- 1Tropical Research and Education Center, Department of Entomology and Nematology, University of Florida, Homestead, FL, United States
- 2School of Agricultural and Veterinarian Sciences (FCAV-Unesp), São Paulo State University, Jaboticabal, São Paulo, Brazil
- 3United States Department of Agriculture APHIS-PPQ-S&T, Miami, FL, United States
- 4Statistical Consulting Unit and Agronomy Department, Institute of Food and Agricultural Sciences, University of Florida, Gainesville, FL, United States
Introduction: The flat mite Brevipalpus yothersi is the main vector of citrus leprosis in South and Central America and Mexico, where vector suppression using conventional acaricides is usually the only disease control method, leading to problems with acaricide resistance. This mite is present in Florida, where several viruses known to cause citrus leprosis were recently detected. This research builds preparedness for managing emerging viral diseases and mitigating potential problems associated with acaricide resistance by addressing the compatibility of chemical and biological control strategies against B. yothersi. The predatory mite Amblyseius largoensis has shown promise as a biological control agent of B. yothersi. However, the effects of acaricides used in citrus on this predator are unknown.
Methods: This study investigated the impact of different routes of acaricide exposure on A. largoensis, including direct contact, pesticide-contaminated prey, residual contact, and combined exposure routes in laboratory settings. Additionally, the efficacy of A. largoensis in controlling B. yothersi, alone and in combination with acaricide applications, was evaluated under greenhouse conditions.
Results and discussion: Commonly used acaricides like abamectin and spirodiclofen were highly effective in controlling B. yothersi and only slightly harmful to A. largoensis. Other acaricides were also effective against B. yothersi but were less compatible with the predatory mite. In the greenhouse trial, all acaricides, alone or in combination with the predators, and the predators alone, effectively suppressed B. yothersi one month after treatment application. However, B. yothersi populations significantly increased one month later in the abamectin-alone and predator-alone treatments. At the same time, the combination of abamectin and predators provided B. yothersi suppression throughout the experiment. The fenpyroximate-predator combination also provided better control than each treatment applied independently. All other acaricides provided prolonged B. yothersi control, hindering biological control probably due to the lack of prey for A. largoensis. Research findings highlight the potential for enhancing the selectivity of acaricides towards A. largoensis by manipulating their exposure route. Combining predatory mites with acaricides shows promise in improving B. yothersi management.
1 Introduction
Brevipalpus-transmitted diseases (BTDs) are caused by single-stranded RNA viruses belonging to the families Kitaviridae (genera Cilevirus and Higrevirus) and Rhabdoviridae (genus Dichorhavirus), transmitted exclusively by flat mites in the genus Brevipalpus (Locali-Fabris et al., 2006; Dietzgen et al., 2018; Quito-Avila et al., 2021). Recently, three of seven viruses known to cause citrus leprosis (CiL), one of the most damaging BTDs, were found infecting multiple ornamental plants in Florida, different species of orchids in California, and hibiscus, passionfruit, and citrus in Hawaii (Melzer et al., 2012; Roy et al., 2013; Roy et al., 2018a; Fife et al., 2021; Olmedo-Velarde et al., 2021a; Olmedo-Velarde et al., 2021b; Alvarez-Quinto et al., 2022; Dey et al., 2022). The flat mite, Brevipalpus yothersi Baker (Acari: Tenuipalpidae), is an important pest of citrus in South and Central America and Mexico (Kitajima et al., 2003; Rodrigues et al., 2003; Castillo et al., 2011; Roy et al., 2015; Ramos-González et al., 2018) due to its ability to transmit Citrus leprosis virus C (CiLV-C, Cilevirus leprosis) and citrus leprosis virus C2 (CiLV-C2, Cilevirus colombiaense) which cause severe yield losses and a reduction in the lifespan of trees, especially in the sweet orange (Citrus sinensis L. Osbeck) (Bastianel et al., 2010; Moreira et al., 2022; Ramos-González et al., 2023). A strain of CiLV-C2 was recently detected in the US associated with B. yothersi, where proactive integrated pest management programs (IPM) are being developed to mitigate viral diseases transmitted by Brevipalpus mites (Peña et al., 2015; Revynthi et al., 2019). This research builds preparedness for managing BTDs by addressing the compatibility of chemical and biological control strategies against B. yothersi.
Acaricide sprays to suppress disease vectors are the main strategy for managing citrus leprosis in South and Central America (Amorim et al., 2006; Andrade et al., 2010; Silva et al., 2012; Della Vechia and Andrade, 2022). In fact, about 10% of the acaricide market worldwide is used to manage Brevipalpus spp. (Van Leeuwen et al., 2015). Given the importance of controlling B. yothersi, the high costs associated with acaricide applications (Bassanezi et al., 2019), and problems associated with acaricide resistance (Alves et al., 2000; Campos and Omoto, 2002; Rocha et al., 2021), an integrated approach combining chemical and biological control methods may be critical to managing these mites effectively (Bielza, 2016; Bielza et al., 2020; Leung et al., 2020). Compatibility between biological and chemical control methods is usually overlooked but critical to the success of integrated pest management (Duso et al., 2020). Several active ingredients are used commercially to target Brevipalpus mites in citrus, including abamectin, bifenazate, cyflumetofen, etoxazole, fenpyroximate, hexythiazox, pyridaben, spirodiclofen, spirotetramat, and sulfur (Della Vechia et al., 2022). Some of these compounds are relatively selective to Phytoseiidae mites, killing the target pest without killing the predatory mites (Cote et al., 2022; Assis et al., 2013; Doker and Kazak, 2019b; Bajda et al., 2022; Barros et al., 2022).
Amblyseius largoensis (Muma) (Acari: Phytoseiidae) is a cosmopolitan and generalist predatory mite that inhabits citrus orchards and other fruit crops around the world (Moraes et al., 2004). This predator has been studied for its potential to control tenuipalpid, eriophyoid, and tarsonemid mites (Galvão et al., 2007; Rodríguez et al., 2011; Carrillo et al., 2012a; Carrillo et al., 2012b; Carrillo et al., 2014; Melo et al., 2015; Rodríguez et al., 2015) and has been reported as an efficient predator of Brevipalpus spp. (Haramoto, 1969; Kamburov, 1971; Galvão et al., 2008; Argolo et al., 2020).
A recent study suggests that A. largoensis could be an effective biocontrol agent of B. yothersi in Florida (Argolo et al., 2020), where this natural enemy has been the most abundant predator in backyard and varietal citrus in multiple surveys conducted in the last decades (Childers et al., 2022). This predator is also dominant in other agricultural systems in Florida (Carrillo et al., 2010; Carrillo et al., 2012), suggesting it must have been subject to continuous exposure to pesticides used in the region. Predators can be exposed to pesticides in different ways that can be more or less harmful (Pozzebon et al., 2011; Bajda et al., 2022). Routes of pesticide exposure include direct contact during spraying, contact with contaminated surfaces during foraging, or feeding on contaminated prey (Longley and Stark, 1996). Understanding the effects of different routes of exposure of A. largoensis to acaricides used to manage B. yothersi may be critical for its successful integration into biocontrol augmentation or conservation programs in the context of IPM.
We hypothesized that A. largoensis may be partially compatible with chemical controls available for B. yothersi. Therefore, the impact of multiple acaricides on A. largoensis administered via different exposure routes (direct contact, pesticide-laced diet (prey), residual contact, and a combination of all exposure routes) was examined under laboratory conditions. In addition, the ability of A. largoensis to control B. yothersi alone and in combination with acaricide applications was assessed under greenhouse conditions.
2 Materials and methods
2.1 Brevipalpus yothersi colony
A pesticide susceptible strain of B. yothersi obtained from citrus plants has been maintained in the Tropical Fruit Entomology laboratory (UF-TREC) for more than five years without pesticide exposure. The mites were reared on common bean seedlings (Phaseolus vulgaris L.) grown on floral foam, following the methods described by Groot and Breeuwer (2006). The colony was kept in environmental chambers (Panasonic MLR-352H-PA, PHC Corporation of North America, Wood Dale, IL) at 27 ± 1°C, 70 ± 5% RH, and 12:12 h (L:D).
2.2 Predatory mite colony
A colony of A. largoensis was established with individuals collected from coconut fronds in the spring of 2018. Standard methods for rearing phytoseiid mites, described by Overmeer (1985), were used to rear A. largoensis. Typha spp. pollen (Nutrimite™, Biobest), Tetranychus urticae Koch (Acari: Tetranychidae), and B. yothersi mites were provided thrice weekly as food for the predatory mites. The colony was kept in an environmental chamber under controlled conditions of 25 ± 1°C, 80 ± 5% RH, and 12:12 h (L:D).
2.3 Laboratory assays
2.3.1 Screening test
2.3.1.1 Screening adulticide and ovicide treatments against Brevipalpus yothersi
Two separate trials were conducted to evaluate ovicides and adulticides on B. yothersi using the bioassays described in Rodrigues et al. (2007). The experimental units were prepared using lime fruit collected from unsprayed trees at UF-TREC. Fruits were submerged in paraffin, leaving a 5.0 cm diameter area without paraffin, representing the experimental arena. The arenas were also “ringed” with entomological glue (Tree Tanglefoot Insect Barrier®, The Tanglefoot Company) to prevent mites from escaping.
For the ovicide assay, 15 B. yothersi adult females (2-3 days old) were transferred to each arena and allowed to oviposit. After 48 h, females were removed, and the number of eggs was adjusted to 15 per arena. Then, the part of the fruit with the experimental arena was submerged in the pesticide (ovicide) solutions described in (Table 1) or in water (control) for two seconds. Nine replicates (fruit) were used for each treatment in the ovicide assay. Egg mortality was determined by counting the number of larvae that emerged daily for ten consecutive days after treatment application. Eggs that did not hatch were considered dead.
New fruits were used to prepare additional experimental arenas with ten newly emerged adult B. yothersi females for the adulticide trial. The infested arenas were dipped in the pesticide solutions described in Table 1 for two seconds, using water as a control. The number of live and dead adults was recorded daily for seven consecutive days after treatment application. Twelve replicates were used for each treatment in the adulticide trial.
2.3.1.2 Mortality of Amblyseius largoensis exposed to acaricides via direct contact
The experimental arenas consisted of 2.5 cm diameter x 1.5 cm height plastic cages with an opening in the lid covered with mite-proof mesh (mesh opening: 0.0385 mm) to allow ventilation. A thin layer of 1% agarose (Fisher Scientific) was placed on the bottom and a bean leaf disk (~ 2.5 cm diam.) was placed on the agar. One A. largoensis adult female (3-4 days old) was released onto the leaf disk and treatments were sprayed directly onto the mites on the arenas before placement of the lids. Acaricide solutions were applied with a manual pressurized sprayer (Porter Cable Air Compressor), delivering 0.8 mL of spray solution per leaf disk at the rates included in Table 1; water was used as a control treatment. A total of 15 replicates were used for each treatment. Predatory mite mortality was recorded daily for seven consecutive days after treatment. Every two days, mites were offered B. yothersi (eggs, immatures, and adults) and Typha spp. pollen ad libitum as food.
Pesticide compatibility with A. largoensis was based on predator mortality on the seventh day after application according to the IOBC (International Organization for Biological and Integrated Control) toxicity categories i.e., class 1 = E < 25% (innocuous); class 2 = 25% < E < 50% (slightly harmful); class 3 = 51% < E < 75% (moderately harmful) e class 4 = E > 75% (harmful) (Hassan et al., 1994).
2.3.2 Effect of acaricide exposure route on predator survival
Abamectin, cyflumetofen, fenpyroximate, and spirodiclofen are among the most used acaricides worldwide (Van Leeuwen et al., 2015). These acaricides provided effective B. yothersi adult control and were either innocuous (Cyflumetofen) or slightly harmful to A. largoensis (abamectin, fenpyroximate, and spirodiclofen) in the screening tests (Sect 2.3.1). Therefore, these acaricides were selected to investigate A. largoensis’s survival after exposure to them via four delivery routes: (i) residual contact, (ii) direct contact, (iii) contaminated prey, and (iv) a combination of residual contact, direct contact, and contaminated prey. Acaricide solutions were applied at the rates in Table 1 and using the same experimental arenas (plastic cages) and spray volumes as in the previous assay.
In the residual contact treatment (i), experimental arenas were constructed with bean leaf disks sprayed with individual acaricides and allowed to dry for 30 minutes before one A. largoensis female (3-4 days old) was released per arena. In addition, 50 B. yothersi eggs (less than 5 days old) were transferred from the stock colony to each arena as a food source for the predators, which were replenished every other day. For direct contact (ii), individual predatory mite females were placed on untreated leaf disks and then sprayed with an acaricide. Treated predators were also offered 50 B. yothersi eggs from the colony, which were replenished every other day. For the acaricide-contaminated prey treatment (iii), B. yothersi infested bean leaves from the colony were sprayed with acaricides and allowed to dry for 30 minutes before transferring groups of 50 treated eggs (less than 5 days old) to untreated arenas. Then, one A. largoensis female was released per arena. Treated B. yothersi eggs offered as food source were replenished every other day. The combined effect of direct contact, residual contact, and acaricide-laced prey (iv) was investigated by first releasing A. largoensis on bean leaf disks arenas with 50 B. yothersi eggs, and then spraying the leaf disks containing predators and prey. The control treatment consisted of an arena with one A. largoensis female and 50 B. yothersi eggs (replenished as before), which were treated with water on the bean leaf disk. The experiment was kept in a climate-controlled chamber at 25 ± 1°C, 80 ± 5% relative humidity (RH), and a 12:12 h (L:D) photoperiod. Predator survival was recorded daily for five days. Predatory mite eggs were counted and removed from the leaf disk daily. Four assays were performed, each with one acaricide. Each experiment comprised five exposure routes (i, ii, iii, iv, and control) and 20 replicates, totaling 100 predatory mites per acaricide.
2.4 Greenhouse assay
The experiment was conducted in a glasshouse with controlled temperature and relative humidity (25 ± 4°C and 75 ± 15% RH) at UF-TREC from March to July 2022. Sixty orange plants (Valencia variety, seeded 1 year before use, approximately 30 cm tall, unsprayed, and pest-free) were used. Each plant was artificially infested with one orange leaf containing 75 B. yothersi adults taken from the colony. The infested leaf was attached to the abaxial surface of a leaf in the center of the experimental plant using a paper clip. Infested plants were then placed in individual cages (62.0 cm x 23.5 cm, 160 μm mesh size) and held for 45 days before being randomly assigned to one of the following treatments: 1) Control (water), 2) Water + predatory mite (A. largoensis), 3) Abamectin (0.9 g a.i.L-1), 4) Abamectin + predatory mite, 5) Cyflumetofen (0.5 g a.i.L-1), 6) Cyflumetofen + predatory mite, 7) Fenpyroximate (5.2 g a.i.L-1), 8) Fenpyroximate + predatory mite, 9) Spirodiclofen (0.7 g a.i.L-1), 10) Spirodiclofen + predatory mite. For the treatments receiving predatory mites, five adult A. largoensis females were transferred from the stock colony into Eppendorf tubes (1.5 mL) that were hung to the experimental plants using a hair clip. This predator-prey ratio effectively suppressed pestiferous tenuipalpid mites in previous research with A. largoensis (Carillo et al., 2014; Argolo et al., 2020). The tubes were then opened, and the predators were allowed to colonize the plant freely. The acaricide applications were made 15 days after the predators were released. Each treatment was replicated on six plants.
Brevipalpus yothersi populations were evaluated four times: 1) before the pesticide application and predatory mite release, 2) 30 days after treatment (acaricide) application (DAT), 3) 62 DAT, and 4) 104 DAT. In the first evaluation, two leaves were randomly removed from each plant, placed in a paper bag, and immediately inspected under a stereomicroscope in the laboratory to record the number of B. yothersi adults, immatures, and eggs. Four and six leaves were collected for the second and third evaluations. The last evaluation comprised the entire plant, which was destructively sampled to record all B. yothersi individuals on the plant.
2.5 Statistical analysis
Abbott’s transformation was used to correct control mortality (Abbott, 1925) in the ovicide and adulticide assays, which was less than 10%. Egg and adult mortality after acaricide applications in the screening test was analyzed using generalized logistic regression techniques implemented in the JMP Fit Model Platform (JMP Statistical Discovery LLC, Cary, NC) using a binomial data distribution. Treatment least squares means were compared using simple t-tests at α = 0.05 based on the recommendations by Milliken and Johnson (2009) and Saville (2015). Least squares means and confidence limits were back-transformed to the data scale.
Survival data in the lab study were analyzed using generalized linear model procedures as implemented in SAS® PROC GLIMMIX (SAS/STAT 15.2; SAS Institute, Cary, NC) using a binomial distribution function and the canonical logit link. Because the control treatment (water) was the same for all acaricides tested, we analyzed it separately to obtain the best estimate for this treatment across all acaricides. The remaining treatments were analyzed using the model Survived/Total = Acaricides + Treatment + Acaricides * Treatment. Interaction least squares means plus 95% confidence limits were calculated and treatments were compared within acaricide using a simple t-test (LSD) based on the recommendations by Milliken and Johnson (2009) and Saville (2015). Least squares means and confidence limits were back transformed to the data scale.
For the greenhouse experiment, count data were analyzed using generalized linear model methodology procedures as implemented in SAS® PROC GLIMMIX (SAS/STAT 15.2; SAS Institute, Cary, NC) with a negative binomial distribution function and the canonical log link function. This factorial experiment consisted of factors treatment (5 levels), predator (2 levels) and DAA (4 levels); the generalized linear model contained all three main effects, all possible two-way interaction effects, and the three-way interaction effect. Least squares means comparisons followed the same approach as described earlier.
3 Results
3.1 Screening adulticide and ovicide treatments against Brevipalpus yothersi
The effects of ovicides and adulticides on B. yothersi varied among the acaricides tested (Tables 2, 3). Etoxazole and spirodiclofen had the highest acute ovicidal activity, causing 100% egg mortality six days after application (DAA). Hexythiazox was also highly effective, reaching 99% mortality 10 DAA. Spirotetramat was the least effective ovicide, reaching approximately 70% egg mortality at the end of the experiment.
Cyflumetofen, abamectin, fenpyroxymate, pyridaben, and sulfur showed high acute activity, causing 100% B. yothersi adult mortality 1-2 days after application. Spirotetramat, spirodiclofen, and tolfenpyrad had a slower activity, reaching 100% mortality 4-7 days after application. Bifenazate had the lowest activity on B. yothersi, causing significantly less mortality (~80% at the end of the experiment) than other acaricides tested on every evaluation date.
3.2 Mortality of Amblyseius largoensis exposed to conventional acaricides via direct contact
Amblyseius largoensis survival decreased slightly after direct contact with the tested acaricides relative to the water control (Figure 1). Abamectin, cyflumetofen, etoxazole, spirodiclofen, and sulfur did not differ significantly from the control (water). Fenpyroximate and pyridaben were also slightly harmful to A. largoensis but caused significantly more mortality than the control. Tolfenpyrad and hexythiazox were the most harmful acaricides, causing more than 50% of A. largoensis mortality. According to the IOBC toxicity categories, cyflumetofen (21.4% mortality seven days after application) can be considered innocuous (class 1) to A. largoensis, while abamectin (33.3%), etoxazole (26.7%), fenpyroximate (40.0%), pyridaben (46.7%), spirodiclofen (35.7%) and sulfur (28.6%) can be considered class 2, slightly harmful acaricides. Hexythiazox (53.3%) and tolfenpyrad (80.0%) can be regarded as class 3 (moderately harmful) and (class 4, harmful), respectively.
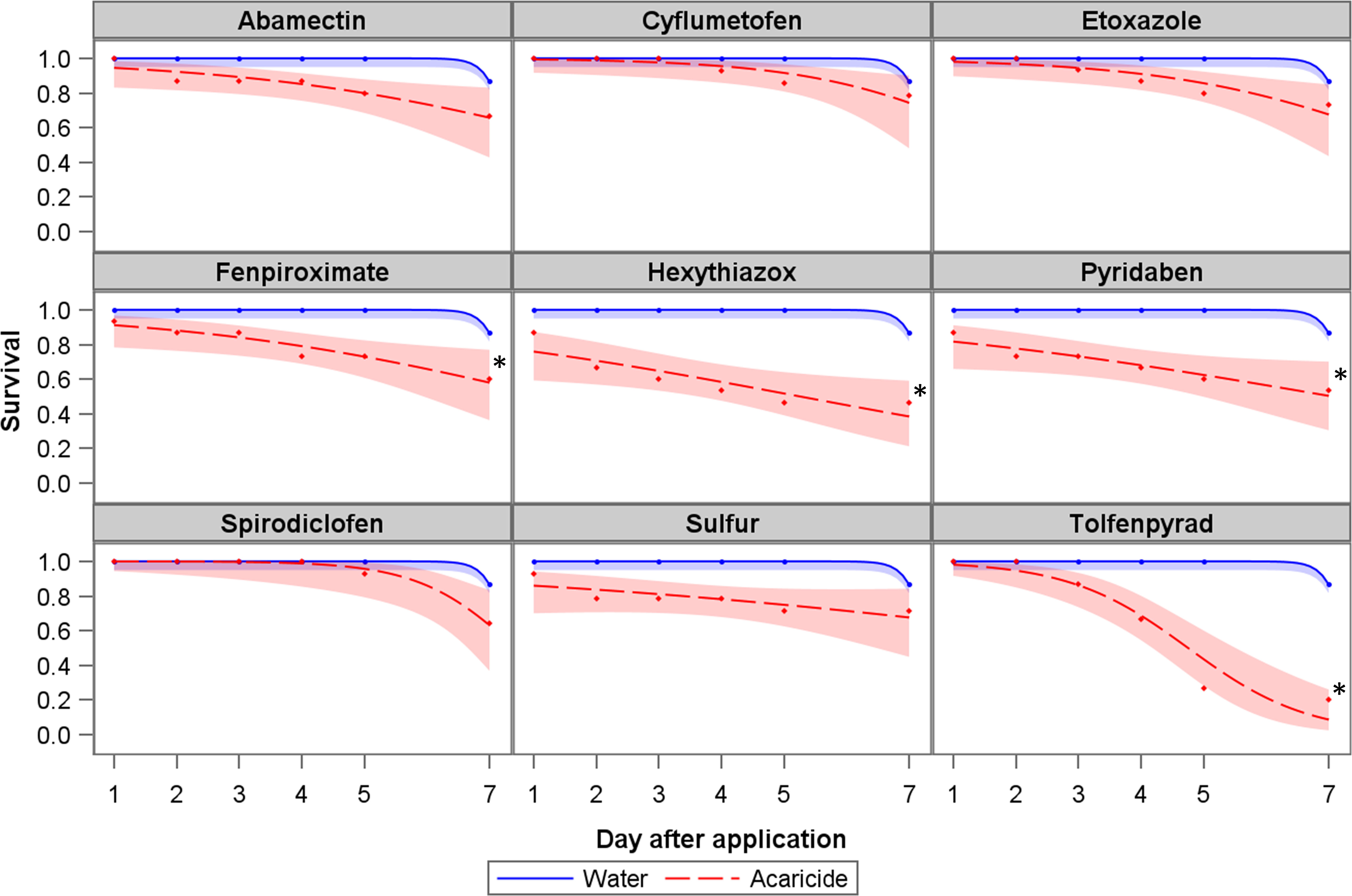
Figure 1 Survival of Amblyseius largoensis exposed to acaricides spirodiclofen (0.7 g a.i.L-1), cyflumetofen (0.5 g a.i.L-1), abamectin (0.9 g a.i.L-1), tolfenpyrad (1.3 g a.i.L-1), fenpyroximate (5.2 g a.i.L-1), pyridaben (0.3 g a.i.L-1), sulfur (3.0 g a.i.L-1), etoxazole (4.5 g a.i.L-1), and hexythiazox (1.5 g a.i.L-1). The shaded areas represent the 95% prediction interval. The asterisk (*) represents a statistically meaningful difference between water and acaricide treatment (α = 0.05).
3.3 Effect of acaricide exposure route on predator survival
All the acaricides, independent of the exposure route, reduced the mite survival rate relative to the water control (Figure 2). Amblyseius largoensis survival decreased between 16% and 48% due to abamectin exposure. This pesticide was significantly more harmful via ingestion of contaminated prey and the combination exposure routes (D+R+I) than through direct or residual contact (Figure 2). Predatory mite survival was 45% to 51% after cyflumetofen exposure, and no differences were observed among the different exposure routes (Figure 2). By contrast, the route of exposure to fenpyroximate significantly affected predator survival. The combination of exposure routes was most harmful to A. largoensis, causing nearly 100% mortality, followed by ingesting fenpyroximate contaminated prey, causing a 93% reduction in predator survival. Direct contact with fenpyroximate was significantly less harmful than the combination of exposure routes or ingestion of contaminated prey, but residual contact was the least harmful of all exposure routes (Figure 2).
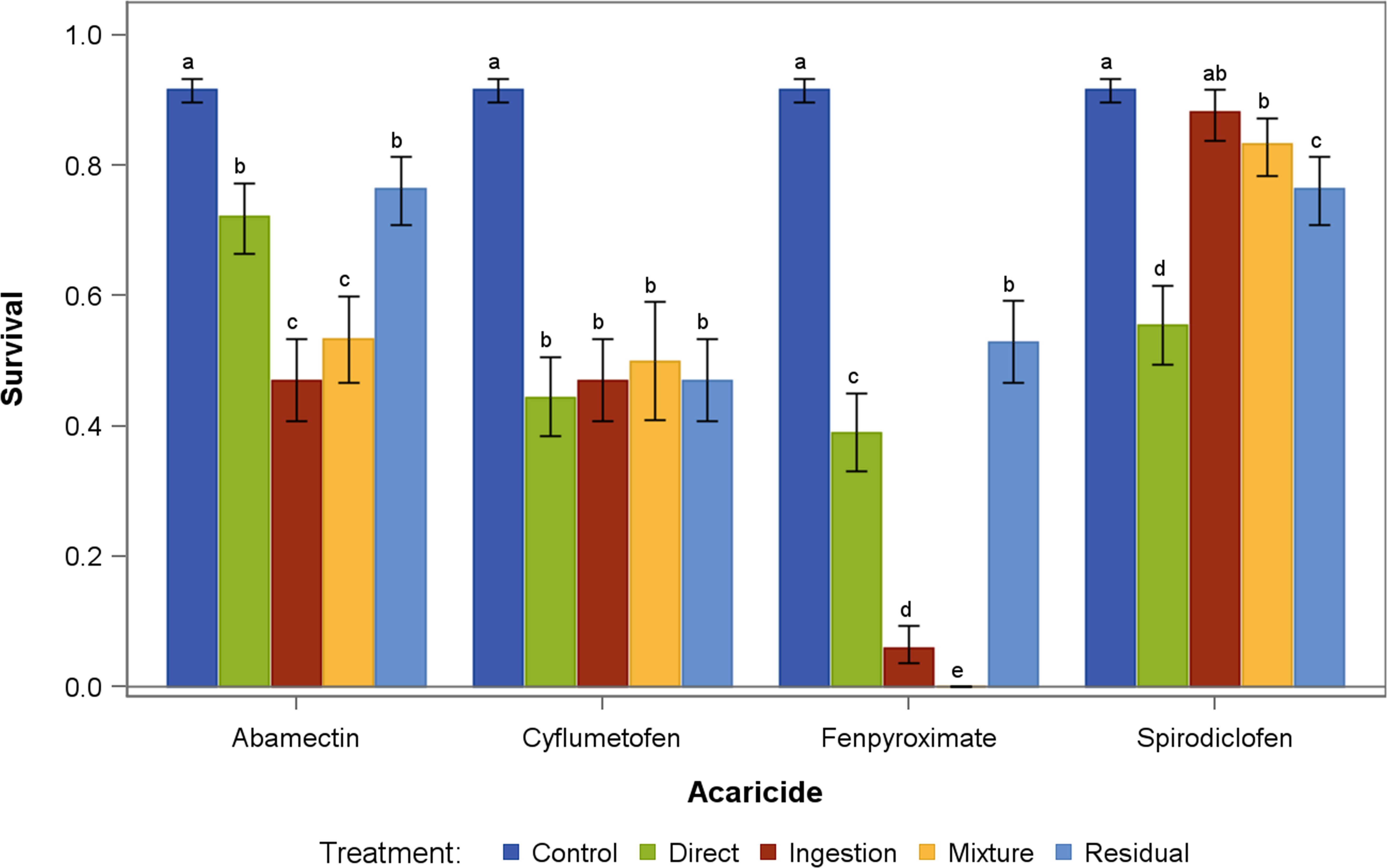
Figure 2 Mean survival of Amblyseius largoensis five days after exposure to four acaricides via different routes (direct contact, acaricide-contaminated prey (ingestion), a combination of exposure routes(D+R+I), and residual contact). Error bars represent 95% confidence intervals. Within acaricide, bars sharing letters are not statistically meaningful different at α = 0.05 based on simple tests, following the recommendations by Milliken and Johnson (2009) and Saville (2015).
Spirodiclofen had a low impact on predator survival rates via ingestion exposure and the combination of exposure routes followed by residual contact. Survival rates of predators subject to the combination of exposures were similar to those exposed via residual contact. Unexpectedly, direct exposure to spirodiclofen resulted in the lowest survival rate of A. largoensis.
3.4 Greenhouse assay
Before treatment, all experimental plants had similar B. yothersi infestation levels. Thirty days after treatment application, B. yothersi populations had increased by approximately 5-fold in the water control treatment (Table 4). By contrast, all acaricide treatments alone or in combination with the predators, and the predators alone, significantly suppressed B. yothersi. However, among the acaricide-only treatments, abamectin and cyflumetofen provided a faster knockdown effect than spirodiclofen and fenpyroximate thirty days after treatment application (Table 4). The predators alone caused a significant reduction in B. yothersi populations relative to the water control. However, this reduction was significantly lower than when predators were combined with any of the acaricides, resulting in nearly 100% control.
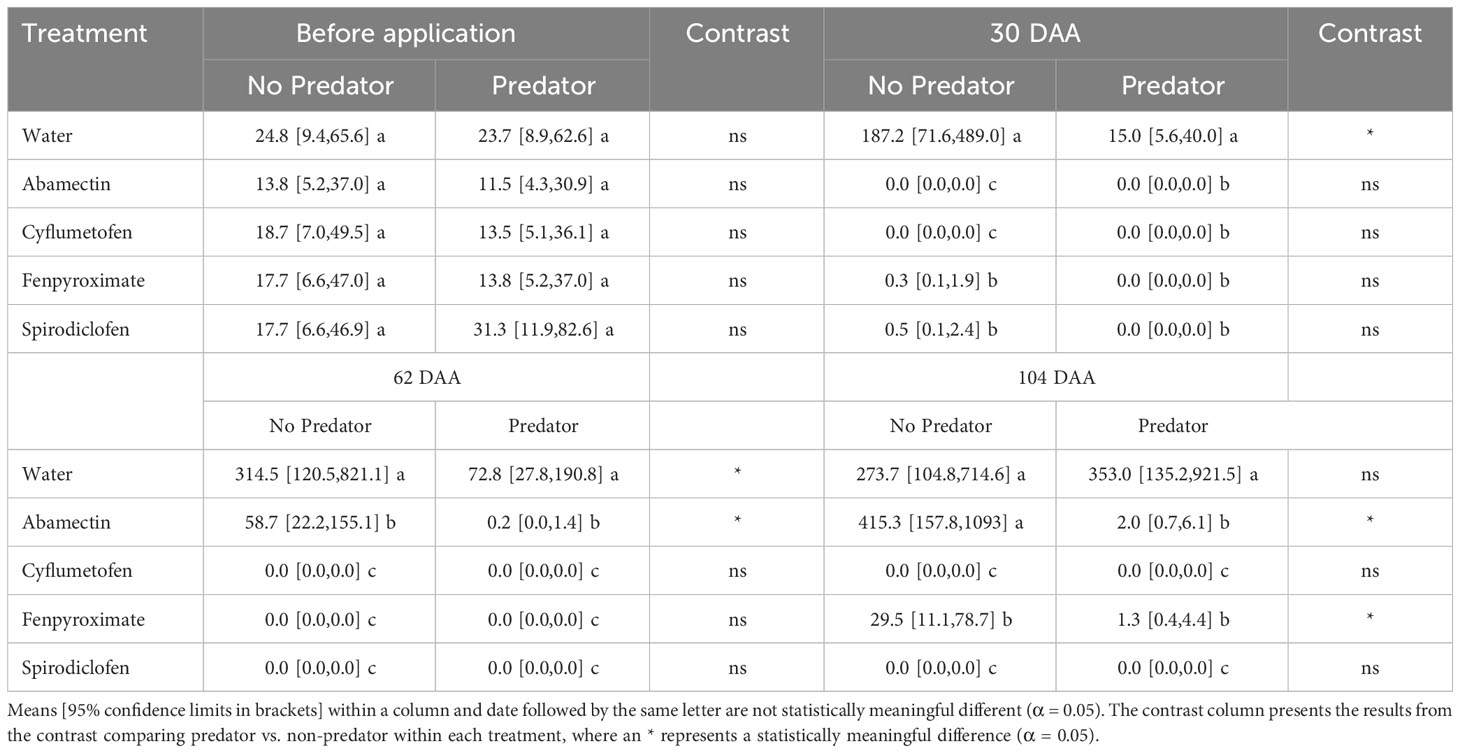
Table 4 Influence of acaricides and their synergy with Amblyseius largoensis on controlling Brevipalpus yothersi infestations in greenhouse citrus plants.
Sixty-two days after treatment application, B. yothersi populations continued to increase in plants subject to the water control treatment. Mite populations on abamectin-alone treated plants also increased at a lower extent than in those treated with water, but significantly more than when other acaricides were applied alone. The predators alone suppressed B. yothersi populations relative to the water control, but B. yothersi suppression by the predators was significantly low compared to treatments that combined predators and acaricides. The abamectin-predator combination reduced B. yothersi populations significantly more than when each treatment was applied separately. When fenpyroximate, cyflumetofen, and spirodiclofen were combined with predators, suppression was almost complete; however, suppression can be attributed to the acaricides because the same levels of B. yothersi suppression were recorded when these acaricides were applied alone.
In the last evaluation, 104 days after treatment application, B. yothersi populations in the water control and predator-alone treatments were similar. Among the treatments involving acaricides only, abamectin-treated plants had similar B. yothersi numbers as the water control, and a slight but significant increase in B. yothersi populations was recorded on fenpyroximate-treated plants. By contrast, the complete B. yothersi suppression by cyflumetofen and spirodiclofen persisted. In plants treated with abamectin and fenpyroximate, the combination with predators resulted in a significantly greater reduction of B. yothersi than the acaricides alone. Treatments that combined cyflumetofen or spirodiclofen with predators provided complete control. However, suppression can be attributed to the acaricides rather than the predators, as in the previous evaluation period.
4 Discussion
Our results suggest that chemical control could be a highly effective tactic for managing viruliferous B. yothersi in the U.S.A. Eggs of Florida populations of B. yothersi were highly susceptible to common mite ovicides used in citrus, such as etoxazole, hexythiazox, spirodiclofen, and spirotetramat. Moreover, abamectin, bifenazate, cyflumetofen, fenpyroximate, pyridaben, sulfur, spirotetramat, spirodiclofen, and tolfenpyrad showed high efficacy in controlling B. yothersi adults. However, overreliance on chemical pesticides may lead to the selection of B. yothersi resistant populations, and the integration of nonchemical strategies like biological control could be fundamental to resistance management (Omoto et al., 2000; Campos and Omoto, 2002; Rocha et al., 2021). One acaricide classified as innocuous (cyflumetofen) and three acaricides classified as slightly harmful to A. largoensis (abamectin, fenpyroximate, and spirodiclofen) were used to study the effects of different pesticide exposure routes on A. largoensis (Hassan et al., 1994). These acaricides are the most commercialized worldwide and have different modes of action (Van Leeuwen et al., 2015). Cyflumetofen showed low to moderate toxicity to A. largoensis regardless of the exposure route. The available literature suggests that cyflumetofen has a low impact through direct and residual contact on several phytoseiids including Neoseiulus fallacis German, Neoseiulus californicus McGregor, Phytoseiulus persimilis Athias-Henriot, Amblyseius eharai Amitai and Swirski, Amblyseius tsugawai Ehara, Euseius sojaensis Ehara, and Typhlodromus vulgaris Ehara (Lee and Kim, 2015; Kishimoto et al., 2018; Bergeron and Schmidt-Jeffris, 2020).
Fenpyroximate has been reported as selective to populations of A. largoensis on coconut (Assis et al., 2013; Barros et al., 2022) and to other phytoseiid mites in other systems (Kim and Paik, 1996; Ghasemzadeh and Qhreshi, 2018). However, we found that this selectivity can vary depending on the exposure route. Direct contact, feeding on fenpyroximate-laced prey, and the combination of exposure routes were highly toxic to A. largoensis (61.1% to 100.0% mortality). Surprisingly, residual contact with fenpyroximate was only slightly harmful to A. largoensis (47.1% mortality). Similarly, Sato et al. (2002) recorded a high survival in N. californicus released on fenpyroximate-contaminated leaves. On the other hand, direct contact with fenpyroximate resulted in 100% Galendromus occidentalis (Nesbitt) mortality (Irigaray and Zalom, 2007). These differences in toxicity among fenpyroximate exposure routes can be related to the amount of pesticide uptake by the predators (Van Leeuwen and Dermauw, 2016). The higher toxicity of fenpyroximate through direct exposure suggests a role of integumental absorption of this pesticide by A. largoensis.
Abamectin was only slightly harmful to A. largoensis via residual or direct contact. Feeding upon abamectin-contaminated prey was more harmful but still allowed more than 50% survival of the predator populations. Abamectin is regarded as harmful to predatory mites of the family Phytoseiidae (Lima et al., 2013; Fernández et al., 2017; Doker and Kazak, 2019a). However, in Florida abamectin is commonly used in citrus and other tropical and subtropical fruit production systems. Therefore, A. largoensis populations may have repeated exposure to this pesticide and likely developed some tolerance/resistance. Our results suggest that A. largoensis can survive on plants treated with abamectin or colonize plants recently treated with this pesticide. In addition, A. largoensis releases after abamectin applications could help maintain B. yothersi populations suppressed longer than when the pesticide is used alone.
Spirodiclofen showed low toxicity to A. largoensis independently of the delivery route, corroborating the results obtained by Assis et al. (2013) on predator populations associated with another tenuipalpid mite, Raoiella indica (Acari: Tenipalpidae). Other phytoseiid species (i.e., Amblyseius swirskii Athias-Henriot and N. californicus) also have reported tolerance to spirodiclofen (Kaplan et al., 2012; Doker and Kazak, 2019a). Our results suggest that spirodiclofen is compatible with A. largoensis and useful for the integrated management of B. yothersi because it effectively controls the pest mite and shows low toxicity to its biocontrol agent. However, direct contact with spirodiclofen resulted in significantly more mortality than ingestion of contaminated prey and the combination of exposure routes, which suggests that spirodiclofen detoxification enzymes or other unknown mechanisms may be present in A. largoensis.
In the greenhouse trial, all acaricides, alone or in combination with the predators, and the predators alone, effectively suppressed B. yothersi one month after treatment application. Abamectin alone showed the shortest control period among the acaricides (approximately 30 days). However, when combined with A. largoensis, the pest mite control was extended throughout the experimental period. Combining abamectin and A. largoensis provided better control (99.9% B. yothersi population reduction) than each control method alone (76.8% and 81.3% B. yothersi population reduction, respectively). Furthermore, plants treated with fenpyroximate in combination with A. largoensis exhibited fewer mites at 104 DAA than plants treated only with this acaricide. Our results suggest that A. largoensis can improve B. yothersi control after abamectin and fenpyroximate applications. All other acaricides were highly effective and provided prolonged B. yothersi control throughout the experiment, hindering biological control by A. largoensis, probably due to the lack of prey. In the predator-alone treatment, A. largoensis initially suppressed B. yothersi, but after 62 days, the pest population increased. This result suggests that periodic releases may be necessary for effective and prolonged biological control by this predatory mite. An alternative explanation relies on the controlled experimental conditions without access to alternative prey. Under natural conditions, A. largoensis utilizes multiple arthropod prey, including other mites and insects inhabiting citrus plants and other tropical and subtropical plants. This predator is classified as a type III generalist phytoseiid mite that feeds on insects and mites as well as alternative food sources like pollen and nectar, allowing them to survive in the field even when pest populations are low (McMurtry and Croft, 1997; Reis and Alves, 1997; Sarmento et al., 2011; Carrillo and Peña, 2012; Gerson and Weintraub, 2012). The presence of alternative prey, or/and the provision of alternative foods (i.e., pollen) in the context of conservation biological control, may play an important role in the ability of A. largoensis to persist on citrus plants and provide long-term B. yothersi suppression.
Research findings highlight the potential for enhancing the selectivity of acaricides towards A. largoensis by manipulating the way predators are exposed to them. For instance, direct and residual contact with abamectin had little effect on A. largoensis; therefore, the predator can be released before or after applications with this pesticide. By contrast, direct contact was the most harmful exposure route for A. largoensis to spirodiclofen. Therefore, predator releases should be done after the spirodiclofen applications. In addition, residual contact with fenpyroximate was slightly harmful to A. largoensis, suggesting that predators can be released safely after fenpyroximate application. The exposure route appears less relevant when applying cyflumetofen, which was moderately harmful to A. largoensis via the different exposure routes.
The results of this study provided an outline to design conservation biocontrol strategies in areas infested with B. yothersi and its associated viruses. In Florida, a strain of citrus leprosis virus C2 infecting hibiscus is highly associated with B. yothersi (Roy et al., 2018b; Roda et al., 2022). In the same area, A. largoensis is the most common predatory mite in backyard-grown citrus, hibiscus, and other plants in the landscape infested with B. yothersi, making it a promising biological control strategy for managing this pest (Argolo et al., 2020. Childers et al., 2022). The present study suggests potential compatibility between acaricides and A. largoensis depending on the route of exposure. Spirodiclofen and abamectin appear safer for A. largoensis than fenpyroximate and cyflumetofen. However, it is essential to highlight that the effects of sublethal concentrations of pesticides can be significant (Desneux et al., 2007), and these are unknown for A. largoensis. Assessing sublethal effects of acaricides on the physiology and behavior of A. largoensis may be crucial for establishing IPM programs combining chemical and biological control strategies to manage citrus leprosis vectors.
Data availability statement
The raw data supporting the conclusions of this article will be made available by the authors, without undue reservation.
Author contributions
JD: Conceptualization, Investigation, Writing original draft, Writing – review & editing. DA: Conceptualization, Investigation, Writing – review & editing. AT: Investigation, Writing – review & editing. AR: Funding acquisition, Writing – review & editing. ES: Formal Analysis, Writing – review & editing. DC: Conceptualization, Funding acquisition, Investigation, Resources, Supervision, Writing – original draft, Writing – review & editing.
Funding
The author(s) declare financial support was received for the research, authorship, and/or publication of this article. This research was funded by two USDA APHIS-UF Cooperative Agreements (FAIN: AP19PPQS&T00C115 & AP18PPQS& T00C125). The U.S. Department of Agriculture has not formally disseminated the findings and conclusions in this preliminary publication and should not be construed to represent any Agency determination or policy. Mention of trade names or commercial products in this publication is solely to provide specific information and does not imply recommendation or endorsement by the USDA; USDA is an equal opportunity provider and employer. We thank the Coordination for the Improvement of Higher Education Personnel (CAPES) for granting the scholarship to DA (88887.571009/2020-00).
Conflict of interest
The authors declare that the research was conducted in the absence of any commercial or financial relationships that could be construed as a potential conflict of interest.
Publisher’s note
All claims expressed in this article are solely those of the authors and do not necessarily represent those of their affiliated organizations, or those of the publisher, the editors and the reviewers. Any product that may be evaluated in this article, or claim that may be made by its manufacturer, is not guaranteed or endorsed by the publisher.
References
Abbott W. S. (1925). A method of computing the effectiveness of insecticide. J. Econ. Entomol. 18, 265–267. doi: 10.1093/jee/18.2.265a
Alvarez-Quinto R. A., Grinstead S., Rott P., Mollov D. (2022). Genome characterization and complete sequence of a new badnavirus from Pandanus amaryllifolius. Arch. Virol. 167, 1717–1720. doi: 10.1007/s00705-022-05480-0
Alves E. B., Omoto C., Franco C. R. (2000). Resistência cruzada entre o dicofol e outros acaricidas em Brevipalpus phoenicis (Geijskes) (Acari: Tenuipalpidae). Anais da Sociedade Entomológica do Brasil 29, 765–771. doi: 10.1590/S0301-80592000000400017
Amorim L. C. S., Silva J. L., Gravena S., Benvenga S. R., Araujo Júnior N. (2006). Efeito de acaricidas sobre ovos do ácaro-da-leprose dos citros, em diferentes idades. Laranja 27, 231–242.
Andrade D. J., Oliveira C. A. L., Santos N. C., Morais M. R. (2010). Toxicidade diferencial de produtos a base de abamectina ao ácaro Brevipalpus phoenicis em citros. Rev. Bras. Fruticultura 32, 82–89. doi: 10.1590/S0100-29452010005000024
Argolo P. S., Revynthi A. M., Canon M. A., Berto M. M., Andrade D. J., Doker I., et al. (2020). Potential of predatory mites for biological control of Brevipalpus yothersi (Acari: Tenuipalpidae). Biol. Control 149, 104330. doi: 10.1016/j.biocontrol.2020.104330
Assis C. P. O., Morais E. G. F., Gondim M. G. C. Jr. (2013). Toxicity of acaricides to Raoiella indica and their selectivity for its predator, Amblyseius largoensis (Acari: Tenuipalpidae: Phytoseiidae). Exp. Appl. Acarol 60, 357–365. doi: 10.1007/s10493-012-9647-5
Bajda S. A., Clercq P. D., Van Leuween T. (2022). Selectivity and molecular stress responses to classical and botanical acaricides in the predatory mite Phytoseiulus persimilis Athias-Henriot (Acari: Phytoseiidae). Pest Manage. Sci. 78, 881–895. doi: 10.1002/ps.6747
Barros M. E. N., Silva F. B., Sousa-Neto E. P., Bisneto M. C. R., Lima D. L., Melo J. W. S. (2022). Acaricide-impaired functional and numerical responses of the predatory mite, Amblyseius largoensis (Acari: Phytoseiidae) to the pest mite Raoiella indica (Acari: Tenuipalpidae). Syst. Appl. Acarol. 27, 33–44. doi: 10.11158/saa.27.1.4
Bassanezi R. B., Czermainski A. B. C., Laranjeira F. F., Moreira A. S., Ribeiro Júnior P. J., Krainski E. T., et al. (2019). Spatial patterns of the Citrus leprosis virus and its associated mite vector in systems without intervention. Plant Pathol. 68, 85–93. doi: 10.1111/ppa.12930
Bastianel M., Novelli V. M., Kitajima E. W., Kubo K. S., Bassanezi R. B., MaChado M. A., et al. (2010). Citrus leprosis: centennial of an unusual mite virus pathosystem. Plant Dis. 94, 284–292. doi: 10.1094/PDIS-94-3-0284
Bergeron P. E., Schmidt-Jeffris R. A. (2020). Not all predators are equal: miticide non-target effects and differential selectivity. Pest Manage. Sci. 76, 2170–2179. doi: 10.1002/ps.5754
Bielza P. (2016). “Insecticide resistance in natural enemies,” in Advances in Insect Control and Resistance Management. Eds. Horowitz R., Ishaaya I. (Switzerland: Springer), 313–329.
Bielza P., Balanza V., Cifuentes D., Mendoza J. E. (2020). Challenges facing arthropod biological control: identifying traits for genetic improvement of predators in protected crops. Pest Manag Sci. 76, 3517–3526. doi: 10.1002/ps.5857
Campos F., Omoto C. (2002). Resistance to hexythiazox in Brevipalpus phoenicis (Acari: Tenuipalpidae) from Brazilian citrus. Exp. Appl. Acarol. 26, 243–251. doi: 10.1023/A:1021103209193
Carrillo D., Amalin D., Hosein F., Roda A., Duncan R. E., Peña J. E. (2012b). Host plant range of Raoiella indica (Acari: Tenuipalpidae) in areas of invasion of the New World. Exp. Appl. Acarol. 57, 271–289. doi: 10.1007/s10493-011-9487-8
Carrillo D., De Coss M. E., Hoy M. A., Peña J. E. (2012a). Variability in response of four populations of Amblyseius largoensis (Acari: Phytoseiidae) to Raoiella indica (Acari: Tenuipalpidae) and Tetranychus gloveri (Acari: Tetranychidae) eggs and larvae. Biol. Control 60, 39–45. doi: 10.1016/j.biocontrol.2011.09.002
Carrillo D., Peña J. E. (2012). Prey-stage preferences and functional and numerical responses of Amblyseius largoensis (Acari: Phytoseiidae) to Raoiella indica (Acari: Tenuipalpidae). Exp. Appl. Acarol. 57, 361–372. doi: 10.1007/s10493-011-9488-7
Carrillo D., Frank J. H., Rodrigues J. C. V., Peña J. E. (2012). A review on the natural enemies of the red palm mite, Raoiella indica (Acari: Tenuipalpidae). Exp. Appl. Acarol. 57, 347–360.
Carrillo D., Peña J. E., Hoy M. A., Frank J. H. (2010). Development and reproduction of Amblyseius largoensis (Acari: Phytoseiidae) feeding on pollen, Raoiella indica (Acari: Tenuipalpidae), and other micro-arthropods inhabiting coconuts in Florida, U.S. Exp. Appl. Acarol. 52, 119–129.
Carrillo D., Hoy M. A., Peña J. E. (2014). Effect of Amblyseius largoensis (Acari: Phytoseiidae) on Raoiella indica (Acari: Tenuipalpidae) by predator exclusion and predator release techniques. Florida Entomologist 97 (1), 256–261.
Castillo I. I., Diaz L. F. Z., Mendez W., Otero-Colina G., Freitas-Astua J., Locali-Fabris E. C., et al. (2011). Confirmation of the presence of the Citrus leprosis virus C (CiLV-C) in Southern Mexico. Trop. Plant Pathol. 36, 400–403.
Childers C. C., Ueckermann E. A., De Moraes G. J. (2022). Phytoseiidae on citrus in Florida dooryard, varietal, and commercial trees between 1951 and 2014, and species recommendations for evaluation in citrus under protective screen (CUPS). Florida Entomologist 105 (1), 27–36. doi: 10.1653/024.105.0105
Cote K. W., Lewis E. E. L., Schultz P. B. (2022). Compatibility of acaricide residues with Phytoseiulus persimilis and their effects on Tetranychus urticae. HortScience 37, 906–909. doi: 10.21273/HORTSCI.37.6.906
Della Vechia J. F., Andrade D. J. (2022). Effect of acaricide and insecticide mixtures against citrus leprosis vector, Brevipalpus yothersi, under laboratory and field conditions. Crop Protect 161, 106074. doi: 10.1016/j.cropro.2022.106074
Della Vechia J. F., Kapp A. B. P., Rocha C. M. (2022). The importance of acaricides in the control of citrus leprosis mite and factors that interfere with the efficacy. Citrus Res. Technol. 43, e1074. doi: 10.4322/crt.23021
Desneux N., Decourtye A., Delpuech J. M. (2007). The sublethal effects of pesticides on beneficial arthropods. Ann. Rev. Entom. 52, 81–106. doi: 10.1146/annurev.ento.52.110405.091440
Dey K. K., Velez-Climent M., Padmanabhan C., Nunziata S., Rivera Y., McVay J., et al. (2022). Smilax auriculata: A new host for Orchid fleck dichorhavirus identified in Florida, USA. Plant Dis. 106, 2271. doi: 10.1094/PDIS-09-21-2085-PDN
Dietzgen R. G., Freitas-Astúa J., Chabi-Jesus C., Ramos-González P. L., Goodin M. M., Kondo H., et al. (2018). Dichorhaviruses in their host plants and mite vectors. Adv. Virus Res. 102, 119–148. doi: 10.1016/bs.aivir.2018.06.001
Doker I., Kazak C. (2019a). Non-target effects of five acaricides on a native population of Amblyseius swirskii (Acari: Phytoseiidae). Int. J. Acarol. 45, 69–74. doi: 10.1080/01647954.2018.1542457
Doker I., Kazak C. (2019b). Toxicity and risk assessment of acaricides on the predatory mite, Euseius scutalis (Athias-Henriot) (Acari: Phytoseiidae) under laboratory conditions. Chemosphere 261, 127760. doi: 10.1016/j.chemosphere.2020.127760
Duso C., Van Leeuwen T., Pozzebon A. (2020). Improving the compatibility of pesticides and predatory mites: recent findings on physiological and ecological selectivity. Curr. Opin. Insect. Sci. 39, 63–68. doi: 10.1016/j.cois.2020.03.005
Fernández M. M., Medina P., Wanumen A., Del Estal P., Smagghe G., Viñuela E. (2017). Compatibility of sulfoxaflor and other modern pesticides with adults of the predatory mite Amblyseius swirskii. Residual contact and persistence studies. BioControl 62, 197–208. doi: 10.1007/s10526-017-9784-1
Fife A., Carrillo D., Knox G., Iriarte F., Dey K., Roy A., et al. (2021). Brevipalpus-transmitted orchid fleck virus infecting three new ornamental hosts in Florida. J. Integrated Pest Manage. 12, 43. doi: 10.1093/jipm/pmab035
Galvão A., Gondim M. G. C. Jr., Moraes G. J., Oliveira J. (2007). Biology of Amblyseius largoensis (Muma) (Acari: Phytoseiidae), a potential predator of Aceria guerreronis Keifer (Acari: Eriophyidae) on coconut trees. Neotrop. Entomol. 36, 465–470. doi: 10.1590/S1519-566X2007000300016
Galvão A. S., Gondim Junior M. G. C., Moraes G. J. D., Oliveira J. V. D. (2008). Exigências térmicas e tabela de vida de fertilidade de Amblyseius largoensis. Ciec. Rural 38, 1817–1823. doi: 10.1590/S0103-84782008000700003
Gerson U., Weintraub P. G. (2012). Mites (Acari) as a factor in greenhouse management. Annu. Rev. Entomol. 57, 229–247. doi: 10.1146/annurev-ento-120710-100639
Ghasemzadeh S., Qhreshi J. A. (2018). Demographic analysis of fenpyroximate and thiacloprid exposed predatory mite Amblyseius swirskii (Acari: Phytoseiidae). PloS One 13 (11), e0206030. doi: 10.1371/journal.pone.0206030
Groot T. V., Breeuwer J. A. (2006). Cardinium symbionts induce haploid thelytoky in most clones of three closely related Brevipalpus species. Exp. Appl. Acarol. 39, 257–271. doi: 10.1007/s10493-006-9019-0
Haramoto F. H. (1969). Biology and control of Brevipalpus phoenicis (Geijskes) (Acarina: Tenuipalpidae). Tech. Bull. - Hawaii Agric. Exp. Stn. 68, 1–63.
Hassan S. A., Bigler F., Bogenschutz H., Boller E., Brun J., Calis J. N. M., et al. (1994). Results of the sixth joint pesticide testing programme of the IOBC/WPRS working group' Pesticides and Beneficial Organisms'. Entomophaga 39, 107–119. doi: 10.1007/BF02373500
IRAC Insecticide Resistance Action Committee. Available at: http://www.iraconline.org/content/uploads/IRAC_MixtureStatement_v1.0_10Sep12.pdf (Accessed 20 jan. 2023).
Irigaray F.J.S.D.C., Zalom F. G. (2007). Selectivity of acaricide exposure on Galendromus occidentalis reproductive potential. Biocontrol Sci. Technol. 17, 541–546. doi: 10.1080/09583150701311820
Kamburov S. S. (1971). Feeding, development, and reproduction of Amblyseius largoensis on various food substances. J. Econ. Entomol. 63, 643–648. doi: 10.1093/jee/64.3.643
Kaplan P., Yorulmaz S., Ay R. (2012). Toxicity of insecticides and acaricides to the predatory mite Neoseiulus californicus (McGregor) (Acari: Phytoseiidae). Int. J. Acarol. 38, 699–705. doi: 10.1080/01647954.2012.719031
Kim S. S., Paik C. H. (1996). Comparative toxicity of fenpyroximate to the predatory mite, Amblyseius womersleyi Schicha and the kanzawa spider mite, Tetranychus kanzawai Kishida (Acarina: Tetranychidae, Phytoseiidae). Appl. Entomol. Zool. 31, 369–377. doi: 10.1303/aez.31.369
Kishimoto H., Yaginuma K., Toyama M. (2018). Effects of pesticides on four native generalist phytoseiid species (Acari: Phytoseiidae). Japan J. Appl. Entomol. Zool. 62, 29–39. doi: 10.1303/jjaez.2018.29
Kitajima E., Chagas C., Rodrigues J. (2003). Brevipalpus-transmitted plant virus and virus-like diseases: cytopathology and some recent cases. Exp. Appl. Acarol. 30, 135–160. doi: 10.1023/B:APPA.0000006546.55305.e3
Lee S. M., Kim S. S. (2015). Susceptibility of the predatory mite, Neoseiulus californicus (Acari: Phytoseiidae) to acaricides.Korean J. Pestic. Sci. 19, 418–423.
Leung K., Ras E., Ferguson K., Ariëns S., Babendreier D. B., Bijma P., et al. (2020). Next generation biological control: the need for integrating genetics and genomics. Biol. Rev. 95, 1838–1854. doi: 10.1111/brv.12641
Lima D. B., Melo J. W. S., Guedes R. N., Siqueira H. A., Pallini A., Gondim M. G. C. Jr. (2013). Survival and behavioural response to acaricides of the coconut mite predator Neoseiulus baraki. Exp. Appl. Acarol. 60, 381–393. doi: 10.1007/s10493-012-9644-8
Locali-Fabris E. C., Freitas-Astúa J., Souza A. D., Takita M. A., Astúa-Monge G., Antonioli-Luizon R., et al. (2006). Complete nucleotide sequence, genomic organization and phylogenetic analysis of Citrus leprosis virus cytoplasmic type. J. Gen. Virol. 87, 2721–2729. doi: 10.1099/vir.0.82038-0
Longley M., Stark J. D. (1996). Analytical techniques for quantifying direct, residual, and oral exposure of an insect parasitoid to an organophosphate insecticide. Bull. Environ. Contam. Toxicol. 57, 683–690. doi: 10.1007/s001289900244
McMurtry J. A., Croft B. A. (1997). Life-styles of phytoseiid mites and their roles in biological control. Annu. Rev. Entomol. 42, 291–321. doi: 10.1146/annurev.ento.42.1.291
Melo J. W. S., Lima D. B., Staudacher H. R., Silva F. R., Gondim M. G. C. Jr., Sabelis M. W. (2015). Evidence of Amblyseius largoensis and Euseius alatus as biological control agent of Aceria guerreronis. Exp. Appl. Acarol 67, 411–421. doi: 10.1007/s10493-015-9963-7
Melzer M. J., Sether D. M., Borth W. B., Hu J. S. (2012). Characterization of a virus infecting Citrus volkameriana with citrus leprosis-like symptoms. Phytopathology 102, 122–127. doi: 10.1094/PHYTO-01-11-0013
Milliken G. A., Johnson D. E. (2009). Analysis of messy data: Volume 1. Designed experiments (2nd) (Boca Roaton, Florida, United States: CRC Press).
Moraes G. J., McMurtry J. A., Denmark H. A., Campos C. B. (2004). A revised catalog of the mite family Phytoseiidae. Zootaxa 434, 1–494. doi: 10.11646/zootaxa.434.1.1
Moreira R. R., MaChado F. J., Lanza F. E., Trombin V. G., Bassanezi R. B., de Miranda M. P., et al. (2022). Impact of diseases and pests on premature fruit drop in sweet orange orchards in São Paulo state citrus belt, Brazil. Pest Manage. Sci. 78 (6), 2643–2656. doi: 10.1002/ps.6894
Olmedo-Velarde A., Hu J., Melzer M. J. (2021b). A virus infecting hibiscus rosa-sinensis represents an evolutionary link between cileviruses and higreviruses. Front. Microbiol. 12, 660237. doi: 10.3389/fmicb.2021.660237
Olmedo-Velarde A., Roy A., Padmanabhan C., Nunziata S., Nakhla M. K., Melzer M. J. (2021a). First report of Orchid fleck virus associated with Citrus leprosis symptoms in rough lemon (Citrus jambhiri) and mandarin (C. reticulata) the United States. Plant Dis. 105, 2258. doi: 10.1094/PDIS-12-20-2736-PDN
Omoto C., Alves E. B., Ribeiro P. C. (2000). Detection and monitoring of resistance in Brevipalpus phoenicis (Geijskes) (Acari: Tenuipalpidae) to dicofol. Anais da Sociedade Entomológica do Brasil 29, 757–764. doi: 10.1590/S0301-80592000000400016
Overmeer W. P. J. (1985). “Rearing and handling,” in World Crop Pests. Spider Mites: Their Biology, Natural Enemies and Control, vol. 1B . Eds. Helle W., Sabelis M. W. (Amsterdam, The Netherlands: Elsevier), 161–169.
Peña J. E., Santos K., Baez I., Carrillo D. (2015). Physical post-harvest techniques as potential quarantine treatments against Brevipalpus yothersi (Acarina: Tenuipalpidae). Florida Entomol 98 (4), 1169–1174.
Pozzebon A., Duso C., Tirello P., Ortiz P. B. (2011). Toxicity of thiamethoxam to Tetranychus urticae Koch and Phytoseiulus persimilis Athias-Henriot (Acari Tetranychidae, Phytoseiidae) through different routes of exposure. Pest Manage. Sci. 67, 352–359. doi: 10.1002/ps.2072
Quito-Avila D. F., Freitas-Astúa J., Melzer M. J. (2021). “Bluner-, cile-, and higreviruses (kitaviridae),” Encyclopedia of Virology, eds Bamford D., Zuckerman M. (Amsterdam: Elsevier), 247–251. doi: 10.1016/B978-0-12-809633-8.21248-X
Ramos-González P. L., Arena G. D., Tassi A. D., Chabi-Jesus C., Kitajima E. W., Freitas-Astúa J. (2023). Kitaviruses: A window to atypical plant viruses causing nonsystemic diseases. Annu. Rev. Phytopathol. 61, 97–118. doi: 10.1146/annurev-phyto-021622-121351
Ramos-González P., Chabi-Jesus C., Arena G. D., Tassi A., Kitajima E. W., Freitas-Astúa J. (2018). Citrus leprosis: a unique multietiologic disease/Leprosis de los cítricos: una enfermedad multietiológica singular. Cítricos en las Américas 1, 4–19.
Reis P. R., Alves E. B. (1997). Biologia de Euseius alatus Deleon (Acari: Phytoseiidae). An. Soc Ent. Bras. 26, 359–363. doi: 10.1590/S0301-80591997000200018
Revynthi A. M., Peña J. E., Moreno J. M., Beam A. L., Mannion C., Bailey W. D., et al. (2019). Effectiveness of hot-water immersion against brevipalpus yothersi (Acari: tenuipalpidae) as a postharvest treatment for lemons. J. . Econ. Entomol 113 (1), 126–133. doi: 10.1093/jee/toz258
Rocha C. M., Della Vechia J. F., Savi P. J., Omoto C., Andrade D. J. (2021). Resistance to spirodiclofen in Brevipalpus yothersi (Acari: Tenuipalpidae) from Brazilian citrus groves: detection, monitoring, and population performance. Pest Manage. Sci. 77, 3099–3106. doi: 10.1002/ps.6341
Roda A. L., Allen C. T., Carrillo D., Nachman G. (2022). Effects of weather and predators on the population Brevipalpus yothersi (Acari: Tenuipalpidae) in south Florida hibiscus hedges. Zoosymposia 22, 74–74. doi: 10.11646/zoosymposia.22.1.39
Rodrigues V., Bastianel M., Kubo K. S., Fadel A. L., Nicolini F., Novelli V. M., et al. (2007). Desenvolvimento de um método para a otimização da transmissão experimental do vírus da leprose dos citros. Laranja 28, 29–38.
Rodrigues J. C. V., Kitajima E. W., Childers C. C., Chagas C. M. (2003). Citrus leprosis virus vectored by Brevipalpus phoenicis (Acari: Tenuipalpidae) on citrus in Brazil. Exp. Appl. Acarol. 30, 161–179. doi: 10.1023/B:APPA.0000006547.76802.6e
Rodríguez H. C., Montoya A., Miranda I., Rodríguez Y. S., Depestre T., Ramos M. S., et al. (2015). Biological control of Polyphagotarsonemus latus (Banks) by the predatory mite Amblyseius largoensis (Muma) on sheltered pepper production in Cuba. Rev. Protección Veg. 30, 70–76.
Rodríguez H. M., Ramos M., Montoya A., Rodríguez Y., Chico R., Miranda I., et al. (2011). Development of Amblyseius largoensis as biological control agent of the broad mites (Polyphagotarsonemus latus). Biotecnol. Appl. 28, 171–175.
Roy A., Choudhary N., Guillermo L. M., Shao J., Govindarajulu A., Achor D., et al. (2013). A novel virus of the genus Cilevirus causing symptoms similar to citrus leprosis. Phytopathology 103, 488–500. doi: 10.1094/PHYTO-07-12-0177-R
Roy A., Hartung J. S., Schneider W. L., Shao J., Leon G., Melzer M. J., et al. (2015). Role bending: complex relationships between viruses, hosts, and vectors related to citrus leprosis, an emerging disease. Phytopathol 105, 1013–1025. doi: 10.1094/PHYTO-12-14-0375-FI
Roy A., Stone A. L., Melzer M. J., Hartung J. S., Mavrodieva V. A., Nakhla M. K., et al. (2018a). First report of a cilevirus associated with green ringspot on senescent hibiscus leaves in Tampa, Florida. Plant Dis. 102, 1181. doi: 10.1094/PDIS-11-17-1699-PDN
Roy A., Stone A. L., Melzer M. J., Shao J., Hartung J. S., Mavrodieva V., et al. (2018b). Complete nucleotide sequence of a novel Hibiscus-infecting Cilevirus from Florida and its relationship with closely associated Cileviruses. Genome Announcements 6, e01521–e01517. doi: 10.1128/genomeA.01521-17
Sarmento R. A., Rodrigues D. M., Faraji F., Erasmo E. A. L., Lemos F., Teodoro A. V., et al. (2011). Suitability of the predatory mites Iphiseiodes zuluagai and Euseius concordis in controlling Polyphagotarsonemus latus and Tetranychus bastosi on Jatropha curcas plants in Brazil. Exp. Appl. Acarol 53, 203–214. doi: 10.1007/s10493-010-9396-2
Sato M. E., Da Silva M., Goncalves L. R., De Souza Filho M. F., Raga A. (2002). Differential toxicity of pesticides to Neoseiulus californicus (McGregor) (Acari: Phytoseiidae) and Tetranychus urticae Koch (Acari: Tetranychidae) on Strawberry. Neotrop. Entomol. 31, 449–456. doi: 10.1590/S1519-566X2002000300016
Saville D. J. (2015). Multiple comparison procedures - cutting the Gordian knot. Agron. J. 107, 730–735. doi: 10.2134/agronj2012.0394
Silva M. Z., Sato M. E., Oliveira C. A. L. (2012). Diversidade e dinâmica populacional de ácaros em pomar cítrico. Bragantia 71, 210–218. doi: 10.1590/S0006-87052012005000013
Van Leeuwen T., Dermauw W. (2016). The molecular evolution of xenobiotic metabolism and resistance in chelicerate mites. Annu. Rev. Entomol 61, 475–498. doi: 10.1146/annurev-ento-010715-023907
Keywords: acaricide selectivity, Amblyseius largoensis, biological control, Brevipalpus yothersi, exposure, integrated pest management
Citation: Vechia JFD, Andrade DJ, Tassi AD, Roda A, van Santen E and Carrillo D (2023) Can predatory mites aid in the management of the citrus leprosis mite? Front. Agron. 5:1304656. doi: 10.3389/fagro.2023.1304656
Received: 29 September 2023; Accepted: 06 November 2023;
Published: 21 November 2023.
Edited by:
David Ezra, Agricultural Research Organization (ARO), IsraelReviewed by:
Qiong Rao, Zhejiang Agriculture and Forestry University, ChinaSpiridon Mantzoukas, University of Ioannina, Greece
Copyright © 2023 Vechia, Andrade, Tassi, Roda, van Santen and Carrillo. This is an open-access article distributed under the terms of the Creative Commons Attribution License (CC BY). The use, distribution or reproduction in other forums is permitted, provided the original author(s) and the copyright owner(s) are credited and that the original publication in this journal is cited, in accordance with accepted academic practice. No use, distribution or reproduction is permitted which does not comply with these terms.
*Correspondence: Daniel Carrillo, dancar@ufl.edu