- 1Department of Plant Pathology, Southwest Florida Research and Education Center, University of Florida, Immokalee, FL, United States
- 2Biologics Department, Bayer Crop Science, Woodland, CA, United States
- 3Ginkgo Bioworks, West Sacramento, CA, United States
- 4Bayer Crop Science, Lyon, France
For over two decades, Florida citrus growers have been heavily using copper sprays to combat citrus canker (Xanthomonas citri subsp. citri; Xcc), but it raises the risk of developing resistant bacterial strains and excessive accumulation in the soil. Alternative methods are needed to reduce copper dependency and better manage Xcc. Therefore, we screened copper-alternative products against canker on young seedlings in the greenhouse and mature trees in a commercial grove in Florida, USA. In the greenhouse, we evaluated the efficacy of single foliar application of new plant defense inducers (PDI), ‘PDI5’, ‘PDI26’, and ‘PDI31’, and a commercially available systemic acquired resistance inducer, Actigard (drench; 2g/tree), applied two days before injection-infiltration with citrus canker bacterium Xcc (104 CFU/ml), on two-year-old Valencia trees. All three PDIs, sprayed at 500 ppm, as well as Actigard (drench), effectively reduced canker severity on the inoculated leaves in the greenhouse (P ≤0.02). In the grove, we tested new antimicrobials, Mastercop ‘MC’ (21.46% copper sulfate pentahydrate; metallic copper equivalent 5.4%) at concentrations of 1.12 kg/ha, 1.68 kg/ha and 2.24 kg/ha, ‘AM’ at 1.68 kg/ha and 2.24 kg/ha, and a nutrient solution (AGRO-MOS) at 1.12 kg/ha for canker suppression on 4–6-year-old Hamlin trees. Kocide 2000 (growers’ standard control; metallic copper equivalent 30%; 3.92 kg/ha) was used as a control check. Although 2021 was not as conducive for citrus canker due to the relatively dry spring months in the field, our results showed that the six sprays of AM (2.24 kg/ha) or MC (1.68 kg/ha, and 2.24 kg/ha), in 2022, provided excellent control of Xcc, with a significant reduction in disease incidence in leaves and fruits, and disease severity in fruits, similar to Kocide 2000. The results indicate that antimicrobials, AM and MC, and plant defense inducers PDI5, PDI26, and PDI31 can be part of an integrated pest management (IPM) program with copper for managing citrus canker. Reducing the frequency and amount of copper applications can help reduce pesticide and application expenses, lower risks of copper buildup in the soil and toxicity for citrus trees and reduce the risk of the emergence of copper-resistant Xcc strains. Further research on these products is needed to assess their long-term effectiveness and potential environmental risks.
1 Introduction
Citrus canker, caused by the bacterium Xanthomonas citri subsp. citri (Xcc), is a contagious plant disease that can affect most commercial citrus cultivars (Graham et al., 2004; Ritenour et al., 2008; Graham et al., 2016b). Early-season sweet oranges (Citrus sinensis), including ‘Hamlin,’ ‘Pineapple,’ and Navels, and Grapefruit (Citrus x paradisi) are most susceptible to citrus canker, which makes it challenging to grow these citrus types profitably in areas with a humid tropical and subtropical climate, that provides suitable environmental conditions for bacterial growth and multiplication (Gottwald et al., 2005; Graham et al., 2016a). The citrus canker spreads hastily over short distances and causes symptoms such as premature leaf and fruit drops, blemished fruit, twig dieback, and general tree decline. The bacteria primarily originate from diseased trees within the grove and spread to uninfected areas through wind-driven rain, irrigation, mechanical transmission (e.g., by farm equipment and contaminated tools), and movement of infected plant materials within and between groves (Gottwald and Irey, 2007).
This disease was introduced to Florida in 1910 through trifoliate rootstock seedlings imported from Japan (Chamberlain et al., 2003). From this first introduction, the disease spread around the Gulf Coast from Texas to Florida and further north to South Carolina. Between 1915 and 1994, various attempts were made to eradicate citrus canker from Florida using quarantines and eradication programs (Schubert et al., 2001; Chamberlain et al., 2003). However, these eradication efforts were unsuccessful as the hurricanes from 2004–2005 spread the disease from ten counties in Florida to all major production areas in twenty-five counties, where the disease is endemic today (Gottwald and Irey, 2007). Each year across the globe, millions of dollars are spent on disease management, quarantines, eradication, and prevention programs designed to prevent citrus canker from becoming endemic (Das, 2003). The biggest economic impacts caused by citrus canker infestation are restrictions on international shipments and the sale of fruit from areas affected by citrus canker (Graham et al., 2016b).
Currently, the main strategy to manage citrus canker is using multiple applications of products that contain copper every twenty-one-day and using windbreaks that limit the dispersal of inoculum (Leite Jr and Mohan, 1990; Graham et al., 2004; Behlau et al., 2008; Graham and Myers, 2013). Although copper bactericide sprays are an effective method for controlling canker, they are costly, provide only preventive care with no curative or systemic activity, and require repeated applications as the fruit tissues expand. They only provide good protection to the expanding fruit from infection if the surface film coverage is continuous with no gaps (Behlau et al., 2008; Behlau et al., 2010; Graham et al., 2010; Graham et al., 2016a). It is crucial to time the copper sprays correctly before late March and early April rainfall to protect fruit that are 0.5–1.0 cm in diameter, when fruits are most susceptible to disease in Florida growing conditions. Using copper bactericides on grapefruit throughout the season, especially during hot and dry periods, can cause phytotoxicity to the fruit’s rind (Graham et al., 2008). Some growers choose not to use bactericides due to the potential risks associated with the long-term use of copper. These risks include the development of a copper-resistant strain of the bacterium and the harmful accumulation of copper in the soil, which can negatively impact the tree’s health and environment (Alva et al., 1995; Canteros et al., 1995; Villamizar and Caicedo, 2020). The use of copper bactericides also negatively affect the useful soil microbiota and overall soil health (Van-Zwieten et al., 2004). Therefore, growers are interested in finding alternative products to reduce copper dependency.
In the past, a few antibiotics have been tested to supplement/alternate copper sprays for citrus canker control, including Streptomycin sulphate (Agrimicina, containing 15% streptomycin and 1.5% oxytetracycline) that was found to be as effective as using intermediate rates of copper hydroxide to manage canker in groves (Graham et al., 2007; Graham et al., 2008; Graham et al., 2010). Alternating the use of Streptomycin sulphate (Firewall, containing 17% streptomycin) with a lower amount of copper hydroxide provided a comparable reduction of fruit symptoms without causing as much copper burn as using the full amount of copper hydroxide or copper sulphate alone. However, the greatest concern about spraying large amounts of streptomycin or other such antibiotics on citrus trees is that it may lead to antibiotic-resistant pathogens emerging in the soil and nearby waterways (Canteros et al., 1995). Apart from antibiotics and antimicrobials, plant defense inducers (PDIs) products have also been developed to reduce the spread of bacterial canker in citrus (Francis et al., 2009; Graham and Myers, 2013; Graham and Myers, 2016). PDIs are compounds that stimulate or enhance the natural plant defense mechanisms before the onset of the disease but have no direct effect on the pathogen (Vallad and Goodman, 2004; Graham and Myers, 2013; Bagio et al., 2016). One common PDI, Actigard (acibenzolar-S-methyl; 50 WP; Syngenta Crop Protection, Greensboro, NC), is a systemic acquired resistance (SAR) inducer, which limits the establishment of citrus canker in the non-bearing stage as well as limit the development of lesions on foliage during the bearing stage, thereby reducing the potential for fruit infection (Dewdney et al., 2022). However, previous studies have shown that the protective effects of Actigard only last a few weeks. In Brazil, spraying Actigard and copper on leaves throughout the season did not provide better disease control than using copper alone (Graham and Leite Jr, 2004). PDIs activate a broad-spectrum defense response that protects against various diseases and is believed to be more environmentally friendly and sustainable than copper-based products (Alva, 1993; Alva et al., 1995; Behlau et al., 2021). Other systemic resistance-inducing products, such as neonicotinoids alone or in rotation with Actigard, also provided good efficacy against citrus canker in the grove (Graham and Myers, 2013). It is necessary to identify new PDIs against citrus canker and compatible with other products to make a long-term control strategy feasible.
This study aims to evaluate new antimicrobials and plant defense inducers (PDIs) for their potential to be used as an alternative or supplement to copper for control of citrus canker in nurseries and in commercial citrus groves without phytotoxicity. Reducing the rates and frequency of copper to manage citrus canker with alternative or supplement products is expected to help minimize the risks of bacterial resistance to copper, copper phytotoxicity (burn) to the fruit rind, and harmful copper accumulation in the soil and environment.
2 Materials and methods
2.1 Bacterial preparations
A bacterial strain X2002-0014 of Xcc isolated from sweet oranges in Dade County, Florida, was used in the greenhouse experiment (Graham et al., 2016b). The pure bacterial culture stored in glycerol at −80°C, and confirmed to be Xcc using PCR and sequencing was streaked in KCB (Kasugamycin-Cephalexin-Bravo) media and incubated at 28°C for 24 hours. A single colony was transferred to the nutrient broth and placed on a shaker at 200 rpm at 28°C. After 18 h, the bacterial suspension in nutrient broth was adjusted to an optical density of 0.1 at 600nm, corresponding to 108 CFU/ml, using PBS buffer. The bacterial suspension was diluted to 104 CFU/ml and used for infiltration inoculation in the greenhouse. The field experiments were not inoculated and were conducted with natural inoculum present in the field with no additional inoculum to the trees.
2.2 Greenhouse experiments
2.2.1 Plant genotype
In the greenhouse experiments, two-year-old ‘Valencia’ sweet orange [Citrus sinensis (L.) Osb.] seedlings, which are susceptible to citrus canker, grafted onto Volkamer (Citrus volkameriana) or sour orange (Citrus aurantium) rootstocks were used. All experiments were done in 15 cm × 41 cm plastic pots with a 3:1:1 ratio of sand, peat moss, and perlite. Three separate experiments were conducted using three plants per treatment and at least three leaves per plant were infiltrated with the bacterium as described below. Plants were supplemented weekly with 20-10-20 fertilizer at one gram per pot. The greenhouse was set to 27°C ± 2°C under natural sunlight conditions.
2.2.2 Test materials
Two separate greenhouse experiments were conducted with different sets of compounds. The test compounds in the first experiment consisted of a novel plant defense inducer (PDI5), sprayed at 500 ppm, and a commonly used defense inducer, Actigard (acibenzolar-S-methyl; Actigard 50 WP), applied as a soil drench (100 ml per plant; 2 gm/tree), two days before injection-infiltration with citrus canker. The second greenhouse experiment was conducted with two additional innovative plant defense inducers, ‘PDI26’ and ‘PDI31’, applied two days before injection-infiltration with citrus canker bacterium. All treatments were applied as a foliar spray using a handheld sprayer at ~20 ml per plant, except Actigard, which was applied as a soil drench. The test materials, PDI5, PDI26, and PDI31, were kindly provided by Bayer (Bayer CropScience, Lyon, France). Since these PDIs are propriety materials, their name, and the active ingredient are not released. Approximately four weeks before spraying the compounds, the plants were pruned and fertilized to induce a new flush of growth susceptible to new infections. When three to four leaves on the shoot reached three-fourths of full expansion, the emerging foliage was sprayed with the test compounds.
2.2.3 Bacterial inoculation
After 48 hours of the spray treatments, the plants were inoculated with Xcc strain (X2002-0014), using 104 colony forming units (CFU) per ml. To inoculate the plants, we injected three leaves per plant that had reached 75% expansion. The injection was done by infiltrating the abaxial surface of each of the three leaves per plant at six sites (three on each side of the mid-vein) until the water-soaked area extended approximately two mm beyond the tip of the syringe, consisting of approximately 10 μl of the bacterial suspension. The trees with the leaves infiltrated with PBS buffer were used as negative controls, as well as untreated trees infiltrated with Xcc were used as positive controls. The infiltration was done around 9 am to 10 am in the morning when the stomata were fully open. Three plants were used per treatment, with three leaves per plant and six inoculation sites per leaf (n = 56 infiltration sites). Following injection, the plants were covered with plastic bags for 24–72 hours to maintain high humidity conducive to bacterial growth and cell infection.
2.2.4 Canker symptoms assessment
Three to four weeks after leaf inoculation, the canker symptoms were assessed on the infiltrated sites, and the lesions were counted with the help of a hand lens (10× magnified). The average number of lesions on the leaf was calculated.
2.3 Field trials
2.3.1 Field trial site and plant genotype
The field experiments were conducted in 2021 and 2022 in a commercial citrus grove in Felda, in Hendry County, FL, using 4–6‐yr-old fruit bearing Hamlin (C. sinensis L. Osbeck) trees grafted onto Carrizo (C. sinensis’ Washington’ x Poncirus trifoliata) rootstock.
2.3.2 Pesticides tested
The test materials in 2021 trials consisted of labeled products Mastercop (Adama Ltd., USA) (21.46% copper sulfate pentahydrate; metallic copper equivalent 5.4%) applied at the concentrations of 1.12 kg/ha, and 1.68 kg/ha, a new antimicrobial compound (AM) (proprietary material provided by Alltech, USA) at 1.68 kg/ha, a nutrition solution (AGRO-MOS; based on Saccharomyces cerevisiae and consisting of 4% copper; Alltech) applied at 1.12 kg/ha, and Kocide 2000 (growers’ standard control; metallic copper equivalent 30%) applied at 3.36 kg/ha.
The test materials in 2022 trials consisted of MC applied at 1.68 kg/ha and 2.24 kg/ha, AM at 1.68 kg/ha and 2.24 kg/ha, and Kocide 2000 at 3.36 kg/ha. The treatment rates and frequencies are listed in Table 1. The test materials, PDIs, and AM, are propriety; thus, names are not released.
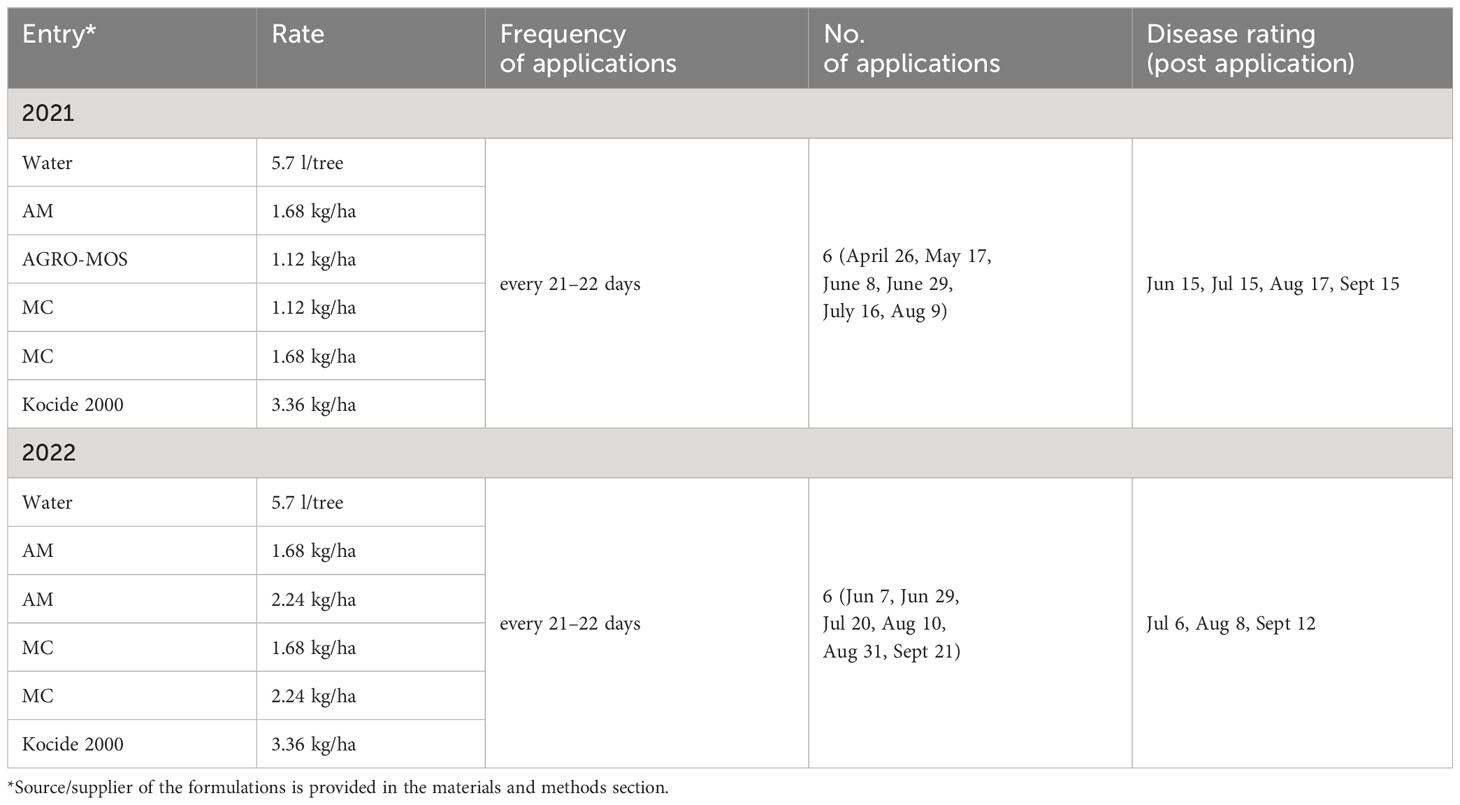
Table 1 Rates, application number, and frequency of antimicrobials, plant defense inducers, and copper formulation tested on the 4–6-year-old Hamlin (C. sinensis) trees in a commercial grove in Felda, FL, in 2021 and 2022.
2.3.3 Treatment of mature citrus trees in the grove
All field treatments were made as foliar spray using a commercial handgun 40-gallon sprayer with 21-day intervals, the first application starting late April in 2021 or early June in 2022, after the spring flush, and a total of 6 spray applications. The experiments were conducted in a completely randomized block design (CRBD) with five plots per treatment and five trees per plot. A single untreated tree was left as a buffer between the plots. The middle three trees in each plot were assessed monthly for fruit and leaf symptoms and fruit drop. The trees in the grove were managed using the grower’s standard pest management, fertilizer, and irrigation practices.
2.3.4 Disease monitoring and rating on fruits
The fruits were assessed monthly for canker lesions on 25 fruits from each side of the middle three trees (50 fruits per tree). The number of symptomatic fruits per tree was expressed as the incidence of fruit with canker lesions. The disease severity in each of the 50 fruits was also rated using a 0–4 disease rating scale (where 0 = no lesions, 1 = lesions present in 1 quarter of the fruit, 2 = lesions present in two-quarters of the fruit, 3 = lesions present in three-quarters of the fruit and 4 = disease present in all quarters of the fruits). The data were expressed as the disease severity index (DSI), which was calculated using the formula, DSI (%) = [sum (class frequency × score of rating class)]/[(total number of plants) × (maximal disease index)] × 100.
2.3.5 Fruit drop
All fruits on the ground under the middle three trees in each plot were evaluated for the presence or absence of canker lesions. After each rating, all the dropped fruits were counted and removed from underneath the tree every month. In 2021, fruit drops were evaluated monthly on June 15th, July 15th, August 17th, and September 15th. In 2022, fruit drops were also evaluated monthly on July 6th, August 8th, and September 12th. In 2021, only the symptomatic dropped fruits were counted, whereas, in 2022, all the dropped fruits with or no canker symptoms were counted.
2.3.6 Leaf symptoms
The number of symptomatic leaves showing canker lesions was counted per meter square canopy area using a 1 m × 1 m square frame from two sides of each of the middle three trees in each plot. In 2021, canker symptoms in the leaves were evaluated monthly on June 15th, July 15th, August 17th, and September 15th. In 2022, leaf symptoms were evaluated monthly on July 6th, August 8th, and September 12th.
2.3.7 Phytotoxicity assessment
All the trees in the greenhouse and field experiments were visually inspected and monitored for phytotoxicity symptoms such as chemical burn, chlorosis, reduced trunk diameter, browning of the leaf tips and overall leaf, twig dieback etc., throughout the experiments. The trunk diameter was measured using a caliper, and the SPAD 502 handheld chlorophyll meter was used to measure the relative chlorophyll content according to the manufacturer’s instructions (Konica Minolta, Tokyo, Japan).
2.4 Weather data
A monthly rainfall, wind speed, and relative humidity data in 2021 and 2022 was recorded at the Florida Agricultural Weather Network (FAWN) site in Immokalee, Florida, located 17 km (10.6 miles) from the trial site and compared with the average for the five years from 2018 to 2022. The weather data was expressed as a percentage of the five-year average (Table 2).
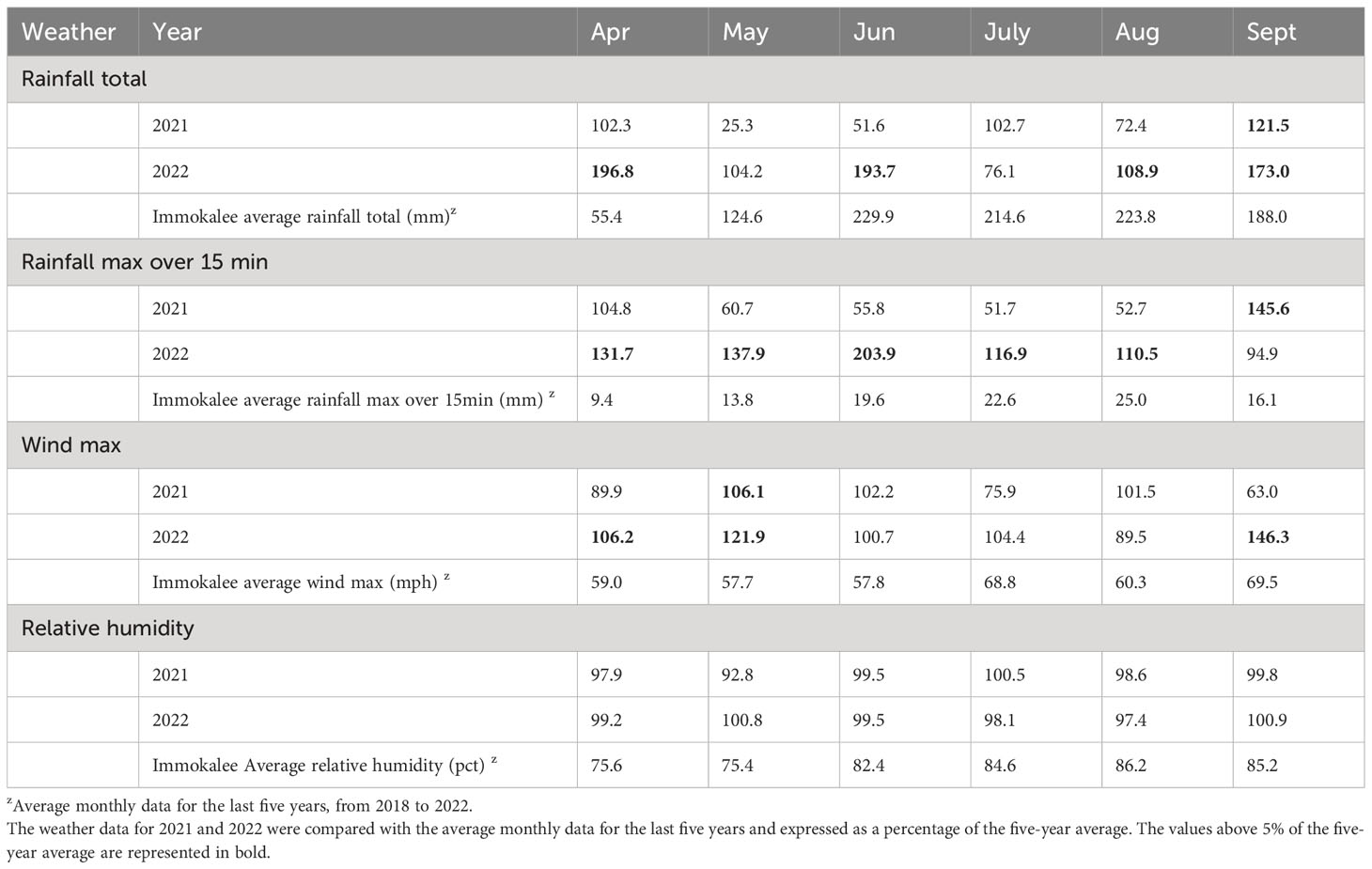
Table 2 Rainfall, wind, and relative humidity data from 2021 and 2022 at the Florida Agricultural Weather Network (FAWN) site in Immokalee, FL.
2.5 Statistical analyses
The greenhouse data were analyzed using one-way analysis of variance (ANOVA) using GraphPad Prism version 8.0.0 for Windows, GraphPad Software, San Diego, California, USA. For each field experiment, the monthly data on the number of symptomatic fruits per tree, number of symptomatic leaves per meter square canopy area, DSI, and total dropped fruits with or without symptoms were combined for analysis and subjected to ANOVA. Mean separation was conducted using Tukey’s multiple comparison test at α = 0.05 wherever a significant treatment effect existed.
3 Results
3.1 Greenhouse experiments
In both greenhouse experiments, the plants that were not treated but infiltrated with Xcc bacteria developed typical canker lesions that were pustular, had a 1–3 mm size, and necrotic erumpent lesions on the canker with an oily margin and a chlorotic halo on edge, four weeks post inoculation (Figures 1B, 2B). The leaves infiltrated with the buffer control showed no canker lesions and remained completely healthy.
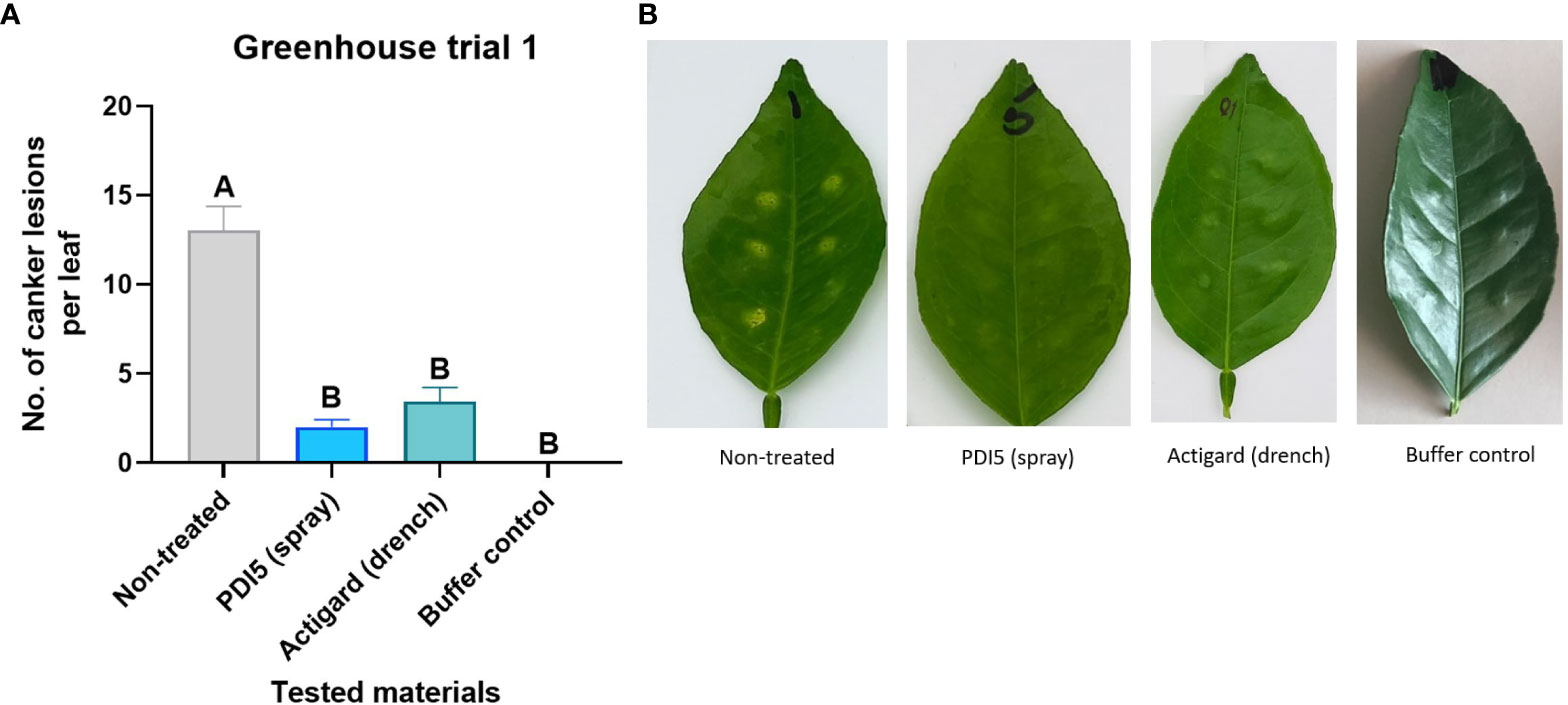
Figure 1 Comparison of the number of canker lesions (A) and visual canker symptoms (B) in the citrus leaves treated with the new plant defense inducer ‘PDI5’ (500 ppm, spray), compared with the Actigard (soil drench; 100 ml per plant; 2 gm/tree), and non-treated and buffer-treated controls, four weeks after injection-infiltration with 1x104 CFU/ml Xanthomonas citri. subspp. citri.
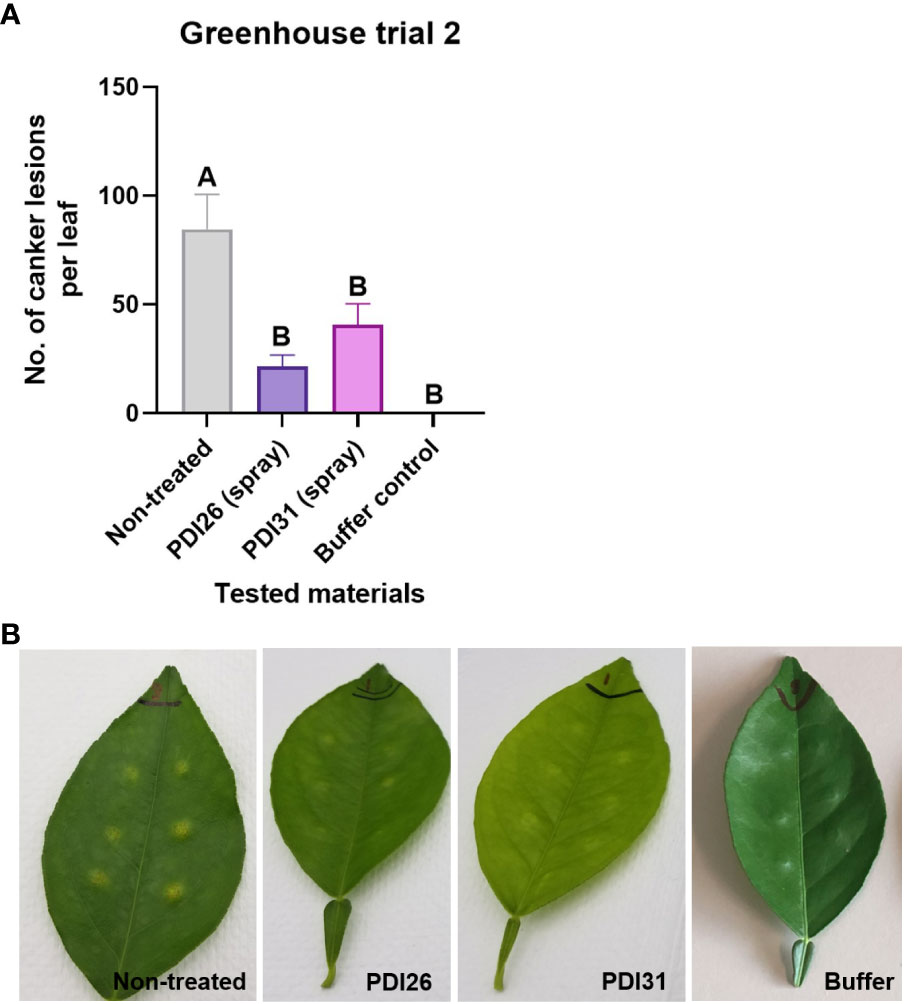
Figure 2 Comparison of the number of canker lesions (A) and visual canker symptoms (B) in the citrus leaves treated with the new plant defense inducers ‘PDI26’ and ‘PDI31’ (500 ppm, spray), compared with non-treated and buffer-treated controls, four weeks after injection-infiltration with 1x104 CFU/ml Xanthomonas citri. subspp. citri.
In the first greenhouse experiment, a foliar spray of the new plant defense inducer PDI5 (500 ppm) had significantly fewer canker lesions on the leaves relative to the non-treated control (P < 0.0001) (Figures 1A, B). The lesions in the PDI5-treated leaves were 84.7% less numerous than non-treated plants. The soil drench application of Actigard was statistically similar to the PDI5 spray treatment in protecting the leaves and had significantly fewer canker lesions relative to the non-treated control (P < 0.0001) (Figures 1A, B). The soil-applied Actigard reduced foliar canker symptoms by 73.7% relative to non-treated plants.
In the second greenhouse experiment, a foliar spray of either of the new plant defense inducers, PDI26 (P = 0.0001) and PDI31 (P = 0.0157), showed significantly fewer canker lesions on the leaves than in the non-treated control (Figures 2A, B). The foliar spray of PDI26 and PDI31 treated leaves had fewer leaf canker lesions by 74.5% and 52.1%, respectively, relative to the non-treated plants.
The appearance of the lesions was different depending on the treatment. Plants treated with the soil-applied Actigard or sprayed with the new plant defense inducers PDI5, PDI26, and PDI31 had smaller lesions with an erumpent center, less necrosis, and minimal chlorosis compared to the untreated leaf symptoms (Figures 1B, 2B).
Phytotoxicity symptoms were not observed with any of the test materials in either greenhouse experiment at any time points throughout the experiment.
3.2 Field trial, 2021
In 2021, during May and June, when fruit was most vulnerable to the disease, significant rainfall events were absent, and the conditions were much drier than usual for that time of year in the field trial site in Florida, USA (Table 2). In addition to the reduced rainfall, the maximum wind speed and the relative humidity in the grove, all of which favored inoculum spread, were also less during the early seasons of 2021 compared to 2022. Hence, the average DSI of fruits in 2021 was only less than 10% for both the water-treated control and copper treatments (Figure 3). Subsequently, six sprays of copper and alternative products from April to September only marginally reduced the number of symptomatic leaves per meter square canopy areas (Figure 4) and the number of dropped fruits (Figure 5) compared to the water-treated control. Overall, the number of fruits dropped due to canker in 2021 was low for all treatments (Figure 5), ranging from 13 in the untreated control to 3.8 in the copper treatments, and there was no significant difference among any of the treatments in terms of the fruit drop due to canker. However, the number of symptomatic fruits per tree (Figure 6) was significantly less in the trees treated with Kocide 2000, as well as the nutrient solution (AGRO-MOS) and the antimicrobial compound (AM) at 1.68 kg/ha and MC at 1.68 kg/ha, when compared to the water-treated control. None of the treatments were significantly better than Kocide 2000 in terms of fruit drop and leaf and fruit symptoms (Figures 3–6).
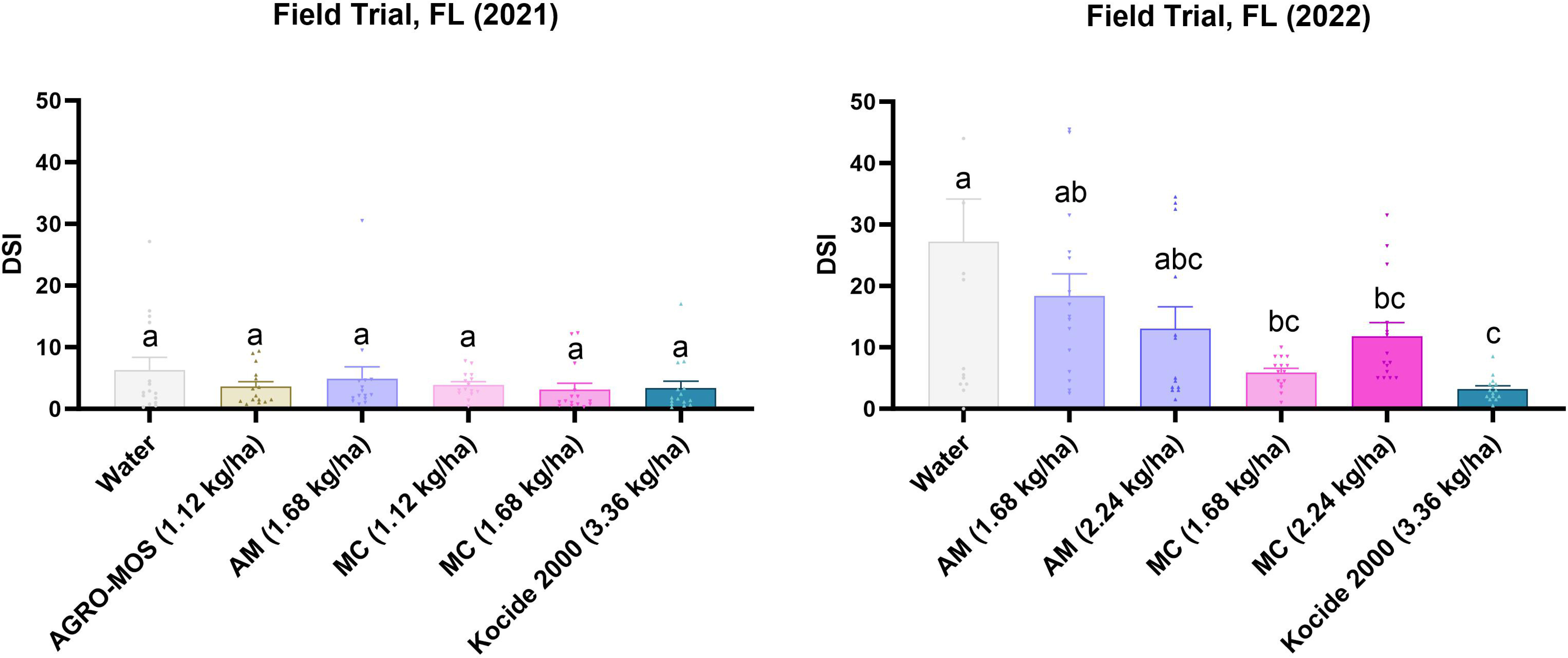
Figure 3 Comparison of the average disease severity index (DSI, %) of the fruits per tree in the trees treated with a nutrient solution (AGRO-MOS), new antimicrobial compounds ‘AM’ and ‘MC’ and Kocide 2000 (growers’ standard control). Different letters above the bar represent significant different values based on one-way ANOVA and Tukey pairwise comparison test. The field trials were conducted in 2021 (left) and 2022 (right).
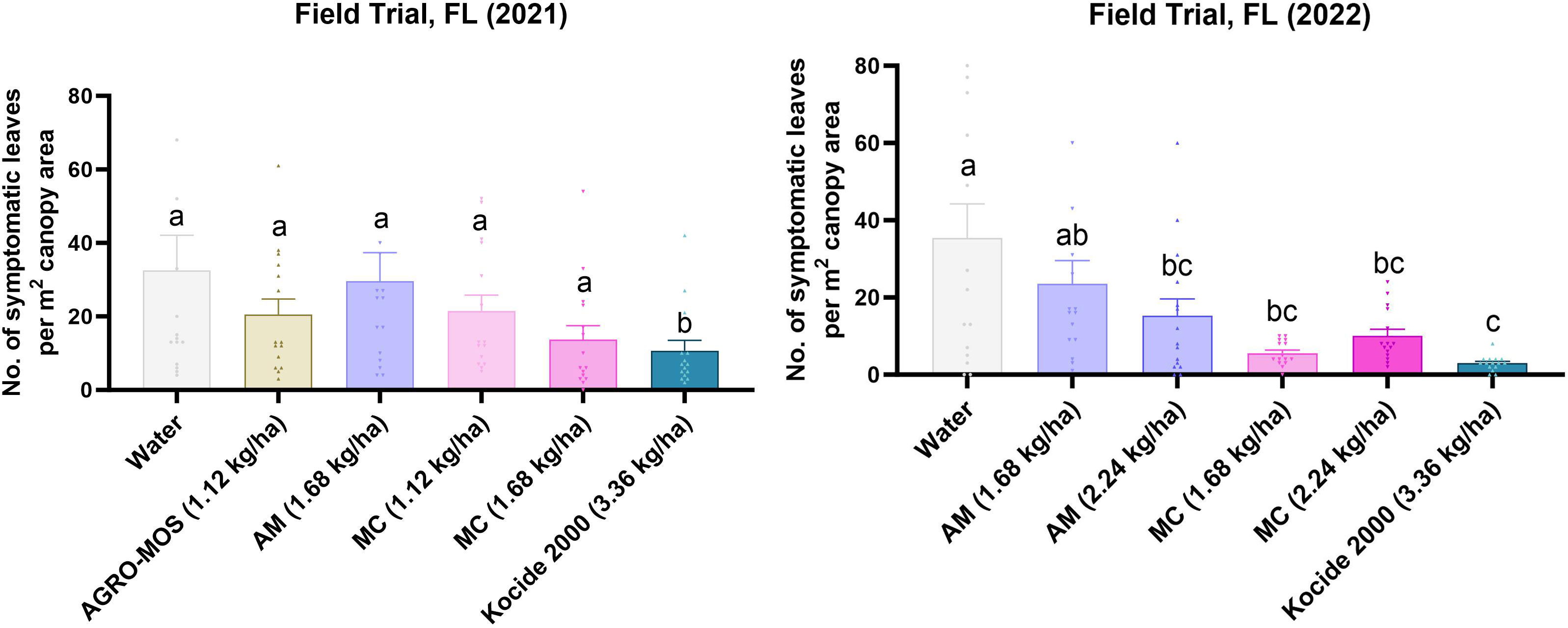
Figure 4 Comparison of the number of symptomatic leaves per meter square canopy areas in the field trees canopy treated with a nutrient solution (AGRO-MOS), new antimicrobial compounds, ‘AM’ and ‘MC’ and Kocide 2000 (growers’ standard control). Each bar represents the means of five replicate plots with three trees per replicate. Different letters above the bar represent significant different values based on one way ANOVA and Tukey multiple comparison test. The field trials were conducted in 2021 (left) and 2022 (right).
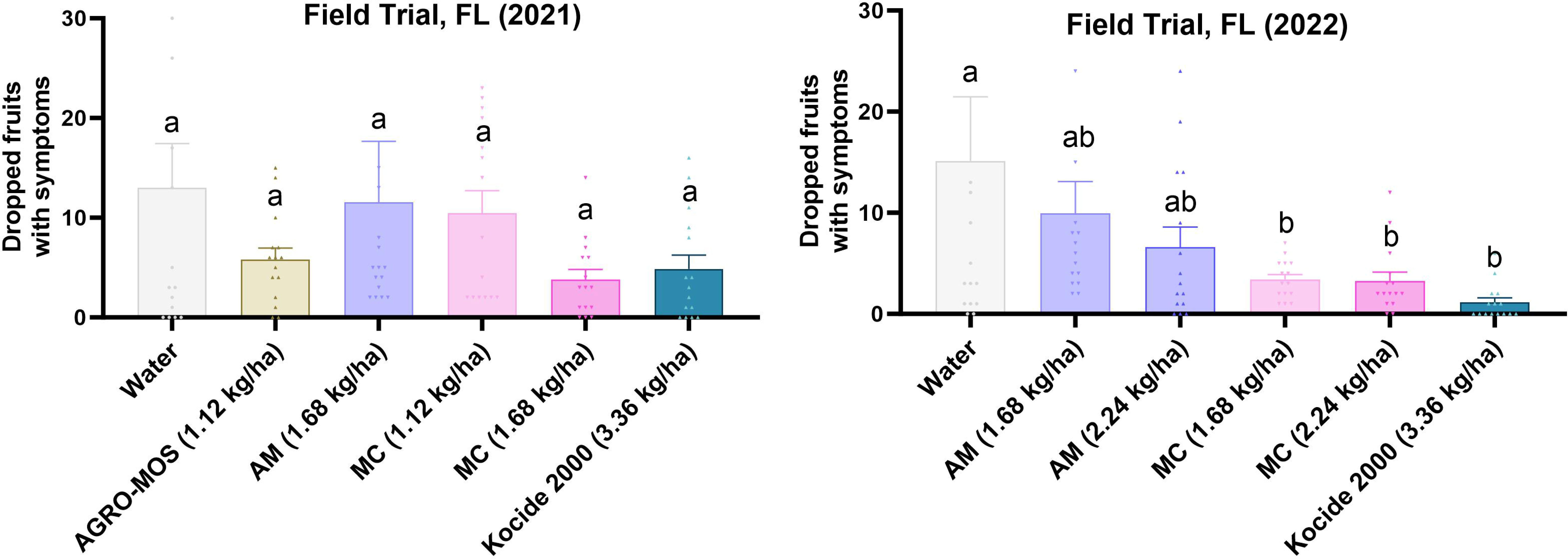
Figure 5 Comparison of the number of dropped fruits with canker lesions on the trees treated with a nutrient solution (AGRO-MOS), new antimicrobial compounds, ‘AM’ and ‘MC’ and Kocide 2000 (growers’ standard control). Each bar represents the means of five replicate plots with three trees per replicate. Different letters above the bar represent significant different values based on one way ANOVA and Tukey multiple comparison test. The field trials were conducted in 2021 (left) and 2022 (right).
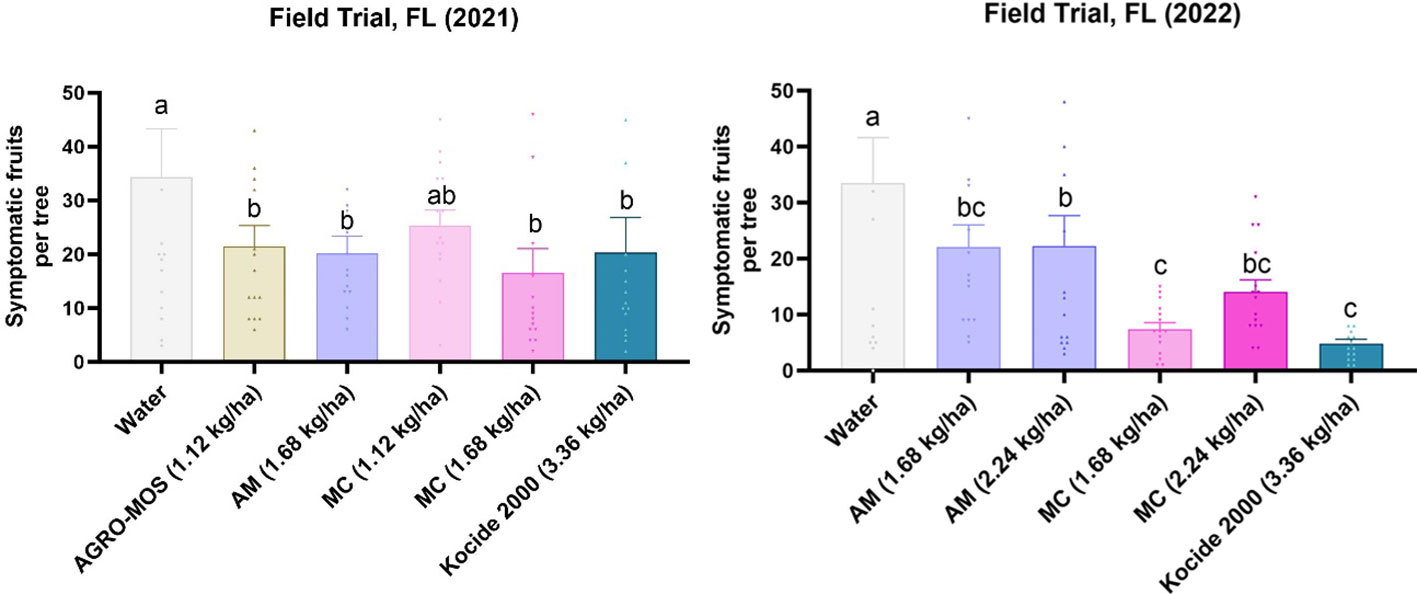
Figure 6 Comparison of the number of symptomatic fruits per tree treated with a nutrient solution (AGRO-MOS), new antimicrobial compounds ‘AM’ and ‘MC’ and Kocide 2000 (growers’ standard control). Different letters above the bar represent significant different values based on Tukey multiple comparison test. The field trials were conducted in 2021 (left) and 2022 (right).
3.3 Field trial, 2022
In 2022, there was relatively higher rainfall throughout the season, accompanied by comparatively high wind and relative humidity (Table 2). Consequently, we observed higher disease incidence in 2022 (Figure 3; Table 2). The average DSI in 2022 was 27.2% in the water treated control, which was significantly higher than in the plants treated with the test compounds, MC, and Kocide 2000. The DSI in the plants treated with MC 1.68 kg/ha and 2.24 kg/ha were 5.9% and 11.8%, respectively, and in plants treated with AM (2.24 kg/ha) was 13%. The DSI of plants treated with the grower’s standard control, Kocide 2000 (3.36 kg/ha) was 3.2%. In terms of the DSI, both rates of MC applied at 1.68 kg/ha (P = 0.9948) and 2.24 kg/ha (P = 0.5436), and the antimicrobial (AM) applied at 2.24 kg/ha (P = 0.4335) performed statistically similarly to Kocide 2000.
In terms of the leaf lesions, the antimicrobial compound AM (2.24 kg/ha) and MC (1.68 kg/ha and 2.24 kg/ha) also significantly reduced the number of leaves with canker lesions per meter square canopy area, as compared to water-treated control (Figure 4). Overall, the number of fruits drop due to canker remained low for all treatments in 2022 (Figure 5). However, unlike in 2021, where we did not see any treatment difference, the number of fruits dropped due to canker was significantly reduced by both concentrations of MC (1.68 kg/ha and 2.24 kg/ha) in 2022, similar to the grower’s standard control, Kocide 2000 (Figure 5). All the treatments, including both rates of AM and MC, also significantly reduced the incidence of fruit infection relative to the water-treated control. Both concentrations of MC as well as AM (1.68 kg/ha) were similar to Kocide 2000 (Figure 6).
Only the Kocide 2000 treatment significantly reduced total fruit drop, regardless of whether canker lesions were present or not (Figure 7), while the other treatments did not exhibit the same effect. Overall, none of the treatments were significantly better than Kocide 2000 in terms of fruit drop and leaf and fruit symptoms (Figures 3–7).
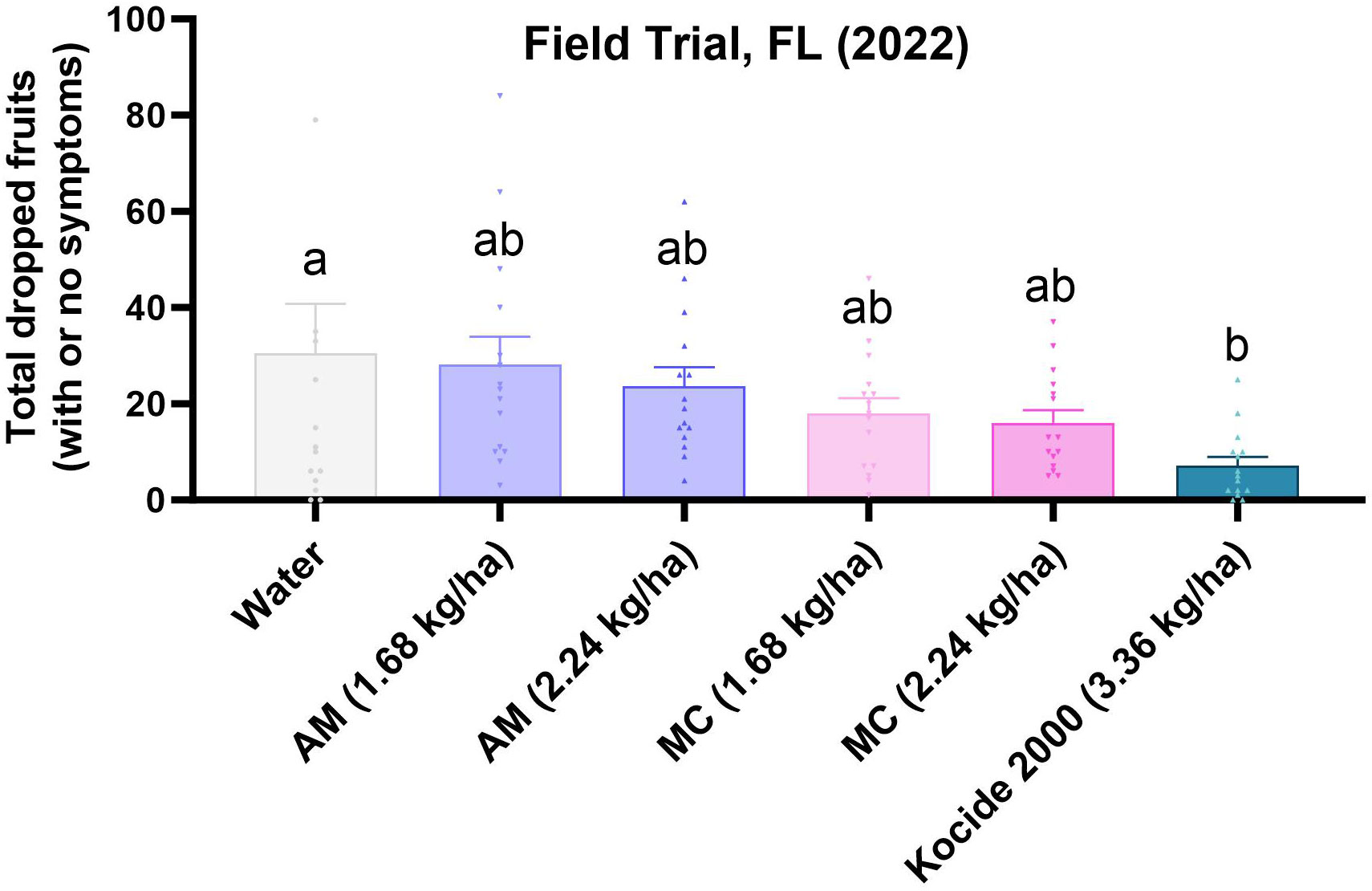
Figure 7 Comparison of total dropped fruits (with or without canker lesions) on the trees treated with a new antimicrobial compounds, ‘AM’ and ‘MC’ and Kocide 2000 (growers’ standard control). Each bar represents the means of five replicate plots with three trees per replicate. Different letters above the bar represent significant different values based on one way ANOVA and Tukey multiple comparison test. The field trial was conducted in 2022.
3.4 Phytotoxicity
No foliar or other symptoms of phytotoxicity were observed in the tree canopy and fruits following treatments in the field trials in both years. However, a small number of leaf burns were noticed in the Kocide treatment, especially a few days after applying on hot days (data not shown).
4 Discussion
The extended use of copper bactericides has several drawbacks, such as developing resistance to copper in Xcc populations and the build-up of copper in citrus soils, which could harm plants and the environment (Alva, 1993; Alva et al., 1995; Canteros et al., 1995). The alternative bactericides for canker control and the commonly used SAR inducers, including Actigard, are not as effective as copper because they cannot provide full-season disease control (Graham and Leite Jr, 2004). The primary aim of this investigation was to identify a better alternating or supplemental partner to copper, aiming to diminish the overall copper accumulation in soil and minimize its environmental impact. Copper remains a prevalent method for controlling a range of bacterial and fungal diseases in citrus groves, including citrus canker, and is routinely used by growers. Although our study does not directly quantify the extent of copper reduction achievable through the application of these complementary agents, we cautiously hypothesize that these agents have the potential to reduce copper reliance in Florida citrus and mitigate its associated environmental consequences, in future.
Our results showed that the new plant defense inducers evaluated in the greenhouse, PDI5, PDI26, and PDI31, applied as a foliar spray, provided excellent control of canker symptoms in citrus leaves. Francis et al. (2009) demonstrated that applying defense inducers such as Actigard, Iso nicotinic acid, and Imidacloprid to citrus plants as a soil drench effectively controlled canker in greenhouse tests. Graham and Myers (2013) and Graham and Myers (2016) also found that applying Actigard, Imidacloprid, and thiamethoxam to young grapefruit trees’ foliar flushes helped prevent canker during epidemic conditions. The PDIs work by enhancing a plant’s natural defense mechanisms against a spectrum of biological and environmental stressors (Hu et al., 2018; Graham and Myers, 2011). While the exact mechanisms can vary depending on the specific inducer and the plant–pathogen interaction, some of the most common and fundamental modes of action of PDIs include: a) hormonal regulation, which includes activation of pivotal plant hormones such as, salicylic acid, jasmonic acid, and ethylene and associated signaling pathways to activate specific defense genes, b) production of pathogenesis related (PR) proteins, c) production of phytoalexin, a secondary metabolite with fungicidal, bactericidal or insecticidal properties, and d) reinforcement of cell walls by promoting deposition of lignin, callose and other structural components thereby preventing pathogen penetration and spread within the host cells. The PDIs included in this study are proprietary and the mode of action of their defense is unknown. However, we are currently evaluating the mode of action of these PDIs through gene expression analysis of the common pathogenesis-related (PR) genes in citrus. This information is expected to shed light on the molecular mechanisms underlying their efficacy, providing a deeper understanding of how these PDIs enhance the plant’s defenses.
The advantages of using plant defense inducers over copper sprays are that they are systemic, less toxic to the environment, are more economical, and can be used as a preventive measure, unlike copper, which is strictly non-systemic and fails to function if there are gaps in the coverage of the surface film (Graham et al., 2016a). Although we do not know the exact mode of action of the plant defense inducers, PDI5, PDI26, and PDI31, they showed promising results in managing citrus canker and reducing canker lesions and warrant being evaluated as part of an IPM program for citrus canker in commercial citrus groves.
Frequent use of foliar-applied SAR inducers such as Actigard has been associated with a negative effect on yield in vegetable and tree crops (Louws et al., 2001; Kunwar et al., 2017), which is thought to be caused by the physiological strain of continuously activating plant defense (van Loon et al., 2006; Walters and Fountaine, 2009). According to a study by Graham and Myers (2011), applying Actigard, Imidacloprid, and thiamethoxam through soil drenches during field trials in grapefruit and oranges had no adverse effects on growth. The new defense inducers that were tested in our greenhouse study (PDI5, PDI26, and PDI31) are currently being trialed in a commercial citrus grove to investigate their ability to provide full season control of citrus canker as well as what factors might affect their efficacy such as timing, application frequencies, and their effects on fruit yield.
Our field results show that the six sprays of the antimicrobial compounds AM (at 2.24 kg/ha) and MC (at 1.68 kg/ha and 2.24 kg/ha) provided excellent control of Xcc, with a significant reduction in disease incidence in leaves and fruits, and disease severity in fruits, similar to Kocide 2000 in 2022. Although 2021 was not as conducive for citrus canker due to relatively dry spring months, AM, MC and the nutrition solution, AGRO-MOS, still numerically reduced fruit and leaf infections. The MC compound also reduced the number of fruit drop due to canker in 2022, similar to Kocide 2000. Although none of the treatments were significantly better than Kocide 2000 in terms of fruit drop, leaf, and fruit symptoms in the field, these were better than the non-treated control (Figures 3–7). These antimicrobials, therefore, have the potential for controlling citrus canker in commercial groves and may be a good alternative to use in IPM as a rotation alternative to copper. Reducing the frequency and amount of copper applications has several advantages, including cutting down on pesticide and application expenses (Behlau et al., 2010; Graham et al., 2010), lower risks of copper buildup in the soil and toxicity for citrus trees (Alva, 1993; Alva et al., 1995), and reduced risk of emergence of copper resistance Xcc strains.
We also cautiously believe that at least AGRO-MOS and MC products will be immediately available to the growers as they are already labeled for citrus in Florida, provided they will continue to hold their efficacy in the repeated future trials in the infected grove. AGRO-MOS is also OMRI listed in the USA for use in organic crop production.
In conclusion, new antimicrobial (AM and MC) and plant defense inducers (PDI5, PDI26, and PDI31) show promise for managing citrus canker and have the potential to be used as part of an IPM program with copper for managing citrus canker. These strategies can potentially reduce dependency on copper sprays and improve crop yields. However, there are still challenges when deploying these strategies that must be addressed before they can be widely adopted. For example, it’s important to optimize the application timing based on the growth stage of the tree, environmental factors such as rainfall and temperature, and disease spread/severity. Moreover, there is a need for further research on greenhouse-evaluated defense inducers (PDI5, PDI26, and PDI31) for their efficacy over long periods, potential risks to the environment, and compatibility with other products. With the right research and implementation, these strategies could provide significant benefits to growers in terms of disease management and crop yield. This information is important for protecting the environment and human health from the harmful effects of the repetitive use of copper bactericides and preventing copper-resistant canker bacterium expansion within and between citrus groves in Florida.
Data availability statement
The original contributions presented in the study are included in the article/supplementary material. Further inquiries can be directed to the corresponding author.
Author contributions
SK: Data curation, Formal Analysis, Investigation, Methodology, Software, Validation, Visualization, Writing – original draft, Writing – review & editing. AR: Data curation, Investigation, Methodology, Project administration, Resources, Software, Supervision, Writing – review & editing. DM: Funding acquisition, Writing – review & editing. MI: Resources, Writing – review & editing. TK: Methodology, Resources, Writing – review & editing. SB: Methodology, Resources, Writing – review & editing. JD: Methodology, Resources, Writing – review & editing. OB: Conceptualization, Funding acquisition, Investigation, Methodology, Project administration, Resources, Supervision, Validation, Visualization, Writing – review & editing.
Funding
The author(s) declare financial support was received for the research, authorship, and/or publication of this article. This project was supported by the Emergency Citrus Diseases Research and Extension grants program, Award numbers 2019-70016-29096 and 2020-70029-33196 from the USDA National Institute of Food and Agriculture.
Acknowledgments
The authors acknowledge growers for supporting this research and providing access to their citrus groves to conduct canker trials. The authors would also like to thank industry collaborators for providing chemicals to conduct the experiments. We would also like to thank the citrus pathology lab members Drs. Kellee Britt and Salih Yilmaz, Kseniya Chumachenko, Juan Balderas, Fetchina Gereus, Jean-Yves, Nicholas Tezna, and Marissa Pequigney, at the Southwest Florida Research and Education Center, University of Florida, for their technical help and support.
Conflict of interest
The authors declare that the research was conducted in the absence of any commercial or financial relationships that could be construed as a potential conflict of interest.
Publisher’s note
All claims expressed in this article are solely those of the authors and do not necessarily represent those of their affiliated organizations, or those of the publisher, the editors and the reviewers. Any product that may be evaluated in this article, or claim that may be made by its manufacturer, is not guaranteed or endorsed by the publisher.
References
Alva A. (1993). Copper contamination of sandy soils and effects on young Hamlin orange trees. Bull. Environ. contamination Toxicol. 51, 857–864. doi: 10.1007/BF00198282
Alva A., Graham J., Anderson C. (1995). Soil pH and copper effects on young ‘Hamlin’orange trees. Soil Sci. Soc. America J. 59 (2), 481–487. doi: 10.2136/sssaj1995.03615995005900020031x
Bagio T. Z., Canteri M. G., Barreto T. P., Júnior R. P. L. (2016). Activation of systemic acquired resistance in citrus to control huanglongbing disease. Semina: Ciências Agrárias 37 (4), 1757–1765. doi: 10.5433/1679-0359.2016v37n4p1757
Behlau F., Belasque J. Jr., Bergamin Filho A., Graham J., Leite R. Jr., Gottwald T. (2008). Copper sprays and windbreaks for control of citrus canker on young orange trees in southern Brazil. Crop Prot. 27 (3-5), 807–813. doi: 10.1016/j.cropro.2007.11.008
Behlau F., Belasque J. Jr., Graham J., Leite (2010). Effect of frequency of copper applications on control of citrus canker and the yield of young bearing sweet orange trees. Crop Prot. 29 (3), 300–305. doi: 10.1016/j.cropro.2009.12.010
Behlau F., Lanza F. E., da Silva Scapin M., Scandelai L. H. M., Silva Junior G. J. (2021). Spray volume and rate based on the tree row volume for a sustainable use of copper in the control of citrus canker. Plant Dis. 105 (1), 183–192. doi: 10.1094/PDIS-12-19-2673-RE
Canteros B. I., Minsavage G. V., Jones J. B., Stall R. E. (1995). Diversity of plasmids in Xanthomonas campestris pv. vesicatoria. Phytopathology 85 (12), 1482–1486. doi: 10.1094/phyto-85-1482
Chamberlain H. L., Roberts P. D., Timmer L. W., Chung K.- R., Zekri M. (2003). CROP ALERT: A citrus canker fact sheet for homeowners: PP194/PP116, 6/2003. EDIS 2003 (11). doi: 10.32473/edis-pp116-2003
Das A. (2003). Citrus canker-A review. J. Appl. Horticulture 5 (1), 52–60. doi: 10.37855/jah.2003.v05i01.15
Dewdney M. M., Walker C., Roberts P. D., Peres N. A. (2022). 2022–2023 florida citrus production guide: citrus black spot: CPG ch. 36, CG088/PP279, rev. 4/2022. EDIS 2022 (CPG). doi: 10.32473/edis-cg088-2022
Francis M., Redondo A., Burns J., Graham J. (2009). Soil application of imidacloprid and related SAR-inducing compounds produces effective and persistent control of citrus canker. Eur. J. Plant Pathol. 124, 283–292. doi: 10.1007/s10658-008-9415-x
Gottwald T., Graham J., Riley T., Sun X., Hughes G., Ferrandino F., et al. (2005). Estimating the increase and spread of citrus canker caused by the interaction of pedestrian versus catastrophic weather events, humans, and bad luck. (Orlando, FL: Second International Citrus Canker and Huanglongbing Workshop) 2005 (C3), 13.
Gottwald T. R., Irey M. (2007). Post-hurricane analysis of citrus canker II: predictive model estimation of disease spread and area potentially impacted by various eradication protocols following catastrophic weather events. Plant Health Prog. 8 (1), 22. doi: 10.1094/PHP-2007-0405-01-RS
Graham J. H., Brooks C., Yonce H. D. (2016a). Importance of early season copper sprays for protection of Hamlin orange fruit against citrus canker infection and premature fruit drop. Proc. Florida State Hortic. Soc., 74–78.
Graham J. H., Dewdney M. M., Myers M. E. (2010). Streptomycin and copper formulations for control of citrus canker on grapefruit. Proc. Florida State Hortic. Soc., 92–99.
Graham J. H., Gottwald T. R., Cubero J., Achor D. S. (2004). Xanthomonas axonopodis pv. citri: factors affecting successful eradication of citrus canker. Mol. Plant Pathol. 5 (1), 1–15. doi: 10.1046/J.1364-3703.2003.00197.X
Graham J. H., Johnson E., Myers M. E., Young M., Rajasekaran P., Das S., et al. (2016b). Potential of nano-formulated zinc oxide for control of citrus canker on grapefruit trees. Plant Dis. 100 (12), 2442–2447. doi: 10.1094/pdis-05-16-0598-re
Graham J., Leite R. Jr. (2004). Lack of control of citrus canker by induced systemic resistance compounds. Plant Dis. 88 (7), 745–750. doi: 10.1094/pdis.2004.88.7.745
Graham J., Leite R., Yonce H. (2007). Streptomycin controls citrus canker in Brazil and Florida and reduces risk of copper phytotoxicity on grapefruit. Phytopathology 97 (7), S42–S42.
Graham J. H., Leite R. P., Yonce H. D., Myers M. (2008). Streptomycin controls citrus canker on sweet orange in Brazil and reduces risk of copper burn on grapefruit in Florida. Proc. Florida State Hortic. Soc., 118–123.
Graham J. H., Myers M. E. (2011). Soil application of SAR inducers imidacloprid, thiamethoxam, and acibenzolar-S-methyl for citrus canker control in young grapefruit trees. Plant Dis. 95 (6), 725–728. doi: 10.1094/PDIS-09-10-0653
Graham J. H., Myers M. E. (2013). Integration of soil applied neonicotinoid insecticides and acibenzolar-S-methyl for systemic acquired resistance (SAR) control of citrus canker on young citrus trees. Crop Prot. 54, 239–243. doi: 10.1016/j.cropro.2013.09.002
Graham J. H., Myers M. E. (2016). Evaluation of soil applied systemic acquired resistance inducers integrated with copper bactericide sprays for control of citrus canker on bearing grapefruit trees. Crop Prot. 90, 157–162. doi: 10.1016/j.cropro.2016.09.002
Hu J., Jiang J., Wang N. (2018). Control of citrus huanglongbing via trunk injection of plant defense activators and antibiotics. Phytopathology 108 (2), 186–195. doi: 10.1094/phyto-05-17-0175-r
Kunwar S., Paret M. L., Freeman J. H., Ritchie L., Olson S. M., Colee J., et al. (2017). Foliar applications of acibenzolar-S-methyl negatively affect the yield of grafted tomatoes in fields infested with Ralstonia solanacearum. Plant Dis. 101 (6), 890–894. doi: 10.1094/PDIS-03-16-0331-RE
Leite R. Jr., Mohan S. (1990). Integrated management of the citrus bacterial canker disease caused by Xanthomonas campestris pv. citri in the State of Paraná, Brazil. Crop Prot. 9 (1), 3–7. doi: 10.1016/0261-2194(90)90038-9
Louws F., Wilson M., Campbell H., Cuppels D., Jones J., Shoemaker P., et al. (2001). Field control of bacterial spot and bacterial speck of tomato using a plant activator. Plant Dis. 85 (5), 481–488. doi: 10.1094/PDIS.2001.85.5.481
Ritenour M., Graham J., Narciso J. (2008). Managing citrus canker for the fresh fruit industry. Citrus Ind. 89 (10), 20–22.
Schubert T. S., Rizvi S. A., Sun X., Gottwald T. R., Graham J. H., Dixon W. N. (2001). Meeting the challenge of eradicating citrus canker in Florida—again. Plant Dis. 85 (4), 340–356. doi: 10.1094/PDIS.2001.85.4.340
Vallad G. E., Goodman R. M. (2004). Systemic acquired resistance and induced systemic resistance in conventional agriculture. Crop Sci. 44 (6), 1920–1934. doi: 10.2135/cropsci2004.1920
van Loon L. C., Rep M., Pieterse C. M. (2006). Significance of inducible defense-related proteins in infected plants. Annu. Rev. Phytopathol. 44, 135–162. doi: 10.1146/annurev.phyto.44.070505.143425
Van-Zwieten L., Merrington G., Van-Zwieten M. (2004). Review of impacts on soil biota caused by copper residues from fungicide application. SuperSoil 2004: 3rd Australian New Zealand Soils Conference (Australia: University of Sydney). Available at http://www.regional.org.au/au/asssi/.
Villamizar S., Caicedo J. C. (2020). Biological control of citrus canker: new approach for disease control. Plant Dis. -Curr. Threat. Manage. Trends 1-12. doi: 10.5772/intechopen.88000
Keywords: sweet orange, copper, systemic acquired resistance inducers, Xanthomonas citri, Xcc, IPM (Integrated Pest Management)
Citation: Kunwar S, Redondo A, Manker D, Iott M, Knobloch T, Brunet S, Dufour J and Batuman O (2023) New antimicrobials and plant defense inducers for managing citrus canker disease. Front. Agron. 5:1292624. doi: 10.3389/fagro.2023.1292624
Received: 11 September 2023; Accepted: 13 November 2023;
Published: 24 November 2023.
Edited by:
David Ezra, Agricultural Research Organization (ARO), IsraelReviewed by:
Zhenchao Wang, Guizhou University, ChinaClive Bock, United States Department of Agriculture (USDA), United States
Copyright © 2023 Kunwar, Redondo, Manker, Iott, Knobloch, Brunet, Dufour and Batuman. This is an open-access article distributed under the terms of the Creative Commons Attribution License (CC BY). The use, distribution or reproduction in other forums is permitted, provided the original author(s) and the copyright owner(s) are credited and that the original publication in this journal is cited, in accordance with accepted academic practice. No use, distribution or reproduction is permitted which does not comply with these terms.
*Correspondence: Ozgur Batuman, obatuman@ufl.edu