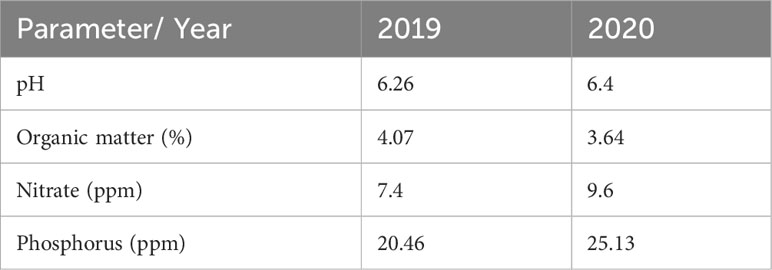
Table 2 Results of the chemical analysis of soil (pH, organic matter, nitrates and phosphorus) for the year 2019 and 2020.
2.2 Meteorological data
The study area is characterized by a dry sub-humid water regime with an average annual total rainfall of 757.8 millimeters (1938-2014 series), being spring and part of autumn the most rainy seasons, while winter the driest. The average annual temperature is 14.9°C, with the warmest month being January and the coldest July (Borda, 2016). Weather data in the study were recorded at the meteorological station of CEI Barrow located in the same experimental site (38° 20” S; 60° 13” W). In 2020, the total rainfall recorded during the CC cycle was 54% higher than in 2019, the latter showing a deficit of 109 mm compared to historical values. Likewise, the latter showed a longer period of minimum temperatures below the historical value (March, July, and September) and earlier occurrence of frosts in the cycle, compared to the year 2020 (Table 3).
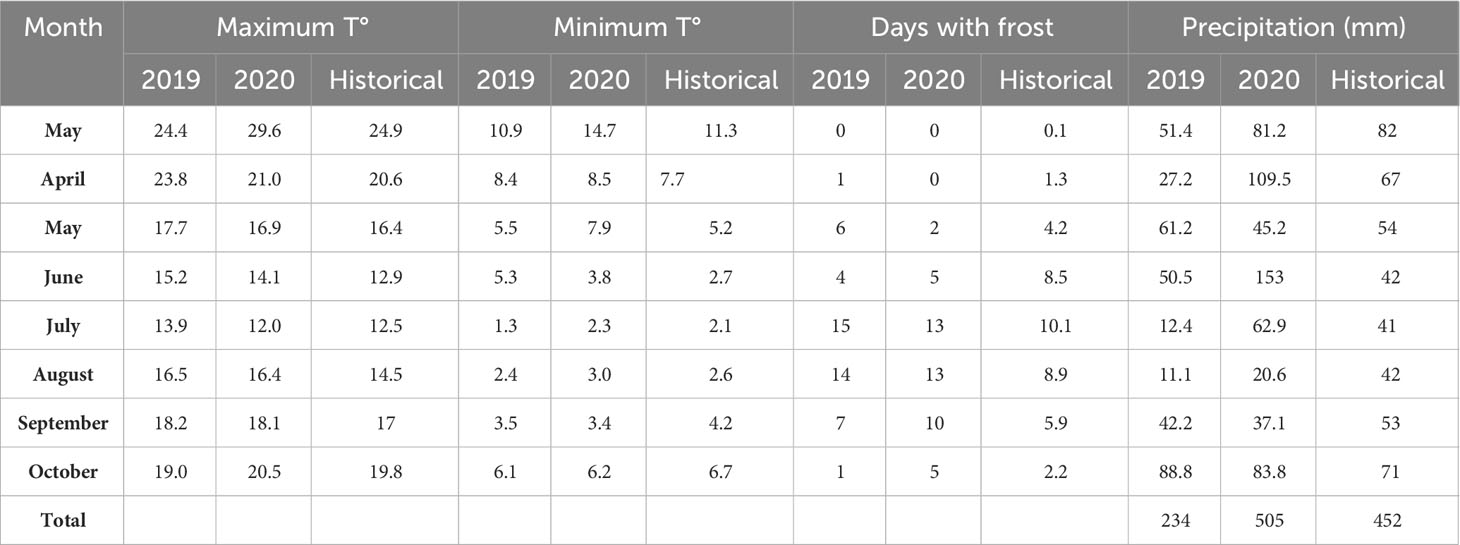
Table 3 Record of average maximum and minimum temperature (T°), days with frost and precipitation (mm) during the CC cycle for the years 2019, 2020 and the historical average of the area (1939-2019 series).
2.3 Data collection
To evaluate the first hypothesis, in the middle of October, vegetation cover percentages (% VC) of CC mixtures were estimated by taking three digital photographs (0.25 m2 each) per EU. The total number of photographs taken was 120, further processed with the CobCal v 2.1 software. Biomass of both CC mixtures and weeds was determined by harvesting the aerial vegetation during mid-spring present in 0.5 m2, resulting from the sum of the biomass contained in 2 quadrats of 0.25 m2 randomly distributed in each EU. Aerial dry biomass values of the different components of the mixtures were obtained after oven drying at 65°C for a week.
To test the second hypothesis, both biomass and density of weed individuals were evaluated. In both years, weekly destructive seedling counts of autumn-winter-spring (A-W-S) species were performed in randomly distributed 0.25 m-2 frames (n=4). Total density (pl m-2) was determined as the sum of seedlings that emerged throughout the CC cycle. In order to characterize the weeds surveyed, the abundance (%) and average frequency of each weed species were assessed. The total number of frames evaluated was 40, one for each EU. Data were represented for those species with abundance or frequency ≥10%.
2.4 Statistical analysis
An analysis of variance (ANOVA) was performed to evaluate the effect of the CC treatments on each study variable. Data were transformed to improve homoscedasticity if necessary. Fisher’s least significant difference test (p≤ 0.05) was used for mean comparison. Statistical analysis was performed using Infostat® software (Di Rienzo et al., 2014).
To evaluate the third hypothesis, a linear regression analysis was performed between each variable measured on the CC (vegetation cover and dry biomass) and the variables of the weed (dry biomass and density).
For all the studied parameters, scatter plots of the observed vs predicted residues were analyzed to assess compliance with the model’s assumptions (normality, homoscedasticity, and independence). Residual plots indicated that the variances were normally distributed and homogenous. At this point, the total emergence density (pl m-2) and the emergence 85 days after planting (DAP) in 2020 of A-W-S and dicotyledons weed species data, were transformed into a log(x) and square root (x+1) to comply with homoscedasticity of variance and normality of data.
3 Results
3.1 Biomass production and vegetation cover of CC mixtures
The average biomass production of the CC was 39% higher in 2020 compared to 2019 (9360 vs. 5678 kg ha-1) (Figure 2). In 2019, aboveground biomass was 54% higher in the TM formed by oats or rye + hairy vetch compared to rye or oats + common vetch (p=0.0006) (Figure 2A). Likewise, hairy vetch showed higher productivity compared to common vetch in all mixtures (p=0.0114). In 2020, no differences were observed in biomass production between CC treatments (Figure 2B). However, it is important to note that rye or oats + hairy vetch were among the mixtures with the highest biomass production in both years, with average values of 6168 and 9551 kg ha-1.
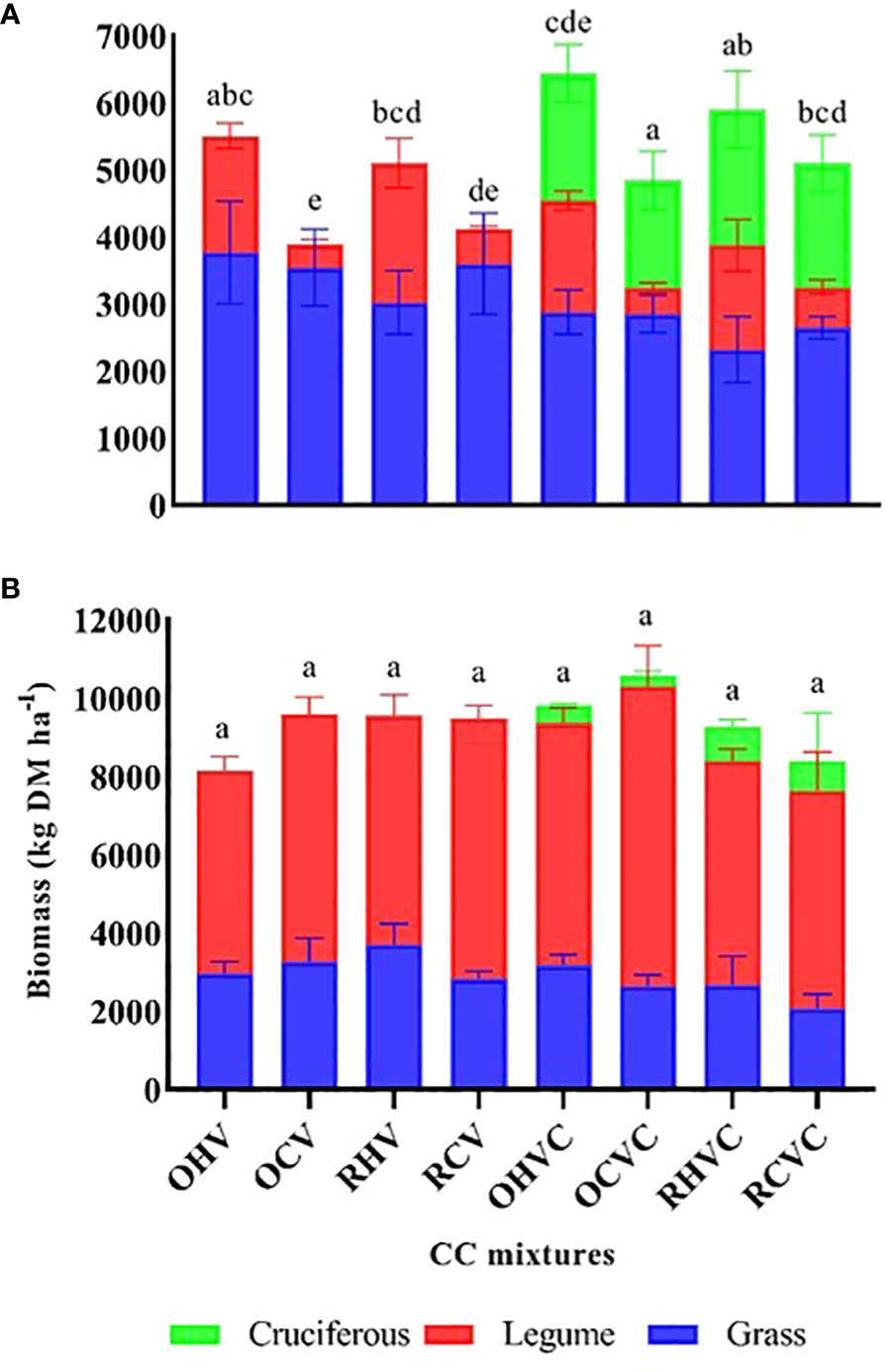
Figure 2 Production of total aerial biomass (kg DM ha-1) of the different CC mixtures and each component: grass (in blue), legume (in red) and cruciferous (in green), for the years 2019 (A) and 2020 (B) in different treatments: OHV (Oats+ Hairy vetch), OCV (Oats+ Common vetch), RHV (Rye+ Hairy vetch), RCV (Rye+ Common vetch), OHVC (Oats+ Hairy vetch+ Canola), OCVC (Oats+ Common vetch+ Canola), RHVC (Rye+ Hairy vetch+ Canola), RCVC (Rye+ Common vetch+ Canola). The bars represent average values and different letters indicate significant differences among treatments in Fisher’s LSD test (p<0.05).
Regarding the composition of the mixtures, a negative relationship (p<0.0001) was found between the percentage of legume and grass (r= -0.71) of the mixture (Figures 3A, C). The contribution of each component to the total biomass varied between years. In 2019, the proportion of grasses was higher, but mixtures with hairy vetch were balanced, while in 2020 legumes were dominant. In 2019, the incorporation of canola in the BM negatively affected the biomass of both grasses and legumes (Figures 2A, B), although in the joint balance, the contributions of aerial biomass of canola exceeded this depression. A negative relationship (p=0.005) was established between the % of grass and the % of canola (r=-0.48) in the mixtures (Figure 3B). In contrast, canola contributed very little biomass to the TM in 2020, which could be due to establishment failures caused by hare damage that reduced the plant stand at the beginning of the cycle.
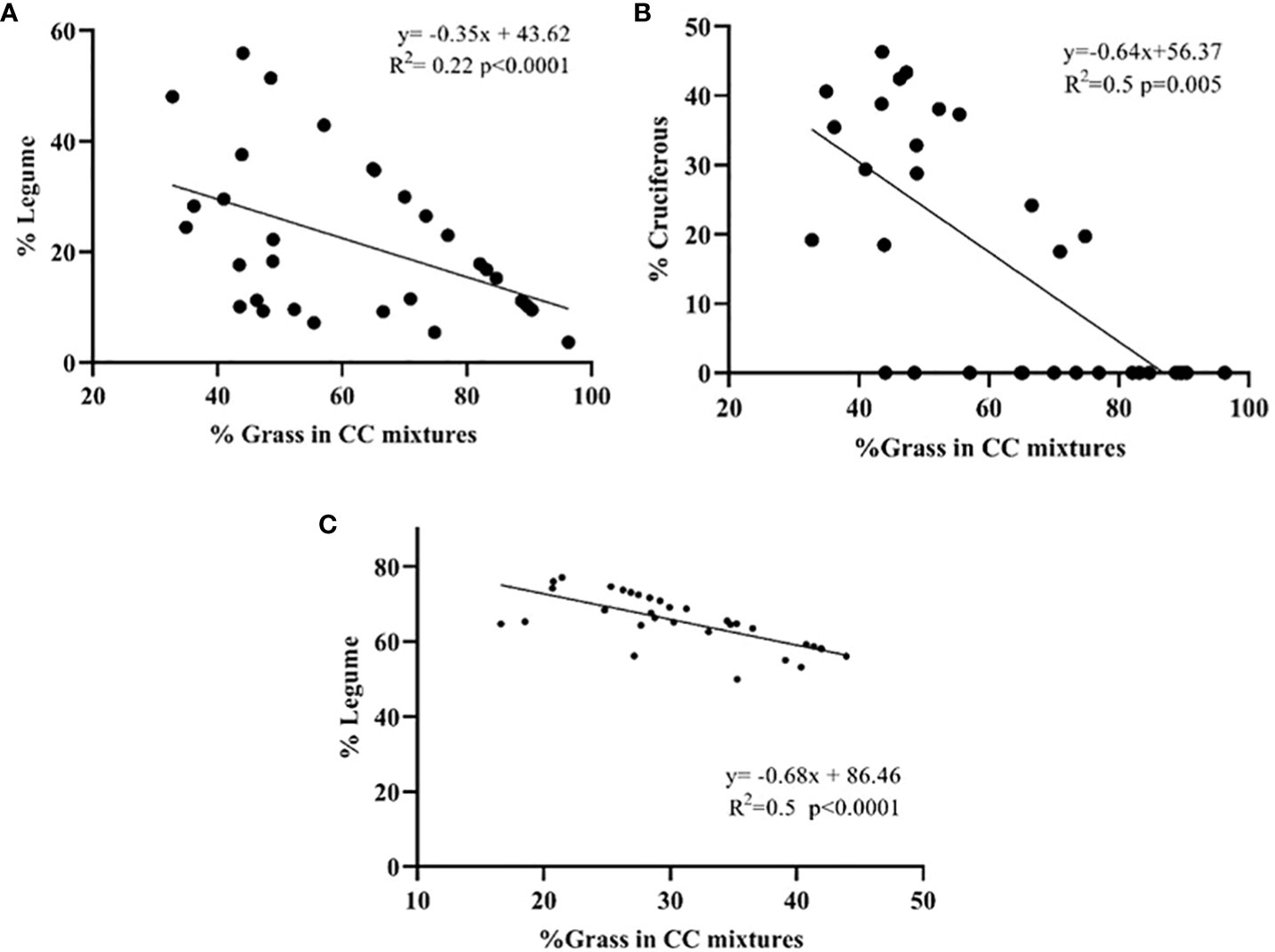
Figure 3 Linear relationships between the percentage (%) of grass in the CC mixtures and the resulting legume and cruciferous percentage for the years 2019 (A, B) and 2020 (C). The points indicate the biomass production of the groups of botanical families for the different CC evaluated.
Regarding VC, in 2019 the TM based on rye + hairy vetch presented the highest values (p<0.0001), followed by the BM oats or rye + hairy vetch and the TM oats + common or hairy vetch. While the BM made up of hairy vetch presented the lowest VC percentage with higher values for the rye-based mixture (Figure 4A). Hairy vetch presented higher (p<0.0001) VC than common vetch in all the mixtures evaluated and the addition of canola (p=0.0001) increased the VC of the BM. In 2020, the TM formed by rye + common or hairy vetch presented higher VC (p=0.0006) compared to all the evaluated mixtures, except for the BM rye + common vetch which did not present significant differences (Figure 4B). An effect of grass was also observed (p=0.0001) and, as in the first year of study, of the canola aggregate in SM (p=0.01). At this point, rye generated higher VC than oats and TM had higher VC than BM (Figure 4B).
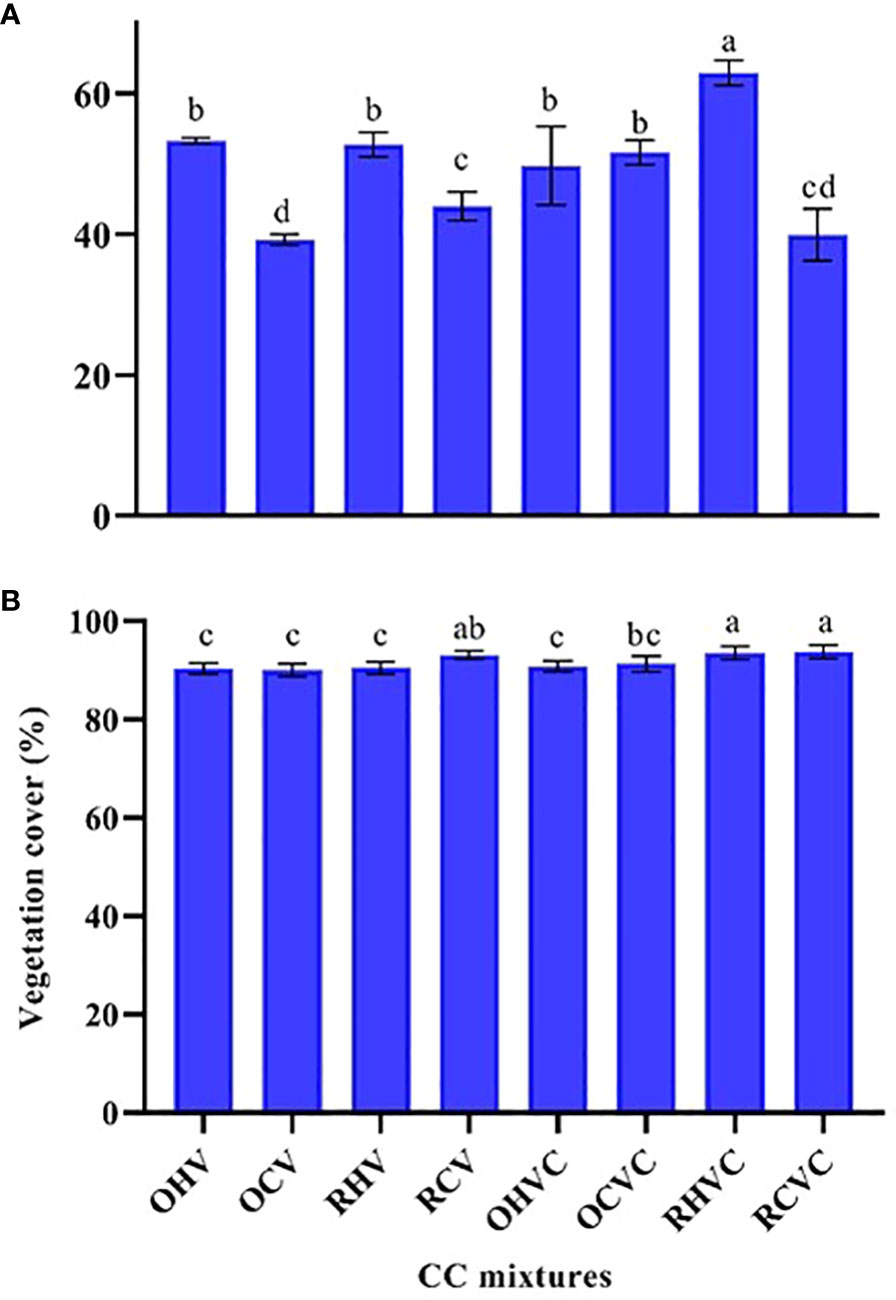
Figure 4 Vegetation cover (%) of the different CC mixtures: OHV (Oats+ Hairy vetch), OCV (Oats+ Common vetch), RHV (Rye+ Hairy vetch), RCV (Rye+ Common vetch), OHVC (Oats+ Hairy vetch+ Canola), OCVC (Oats+ Common vetch+ Canola), RHVC (Rye+ Hairy vetch+ Canola), RCVC (Rye+ Common vetch+ Canola), for the years 2019 (A) and 2020 (B). The bars represent mean values and the same letters indicate non-significant differences among treatments determined by Fisher’s LSD test (p<0.05).
The average VC was higher in 2020 than in 2019, highlighting that, in the second year, all mixtures achieved VC > 90%. While, in 2019, the maximum value of VC reached 63%, thus suggesting that the low rainfall regime was a limiting environmental factor.
For both years negative relationships were found between VC and the % of grasses in the CC (r=-0.44 and -0.36). VC decreased (p=0.01 and p= 0.04) when the proportion of grasses in the mixtures increased (Figures 5A, B). This variable also showed a positive correlation with the % of legumes (r=0.45) and Canola (r=0.62), for the years 2019 (p=0.01) and 2020 (p=0.009) respectively. This would determine that the proportion of legumes and cruciferous plants was important (p=0.01 and 0.009) to increase VC (Figures 5C, D).
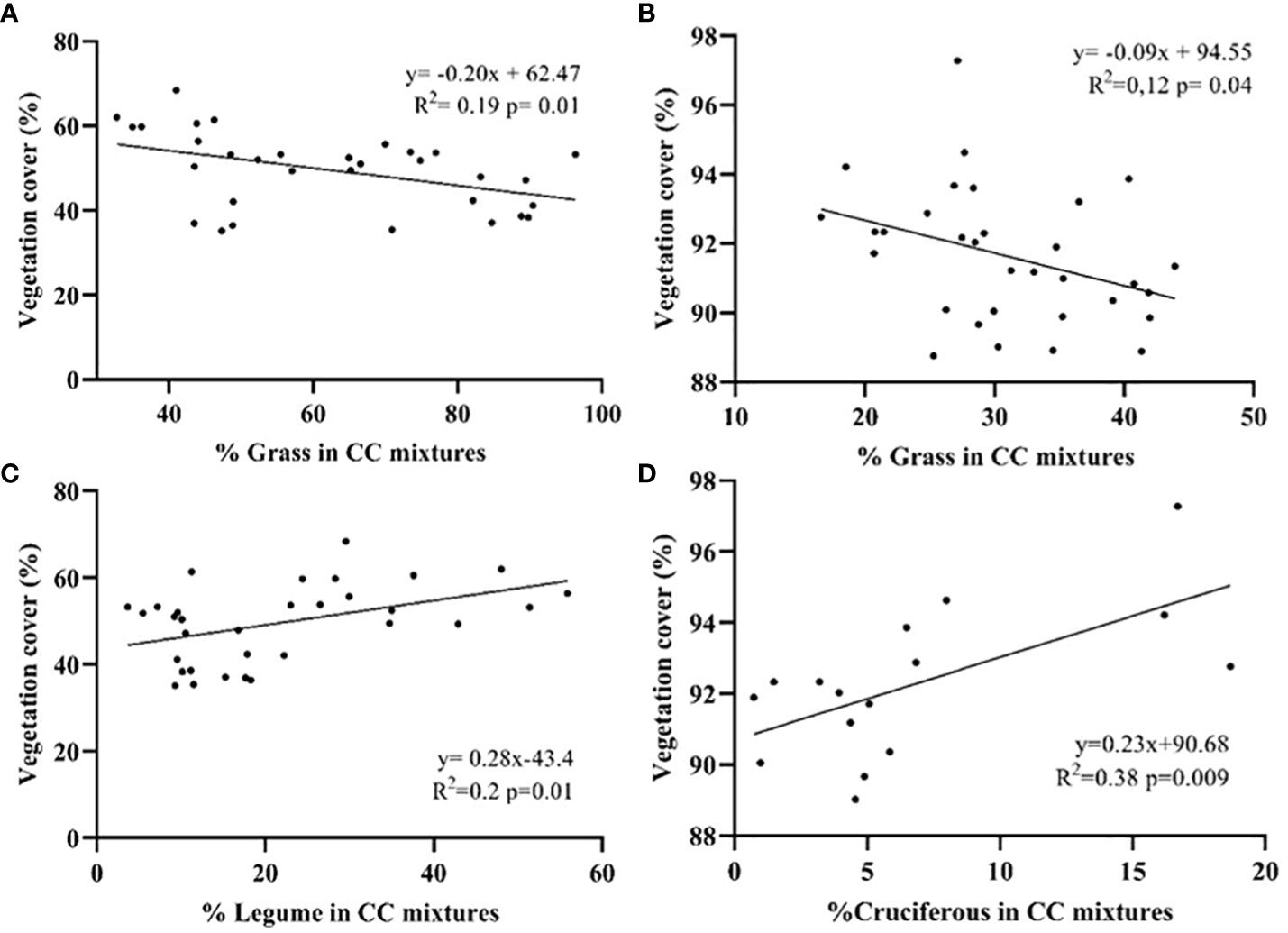
Figure 5 Linear relationships between vegetation cover (%) at the end of the cycle based on the % of grass for the years 2019 (A) and 2020 (B), legume and cruciferous in the mixtures for the years 2019 (C) and 2020 (D). The points indicate the vegetation cover generated for the % of grass, legume, or cruciferous in the different CC evaluated.
3.2 Characterization of the weed species surveyed
The A-W-S weed surveyed during 2019 and 2020 consisted predominantly of annual dicotyledonous species. Under conventional tillage (2019), Anagallis arvensis presented the highest relative abundance and average frequency of occurrence in all CC and sampling dates, followed by Conyza sumatrensis and Polygonum aviculare (Table 4). Under no-tillage (2020), P. aviculare showed the highest relative abundance followed by C. sumatrensis. In turn, these had the highest average frequency of occurrence while A. arvensis, G. spicata, Cirsium vulgare and Lolium spp. showed the lowest values.
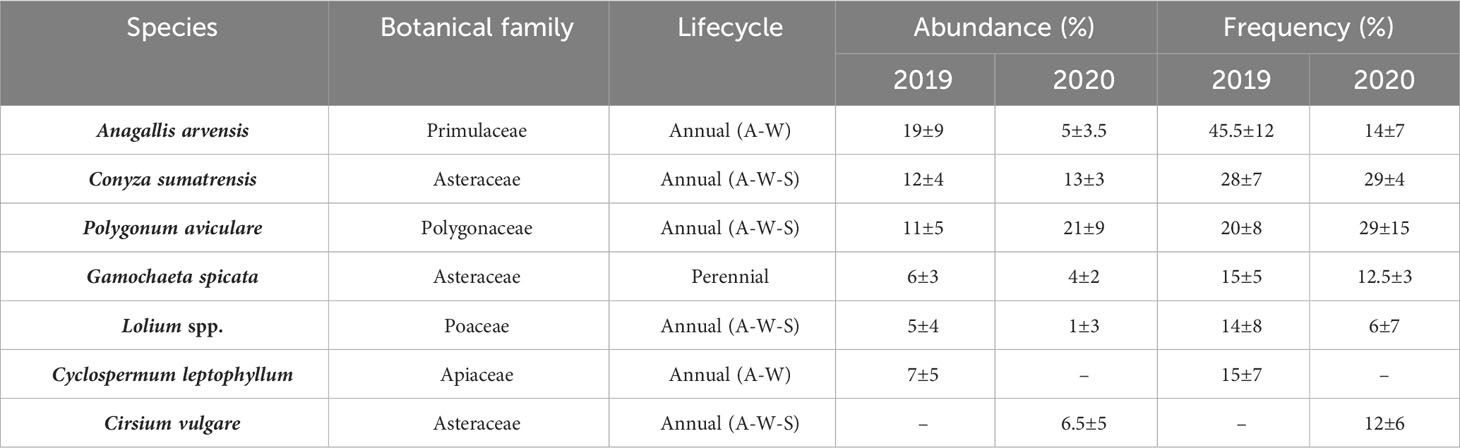
Table 4 Average frequency of occurrence (%) and abundance (%) of the different autumn-winter-spring (A-W-S) and autumn-winter (A-W) weed species: surveyed in all mixtures of CC and controls for 2019 (conventional tillage) and 2020 (non-tillage).
P. aviculare and C. sumatrensis were among the most abundant weeds regardless of the period considered. The variation found in the relative abundance of weed species surveyed between study years could be due to the differences between tillage systems and/or the contrasting rainfall regime between years.
3.3 Weed emergence density
In 2019, no significant differences were observed between CC and the control treatments in A-W-S weed emergence density. Also, no significant differences between the different types of CC mixtures (Figure 6A). In part, this could be due to the limiting water conditions prevailing this year (Table 3).In contrast, in 2020, all the CC mixtures suppressed weed emergence by 88-98% (p<0.0001) compared to chemical fallow and weedy plots, respectively (Figure 6B). Also, it is important to note that in both years, the CC generated an early suppression of weed emergence. As showed in Figure 7A, in 2019, at the beginning of the cycle (53 DAP), a greater emergence of A-W-S weeds (p=0.009) was observed in weedy plots compared to CC (polled data) (48 vs 17 pl m-2). In addition, no significant differences were observed between most CC mixtures and the chemical fallow, with the exception of BM oat + hairy vetch which presented the greatest emergence (p=0.01), comparable to weedy plots (Figure 7A). In 2020 (85 DAP), both controls showed a greater emergence of A-W-S weeds (p=0.0007), which were mainly dicotyledonous species, compared to the CC (polled data) (30 vs 9 pl m-2) and the BM rye + hairy vetch was the mixture with the lowest emergence (p=0.04) of weeds (Figure 7B). No significant relationship was observed between CC biomass production (or vegetation cover) and weed emergence density (p=0.6).
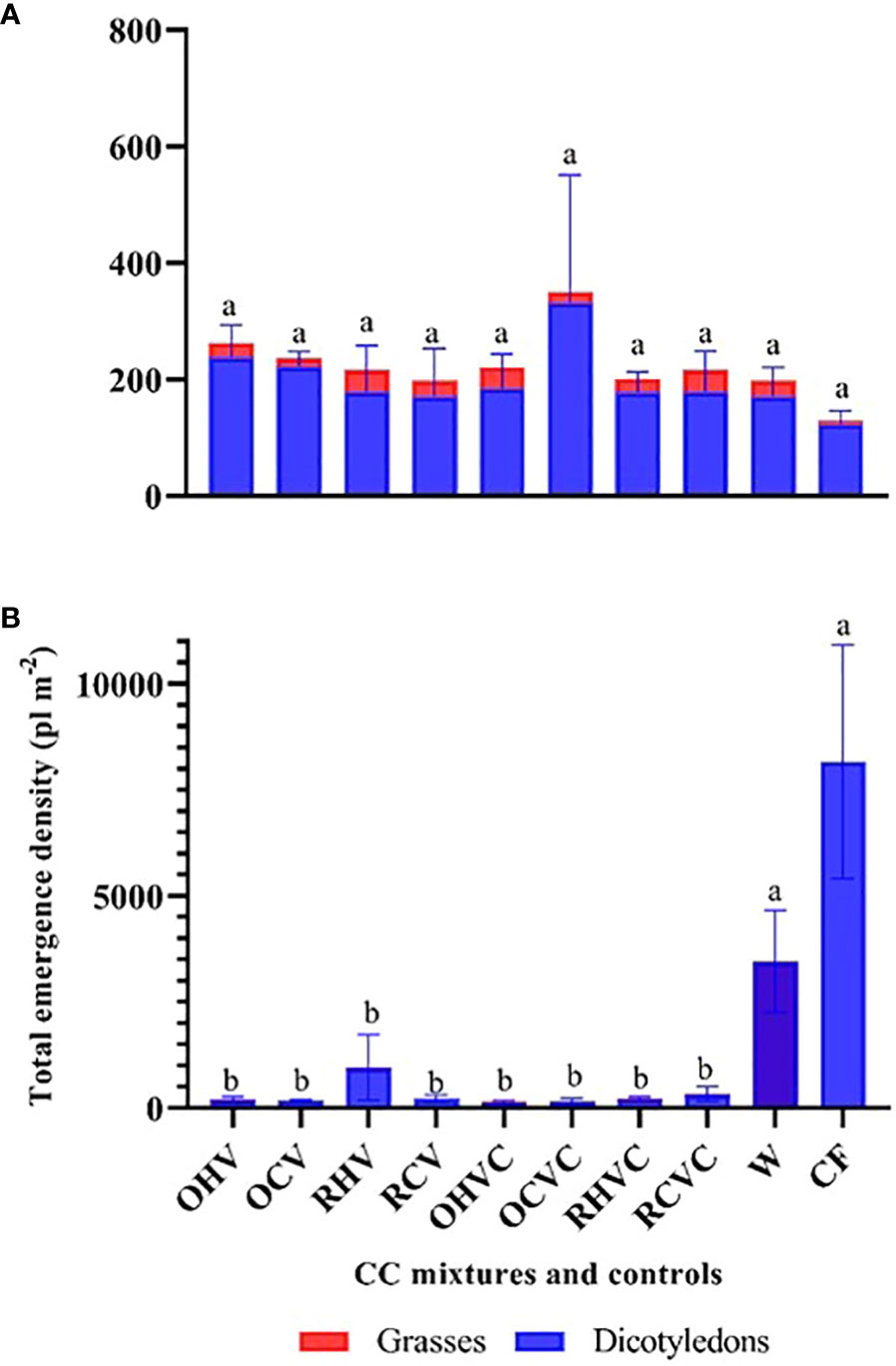
Figure 6 Total emergence density (pl m-2) of A-W-S weeds: dicotyledons (in blue) and grasses (in red) for the different mixtures and controls: OHV (Oats+ Hairy vetch), OCV (Oats+ Common vetch), RHV (Rye+ Hairy vetch), RCV (Rye+ Common vetch), OHVC (Oats+ Hairy vetch+ Canola), OCVC (Oats+ Common vetch+ Canola), RHVC (Rye+ Hairy vetch+ Canola), RCVC (Rye+ Common vetch+ Canola), CF (Chemical fallow), W (Weedy fallow), for the years 2019 (A) and 2020 (B). A-W-S and dicot weed data were transformed into a log(x) and square root (x+1). Mean values of untransformed data are shown in bars, significant differences among treatments are indicated by different letters) using Fisher’s LSD test (p<0.05).
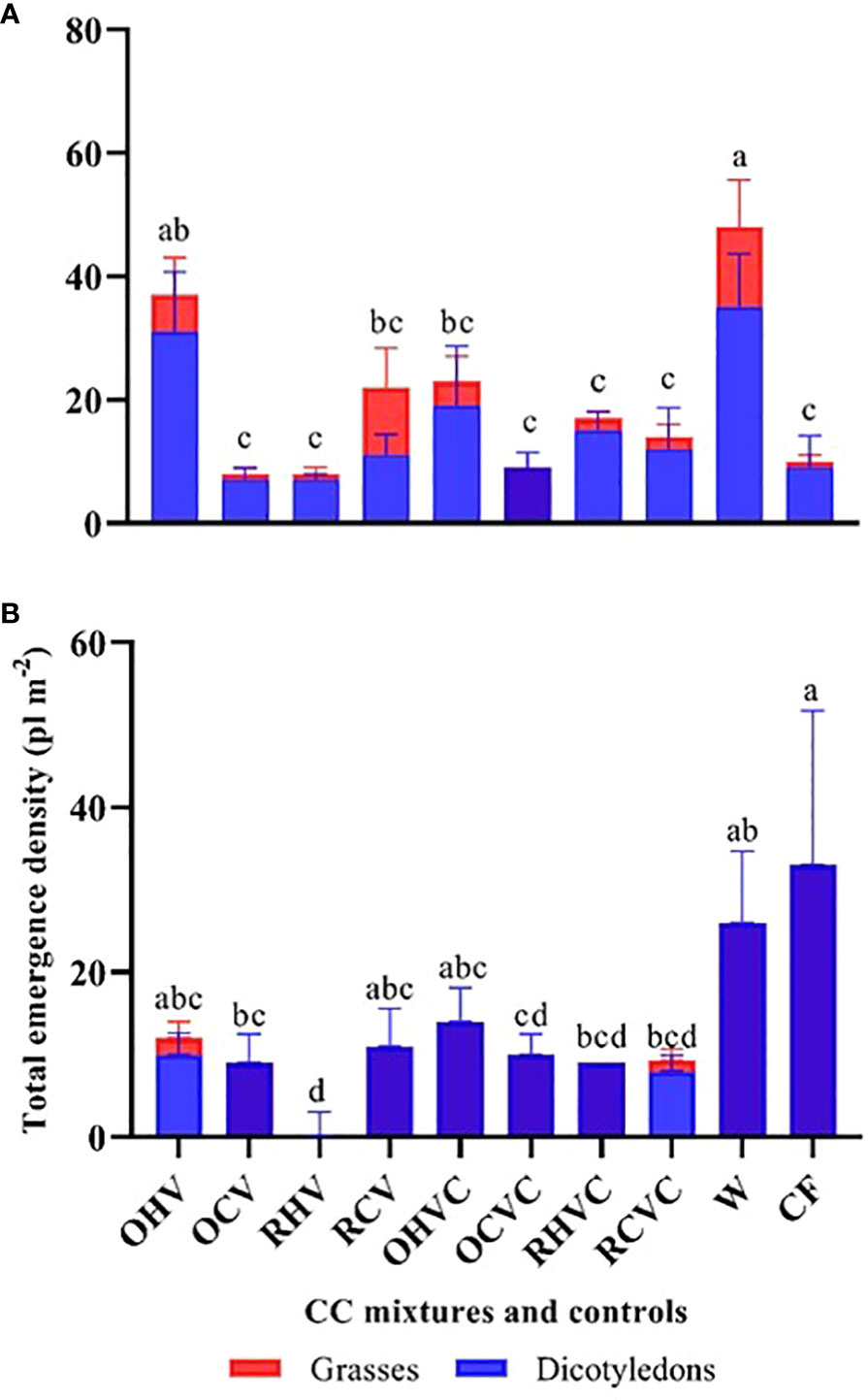
Figure 7 Emergence density (pl m-2) of A-W-S weeds: dicotyledons (in blue) and grasses (in red) for the different mixtures and controls: OHV (Oats+ Hairy vetch), OCV (Oats+ Common vetch), RHV (Rye+ Hairy vetch), RCV (Rye+ Common vetch), OHVC (Oats+ Hairy vetch+ Canola), OCVC (Oats+ Common vetch+ Canola), RHVC (Rye+ Hairy vetch+ Canola), RCVC (Rye+ Common vetch+ Canola), CF (Chemical fallow), W (Weedy fallow), at the beginning of the CC cycle for the years 2019 (A) and 2020 (B). 2020 A-W-S and dicot weed data were transformed into a square root (x+1). Mean values of untransformed data are shown in bars, significant differences among treatments are indicated by different letters) using Fisher’s LSD test (p<0.05).
3.4 Weed biomass
CC mixtures reduced weed biomass by 94.5 and 98% compared to the weedy plots for 2019 and 2020, respectively (Figure 8). These values were comparable to those obtained under chemical fallow and, in general, all mixtures showed low biomass levels except oats-hairy vetch and rye-common vetch BM in 2019. In terms of differences between CC mixtures, for the first year, the oats-hairy vetch BM presented higher biomass (p<0.0001) compared to the rye-common vetch TM, the BM, and TM based on rye-hairy vetch and the TM made up of oats-common vetch. Likewise, BM showed higher biomass (p=0.0468) compared to TM (Figure 8A). In 2020, the TM based on rye-hairy vetch and the BM of the same species with common vetch showed a higher biomass of spontaneous plants (p<0.0001) compared to the oats- common vetch BM (Figure 8B). Rye had a higher biomass (p=0.0325) compared to oats (Figure 8B). No significant relationship was observed between CC biomass production (or vegetation cover) and weed biomass (p= 0.67 and 0.15).
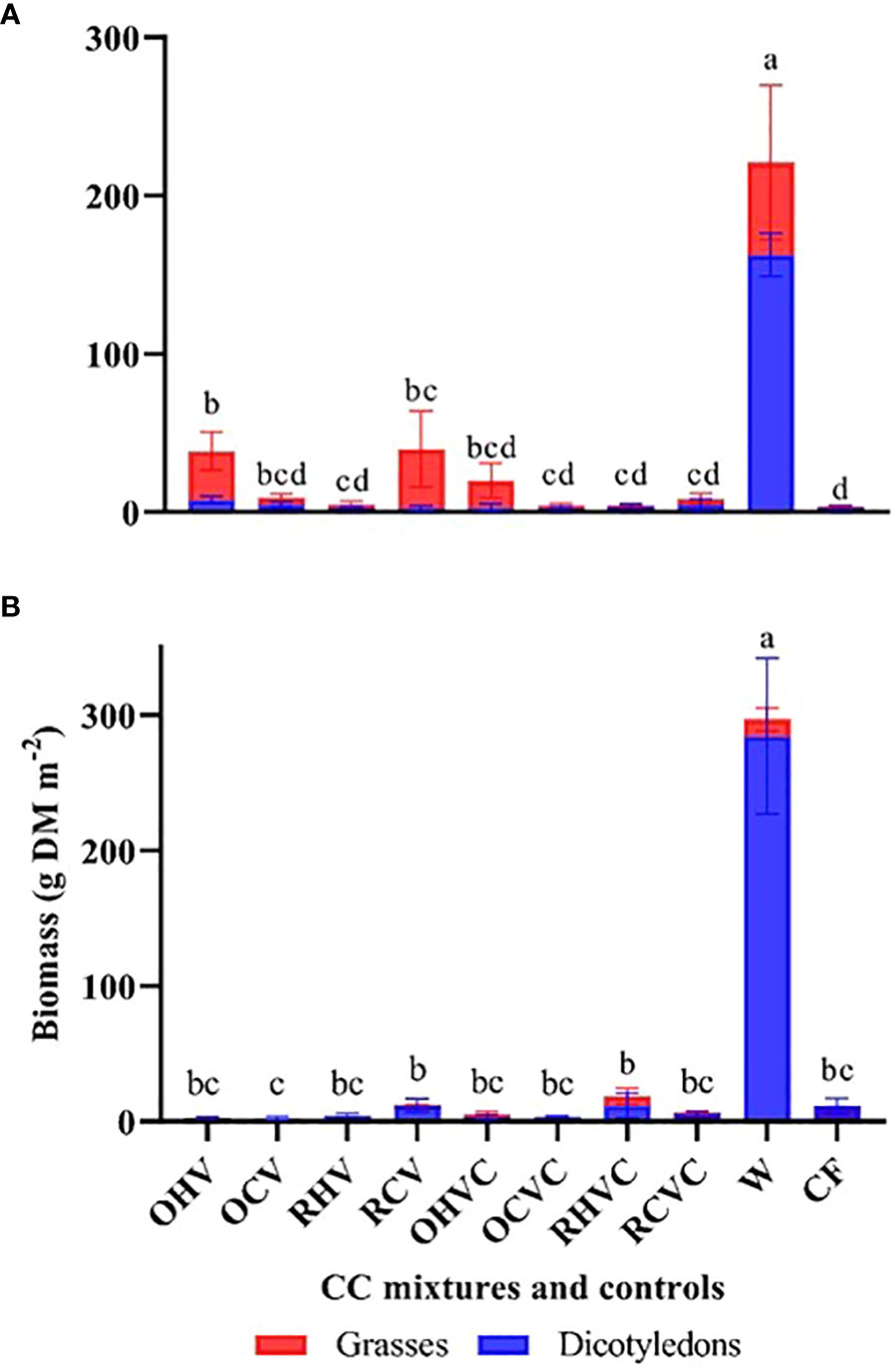
Figure 8 Aerial biomass (g m-2) of A-W-S weeds: dicotyledons (in blue) and grasses (in red) at the end of the cycle for the different mixtures and controls: OHV (Oats+ Hairy vetch), OCV (Oats+ Common vetch), RHV (Rye+ Hairy vetch), RCV (Rye+ Common vetch), OHVC (Oats+ Hairy vetch+ Canola), OCVC (Oats+ Common vetch+ Canola), RHVC (Rye+ Hairy vetch+ Canola), RCVC (Rye+ Common vetch+ Canola), CF (Chemical fallow), W (Weedy fallow), for the years 2019 (A) and 2020 (B). Average values of untransformed data are presented in the bars, and differences among treatments are indicated by different letters using Fisher’s LSD test (p<0.05).
4 Discussion
Results obtained in this contribution suggest that the composition of the CC mixtures would have a greater influence on total biomass production in years with a limited rainfall regime. Under such environmental conditions, the addition of canola to the CC mixture showed a tendency to increase productivity, while V. villosa showed greater stability compared to V. sativa. This could be explained by the differential behavior of both types of vetch, since V. villosa is more tolerant to prolonged periods of water deficit and low temperatures (Renzi, 2013; Renzi et al., 2019). For both years of study, mainly the type of vetch used, the addition of canola in BM, and the proportion of grasses affected the vegetation cover. This reveals the strong competitive capacity of grass species which must be taken into account when designing CC mixtures if the goal is to achieve a good diversity of its components. Grass seedling rates should be reduced by half to a quarter in a mixture (compared to monocultures) to achieve a balanced ratio with legumes, which tend to be weak competitors and must be planted at the same densities as monocultures to ensure establishment (White et al., 2016). In addition, the vertical orientation of the grass leaves would allow the passage of light through the upper strata (Elhakeem et al., 2021). Therefore, it is important to add other species to the mix with different strategies for using space, mainly those species with horizontal canopy architecture (MacLaren et al., 2019). Thus, the specific composition of the CC mixtures would have a greater influence on the vegetation cover than on the total biomass production, which would depend mainly on the prevailing environmental conditions, confirming the first hypothesis. The environmental conditions played a pivotal role in the results as indicated by the differences observed between years.
In this contribution we found evidence of an early suppressive effect of the CC mixtures on the emergence dynamics and growth of A-W-S weeds (Figures 6–8). Weed suppression values (66 and 94%) were similar to those provided by chemical methods (see Teasdale and Mohler, 1992; Osipitan et al., 2018) and are considered sufficiently high to prevent seed bank replenishment (Liebman and Nichols, 2020). In addition, an early weed emergence reduction would clearly decrease weed-crop competition (Hock et al., 2006). Based on these results, we decided to accept the second hypothesis. However, the study demonstrates that for both years of the study, CC mixtures were consistently more effective to suppress weed biomass compared to weed density. The latter was only lower than the control treatments in 2020. Despite this fact, both weed biomass production and final density levels were similar to those obtained by chemical fallow. These results are comparable to those reported by Piñeiro et al. (2019) for different types of CC and sites in Argentina. Conversely, Buratovich and Acciaresi (2019) observed a greater reduction in weed biomass in CC compared to the use of herbicides. The benefits of CC mixtures should be addressed within integrated weed management scenarios, considering the potential impact of these results on favoring seedbank depletion (Liebman and Nichols, 2020; Tiwari et al., 2021).
Finally, although many contributions have cited a positive relationship between biomass production (Finney et al., 2016; Florence et al., 2019; MacLaren et al., 2019) or vegetation cover (Kruidhof et al., 2008; Uchino et al., 2011; Dorn et al., 2015; Elhakeem et al., 2021) and weed suppression, no such relationships were observed in this work. Therefore, we decided to reject the third hypothesis. However, evidence was found that the composition of the mixtures affected the performance of CC. Therefore, these results could indicate that resources complementarity among CC species would influence on the weed suppressive capacity of the mixture. However, more studies should be conducted to comprehensively address the effect of CC mixtures on the weed dynamics.
5 Conclusions
The suppression of weed emergence and biomass exerted by CC mixtures were similar to (or even greater than) the chemical-based control. Average weed biomass figures for all CC mixtures were highly reduced when compared to the weedy control. In this sense, the mixture S. cereale + V. villosa + canola stood out for presenting the highest performance (productivity and vegetation cover) and high weed suppression. Obtained results support the idea that the use of species with functional differences is a practical recommendation criterion when designing better mixtures. Therefore, the implementation of CC within cropping sequences should be considered as an efficient and complementary tool, to promote IWM tactics and the design of more sustainable agricultural practices in the south-central region of Buenos Aires province, Argentina. From the authors perspective, this contribution provides novel results showing for the first time the effect of different CC mixtures on weed suppression at a community level in a dry subhumid environment of Argentina. It also provides valuable information in a poorly studied field,as weed emergence dynamics in CC. Future research should incorporate new variables in order to understand the relationship between the CC mixtures and weed suppression, such as: (i) the biomass production of both weeds and CC in the initial stages of the crop, (ii) the photosynthetic activity index active radiation as a complement to the vegetal cover, and (iii) the level of the weed seed bank before and after the implementation of the practice.
Data availability statement
The raw data supporting the conclusions of this article will be made available by the authors, without undue reservation.
Author contributions
MM: Writing – original draft. GC: Conceptualization, Formal analysis, Methodology, Supervision, Validation, Writing – review & editing. MY: Conceptualization, Formal analysis, Methodology, Supervision, Validation, Writing – review & editing.
Funding
The author(s) declare financial support was received for the research, authorship, and/or publication of this article. This work was partially supported by Instituto Nacional de Tecnología Agropecuaria (INTA) and Universidad Nacional del Sur (PGI 24/A254, PGI-TIR 80020190100001SU).
Conflict of interest
The authors declare that the research was conducted in the absence of any commercial or financial relationships that could be construed as a potential conflict of interest.
Publisher’s note
All claims expressed in this article are solely those of the authors and do not necessarily represent those of their affiliated organizations, or those of the publisher, the editors and the reviewers. Any product that may be evaluated in this article, or claim that may be made by its manufacturer, is not guaranteed or endorsed by the publisher.
References
Acciaresi H. A., Buratovich M. V., Cena M. E., Picapietra G., Restovich S. B. (2016). Cultivos de cobertura y su relación con la intercepción de la radiación fotosintéticamente activa y la materia seca aérea de las malezas. Rev. Tecnología Agropecuaria 10 (30), 45–48.
Akemo M. C., Regnier E. E., Bennett M. A. (2000). Weed Suppression in Spring-Sown Rye (Secale cereale)–Pea (Pisum sativum) Cover Crop Mixes 1. Weed Technol. 14 (3), 545–549. doi: 10.1614/0890-037x(2000)014[0545:wsissr]2.0.co;2
Baigorria T., Cazorla C., Santos Sbuscio D., Aimetta B., Belluccini P. (2013). Efecto de triticale (× Triticosecale Wittman) rolado como cultivo de cobertura en la supresión de malezas, rendimiento y margen bruto de soja. Siembra directa. Rev. técnica. Aapresid, 94–100.
Baigorria T., Gómez D., Cazorla C., Lardone A., Bojanich M., Aimetta B., et al. (2011). Bases para el manejo de vicia como antecesor del cultivo de maíz. Sitio Argentino Producción Anim. Available at: https://www.produccion-animal.com.ar/produccion_y_manejo_pasturas/pasturas%20artificiales/232-vicia.pdf.
Baraibar B., Hunter M. C., Schipanski M. E., Hamilton A., Mortensen D. A. (2017). Weed suppression in cover crop monocultures and mixtures. Weed Sci. 66 (1), 121–133. doi: 10.1017/wsc.2017.59
Baraibar B., Mortensen D. A., Hunter M. C., Barbercheck M. E., Kaye J. P., Finney D. M., et al. (2018). Growing degree days and cover crop type explain weed biomass in winter cover crops. Agron. Sustain. Dev. 38 (6), 1–9. doi: 10.1007/s13593-018-0543-1
Björkman T., Lowry C., Shail J. W., Brainard D. C., Anderson D. S., Masiunas J. B. (2015). Mustard cover crops for biomass production and weed suppression in the great lakes region. Agron. J. 107 (4), 1235–1249. doi: 10.2134/agronj14.0461
Brainard D. C., Bellinder R. R., Kumar V. (2011). Grass–legume mixtures and soil fertility affect cover crop performance and weed seed production. Weed Technol. 25 (3), 473–479. doi: 10.1614/wt-d-10-00134.1
Brennan E. B., Smith R. F. (2005). Winter cover crop growth and weed suppression on the central coast of California 1. Weed Technol. 19 (4), 1017–1024. doi: 10.1614/wt-04-246r1.1
Brooker R. W., George T. S., Homulle Z., Karley A. J., Newton A. C., Pakerman R. J., et al. (2021). Facilitation and biodiversity–ecosystem function relationships in crop production systems and their role in sustainable farming. J. Ecol. 1–14. doi: 10.1111/1365-2745.13592
Buratovich M. V., Acciaresi H. A. (2017). Cultivos de cobertura como moduladores de la emergencia de malezas naturales. Revista de Tecnología Agropecuaria 10 (35), 47–50.
Buratovich M. V., Acciaresi H. (2019). Manejando malezas con cultivos de cobertura: una alternativa tecnologica para disminuir el uso de herbicidas. Rev. Tecnología Agropecuaria-RTA 10, 51–54.
Capurro J., Dickie M. J., Ninfi D., Zazzarini A., Tosi E., Gonzalez M. C. (2012). Gramíneas Y leguminosas como cultivos de cobertura. Para Mejorar La Producción-INTA EEA Oliveros 47 (1), 83–88.
Cazorla C., Lardone A., Bojanich M., Aimetta B., Vilches D., Baigorria T. (2012). “Antecesores de maíz: ¿Barbecho o cultivos de cobertura?“ En: Contribución de los cultivos de cobertura a la sustentabilidad de los sistemas de producción. (Eds) Álvarez C., Quiroga A., Santos D., Bodrero M., (La Pampa, Argentina: Ediciones INTA). 1, 181–185 pp.
Cong W. F., Suter M., Lüscher A., Eriksen J. (2018). Species interactions between forbs and grass-clover contribute to yield gains and weed suppression in forage grassland mixtures. Agriculture Ecosyst. Environ. 268 (February), 154–161. doi: 10.1016/j.agee.2018.09.019
Davis C., Presley D., Farney J. K., Sassenrath G. (2016). Evaluating multi-species cover crops for forage production. Kansas Agric. Experiment Station Res. Rep. 2 (3), 8. doi: 10.4148/2378-5977.1204
Deguine J. P., Aubertot J. N., Bellon S., Côte F. X., Lauri P. E. P. E., Lescourret F., et al. (2023). Agroecological crop protection for sustainable agriculture. Adv. Agron. 178, 1–59. doi: 10.1016/bs.agron.2022.11.002
den Hollander N. G., Bastiaans L., Kropff M. J. (2007). Clover as a cover crop for weed suppression in an intercropping design. I. Characteristics of several clover species. Eur. J. Agron. 26 (2), 92–103. doi: 10.1016/j.eja.2006.08.011
Dhima K. V., Lithourgidis A. S., Vasilakoglou I. B., Dordas C. A. (2007). Competition indices of common vetch and cereal intercrops in two seeding ratio. Field Crops Res. 100 (2–3), 249–256. doi: 10.1016/j.fcr.2006.07.008
Di Rienzo J. A., Casanoves F., Balzarini M. G., González L., Tablada M., Robledo C. W. (2014). InfoStat version (Argentina: Professional. Group InfoStat, FCA, Universidad Nacional de Córdoba). Available at: http://www.infostat.com.ar (Accessed September 15, 2022).
Döring T. F., Storkey J., Baddeley J. A., Crowley O., Howlett S. A., McCalman H., et al. (2012). Legume based plant mixtures for delivery of multiple ecosystem services: weed diversity and weed control. Agric. Environ. 9, 163–168. Valuing Ecosystems: Policy, Economic and Management Interactions.
Dorn B., Jossi W., van der Heijden M. G. A. (2015). Weed suppression by cover crops: Comparative on-farm experiments under integrated and organic conservation tillage. Weed Res. 55 (6), 586–597. doi: 10.1111/wre.12175
Elhakeem A., van der Werf W., Baastiaans L. (2021). Radiation interception and radiation use efficiency in mixtures of winter cover crops. Field Crops Res. 264 (December 2020), 108034. doi: 10.1016/j.fcr.2020.108034
Finn J. A., Kirwan L., Connolly J., Sebastià M. T., Helgadottir A., Baadshaug O. H., et al. (2013). Ecosystem function enhanced by combining four functional types of plant species in intensively managed grassland mixtures: A 3-year continental-scale field experiment. J. Appl. Ecol. 50 (2), 365–375. doi: 10.1111/1365-2664.12041
Finney D., Kaye J. P. (2017). Functional diversity in cover crop polycultures increases multifunctionality of an agricultural system. J. Appl. Ecol. 54 (2), 509–517. doi: 10.1111/1365-2664.12765
Finney D. M., White C. M., Kaye J. P. (2016). Biomass production and carbon/nitrogen ratio influence ecosystem services from cover crop mixtures. Agron. J. 108 (1), 39–52. doi: 10.2134/agronj15.0182
Florence A. M., Higley L. G., Drijber R. A., Francis C. A., Lindquist J. L. (2019). Cover crop mixture diversity, biomass productivity, weed suppression, and stability. PloS One 14 (3), 1–18. doi: 10.1371/journal.pone.0206195
Groff S. (2008). Mixtures and cocktails: Soil is meant to be covered. J. Soil Water Conserv. 63 (4), 110–111. doi: 10.2489/jswc.63.4.110
Haramoto E. R., Gallandt E. R. (2004). Brassica cover cropping for weed management: A review. Renewable Agric. Food Syst. 19 (04), 187–198. doi: 10.1079/rafs200490
Hayden Z. D., Brainard D. C., Henshaw B., Ngouajio M. (2012). Winter annual weed suppression in rye–vetch cover crop mixtures. Weed Technol. 26 (4), 818–825. doi: 10.1614/wt-d-12-00084.1
Hayden Z. D., Ngouajio M., Brainard D. C. (2014). Rye-vetch mixture proportion tradeoffs: Cover crop productivity, nitrogen accumulation, and weed suppression. Agron. J. 106 (3), 904–914. doi: 10.2134/agronj2013.0467
Hock S. M., Knezevic S. Z., Martin A. R., Lindquist J. L. (2006). Soybean row spacing and weed emergence time influence weed competitiveness and competitive indices. Weed Sci. 54 (1), 38–46. doi: 10.1614/ws-05-011r.1
Holmes A. A., Thompson A. A., Wortman S. E. (2017). Species-specific contributions to productivity and weed suppression in cover crop mixtures. Agron. J. 109 (6), 2808–2819. doi: 10.2134/agronj2017.06.0309
INTA. (2014). Carta de Suelos de la provincia de Buenos Aires. Available at: https://anterior.inta.gob.ar/suelos/cartas/series/Tres_Arroyos.htm (Accessed November, 2023).
Kahl M., De Carli R., Behr E. (2016). Dinámica de las malezas de ciclo invernal sobre cultivos de cobertura y en barbecho químico en el centro-oeste de Entre Ríos. Serie Extensión INTA Paraná 78, 9–16.
Kato-Noguchi H., Kosemura S., Yamamura S., Mizutani J., Hasegawa K. (1994). Allelopathy of oats. I. Assessment of allelopathic potential of extract of oat shoots and identification of an allelochemical. J. Chem. Ecol. 20 (2), 309–314. doi: 10.1007/BF02064439
Kruidhof H. M., Bastiaans L., Kropff M. J. (2008). Ecological weed management by cover cropping: effect on winter weeds and summer weeds establishment in potato. Weed Res. 48 (6), 492–502. doi: 10.1111/j.1365-3180.2008.00665.x
Lawson A., Cogger C., Bary A., Fortuna A. M. (2015). Influence of seeding ratio, planting date, and termination date on rye-hairy vetch cover crop mixture performance under organic management. PloS One 10 (6), 1–19. doi: 10.1371/journal.pone.0129597
Liebman M., Dyck E. (1993). Crop rotation and intercropping strategies for weed management. Ecol. Appl. 3 (1), 92–122. doi: 10.2307/1941795
Liebman M., Nichols V. (2020). Cropping system redesign for improved weed management: A Modeling Approach Illustrated with Giant Ragweed (Ambrosia trifida). Agronomy 10 (2), 262. doi: 10.3390/agronomy10020262
Lobos M. H., Rampo M., Miranda W. (2019). Influencia de cultivos de cobertura invernales sobre la emergencia de malezas otoño- invernales y primavero-estivales. MEMORIA TÉCNICA 2018-2019. EEA INTA Gen. Villegas 1, 1–3.
Lorin M., Jeuffroy M. H., Butier A., Valantin-Morison M. (2015). Undersowing winter oilseed rape with frost-sensitive legume living mulches to improve weed control. Eur. J. Agron. 71, 96–105. doi: 10.1016/j.eja.2015.09.001
MacLaren C., Swanepoel P., Bennett J., Wright J., Dehnen-Schmutz K. (2019). Cover crop biomass production is more important than diversity for weed suppression. Crop Sci. 59 (2), 733–748. doi: 10.2135/cropsci2018.05.0329
Mesbah A., Nilahyane A., Ghimire B., Beck L., Ghimire R. (2019). Efficacy of cover crops on weed suppression, wheat yield, and water conservation in winter wheat–sorghum–fallow. Crop Sci. 59 (4), 1745–1752. doi: 10.2135/cropsci2018.12.0753
Miranda W., Girón P., Pérez M., Barraco M. (2014). “Cultivo De Cobertura: Espaciamiento Entre Hileras De Siembra y Manejo De Malezas,” in Memoria Técnica 2014-2015 ISSN 1850-6038. (General Villegas, Buenos Aires, Argentina: INTA Ediciones 11–14.
Murrell E. G., Schipanski M. E., Finney D. M., Hunter M. C., Burgess M. H., Lachance J. C., et al. (2017). Achieving diverse cover crop mixtures: Effects of planting date and seeding rate. Agron. J. 109 (1), 259–271. doi: 10.2134/agronj2016.03.0174
Ngouajio M., Mennan H. (2005). Weed populations and pickling cucumber (Cucumis sativus) yield under summer and winter cover crop systems. Crop Prot. 24 (6), 521–526. doi: 10.1016/j.cropro.2004.10.004
Norsworthy J. K., Malik M. S., Jha P., Riley M. B. (2007). Suppression of Digitaria sanguinalis and Amaranthus palmeri using autumn-sown glucosinolate-producing cover crops in organically grown bell pepper. Weed Res. 47 (5), 425–432. doi: 10.1111/j.1365-3180.2007.00586.x
Osipitan O. A., Dille J. A., Assefa Y., Knezevic S. Z. (2018). Cover crop for early season weed suppression in crops: systematic review and meta-analysis. Agron. J. 110 (6), 2211–2221. doi: 10.2134/agronj2017.12.0752
Piñeiro G., Pinto P., Della Chiesa T., Madias A. (2019). Informe Final de la Red de Cultivos de Servicios AAPRESID-BASF campaña 2018-2019. Red Cultivos Servicios AAPRESID-BASF. 28, 19–20.
Reeves D. W. (1994). “Cover crops and rotations,” in Crops Residue Management. Advances in Soil Science. Ed. Hatfield J. L. (United States: Lewis Publ), 125–172). doi: 10.1201/9781351071246
Renzi J. P. (2013). “Adaptación, Crecimiento y Desarrollo,” in Vicias: Bases agronómicas para el manejo de la Región Pampeana. Ed. Renzi J.P.M. Á. C. (Buenos Aires, Argentina: Ediciones), 101–126).
Renzi J. P., Chantre G. R., González-Andújar J. L., Cantamutto M. A. (2019). Development and validation of a simulation model for hairy vetch (Vicia villosa Roth) self-regeneration under different crop rotations. Field Crops Res. 235, 79–86. doi: 10.1016/j.fcr.2019.02.020
Restovich S. B., Andriulo A. E., Portela S. I. (2012). Introduction of cover crops in a maize-soybean rotation of the Humid Pampas: Effect on nitrogen and water dynamics. Field Crops Res. 128, 62–70. doi: 10.1016/j.fcr.2011.12.012
Ruffo M. L., Parsons A. T. (2004). Cultivos de Cobertura en Sistemas Agrícolas. Informaciones Agronómicas Del Cono Sur 21. Available at: https://fertilizar.org.ar/wp-content/uploads/2004/03/Cultivo-Cobertura-Matias-Ruffo.pdf.
Scavo A., Fontanazza S., Restuccia A., Pesce G. R., Abbate C., Mauromicale G. (2022). The role of cover crops in improving soil fertility and plant nutritional status in temperate climates. A review. Agron. Sustain. Dev. 42, 93. doi: 10.1007/s13593-022-00825-0
Schappert A., Schumacher M., Gerhards R. (2019). Weed control ability of single sown cover crops compared to species mixtures. Agronomy 9 (6), 294. doi: 10.3390/agronomy906029
Schipanski M. E., Barbercheck M., Douglas M. R., Finney D. M., Haider K., Kaye J. P., et al. (2014). A framework for evaluating ecosystem services provided by cover crops in agroecosystems. Agric. Syst. 125 (March), 12–22. doi: 10.1016/j.agsy.2013.11.004
Schonbeck B. M., Jerkins D., Ory J. (2017). Soil Health and Organic Farming Practical Conservation Tillage (Santa Cruz, California, United States: Organic Farming Research Foundation (Organic Fa)). Available at: https://www.pivotandgrow.com/wp-content/uploads/2017/08/Cover-Crops-Selection-and-Management.pdf.
Schulz M., Marocco A., Tabaglio V., Macias F. A., Molinillo J. M. G. (2013). Benzoxazinoids in rye allelopathy - from discovery to application in sustainable weed control and organic farming. J. Chem. Ecol. 39 (2), 154–174. doi: 10.1007/s10886-013-0235-x
Suter M., Hofer D., Luscher A. (2017). Weed suppression enhanced by increasing functional trait dispersion and resource capture in forage ley mixtures. Agriculture Ecosyst. Environ. 240, 329–339. doi: 10.1016/j.agee.2017.01.007
Teasdale J. R., Mohler C. L. (1992). Weed suppression by residue from hairy vetch and rye cover crops. Proc. First Int. Weed Congress 2, 516–528.
Tiwari R., Reinhardt Piskackova T. A., Devkota P., Mulvaney M. J., Ferrell J. A., Leon R. G. (2021). Emergence patterns of winter and summer annual weeds in Ethiopian mustard (Brassica carinata) cropping system. Weed Sci. 69 (4), 446–453. doi: 10.1017/wsc.2021.20
Uchino H., Iwama K., Jitsuyama Y., Ichiyama K., Sugiura E., Yudate T. (2011). Stable characteristics of cover crops for weed suppression in organic farming systems. Plant Production Sci. 14 (1), 75–85. doi: 10.1626/pps.14.75
USDA (1975). “Soil Taxonomy: A Basic System of Soil Classification for Making and Interpreting Soil Surveys. Soil Survey Staff, Coord., Soil Conservation Service,” in Agriculture Handbook, vol. 436. (Washington DC: US Department of Agriculture). 754 p.
Vanzolini J. I., Galantini J., Agamenonni R. (2013). “Cultivos de cobertura de Vicia villosa Roth. en el valle bonaerense del Río Colorado,” in Contribuciones de los cultivos de cobertura a la sostenibilidad de los sistemas de producción, 1st ed (La Pampa, Argentina: Ediciones INTA, Anguil), vol. 04.
Wayman S., Cogger C., Benedict C., Burke I., Collins D., Bary A. (2015). The influence of cover crop variety, termination timing and termination method on mulch, weed cover and soil nitrate in reduced-tillage organic systems. Renewable Agric. Food Syst. 30 (5), 450–460. doi: 10.1017/S1742170514000246
Wendling M., Büchi L., Amossé C., Jeangros B., Walter A., Charles R. (2017). Specific interactions leading to transgressive overyielding in cover crop mixtures. Agriculture Ecosyst. Environ. 241, 88–99. doi: 10.1016/j.agee.2017.03.003
Weston L. A. (1996). Utilization of allelopathy for weed management in agroecosystems. Agron. J. 88 (6), 860–866. doi: 10.2134/agronj1996.00021962003600060004x
White C., Barbercheck M., DuPont T., Finney D., Hamilton A., Hartman D., et al. (2016). Making the most of mixtures: considerations for winter cover crops in temperate climates. Extension.Org 1–32.
Keywords: service crops, integrated management, weed suppression, emergence density, weed biomass, biomass production, vegetation cover
Citation: Malaspina M, Chantre GR and Yanniccari M (2024) Effect of cover crops mixtures on weed suppression capacity in a dry sub-humid environment of Argentina. Front. Agron. 5:1330073. doi: 10.3389/fagro.2023.1330073
Received: 30 October 2023; Accepted: 18 December 2023;
Published: 16 January 2024.
Edited by:
Elba De La Fuente, University of Buenos Aires, ArgentinaReviewed by:
Aurelio Scavo, University of Messina, ItalyIoannis Roussis, Agricultural University of Athens, Greece
Copyright © 2024 Malaspina, Chantre and Yanniccari. This is an open-access article distributed under the terms of the Creative Commons Attribution License (CC BY). The use, distribution or reproduction in other forums is permitted, provided the original author(s) and the copyright owner(s) are credited and that the original publication in this journal is cited, in accordance with accepted academic practice. No use, distribution or reproduction is permitted which does not comply with these terms.
*Correspondence: Micaela Malaspina, bWFsYXNwaW5hLm1pY2FlbGFAaW50YS5nb2IuYXI=