- 1Rothamsted Research, West Common, Harpenden, United Kingdom
- 2School of Biosciences, University of Nottingham, Loughborough, United Kingdom
- 3International Maize and Wheat Improvement Centre (CIMMYT), Addis Ababa, Ethiopia
- 4Alliance for a Green Revolution in Africa (AGRA), Nairobi, Kenya
Aim: Zinc deficiencies are widespread in many soils, limiting crop growth and contributing to Zn deficiencies in human diets. This study aimed at understanding soil factors influencing grain Zn concentrations and uptake of crops grown in different landscape positions in West Amhara, Ethiopia.
Methods: On-farm experiments were conducted in three landscape positions, with five farmers’ fields as replicates in each landscape position, and at three sites. Available Zn from the soil (Mehlich 3, M3, Zn) and applied fertilizer (NET_FERT Zn, estimated based on adsorption/desorption characteristics and applied Zn) were related to the actual grain Zn concentration and uptake of teff, wheat, and maize. Zinc fertilizer treatments tested were Zn applied at planting (basal), basal plus side dressing and a control with no Zn applied.
Results: Zn treatments had a significant effect on grain Zn concentration (increase by up to 10%) but the effect on grain yield was variable. Differences in crop Zn concentrations along the landscape positions were observed but not at all sites and crops. Trial results showed that soils with higher soil pH and Soil Organic Carbon (SOC) (typical of footslope landscape positions) tended to adsorb more applied Zn (reduce NET_FERT Zn) than soils with lower soil pH and SOC (typical of upslope landscape positions). Zn availability indicators (M3, NET_FERT Zn, clay%) explained 14-52% of the observed variation in grain Zn concentrations, whereas macronutrient indicators (Total N, exchangeable K) together with M3 Zn were better in predicting grain Zn uptake (16 to 32% explained variability). Maize had the lowest grain Zn concentrations but the highest grain Zn uptake due to high yields.
Conclusion: We found that the sum of indigenous and fertilizer Zn significantly affects grain Zn loadings of cereals and that the associated soil parameters differ between and within landscape positions. Therefore, knowledge of soil properties and crop characteristics helps to understand where agronomic biofortification can be effective.
1 Introduction
Zinc (Zn) is an essential plant nutrient required for optimum quality of food crops and can affect crop yield when there is severe deficiency (Broadley et al., 2007). Zinc deficiency is widespread in agricultural soils (Alloway, 2009), diminishing the nutritional quality of crops grown on these soils (Alloway, 2004; Bereket et al., 2011). It is estimated that nearly 20% of the world’s population is severely affected by inadequate Zn intake, living on a cereal-dominated diet with inherently low grain Zn and this figure is closer to 25% in Africa (Hambridge, 2000; Wessells and Brown, 2012). A survey in Ethiopia (1,389 locations) showed that the grain Zn concentrations in wheat, maize, and teff were highly variable due to spatial variation of soils and landscape factors, but it was found to be mostly < 30 mg kg-1 for all crops at all sites (Gashu et al., 2021). This is well below the target concentration of 38 mg kg-1, considered adequate for reducing human Zn deficiency (Bouis and Welch, 2010). Therefore, increasing Zn concentration in staple cereals through the application of Zn-containing fertilizers (also called agronomic biofortification) could help to reduce dietary Zn deficiencies (Welch and Graham, 2004; White and Broadley, 2005; White and Broadley, 2009; Joy et al., 2014; Manzeke et al., 2014; Joy et al., 2015; De Groote et al., 2021).
It has been shown that the application of Zn fertilizer improves wheat and teff crop yields on low Zn soils of the Tigray region in Ethiopia (Bereket et al., 2018). The response of different levels of ZnSO4 on growth and yield of hybrid maize (NK 6240) on farmers’ fields showed that the highest plant height, thousand grain weight, cob yield, stover and grain yield were obtained with the application of 7.5 kg Zn ha-1 in areas where the initial Zn status was low, while 5.0 kg Zn ha-1 was sufficient for soils with medium and high initial Zn status (Panneerselvam et al., 2014). The recommended rate for wheat is 23 kg Zn ha-1 in Turkey (Cakmak, 2008) but only 2 - 5 kg Zn ha-1 in the Middle East (Rashid and Ryan, 2008). For field crops on calcareous soils in South Australia, an application of 9 - 22 kg Zn ha-1 has a beneficial residual effect for about 10 years (Alloway et al., 2008). These studies showed that the efficiency of application methods and rates vary from location to location, driven by the soil characteristics, past uses, management, and environmental conditions.
An international soil test to evaluate the indigenous Zn supply in soils and possible Zn fertilizer response is the Mehlich 3 (M3) method (Mehlich, 1984). But the M3 test does not predict the availability of applied Zn. The availability of Zn from the application of fertilizer is controlled by soil adsorption-desorption characteristics which vary among soil types and landscape positions (Kabata-Pendias, 2010; Stietiya, 2010; Joy et al., 2015; Desta et al., 2021; Mossa et al., 2021). Adsorption-desorption studies have been carried out in many soil types, ranging from acidic to calcareous soils (Imtiaz et al., 2006; Ashraf et al., 2008; Hashemi and Baghernejad, 2009; Muhammad et al., 2006; Mohammed, 2010; Reyhanitabar et al., 2010; Harrah et al., 2012; Desta et al., 2021), but only rarely has this been linked to the Zn availability to plants (Stanton and Burger, 1967; Chaudhry and Loneragan, 1972). Other known soil properties affecting the adsorption of Zn and influencing its availability for crop uptake are soil pH, soil organic carbon (SOC) content, and cation exchange capacity (CEC) (Catlett et al., 2002; Aysen and Behnam, 2012; Bereket, 2018; Van Eynde et al., 2022). Soils with a high pH (Dhanwinder et al., 2008; Desta et al., 2021), high clay content (Ashraf et al., 2008; Mohammed, 2010), and/or high organic matter concentration (Fan et al., 2016; Desta et al., 2021; Van Eynde et al., 2022) tend to increase Zn adsorption and reduce Zn availability to plants. Therefore, it seems necessary to adjust Zn fertilizer application strategies to these soil characteristics accordingly.
The importance of landscape position for crop performance in our target area has recently been highlighted (Amede et al., 2020) but little is known about the effects on Zn uptake for major crops in the region. For example, a study in the Tigray region of Ethiopia has shown that positive and significant correlations of leaf Zn concentration were detected only with total soil Zn (r2 0.30) and SOC (r2 0.52) while DTPA-Zn explained less than 10% of the variability in teff and bread wheat (Bereket, 2018). Similar results were also reported from Malawi where only 3% of the observed variation of maize grain Zn concentration could be explained from soil characteristics (i.e., DTPA-Zn), however this study covered a very wide variation of soils (Botoman et al., 2022). The absence of a significant relationship between grain Zn uptake by plants and the DTPA extractable Zn has also been reported for Mediterranean soils and climate (Moreno-Lora and Delgado, 2020).
A recent aligned study has reported the effects of landscape on grain Zn concentration in wheat and teff in Ethiopia, alongside the responsiveness of grain Zn concentration to Zn fertiliser (Manzeke et al., 2023). A landscape effect on grain Zn concentration was observed in wheat, but not in teff; grain Zn concentration was greater when wheat was grown on footslope soils than on midslope or hillslope/upslope. Landscape position did not influence the response of wheat or teff to Zn fertiliser application.
In another linked study we identified that adsorption-desorption characteristics of the soil could explain a large part of the effects of landscape position on soil Zn availability (Desta et al., 2021). Soil pH and SOC played a significant role in these adsorption-desorption processes and could determine the potentially available Zn from applied Zn fertilizer in the soil (which we called NET_FERT Zn). Therefore, the primary objective of the current study was trying to link indigenous, plant available soil Zn (M3), NET_FERT Zn and basic soil characteristics (e.g., clay concentration, total soil N, exchangeable K) with the actual grain Zn concentrations and uptake of teff, wheat and maize grown across the landscape (upslope, midslope and footslope) in Ethiopia.
2 Materials and methods
2.1 Site description
Experimental sites were at locations in three districts of the Amhara Region (Bahir Dar Zuriya, Enarj Enawega and Bure Districts), named Aba Gerima, Debre Mewi, and Markuma, respectively (Figure 1). The climate in the region is subtropical with annual minimum and maximum temperatures of 12 and 30°C, and an average annual rainfall of 1022 mm at Aba Gerima, 1240 mm at Debre Mewi, and 1450 mm at Markuma (https://www.meteoblue.com). The experimental region is characterized by a hilly landscape on a plateau of about 1800 to 2200 MASL. Experimental fields were chosen based on landscape position which in this region has been reported to have strong effects on soil characteristics (Amede et al., 2020). Nitisols are the dominant soil type for all sites and landscape positions, except for the footslope of Debre Mewi which is dominated by Vertisols (Supplementary Table 1).
2.2 On-farm field trials
On-farm trials in farmer’s fields were established at Aba Gerima, Debre Mewi and Markuma in the 2018/19 cropping season. Each site was divided into three landscape positions (upslope, midslope, and footslope) and in each landscape position, five on-farm trials were established as replications. Therefore, at each site, there were fifteen farmers participating, which also covered different crops. At Aba Gerima two crops were tested (teff and maize), at Debre Mewi three crops (teff, maize and wheat), and at Markuma two crops (maize and wheat), resulting in 30, 45, and 30 on-farm trials, respectively, adding up to a total of 105 trials. Geo-referenced representative top-soil soil samples (0-0.2 m depth) were collected from each on-farm trial (5 sub-samples combined). Except at Aba Gerima where the two crops were planted at different locations with different farmers resulting in 30 soil samples, the same farmers hosted the different crops at Debre Mewi and Markuma, thus resulting in a total of 60 soil samples for this study.
The plot size for each treatment was 5 by 5 m with a 1 m spacing between plots. The Zn fertilizer treatments in the on-farm trials for all three crops were: (1) basal at 5.5 kg Zn ha-1 at planting, (2) basal 5.5 kg Zn ha-1 plus side dressing of 2.75 kg Zn ha-1 at tillering (teff and wheat) or knee-height (maize), and (3) the control treatment where no Zn was applied. All experimental plots received the same recommended NPKS rates according to the research recommendations for each crop. The N, P2O5, K2O, and SO4 rates applied for wheat (variety TAY) and maize (variety BH540 at Aba Gerima and Debre Mewi, and BH660 at Markuma) were 138, 92, 40 and 50.9 kg ha-1, respectively. However, teff (variety Kuncho) growing on Nitisols received 40, 60, 40 and 33.3 kg ha-1, whereas on Vertisols it received 80, 46, 40 and 25.5 kg of N, P2O5, K2O, and SO4 ha-1, respectively. The full amount of P2O5, K2O, and SO4 was applied at planting along with any basal application of Zn. In contrast, N was applied in two equal splits at planting and at tillering/knee height. The harvest was collected from 3 m by 3 m in the centre of the sub-plot to avoid border effects and the yields are expressed at 12.5% moisture content for all three crops.
2.3 Sample analysis
The soil samples were ground and sieved through a 2 mm mesh. All samples were subjected to wet chemistry analysis following standard procedures. The soil pH was measured in deionized water with a soil/water ratio of 1:2.5 (10 g of soils with 25 mL of water) and with a temperature-compensated pH electrode. Total carbon and nitrogen concentrations were determined by dry combustion (Tiessen et al., 1981) using a Leco TruMac CN Combustion Analyser (Leco Corporation, St Joseph, Michigan). And because the pH of all soils was below 6.5, total carbon was equivalent to soil organic carbon (SOC). Total elemental concentrations were measured after an aqua regia extraction (McGrath and Cunliffe, 1985), followed by inductively coupled plasma optical emission spectrometry (ICP-OES; model, Perkin Elmer Life and Analytical, Shelton, USA). For analysis of major and trace elements in the plant, the plant samples were digested using a mixture of nitric acid and perchloric acid (85:15 V/V) in open tube digestion blocks. The acids were removed by volatilisation and the residue dissolved in nitric acid (5% V/V) and the total concentrations of trace elements were measured by ICP-OES. The effective cation exchange capacity (eCEC) determination was a one-step extraction with a 0.0166 M cobalt (III) hexamine chloride solution (Cohex) [Co[NH3]6]Cl3. All exchangeable cations were measured in the extract while the decrease in Co concentration is a measure of the eCEC, and concentrations were measured by ICP-OES analysis (Ciesielski and Sterckeman, 1977). Available soil Zn (indigenous Zn) was determined with the Mehlich 3 (M3) method (Mehlich, 1984). Soil texture was analysed using a Laser Scattering Particle Size Distribution Analyser (LA-960, Horiba Ltd., Kyoto, Japan) and the size of particles were computed based on Fisher et al. (2017).
2.4 Linking the adsorption-desorption characteristics with grain Zn concentrations
The adsorption and desorption experiments were conducted as described in detail by Desta et al. (2021). For each soil sample, an adsorption and desorption curve were determined. Multiple linear regression models were then developed best explaining the observed adsorption and desorption trends, using all soil parameters (35) but eliminating those which were not significant through backward elimination. In both cases, only soil pH and Soil Organic Carbon (SOC) significantly affected the adsorption/desorption functions (Desta et al., 2021). These predictive models of adsorption-desorption characteristics were used in this study to estimate the fractions of Zn adsorbed (Equation 1) and desorbed (Equation 2).
Next, the amount of adsorbed (Equation 3) and desorbed (Equation 4) Zn from the applied fertilizer was computed in kg ha-1.
And lastly, plant available fertilizer Zn (NET_FERT Zn) was computed as the sum of the difference between the applied fertilizer Zn (kg) minus the amount adsorbed (kg) and plus the amount of desorbed Zn in kg (Equation 5).
where Znf, Znad and Znde refers to the amount of Zn applied as a fertilizer, adsorbed, and desorbed (kg ha-1), respectively.
Different approaches were tested to link indigenous soil Zn (M3 Zn) and NET_FERT Zn (Equation 5) to the grain Zn concentration and uptake of teff, wheat, and maize. The first approach was to use “crop data per site” and seven multiple regression models were developed. But because the number of observations for each model was limited to fifteen, the explanatory value of the model measured by the adjusted regression coefficient (r2) was very low. The second approach was to link M3 Zn, NET_FERT Zn (Equation 5) and clay percentage with grain Zn concentration across sites but separated for each crop (see process below). This doubled the observations for teff, and wheat planted in two locations, and tripled them for maize planted in three locations, allowing better prediction power as compared to the previous approach. The same procedures were followed for grain Zn uptake where Total soil N and Total Soil K were identified as the best further explanatory variables.
2.5 Statistical analysis
Prior to statistical analysis, the data were checked for the assumptions of ANOVA using graphs such as residual vs fitted values for homogeneity of variance, theoretical quantiles vs standardized residuals for normality assumption, and the bell-shape of the histogram of residuals, always meeting the assumptions of ANOVA.
Then, ANOVA with linear models for location, landscape position and treatments and possible interactions among these on the significance effect on the yield, grain Zn concentrations and uptake of teff, maize and wheat crops were prepared using the R packages Agricole (Statistical Procedures for Agricultural Research), carData (Comparison of Applied Regression), car (Comparison of Applied Regression) and predictmeans (making inferences such as predicted means and standard errors, contrasts, multiple comparisons, permutation tests and graphs). All the statistical analyses were performed using RStudio (Version 4.1.2).
Finally, predictive models for grain Zn concentration were developed based on indigenous (M3) Zn, NET_FERT Zn and other soil parameters significantly affecting the actual grain Zn concentration of each crop using linear models (lm, RStudio). The same process was used to develop predictive models for grain Zn uptake (grain Zn concentration times grain yield).
3 Results
3.1 Soil characteristics of the study sites
The soils were all classified as strongly to moderately acidic and SOC contents were classified by the same author as low to moderate (Tekalign, 1991). In general, the study sites were characterized by lower pH and higher SOC in the upslope compared with the footslope except for teff at Aba Gerima (Table 1). Total soil Zn falls in the medium class (Jones, 2003) while the available Zn measured by Mehlich 3 (M3) was classified as low to medium (Mehlich, 1984). The effective CEC (eCEC) varied but can be considered low to medium (Landon, 1991; FAO, 2006). Across sites, the soil texture ranged from clay-loam to clay and usually showed increasingly finer texture (more clay) from the upper to the lower field positions (Table 1). There were differences between sites, but the texture trends are similar.
Although usually the CEC of soil increases with its clay content, it also depends on the type of clay, as well as soil pH and the amount of organic matter. Soils with a low CEC are more likely to acidify, and at all sites lower eCEC values were linked to lower pH values. Soil organic carbon concentrations did not indicate a clear trend with respect to landscape position but were often increasing with decreasing soil pH (Sun et al., 2023). The higher CEC of the upslope, teff-planted fields at Aba Gerima and the maize-planted footslope fields at the same site was associated with increasing soil pH and decreasing SOC content while at Debre Mewi it was also associated with increasing clay content. Total soil Zn and M3 available Zn are positively linked, and the lowest values were observed at Markuma.
3.2 Treatment effects on grain yield
Analyses of variance (ANOVA) with linear models for effects of location, landscape position and treatment, and possible interactions among these, on the yield of teff, maize and wheat were established. Significant differences were observed among the locations for the mean grain yield of teff (df 70, F -42.68, p < 0.001) and maize (df 74, F -67.24, p < 0.001) but not for wheat (df 57, F -2.68, p = 0.107). For all sites, landscape positions were found to significantly influence the mean grain yield of teff (F -31.22, p < 0.001) and wheat (F -5.80, p < 0.01), but for maize only at Debre Mewi (df 9, F-60.21, p < 0.001).
The grain yield was generally higher for teff on the upslope and for wheat on the footslope. Only marginal yield differences were observed for maize at Aba Gerima and Markuma and landscape position had no consistent effect (Table 2). Zinc treatments did not show any significant effect on the mean grain yield of any crop (teff F- 0.33, p = 0.719; wheat F- 0.42, p = 0.657; maize F- 0.10, p = 0.902) but the control was usually the lowest yielding treatment; Possible reasons are the relatively low application rates of Zn fertilizer and the large variation between fields (Table 2). Interaction effects were found to be non-significant for all crops.
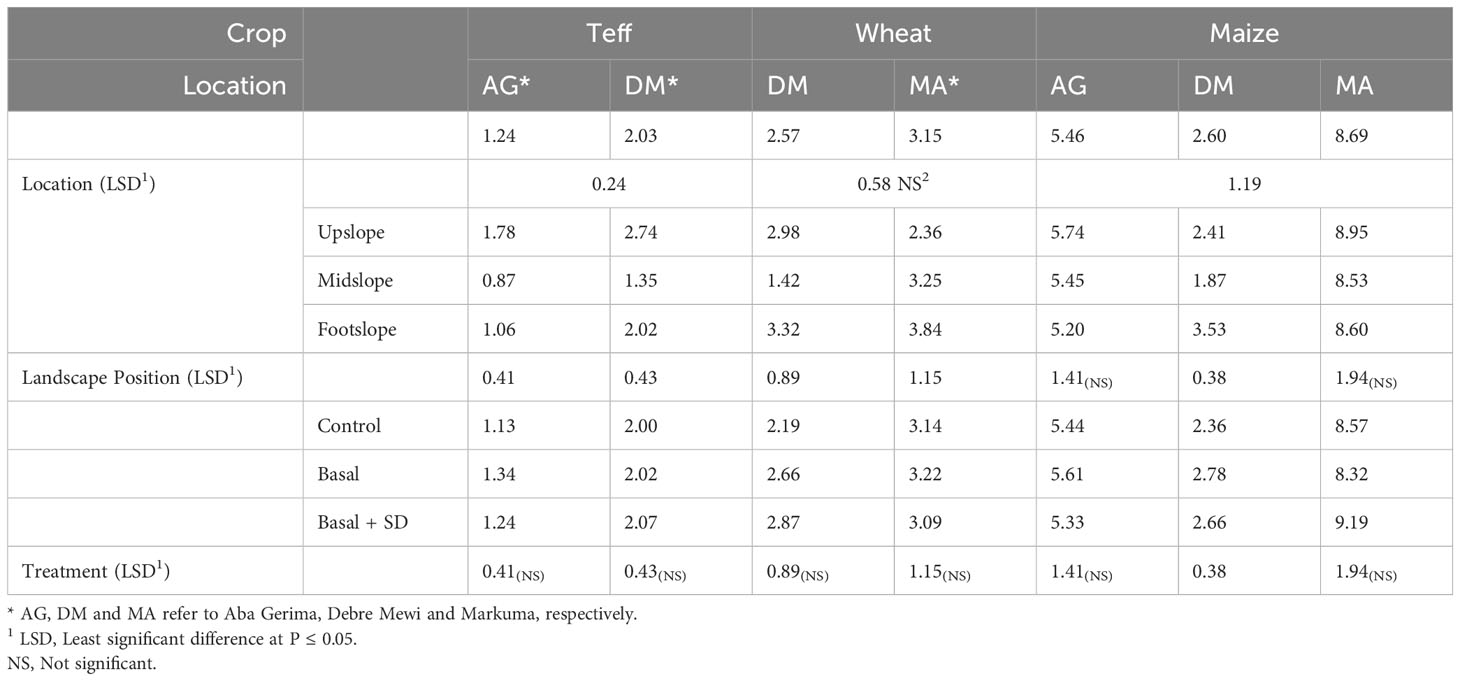
Table 2 Mean grain yield (t ha-1) of teff, wheat and maize depending on location, landscape position and zinc treatment in the 2018 cropping season.
3.3 Treatment effects on grain Zn concentrations
Location significantly affected the mean grain Zn concentrations of teff (df 70, F-17.77, p < 0.001), wheat (df 57, F -23.26, p < 0.001) and maize (df 74, F -32.28, p < 0.001) (Table 3). Similarly, significant differences were observed for landscape positions and teff at Aba Gerima (F -14.12, p < 0.001), for wheat at both locations (F -6.96, p < 0.01) and for maize at Debre Mewi (Table 3), with highest grain concentrations usually on the footslope. Unlike with the grain yield, the Zn treatments did significantly affect the mean grain Zn concentrations of teff (F -17.77, p < 0.001), wheat (F -15.88, p < 0.001), and maize (F -4.94, p < 0.01) at Debre Mewi and Markuma. As expected, Zn concentrations increased in the sequence from the control to basal and basal plus side dressing treatments. The application of basal Zn increased grain Zn concentrations substantially (4 - 20%, mean of 11%) whereas the further increase with additional side dressing had little to no effect (Table 3). The higher yield of maize (Table 2) and grain Zn concentrations (Table 3) at Markuma as compared to Aba Gerima and Debre Mewi could be associated with the varietal difference and its efficiency of using soil Zn.
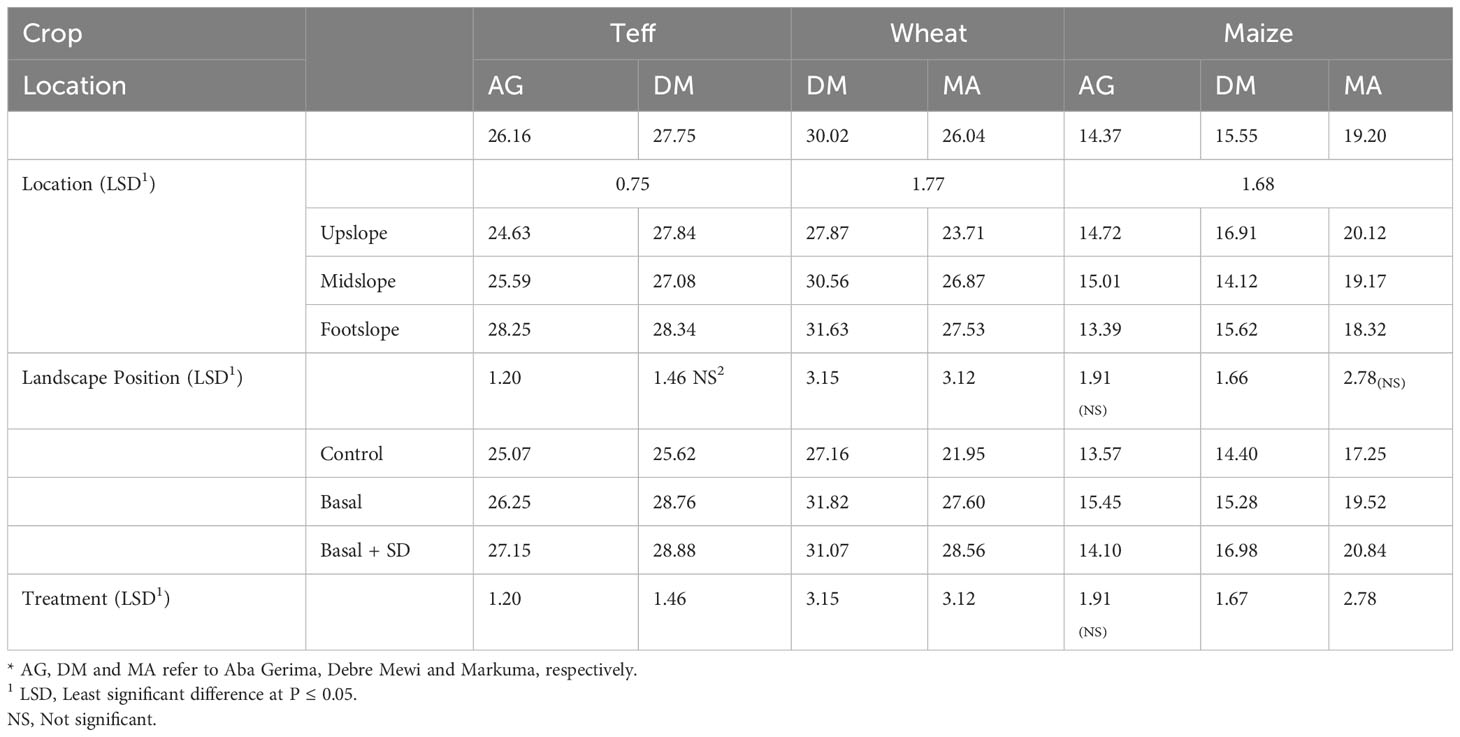
Table 3 Mean grain Zn concentrations (mg kg-1) of teff, wheat and maize depending on location, landscape position and zinc treatment in the 2018 cropping season.
3.4 Treatment effects on grain Zn uptake
Grain Zn uptake, which is a function of yield and grain Zn concentrations, followed the combined trends of significant effects for location, landscape position and Zn treatment for the three crops (Table 4). Location affected the uptake of teff (df 70, F -60.14, p < 0.001) and maize (df 74, F -69.28, p < 0.001) but not for wheat (df 57, F -0.06, p < 0.807). Significant effects of landscape were detected for teff (df 70, F -32.80, p < 0.001), wheat (df 57, F -7.59, p < 0.01) and maize at Debre Mewi (df 9, F -379, p < 0.001). The uptake was high in the upslope for teff at both locations and for maize at Aba Gerima and Markuma whilst wheat uptake was higher towards the footslope at both locations (Table 4). Increased Zn application tended to increase Zn uptake, but the effect was rarely significant due to considerable variability between fields.
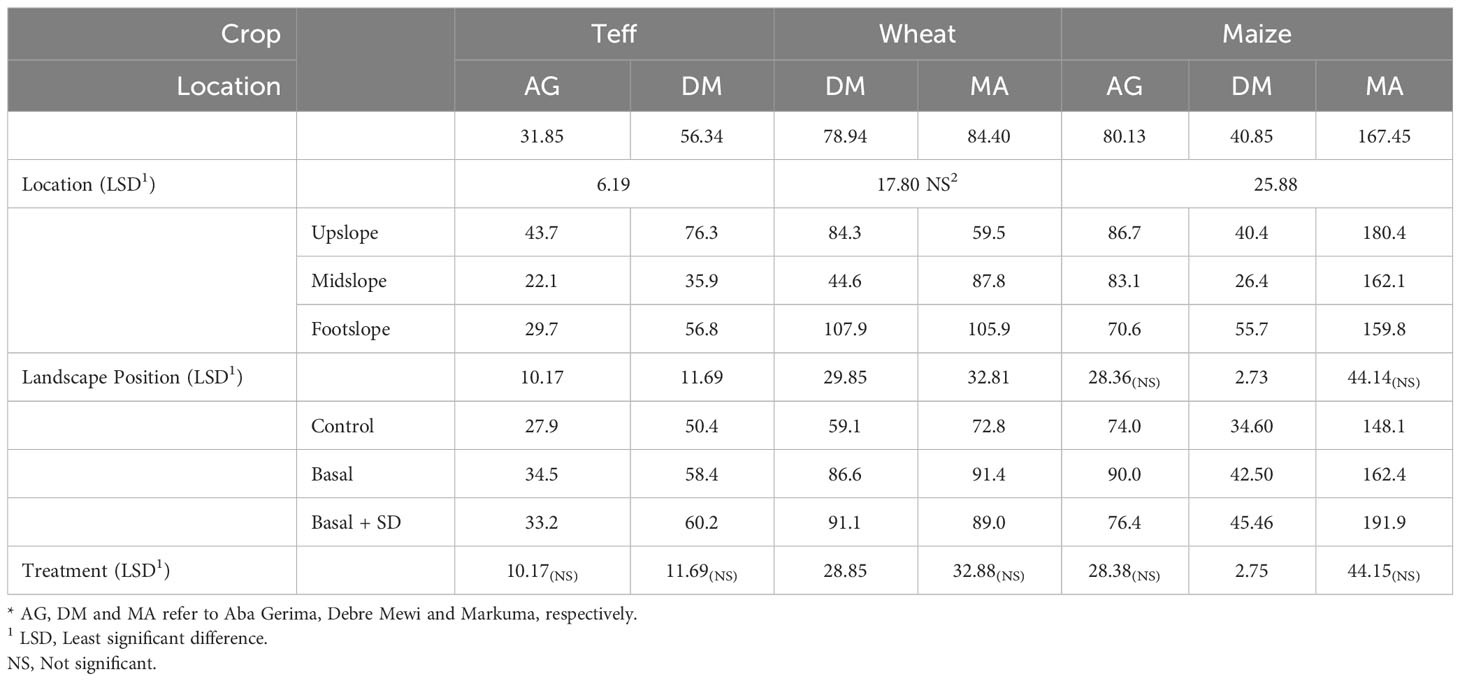
Table 4 Mean grain Zn uptake (g ha-1) of teff, wheat and maize depending on location, landscape position and zinc treatment in the 2018 cropping season.
3.5 Linking indigenous and fertilizer Zn with grain Zn concentrations and uptake
Our objective was to test models estimating grain Zn concentration combining a measure of indigenous soil Zn availability (M3 extraction), availability of Zn from applied Zn fertilizer (NET_FERT Zn), and any additional soil factor explaining grain Zn of the three crops. From the summary of the linear regression model, NET_FERT Zn (see Eq. 5) alone explained some of the observed grain Zn variation, and the explained variation decreased from teff (15%) to wheat (9%) and maize (7%). Similarly, M3 alone explained only a small part of the observed grain Zn concentration variation, decreasing from teff (9%) to maize (6%) and wheat (4%). The only other soil characteristic significantly linked to grain Zn concentration was clay concentration, and the best models including all three components were for maize (19.38 - 1.81Mehlich-3 + 0.46NET_FERT Zn – 0.06Clay), for teff (19.05 + 0.38 Mehlich-3 + 0.58NET_FERT Zn + 0.11 Clay) and for wheat (10.60 + 0.51 Mehlich-3 + 1.07NET_FERT Zn + 0.28Clay) (Figure 2). These models improved the prediction of observed grain Zn variability to 14% for maize (p ≤ 0.05), 52% for teff (p ≤ 0.001) and to 47% for wheat (p ≤ 0.001) (Figure 2). As NET_FERT Zn was computed by including soil pH and SOC (Equation 1 and 2) along with the fertilizer rate, changes in these soil characteristics (Table 1) were also associated with Zn availability in the soil and subsequent concentrations in the grain from applied fertilizer. For example, NET_FERT Zn was higher for the footslope of teff and upslope of maize which was highly associated with increased grain Zn concentrations of these crops. As the results show, predictions are least good in maize which partially might be due to the distinctly different Zn grain concentrations of the two varieties used, i.e., BH660 which was planted at Markuma and BH540 planted at Aba Gerima and Debre Mewi (Table 3). Across landscape positions and treatments, BH660 had higher grain Zn concentrations as compared with BH540 (Table 3).
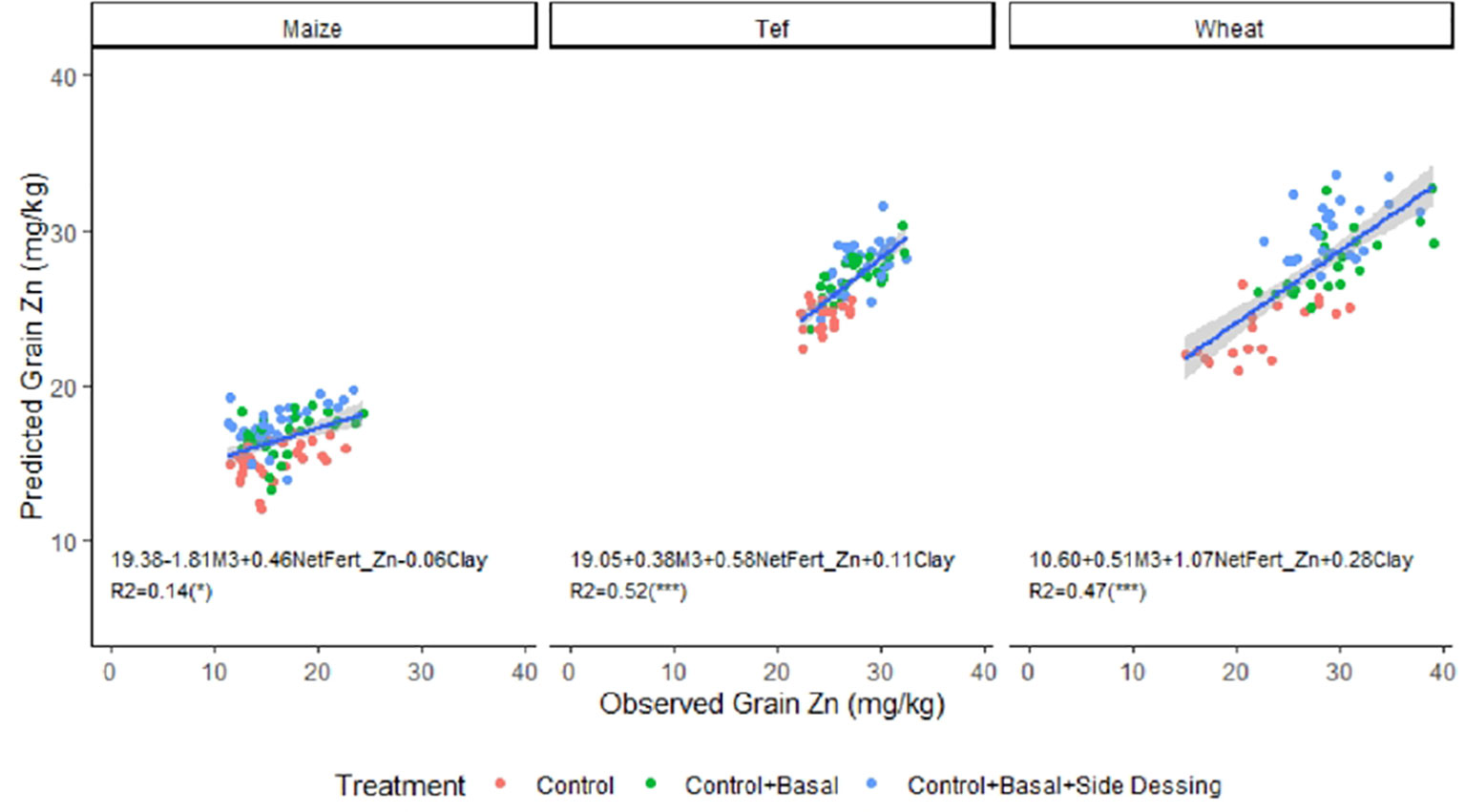
Figure 2 Observed versus predicted grain Zn concentrations for the control and the Zn fertilizer treatments in maize, teff and wheat across sites and landscape positions. The equation describes the best fitting predictive model based on Mehlich 3 (M3), NET_FERT Zn (see equation 5) and the clay concentration (Clay). The related regression coefficient R2 is also shown.
Although the Zn treatments (and therefore NET_FERT Zn) did not significantly influence Zn uptake in the grain (Table 4), the model inclusion of Total Soil N, Mehlich 3 Zn and Total Soil K were found to explain a significant part of the variation in grain Zn uptake for the three crops. Models explaining grain Zn uptake were for maize (47 + 953Total Soil N - 56M3 - 0.01 Total Soil K), for teff (10 + 98Total Soil N - 0.7M3 + 0.01Total Soil K) and for wheat (-37 - 122Total Soil N - 9M3 + 0.05 Total Soil K), with the adjusted r2 of 0.32, 0.16 and 0.24, respectively (Figure 3). Total Soil K was the main predictive soil parameter for Zn uptake in teff and wheat. The consistent and positive significant regressions between Total Soil K and Zn uptake indicates that it had an important role in grain Zn uptake, although it did not for grain Zn concentration. Generally, grain Zn uptake was less predictable than grain Zn concentration (Figure 3) and the uptake values for the control treatment were spread evenly across the dataset from each crop (Figure 3).
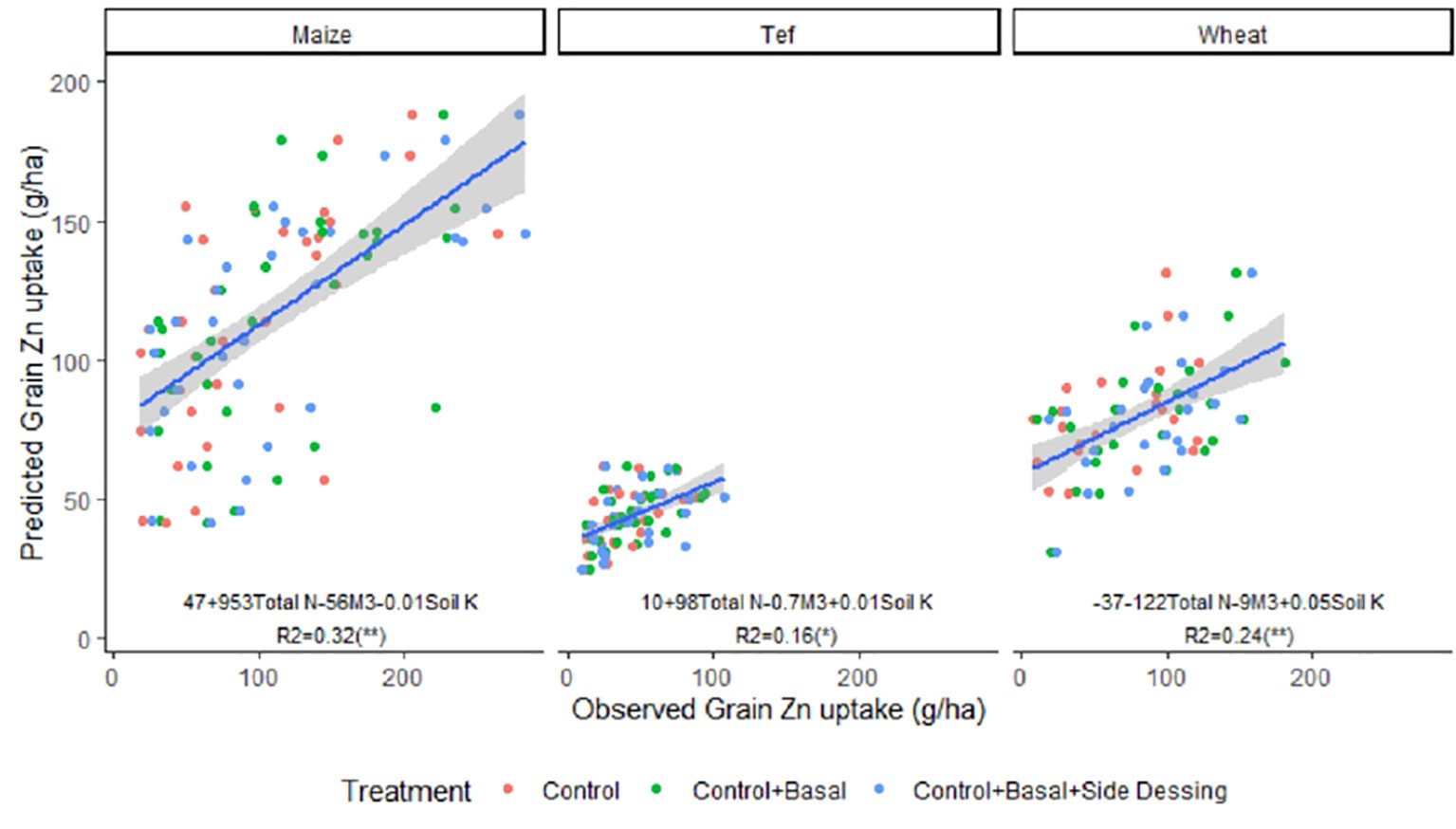
Figure 3 Observed versus predicted grain Zn uptake for the control and the Zn fertilizer treatments in maize, teff and wheat across sites and landscape positions. The equation describes the best fitting predictive model based on Total soil N (Total N), Mehlich 3 Zn (M3) and Total soil K (Soil K). The related regression coefficient R2 for each model is also shown.
4 Discussion
4.1 Soil characteristics in landscape positions and their effect on yield and Zn concentrations
Landscape positions are associated with a range of soil characteristics, which affect the solubility and amount of nutrients available for plant uptake (Singh et al., 2008; Clemens et al., 2010; Bufebo et al., 2021; Sun et al., 2021). Most of these effects are explained by water, particle and nutrient transport downhill, with an accumulation towards the bottom of the slope (Hao et al., 2002; Hu et al., 2019; Sun et al., 2021). General trends of higher CEC, pH, SOC, N, P, K and Zn in footslopes were described for the same region by Amede et al. (2020) but in their as well in our study, these trends were not always consistent, most likely because of considerable variability within landscape positions (Desta et al., 2022). These characteristics and processes also affect the availability of indigenous as well as applied nutrients, including the retention and release of Zn through adsorption-desorption processes across the landscape positions (Desta et al., 2021).
Generally, more favorable conditions in lower landscape positions with respect to water and nutrients (excluding flooding in the valley bottoms) often support higher yields and fertilizer response, as has been shown in the region for wheat by Amede et al. (2020). This study found a similar yield trend for wheat, evenly distributed yields for maize, and higher yields of teff on the upslope. In the case of maize, two sites (Aba Gerima and Markuma) had very high yields across the landscape indicating few soil-related growth limitations for maize, whereas teff is known to be adapted to poor environments with a tendency to lodge under improved N nutrition (Assefa et al., 2011).
Yield response to macro nutrient application (NPK) was not investigated in this study because we only analysed treatments with differences in Zn application. But experimental evidence for wheat grown over three years in Ethiopia showed a pronounced effect of landscape position on the yield response of wheat, increasing up to 3-fold from the upper slope to the foot slope (Amede et al., 2020). With regards to Zn, Zn application generally increased grain Zn concentration significantly and the application of one basal Zn application increased grain Zn concentrations substantially by 4 to 20%, with a mean increase of 11%. But this improved Zn nutrition did not translate into a consistent yield increase, indicating that only a subset of the trial fields were Zn deficient (both results are confirmed by Manzeke et al., 2023). Similar results were reported recently by van Eynde et al. (2023) who found that soil zinc fertilisation did not increase maize yields in 17 out of 19 sites in Sub-Saharan Africa although the application did increase Zn uptake and grain Zn concentrations at the majority of sites (especially in soils with low pH and organic carbon contents).
As already indicated above, the effects of landscape position on yield and Zn fertilizer yield response in our study were less clear and often not significant. Possible reasons were a considerable variability of grain yields within each landscape position and the fact that all treatments tested did receive an equal amount of NPKS, thus covering the main limiting nutrient elements. A recent study on sorghum growing across landscape positions in the same region, also found a positive, although non-significant, response to Zn applications (Desta et al., 2022). Our results also indicate that the change in pH and SOC along with clay contents played an important role in influencing Zn availability to cereal crops grown in different landscape positions, possibly concealing treatment effects. The chemical soil properties such as pH, SOC, and CEC are dominant factors in the control of Zn activity in soils as reported by many authors (Ashraf et al., 2008, Mohammed, 2010). In addition, clay, eCEC, and SOC are involved in the adsorption-desorption process which also affect Zn availability (Alloway, 2009; Aysen and Behnam, 2012). Another recent study showed that the soil Zn distribution between its exchangeable Zn and soluble Zn fractions was controlled by pH, and that isotopic Zn exchangeability increased with soil pH (Mossa et al., 2021). This confirms the results reported by Desta et al. (2021), although that study identified SOC as an additional soil parameter driving Zn availability in soils of different landscape positions. And it leads to the second part of the analysis which intended to determine the main factors affecting grain Zn concentration and uptake across the landscape.
4.2 Predicting grain Zn concentrations and uptake from soil applied fertilizer
Although many independent studies showed that soil pH, SOC, clay content and the linked CEC influence adsorption-desorption process of Zn in the soil and the related availability of Zn to the plant, few of them used the combined effects of these parameters to predict the actual grain Zn concentrations of crops grown on these soils. For example, Zn partitioning between the solid (adsorbed ZnE) and the solution (ZnSoln) phases and the variations in soil solution was highly pH dependent, where 94 and 86% of the variation were explained by soil pH, respectively (Mossa et al., 2021). But the link with the actual grain Zn concentrations was not investigated in this study.
In our study, we hypothesized that the grain Zn concentration would be related to plant available Zn in the three fertilizer treatments, and therefore could be described as a combination of indigenous Zn, determined with the M3 method, and the available fertilizer Zn (NET_FERT Zn; Eq. 5), calculated based on previously established adsorption/desorption functions (Eq. 1 and 2) and the applied Zn amount (Eq. 3 and 4). When combining both of these indicators with clay concentration a considerable part of the variation of grain Zn (14 to 52%) was explained. Therefore, our results indicate that there is a strong link between Zn supply from the soil and Zn concentration in cereal grains, although the effect differed between the crops tested. The much lower explanatory value of available soil Zn for grain Zn concentration in maize could be explained by 1) the considerable differences between the two maize varieties used in the trials, 2) the fact that maize roots are known not to release mugineic acid (MA) which chelate Zn in the rhizosphere (Cakmak et al., 1998), and 3) the much lower Zn concentrations in maize grains at least partially related to the higher yields of maize. Our approach to use adsorption-desorption characteristics to estimate plant available soil Zn is comparable to the approach used by e.g., (Krauss et al., 2002), who also used Freundlich-type functions, but is new for the inclusion of applied Zn fertilizer. In agreement with our study, Bereket (2018) reported that soil pH and SOC, which are part of the NET_FERT Zn estimate in our study, significantly influenced teff and bread wheat leaf Zn concentrations. Similarly, a study from 2010 confirms our observation that soil organic carbon affects Zn adsorption in soils (Mohammed, 2010). And although clay did not affect the adsorption/desorption processes in our study in contrast to Ashraf et al. (2008), the clay concentration was also important to explain grain Zn concentration in our study. Therefore, we believe that our approach has the potential to explain a large part of the observed variation in Zn availability to crops and could be used to fine tune Zn fertilizer recommendations, at least for wheat and teff, respectively.
Given these promising results for grain Zn concentration, we expected similar results for grain Zn uptake (calculated as grain Zn concentration x grain yield). However, neither NET_FERT Zn nor clay concentration contributed anything to explain the variability of grain Zn uptake. Instead, Total soil N and Total soil K together with M3 were identified as the most important/significant characteristics determining grain Zn uptake. But after reconsideration, this seems to make sense. Total soil N and K are linked to important macro nutrients determining grain yield, which is obviously the most important factor determining grain Zn uptake. This is also confirmed by the distribution of the three Zn treatments across the whole range of grain Zn uptake (Figure 3). If Zn supply would be an important factor in grain Zn uptake, it should have been distributed in three clusters similar to the distribution in Figure 2. Nevertheless, indigenous Zn as determined by M3 was still included in the predictive model for grain Zn uptake, indicating that it does have some effect, even if small.
These results might explain the often contradicting reports on the important soil parameters for grain Zn uptake identified by different authors. For example, in a greenhouse experiment the DTPA extractable Zn at the beginning of the experiment explained on average 73 and 56% of the variance in the cumulative Zn uptake of wheat and sunflower, respectively (Recena et al., 2021). But previous work showed that there was no relationship between Zn uptake and the DTPA extractable Zn on durum wheat (Triticum durum L.) grown on a set of Mediterranean soils, and was therefore rejected as an availability index for assessing Zn availability in soils (Moreno-Lora and Delgado, 2020). Such contrasting results could be explained by the varying yield limiting factors determining grain Zn uptake. Another study supporting some of our results include an analysis of 599 wheat plant and corresponding soil samples in China during 2015 and 2016 showed a large variation in grain Zn within different regions. But grain Zn was positively correlated with soil available potassium in wheat systems while soil available Zn was a good indicator in the wheat-maize region, and soil ammonium nitrogen worked well in a rice-wheat region (Huang et al., 2019). Zn uptake, translocation, and remobilization to grain of winter wheat were affected by root growth and were matching with the availability of soil Zn (Liu et al., 2019). However, none of these approaches included the estimation of available Zn fertilizer based on adsorption/desorption functions combined with Mehlich 3 as an indicator of indigenous soil Zn.
Another observation is that although maize had the lowest grain Zn concentrations, it had the highest Zn uptake. Thus, more available soil Zn and fertilizer rates might be needed for (higher yielding) maize crops whereas humans mostly eating maize will have a hard time to fulfil their dietary Zn requirements. Our results confirmed previous studies reporting higher grain Zn concentrations in wheat than maize under low Zn supply (Rehman et al., 2021). Another research report also confirmed that Zn concentration in maize grains was less responsive to Zn application (9%) than in wheat (86%) and rice (27%) (Cakmak and Kutman, 2018). However, we could not find any related studies on teff to confirm our findings.
4.3 Implications for Zn management
It has been shown before that the application of Zn containing fertilizers enhanced yield and grain Zn concentrations of crops on Zn deficient soils. But variability of soil Zn supplies within and between different landscape positions in our trial area cause inconsistent response of Zn soil + fertilizer supply particularly in the case of grain yield. Another issue to consider is the residual effect of applied fertilizers in highly adsorptive soils, important to maximize the benefit of applied Zn as well as to avoid toxicities, both also depending on the crop. As our trials show, only a small amount of soil applied Zn fertilizers are taken up by a crop and the majority remains in the field, potentially accumulating with subsequent applications. Therefore, by considering basic soil parameters, choice of crop type, and target Zn concentrations in the grain of the crop, appropriate Zn fertilizer additions could be calculated using predictive models. This would help to further improve current fertilizer recommendations schemes which do not consider soil adsorption-desorption process affecting the availability of applied Zn as well as the subsequent accumulations in the soil. It also provides additional information about the residual Zn that can be exploited in the following seasons.
5 Conclusions
Predicting nutrient availability to crops based on soil analysis is a constant challenge in agronomy because nutrient availability in soils is dependent on several soil properties, environmental factors, and crop characteristics. Nevertheless, it is a necessary task for the management of inorganic fertilizers to optimize crop growth as well as the nutritional value of the produce. The objective of this study was to better understand Zn uptake of three important cereals in farmers’ fields and a typical cropping system in the West Amhara region of Ethiopia. Based on previous work studying adsorption-desorption functions from the same fields, we developed a model to estimate available Zn from soil supplies and applied fertilizer, and linked it with grain Zn concentrations and uptake by teff, wheat and maize. The analysis showed a good relation between the estimated Zn supply and grain Zn concentration across landscape positions but the strength/shape of the relationship differed between crops. Practical implications are that a) knowledge of soil characteristics can help to better understand Zn availability to the crop, b) that such relations are dependent on the crop and its achievable yields in an agricultural system and c) that agronomic biofortification can help to increase the nutritional value of all three crops studied.
Data availability statement
The raw data supporting the conclusions of this article will be made available by the authors, without undue reservation.
Author contributions
MD: Conceptualization, Formal Analysis, Writing – original draft. MB: Funding acquisition, Project administration, Supervision, Writing – review & editing. SM: Supervision, Writing – review & editing. JH: Methodology, Writing – review & editing. KH: Methodology, Writing – review & editing. SG: Writing – review & editing. TA: Writing – review & editing. SH: Funding acquisition, Supervision, Writing – review & editing.
Funding
The author(s) declare financial support was received for the research, authorship, and/or publication of this article. This work was supported by the Nottingham-Rothamsted Future Food Beacon Studentships in International Agricultural Development and the GeoNutrition project funded by the Bill & Melinda Gates Foundation (BMGF) [INV-009129]. SPM, SMH, JHA and KLH were also partially funded by the Institute Strategic Programme grant “Soils to Nutrition”, UK-BBSRC grant number BBS/E/C/000I0310 and “Growing Health”, BB/X010953/1.
Acknowledgments
The support of the Soil and Water Research staff of Adet Agricultural Research Centre was highly appreciated, and especially Dr. Tadele Amare, and Mr. Beamlaku Alemayehu of the Amhara Regional Agricultural Research Institute (ARARI) are thanked for the help in establishing and managing the field trials. Rothamsted Research is also highly acknowledged for providing access to the laboratory facilities needed for this study.
Conflict of interest
The authors declare that the research was conducted in the absence of any commercial or financial relationships that could be construed as a potential conflict of interest.
Publisher’s note
All claims expressed in this article are solely those of the authors and do not necessarily represent those of their affiliated organizations, or those of the publisher, the editors and the reviewers. Any product that may be evaluated in this article, or claim that may be made by its manufacturer, is not guaranteed or endorsed by the publisher.
Supplementary material
The Supplementary Material for this article can be found online at: https://www.frontiersin.org/articles/10.3389/fagro.2023.1285880/full#supplementary-material
Supplementary Table 1 | Locations, geographical data, landscape position and soil types.
References
Alloway B. J. (2004). Zinc in soils and crop nutrition (Brussels: IZA Publications. International Zinc Association), 135.
Alloway B. J. (2009). Soil factors associated with zinc deficiency in crops and humans. Environ. Geochem Health 31, 537–548. doi: 10.1007/s10653-009-9255-4
Alloway B. J., Graham R. D., Stacey S. P. (2008). “Micronutrient deficiencies in Australian field crops,” in Micronutrient deficiencies in global crop production, vol. 2008 . Ed. Alloway B. J. (Netherlands: Springer), 63–92.
Amede T., Gsashaw T., Legesse G., Tamene L., Mekonen K., Thorne P., et al. (2020). Landscape positions dictating crop fertilizer responses in wheat-based farming systems of East African Highlands. Renewable Agric. Food Syst. 37, 4–16. doi: 10.1017/S1742170519000504
Ashraf M. S., Ranjha A. M., Yaseen M., Ahmad N., Hannan A. (2008). Zinc adsorption behaviour of different textured calcareous soils using Freundlich and Langmuir models. Pakistan J. Agric. Sci. 45, 6–10.
Assefa K., Yu J. K., Zeid M., Belay G., Tefera H., Sorrells M. E. (2011). Breeding tef [Eragrostis tef (Zucc.) trotter]: conventional and molecular approaches. Plant Breed. 130, 1–9. doi: 10.1111/j.1439-0523.2010.01782.x
Aysen A., Behnam D. (2012). The effect of soil properties on Zn adsorption. J. Int. Environ. Appl. Sci. 4, 151–160.
Bereket H. (2018). Assessment of zinc and iron, zinc adsorption, and effects of zinc and iron on yields and grain nutrient concentration of teff and bread wheat in some soils of Tigray (Northern Ethiopia. PhD Dissertation: Haramaya University).
Bereket H., Bobe B., Selamyihun K., TekalignGN M. (2018). Effect of zinc containing fertilizers on yield and grain quality of Tef [(Eragrostis tef (Zucc.) trotter] in some soils of tigray region, Ethiopia. Ethiop. J. Agric. Sci. 28, 23–35.
Bereket H., Stomph T. J., Hoffland E. (2011). Teff (Eragrostis tef) production constraints on Vertisols in Ethiopia: farmers' perceptions and evaluation of low soil zinc as yield-limiting factor. Soil Sci. Plant Nutr. 57, 587–596. doi: 10.1080/00380768.2011.593482
Botoman L., Chagumaira C., Mossa A. W., Amede T., Ander E. L., Bailey E. H., et al. (2022). Soil and landscape factors influence geospatial variation in maize grain zinc concentration in Malawi. Sci. Rep. 12 (1), 7986. doi: 10.1038/s41598-022-12014-w
Bouis H. E., Welch R. M. (2010). Biofortification—A sustainable agricultural strategy for reducing micronutrient malnutrition in the global south. Crop Sci. 50, S 20–S 32. doi: 10.2135/cropsci2009.09.0531
Broadley M. R., White P. J., Hammond J. P., Zelko I., Lux A. (2007). Zinc in plants. New Phytol. 173, 677–702. doi: 10.1111/j.1469-8137.2007.01996.x
Bufebo B., Elias E. A., Agegnehu G. (2021). Effects of landscape positions on soil physicochemical properties at Shenkolla Watershed, South Central Ethiopia. Environ. Syst. Res. 10, 14. doi: 10.1186/s40068-021-00222-8
Cakmak I. (2008). “Zinc deficiency in wheat in Turkey,” in Micronutrient deficiencies in global crop production, vol. 2008 . Ed. Alloway B. J. (Dordrecht: Springer), 181–200.
Cakmak I., Kutman U. B. (2018). Agronomic biofortification of cereals with zinc: A review. Eur. J. Soil Sci. 69, 172–180. doi: 10.1111/ejss.12437
Cakmak I., Torun B., Erenoglu B., Oztürk L., Marschner H., Kalayci M., et al. (1998). Morphological and physiological differences in cereals in response to zinc deficiency. Euphytica 100, 349–357. doi: 10.1023/A:1018318005103
Catlett K. M., Heil D. M., Lindsay W. L., Ebinger M. H. (2002). Soil chemical properties controlling zinc activity in 18 Colorado soils. Soil Sci. Soc America J. 66, 1182–1189. doi: 10.2136/sssaj2002.1182
Chaudhry F. M., Loneragan J. F. (1972). Zinc absorption by wheat seedlings: II. Inhibition by hydrogen ions and by micronutrient cations 1. Soil Sci. Soc Am. Proc. 36, 327–331. doi: 10.2136/SSSAJ1972.03615995003600020035X
Ciesielski H. A., Sterckeman T. (1977). Determination of cation exchange capacity and exchangeable cations in soils by means of cobalt hexamine trichloride. Effect of experimental conditions. Agronomie 17, 1–7. doi: 10.1051/agro:19970101
Clemens G., Fiedler S., Nguyen D. C., Nyuyen V. D., Schuler U. A., Stahr K. (2010). Soil fertility affected by land use history, relief position, and parent material under a tropical climate in NW-Vietnam. Catena 81, 87–96. doi: 10.1016/j.catena.2010.01.006
De Groote H., Tessema M., Gameda S. A., Gunaratna N. S. (2021). Soil zinc, serum zinc, and the potential for agronomic biofortification to reduce human zinc deficiency in Ethiopia. Sci. Rep. 11, 8770. doi: 10.1038/s41598-021-88304-6
Desta G., Amede T., Gashaw T., Legesse G., Agegnehu G., Mekonnen K., et al. (2022). Sorghum yield response to NPKS and NPZn nutrients along sorghum-growing landscapes. Exp. Agric. 58, 1–16. doi: 10.1017/S0014479722000072
Desta M. K., Broadley M. R., McGrath S. P., Hernandez-Allica J., Hassall K. L., Gameda S., et al. (2021). Plant available zinc is influenced by landscape position in the Amhara region, Ethiopia. Plants 10, 254. doi: 10.3390/plants10020254
Dhanwinder S., Ronald G. M. A., Keith C. C. (2008). Effect of pH on zinc sorption–desorption by soils. J. Commun. Soil Sci Plant Anal. 39, 2971–2984.
Fan T. T., Wang Y. J., Li C. B., He J. Z., Gao J., Zhou D. M., et al. (2016). Effect of organic matter on sorption of Zn on soil: elucidation by Wien effect measurements and EXAFS spectroscopy. Environ. Sci. Technol. 50, 2931–2937. doi: 10.1021/acs.est.5b05281
FAO (2006). Plant nutrition for food security: A guide for integrated nutrient management (Rome: Food and Agriculture Organization).
Fisher P., Aumann C., Chia K., O'Halloran N., Chandra S. (2017). Adequacy of laser diffraction for soil particle size analysis. PLoS One 12, e0176510. doi: 10.1371/journal.pone.0176510
Gashu D., Nalivata P. C., Amede T., Ander E. L., Bailey E. H., Botoman L., et al. (2021). The nutritional quality of cereals varies geospatially in Ethiopia and Malawi. Nature 594, 71–76. doi: 10.1038/s41586-021-03559-3
Hao Y., Lal R., Owens L. B., Izaurralde R. C., Post W. M., Hothem D. L. (2002). Effect of cropland management and slope position on soil organic carbon pool at the North Appalachian Experimental Watersheds. Soil Tillage Res. 68, 133–142. doi: 10.1016/S0167-1987(02)00113-7
Harrah M. A., Al-Nasir F., El-Hasan T., Al-Muhtaseb A. H. (2012). Zinc adsorption–desorption isotherms: possible effects on the calcareous Vertisols from Jordan. Environ. Earth Sci. 65, 2079–2085. doi: 10.1007/s12665-011-1188-4
Hashemi S. S., Baghernejad M. (2009). Zinc sorption by acid, calcareous and gypsiferous soils as related to soil mineralogy. Iran Agric. Res. 28, 1–16.
Hu C., Wright A. L., Lian G. (2019). Estimating the spatial distribution of soil properties using environmental variables at a catchment scale in the loess hilly area, China. Int. J. Environ. Res. Public Health 16, 491. doi: 10.3390/ijerph16030491
Huang T., Huang Q., She X., Ma X., Huang M., Cao H., et al. (2019). Grain zinc concentration and its relation to soil nutrient availability in different wheat cropping regions of China. Soil Tillage Res. 191, 57–65. doi: 10.1016/j.still.2019.03.019
Imtiaz M., Alloway B. J., Aslam M., Memon M. Y., Khan P., Siddiqui S.-U.-H.A., et al. (2006). Zinc sorption in selected soils. Communication Soil Sci. Plant Anal. 37, 1675–1688. doi: 10.1080/00103620600710330
Jones J. B. (2003). Agronomic Handbook: Management of Crops, Soils, and Their Fertility (FL, USA: CRC Press LLC, Boca Raton).
Joy E. J. M., Ander E. L., Young S. D., Black C. R., Watts M. J., Chilimba A. D. C., et al. (2014). Dietary mineral supplies in Africa. Physiol. Plantarum 151, 208–229. doi: 10.1111/ppl.12144
Joy E. J. M., Broadley M. R., Young S. D., Black C. R., Chilimba A. D. C., Ander E. L., et al. (2015). Soil type influences crop mineral composition in Malawi. Sci. Total Environ. 505, 587–595. doi: 10.1016/j.scitotenv.2014.10.038
Kabata-Pendias A. (2010). Trace Elements in Soils and Plants (Boca Raton, Florida: CRC Press), 548 p. doi: 10.1201/b10158
Krauss M., Wilcke W., Kobza J., Zech W. (2002). Predicting heavy metal transfer from soil to plant: potential use of Freundlich-type functions. J. Plant Nutr. Soil Sci. 165, 3–8. doi: 10.1002/1522-2624(200202)165:1<3::AID-JPLN3>3.0.CO;2-B
Landon J. R. (1991). Booker Tropical Soil Manual: A Handbook for Soil Survey and Agricultural Land Evaluation in the Tropics and Subtropics. 1st ed (London: Routledge), 530 p. doi: 10.4324/9781315846842
Liu D.-Y., Liu Y.-M., Zhang W., Chen X.-P. A., Zou C.-Q. (2019). Zinc uptake, translocation, and remobilization in winter wheat as affected by soil application of Zn fertilizer. Front. Plant Sci. 10. doi: 10.3389/fpls.2019.00426
Manzeke G. M., Amede T., Bailey E. H., Wilson L., Mossa A. W., Tirfessa D., et al. (2023). Landscape and micronutrient fertilizer effect on agro-fortified wheat and teff grain nutrient concentration in Western Amhara. Agronomy 13, 2598. doi: 10.3390/agronomy13102598
Manzeke G. M., Mtambanengwe F., Nezomba H., Mapfumo P. (2014). Zinc fertilization influence on maize productivity and grain nutritional quality under integrated soil fertility management in Zimbabwe. Field Crops Res. 166, 128–136. doi: 10.1016/j.fcr.2014.05.019
McGrath S. P. A., Cunliffe C. H. (1985). A simplified method for the extraction of the metals Fe, Zn, Cu, Ni, Cd, Pb, Cr, Co and Mn from soils and sewage sludges. J. Sci. Food Agric. 36, 794–798. doi: 10.1002/jsfa.2740360906
Mehlich A. (1984). Mehlich 3 soil test extractant: A modification of Mehlich 2 extractant. Commun. Soil Sci. Plant Anal. 15 (12), 1409–1416. doi: 10.1080/00103628409367568
Mohammed H. S. (2010). Sorption Mechanisms of Zn in Different Clay Minerals and Soil Systems as Influenced by Various Natural Ligands. Ph.D. Thesis. Baton Rouge, LA, USA, Louisiana State University and Agricultural and Mechanical College.
Moreno-Lora A., Delgado A. (2020). Factors determining Zn availability and uptake by plants in soils developed under Mediterranean climate. Geoderma 376, 114509. doi: 10.1016/j.geoderma.2020.114509
Mossa A. W., Gashu D., Broadley M. R., Dunham S. J., McGrath S. P., Bailey E. H., et al. (2021). The effect of soil properties on zinc lability and solubility in soils of Ethiopia - an isotopic dilution study. SOIL 7, 255–268. doi: 10.5194/soil-7-255-2021
Muhammad I., Alloway B. J., Aslam M., Memon M. Y., Khan P., Siddiqui S.-U.-H., et al. (2006). Zn sorption in selected soils. Comm. Soil Sci. Plant. Anal. 37, 1675–1688.
Panneerselvam Selva P., Palaniyandi S. (2014). Response of maize to soil applied zinc fertilizer under varying available zinc status of soil. Indian J. Sci. Technol. 7, 939–944.
Rashid A., Ryan J. (2008). “Micronutrient constraints to crop production in the near east,” in Micronutrient deficiencies in global crop production. Ed. Alloway B. J. (Netherlands: Springer), 2008 149–180.
Recena R., Garcia-Lopez A. M., Delgado A. (2021). Zinc uptake by plants as affected by fertilization with Zn sulfate, phosphorus availability, and soil properties. Agronomy 11, 390. doi: 10.3390/agronomy11020390
Rehman R., Muhammad A., Ismael C. A., Levent O. (2021). Differences in uptake and translocation of foliar-applied Zn in maize and wheat. Plant Soil 462, 1–10. doi: 10.1007/s11104-021-04867-3
Reyhanitabar A., Ardalan M., Gilkes R. J., Savaghebi G. (2010). Zinc sorption characteristics of some selected calcareous soils of Iran. J. Agr. Sci. Tech 12, 99–110.
Singh D., Mclaren R. G., Cameron K. C. (2008). Effect of pH on zinc sorption–desorption by soils. J. Commun. Soil Sci. Plant Anal. 39, 2971–2984. doi: 10.1080/00103620802432873
Stanton D. A., Burger R. D. T. (1967). Availability to plants of zinc sorbed by soil and hydrous iron oxides. Geoderma 1, 13–17. doi: 10.1016/0016-7061(67)90010-9
Stietiya M. H. (2010). Sorption mechanisms of zinc in different clay minerals and soil systems as influenced by various natural ligands (Louisiana, USA: Louisiana State University Doctoral Dissertations), 3592.
Sun X.-L., Minasny B., Wu Y.-J., Wang H.-L., Fan X.-H., Zhang G.-L. (2023). Soil organic carbon content increase in the east and south of China is accompanied by soil acidification. Sci. Total Environ. 857, Part 1. doi: 10.1016/j.scitotenv.2022.159253
Sun S., Zhang G., He T., Song S., Chu X. (2021). Effects of landscape positions and landscape types on soil properties and chlorophyll content of citrus in a sloping orchard in the three gorges reservoir area, China. Sustainability 13, 4288. doi: 10.3390/su13084288
Tekalign T. (1991). “Soil, plant, water, fertilizer, animal manure and compost analysis,” in Working Document No. 13. Addis Ababa: International Livestock Research Center for Africa, Addis Ababa, Ethiopia. Available at: https://hdl.handle.net/10568/4448
Tiessen H., Bettany J. R., Stewart J. W. B. (1981). An improved method for the determination of carbon in soils and soil extracts by dry combustion. Commun. Soil Sci. Plant Anal. 12, 21l–218. doi: 10.1080/00103628109367142
van Eynde E., Breure M. S., Chikowo R., Njoroge S., Comans R. N. J., Hoffland E. (2023). Soil zinc fertilisation does not increase maize yields in 17 out of 19 sites in Sub-Saharan Africa but improves nutritional maize quality in most sites. Plant Soil. doi: 10.1007/s11104-023-06050-2
Van Eynde E., Groenenberg J. E., Hoffland E., Comans R. N. J. (2022). Solid-solution partitioning of micronutrients Zn, Cu and B in tropical soils: Mechanistic and empirical models. Geoderma 414, 115773. doi: 10.1016/j.geoderma.2022.115773
Welch R. M., Graham R. D. (2004). Breeding for micronutrients in staple food crops from a human nutrition perspective. J. Exper. Bot. 55, 353–364. doi: 10.1093/jxb/erh064
Wessells K. R., Brown K. H. (2012). Estimating the global prevalence of zinc deficiency: results based on zinc availability in national food supplies and the prevalence of stunting. PLoS One 7, e50568. doi: 10.1371/journal.pone.0050568
White P. J., Broadley M. R. (2005). Biofortifying crops with essential mineral elements. Trends Plant Sci. 10, 586–593. doi: 10.1016/j.tplants.2005.10.001
Keywords: agronomic biofortification, cereals, East Africa, grain zinc, malnutrition, soil zinc
Citation: Desta MK, Broadley MR, McGrath SP, Hernandez-Allica J, Hassall KL, Gameda S, Amede T and Haefele SM (2023) Linking soil adsorption-desorption characteristics with grain zinc concentrations and uptake by teff, wheat and maize in different landscape positions in Ethiopia. Front. Agron. 5:1285880. doi: 10.3389/fagro.2023.1285880
Received: 30 August 2023; Accepted: 17 October 2023;
Published: 13 November 2023.
Edited by:
Esther Nyaradzo Masvaya, Marondera University of Agricultural Sciences and Technology (MUAST), ZimbabweReviewed by:
Asif Naeem, Nuclear Institute for Agriculture and Biology, PakistanSadia Bibi, University of Agriculture, Pakistan
Copyright © 2023 Desta, Broadley, McGrath, Hernandez-Allica, Hassall, Gameda, Amede and Haefele. This is an open-access article distributed under the terms of the Creative Commons Attribution License (CC BY). The use, distribution or reproduction in other forums is permitted, provided the original author(s) and the copyright owner(s) are credited and that the original publication in this journal is cited, in accordance with accepted academic practice. No use, distribution or reproduction is permitted which does not comply with these terms.
*Correspondence: Stephan M. Haefele, c3RlcGhhbi5oYWVmZWxlQHJvdGhhbXN0ZWQuYWMudWs=