- 1Department of Crop Science and Horticulture, College of Agriculture, Sokoine University of Agriculture, Chuo-Kikuu, Morogoro, Tanzania
- 2Department of Soil and Geological Sciences, College of Agriculture, Sokoine University of Agriculture, Chuo-Kikuu, Morogoro, Tanzania
Cotton productivity continues to be disputed, despite rapid advancements and widespread technologies. These uncertainties remain to be critically addressed in a broad spectrum focusing on domains at the global level. Therefore, this systematic review provides an overview of the existing advancements in knowledge, skills, and technologies for sustainable cotton production on small landholdings. Specifically, the areas of the cotton chain examined are threefold: - (1) Explore disguised agronomic practices to be endowed for sustainable cotton production on small landholdings; (2) Explore socioeconomic settings based on their disparities in contributing to sustainable cotton production on small landholdings; and (3) Explore existing and feasible institutional policies to be enforced for sustainable cotton production on small landholdings. This review shows that worldwide cotton production involves conventional and organic systems, at the expense of the traditional system. Heavy uses of nitrogenous fertilizers and pesticides are the common practices in conventional systems, with some adoptions of precision agriculture practices, and genetically modified varieties. Rotation and intercropping with early-maturing food crops are also identified viable options to improve farmers’ attitudes toward adopting cotton-producing technologies. In socioeconomics, farmers’ livelihoods are improved by income generation from sales of cotton and labour in the cotton industry. Gender equity in the cotton industry prioritizes females over males, as females display a group with a higher level of technology adoption. Generally, clear institutional policies governing the cotton industry are globally paucity. Furthermore, efforts to sensitize sustainable cotton production are still highly questionable and challenged by the superseding climate changes.
1 Introduction
Cotton (Gossypium sp.), one of the world’s oldest cultivated crops, plays a crucial role in the global textile industry (Shahrajabian et al., 2020). The cotton plant is divided into four species in the genus Gossypium, including G. arboreum, G. barbadense, G. herbaceum, and G. hirsutum (Wendel and Cronn, 2003; Wendel and Grover, 2015). About 95% of global cotton production constitutes G. hirsutum cotton (Trapero et al., 2016). It is a warm-season fibre crop that belongs to the Malvaceae family. Cotton is cultivated for its soft, fluffy fibres that are used to manufacture textiles, clothing, and various industrial products. The crop is a significant contributor to the economies of many countries, providing income to farmers and supporting a wide range of industries (Anwar et al., 2009; Yu et al., 2012). With its versatile applications and economic significance, cotton production has evolved over the years, incorporating modern agronomic practices to enhance yield, quality, and sustainability. In this review, we delved into the basics of cotton production and explore the current agronomic practices that contribute to its successful cultivation.
Cotton has woven itself into the fabric of human civilization for thousands of years. Its history is a tapestry of cultivation and trade that spans continents and millennia. The earliest traces of cotton cultivation can be traced back to ancient civilizations, notably the Indus Valley and Egypt, as evidenced by archaeological findings (Yafa, 2005). South America joined this ancient cotton saga, with evidence suggesting cultivation as early as 4500 BCE. The roots of domestication dig deep into the soils of India and Mexico, where wild plants first gave way to cultivation around 4000 BCE (Coppens d'Eeckenbrugge and Lacape, 2014; Suomela et al., 2023). Mehrgarh in Pakistan provides a glimpse into the sixth millennium BCE, where archaeological remains hint at the early use of cotton (Moulherat et al., 2002; Viot, 2019). Moving to Africa, the third millennium BCE reveals the earliest cotton textiles in Nubia, though their precise date and origin remain uncertain (Viot, 2019). In the Classical Antiquity era, the north-eastern part of Africa and the Arabian Peninsula embraced cotton cultivation, as indicated by Bouchaud et al. (2018). Cotton extends its fibres to Central Asia, where cotton textiles from the Later Han period (25–220 CE) in Khotan and the Turfan basin in north-western China paint a vivid picture (Viot, 2019). The cotton plant’s migration continued eastward, reaching west Yunnan and Szechwan in Southern China by the 1st century CE. By the Sung times (5th c. CE), it had established itself in the Chiang-nan region, south of Shanghai (Viot, 2019). This rich history is a testament to cotton’s versatility and adaptability, not only as a textile but also as a cultural and economic force shaping societies across the globe.
The industrial revolution marked a turning point in cotton production, as mechanization and technological advancements led to increased efficiency in processing raw cotton into usable fibres (Huang et al., 2021). Today, cotton remains a vital global commodity (Iqbal et al., 2020). Modern cotton production incorporates a combination of traditional knowledge and advanced techniques to optimize yields while minimizing environmental impacts (AGOA, 2011; Arshad et al., 2022). Some key agronomic practices include variety selection, sowing, soil management, irrigation, fertilization, pest and disease management, weed control, harvesting, post-harvest processing, and sustainability initiatives (Arshad et al., 2022; De Araújo et al., 2022). Therefore, cotton production has evolved significantly over time, incorporating advanced agronomic practices to meet the demands of the textile industry while addressing environmental and social concerns. As the world continues to prioritize sustainability, cotton farmers and researchers work together to develop innovative approaches that ensure a balance between productivity and responsible cultivation (Chen et al., 2022a; Lu et al., 2022).
2 The rationale of the review
The rationale of this review resides in the fact that cotton farmers live and work in developing countries, accounting for about 99% of the world’s cotton farmers (EJF, 2007). Over the past two decades, it has been indicated that almost 66% of cotton farmers reside in India, China, Pakistan, Uzbekistan, Turkmenistan, and Tajikistan with the rest (33%) found in West Africa (i.e., Ghana, Benin, Mali, Burkina Faso, Cote d’Ivoire, Chad, and Togo), Egypt, and/or South America, particularly Brazil (International Cotton Advisory Committee (ICAC), 2005; EJF, 2007). Farmers in these areas are characteristically poor exercising rural settings and cultivating cotton on fields of less than 0.5 hectares, or on small parts of their fields, with the purpose of supplementing income, unlike cotton farmers in the US and Australia (EJF, 2007).
Approximately 2.5% of arable land worldwide, equivalent to 30 million hectares is under cotton production (Johnson et al., 2022; Voora et al., 2023). Literature shows that while 30 million farmers depend on cotton cultivated in rotation with other crops, about 20 million farmers completely depend on cotton production (Hoehn, 2010; Johnson et al., 2022; Riello, 2022; Voora et al., 2022). Whereas cotton has been regarded as an engine of economic growth in rural areas in developing countries, farm inputs related to cotton production have been highly variable with the environments, type of cotton produced, socioeconomic settings of the farmers, and institutional policy (Riello, 2022; Voora et al., 2022). However, the introduction of conventional approaches has exacerbated the haphazard use of tremendous farm inputs, thereby increasing the rate of environmental degradation (Riar et al., 2017; Mandumbu et al., 2021). It is by coincidence that poor institutional policies tailored with the dismantling of cotton boards, plus high fluctuations in fibre prices in international markets have been decreasing areas under cotton in some regions of the globe (Tittonell, 2010; Mandumbu et al., 2021; Bwana et al., 2021).
Deregulation in cotton production by smallholder farmers is reported to increase a shift to the production of maize (Zea mays L.) or sorghum (Sorghum bicolor L.) as food crops for subsistence (Tittonell, 2010; Opee, 2018; Voora et al., 2023). The socioeconomic impacts of cotton production by conventional systems have been analyzed through agronomic and experimental shreds of evidence (Voora et al., 2023). On the other hand, there exist concerns about the environment (land and water resources), farmers’ health, reducing factors (i.e., insect pests, weeds, and disease pathogens), and social settings (Johnson et al., 2022). Global cotton demand and supply have been fluctuating over the last five years (Food and Agriculture Organization of the United Nations, 2019; Johnson et al., 2022). Despite the estimated global increase in cotton production by 3% for the 2022/23 period, demand will outstrip supply since the world economy rebounds due to the pandemic (Grain Central, 2020), thereby reducing cotton stocks by approximately 0.5 Mt (Johnson et al., 2022). Nevertheless, it was predicted to be a relatively balanced market for cotton until 2030 (Food and Agriculture Organization of the United Nations, 2021). There are still uncertainties associated with the effects of the Russia–Ukraine war, and risks of a global recession in 2023 (World Bank Group, 2022). These challenges remain to be an important debate to the whole spectrum of cotton production, including policy-making institutions, production agronomists, researchers, extension officials, and economists (Wakhungu and Wafula, 2013). The decline in cotton production due to limited challenges also is reported to coincide with poverty levels for smallholder farmers, where it is designated as a major cash crop (Lisa and Minot, 2014). For instance, some developing countries realized a tremendous drop in exported revenues from cotton at an average of 34% (Sub-Committee on Cotton, 2021). These countries include Mali, Togo, Benin, Burkina Faso, Malawi, Zambia, Chad, Mozambique, Uganda, and Tanzania, resulting in reduced total area under cotton production (Kone et al., 2020; Sub-Committee on Cotton, 2021; Wright, 2022).
Climate change is another threat to global cotton production, since cotton plants require certain temperatures and moisture levels for fibre production (Devi et al., 2019; Hughes, 2021). In contrast, the productivity of cotton is favoured by increased carbon dioxide levels, but the elevated temperature could be detrimental to global cotton production (Hughes, 2021). Extreme weather events such as extreme rainfall or floods and storms, persistent droughts, heat stress, and extreme winds are reported to affect cotton production (Jans et al., 2020; Cunneen and Owain, 2021). An increase in temperature of 2°C is expected by 2045 to 2065, and at least one of these extreme climate hazards will drastically affect about 50% of all areas growing cotton by 2040 (Cunneen and Owain, 2021; Voora et al., 2023). In line with the impact of climate change, is a dilemma observation that an increase in atmospheric concentrations of carbon dioxide may offset climate-related cotton losses, thereby improving cotton yields (Jans et al., 2020). The cotton crop exhibits a noteworthy tolerance to drought conditions, particularly thriving in temperatures ranging from 32.2°C to 35°C (Sharma et al., 2021). However, regional disparities exist in defining temperature thresholds. In the United States and China, cotton is deemed under heat stress at temperatures exceeding 38°C, whereas in Pakistan and India, temperatures up to 46°C are considered optimal (Zahid et al., 2016; Majeed et al., 2021). For cotton seed germination, an optimal temperature range of 28 to 30°C is crucial. Deviations from this range, either higher or lower, negatively impact germination rates. Temperatures below 20°C, for instance, result in notably poor seed germination (Zahid et al., 2016). Conversely, elevated temperatures beyond the optimum, especially exceeding 38°C, have been linked to decreased germination of cotton seeds (Majeed et al., 2021). The impact of temperature extends to seedling growth. Sub-optimal temperatures between 10°C and 25°C considerably impede seedling growth and temporarily inhibit net assimilation (Snider et al., 2022). Maximum seedling emergence with robust growth is observed at 30°C (Virk et al., 2021). Notably, genotypes developed for hot tropical environments, characterized by larger seed weights, display vigorous seedlings even at 40°C compared to genotypes with smaller seed size and weight (Majeed et al., 2021; Çelik, 2023). However, cotton seedlings do not emerge at an extreme temperature of 50°C (Raphael et al., 2017).
Despite various efforts of improving and sustaining cotton production on smallholder farming systems, factors affecting cotton production and multi-options for overcoming them have not been adequately addressed in broad spectra (Voora et al., 2023). Some of these options could be to develop cotton by-product value chains, including diversifying the use of cottonseed oil, and cotton stalks, as biofuel and providing complementary sources of revenue for farmers (Voora et al., 2023). Given that the average cotton lint yield on smallholder land-holdings is only ~ 0.4 t ha-1, improvement in profitability would be achieved even with production at 50% of the yield potential > 1.0 t ha-1 (Wakhungu and Wafula, 2013; Constable and Bange, 2015; Khan et al., 2019). Therefore, the driving themes of this review are threefold: - (1) Explore disguised agronomic practices to be endowed for sustainable cotton production on small landholdings; (2) Explore socioeconomic settings based on their disparities in contributing to sustainable cotton production on small landholdings; and (3) Explore existing and feasible institutional policies to be enforced for sustainable cotton production on small landholdings. The findings from this study are expected to shed light on the unravelled options for improving the productivity of cotton systems and farmers’ livelihoods, without compromising the environment in the context of climate change. This review is guided by the assumptions that: - (1) the varieties of cotton used by the smallholder farmers based on the reviewed literature are the most appropriate. (2) Unfavourable climates and extreme weather events would not come into play as erratic under smallholders’ settings since production is mainly through rainfed, without, or with only a little irrigation, and water for irrigation is available throughout the production season whenever required. (3) The information drawn from reviews is as credibly reliable as the information drawn from articles. A detailed search for additional information was done to verify some reviews where the data/information was held in doubt.
The conceptual framework guiding this study is presented in Figure 1. Whereas only nine areas are shown in the presentation, the six areas are discussed as components of three major, namely agronomic practices, socioeconomic settings, and existing and contribution of policy to the cotton-producing systems. The keywords used in the search for relevant literature for this review were borrowed from the conceptualized framework.
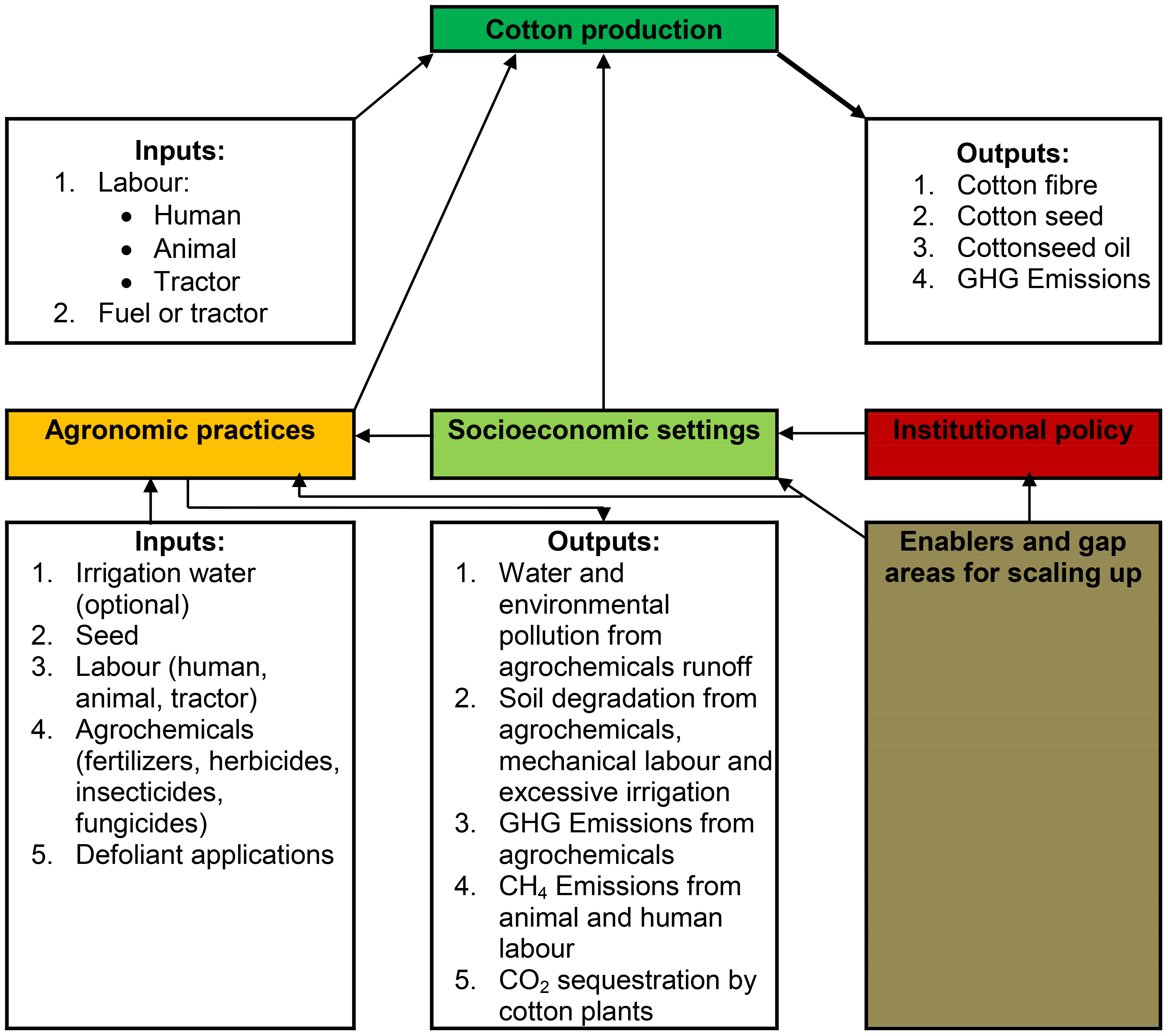
Figure 1 Interlace dependence of cotton producing systems. Partly borrowed and modified from Hoehn (2010), then own conceptualization with the support of literature synthesis.
3 Literature search
This study adopted the Preferred Reporting Items for Systematic Reviews and Meta-Analyses (PRISMA) approach (Page et al., 2020; Nassary et al., 2022) as presented in Figure 2. The PRISMA enhances transparently reporting the reasons for conducting (it answers “why”)?, the activities done by the authors (it answers “what”)?, and the findings of the review (it answers “what”)? (Moher et al., 2009; Page et al., 2020). Page et al. (2020) modified the PRISMA statement of 2009 by including new guidance for reporting reviews that reflects advances in the identification, selection, evaluation, and synthesizing of studies. Other areas of the PRISMA modified by Page et al. (2020) are the structure of the items and their presentation, the inclusion of a 27-item checklist, and flow diagrams for original and updated reviews.
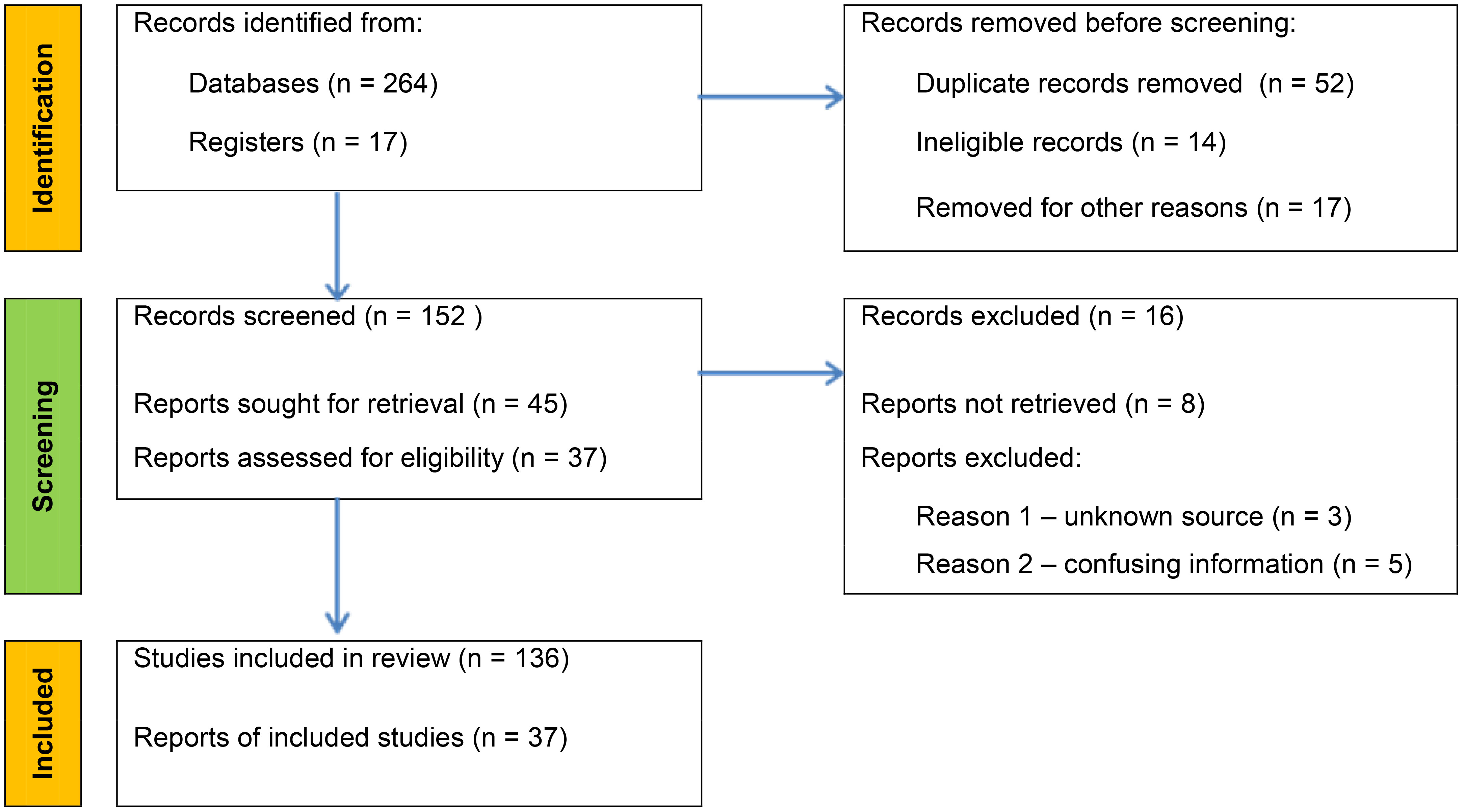
Figure 2 Number of studies identified and included in the study. Modified from PRISMA 2020 flow diagram for new systematic reviews of Page et al. (2020).
Page et al. (2020) provide the checklist for a systematic literature review report, covering various sections such as title, abstract, introduction, methods, results, discussion, and other relevant information, following the PRISMA 2020 for checklist. The title should clearly identify the report as a literature review, whereas the abstract needs to provide a structured summary. The introduction should discuss the rationale and objectives of the review. Methods include specifying eligibility criteria, information sources, search strategy, selection process, and risk of bias assessment. Results involve detailing the study selection process, characteristics, risk of bias, and individual study results. The discussion should interpret results, discuss limitations, and address implications. Other information includes registration details, support sources, competing interests, and the availability of data and materials.
The databases deployed in the present study are MEDLINE (to capture health-related issues of using agro-chemicals in cotton production), the Cochrane Library (to gather information on healthcare decision-making related to the use of agro-chemicals in cotton production), Scopus, and Web of Science. Comprehensively, we also captured studies from Google Scholar and other Grey Literature (Haddaway et al., 2015; Adams et al., 2016). The main search terms used include “cotton + production”; “cotton + global”; “cotton + smallholders”; “cotton + agrochemicals”; “cotton + environments”; “cotton + market”; “cotton + price”; “cotton + climate change”; “cotton + health”; “cotton + livelihoods”; “cotton + soil”; “cotton + microbes”; “cotton + nutrients”; “cotton + policy”; and “cotton + agronomics”. Finally, the total number of studies included after the exclusion of duplicates and redundant sources is 173 (Figure 2).
4 Findings and discussion
The results of the present review are presented in three major categories, which include agronomic practices endowment, socioeconomic settings, and institutional policy enforcement as the elements for sustainable cotton production on small land-holdings.
4.1 Agronomic practices endowment for sustainable cotton production
Important aspects obtained under agronomic practices are summarized in Table 1. Integrated nutrient management practices should be encouraged to promote sustainability in cotton production. These practices include the appropriate use of organic or slow-release fertilizers, precision agriculture techniques to reduce fertilizer use while maintaining or increasing crop productivity, crop rotation, and cover cropping. It is crucial to optimize nutrient uptake by cotton plants and minimize losses to the environment. The details of these agronomic practices are provided in this section.
Fertilization is a key aspect of cotton cultivation that involves providing essential nutrients to the crop for healthy growth, improved yields, and fibre quality. Sustainable fertilization practices aim to optimize nutrient use efficiency while minimizing environmental impacts. A more detailed exploration is provided as the key factors and considerations involved in fertilization for cotton production, with an emphasis on sustainability, depending on soil nutrient management and soil testing; nutrient types such as nitrogen, phosphorus, and potassium (Gascho and Parker, 2001; Savoy and Joines, 2009; Raper et al., 2014; Gabion, 2020). As a first rule of thumb, the cotton plant consumes nitrogen at double the quantity compared to phosphorus and potassium (Duncan and Raper, 2019). Micronutrients such as zinc, iron, and boron are also essential for cotton in small quantities. Other factors important under fertilization include timing of fertilizer application, fertilizer formulation, precision fertilization, sustainable nutrient sources, nutrient efficiency, integrated nutrient management, fertilizer placement, avoiding nutrient overuse, and nutrient cycling (Savoy and Joines, 2009). The different growth stages of cotton plants require nutrients in different amounts. For instance, the highest cotton requirement for nitrogen is during boll filling, with detrimental carry-over into the harvest (Savoy and Joines, 2009). In addition to nitrogen, phosphorus, potassium, sulphur, and boron are nutrients that must be managed appropriately for optimum fibre quality and lint yield of cotton (Yin, 2016; Duncan and Raper, 2019). The highest requirement for potassium and boron occurs during boll filling (Savoy and Joines, 2009). Whereas phosphorus, potassium, calcium, and magnesium are not easily moved in the soil system unless disturbance occurs, nitrogen, boron, and sulphur are reported to be highly vulnerable to losses from the root zone before being taken up by the plant (Savoy and Joines, 2009; Yin, 2016). Given the high demand for nitrogen, phosphorus, and potassium in cotton, the recommendation is to apply these nutrients in splits of N-P-K 20-10-10 at 200 kg ha-1 during sowing and another 200 kg ha-1, during flowering (Savoy and Joines, 2009; Duncan and Raper, 2019). However, regular soil analysis is important before sowing and harvesting, thereby signalling the actual amount of nutrients to be applied (See Table 2).
Other important micronutrients required for cotton production include iron, manganese, zinc, copper, molybdenum, and chlorine (Dordas, 2009; Alejandro et al., 2020). In alkaline and calcareous soils, iron and zinc availability to cotton plants may be reduced due to increased soil pH, leading to deficiencies in plants. Conversely, in acidic soils, iron toxicity can be a concern (Alejandro et al., 2020). In soils with high organic matter content, copper availability may be reduced (Wang et al., 2022). While cotton is not a nitrogen-fixing crop, molybdenum still plays a role in nitrogen metabolism and overall plant health (Rana et al., 2020; Wang et al., 2022). Although required in small amounts, chlorine is involved in water movement within plants and serves as an essential component of photosynthesis (Chen et al., 2010). Besides, not soil test alone or plant tissue analysis alone will provide information on the potential factors that contribute to nutrient deficiency. So, both analyses, plus management history, are needed to better understand the cause of nutrient deficiencies in cotton fields (Savoy and Joines, 2009). Sufficient ranges used for interpreting tissue tests of nutrient concentrations in cotton are adequate for potential yields (Campbell and Plank, 2013; Gabion, 2020). According to Savoy and Joines (2009), these ranges serve as indicators, and values close but outside to this range may not affect cotton performance (See Table 3).
Intensification of agricultural systems is meaningfully productive if it is operated in the context of sustainability, without or with little effect on the environment and biodiversity. However, it becomes pertinent to consider the environmental conservation aspect due to the heavy and inappropriate use of agrochemicals in cotton production by smallholder farmers (EJF, 2007). In contrast to what many think, environmental health is not great for conventional cotton production. Whereas cotton fibres will eventually decompose into the soil and release essential nutrients, the process by which fibres decompose does not make it environmentally inconsequential (EJF, 2007). Given intensification, soil degradation, nutrient runoff, and water pollution are the common environmental issues of excessive and improper use of fertilizers in cotton production (Delate et al., 2021).
The world’s uses of insecticides and pesticides in cotton production are reported to revolve around 25% and 10%, respectively, while the same crop consumes 4% of the world’s synthetic nitrogenous and phosphate fertilizers (Shepherd, 2020). In the US, for example, cotton is reported to be the third crop with the highest pesticide consumption (EJF, 2007; Shepherd, 2020). Delate et al. (2021) reported that diuron, glyphosate, and tribufos out of the top ten highly consumed pesticides in cotton in 2017 were the most carcinogenic to humans. Parvez et al. (2018) reported that the use of glyphosate in cotton production was associated with genetic disruption and congenital disabilities in farmers living in cotton areas. There is limited awareness of the environmental responses associated with pristine white cotton among many people. Fashioning in the textile industry, which involves the use of pesticides, toxic dyes, and bleaching chlorine contributes to about 20% of industrial water pollution (Parvez et al., 2018). The sinks of these pollutants are oceans, underground aquifers, lakes, rivers, and wetlands (EJF, 2007; Parvez et al., 2018; Shepherd, 2020). As a response to these pollutants, environments, wildlife, and humans are directly affected by polluted systems resulting from industries responsible for the processing of fibres and clothes.
Cotton plants serve as a sink for carbon dioxide sequestration through its use as a raw material during photosynthesis. However, fertilizer production and heavy applications in cotton can result in the release of greenhouse gases (GHG), such as nitrous oxide (N2O) and carbon dioxide (CO2). The N2O, in particular, is a potent greenhouse gas, contributing to climate change and global warming (Butterbach-Bahl et al., 2013; Richards et al., 2016; Tian et al., 2019). The impact of 0.5 kg of N2O on warming the atmosphere is ~ 300 times that of 0.5 kg of CO2, and 40% of total world N2O emissions come from human and microbial activities (Parker and Behringer, 2009; Xu et al., 2020; Aryal et al., 2022; Huang et al., 2024). Butterbach-Bahl et al. (2013) indicated that microbial decomposition and production processes are the potential sources of N2O, in water bodies, soils, and sediments. Syakila and Kroeze (2011) reported that the use of nitrogenous fertilizers and farm management with manures in agriculture contribute to 4–6 Tg N2O–N yr−1 while emissions from in situ soils contribute to 6–7 Tg N2O–N yr−1, which accounts for the 60–70% of total global N2O sources.
The use of nitrogenous fertilizers in crop production is contributing to a ~ 20% increase in the concentration of N2O in the atmosphere (Xu et al., 2020). Xu et al. (2020) examined the spatial variability of N2O emissions from cropland during 1961–2014 by portioning the world into 11 regions namely Africa, America (North and South), Asia (i.e., Central, East, North, South, Southeast, and West), Europe, and Oceania. By deploying a process-based dynamic land ecosystem model (DLEM) Xu et al. (2020) found that cropland increased global N2O emissions by 180% (i.e., from 1.1 ± 0.2 to 3.3 ± 0.1 Tg N year−1; mean ±1 standard deviation) during 1961–2014. Tian et al. (2019) examined the combined effects of nitrogenous fertilizers and organic N application in agriculture, deposition of atmospheric N, climate change, changes in land cover, and elevated CO2 concentration in the atmosphere on global soil N2O emissions for the period 1861-2016. Tian et al. (2019) found that global soil N2O emissions increased from 6.3 ± 1.1 Tg N2O-N yr-1 in the pre-industrial period (i.e., the 1860s) to 10.0 ± 2.0 Tg N2O-N yr-1 recently (i.e., 2007-2016), with the cropland accounting for ~ 80% of the total increase. Furthermore, Tian et al. (2019) observed that countries like China and those in South Asia and Southeast Asia had undergone rapid N2O emissions increase in cropland since the 1970s, with the US N2O emissions from cropland being relatively uniform since 1980 while N2O emissions in cropland for the EU decreased by 14% during the same period. The evidence of an increase in the atmospheric N2O concentrations as a result of human activities, and temporal and spatial differences in the existing data sets of nitrogenous fertilizer increase uncertainties. Furthermore, the continuous concentration of N2O emissions to the atmosphere as a result of the combined effects of environmental changes and the use of nitrogenous fertilizer in agriculture remains highly questionable.
Smallholder farmers often face challenges in using synthetic fertilizers for cotton production due to several reasons. First, cost can be a significant barrier. Synthetic fertilizers are often expensive, and smallholder farmers may struggle to afford them (Altenbuchner et al., 2016). Additionally, the initial investment in equipment for applying these fertilizers, such as sprayers, can be a financial burden. Moreover, the knowledge and technical know-how required to properly use synthetic fertilizers might be lacking in some smallholder farming communities. Precision in application is crucial to prevent overuse or misuse, which can lead to environmental degradation and decreased soil fertility over time (Chen et al., 2022b). Access to markets and credit is another issue. Smallholder farmers may find it difficult to access markets that provide quality synthetic fertilizers, and they may lack the financial resources to secure credit for purchasing inputs. Environmental concerns also come into play, as improper use of synthetic fertilizers can contribute to soil and water pollution (Wang et al., 2018). Smallholder farmers, often relying on sustainable and traditional farming practices, may be hesitant to adopt synthetic fertilizers due to environmental awareness or concerns about the long-term impact on soil health (Chen et al., 2022b).
Sowing dates depend on the onset of rainy seasons in rainfed systems or beginning of the cropping season for the irrigated systems, and are important factors affecting overall cotton production, including planting density, boll formation, and lint quality (Tak et al., 2021; Adombilla et al., 2023). Unreliable weather forecast technology and the inability of smallholder farmers to predict the onset of rains in rainfed are a great challenge in deciding when is the appropriate date for sowing cotton (Adombilla et al., 2023). The impact of sowing dates on cotton production needs need to be discussed depending on the production systems as there is no reliance on the onset of rains under irrigated systems. In Pakistan, for example, smallholder farmers depend heavily on the Indus River’s irrigation system, which contributes 97% of the water essential for cotton cultivation, a vital sector that positions the country as the fourth-largest cotton producer globally, following China, India, and the United States (Watto and Mugera, 2015). The reliance on irrigation is necessitated by the brief and unpredictable nature of the rainy season, with precipitation ranging from 155 to 755 mm (Shuli et al., 2018). This circumstance, as highlighted by researchers such as Watto and Mugera (2015) and Shuli et al. (2018), creates a challenge for farmers who are unable to capitalize on water resource for sowing their cotton. The juxtaposition of impressive global cotton demand with the constraints faced by smallholder farmers underscores the complex interplay between agricultural practices, water resources, and climate conditions.
Sowing of cotton too early or too late relative to the usual dates is critical to cotton performance (Tak et al., 2021). For instance, there is more vegetative growth than yield for the early sown cotton (Iqbal et al., 2012). On the other hand, late-sown cotton may be subjected to the opening of bolls during maturity resulting from moisture stress and damage by insect pests (Ali et al., 2009). The selection of appropriate varieties and timing of the sowing dates optimize cotton production, although the overall performance of each variety may vary with agroecologies (Adombilla et al., 2023). Therefore, while the supply of other inputs is optimized, it is important to understand the interactions of those inputs with varieties and sowing dates for optimum cotton production in terms of yield and lint quality. Adombilla et al. (2023) evaluated the interactions of four sowing dates (i.e., mid to late June, early to mid-July, mid to late July, and early August) and three cotton varieties (i.e., FK37, SARCOT1, and SARCOT5). According to Adombilla et al. (2023), sowing mid to late June was superior to other sowing dates, and cotton variety SARCOT5 produced the highest seed cotton yield. Given that sowing dates and cotton variety interactions remain to be clearly evaluated, it is also imperative that these interactions are evaluated along with management options for the specific physiographic locations and the context of climate change.
In addition to the varieties released by research institutions, there are widely genetically modified (GM-GMO) cotton varieties for some qualities including tolerance to herbicides (i.e., glyphosate-based) and resistance to insects (i.e., bollworm) (Rocha-Munive et al., 2018). Whereas GMO cotton is widely accepted worldwide, about 90% is grown in China, the United States, Australia, Argentina, India, Mexico, Pakistan, Paraguay, and South Africa (Terán-Vargas et al., 2005; Rocha-Munive et al., 2018). Mexico, for example, introduced GM cotton because it was difficult to grow conventional cotton varieties (Martínez-Carrillo, 2005; James, 2016). In other observations, Terán-Vargas et al. (2005) emphasized that commercialization and cultivation of GM cotton are escalated by the severe pest pressure on conventional cotton varieties. Rocha-Munive et al. (2018) reported that GM cotton has been commercialized in the United States, Australia, Argentina, Brazil, Burma, Burkina Faso, China, Colombia, Costa Rica, India, Mexico, Pakistan, Paraguay, South Africa, and Sudan). The benefits of GM cotton are yet to be trapped by other countries, which are vulnerable to insect pests and high weed infestation. Furthermore, the environmental pitfalls associated with GM cotton have rarely been addressed in countries where commercialization and cultivation are already in practice (Rocha-Munive et al., 2018).
Precision agriculture technologies, such as remote sensing and GPS-guided equipment, can help farmers apply fertilizers and other inputs more efficiently (Lambert et al., 2015). Lambert et al. (2015) analyzed the adoption patterns of precision agriculture technologies by deployment of a Multiple Indicator Multiple Causation regression model among cotton farmers through remote sensing, yield monitors, soil testing, and soil electrical conductivity and found that the propensity to adopt technology was dependent on the information acquisition and its sources about precision farming. The important identified practices include the rotation of cotton with other crops, the adoption of irrigation systems for cotton, and the availability of programs for environmental conservation (Lambert et al., 2015). Precision technology bundles have been reported to assist farmers in the adoption of appropriate farming practices (Lambert et al., 2015). The widely reported precision bundles are Geographical Positioning System (GPS) and Computerized Cotton Management System (COTMAN), which are decision support software (Bange et al., 2004; Mooney et al., 2010; Pandit et al., 2011; Lambert et al., 2015).
Literature shows that cotton-producing farmers used handheld GPS and COTMAN to map and record growth variables in cotton plants (Bange et al., 2004; Pandit et al., 2011; Zhou et al., 2017). These digitally supported technologies are reported in the USA and Australia, where twelve states in the U.S. out of 1,800 of the studied cotton farmers, the adoption varied within precision farming (Pandit et al., 2011). Based on the study conducted in the twelve U.S. states namely Alabama, Arkansas, Florida, Georgia, Louisiana, Missouri, Mississippi, North and South Carolina, Tennessee, Texas, and Virginia farmers’ constraints to adoption of precision agriculture practices were the cost of inputs, time constraint, and satisfaction with the practice (Paudel et al., 2021). According to Paudel et al. (2021), farmers are more likely to adopt precision farming practices with large cultivated fields, high returns from the total cultivated field, and use computers by the farmers. In Australia, Bange et al. (2004) reported that EntomoLOGIC, part of the CottonLOGIC software has been used to predict future pest infestation in the context of weather data, which provides insight into when pest infestation on cotton is defined in economic thresholds. These bundling technologies remain to be distributed to smallholder farmers in other areas, including those residing in developing countries. Literature has also indicated the significance of precision farming technologies as they accommodate a series of spatial-related information meant to improve farming systems’ profitability through increasing yields while reducing costs incurred from inputs (Larson et al., 2016; Zhou et al., 2017). According to Bongiovanni and Lowenber-Deboer (2004), precision farming technologies provide information about soil and input requirements by the crop within-field and specific to the site. Precision agriculture practices provide farmers with the right amount of agro-input to be applied in the right place and at the right time (Roberts et al., 2004; Larson et al., 2016).
The adoption of technologies in agriculture is highly variable, as it is dependent on the geographical location, the mindset of farmers’ higher expectations from the deployment of the technologies, the social settings of the farming community, and partly the existing policy. Pokhrel et al. (2018) reported a higher adoption rate of water-efficient irrigation technologies, including trickle and sub-surface drip irrigation technologies by farmers after realizing a higher yield from irrigation through the data of a 2013 survey in fourteen states of the United States. According to Pokhrel et al. (2018), whereas higher cotton yields realized from irrigation are one of the reasons for technology adoption, other factors driving technology adoption are the total size of land holding, level of education and literacy in computer use, and the social settings where the farmer is originating. These findings suggest that spatial displacement of the farmer and awareness of technologies in precision cotton production, plus land tenure and its use for cotton production are critical factors in sustainability of the crop. Therefore, creating awareness of farmers to technologies and sensitization on skills impartment is inevitable towards the prosperity of the cotton industry.
Over-reliance on synthetic fertilizers can lead to a decline in soil health and biodiversity. Beneficial soil organisms and microbial communities may be negatively affected, which could reduce soil fertility and overall ecosystem resilience (Bwana et al., 2020). Cotton grown organically does not use chemical pesticides and fertilizers; instead, it uses natural pest management methods, and builds biologically diverse agriculture (Textile Exchange, 2018; Mandumbu et al., 2021). Microbes-root interactions play a crucial role in cotton production by influencing plant growth, nutrient uptake, disease resistance, and overall plant health. Some key aspects of microbes-root interactions in cotton are summarized in Table 4. Plant Growth-Promoting Rhizobacteria (PGPR) are the bacteria that colonize the root zone and stimulate plant growth through various mechanisms (Backer et al., 2018; Verma et al., 2018; Lyu et al., 2023). PGPR can produce plant hormones, solubilize nutrients, enhance nutrient availability, and improve water uptake efficiency. Furthermore, these PGPR can improve the tolerance of crops to environmental stresses (e.g., salinity, heavy metal contamination, heat, and drought), which are partly likely to be exacerbated by the impact of climate change (Bhattacharyya and Jha, 2012; Vocciante et al., 2022; Lyu et al., 2023).
Cotton plants can form symbiotic relationships with nitrogen-fixing bacteria such as Bradyrhizobium and Rhizobium species. These bacteria convert atmospheric nitrogen into a form that cotton plants can use, reducing the need for nitrogen fertilizers. Symbiotic relationships with cotton roots are widely reported including Arbuscular mycorrhizal (AM) fungi that enhance nutrient uptake, especially phosphorus, by extending their hyphae into the soil and increasing the root’s surface area for nutrient absorption (Pereg and McMillan, 2015). Nitrogen-fixing bacteria like rhizobia establish a symbiotic relationship with leguminous cover crops that are often intercropped with cotton (Saghafi et al., 2020; Sharath et al., 2021). These bacteria convert atmospheric nitrogen into a form that the plants can utilize, promoting soil fertility and reducing the need for synthetic fertilizers. Inoculation with N-fixing, P-solubilising, and indole-3- acetic acid (IAA)-producing bacteria such as Acetobacter, Azotobacter, Azospirillum, and Pseudomonas has been reported to increase the number and weight of boll in cotton (Narula et al., 2005; Gomathy et al., 2008). The agronomic performance of cotton has been improved by inoculation with Azospirillum sp., and significant enhancement was on shoot, root, and fibre quality (Nalayini et al., 2010; Dhale et al., 2011). Treatment of cottonseeds with rhizobia isolated from cotton is reported to improve the yield of cotton in Pakistan under low inputs of synthetic fertilizers (Yasmin et al., 2013).
Beneficial microbes, such as certain bacteria and fungi, can act as biocontrol agents against soil-borne pathogens. Pereg and McMillan (2015) compiled the biocontrol agents often used to control common cotton pathogens including Trichoderma virens, Pseudomonas fluoroscens, Streptomyces lydicus, Burkholderia cepacia, Trichoderma harzianum, Cladorrhium foecundissimum, and Bacillus subtilis. Existing evidence of these bicontrol agents in cotton is widely documented in the USA, India, Israel, and Argentina (Howell, 2002; Mansoori et al., 2013). Biocontrol agents compete with pathogens for resources, produce antimicrobial compounds, and stimulate the plant’s defense mechanisms, helping to suppress disease (Pereg and McMillan, 2015). Furthermore, some beneficial microbes can trigger a response in the plant that enhances its resistance to diseases and pests. This phenomenon, known as induced systemic resistance (ISR), involves the activation of the plant’s defense mechanisms by specific microbial signals (Mansoori et al., 2013).
Soil organic matter decomposition presents another important component of microbes-root interactions in cotton plants. Microbes in the root zone play a crucial role in breaking down organic matter, releasing nutrients that become available to plants. This decomposition process contributes to improvement in soil fertility, thereby ensuring a vital contribution to the productivity of the system (Ma et al., 2021a; Ma et al., 2021b; Mooshammer et al., 2022; Zong et al., 2022). Along with these contributions are microbial inoculants. Some farmers use microbial inoculants containing beneficial microorganisms to improve soil health and crop performance. Inoculants can be applied directly to seeds or the soil to establish a beneficial microbial community around the roots (Pereg and McMillan, 2015). Understanding and harnessing these interactions can lead to improved cotton yield, quality, and sustainability. Research into specific microbial strains, their effects on cotton growth, and their interactions with different environmental conditions continues to provide valuable insights for optimizing cotton production (Yang et al., 2022). The information on microbes-roots interactions in cotton plants is presented in a simplified format in Plate 1. It is important to note that this illustration provides a simplified overview of the interactions since the interactions between microbes and roots are complex and can involve various mechanisms and species.
Plate 1: Microbes-roots interactions in cotton plants. These are our own designed typical illustrations for which ChatGPT support is highly acknowledged.
Intercropping, a practice of growing more than one crop simultaneously on the same piece of land is widely practiced by smallholder farmers to diversify food basis, and income and avoid risks of complete failure of one crop before attaining maturity (Reddy and Shaik, 2009; Biswas et al., 2018; Salama et al., 2022). Also, intercropping saves land resources by increasing its productivity, often measured by the land equivalent ratio and relative yield or net returns (Koraddi et al., 1991; Singh and Ahlawat, 2011; Vaghasia and Dobariya, 2019). Intercropping ensures complementarity and sharing of growth resources (i.e., light, nutrients, and moisture), and it optimizes resources use efficiency (Lv et al., 2023). According to Lv et al. (2023), intercropping that involves the adoption of alternate rows between the component crops can increase yield and net return by ~ 20%, and the land equivalent ratio by ~ 30% compared with traditional crop mixtures or rotations.
Cotton is a long-duration and widely spaced crop, occupying large land areas hence its sustainability on small land-holdings can be easily up-scaled through intercropping using compatible and early maturing crop species (Surendran et al., 2016; Kumar et al., 2017; Biswas et al., 2018; Lv et al., 2023). Surendran et al. (2016) reported that the suitable intercrops with cotton plants could be those of grain legumes, including Phaseolus beans, cowpea (Vigna unguiculata (L.) Walp.), green gram (Vigna radiata L. Wildzek), black gram (Vigna mungo (L.) Hepper), soybean (Glycine max), and groundnut (Arachis hypogaea L.), and sesame (Sesamum indicum L.). Metwally et al. (2012) reported the benefits of intercropping cotton with maize (Zea mays L.) in Egypt. According to Sankaranarayanan et al. (2012), additional benefits can be derived from intercrops of cotton with radish (Raphanus sativus L.), coriander (Coriandrum sativum L.), cluster bean (Cyamopsis tetragonoloba L.), beet root (Beta vulgaris subsp. vulgaris), and dolichos (Lablab purpureus (L.) Sweet [Fabaceae]). Intercropped cotton can yield 8% to 31% yields lower than those of sole cultivated cotton, but the total systems’ productivity and net income of intercropping is higher than its counterpart (Reddy and Shaik, 2009; Tariq et al., 2018; Lv et al., 2023). Other studies have shown that the income generated from different intercropping systems of cotton increases by 30% to 40% (Ali et al., 2011; Tariq et al., 2018; Lv et al., 2023).
There exists evidence of benefits derived from cotton intercropped with crops differing in their maturity cycles. Surendran et al. (2016) studied the benefits of intercropping cotton with cowpea, black gram, and green gram and found significant total productivity of the systems to yield the equivalent of cotton relative to the sole cotton system. Vaghasia and Dobariya (2019) assessed the optimum nutrient requirement for the intercrops between Bt cotton and groundnut aiming at higher productivity and profitability. According to the findings obtained by Vaghasia and Dobariya (2019), plant growth and yield attributes were increased in both crops under intercropping. Biswas et al. (2018) examined the benefits of intercropping cotton with black gram and found that optimum uptake of the nutrients N, P, and K was recorded in the intercropping system relative to the sole systems of the two crops. Also, Biswas et al. (2018) indicated that only 75% of the recommended rates of N, P, and K nutrients can optimize nutrient uptake by crops, cotton equivalent yield, seed-cotton yield, and net production value in cotton-black gram intercrops. Apart from the advantages of reducing costs of purchasing full doses of fertilizers containing these nutrients, additional benefits are the provision of food and income generation from black gram and reduced environmental pitfalls resulting from higher doses of N. On the other view, literature shows that most cotton intercrops with other crops are not necessarily meant to alter cotton production but to reduce inputs related to fertilizers, herbicides, insecticides, and fungicides, and maximize land utilization (Miller and Greene, 2018). For example, Sankaranarayanan et al. (2012) reported that fibre maturity, length, ginning percentage, and fineness were not altered by the intercrops with coriander, radish, beet root, cluster bean, vegetable cowpea, and dolichos, whose growth variables were altered by the intercropping systems. There are still many unanswered questions pertaining to crop selection for compatibility with cotton-based intercrops in resource facilitation, complementarity, and sharing for efficient use between companion crops. Furthermore, additional economic and biodiversity benefits to be derived between intercrops of cotton with various food crops under diverse agro-ecologies remain to be critically examined.
Crop rotation is widely known for its agronomic and economic benefits. Crop rotations play a role in soil conservation, improvement of soil nutrients, management of crop-reducing factors (i.e., diseases, insects, and weeds), and enhancement of soil biodiversity (Arnold Bruns, 2017; Arnold Bruns et al., 2018; Xi et al., 2021). Given the nature of the cotton crop, occupying a large land area and taking a relatively longer period in the field, rotational cropping is not common in smallholder settings. However, there is literature finding showing the benefits derived from rotational systems which involve cotton crops. For instance, a common disease of cotton Verticillium wilt caused by Verticillium dahlia is reported to be controlled through cotton-maize rotation in China (Xi et al., 2019; Xi et al., 2021). The fixation of atmospheric N for improvement of the soil nitrogen budget is another widely report benefit of legumes inclusion in rotation with cotton plants (Pettigrew et al., 2016; Arnold Bruns et al., 2018). Bordovsky et al. (1994) examined the productivity of cotton and wheat (Triticum aestivum L.) rotation and found increased lint yields by ~ 13%, relative to sole cotton. Sehgal et al. (2023) evaluated the yield and profitability effects of 17-year rotations of cotton, maize, and soybean and found that lint yields and cotton seed were highest in the maize-cotton-cotton system followed by the maize-cotton system. Khaitov and Allanov (2014) using rotational cropping of cotton–wheat-maize and cotton–wheat–soybean found that the latter combination was superior to sole cotton in soil quality management. A series of examples involving cotton rotation with various crops exist. However, the benefits to be derived by integrating precision agriculture technologies into the system remain to be an important area for further investigation. Furthermore, the interactions between cotton rotational systems with the impact of climate change and extreme weather events are rarely documented. Harnessing precision agriculture technologies in cotton rotational systems may not be practically profitable if the challenges of climate change on systems productivity are not critically addressed. For example, there are concerns that farmers in Uzbekistan often practice the rotation of cotton with wheat (Khaitov and Allanov, 2014), which is not an appropriate choice due to escalated soil degradation. The inclusion of a legume crop that fixes atmospheric N in the systems would be important for sustainable soil conservation.
4.2 Socioeconomic settings for sustainable cotton production
The socioeconomic implications of cotton production are significant and can have wide-ranging effects on various aspects of society, the economy, and the environment. Employment and livelihoods are some of the key socioeconomic implications of cotton production. Cotton production is a labour-intensive industry that provides employment opportunities for millions of people, especially in developing countries (Ahmad et al., 2021). Many smallholder farmers rely on cotton cultivation as a primary source of income. Cotton farming creates both on-farm jobs (i.e., planting, cultivation, and harvesting) and off-farm jobs (i.e., processing, transportation, and marketing), thereby contributing to rural economies (Bachmann, 2012). While cotton production can provide income to farmers and workers, income disparities can exist within the cotton value chain. Farmers’ incomes may fluctuate due to market prices, weather conditions, and input costs, leading to economic instability for some. Additionally, issues like unfair trade practices and exploitative labour conditions can exacerbate income disparities (Bachmann, 2012; Altenbuchner et al., 2016).
All activities related to the cotton chain are important sources of employment and income in many countries, including West and Central Africa, India, and Pakistan (Fei et al., 2022). Many smallholder farmers in cotton-producing areas live below the poverty line and are dependent on the middlemen or ginners who buy their cotton, often at prices below the cost of production (Tarazi et al., 2019; Ahmad et al., 2021). According to Fei et al. (2022), there is a strongly positive linkage between cotton export and economic growth, and its impact on human capital. Worldwide, cotton is the leading produced and commercialized natural fibre (Ahmad, 2014). Over 150 countries are involved in the industrialization of the cotton sector while providing income to more than 100 million households (Tarazi et al., 2019; Meyer, 2020). Fei et al. (2022) indicated that output from cotton production in 2019 was worth ~ USD 46 billion and worth ~ USD 15 billion in global trade. The use of global cotton mills reached 26.7 million tons for the period of 10 yrs compared to 2007/08 and 2018/19 (Fei et al., 2022).
Sex, age, education level, income of farmers, distance from households to fields, and extension services affect farmers’ decision, ability, and capacity to engage in and execute cotton production. Tzouvelekas et al. (2001) conducted an empirical analysis to compare the economics of allocating technologies to conventional and organic cotton-producing systems in Greece and found that the allocation was efficiently similar between the two systems. According to Tzouvelekas et al. (2001), the age and education level of farmers and farm size well explain the discrepancies in resource allocation between contrasting cotton-producing systems. Sodjinou et al. (2015) assessed farmers’ decisions to adopt cotton production practices as affected by socioeconomic and institutional factors through a probit model in Benin. Sodjinou et al. (2015) found that socioeconomic factors, distance from households to fields, and extension services affect farmers’ decision to adopt cotton production technologies. Gender sensitization is another important social factor determining cotton production in smallholder farming settings. For example, Sodjinou et al. (2015) reported that females are more attracted to cotton farming compared with males, thereby increasing their economic independence. Cotton producers are characterized by old age, low levels of education, and low income (Sodjinou et al., 2015). Besides, explorative and situational analysis studies on cotton production should be conducted by considering options of strengthening farmers’ livelihoods, while preserving the environmental resources. In Tanzania, as in other countries dominated by smallholder farmers, cotton production is reported to be an important source of generating income but is accompanied by costs incurred in high pesticide consumption and intensive land cultivation (Altenbuchner et al., 2016). Focusing on smallholder farmers in the rural Meatu district of Tanzania, Altenbuchner et al. (2016) explored farmers’ attitude change to adopt conventional practices of cotton production practices and found that training and extension services are the important factors. According to Altenbuchner et al. (2016), the poverty level displaces the priorities of the farmers from environmental conservation. Altenbuchner et al. (2016) also identified that females display a positive contribution to cotton production, but polygamy and gender disparities are the common cultural preconditions challenging cotton farmers. Other important social problems facing cotton smallholder farmers reported by Altenbuchner et al. (2016) are high birth rates and poor levels of education.
Despite its advantages of generating income for smallholder farmers through labour, cotton-producing systems are reported to involve children in field operations, including cultivating, spraying of pesticides, harvesting of cotton, ginning fibres and manufacturing of clothes (Herring and Grodzins Gold, 2005; WHO, 2005; EJF, 2007). Literature shows that the cotton industry drives child labour and forced labour in at least 18 countries (Human Rights Watch, 2010; United States Department of Labour, 2011; World Vision Australia, 2012). Globally, the countries with high child labour record in the cotton industry include Kazakhstan, China, USA, Uzbekistan, Azerbaijan, Turkey, Turkmenistan, Tajikistan, Egypt, Kyrgyzstan, Pakistan, India, Mali, Burkina Faso, Benin, Brazil, Paraguay, Zambia, and Argentina (World Vision Australia, 2012; Kazianga and Makamu, 2016). Apart from low pays, children are also vulnerable to poisonings occurring during cotton production. According to EJF (2007), children in Uzbekistan and India are involved directly in spraying of pesticide. In other countries such as Egypt, India, Pakistan, Tajikistan, Turkmenistan, and Uzbekistan children often work during, or immediately after, the spraying season thereby coinciding with high levels of pesticide residues (EJF, 2007). Besides these scenarios there is limited evidence of ill-health documented among children involved in spraying pesticides in cotton fields, and this increase a need for detailed studies to establish grounds of reliable evidence.
Nevertheless, global trading schemes and climate-environmental factors such as water scarcity, floods, high temperatures, and drought are not likely to be offset by the adoption of cotton production technologies by smallholder farmers. Altenbuchner et al. (2018) evaluated intensively cultivated cotton by smallholder farmers in India and identified that training for capacity building and institutional policy were the important areas impacting the system’s productivity for the crop. According to Altenbuchner et al. (2018), strengthening cotton production is gaining acceptance by females more than males, although environmental factors are increasingly challenging. However, Salihu and Singh (2020) studied 120 cotton farmers through a multistage sampling technique in four villages in India and found that gender, farm size owned and cultivated and education levels of the farmers were not the only factors driving higher cotton output. According to Salihu and Singh (2020), profitable cotton production is a combination of cross-cutting factors ranging from agronomic to policy through social settings. The interactions among agronomic factors, socioeconomics, and policy aspects remain to be critically evaluated among cotton small landholdings in diverse areas of the world, with much focus on developing countries.
Fair trade and market prices for cotton improve livelihoods for smallholder farmers through higher total returns, with low costs incurred in inputs and little risks (Bachmann, 2012). However, there is a limited study comparing the profitability of various cotton-producing systems among developing countries and/or against developed countries. Bachmann (2012) examined socioeconomic and environmental factors affecting cotton production in Central Asia and found that cotton yields were 20% higher relative to lower costs of inputs. Availability and access to credits, appropriate seed, extension services, and diversified uses of processed cotton produce improve farmers’ attitudes toward the adoption of technologies of cotton production (Bachmann, 2012). It is also challenging that cotton production is labour-intensive depending on the system of production, including traditional, conventional, and/or organic (Bachmann, 2012; Altenbuchner et al., 2016; Altenbuchner et al., 2018). Therefore, factors of production such as those involved in inputs, field operations, and processing altogether remain to be studied through a multi-factor model that describes their main and interaction effects on cotton production. For example, Bachmann (2012) reported that organic cotton production is characterized by a higher workload, which is counteracted by the improvement in the livelihoods of smallholder farmers. Altenbuchner et al. (2018) also reported that the profitability of cotton-producing technologies to smallholder farmers was associated with lower costs incurred in production, reduced dependency on credit lenders, improved soil conditions, and little exposure to toxic agrochemicals.
The socioeconomic implications of cotton production are closely tied to environmental concerns (Ahmad et al., 2021). Conventional cotton farming often involves the use of pesticides, herbicides, and synthetic fertilizers, which can lead to environmental degradation, soil degradation, and water pollution. Transitioning to more sustainable and organic farming practices can mitigate these negative effects. Pesticide use in conventional cotton farming can have adverse health effects on farmers, farm workers, and nearby communities. Exposure to these chemicals can lead to health issues and even chronic diseases (EJF, 2007). Addressing these health concerns is essential for ensuring the well-being of individuals involved in cotton production. Cotton is a globally traded commodity, and its production can significantly impact the economies of both producing and consuming countries (Bachmann, 2012). Fluctuations in cotton prices can influence the income of farmers and the profitability of cotton-related industries. Changes in global trade dynamics, including tariffs and subsidies, can affect the competitiveness of cotton-producing nations.
The cotton industry’s socioeconomic implications extend to social welfare issues such as child labour, forced labour, and poor working conditions (Mi et al., 2020). In some regions, unethical labour practices have been reported in cotton farming and textile industries (EJF, 2007). Addressing these issues is crucial for ensuring ethical and sustainable cotton production. Cotton is a major raw material for the textile and apparel industry, which is a significant contributor to economies worldwide. The success of cotton production directly impacts the textile industry’s supply chain, affecting jobs and economic growth in this sector. Advancements in agricultural technology can impact cotton production’s socioeconomic implications (Khor and Feike, 2017). The adoption of genetically modified cotton varieties, for example, can affect yields, pest resistance, and overall productivity. However, the adoption of such technologies also raises debates about environmental and health impacts, as well as concerns over seed patents and control.
4.3 Institutional policy enforcement for sustainable cotton production
Herein, we capture the key policies from relevant authorities that align with sustainable agriculture, and have a direct or indirect impact on cotton production. This section seeks to explore existing opportunities emanating from collaboration between players of cotton industry including governments, non-government agencies, and private sectors. Policies associated with cotton production are summarized in Table 5. These policies include initiatives that have also been critically mapped in the present study including land and/or soil management, inputs usage, water and/or moisture management, and return from labour practices, etc.
Policy implications in cotton production have far-reaching impacts on both the agricultural sector and the socioeconomic fabric of societies (Baffes, 2007). Here is an exploration of some key policy considerations and their effects on cotton production and socioeconomic aspects. Government subsidies provided to cotton farmers can influence production decisions, input use, and market prices. These subsidies can incentivize cotton cultivation, affecting the supply-demand dynamics and global cotton markets. However, overreliance on subsidies may distort market signals and impact trade relations. Policies promoting sustainable practices such as organic farming, reduced chemical use, and soil conservation have the potential to enhance long-term productivity while minimizing environmental impacts (Fei et al., 2022). These policies on sustainable practices can improve soil health, reduce water pollution, and contribute to ecosystem conservation. However, transition to these practices may require education, training, and financial support for farmers. Investment in agricultural research and development can lead to the development of high-yield, disease-resistant cotton varieties and innovative farming techniques (Najib et al., 2022). Research-driven advancements can boost productivity, improve fibre quality, and enhance pest and disease management. However, access to these technologies should be equitable to prevent inequalities among farmers. Water-use policies, including regulations on irrigation practices and water pricing, can influence water management in cotton cultivation (Djanibekov et al., 2013; Khor and Feike, 2017; Fan et al., 2022). Efficient water management ensures sustainable use and prevents over-extraction, particularly in water-scarce regions. Well-managed policies can balance agricultural needs with broader water resource conservation. Integrated Pest Management (IPM) policies that promote the judicious use of pesticides and encourage biological control methods can minimize chemical inputs (Najib et al., 2022). IPM can reduce environmental pollution, safeguard human health, and prevent pest resistance. Implementation requires farmer education and support for adopting IPM strategies.
Policies on trade and export agreements and tariffs can influence cotton exports and imports, impacting market access and price stability. Favourable trade policies can enhance market opportunities for cotton producers, contributing to economic growth (Partzsch and Kemper, 2019; Meyer, 2020). However, fluctuating trade conditions can pose challenges to stability. These policies are also linked to the policies supporting fair pricing, market access, and value chain development can ensure a stable income for cotton farmers (Amao et al., 2021). Policies on fair pricing mechanisms help safeguard farmers’ livelihoods and support rural economies (Partzsch et al., 2019). However, market fluctuations and global competition can still pose challenges. Policies focusing on rural development, infrastructure improvement, and skill enhancement in cotton-growing regions can strengthen local economies (Ahmad and Afzal, 2020; Kothari et al., 2021). Such policies can reduce rural-urban migration, create employment opportunities, and enhance overall living conditions in cotton-producing communities. Programs targeting cotton farmers, such as insurance schemes, credit availability, and social safety nets, can mitigate risks and improve farmers’ resilience (Sabesh and Prakash, 2018). These programs provide support during adverse conditions, enhancing farmers’ ability to cope with uncertainties and promoting social equity. Similarly, this is picked by policies promoting gender equality and labour rights in cotton farming and ensuring fair labour practices and that can improve working conditions and empower marginalized groups (Singh and Dusanj-Lenz, 2019; Wang et al., 2021). The impact of such policies is to contribute to social development, reduce exploitation, and enhance the overall well-being of cotton farming communities (Wang et al., 2021).
Policy set-up on cotton worldwide is diversely presented given the need for adoption of conventional farming. A study conducted by Hoehn (2010) indicated that the global cotton production is conventionally exercised by 80%. However, earlier than 2010, Kooistra et al. (2006) reported that the majority of conventional cotton production was from China (24%), USA (19%) and India (16%). Emphasis on the same policy was reported by Marquardt (2011) insisting global position of convention cotton production by ~0.8%. So far reported as developing countries in parts of West Africa, Pakistan, India, Uzbekistan, and China have quite an independent policy on cotton production, with cultivation practised on intensively small and mixed cropped farms by 2009. Leaning more on cultivation of cotton in these farms remains the policy because of its high value, with other crops integrated throughout the year (Conrad et al., 2016). According to Hoehn (2010), regulations on the use of agro-chemicals in developing countries are quietly displaced, thereby increasing health risks and environmental jeopardy. In contrast, developed countries such as Europe, United States, Turkey, and Australia are characterized by large farms usually over 20 hectares, with cotton produced as sole crop (Hoehn, 2010). Government’s policy is profit maximization through cotton, with heavy investment in mechanization and chemical defoliants (Kooistra et al., 2006). Pantzios et al. (2006) examined efficient resource use by 172 cotton-growing farms under the EU policy regime and found that cotton farms are not efficient attributed to the policy governing cotton sector throughout EU.
5 Conclusions
Farming systems significantly influence cotton production, encompassing various impacts on yield, resource efficiency, soil health, pest management, environmental sustainability, economic viability, social and ethical considerations, climate resilience, and market access. By adopting sustainable, efficient, and climate-smart practices, the cotton industry can foster long-term success and contribute to a more sustainable global agricultural landscape. Policymakers, stakeholders, and cotton growers should collaborate to promote farming systems that balance productivity, profitability, and environmental stewardship. Appropriate fertilizer use in cotton production can significantly impact sustainability. Balancing the need for increased crop yields with environmental conservation is essential to ensure the long-term viability of cotton farming. Sustainable practices, precision agriculture, and ongoing research are vital for minimizing the negative environmental effects of fertilizer use while maintaining productivity and promoting the sustainable growth of the cotton industry. It is important to note that nutrient distribution in soils can be highly influenced by the specific context, geographical location, and agricultural practices employed in cotton production. By implementing precision techniques, utilizing organic inputs, and focusing on nutrient use efficiency, farmers can promote both productivity and sustainability in their cotton fields while minimizing negative impacts on ecosystems and water resources.
The socioeconomic implications of cotton production are complex and multifaceted, involving various stakeholders from farmers and workers to policymakers, consumers, and environmentalists. Balancing economic growth, environmental sustainability, and social equity within the cotton industry requires collaborative efforts and a holistic approach to address the challenges and opportunities it presents.
Policy implications in cotton production extend beyond agricultural considerations to socio-economic dimensions. Well-designed policies that balance environmental sustainability, economic growth, social equity, and market stability are essential for fostering a resilient and thriving cotton sector that benefits both farmers and society as a whole. It is also important to beware that the cotton industry is dynamic and can be influenced by various factors, including technological advancements, economic shifts, and policy changes. To get the most accurate and up-to-date information about cotton production, this study recommends continuously consulting recent and advancing agricultural research papers, industry reports, and news from reputable sources in the field of agriculture and textiles. Therefore, systematic reviews that compile and analyze multiple studies on this topic can provide valuable insights into the variations and trends in cotton production.
This systematic review provides valuable insights but also highlights several limitations and areas for future research. The limitations include issues of expanded coverage of the review, lack of specific trial data on some methods and economic considerations, high economic disparity among farmers in regions where data was acquired, and a focus on certain regions and farmer perspectives.
Based on the findings of his review, the suggested research directions encompass a range of topics, from precision agriculture and soil-specific recommendations to exploring the role of microbial interactions and the socio-economic impact of genetically modified cotton. Future studies could also delve into educational interventions, policy implications, and market access for smallholder farmers, ultimately aiming for a more comprehensive and globally applicable understanding of sustainable cotton production.
Author contributions
JT: Conceptualization, Data curation, Investigation, Methodology, Resources, Software, Validation, Visualization, Writing – original draft, Writing – review & editing. GM: Data curation, Formal Analysis, Methodology, Resources, Software, Supervision, Validation, Visualization, Writing – review & editing. EN: Data curation, Formal Analysis, Methodology, Software, Supervision, Validation, Visualization, Writing – review & editing.
Funding
The author(s) declare that no financial support was received for the research, authorship, and/or publication of this article.
Conflict of interest
The authors declare that the research was conducted in the absence of any commercial or financial relationships that could be construed as a potential conflict of interest.
Publisher’s note
All claims expressed in this article are solely those of the authors and do not necessarily represent those of their affiliated organizations, or those of the publisher, the editors and the reviewers. Any product that may be evaluated in this article, or claim that may be made by its manufacturer, is not guaranteed or endorsed by the publisher.
References
Adams J., Hillier-Brown F. C., Moore H. J., Lake A. A., Araujo-Soares V., White M., et al. (2016). Searching and synthesising ‘grey literature’ and ‘grey information’ in public health: critical reflections on three case studies. Systematic Rev. 5, 164. doi: 10.1186/s13643-016-0337-y
Adombilla R., Chamba E. B., Kangben F. Y., Sesay M. J. (2023). Optimum sowing dates for cotton varieties in the Guinea Savanna agroecological zone of Ghana. Afr. Crop Sci. J. 31 (2), 175–189. doi: 10.4314/acsj.v31i2.4
AGOA (2011). “Tanzania: Up cotton, textile production,” in THE CITIZEN. (The Citizen, Tanzania: US Africa Growth and Opportunity Act). Available at: www.agoa.info/news/article/4683-Tanzania-up-cotton-textile-production.html.
Ahmad S. (2014). “Cotton production and uses agronomy,” in Crop protection and postharvest technologies (Singapore: Springer Nature). doi: 10.1002/14356007.a22
Ahmad D., Afzal M. (2020). Climate change adaptation impact on cash crop productivity and income in Punjab province of Pakistan. Environ. Sci. pollut. Res. International 27 24, 30767–30777. doi: 10.1007/s11356-020-09368-x
Ahmad S., Huifang W., Akhtar S., Imran S., Yousaf H., Wang C., et al. (2021). Impact assessment of better management practices of cotton: a sociological study of southern Punjab, Pakistan. Pakistan J. Agric. Sci. 58 (1), 291–300. doi: 10.21162/PAKJAS/21.227
Alejandro S., Höller S., Meier B., Peiter E. (2020). Manganese in plants: From acquisition to subcellular allocation. Front. Plant Sci. 11. doi: 10.3389/fpls.2020.00300
Ali H., Afzal M. N., Ahmad F. S., Atif R. (2011). Effect of sowing dates, plant spacing and nitrogen application on growth and productivity on cotton crop. Int. J. Sci. Eng. Res. 2, 1–6.
Ali H., Afzal M. N., Ahmad S., Muhammad D. (2009). Effect of cultivars and sowing dates on yield and quality of Gossypium hirsutum L. crop. J. Food Agric. Environ. 7, 244–247.
Altenbuchner C., Larcher M., Vogel S. (2016). The impact of organic cotton cultivation on the livelihood of smallholder farmers in Meatu district, Tanzania. Renewable Agric. Food Syst. 31 (1), 22–36. doi: 10.1017/S1742170514000416
Altenbuchner C., Vogel S., Larcher M (2018). Social, economic and environmental impacts of organic cotton production on the livelihood of smallholder farmers in Odisha, India. Renewable Agric. Food Syst. 33 (4), 373–385. doi: 10.1017/S174217051700014X
Amao O. D., Antwi M. A., Oduniyi O. S., Oni T. O., Rubhara T. T. (2021). Performance of agricultural export products on economic growth in Nigeria. Asian J. Agric. Rural Dev. 11 (1), 47–52. doi: 10.18488/journal.ajard.2021.111.47.52
Anwar M., Chaudhry I. S., Khan M. B. (2009). Factors affecting cotton production in Pakistan: empirical evidence from Multan District. J. Qual. Technol. Manage. 5 (2), 91–100.
Arnold Bruns H. (2017). Effects of boron foliar fertilization on irrigated soybean (Glycine max L. Merr.) in the Mississippi River Valley Delta of the mid-south, USA. Arch. Agric. Environ. Sci. 2 (3), 167–169.
Arnold Bruns H. A., Reddy K., Pettigrew W. T. (2018). Effects of rotation of cotton (Gossypium hirsutum L.) and soybean [Glycine max (L.) Merr.] crops on soil fertility in Elizabeth, Mississippi, USA. Arch. Agric. Environ. Sci. 3 (1), 86–88. doi: 10.26832/24566632.2018.0301013
Arshad M. U., Zhao Y., Hanif O., Fatima F. (2022). Evolution of overall cotton production and its determinants: implications for developing countries using Pakistan case. Sustainability 14 (2), 840. doi: 10.3390/su14020840
Aryal B., Gurung R., Camargo A. F., Fongaro G., Treichel H., Mainali B., et al. (2022). Nitrous oxide emission in altered nitrogen cycle and implications for climate change. Environ. pollut. 314, 120272. doi: 10.1016/j.envpol.2022.120272
Bachmann F. (2012). Potential and limitations of organic and fair trade cotton for improving livelihoods of smallholders: Evidence from Central Asia. Renewable Agric. Food Syst. 27 (2), 138–147. doi: 10.1017/S1742170511000202
Backer R., Rokem J. S., Ilangumaran G., Lamont J., Praslickova D., Ricci E., et al. (2018). Plant growth-promoting rhizobacteria: context, mechanisms of action, and roadmap to commercialization of biostimulants for sustainable agriculture. Front. Plant Sci. 9. doi: 10.3389/fpls.2018.01473
Baffes J. (2007). The cotton problem in West and Central Africa: the case for domestic reforms. CATO Institute. Econ. Dev. Bull. No. 11. Available at: http://www.cato.org/pubs/edb/edb11.html.
Bange M. P., Deutscher S. A., Larsen D., Linsley D., Whiteside S. (2004). A handheld decision support system to facilitate improved insect pest management in Australian cotton systems. Comput. Electron. Agriculture 43, 131–147. doi: 10.1016/j.compag.2003.12.003
Bell A., Howell C., Stipanovic R. (2010). “Cotton host-microbe interactions,” in Physiology of cotton. Eds. Stewart J. M., Oosterhuis D. M., Heitholt J. J., Mauney J. R. (Dordrecht: Springer). doi: 10.1007/978-90-481-3195-2_18
Bhattacharyya P. N., Jha D. K. (2012). Plant growth-promoting rhizobacteria (PGPR): emergence in agriculture. World J. Microbiol. Biotechnol. 28 (4), 1327–1350. doi: 10.1007/s11274-011-0979-9
Biswas S., Saha A., Mondal S. S. (2018). Response of integrated nutrient management and intercropping in cotton in new alluvial zone of West Bengal. J. Pharmacognosy Phytochem. 7 (6), 1334–1337.
Bongiovanni R., Lowenber-Deboer J. (2004). Precision agriculture and sustainability. Precis. Agric. 5, 359–387. doi: 10.1023/B:PRAG.0000040806.39604.aa
Bordovsky J. P., Lyle W. M., Keeling J. W. (1994). Crop rotation and tillage effects on soil water and cotton yield. Agron. J. 86 (1), 1–6. doi: 10.2134/agronj1994.00021962008600010001x
Bouchaud C., Clapham A., Newton C., Thanheiser U., Tallet G. (2018). Cottoning on to cotton (spp.) in Arabia and Africa during Antiquity. Eds. Mercuri A. M., D’Andrea A. C., Fornaciari R., Höhn A. (Cham: Springer International Publishing), 380–426. doi: 10.1007/978-3-319-89839-1_18
Butterbach-Bahl K., Baggs E. M., Dannenmann M., Kiese R., Zechmeister-Boltenstern S. (2013). Nitrous oxide emissions from soils: how well do we understand the processes and their controls? Phil. Trans. R. Soc B 368 (30122), 20130122. doi: 10.1098/rstb.2013.0122
Bwana T. N., Amuri N. A., Semu E., Olesen J. E., Henningsen A., Baha M. R., et al. (2020). “Yield and profitability of cotton grown under smallholder organic and conventional cotton farming systems in Meatu District, Tanzania,” in Climate impacts on agricultural and natural resource sustainability in africa. Eds. Singh B., Safalaoh A., Amuri N., Eik L., Sitaula B., Lal R. (Cham: Springer). doi: 10.1007/978-3-030-37537-9_10
Bwana T. N., Amuri N. A., Semu E., Elsgaard L., Butterbach-Bahl K., Pelster D. E., et al. (2021). Soil N2O emission from organic and conventional cotton farming in Northern Tanzania. Sci. Total Environ. 1 (785), 147301. doi: 10.1016/j.scitotenv.2021.147301
Campbell R. A., Plank C. O. (2013). “Corn,” in Reference sufficiency ranges for plant analysis in the southern region of the United States. South. Coop. Ser. Bull. 394. Ed. Campbell C. R. (NC: South Region Agric. Experiment Stn.), 11–14. Available at: http://www.ncagr.gov/agronomi/saaesd/scsb394.pdf.
Çelik S. (2023). Assessing drought tolerance in a large number of upland cotton plants (Gossypium hirsutum L.) under different irrigation regimes at the seedling stage. Life 13 (10), 2067. doi: 10.3390/life13102067
Chen Y., Fu X., Liu Y. (2022b). Effect of farmland scale on farmers’ application behavior with organic fertilizer. Int. J. Environ. Res. Public Health 19, 9. doi: 10.3390/ijerph19094967
Chen W., He Z. L., Yang X. E., Mishra S., Stoffella P. J. (2010). Chlorine nutrition of higher plants: Progress and perspectives. J. Plant Nutr. 33 (7), 943–952. doi: 10.1080/01904160903242417
Chen D., Lu Y., Li Z., Young S. (2022a). Performance evaluation of deep transfer learning on multi-class identification of common weed species in cotton production systems. Comput. Electron. Agric. 198, 107091. doi: 10.1016/j.compag.2022.107091
Conrad C., Lamers J. P. A., Ibragimov N., Löw F., Martius C. (2016). Analysing irrigated crop rotation patterns in arid Uzbekistan by the means of remote sensing: A case study on post-Soviet agricultural land use. J. Arid Environments 124, 150–159. doi: 10.1016/j.jaridenv.2015.08.008
Constable G. A., Bange M. P. (2015). The yield potential of cotton (Gossypium hirsutum L.). Field Crops Res. 182, 98–106. doi: 10.1016/j.fcr.2015.07.017
Coppens d'Eeckenbrugge G., Lacape J. M. (2014). Distribution and differentiation of wild, feral, and cultivated populations of perennial upland cotton (Gossypium hirsutum L.) in Mesoamerica and the Caribbean. Plos One 9 (9), e107458. doi: 10.1371/journal.pone.0107458
Cunneen H., Owain E. (2021). “Physical climate risk for global cotton production: Global analysis,” in Forum for the future cotton 2040 & Acclimatise. (London, UK: Forum for the Future and Willis Towers Watson). Available at: https://www.google.com/url?sa=t&rct=j&q=&esrc=s&source=web&cd=&cad=rja&uact=8&ved=2ahUKEwi0u7-p1oj8AhXQK0QIHRawDVcQFnoECAkQAQ&url=https%3A%2F%2Fwww.wtwco.com%2F-%2Fmedia%2FWTW%2FInsights%2Fcampaigns%2FWTW-9650-Cotton-2040-May21-ExecSummaryGA-v9.pdf%3Fmo.
De Araújo A. F., Cavalcante E. S., Lacerda C. F., De Albuquerque F. A., Richeds J., Lopes F. B., et al. (2022). Fiber quality, yield, and profitability of cotton in response to supplemental irrigation with treated wastewater and NPK fertilization. Agronomy 12 (10), 2527. doi: 10.3390/agronomy12102527
Delate K., Heller B., Shade J. (2021). Organic cotton production may alleviate the environmental impacts of intensive conventional cotton production. Renewable Agric. Food Syst. 36, 405–412. doi: 10.1017/S1742170520000356
Devi M., Mishra P., Malik D. P., Mehala V., Mehta V. P., Bhardwaj N. (2019). Study of climatic factors affecting the productivity of cotton and its instability. Economic Affairs 64 (4), 761–767. doi: 10.30954/0424-2513.4.2019.11
Dhale D., Chatte S., Jadhav V. T. (2011). Response of bioinoculents on growth, yield and fiber quality of cotton under irrigation. Agric. Biol. J. North America 2 (2), 376–386. doi: 10.5251/abjna.2011.2.2.376.386
Djanibekov N., Sommer R., Djanibekov U. (2013). Evaluation of effects of cotton policy changes on land and water use in Uzbekistan: Application of a bio-economic farm model at the level of a water users association. Agric. Syst. 118, 1–13. doi: 10.1016/j.agsy.2013.02.004
Dordas C. (2009). Foliar application of manganese increases seed yield and improves seed quality of cotton grown on calcareous soils. J. Plant Nutr. 32 (1), 160–176. doi: 10.1080/01904160802609013
Duncan L. A., Raper T. B. (2019). Cotton nitrogen management in tennessee UT extension, W. (US: Extension Institute of Agriculture, The University of Tennessee), Vol. 783.
EJF (2007). The deadly chemicals in cotton: environmental justice foundation in collaboration with pesticide action network UK (London, UK: Environmental Justice Foundation and Pesticide Action Network).
Estur G., Gergely N. (2010). The economics of roller ginning technology and implications for African Cotton Sector. Econ. Roll. Ginning Technol. Implications Afr. Cotton Sector 129, 129. doi: 10.1596/27585
Fan Y., Himanshu S. K., Ale S., DeLaune P. B., Zhang T., Park S. C., et al. (2022). The synergy between water conservation and economic profitability of adopting alternative irrigation systems for cotton production in the Texas High Plains. Agric. Water Manage. 262, 107386. doi: 10.1016/j.agwat.2021.107386
Fei C., Dilanchiev A., Romaric S. (2022). Modeling the impact of cotton production on economic development in Benin: A Technological Innovation Perspective. Front. Environ. Sci. 10. doi: 10.3389/fenvs.2022.926350
Food and Agriculture Organization of the United Nations (2019) FAOSTAT-data. Available at: http://www.fao.org/faostat/en/#data.
Food and Agriculture Organization of the United Nations (2021) Recent trends and prospects in the world cotton market and policy developments. Available at: https://www.fao.org/3/cb4589en/cb4589en.
Gabion L. (2020). Fertilization: 2020 cotton information. (North Carolina: NC State Extension, NC State University).
Gascho G. J., Parker M. B. (2001). Long-term liming effects on coastal plain soils and crops. Agron. J. 93 (6), 1305–1315. doi: 10.2134/agronj2001.1305
Gomathy M., Sathya Prakash D., Thangaraju M., Sundaram S. P., Manicka Sundaram P. (2008). Impact of biofertigation of Azophosmet on cotton yield under drip irrigation. Res. J. Agric. Biol. Sci. 4 (6), 695.
Grain Central (2020) COVID-19 impacts global cotton sector: USDA. Available at: https://www.graincentral.com/cropping/cotton/covid-19-impacts-global-cotton-sector-usda/.
Haddaway N. R., Collins A. M., Coughlin D., Kirk S. (2015). The role of google scholar in evidence reviews and its applicability to grey literature searching. PloS One 10, 9. doi: 10.1371/journal.pone.0138237
Herring R., Grodzins Gold A. (2005). Is there a case for growing cotton in India? Indian cotton: biology and utility, meanings and histories workshop, Cornell University, April 29-30, 2005. A joint venture of development, governance and nature and the title VI national resource center for South Asia, Syracuse and Cornell Universities.
Hoehn R. L. (2010) From seed to harvest: A comparative life cycle assessment of conventional vs. organic cotton agriculture. Masters Theses. Available at: https://commons.lib.jmu.edu/master201019/425.
Howell C. (2002). Cotton seedling preemergence damping-off incited by Rhizopus oryzae and Pythium spp. and its biological control with Trichoderma spp. Phytopathology 92 (2), 177–180. doi: 10.1094/phyto.2002.92.2.177
Huang G., Huang J.-Q., Chen X.-Y., Yu-Xian Zhu Y.-X. (2021). Recent advances and future perspectives in cotton research. Annu. Rev. Plant Biol. 72 (1), 437–462. doi: 10.1146/annurev-arplant-080720113241
Huang R., Tao Y., Chen W., Lei L., Li S., Yang L. (2024). Enhancing aerosol emission reduction in an ammonia-based WFGD system with tray implementation. Fuel 355, 129522. doi: 10.1016/j.fuel.2023.129522
Hughes K. (2021). “Climate change – Impacts and mitigation in the cotton sector [Interview],” in Bremen cotton exchange. (Bremen, Germany: Fibre Institute Bremen). Available at: https://neu.baumwollboerse.de/en/2021/06/22/climate-change-impactsand-mitigation-in-the-cotton-sector/.
Human Rights Watch (2010) Fields of peril: child labour in US agriculture. Available at: http://www.hrw.org/sites/default/files/reports/crd0510webwcover_1.
International Cotton Advisory Committee (ICAC) (2005) Cotton production practices. Available at: http://www.icac.org.
Iqbal B., Kong F., Ullah I., Ali S., Li H., Wang J., et al. (2020). Phosphorus application improves the cotton yield by enhancing reproductive organ biomass and nutrient accumulation in two cotton cultivars with different phosphorus sensitivity. Agronomy 10 (2), 153. doi: 10.3390/agronomy10020153
Iqbal J., Wajid S. A., Ahmad A., Arshad M. (2012). Comparative studies on seed cotton yield in relation to nitrogen rates and sowing dates under diverse agroenvironment of Punjab. Pakistan J. Agric. Sci. 64 (1), 59–63.
James C. (2016). Global status of commercialized biotech/GM crops: 2016 (Ithaca, NY: ISAAA Brief No. 52. ISAAA).
Jans Y., von Bloh W., Schaphoff S., Müller C. (2020). “Global cotton production under climate change – Implications for yield and water consumption,” in Hydrology and earth system sciences. (US: Committee on Environment, Geography and Urbanization (CEGU), University of Chicago). Available at: https://hess.copernicus.org/preprints/hess-2019-595/hess-2019-595.pdf.
Johnson J., MacDonald S., Meyer L., Soley G. (2022). “The world and United States cotton outlook [Presentation],” in United states department of agriculture’s 9th annual agricultural outlook forum ([virtual]. (US: U.S. Department of Agriculture). Available at: https://www.usda.gov/sites/default/files/documents/2022AOF-cotton-outlook.pdf.
Kazianga H., Makamu F. (2016). Crop choice, school participation, and child labor in developing countries: Cotton expansion in Burkina Faso. Am. J. Agric. Economics 99 (1), 34–54. doi: 10.1093/ajae/aaw061
Khaitov B., Allanov K. (2014). Crop rotation with no-till methods in cotton production of Uzbekistan. Eurasian J. Soil Sci. 3, 28–32. doi: 10.18393/ejss.52631
Khan N., Han Y., Xing F., Feng L., Wang Z., Wang G., et al. (2019). Plant density influences reproductive growth, lint yield and boll spatial distribution of cotton. Agronomy 10 (1), 14. doi: 10.3390/agronomy10010014
Khor L. Y., Feike T. (2017). Economic sustainability of irrigation practices in arid cotton production. . Water Resour. Economics 20, 40–52. doi: 10.1016/j.wre.2017.10.004
Kone Y., Sissoko M., Assima A., Keita N. (2020). “Why could the COVID-19 cotton crisis lead to an economic and social crisis in Mali,” in Food security group, department of agricultural, food, and resource economics, michigan state university. (US: Department of Agricultural, Food, and Resource Economics Food Security Group, Michigan State University). Available at: https://www.canr.msu.edu/news/whycould-the-covid-19-cotton-crisis-lead-to-an-economic-and-social-crisis-in-maliIs.
Kooistra K. J., Termorshuizen A. J., Pyburn R. (2006). “The sustainability of cotton: Consequences for man and environment (Report No. 223),” in Science shop wageningen university and research centre. (Netherlands: Wageningen University & Research). Available at: https://edepot.wur.nl/17214.
Koraddi V. R., Channal S. K., Guggari A. K., Hamath K. S. (1991). Studies on planting pattern and fertilizer requirements for intercropping of cotton and groundnut under assured rainfall condition. Karnataka J. Agric. Sci. 3&4, 126–128.
Kothari K., Ale S., Bordovsky J. P., Munster C. L., Singh V. P., Nielsen-Gammon J., et al. (2021). Potential genotype-based climate change adaptation strategies for sustaining cotton production in the Texas High Plains: A simulation study. Field Crops Res. 271, 108261. doi: 10.1016/j.fcr.2021.108261
Kumar R., Turkhede A. B., Nagar R. K., Nath A. (2017). Effect of different intercrops on growth and yield attributes of American cotton under dryland condition. Int. J. Curr. Microbiol. Appl. Sci. 6 (4), 754–761. doi: 10.20546/ijcmas.2017.604.093
Lambert D. M., Paudel K. P., Larson J. A. (2015). Bundled adoption of precision agriculture technologies by cotton producers. J. Agric. Resource Economics 40 (2), 325–345.
Larson J. A., Velandia M. M., Buschermohle M. J., Westland S. M. (2016). Effect of field geometry on profitability of automatic section control for chemical application equipment. Precis. Agric. 17, 18–35. doi: 10.1007/s11119-015-9404-y
Lisa D., Minot N. (2014). Impact of global cotton markets on rural poverty in Benin. Agric. Economics 33 (3), 453–466. doi: 10.1111/j.1574-0864.2005.00415.x
Lu F., Bao-jie C., He-Zhong D. (2022). Cotton cultivation technology with Chinese characteristics has driven the 70-year development of cotton production in China. J. Integr. Agric. 21 (3), 597–609. doi: 10.1016/S2095-3119(20)63457-8
Lv Q., Chi B., He N., Zhang D., Dai J., Zhang Y., et al. (2023). Cotton-based rotation, intercropping, and alternate intercropping increase yields by improving root–shoot relations. Agronomy. 13 2, 413. doi: 10.3390/agronomy13020413
Lv N., Liu Y., Guo T., Liang P., Li R., Liang P., et al. (2022). The influence of Bt cotton cultivation on the structure and functions of the soil bacterial community by soil metagenomics. Ecotoxicology Environ. Saf. 236, 113452. doi: 10.1016/j.ecoenv.2022.113452
Lyu D., Backer R., Berrué F., Martinez-Farina C., Hui J. P. M., Smith D. L. (2023). Plant Growth-Promoting Rhizobacteria (PGPR) with microbial growth broth improve biomass and secondary metabolite accumulation of. Cannabis sativa L. J. Agric. Food Chem. 71 (19), 7268–7277. doi: 10.1021/acs.jafc.2c06961
Ma F., Wang Y., Yan P., Wei F., Duan Z., Yang Z., et al. (2021a). Effect of cotton residues incorporation on soil properties, organic nitrogen fractions, and nitrogen-mineralizing enzyme activity under long-term continuous cotton cropping. PeerJ. 9, e11053. doi: 10.7717/peerj.11053
Ma Z., Zhang Y., Wu L., Zhang G., Sun Z., Li Z., et al. (2021b). High-quality genome assembly and resequencing of modern cotton cultivars provide resources for crop improvement. Natural Genet. 53, 1385–1391. doi: 10.1038/s41588-021-00910-2
Majeed S., Rana I. A., Mubarik M. S., Atif R. M., Yang S., Chung G., et al. (2021). Heat stress in cotton: A review on predicted and unpredicted growth-yield anomalies and mitigating breeding strategies. Agronomy 11 (9), 1825. doi: 10.3390/agronomy11091825
Mandumbu R., Nyawenze C., Rugare J. T., Nyamadzawo G., Parwada C., Tibugari H. (2021). “Tied ridges and better cotton breeds for climate change adaptation,” in African handbook of climate change adaptation. Eds. Oguge N., Ayal D., Adeleke L., da Silva I. (Cham: Springer). doi: 10.1007/978-3-030-45106-6_23
Mansoori M., Heydari A., Hassanzadeh N., Rezaee S., Naraghi L. (2013). Evaluation of Pseudomonas and Bacillus antagonists for biological control of cotton Verticillium wilt disease. Plant Prot. Res. 53 (2), 154–157. doi: 10.2478/jppr-2013-0023
Marquardt S. (2011). “Organic cotton: production and marketing trends in the U.S and globally-2010,” in 2011 Beltwide cotton conferences. (US: The National Cotton Council of America).
Martínez-Carrillo J. L. (2005). “Evolución del algodón transgénico en México,” in VII congreso internacional de ciencia agrícolas (Mexicali: Universidad Autónoma de Baja California), 1–5.
Metwally A.E.-A.A., Shafik M. M., Sherief M. N., Abdel-Wahab T. I. (2012). Effect of intercropping corn on Egyptian cotton characters. J. Cotton Sci. 16, 210–219.
Meyer L. A. (2020). Cotton and wool outlook global cotton imports projected at 7-year high. (US: U.S. Department of Agriculture), 14–19.
Mi Q., Li X., Gao J. (2020). How to improve the welfare of smallholders through agricultural production outsourcing: Evidence from cotton farmers in Xinjiang, Northwest China. J. Cleaner Production 256, 120636. doi: 10.1016/j.jclepro.2020.120636
Miller G., Greene J. (2018). Intercropping seedless watermelon and cotton. HortSci. Horts. 53 (12), 1799–1803. doi: 10.21273/HORTSCI13428-18
Moher D., Liberati A., Tetzlaff J., Altman D. G. (2009). PRISMA Group. Preferred reporting items for systematic reviews and meta-analyses: the PRISMA statement. Ann. Internal Med. 151, 264–9, W64. doi: 10.7326/0003-4819-151-4-200908180-00135pmid:19622511
Mooney D. F., Roberts R. K., English B., Lambert D. M., Larson J., Velandia M., et al. (2010). “Precision farming by cotton producers in twelve southern states: results from the 2009 southern cotton precision farming survey,” in Research series 10-02, university of tennessee, knoxville, department of agricultural and resource economics. (Tennessee, US: The University of Tennessee Knoxville). Available at: http://ageconsearch.umn.edu/bitstream/91333/2/2009.
Mooshammer M., Stuart Grandy A., Calderón F., Culman S., Deen B., Drijber R. A., et al. (2022). Microbial feedbacks on soil organic matter dynamics underlying the legacy effect of diversified cropping systems. Soil Biol. Biochem. 167, 108584. doi: 10.1016/j.soilbio.2022.108584
Moulherat C., Tengberg M., Haquet J., Mille B. (2002). First evidence of cotton at Neolithic Mehrgarh, Pakistan: Analysis of mineralized fibres from a copper bead. J. Archaeological Sci. 29 (12), 1393–1401. doi: 10.1006/jasc.2001.0779
Najib D. C. S., Fei C., Dilanchiev A., Romaric S. (2022). Modeling the impact of cotton production on economic development in Benin: A Technological Innovation Perspective. Front. Environ. Sci. 10. doi: 10.3389/fenvs.2022.926350
Nalayini P., Sankaranarayanan K., Anandham R. (2010). Bio inoculants for enhancing the productivity and nutrient uptake of winter irrigated cotton (Gossypium hirsutum) under graded levels of nitrogen and phosphatic fertilizers. Indian J. Agron. 55 (1), 64–67.
Narula N., Saharan B., Kumar V., Bhatia R., Bishnoi L. K., Lather B., et al. (2005). Impact of the use of biofertilizers on cotton (Gossypium hirsutum) crop under irrigated agro-ecosystem. Arch. Agron. Soil Sci. 51 (1), 69–77. doi: 10.1080/03650340400029275
Nassary E. K., Msomba B. H., Masele W. E., Ndaki P. M., Kahangwa C. A. (2022). Exploring urban green packages as part of Nature-based Solutions for climate change adaptation measures in rapidly growing cities of the Global South. J. Environ. Manage. 310, 114786. doi: 10.1016/j.jenvman.2022.114786
Opee O. P. (2018). Selected factors influencing cotton production among smallholder farmers in Bura irrigation and settlement scheme, Kenya. Master’s Dissertation. (Kenya: Egerton University).
Page M. J., McKenzie J. E., Bossuyt P. M., Boutron I., Hoffmann T. C., Mulrow C. D., et al. (2020). The PRISMA 2020 statement: an updated guideline for reporting systematic reviews. BMJ 2021, 372, n71. doi: 10.1136/bmj.n71
Pandit M., Paudel K., Mishra A., Segarra E. (2011). “Precision farming technology adoption in cotton farming: duration analysis,” in Selected poster presented at the 2011 Annual Meeting of the Agricultural and Applied Economics Association, Pittsburgh, PA, July 24-26, Agricultural and Applied Economics Association. (US: LSU and LSU Agricultural Center Eduardo Segarra, Texas Tech University).
Pantzios C., Rozakis S., Tzouvelekas V. (2006). Evading farm support reduction via efficient input use: The Case of Greek Cotton Growers. J. Agric. Appl. Economics 38 (3), 555–574. doi: 10.1017/S1074070800022616
Parker N. W., Behringer E. C. (2009). Nitrous oxide: a global toxicological effect to consider. Anesthesiology 110, 1195. doi: 10.1097/ALN.0b013e31819faca9
Partzsch L., Kemper L. (2019). Cotton certification in Ethiopia: Can an increasing demand for certified textiles create a 'fashion revolution'? Geoforum 99, 111–119. doi: 10.1016/j.geoforum.2018.11.017
Partzsch L., Zander M., Robinson H. (2019). Cotton certification in Sub-Saharan Africa: Promotion of environmental sustainability or greenwashing? Global Environ. Change 57, 101924. doi: 10.1016/j.gloenvcha.2019.05.008
Parvez S., Gerona R. R., Proctor C., Friesen M., Ashby J. L., Reiter J. L., et al. (2018). Glyphosate exposure in pregnancy and shortened gestational length: a prospective Indiana birth cohort study. Environ. Health 17, 23. doi: 10.1186/s12940-018-0367-0
Paudel K. R., Mishra A. K., Pandit M., Segarra E. (2021). Event dependence and heterogeneity in the adoption of precision farming technologies: A case of US cotton production. Comput. Electron. Agric. 181, 105979. doi: 10.1016/j.compag.2020.105979
Pereg L., McMillan M. (2015). Scoping the potential uses of beneficial microorganisms for increasing productivity in cotton cropping systems. Soil Biol. Biochem. 80, 349–358. doi: 10.1016/j.soilbio.2014.10.020
Pettigrew W. T., Bruns H. A., Reddy K. N. (2016). Growth and agronomic performance of cotton when grown in rotation with soybean. J. Cotton Sci. 20, 299–308.
Pokhrel B., Paudel K., Segarra E. (2018). Factors affecting the choice, intensity, and allocation of irrigation technologies by U.S. cotton farmers. Water 10 (6), 706. doi: 10.3390/w10060706
Rana M. S., Hu C. X., Shaaban M., Imran M., Afzal J., Moussa M. G., et al. (2020). Soil phosphorus transformation characteristics in response to molybdenum supply in leguminous crops. J. Environ. Manage. 268, 110610. doi: 10.1016/j.jenvman.2020.110610
Raper T. B., McClure A. T., Yin F., Brown B. (2014). Sulfur and Tennessee row crops Vol. W435 (Tennessee, US: The University of Tennessee Knoxville).
Raphael J. P. A., Gazola B., Nunes J. G. S., Macedo G. C., Rosolem C. A. (2017). Cotton germination and emergence under high diurnal temperatures. Crop Sci. 57 (5), 2761–2769. doi: 10.2135/cropsci2017.03.0182
Reddy P. R. R., Shaik M. (2009). Evaluation of cotton (Gossypium hirsutum)-based intercropping system through different approaches under rainfed conditions. Indian J. Agric. Sci. 79 (3), 210–214.
Riar A., Mandloi L. S., Poswal R. S., Messmer M. M., Bhullar G. S. (2017). A diagnosis of biophysical and socio-economic factors influencing farmers’ choice to adopt organic or conventional farming systems for cotton production. Front. Plant Sci. 8. doi: 10.3389/fpls.2017.01289
Richards M., Metzel R., Chirinda N., Ly P., Nyamadzawo G., Vu Q. D., et al. (2016). Limits of agricultural greenhouse gas calculators to predict soil N2O and CH4 fluxes in tropical agriculture. Sci. Rep. 6, 26279. doi: 10.1038/srep26279
Riello G. (2022). Cotton textiles and the industrial revolution in a global context. Past Present 255 (1), 87–139. doi: 10.1093/pastj/gtab016
Roberts R. K., English B. C., Larson J. A., Cochran R. L., Goodman W. R., Larkin M. C. S. L., et al. (2004). Adoption of site-specific information and variable-rate technologies in cotton precision farming. J. Agric. Appl. Economics 36 (1), 143–158. doi: 10.1017/S107407080002191X
Rocha-Munive M. G., Soberón M., Castañeda S., Niaves E., Scheinvar E., Eguiarte L. E., et al. (2018). Evaluation of the impact of genetically modified cotton after 20 years of cultivation in Mexico. Front. Bioengineering Biotechnol. 6. doi: 10.3389/fbioe.2018.00082
Sabesh M., Prakash A. H. (2018). Higher cotton productivity in Africa a socio economic analysis. ICAC Rec. 36 (4), 1–34.
Saghafi D., Asgari Lajayer B., Ghorbanpour M. (2020). “Engineering bacterial ACC deaminase for improving plant productivity under stressful conditions,” in Molecular aspects of plant beneficial microbes in agriculture. Eds. Sharma V., Salwan R., Tawfeeq Al-ani L. K. (Amsterdam: Elsevier), 259–277. doi: 10.1016/B978-0-12-818469-1.00022-5
Salama H. S. A., Nawar A. I., Khalil H. E. (2022). Intercropping pattern and N fertilizer schedule affect the performance of additively intercropped maize and forage cowpea in the Mediterranean Region. Agronomy 12, 107. doi: 10.3390/agronomy12010107
Salihu A., Singh H. P. (2020). Cotton production and socio-economic characteristics of farmers in Maharashtra, India. J. Environ. Issues Agric. Developing Countries 12 (1), 21–29. doi: 10.20546/ijcmas.2021.1001.100
Sankaranarayanan K., Nalayini P., Praharaj C. S. (2012). Multi-tier cropping system to enhance resource utilization, profitability and sustainability of Bt cotton (Gossypium hirsutum) production system. Indian J. Agric. Sci. 82 (12), 1044–1050.
Savoy H. J., Joines D. (2009). “Lime and fertilizer recommendations for the various crops of Tennessee,” in Chapter II. Agronomic crops. (John Wiley & Sons, Inc.). Available at: http://soilplantandpest.utk.edu/pdffiles/soiltestandfertrecom/chap2-agronomic_mar2009.pdf.
Sehgal A., Singh G., Quintana N., Kaur G., Ebelhar W., Nelson K. A., et al. (2023). Long-term crop rotation affects crop yield and economic returns in humid subtropical climate. Field Crops Res. 298, 108952. doi: 10.1016/j.fcr.2023.108952
Shahrajabian M. H., Sun W., Cheng Q. (2020). Considering white gold, cotton, for its fiber, seed oil, traditional and modern health benefits. J. Biol. Environ. Sci. 14 (40), 25–39.
Sharath S., Triveni S., Nagaraju Y., Latha P. C., Vidyasagar B. (2021). The role of phyllosphere bacteria in improving cotton growth and yield under drought conditions. Front. Agron. 3. doi: 10.3389/fagro.2021.680466
Sharma A., Deepa R., Sankar S., Pryor M., Stewart B., Johnson E., et al. (2021). Use of growing degree indicator for developing adaptive responses: A case study of cotton in Florida. Ecol. Indic. 124, 107383. doi: 10.1016/j.ecolind.2021.107383
Shepherd H. (2020). Thirst for fashion? How organic cotton delivers in a water-stressed world. UK: Soil Association. Available at: http://library.unccd.int/Details/books/1352.
Shuli F., Jarwar A. H., Wang X., Wang L., Ma Q. (2018). Overview of the cotton in Pakistan and its future prospects. Pakistan J. Agric. Res. 31 (4), 396–407. doi: 10.17582/journal.pjar/2018/31.4.396.407
Singh R. J., Ahlawat I. P. S. (2011). Productivity, competition indices and soil fertility changes of Bt cotton (Gossypium hirsutum L.) – groundnut (Arachis hypogaea L.) intercropping system using different fertility levels. Indian J. Agric. Sci. 81 (7), 606–611.
Singh S., Dusanj-Lenz S. (2019). “Gender equity and its impact on sustainability in cotton farming in India,” in Fashion revolution India. (India: Fashion Revolution India). Available at: https://issuu.com/fashionrevolution/docs/gender_equity_cotton_farming_policy_pilot_fr_bc.
Snider J. L., Pilon C., Hu W., Wang H., Tishchenko V., Slaton W., et al. (2022). Net photosynthesis acclimates to low growth temperature in cotton seedlings by shifting temperature thresholds for photosynthetic component processes and respiration. Environ. Exp. Bot. 196, 104816. doi: 10.1016/j.envexpbot.2022.104816
Sodjinou E., Glin L. C., Nicolay G., Tovignan S., Hinvi J. (2015). Socioeconomic determinants of organic cotton adoption in Benin, West Africa. Agric. Food Economics 3 (1), 1–22. doi: 10.1186/s40100-015-0030-9
Sub-Committee on Cotton (2021). “Impacts of the COVID-19 pandemic on cotton and its value chains: The case of the C-4 and other LDCs (Report No.: TN/AG/SCC/W/40-WT/CFMC/W/97),” in Agriculture and commodities division (TN/AG/SCC/W/40 - WT/CFMC/W/97 21-8525: World Trade Organization). Available at: https://www.wto.org/english/tratop_e/agric_e/covidinpactstudyc4ldcscotton_e.pdf.
Suomela J. A., Viljanen M., Svedström K., Wright K., Lipkin S. (2023). Research methods for heritage cotton fibres: Case studies from archaeological and historical finds in a Finnish context. Heritage Sci. 11 (1), 1–18. doi: 10.1186/s40494-023-01022-2
Surendran U., Subramoniam S. R., Raja P., Kumar V., Murugappan V. (2016). Budgeting of major nutrients and the mitigation options for nutrient mining in semi-arid tropical agro-ecosystem of Tamil Nadu, India using NUT-MON model. Environ. Monit. Assess. 188, 250. doi: 10.1007/s10661-016-5202-x
Syakila A., Kroeze C. (2011). The global nitrogen budget revisited. Greenhouse Gas Measurement Manage. 1, 17–26. doi: 10.3763/ghgmm.2010.0007
Tak V., Pandey V., Parmar P. K. (2021). Effect of date of sowing and varieties on yield of Kharif Bt-cotton in Middle Gujarat Agro-Climatic Region. Int. J. Curr. Microbiol. Appl. Sciences 10 01, 2217–2222. doi: 10.20546/ijcmas.2021.1001.254
Tarazi R., Jimenez J. L. S., Vaslin M. F. S. (2019). Biotechnological solutions for major cotton (Gossypium hirsutum) pathogens and pests. Biotechnol. Res. Innovation 3, 19–26. doi: 10.1016/j.biori.2020.01.001
Tariq M., Afzal M. N., Muhammad D., Ahmad S., Shahzad A. N., Kiran A., et al. (2018). Relationship of tissue potassium content with yield and fiber quality components of Bt cotton as influenced by potassium application methods. Field Crops Res. 229, 37–43. doi: 10.1016/j.fcr.2018.09.012
Terán-Vargas A. P., Rodríguez J. C., Blanco C. A., Martínez-Carrillo J. L., Cibrián-Tovar J., Sánchez-Arroyo H., et al. (2005). Bollgard cotton and resistance of tobacco budworm (Lepidoptera: Noctuidae) to conventional insecticides in southern Tamaulipas. Mexico. J. Economic Entomology 98, 2203–2209. doi: 10.1093/jee/98.6.2203
Textile Exchange (2018) Organic cotton market report 2018. Available at: https://textileexchange.org/wp-content/uploads/2018/11/2018-Organic-Cotton-Market-Report.pdf.
Tian H., Yang J., Xu R., Lu C., Canadell J. G., Davidson E. A., et al. (2019). Global soil nitrous oxide emissions since the preindustrial era estimated by an ensemble of terrestrial biosphere models: Magnitude., attribution., and uncertainty. Global Change Biol. 25 (2), 640–659. doi: 10.1111/gcb.14514
Tittonell P. (2010). “Sustaining soil productivity of cotton-based cropping systems in the savannahs of West and Central Africa: challenges and opportunities,” in Proceedings of the 30th International Cotton Conference, Bremen, March 2010, 140–145 (Brême: Faserinstitut Bremen), International Cotton Conference. 30, 2010-03-24/2010-03-27, Brême (Allemagne).
Trapero C., Wilson I. W., Stiller W. N., Wilson L. J. (2016). Enhancing integrated pest management in GM cotton systems using host plant resistance. Front. Plant Sci. 7. doi: 10.3389/fpls.2016.00500
Tuomisto H. L., Hodge I. D., Riordan P., Macdonald D. W. (2012). Does organic farming reduce environmental impacts? – a meta-analysis of European research. J. Environ. Manage. 112, 309–320. doi: 10.1016/j.jenvman.2012.08.018
Tzouvelekas V., Pantzios C., Fotopoulos C. (2001). Economic efficiency in organic farming: evidence from cotton farms in Viotia, Greece. J. Agric. Appl. Economics 33 (1), 35–48. doi: 10.1017/S1074070800020769
United States Department of Labour (2011) List of goods produced by child labour or forced labour. Available at: http://www.dol.gov/ilab/programs/ocft/PDF/2011TVPRA.pdf.
Vaghasia P. M., Dobariya K. L. (2019). Nutrient management in groundnut (Arachis Hypogaea L.) - Bt cotton (Gossypium Hirsutum L.) intercropping system. Legume Res. 44 (3), 334–338. doi: 10.18805/LR-4108
Verma M., Mishra J., Arora N. K. (2019). “Plant growth-promoting rhizobacteria: diversity and applications,” in Environmental biotechnology: for sustainable future. Eds. Sobti R., Arora N., Kothari R. (Singapore: Springer). doi: 10.1007/978-981-10-7284-0_6
Viot C. (2019). Domestication and varietal diversification of Old World cultivated cottons (Gossypium sp.) in the Antiquity. Rev. d’ethnoeícologie 15, 2019. doi: 10.4000/ethnoecologie.4404
Virk G., Snider J. L., Chee P., Jespersen D., Pilon C., Rains G., et al. (2021). Extreme temperatures affect seedling growth and photosynthetic performance of advanced cotton genotypes. Ind. Crops Products 172, 114025. doi: 10.1016/j.indcrop.2021.114025
Vocciante M., Grifoni M., Fusini D., Petruzzelli G., Franchi E. (2022). The role of Plant Growth-Promoting Rhizobacteria (PGPR) in mitigating plant’s environmental stresses. Appl. Sci. 12 (3), 1231. doi: 10.3390/app12031231
Voora V., Bermudez S., Farrell J. J., Larrea C., Luna E. (2023). “Global market report. Cotton prices and sustainability,” in Sustainable commodities marketplace series. (Winnipeg, Manitoba, Canada: The International Institute for International Development and International Trade Centre). Available at: https://www.iisd.org/system/files/2023-01/2023-global-market-report-cotton.pdf.
Voora V., Larrea C., Bermudez S. (2020). “Global market report: Cotton,” in International institute for sustainable development. (Winnipeg, Manitoba, Canada: The International Institute for International Development and International Trade Centre). Available at: https://www.iisd.org/sites/default/files/publications/ssiglobal-market-report-cotton.pdf.
Voora V., Larrea C., Huppé G., Nugnes F. (2022). “Standards and investments in sustainable agriculture,” in International institute for sustainable development. Available at: https://www.iisd.org/system/files/2022-04/ssi-initiatives-review-standards-investments-agriculture.pdf.
Wakhungu J., Wafula D. (2013). Introducing BT cotton: policy lessons for smallholder farmers in Kenya (Nairobi: Kenya).
Wang Y., Liu Z., Liang H., Wang X., Wang Z., Ma C., et al. (2022). Chelated copper reduces yet manganese fertilizer increases calcium-silica fouling in brackish water drip irrigation systems. Agric. Water Manage. 269, 107655. doi: 10.1016/j.agwat.2022.107655
Wang W., Qiu Z., Tan H., Cao L. (2014). Siderophore production by actinobacteria. Biometals 27, 623–631. doi: 10.1007/s10534-014-9739-2
Wang W., Zhang C., Song J., Xu D. (2021). The impact of target price policy on cotton cultivation: analysis of county-level panel data from China. Agriculture 11 (10), 988. doi: 10.3390/agriculture11100988
Wang Y., Zhu Y., Zhang S., Wang Y. (2018). What could promote farmers to replace chemical fertilizers with organic fertilizers? J. Cleaner Production 199, 882–890. doi: 10.1016/j.jclepro.2018.07.222
Watto M. A., Mugera A. W. (2015). Econometric estimation of groundwater irrigation efficiency of cotton cultivation farms in Pakistan. J. Hydrology: Regional Stud. 4, 193–211. doi: 10.1016/j.ejrh.2014.11.001
Wei F., Feng H., Zhang D., Feng Z., Zhao L., Zhang Y., et al. (2021). Composition of rhizosphere microbial communities associated with healthy and Verticillium wilt diseased cotton plants. Front. Microbiol. 12. doi: 10.3389/fmicb.2021.618169
Wei F., Zhao L., Xu X., Feng H., Shi Y., Deakin G., et al. (2019). Cultivar dependent variation of the cotton rhizosphere and endosphere microbiome under field conditions. Front. Plant Sci. 10. doi: 10.3389/fpls.2019.01659
Wendel J. F., Cronn R. C. (2003). Polyploidy and the evolutionary history of cotton. Adv. Agron. 78, 139–186.
Wendel J. F., Grover C. E. (2015). “Taxonomy and evolution of the cotton genus, Gossypium,” in Cotton. Eds. Fang D. D., Percy R. G. (Madison: American Society of Agronomy Inc.), 25–44. doi: 10.2134/agronmonogr57.2013.0020
WHO (2005) The WHO recommended classification of pesticides by hazard and guidelines to classification 2004. Available at: http://www.who.int/ipcs/publications/pesticides-hazard/en.
World Bank Group (2022) Is a global recession imminent?Available at: https://openknowledge.worldbank.org/bitstream/handle/10986/38019/Global-Recession.pdf.
World Vision Australia (2012) Forced and child labour in the cotton industry. Available at: https://www.worldvision.com.au/docs/default-source/buy-ethical-fact-sheets/forced-and-child-labour-in-the-cotton-industry-fact-sheet.pdf?sfvrsn=2#:~:text=Children%20work%20in%20ginning%20factories,they%20inhale%2C%20causing%20respiratory%20problems37.&text=Children%20spread%20cotton%20on%20platforms,factory%20and%20remove%20cotton%20seed38.
Wright B. (2022) Video interview: Is West Africa the next frontier for apparel sourcing? Just Style. Available at: https://www.just-style.com/interviews/video-interview-is-west-africa-the-next-frontier-forapparel-sourcing/.
Xi H., Shen J., Qu Z., Yang D., Liu S., Nie X., et al. (2019). Effects of long-term cotton continuous cropping on soil microbiome. Sci. Rep. 9, 18297. doi: 10.1038/s41598-019-54771-1
Xi H., Zhang X., Qu Z., Yang D., Alariqi M., Yang Z., et al. (2021). Effects of cotton-maize rotation on soil microbiome structure. Mol. Plant Pathol. 22 (6), 673–682. doi: 10.1111/mpp.13053
Xu R., Tian H., Pan S., Prior S. A., Feng Y., Dangal S. R. (2020). Global N2O emissions from cropland driven by nitrogen addition and environmental factors: Comparison and Uncertainty Analysis. Global Biogeochemical Cycles 34 (12), e2020GB006698. doi: 10.1029/2020GB006698
Yang C., Yue H., Ma Z., Feng Z., Feng H., Zhao L., et al. (2022). Influence of plant genotype and soil on the cotton rhizosphere microbiome. Front. Microbiol. 13. doi: 10.3389/fmicb.2022.1021064
Yasmin S., Hafeez F., Schmid M., Hartmann A. (2013). Plant-beneficial rhizobacteria for sustainable increased yield of cotton with reduced level of chemical fertilizer. Pakistan J. Bot. 45 (2), 655–662.
Yin X. (2016). Small scale spatio-temporal variabilities in soil nitrogen, leaf nitrogen, and canopy normalized difference vegetation index of cotton. J. Geosci. Environ. Prot. 4 (12), 56–74. doi: 10.4236/gep.2016.412005
Yu K., Yu S., Fan S., Song M., Zhai H., Li X., et al. (2012). Mapping quantitative trait loci for cotton seed oil, protein and gossypolcontentina Gossypium hirsutum × Gossypium barbadense backcross inbred line population. Euphytica 187, 191–201.
Zahid K. R., Ali F., Shah F., Younas M., Shah T., Shahwar D., et al. (2016). Response and tolerance mechanism of cotton Gossypium hirsutum L. to elevated temperature stress: A review. Front. Plant Sci. 7, 937. doi: 10.3389/fpls.2016.00937
Zhou X. V., English B. C., Larson J. A., Lambert D. M., Roberts R. K., Boyer C. N., et al. (2017). Economics and marketing: precision farming adoption trends in the southern U.S. J. Cotton Sci. 21, 143–155.
Keywords: cotton industry, livelihoods, global, small landholdings, sustainable systems
Citation: Tlatlaa JS, Tryphone GM and Nassary EK (2023) Unexplored agronomic, socioeconomic and policy domains for sustainable cotton production on small landholdings: a systematic review. Front. Agron. 5:1281043. doi: 10.3389/fagro.2023.1281043
Received: 23 August 2023; Accepted: 30 October 2023;
Published: 20 November 2023.
Edited by:
Min Huang, Hunan Agricultural University, ChinaReviewed by:
Imran Arshad, Saa Technical And Specialized Services Establishment, United Arab EmiratesMuhammad Umer Arshad, Inner Mongolia Hongde College of Arts and Sciences, China
Copyright © 2023 Tlatlaa, Tryphone and Nassary. This is an open-access article distributed under the terms of the Creative Commons Attribution License (CC BY). The use, distribution or reproduction in other forums is permitted, provided the original author(s) and the copyright owner(s) are credited and that the original publication in this journal is cited, in accordance with accepted academic practice. No use, distribution or reproduction is permitted which does not comply with these terms.
*Correspondence: Eliakira Kisetu Nassary, a2VsaWFraXJhQHlhaG9vLmNvbQ==