- 1Plant Protection Institute, Hebei Academy of Agriculture and Forestry Sciences, Baoding, Hebei, China
- 2College of Horticulture, Hebei Agricultural University, Baoding, Hebei, China
- 3Hebei Agricultural Characteristic Industry Technical Guidance Station, Shijiazhuang, Hebei, China
- 4Shunping Meteorological Bureau, Baoding, Hebei, China
- 5School of Agriculture and Environment, Massey University, Palmerston North, New Zealand
The successful management of lepidopteran moths in orchards usually depends on the precise forecast of adult activity. However, the seasonal phenology of moths varies between crop cultivars and years, making it difficult to schedule the control measures. Here, we monitored male flight activity of oriental fruit moth Grapholita molesta and summer fruit tortrix moth Adoxophyes orana by using sex pheromone traps in peach orchards of three different cultivars for three successive years. We developed a logistic multiple-peaks model to fit data and then calculated degree-days (DD) required for male activity and neonate emergency. Results show that G. molesta and A. orana males had 4–5 and 3 flight peaks per year, respectively. The seasonal phenology of G. molesta or A. orana was quite stable with an identical timing of each flight peak between cultivars in a year. The flight activity was usually higher in the second and third peaks for both moths, with a higher cumulative number of G. molesta males captured than that of A. orana. Compared to A. orana, G. molesta emerged early in spring and required lower degree-days to reach the subsequent flight peaks and for neonate emergency. Our results suggest that to decline the possibility of outbreaks of moths during the growing seasons, pheromone traps should be scheduled in April with a cumulative DD between 49.6 and 207.1 for G. molesta and in mid-May–early June with a cumulative DD between 450.4 and 866.7 for A. orana, aiming to trap the newly emerged male adults or disrupting female mating success of overwintered moths in orchards. Based on the thermal requirement for egg hatching (i.e., 79.4 DD for G. molesta and 90.0 DD for A. orana), insecticide treatments would be applied in late-April–early May and late May–early June to reduce the field population density of neonates of G. molesta and A. orana, respectively, to reduce fruit damage in orchards. Furthermore, pheromone traps set up in late July–early August (573.8–1025.2 DD) for G. molesta and in mid-September (1539.7–1788.9 DD) for A. orana may suppress overwintering populations and thus decrease pest infestation in next year.
Introduction
The oriental fruit moth Grapholita molesta (Busck) and summer fruit tortrix moth Adoxophyes orana (Fischer von Röslerstamm) (Lepidoptera: Tortricidae) are two of the most important lepidopteran pests that continually cause significant damage to commercial pome and stone fruits, including peach, apple, pear, nectarines, cherries, quince, and persimmons (Rothschild and Vickers, 1991; van der Geest and Evenhuis, 1991; Kocourek and Stara, 2005; Myers et al., 2007; Piñero and Dorn, 2009; Kirk et al., 2013). Grapholita molesta is assumed to be native to Asia (Rothschild and Vickers, 1991; Kirk et al., 2013) and A. orana is originally reported from Europe (Byun et al., 2012); they are now distributed throughout temperate regions of Asia, Europe, Americas, Africa, and Australia (Rothschild and Vickers, 1991; Li et al., 2021).
Grapholita molesta overwinters as mature fifth instar larvae inside the cocoons (Rothschild and Vickers, 1991; Notter-Hausmann and Dorn, 2010). The overwintered larvae pupate in the spring, and adults emerge and oviposit on host plants or near the overwintering habitats (Sarai, 1970; Ellis and Hull, 2013). Upon hatching, G. molesta neonates excavate tunnels and feed inside the tender twigs in spring (Neven et al., 2018; Liu et al., 2022), and the developing larvae are internal feeders in fruits in the mid- and late-growing seasons (Rothschild and Vickers, 1991; Neven et al., 2018). Adoxophyes orana also has five larval instars but enter overwinter at the third instar (Oku, 1966), and the overwintered larvae develop again in spring and feed on host plant flower buds and clusters, and young shoots and fruits (Oku, 1966; Damos et al., 2022). Apart from attacking the fruit, A. orana is a leafroller as the young shoots and leaves fed by the larvae may stick together (Pehlevan and Kovanci, 2014; Damos et al., 2022). Grapholita molesta larval feeding may cause up to 50–60% fruit losses in Texas State, USA (Anon, 2014) or even 90% in Santa Catarina state, Brazil (Reis Fo et al., 1988). Direct damage on fruits by A. orana larvae may result in yield losses varying from 10% to 80% in apple, peach, and pear (de Jong et al., 1971; Whittle, 1985; Stamenkovic and Pesic, 1998; CAB International, 2008). Moreover, fruits damaged by G. molesta and A. orana larvae may attract secondary pests, such as the nitidulid beetles Carpophilus spp., which act as vectors of brown rot (Monilinia spp.) fungal infection (Hossain et al., 2006). The damaged or infected fruits could not access the markets.
So far, the management of field populations of G. molesta and A. orana is mainly relied on the applications of insecticides and sex pheromone traps (mating disruption) (Trimble et al., 2001; Pastori et al., 2012) basing on a year-by-year calendar schedule programme and/or the phenological stage of the moths (Damos and Savopoulou-Soultani, 2010; Damos and Karabatakis, 2012; Damos and Savopoulou-Soultani, 2012a). Unfortunately, the efficacy of insecticides is usually lower than expected due to the feeding habits protecting larvae from insecticides (Rothschild and Vickers, 1991; Pehlevan and Kovanci, 2014; Neven et al., 2018; Liu et al., 2022; Damos et al., 2022), and the rapid development of insecticide resistance (Pree et al., 1998; de Lame et al., 2001; Kanga et al., 2003; Navarro-Roldán et al., 2017). Furthermore, mating disruption using sex pheromone may have a low control efficacy when the field populations are large (Kong et al., 2020; Ferracini et al., 2021; Liu et al., 2022), as males can easily locate their mates using visual cues as well as by chance encounters (Thorpe et al., 2006). For G. molesta and A. orana, the eggs/neonates and male adults of moths are the targets of pest control using insecticide and sex pheromone measures, respectively (Philips, 1973; Wang et al., 2017); therefore, the effective management of these species largely depends on precise prediction of timings of egg laying and hatching and flight peaks of male adults, especially in the overwintered generation.
Insects are poikilothermic, and temperature is the major factor regulating their development, growth and thus population dynamics. Degree-days (DD) are the most convenient way to predict a phenological event in insects (Prues, 1983; Damos and Savopoulou-Soultani, 2012b). Previous studies have acknowledged that degree-days models could be a useful tool in forecasting the phenology of many lepidopterous pests (e.g., Hrdý et al., 1996; Godin and Boivin, 1998; Del Tío et al., 2001; Milonas et al., 2001; Tobin et al., 2003; Pehlevan and Kovanci, 2017; Ivezić et al., 2023), including G. molesta and A. orana (Damos and Savopoulou-Soultani, 2010; Jones et al., 2013; Damos et al., 2022).
In this study, we monitored the seasonal flight dynamics of G. molesta and A. orana males using pheromone traps in peach orchards of different cultivars over three successive years. We then calculate the cumulative degree-days basing on the low temperature thresholds of the two pests to predict the thermal requirement for the first male captured in early spring, and male flight peaks and egg hatching during the growing seasons. Our results may deliver critical knowledge for the development of pest management strategies aiming to supress the populations of G. molesta and A. orana in field.
Materials and methods
Study site and local climate conditions
The field experiment was conducted in peach growing region in Shunping County, Hebei Province, China (38.84°N, 115.13°E). The mean annual temperature during the growing seasons (March–October) is 19.1°C (19.2, 19.4 and 18.8°C for 2018, 2019 and 2020, respectively), and the mean annual precipitation is 577.1 mm (623.6, 558.2 and 458.9 for 2018, 2019 and 2020, respectively), occurring mainly in June and July (> 74.4%) (Figure 1). Pest control of the target pest moths by using pesticides or natural enemies was not performed in orchards during the experiment.
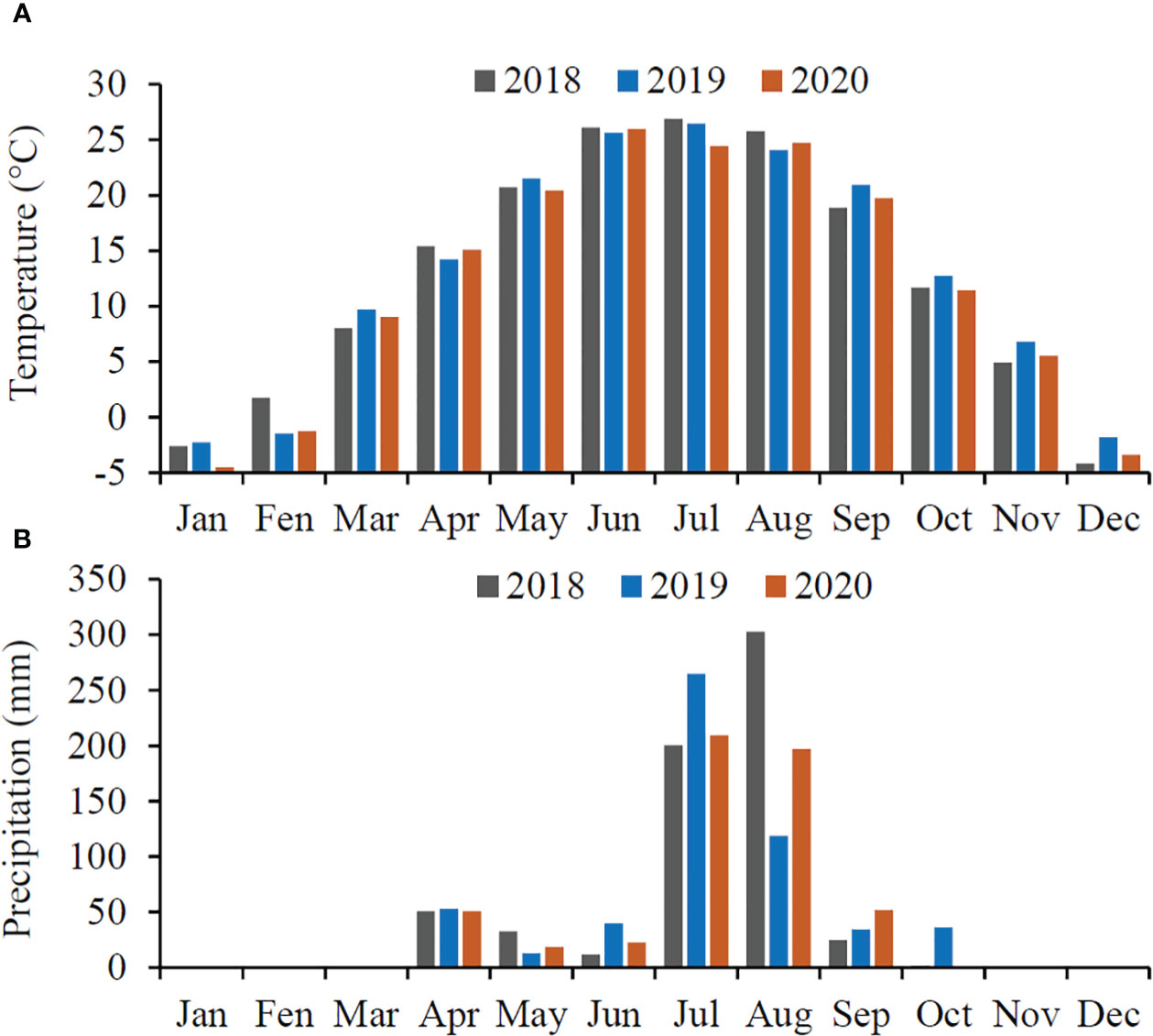
Figure 1 Mean temperature (A) and total precipitation (B) per month in three consecutive years in Shunping County where the experiments were carried out.
Field trials
The population dynamics of G. molesta and A. orana were monitored in peach orchards of three cultivars (i.e., Jinghong, Jiubao and Luhua9) for three consecutive years (i.e., 2018, 2019 and 2020). For each cultivar, we selected three orchards (replicates, each > 0.33 hectare). In each orchard, we set up two Delta traps (23.5 cm length × 20.5 cm width × 12.5 cm heigh, one trap for G. molesta and one trap for A. orana) baited with one species-specific commercial pheromone lure (Pherobio Technology Co., Ltd., Beijing, China) hung 1.0–1.5 cm above the white sticky board (20 cm length × 19 cm width), in mid-March each year. The two traps were hung in two trees approximately 1.5 m from the ground, 40 m apart, and > 20 m from borders of the orchard.
The sticky boards were replaced and the number of male adults of G. molesta and A. orana captured was counted every 7 days. The pheromone lures were replaced monthly, and observation was terminated in late October.
Statistical analysis
We performed analyses using SAS v. 9.4 software (SAS Institute Inc., NC, USA). Data were normally distributed (Shapiro-Wilk test, UNIVARIATE Procedure). Basing on a non-linear Gaussian functional model of Archontoulis and Miguez (2015), we developed a seasonal phenology model with multiple peaks to fit the seasonal activity of adult males of both species (NLIN Procedure) in orchards of each cultivar or overall cultivars in different years: no. moths = , where n is the number of peaks, t is the time (days) since the early male adult captured on 02 April 2020 during the experiment, is the i peak at time , is the coefficients controlling the width of i peak, and 0.5 is defaulted for the Gaussian function. The difference in each estimated parameter was compared between different cultivars or years: there is no significant difference if the 95% confidence limits (CLs) overlap (Julious, 2004). The mean total number of male adults of a give species between the combination treatments of cultivars and years was analyzed using ANOVA (GLM Procedure) with LSD for multiple comparisons. For a given cultivar and year, the mean total number of male adults of G. molesta and A. orana was also compared using ANOVA.
Based on the low temperature threshold, i.e., = 9.5 and 7.2°C for G. molesta and A. orana, respectively (Damos et al., 2022), we calculated the cumulative degree-days (DD) for the first male adult moth captured by the pheromone traps in orchards and for the seasonal flight peaks of moths, using a degree-day model: , where Ti is the mean daily air temperature at date i, and n is the number of days since on 13 March, 05 March and 15 March in 2018, 2019 and 2020, respectively. We also estimated the hatching date of eggs (presence of larvae or start of larva feeding) for the first captured male adults and that at flight peaks basing on the degree-days for egg hatching, i.e., 79.4 DD for G. molesta (Croft et al., 1980) and 90.0 DD for A. orana (Charmillot and Megevand, 1983). The timings of first male captured and subsequent flight peaks of moths estimated from the overall model of three cultivars were used for degree-days calculation.
Results
Seasonal phenology
The regression coefficient (i.e., R2) of the models ranged from 0.9114 to 0.9853 for G. molest and from 0.8957 to 0.9975 for A. orana (Figures 2, 3), indicating the good fitness of the models to fit the data of number of moths captured by the pheromone traps in peach orchards of different cultivars in different years. As shown in Figure 2, G. molesta generally had four male flight peaks per year in peach orchards of three different cultivars during the three successive years, with a small additional peak detected for Jinghong in 2019 and for all cultivars in 2020. In 2018, the peak was significantly higher in the 2nd and 3rd active peaks than in the 1st and 4th ones for each cultivar or overall cultivars (non-overlapped 95% CLs) (Figure 2A; Table 1); however, in 2019 the active peak was significantly higher in the 4th and 5th peaks for the Jinghong, in the 4th peak for Jiubao and in the 3rd peak for Luhua9 (non-overlapped 95% CLs) (Figure 2B; Table 1), and in 2020 it was significantly higher in the 2nd and 3rd peaks for Jinghong and Luhua9 and in 3rd peak for Jiubao (non-overlapped 95% CLs) (Figure 2C; Table 1). The timing of flight peak of G. molesta male adults was significantly different between peaks for a given cultivar in each year (non-overlapped 95% CLs); however, for a given flight peak, the timing was not significantly different between cultivars in each year (overlapped 95% CLs) (Figure 2; Table 1).
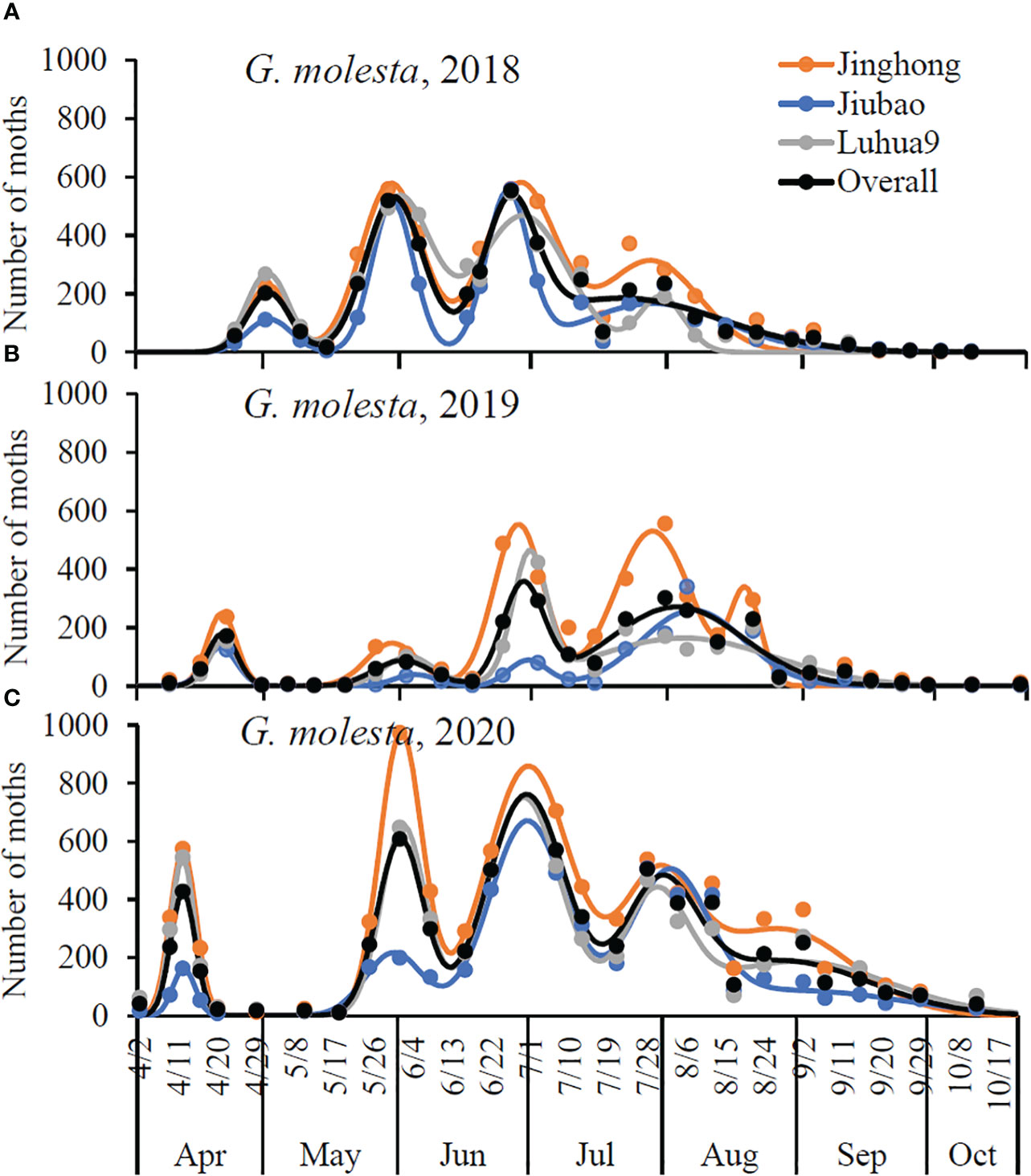
Figure 2 Seasonal activity of Grapholita molesta male adults in peach orchards of different cultivars in three consecutive years (see Table 1 for details): (A) 2018, (B) 2019, and (C) 2020. R2 = 0.9114–0.9853 for models of different cultivars in different years, and R2 = 0.8509–0.9288 for models of overall cultivars in different years; P< 0.0001. The solid circles are the number of male adults captured by the pheromone traps, and the smooth lines are the number of captured male adults predicted by the multiple-peaks models.
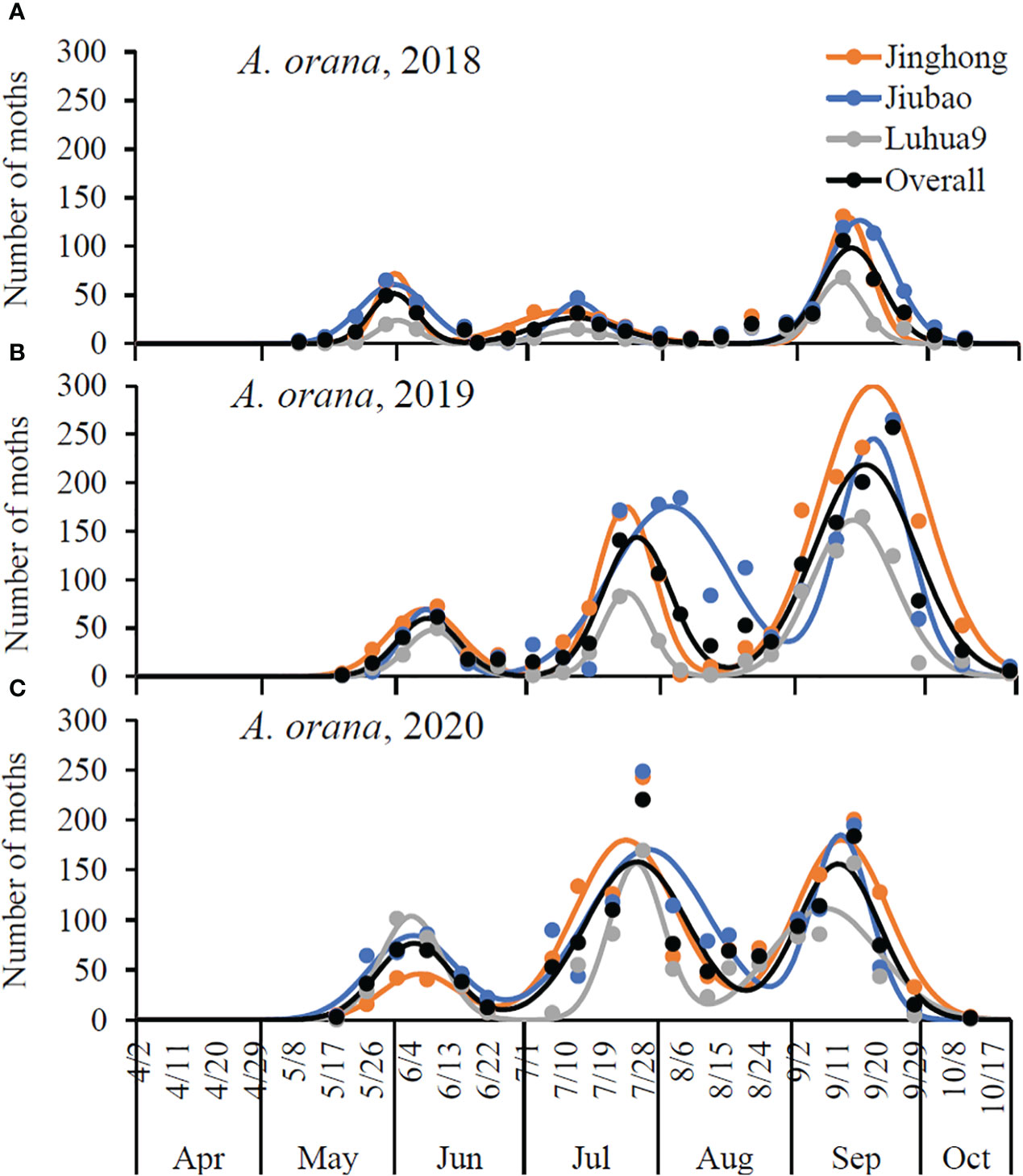
Figure 3 Seasonal activity of Adoxophyes orana male adults in peach orchards of different cultivars in three consecutive years (see Table 2 for details): (A) 2018, (B) 2019, and (C) 2020. R2 = 0.8957–0.9975 for models of different cultivars and different years, and R2 = 0.8014–0.8086 for models of overall cultivars in different years; P< 0.0001. The solid circles are the number of male adults captured by the pheromone traps, and the smooth lines are the number of captured male adults predicted by the multiple-peaks models.
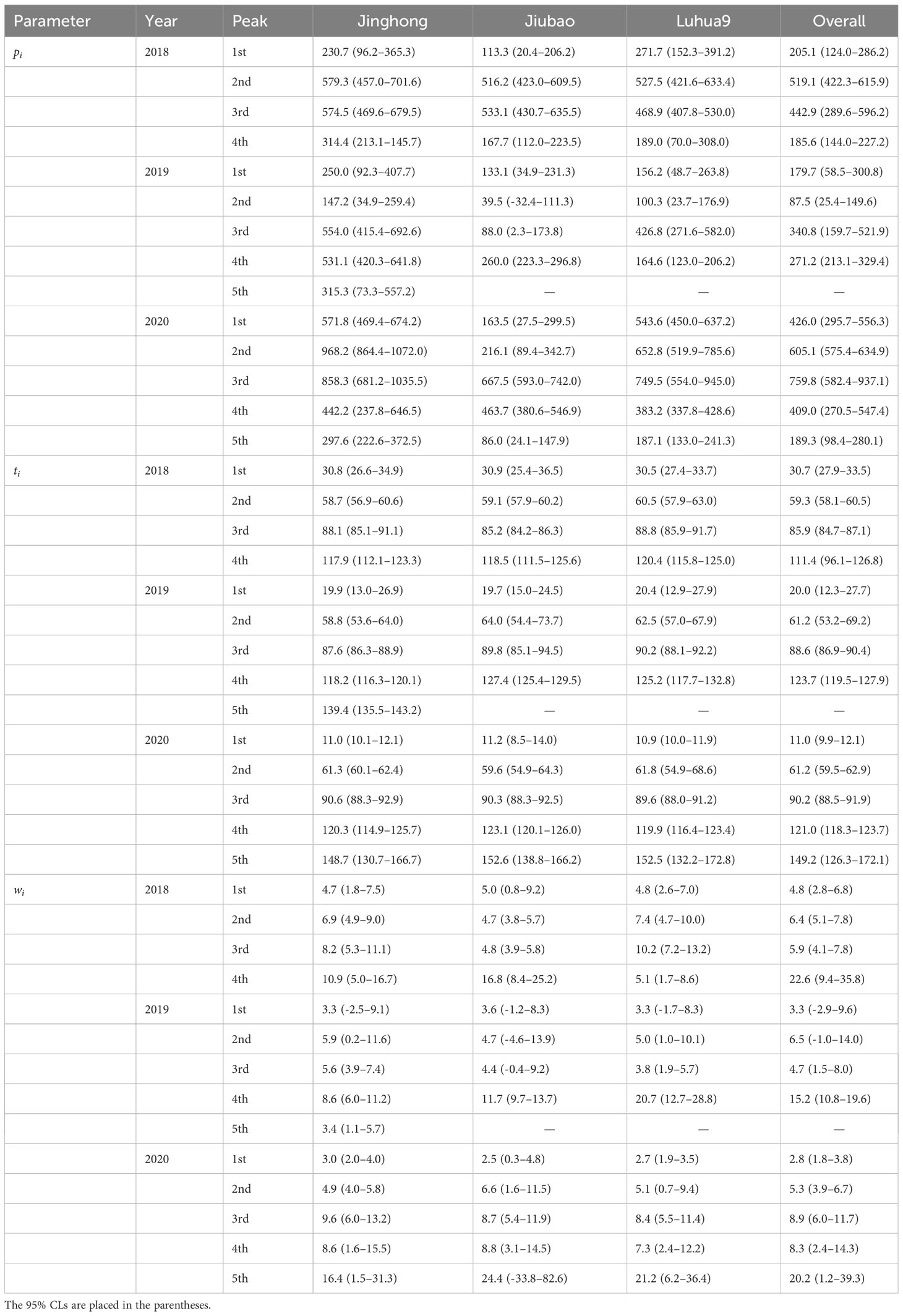
Table 1 Estimated number of moths captured (pi) and timing (ti, days since 02 April) of flight peak of Grapholita molesta males in peach orchards of different cultivars in three consecutive years: y = , where is the coefficients controlling the width of i peak.
Adoxophyes orana had three male flight peaks per year (Figure 3). Regardless of the cultivars, the population peak was significantly higher in the last peak in 2018 and 2019 (non-overlapped 95% CLs); however, in 2020, it was significantly higher in the 2nd and 3rd peaks in the Jinghong orchards with no significant difference in peak height between the three peaks in Jiubao and Luhua9 orchards (non-overlapped 95% CLs) (Figure 3; Table 2). The timing of flight peak of A. orana male adults was significantly different between peaks for a given cultivar in each year (non-overlapped 95% CLs); however, for a given flight peak, the timing was not significantly different between cultivars in each year (overlapped 95% CLs) (Figure 3; Table 2).
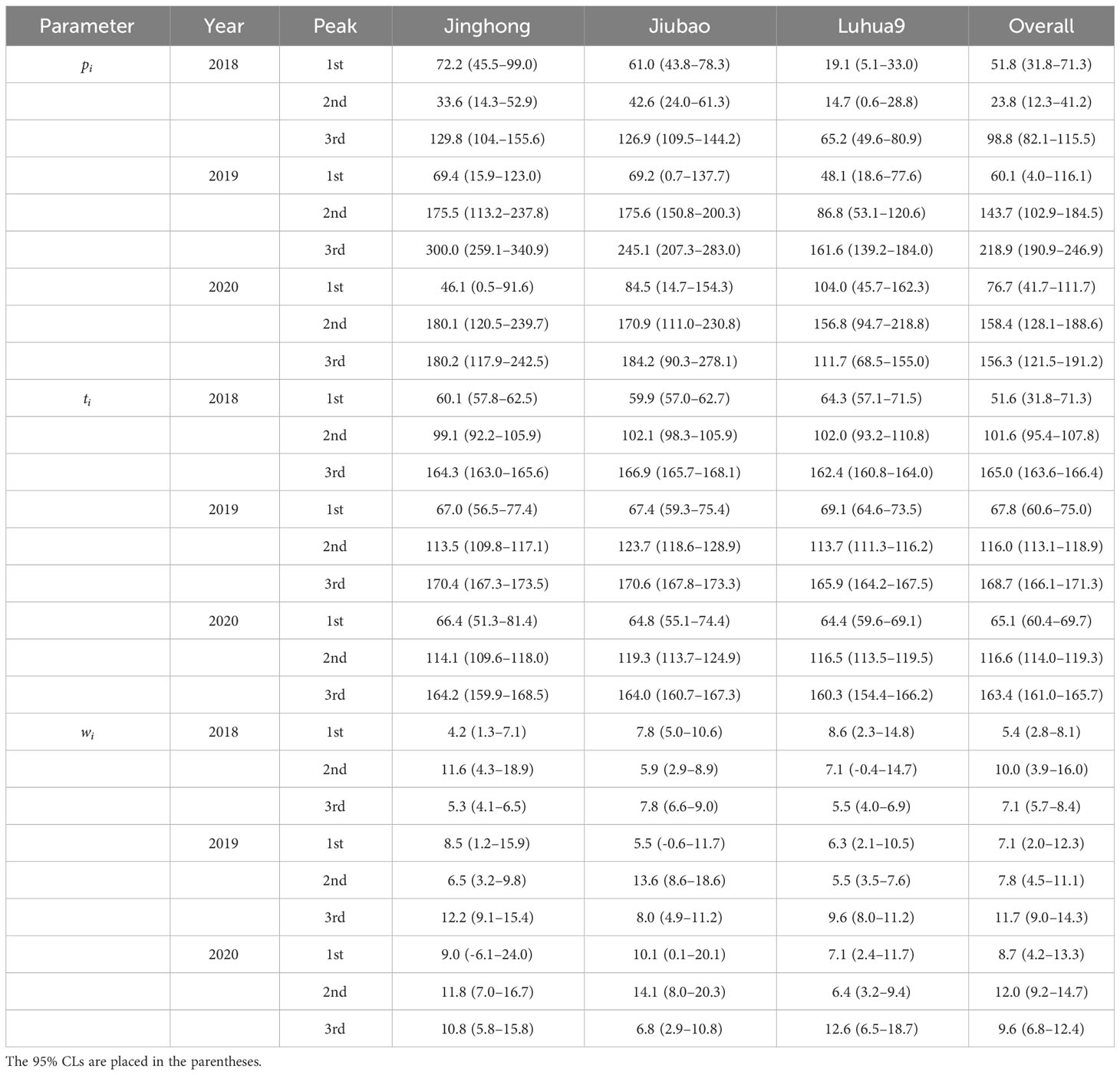
Table 2 Estimated number of moths captured (pi) and timing (ti, days since 02 April) of flight peak of Adoxophyes orana males in peach orchards of different cultivars in three consecutive years: y = , where is the coefficients controlling the width of i peak.
The total number of G. molesta male adults captured was significantly higher in 2020 than in 2018 and 2019, and it was significantly higher in Jinghong and Luhua9 orchards than in the Jiubao one in 2018 and 2020 (F8,18 = 31.29, P< 0.0001) (Figure 4A). For A. orana, the number of males captured was significantly higher in Jinghong and Jiubao orchards in 2019 and 2020 (F8,18 = 20.97, P< 0.0001) (Figure 4B). For a given cultivar and year, the number of G. molesta male adults was significantly higher than that of A. orana (F1,4 = 9.93–222.46, P< 0.05), except that in the Jiubao orchard in 2019 (F1,4 = 1.45, P = 0.2820).
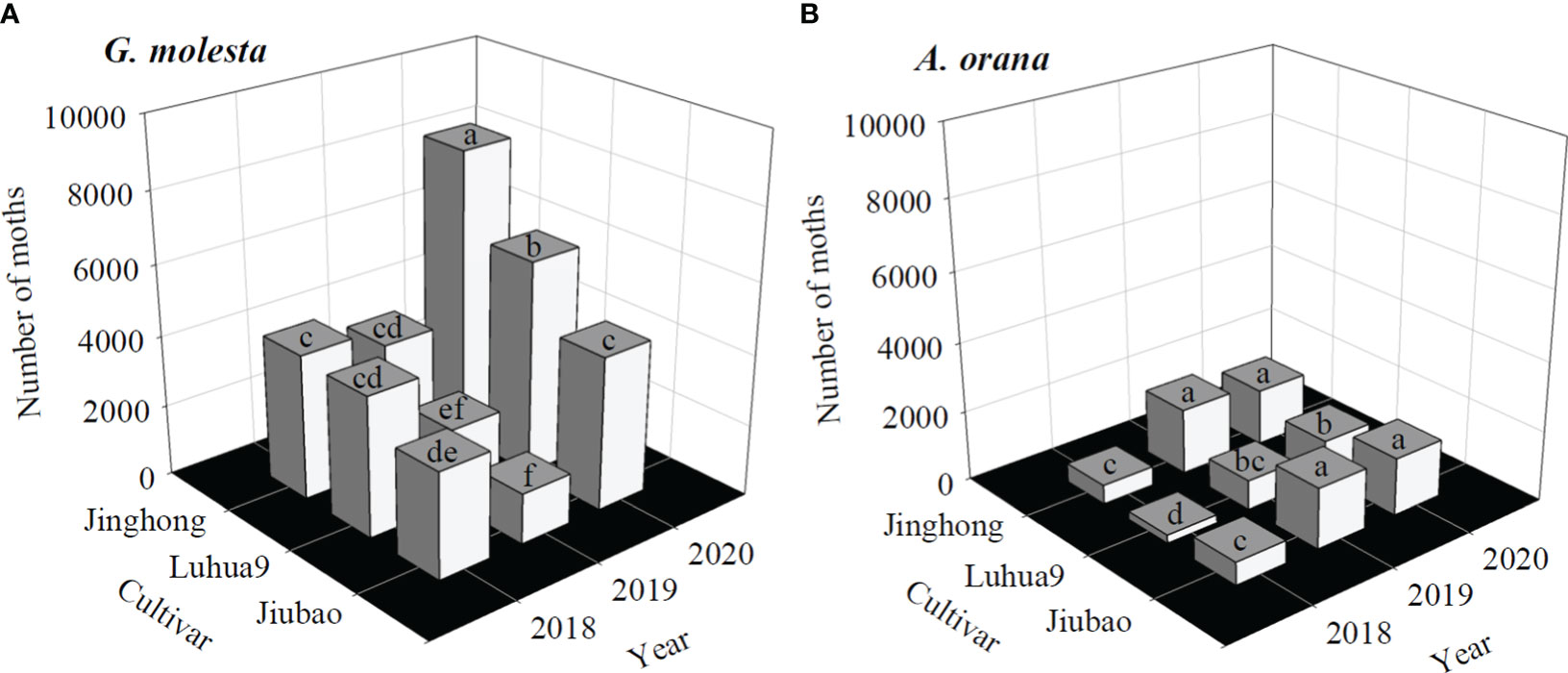
Figure 4 Cumulative number of male adults captured in peach orchards of different cultivars in three successive years: (A) Grapholita molesta, and (B) Adoxophyes orana. For each moth species, columns with the same letter are not significantly different (ANOVA: P > 0.05).
Degree-days
The first G. molesta male adult was expected to emerge from early to mid-April with a cumulative DD of 50–136, while the first A. orana male adult emerged one month later with a cumulative DD of about 460 (Table 3). Generally, A. orana required more DD to reach the subsequent flight peaks and egg hatching than did G. molesta (Table 3).
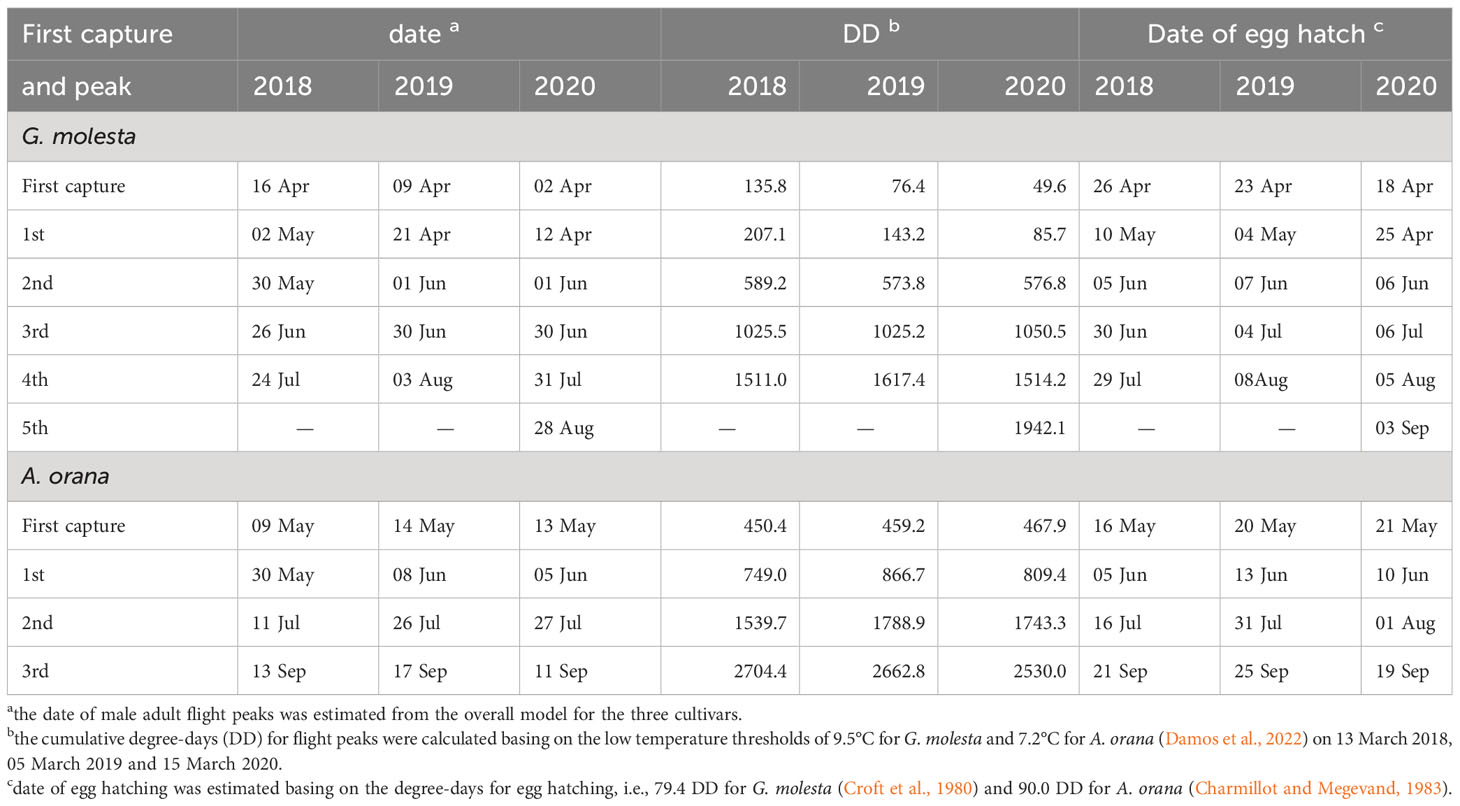
Table 3 Timing (date) of first adult captured/male adult flight peaks (from the overall model), cumulative degree-days (DD) for flight peaks, and timing (date) of egg hatching of Grapholita molesta and Adoxophyes orana in different years.
Discussion
The first step to integrated pest management (IPM) of the identified species is the timely detection of a pest infestation. Sex pheromone traps have long been used for detecting presence of insects in the early season and long-term monitoring of pest population size during the growing seasons. We detect three distinct flight peaks for A. orana and 4–5 flight peaks G. molesta during the growing seasons (Figures 2, 3). According to previous studies (Milonas et al., 2001; Kumral et al., 2005; Damos et al., 2022), the number of flight peaks in lepidopteran moths may roughly reflect the number of field generations. However, Damos et al. (2022) argue that as the season progresses, the higher variation in the last moth flights could be observed, probably due to the extreme high temperatures occurring during the summer which slow down the development and cause bimodal peaks. In this study we did not record a high temperature during the 3rd flight peak in July in 2019 and 2020 compared to that in 2018 (Figure 1A). Alternately, according to Zhao et al. (2017), the continuous low soil water content due to the low precipitation in August 2019/2020 (Figure 1B) may result in the bimodal adult emergence peaks. Therefore, the additional fifth flight peaks of G. molesta in late season in 2019 (for Jinghong only) and 2020 (for all cultivars) could be from the same generation, and there were three and four generations detected for A. orana and G. molesta, respectively. The various number of flight peaks or generations between these two species may be attributed to their natural phenology, i.e., G. molesta overwinter as the mature instar larvae (Rothschild and Vickers, 1991; Notter-Hausmann and Dorn, 2010) and overwintered larvae pupate and adults emerge in the spring (Sarai, 1970; Ellis and Hull, 2013), while A. orana overwinters at the 3rd instar (Oku, 1966) and overwintered larvae feed and develop again before adult emergence and thus require more time to complete the lifecycle and start a new generation.
Invasive herbivores usually utilize a broad range of host plants. Previous research reveals that both G. molesta and A. orana prefer peach over other host plants, such as apple and/or pear, for larval feeding, development or oviposition (Myers et al., 2007; Piñero and Dorn, 2009; Najar-Rodriguez et al., 2013a; Najar-Rodriguez et al., 2013b; Li et al., 2021). In the precent study, we further show that among different peach cultivars, the total number of G. molesta male adults captured was significantly higher in Jinghong than in Luhua9 with significantly lesser male adults trapped in Jiubao (Figure 4A), while the A. orana population size was significantly higher in Jinghong and Jiubao than in Luhua9 (Figure 4B). We suggest a variation in plant nutrients existing between peach cultivars, which may impact the interactions between host plants and insect herbivores, regulating the herbivore fecundity (Awmack and Leather, 2002) and affecting their population and community dynamics (Joern et al., 2012). In addition, we also show that the population size of G. molesta was usually significantly higher than that of A. orana (Figure 4), suggesting that G. molesta performed better on peaches than did A. orana. To understand the mechanisms behind the varying population dynamic between cultivars and varying population size between herbivore species, future studies should assess development and reproduction of G. molesta and A. orana reared on growing shoots/leaves and peach fruit of different cultivars. Furthermore, it should be noted that the differences in environmental conditions across years and possible in the relative effectiveness of pheromone traps/lures for these two species may lead to the variations of moth populations between different years (Figures 2, 3) and between species (Figure 4). However, these assumptions require further investigations, e.g., independently testing the specific pheromone traps/lures in successive years and separated orchards.
Although the number of moths captured in orchards of each peach cultivar varied between flight peaks, the seasonal phenology of G. molesta or A. orana was quite stable with an identical timing of each flight peak between cultivars in a year (Figures 2, 3; Tables 1, 2). The identical flight timing allows us to fit the overall data of different cultivars using a single phenology model. The precise prediction of flight timing coordinating with the degree-days technique (e.g., Rice et al., 1984; Tobin et al., 2003; Pehlevan and Kovanci, 2017; Rowley et al., 2017; Graf et al., 2018; Damos et al., 2022) may provide critical information to optimize the schedule of pheromone trap or pesticide applications. For example, the flight peak of overwintered G. molesta or A. orana was usually lower than the consequent peaks (i.e., the 2nd and 3rd peaks) (Figures 2, 3; Tables 1, 2), which provides opportunities to prevent the outbreaks of field populations in the later seasons when the overwintered population size is low in early spring (Tshernyshei, 1995; Parry et al., 2019). Therefore, to trap the newly emerged male adults or disrupting female mating success in the 1st generation in peach orchards, deploying pheromone traps would be scheduled in April with a cumulative DD between 49.6 (for the earliest male captured in 2020) and 207.1 (for the 1st flight peak in 2018) for G. molesta and in mid-May–early June with a cumulative DD between 450.4 (for the earliest male captured in 2018) and 866.7 (for the 1st flight peak in 2019) for A. orana (Table 3). The high variation of degree day calculations for the first emergence and first flight peak for G. molesta in different years (Table 3) may be attributed to the various environmental temperatures before adult emergence (Figure 1). The precise predictions of first adult flight and larval emergence basing on the calculated cumulative DD need future confirmation in orchards in early spring. Additionally, according to the thermal requirement for egg hatching [i.e., 79.4 DD for G. molesta (Croft et al., 1980) and 90.0 DD for A. orana (Charmillot and Megevand, 1983)], insecticide treatments would be applied in late-April–early May and late May–early June to reduce the field population density of neonates of G. molesta and A. orana, respectively.
Our results also demonstrate that compared to the overwintered population, the moth populations were higher during the growing seasons (Figures 2, 3). Therefore, to reduce the fruit damage, pheromone traps would be deployed in June to suppress G. molesta populations in the 2nd and 3rd generations and in mid- to early July to suppress A. orana populations in 2nd generation when the cumulative DD reached 573.8–1025.2 for G. molesta and 1539.7–1788.9 for A. orana (Table 3). In addition, applications of short term-residue insecticides in early June to early July and mid-July to late July (Table 3) might respectively suppress populations of G. molesta and A. orana neonate feeding on peach fruit. Furthermore, it is well known that there is positive relationship between the overwintering and next spring populations (Hu et al., 2015; Ju et al., 2017; Jorgensen et al., 2020). It is thus suggested that pheromone traps could be set up in late July–early August to trap G. molesta of the 4th generation and in mid-September to trap A. orana of the 3rd generation when the cumulative DD reached 1511.0–1617.4 for G. molesta and 2704.4–2662.8 for A. orana, which may reduce overwintering population size and thus decrease pest infestation in next year. However, application of chemical treatments to reduce the neonate population is not encouraged just before fruit harvest. Furthermore, mating disruption using sex pheromones may be less effective during the last generation, because moth survival is largely determined by some other factors such as the temperature, moisture and predators during overwintering.
In conclusion, results of this study show that there are three clear flight peaks reflecting three generations for A. orana, with four to five flight peaks observed but probably only four generations for G. molesta. We find that the population size of G. molesta in peach cultivars of Jinghong and Luhua9 is higher than that in Jiubao, while A. orana population is higher in peach cultivars of Jinghong and Jiubao than in Luhua9. We develop a logistic multiple-peaks model to predict the seasonal phenology of moths and integrate the results with the degree-days calculation, which may help optimize the precise timings of pheromone trap and insecticide applications aiming to suppress the male adult population size and reduce neonate population density of both G. molesta and A. orana. Future research will investigate the efficiency of precise timing and number of pheromone traps and insecticide applications in suppressing pest populations in field.
Data availability statement
The raw data supporting the conclusions of this article will be made available by the authors, without undue reservation.
Ethics statement
The manuscript presents research on animals that do not require ethical approval for their study.
Author contributions
HZ: Conceptualization, Data curation, Investigation, Methodology, Validation, Writing – original draft, Writing – review & editing. AM: Conceptualization, Investigation, Methodology, Supervision, Validation, Writing – original draft, Writing – review & editing. HR: Investigation, Validation, Writing – review & editing. XY: Investigation, Validation, Writing – review & editing. JH: Investigation, Validation, Writing – review & editing. JZ: Investigation, Validation, Writing – review & editing. HL: Investigation, Validation, Writing – review & editing. ZY: Investigation, Validation, Writing – review & editing. XW: Investigation, Validation, Writing – review & editing. XH: Data curation, Formal Analysis, Software, Validation, Writing – original draft, Writing – review & editing. JL: Conceptualization, Funding acquisition, Methodology, Project administration, Resources, Supervision, Validation, Writing – original draft, Writing – review & editing.
Funding
The authors declare financial support was received for the research, authorship, and/or publication of this article. This work was partly supported by the Earmarked Fund for Hebei Peach Innovation Team of Modern Agro-industry Technology Research System (HBCT2021220205).
Acknowledgments
We would like to thank Shunping Wangrui Farmer Professional Cooperativeal allowed us to set up the experiments and collect data in the peach orchards, and Mrs. Rui Jiao for her technical support during the early stage of statistical analysis. We are also very grateful to two reviewers for their constructive comments on an earlier manuscript, which have significantly improved the quality of the paper.
Conflict of interest
The authors declare that the research was conducted in the absence of any commercial or financial relationships that could be construed as a potential conflict of interest.
Publisher’s note
All claims expressed in this article are solely those of the authors and do not necessarily represent those of their affiliated organizations, or those of the publisher, the editors and the reviewers. Any product that may be evaluated in this article, or claim that may be made by its manufacturer, is not guaranteed or endorsed by the publisher.
References
Anon (2014). Texas invasive species: oriental fruit moth (San Marcos, USA: Texas State University). Available at: https://www.tsusinvasives.org/home/database/grapholita-molesta.
Archontoulis S. V., Miguez F. E. (2015). Nonlinear regression models and applications in agricultural research. Agron. J. 107, 786–798. doi: 10.2134/agronj2012.0506
Awmack C. S., Leather S. R. (2002). Host plant quality and fecundity in herbivorous insects. Ann. Rev. Entomol. 47, 817–844. doi: 10.1146/annurev.ento.47.091201.145300
Byun B.-K., Lee B.-W., Lee E.-S., Choi D.-S., Park Y. M., Yang C. Y., et al. (2012). A review of the genus Adoxophyes (Lepidoptera Tortricidae) in Korea, with description of A. paraorana sp. nov. Anim. Cells Syst. 16, 154–161. doi: 10.1080/19768354.2011.611175
CAB International (2008). Crop Protection Compendium (Wallingford, UK: International Plant Protection Convention). Available at: http://www.cabicompendium.org/cpc/home.asp.
Charmillot P. J., Megevand B. (1983). Development of Adoxophyes orana in relation to temperature and consequences for practical control. Bull. OEPP/EPPO Bull. 13, 145–151.
Croft B. A., Michels M. F., Rice R. E. (1980). Validation of a PETE timing model for the oriental fruit moth in Michigan and central California (Lepidoptera: Olethreutidae). Great Lakes Entomol. 13, 211–217.
Damos P., Karabatakis S. (2012). “Real time pest population modeling through the World Wide Web: decision making from theory to praxis,” in Proceedings of the 8th International Conference on Integrated Fruit Production, Kusadasi (Turkey, October 7–12. 223–228 (IOBC wprs Bulletin OILB srop).
Damos P., Savopoulou-Soultani M. (2010). Development and statistical evaluation of models in forecasting moth phenology of major lepidopterous peach pest complex for integrated pest management programs. Crop Prot. 29, 1190–1199. doi: 10.1016/j.cropro.2010.06.022
Damos P., Savopoulou-Soultani M. (2012a). “Microlepidoptera of economic significance in fruit production: challenges, constrains and future perspectives for integrated pest management,” in Moths: Types, Ecological Significance and Control Methods. Ed. Cauterrucio L.. New York: Nova Science Publishers, 75–113.
Damos P., Savopoulou-Soultani M. (2012b). Temperature-driven models for insect development and vital thermal requirements. Psyche 2012, 123405. doi: 10.1155/2012/123405
Damos P. T., Soulopoulou P., Gkouderis D., Monastiridis D., Vrettou M., Sakellariou D., et al. (2022). Degree-day risk thresholds for predicting the occurrence of Anarsia lineatella, Grapholita molesta and Adoxophyes orana in northern Greece peach orchards. Plant Protect. Sci. 58, 234–244. doi: 10.17221/137/2021-PPS
de Jong D. J., Ankersmit G. W., Barel C. J. A., Minks A. K. (1971). “Summer fruit tortrix moth, Adoxophyes orana F.R. studies on biology, behavior, and population dynamics in relation to the application of the sterility principle,” in Proceedings of a panel on the Application of Induced Sterility for Control of Lepidopterous Populations, Vienna, 1–5 June 1970. Wien, Austria: International Atomic Energy Agency (IAEA), 27–39.
de Lame F. M., Hong J., Shearer P. W., Brattsten L. B. (2001). Sex-related differences in the tolerance of oriental fruit moth (Grapholita molesta) to organophosphate insecticides. Pest Manage. Sci. 57, 827–832. doi: 10.1002/ps.368
Del Tío R., Martínez J. L., Ocete R., Ocete M. E. (2001). Study of the relationship between sex pheromone trap catches of Lobesia botrana (Den. & Schiff.) (Lep., Tortricidae) and the accumulation of degree-days in Sherry vineyards (SW of Spain). J. Appl. Entomol. 125, 9–14. doi: 10.1111/j.1439-0418.2001.00507.x
Ellis N. H., Hull L. A. (2013). Factors influencing adult male Grapholita molesta dispersal in commercial Malus and Prunus host crops. Entomol. Exp. Appl. 146, 232–241. doi: 10.1111/eea.12015
Ferracini C., Pogolotti C., Rama F., Lentini G., Saitta V., Mereghetti P., et al. (2021). Pheromone-mediated mating disruption as management option for Cydia spp. in Chestnut Orchard. Insects 12, 905. doi: 10.3390/insects12100905
Godin C., Boivin G. (1998). Seasonal occurrence of lepidopterous pests of cruciferous crops in Southwestern Quebec in relation to degree-day accumulations. Can. Entomol. 130, 173–185. doi: 10.4039/Ent130173-2
Graf B., Höhn H., Höpli H. U., Kuske S. (2018). Predicting the phenology of codling moth, Cydia pomonella, for sustainable pest management in Swiss apple orchards. Entomol. Exp. Appl. 166, 618–627. doi: 10.1111/eea.12717
Hossain M. S., Williams D. G., Mansfield C., Bartelt R. J., Callinan L., Il’ichev A. L. (2006). An attract-and-kill system to control Carpophilus spp. in Australian stone fruit orchards. Entomol. Exp. Appl. 118, 11–19. doi: 10.1111/j.1570-7458.2006.00354.x
Hrdý I., Kocourek F., Berankova J., Kuldova J. (1996). Temperature models for predicting the flight activity of local populations of Cydia funebrana (Lepidoptera: Tortricidae) in Central Europe. Eur. J. Entomol. 93, 569–578.
Hu S. J., Fu D. Y., Han Z. L., Ye H. (2015). Density, demography, and influential environmental factors on overwintering populations of Sogatella furcifera (Hemiptera: Delphacidae) in Southern Yunnan, China. J. Insect. Sci. 15, 58. doi: 10.1093/jisesa/iev041
Ivezić A., Mimić G., Trudić B., Blagojević D., Kuzmanović B., Kaitović Ž., et al. (2023). Development of a predictive model of the flight dynamics of the European corn borer, Ostrinia nubilalis Hübner 1796 (Lepidoptera: Pyralidae), in the Vojvodina Region, Serbia – implications for integrated pest management. Agronomy 13, 1494. doi: 10.3390/agronomy13061494
Joern A., Provin T., Behmer S. T. (2012). Not just the usual suspects: insect herbivore populations and communities are associated with multiple plant nutrients. Ecology 93, 1002–1015. doi: 10.1890/11-1142.1
Jones V. P., Hilton R., Brunner J. F., Bentley W. J., Alston D. G., Barrett, et al. (2013). Predicting the emergence of the codling moth, Cydia pomonella (Lepidoptera: Tortricidae), on a degree-day scale in North America. Pest Manage. Sci. 69, 1393–1398. doi: 10.1002/ps.3519
Jorgensen A., Otani J., Evenden M. L. (2020). Assessment of available tools for monitoring wheat midge (Diptera: Cecidomyiidae). Environ. Entomol. 49, 627–637. doi: 10.1093/ee/nvaa017
Ju R. T., Gao L., Wei S. J., Li B. (2017). Spring warming increases the abundance of an invasive specialist insect: links to phenology and life history. Sci. Rep. 7, 14805. doi: 10.1038/s41598-017-14989-3
Julious S. A. (2004). Using confidence intervals around individual means to assess statistical significance between two means. Pharm. Stat. J. Appl. Stat. Pharm. Indust. 3, 217–222. doi: 10.1002/pst.126
Kanga L. H. B., Pree D. J., van Lier J. L., Walker G. M. (2003). Management of insecticide resistance in oriental fruit moth (Grapholita molesta; Lepidoptera: Tortricidae) populations from Ontario. Pest Manage. Sci. 59, 921–927. doi: 10.1002/ps.702
Kirk H., Dorn S., Mazzi D. (2013). Worldwide population genetic structure of the oriental fruit moth (Grapholita molesta), a globally invasive pest. BMC Ecol. 13, 12. doi: 10.1186/1472-6785-13-12
Kocourek F., Stara J. (2005). Predictive value of a model of the flight activity of Adoxophyes orana (Lep.: Tortricidae). J. Pest Sci. 78, 205–211. doi: 10.1007/s10340-005-0093-6
Kong W. N., Wang Y., Guo Y. F., Chai X. H., Niu G. F., Li Y., et al. (2020). Effects of disruption of Grapholita molesta (Lepidoptera: Tortricidae) using sex pheromone on moth pests and insect communities in orchards. Appl. Entomol. Zool. 55, 367–377. doi: 10.1007/s13355-020-00691-w
Kumral N. A., Kovanci B., Akbudak B. (2005). Pheromone trap catches of the olive moth Prays oleae (Bern.) (Lep., Plutellidae) in relation to olive phenology and degree-day models. J. Appl. Entomol. 129, 375–381. doi: 10.1111/j.1439-0418.2005.00985.x
Li G.-W., Wang H.-M., Yang W.-T., Chen X.-L., Li B.-L., Chen Y.-X. (2021). Influence of host plants on the development, survivorship, and fecundity of the summer fruit tortrix moth, Adoxophyes orana (Lepidoptera: Tortricidae). Entomol. Res. 51, 499–508. doi: 10.1111/1748-5967.12542
Liu C.-M., Phukhahad S., Auamcharoen W., Matsuyama S., Kainoh Y. (2022). Oviposition preferences of the oriental fruit moth Grapholita molesta (Lepidoptera: Tortricidae) to pear HIPVs. Arthropod-Plant Interact 16, 517–523. doi: 10.21203/rs.3.rs-1094673/v1
Milonas P. G., Savopoulou-Soultani M., Stavridis D. G. (2001). Day-degree models for predicting the generation time and flight activity of local populations of Lobesia botrana (Den. & Schiff.) (Lep., Tortricidae) in Greece. J. Appl. Entomol. 125, 515–518. doi: 10.1046/j.1439-0418.2001.00594.x
Myers C. T., Hull L. A., Krawczyk G. (2007). Effects of orchard host plants (apple and peach) on development of oriental fruit moth (Lepidoptera: Tortricidae). J. Econ Entomol 100, 421–430. doi: 10.1603/0022-0493(2007)100[421:eoohpa]2.0.co;2
Najar-Rodriguez A., Bellutti N., Dorn S. (2013a). Larval performance of the oriental fruit moth across fruits from primary and secondary hosts. Physiol. Entomol. 38, 63–70. doi: 10.1111/phen.12003
Najar-Rodriguez A., Orschel B., Dorn S. (2013b). Season-long volatile emissions from peach and pear trees in situ, overlapping profiles, and olfactory attraction of an oligophagous fruit moth in the laboratory. J. Chem. Ecol. 39, 418–429. doi: 10.1007/s10886-013-0262-7
Navarro-Roldán M. A., Avilla J., Bosch D., Valls J., Gemeno C. (2017). Comparative effect of three neurotoxic insecticides with different modes of action on adult males and females of three tortricid moth pests. J. Econ. Entomol. 110, 1740–1749. doi: 10.1093/jee/tox113
Neven L. G., Kumar S., Yee W. L., Wakie T. (2018). Current and future potential risk of establishment of grapholita molesta (Lepidoptera: tortricidae) in Washington State. Environ. Entomol. 47, 448–456. doi: 10.1093/ee/nvx203
Notter-Hausmann C., Dorn S. (2010). Relationship between behavior and physiology in an invasive pest species: oviposition site selection and temperature dependent development of the oriental fruit moth (Lepidoptera: Tortricidae). Environ. Entomol. 39, 561–569. doi: 10.1603/EN09231
Oku T. (1966). Diapause of some apple leaf rollers at an earlier larval instar (Lepidoptera: Tortricidae): pre-diapause behavior and peculiarity of life-cycle. Kontyu 34, 144–153.
Parry H., Capon T., Clarke A., Crisp P., Hulthen A., Kandulu J., et al. (2019). “Area-wide management guidelines for sterile insect technique developed through interdisciplinary modelling research,” in MODSIM2019, 23rd International Congress on Modelling and Simulation. Ed. Elsawah S. (Australia: Modelling and Simulation Society of Australia and New Zealand Inc. (MSSANZ), 652–657.
Pastori P. L., Arioli C. J., Botton M., Monteiro L. B., Stoltman L., Mafra-Neto A. (2012). Integrated control of two tortricids (Lepidoptera) pests in apple orchards with sex pheromones and insecticides. Rev. Colomb. Entomol. 38, 224–230. doi: 10.25100/socolen.v38i2.8996
Pehlevan B., Kovanci O. B. (2014). First report of Adoxophyes orana in northwestern Turkey: population fluctuation and damage on different host plants. Turk. J. Agric. For. 38, 9. doi: 10.3906/tar-1402-11
Pehlevan B., Kovanci O. B. (2017). Using degree-day and nonlinear regression models to predict seasonal flights of Adoxophyes orana (Fischer von Röslerstamm 1834) (Lepidoptera: Tortricidae) in plum orchards. Turk. J. Entomol. 41, 75–86. doi: 10.16970/ted.87203
Philips J. H. H. (1973). Monitoring for the oriental fruit moth with synthetic sex pheromone. Environ. Entomol 2, 1039–1042. doi: 10.1093/ee/2.6.1039
Piñero J. C., Dorn S. (2009). Response of female oriental fruit moth to volatiles from apple and peach trees at three phenological stages. Entomol. Exp. Appl. 131, 67–74. doi: 10.1111/j.1570-7458.2009.00832.x
Pree D .J., Whitty K. J., van Driel L., Walker G .M. (1998). Resistance to insecticides in oriental fruit moth populations (Grapholita molesta) from the Niagara Peninsula of Ontario. Can. Entomol. 130, 245–256. doi: 10.4039/Ent130245-3
Prues K. P. (1983). Day-degree methods for pest management. Environ. Entomol. 12, 613–619. doi: 10.1093/ee/12.3.613
Reis Fo W., Nora I., Melzer R. (1988). Population dynamics of Grapholita molesta, Busk 1916, and its adaptation on apple in South Brazil. Acta Hortic. 232, 204–208. doi: 10.17660/ActaHortic.1988.232.27
Rice R. E., Weakley C. V., Jones R. A. (1984). Using degree-days to determine optimum spray timing for the oriental fruit moth (Lepidoptera: Tortricidae). J. Econ. Entomol. 77, 698–700. doi: 10.1093/jee/77.3.698
Rothschild G. H. L., Vickers R. A. (1991). “Biology, ecology and control of the oriental fruit moth,” in Tortricid pests: their biology, natural enemies and control. Eds. van der Geest L. P. S., Evenhuis H. H. (Amsterdam: Elsevier), 389–412.
Rowley C., Cherrill A., Leather S. R., Pope T. W. (2017). Degree-day based phenological forecasting model of saddle gall midge (Haplodiplosis marginata) (Diptera: Cecidomyiidae) emergence. Crop Prot. 102, 154–160. doi: 10.1016/j.cropro.2017.08.025
Sarai D. S. (1970). The seasonal history of the oriental fruit moth in southern Missouri. J. Econ. Entomol. 63, 301–302. doi: 10.1093/JEE/63.1.301
Stamenkovic S., Pesic M. (1998). Economically important Tortricidae of pear. Acta Entomol. Serbica. 3, 149–157.
Thorpe K. W., Reardon R., Tcheslavskaia K., Leonard D., Mastro V. (2006). A review of the use of mating disruption to manage gypsy moth, Lymantria dispar (L.) (Washington, DC, USA: U.S. Department of Agriculture, Forest Service, Forest Health Technology Enterprise Team). 114 p.
Tobin P. C., Nagarkatti S., Saunders M. C. (2003). Phenology of grape berry moth (Lepidoptera: Tortricidae) in cultivated grape at selected geographic locations. Environ. Entomol. 32, 340–346. doi: 10.1603/0046-225X-32.2.340
Trimble R. M., Pree D. J., Carter N. J. (2001). Integrated control of oriental fruit moth (Lepidoptera: Tortricidae) in peach orchards using insecticide and mating disruption. J. Econ. Entomol. 94, 476c485. doi: 10.1603/0022-0493-94.2.476
Tshernyshei W. B. (1995). Ecological pest management (EPM): general approaches. J. Appl. Entomol. 119, 379–381. doi: 10.1111/j.1439-0418.1995.tb01304.x
van der Geest L. P. S., Evenhuis H. H. (1991). “World crop pests,” in Tortricid pests: their biology, natural enemies and control, vol. 5. (New York: Elsevier). 816 p.
Wang X., Li Y. S., Zhang J., Zhang Q. W., Liu X. X., Li Z. (2017). De novo characterization of microRNAs in oriental fruit moth Grapholita molesta and selection of reference genes for normalization of microRNA expression. PloS One 12, e0171120. doi: 10.1371/journal.pone.0171120
Whittle K. (1985). Summer fruit tortrix moth: Adoxophyes orana (Fischer von Roeslerstamm) (Hyattsville, MD, USA: United States Department of Agriculture, Animal and Plant Health Inspection Service).
Keywords: lepidopteran pest, population dynamics, sex pheromone trap, flight activity modeling, degree-days, pest management
Citation: Ma A, Zhang H, Ran H, Yang X, Hao J, Zhang J, Li H, Yu Z, Wang X, He XZ and Li J (2023) Prediction of seasonal population dynamics of Grapholita molesta (Busck) and Adoxophyes orana (Fischer von Röslerstamm) in peach orchards using sex pheromone trap and degree-days and its implications in pest management. Front. Agron. 5:1269977. doi: 10.3389/fagro.2023.1269977
Received: 31 July 2023; Accepted: 19 September 2023;
Published: 04 October 2023.
Edited by:
Murray B. Isman, University of British Columbia, CanadaReviewed by:
David Robert Hall, University of Greenwich, United KingdomOdair Aparecido Fernandes, São Paulo State University, Brazil
Copyright © 2023 Ma, Zhang, Ran, Yang, Hao, Zhang, Li, Yu, Wang, He and Li. This is an open-access article distributed under the terms of the Creative Commons Attribution License (CC BY). The use, distribution or reproduction in other forums is permitted, provided the original author(s) and the copyright owner(s) are credited and that the original publication in this journal is cited, in accordance with accepted academic practice. No use, distribution or reproduction is permitted which does not comply with these terms.
*Correspondence: He Zhang, zhangheau@163.com ; Xiong Zhao He, x.z.he@massey.ac.nz; Jiancheng Li, lijiancheng08@163.com