- 1Land Resources and Environmental Sciences, Montana State University, Bozeman, MT, United States
- 2Central Agriculture Research Center, College of Agriculture, Montana State University, Moccasin, MT, United States
Cirsium arvense (Canada thistle) is a perennial weed that causes significant economic losses in agriculture. An extensive rhizomatous root system makes C. arvense difficult to manage, particularly in agricultural systems that use tillage as a primary management tool. There is a need for the development of integrated weed management toolsets that include C. arvense biological controls. Puccinia punctiformis (thistle rust) is an autoecious fungal pathogen that systemically infects C. arvense, with the potential to reduce host vigor over time. The goal of this study was to integrate the P. punctiformis biocontrol with a simulated annual cropping sequence in a greenhouse environment and evaluate C. arvense’s above-and belowground biomass production, and its competitive ability. Repeated P. punctiformis inoculations produced systemically infected C. arvense stems in greenhouse pots over time. Cirsium arvense that was inoculated with P. punctiformis had 1.6 grams/pot (p = 0.0019) less aboveground biomass and 5.6 grams/pot (p< 0.001) less belowground biomass, compared to the non-inoculated (control). Puccinia punctiformis and crop competition interacted additively to lower aboveground (p<0.001) and belowground (p<0.001) C. arvense biomass more than individual use of either the biocontrol or competition alone. The aboveground competition intensity of C. arvense in a mixed crop sequence, relative to non-inoculated C. arvense grown in a monoculture, was moderately impacted by the P. punctiformis biocontrol (p = 0.0987). These results indicate that systemic infection can reduce biomass production and the competitive ability of C. arvense. Overall, P. punctiformis can be integrated into competitive annual cropping sequences with the potential to reduce C. arvense vigor over time.
1 Introduction
Cirsium arvense (L.) Scop. (Canada thistle) is a problematic weed that causes large economic losses in agriculture, driving the need for integrated weed management tools that include biological control agents (Orloff et al., 2018). Cirsium arvense can be found throughout temperate climates of the world, where it exists as a perennial herb that reproduces through an extensive rhizomatous root system and wind dispersed seeds (Tiley, 2010). Clonal rhizomes make C. arvense resilient to disturbance, particularly in tilled organic cropping systems that do not use synthetic herbicides for weed management (Moore, 1975). Organic producers in the Northern Great Plains region of the United States generally depend on tillage as a primary weed management tool, however this practice increases soil erosion due to wind and water and depletes soil organic matter over time (Lenhoff et al., 2017). Additionally, tillage can disperse vigorous C. arvense rhizomes, causing a rapid increase of the weed’s population (Tiley, 2010). As a result, C. arvense has become a serious management problem within organic cropping systems, where alternative management tools need to be explored (Tautges et al., 2016; Orloff et al., 2018).
The use of competitive annual crops is another common approach used to manage weeds in organic cropping systems (Bullock, 1992; Liebman and Dyck, 1993). Competitive crops can disrupt weed growth by reducing resource availability and niche dominance of weed species (Liebman and Dyck, 1993). However, the difficult nature of reducing C. arvense rhizomes, particularly in organic agriculture (Tautges et al., 2016; Orloff et al., 2018), has led to a search for alternative and integrated tactics, including biocontrol agents that inhibit root development (Berner et al., 2013; Cripps et al., 2014). The use of biocontrol agents can be challenging due to a lack of host specificity, varied responses to environmental conditions, and mismanagement. However, continued exploration of biocontrols for C. arvense has the potential to yield low-cost, long-term, host-specific options that can be integrated into existing weed management toolsets (Berner et al., 2013).
Puccinia punctiformis (F. Strauss) Rohl. (thistle rust) is a heterotrophic fungal pathogen of C. arvense that acts as a long-term systemic parasite (Buller, 1950; Menzies, 1953; Berner et al., 2013; Kentjens et al., 2023). As a parasite that consumes resources and weakens the root structure (Buller, 1950; Menzies, 1953), P. punctiformis is specific to C. arvense (Berner et al., 2013; Kentjens et al., 2023) and has been identified in temperate habitats around the globe (Berner et al., 2013; Kentjens et al., 2023). Once established in the roots, infected C. arvense can develop chlorotic leaf tissue with lesions, elongated stems, and growth irregularities which can reduce fitness and cause death (Buller, 1950; Berner et al., 2013). Diseased stems act as aboveground carriers for P. punctiformis spores, appearing as orange to dark-red pustules on leaves, where the fungus completes most of its five-stage heterothallic life cycle during summer months, eventually producing transmissible teliospores (Buller, 1950; Menzies, 1953; Kentjens et al., 2023). Teliospore-bearing thistle leaves senesce and abscise as precipitation and temperatures decline, where they can contact healthy C. arvense rosettes through wind or mechanical dispersion, leading to long-term systemic infection in new C. arvense hosts under ideal environmental conditions (French and Lightfield, 1990; Berner et al., 2013).
Puccinia punctiformis’ impact on C. arvense abundance has been well documented (French, 1990; Thomas et al., 1994; Berner et al., 2013; Cripps et al., 2014; Kentjens et al., 2023). However, to our knowledge, the effects of integrating the P. punctiformis biocontrol with a competitive crop sequence on C. arvense growth have not been studied. We addressed this gap in knowledge using greenhouse experiment, which assessed the impact of P. punctiformis on C. arvense growth and competitiveness. Specifically, our questions were: 1) What is the probability of observing P. punctiformis infected C. arvense over time, and does the percentage of infected C. arvense stems increase over time? 2) How does P. punctiformis affect C. arvense above- and belowground biomass, and does crop competition interact with the effects? 3) Using a relative competition intensity index (RCI), is the competitive ability of C. arvense reduced when P. punctiformis is integrated into a sequence of competitive annual crops? We hypothesized that the integration of P. punctiformis with a competitive crop sequence would lead to a significant reduction in above- and belowground C. arvense biomass, compared to individual effects from P. punctiformis or crop competition when used alone.
2 Materials and methods
2.1 Experimental design
A greenhouse study with three independent trials was conducted at the Montana State University Plant Growth Center in Bozeman, Montana, between 2020 and 2022. A nested full factorial (2 x 2) design was used to assess the integration of P. punctiformis and crop competition. The primary treatment was P. punctiformis inoculation, with two levels: C. arvense inoculated with P. punctiformis (n = 20) and non-inoculated C. arvense grown as a control (n = 20). Nested within each level of the inoculation treatment was a competition treatment, split into two levels: C. arvense grown in monoculture (n = 10) and C. arvense grown in competition with a common crop species (n = 10; Supplementary Figure 1).
The competition treatment was a four-phase crop sequence that incorporated common crops used by organic farmers in the dryland areas of the Northern Great Plains. The sequence included the following four phases, with seeding depths and seeding rates scaled for greenhouse pots: 1) Fallow: 1-gram C. arvense rhizome planted ~ 10 cm deep; 2) spring wheat: 100 kg/hectare planted ~ 5 cm deep (18 plants/pot); 3) forage pea: 89 kg/hectare planted ~ 5 cm deep (8 plants/pot); and 4) safflower: 33 kg/hectare planted ~ 3 cm deep (2 plants/pot). Cirsium arvense rhizomes were planted in the approximate center of each pot during the first phase. Crops were planted in a manner that provided approximately equal space between individuals, with at least 5 cm of distance from pot edges.
Two separate greenhouse spaces were used to prevent movement of P. punctiformis spores between the P. punctiformis inoculated treatment and the non-inoculated (control) treatment. Internal greenhouse temperatures for both spaces were set at a range of 18°C to 26.5°C during the day, and 10°C to 24°C at night. To ensure consistent lighting, passive solar lighting with supplemental 1000-watt metal halide lamps, set to 12 hour intervals, were used throughout the course of the study.
2.2 Cirsium arvense and Puccinia punctiformis establishment
Cirsium arvense rhizomes were acquired from naturally occurring populations in Gallatin County and Hill County, Montana during the summer of 2019. Rhizomes were maintained in greenhouse pots and used as the source of rhizome transplants for the study. Pots (25.4 cm diameter x 20.3 cm deep) were sown with 1-gram cuttings of C. arvense rhizome and randomly assigned to a treatment. Rhizomes were planted into a pasteurized soil mixture consisting of equal parts (by volume) of loam soil, washed sand, and Canadian sphagnum peat moss. Pots were watered every two days or as needed, for ten seconds per pot using the shower setting on a conventional garden hose wand. A soluble all-purpose fertilizer (20-20-20 NPK) was added to pots bi-weekly, by mixing 2.5 ml of fertilizer with 3.8 L of water in a watering can, and hand watering for ten seconds per pot. Cirsium arvense was grown for an average of 4.5 months during the first phase (fallow) in all treatments, which was approximately timed with the development of flower buds in all pots. In subsequent phases of each trial, C. arvense was allowed to grow until harvest at the maturity stage of the crop within each crop phase.
Puccinia punctiformis inoculum was collected from naturally occurring populations of infected C. arvense in Gallatin County, Montana and prepared as described by Berner et al. (2013). Systemically infected C. arvense stems were harvested in the autumns of 2020 and 2021, and dried in paper bags in a dark room at ambient temperatures. From the dried stems, leaf tissue containing signs of teliospores were gathered, and ground into a coarse powder inoculum using a household blender. The ground teliospore-bearing inoculum was immediately used or stored for future use in a -80°C freezer. Inoculation methodology followed Berner et al. (2013), where 5 ml of dry rust inoculum was dispersed evenly on the crowns of C. arvense rosettes at the beginning of each phase, for a total of four inoculations per pot in each trial. Cripps et al. (2014) estimated that the concentration of teliospores, using the same methodology, was 1.14x107 teliospores g-1. The inoculated rosettes were misted with deionized water once a day for three days post inoculation to maintain humidity for spore germination. This method was repeated after the harvest of each phase and subsequent regrowth of C. arvense, for a total of four inoculations per pot in each trial.
2.3 Data collection
To address our first question, the density of C. arvense stems with signs of systemic P. punctiformis infection was recorded from each pot at the termination of each crop phase. Cirsium arvense stems were identified as systemically infected when spore structures were observed on leaves and stems. To address our second and third questions, C. arvense and crop stems were counted and cut at soil level at the termination of each crop phase. To obtain dry weight, the harvested biomass was oven dried for 72 hours at ~40.5°C and weighed to the nearest 0.01-gram. After each harvest, pots containing thistle rhizomes were left undisturbed and the next crop phase was seeded into pots assigned to the mixed competition treatment. After the aboveground harvest of final the crop phase (safflower) of each trial, C. arvense rhizome biomass was removed from the soil of each pot, cleaned of soil and residue with cool water, dried for 72 hours at ~40.5°C, and weighed to the nearest 0.01-gram Cirsium arvense pots assigned to the monoculture level of the competition treatment were harvested using the same methodology and at the same time as the mixed pots.
2.4 Data analysis
The probability of observing systemic P. punctiformis infection in pots was calculated at each phase in the crop sequence and was modeled using a generalized linear mixed effects model with a binomial distribution (“glmer” function in the R-Package “lmerTest”; Kuznetsova et al., 2017). The fixed effect in this model was crop phase, and pot ID was included as a random effect to account for repeated observations within each pot over the three trials. Model selection followed a backwards selection from a full model containing all potential explanatory variables using a ‘Drop in Deviance’ test (Ramsey and Schafer, 2012). Model overdispersion was checked by calculating the sum of squared Pearson residuals and comparing it to the residual degrees of freedom, and assumptions homoscedasticity, normality, or influential observations were visually assessed (Ramsey and Schafer, 2012).
The percentage of C. arvense stems with signs of systemic P. punctiformis infection within the inoculated treatment was calculated out of the total density of C. arvense stems per pot and was modeled using a linear mixed effects model (“lmer” function in the R-Package “lmerTest”; Kuznetsova et al., 2017). The fixed effects and random effects in this model were the same as previously described. Explanatory variables were backwards selected from a full model containing all potential explanatory variables (“step” function in the R-Package “lmerTest”; Ramsey and Schafer, 2012). Model assumptions of homoscedasticity, normality, and influential observations were visually assessed (Ramsey and Schafer, 2012).
Differences in C arvense above- and belowground biomass was evaluated using separate linear mixed effects models. In the model for aboveground biomass, the fixed effects were inoculation treatment, competition treatment, and crop phase, with pot ID as a random effect. In the model for belowground biomass, the fixed effects were inoculation treatment and competition treatment, with trial as a random effect to account for repeated observations within each trial. In both models, explanatory terms were selected, and assumptions were checked using methods described previously.
To assess the competitive ability of C. arvense, a relative competition intensity (RCI; Weigelt and Jolliffe, 2003) was used to evaluate the impacts of competition between the P. punctiformis inoculated and non-inoculated (control) treatments was calculated as:
Where “monoculture” was the aboveground biomass of C. arvense from the non-inoculated (control) monoculture treatment, and “mixed” was the aboveground biomass of the mixed pots for either the P. punctiformis inoculated or non-inoculated (control) treatment. RCIcontrol was calculated using aboveground biomass from the control monoculture and mixed pots that were not inoculated with P. punctiformis. RCIinoculated was calculated using aboveground biomass from the non-inoculated (control) monoculture and the aboveground biomass from the mixed pots in the P. punctiformis inoculated treatment. An RCI value ≤ 0 indicates that C. arvense grown in mixed pots produced as much or more aboveground biomass compared to C. arvense grown in a monoculture. In contrast, RCI > 0 indicates that aboveground biomass of C. arvense was reduced when grown in mixed pots, and RCI = 100 indicates that no aboveground C. arvense biomass was produced in the mixed treatment.
The relationship between RCIcontrol and RCIinoculated was evaluated using a linear mixed effects model, with fixed effects of inoculation treatment and crop phase, and pot ID included as a random effect. Model selection was completed by comparing all potential models with an Extra Sums of Squares F-Test. All model assumptions were visually assessed.
3 Results
3.1 Puccinia punctiformis establishment
The overall frequency of P. punctiformis inoculated pots with systemically infected C. arvense stems over the three trials was 52% with no infection observed in the non-inoculated (control) treatment. Systemically infected C. arvense stems were observed in 15% of pots in the fallow phase, 65% of pots in the wheat phase, 60% of pots in the pea phase, and 67% of pots in the safflower phase (F = 14.159; p<0.001; Figure 1A). The percentage of P. punctiformis infected stems in the inoculated treatment, out of all C. arvense stems produced per pot, increased as the crop sequence progressed, with the largest increase occurring after the fallow phase (F = 8.58; p<0.001). The overall mean percentage of P. punctiformis infected stems per pot was 12%. Out of all stems produced per pot, 4% were systemically infected in the fallow phase, 14% were systemically infected in the wheat phase, 16% were systemically infected in the pea phase, and 14% were systemically infected in the safflower phase (Figure 1B).
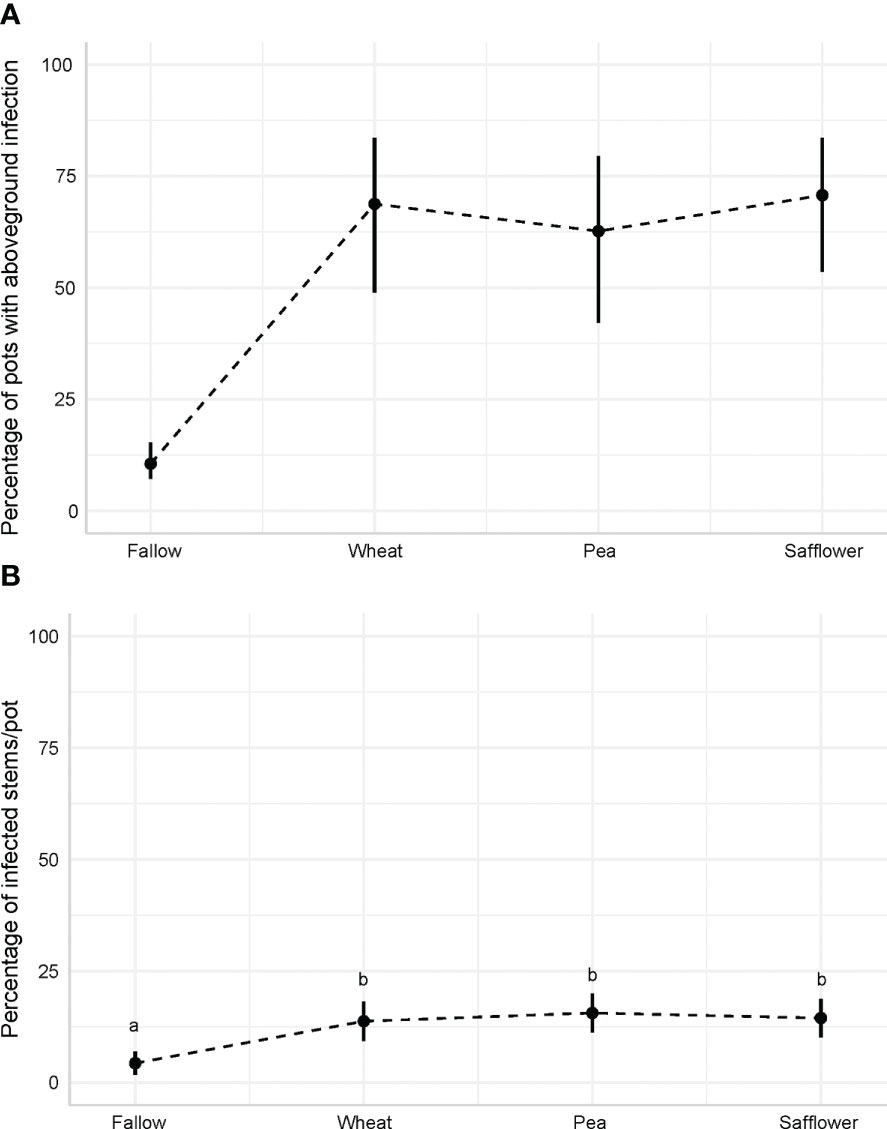
Figure 1 (A) Model predicted percentage of greenhouse pots with signs of systemically infected C arvense stems throughout the simulated crop sequence in the P. punctiformis inoculated treatment. (B) Model predicted percentage of systemically infected stems, out of the total C arvense stems produced per pot, in the P. punctiformis inoculated treatment throughout the simulated crop sequence. Letters (a & b) are representative of the statistical differences in percentage of infected stems/pot between crop phases, where phases that share the same letter are not statistically different.
3.2 Cirsium arvense above-and belowground biomass
Cirsium arvense that was inoculated with P. punctiformis had (± SE) 1.6 (± 0.52) grams/pot less aboveground biomass compared to non-inoculated (control) C. arvense (F = 9.965; p = 0.0020). Cirsium arvense grown with crop competition produced ( ± SE) 3.1 ± 0.52 grams/pot less aboveground biomass than C. arvense grown in monoculture (F = 36.396; p< 0.001). Cirsium arvense biomass in the integrated P. punctiformis inoculated and crop competition treatment was ( ± SE) 4.8 ± 0.74 grams/pot less than C. arvense biomass in the monoculture, non-inoculated treatment (t = 6.506; p< 0.001; Figure 2, Table 1).
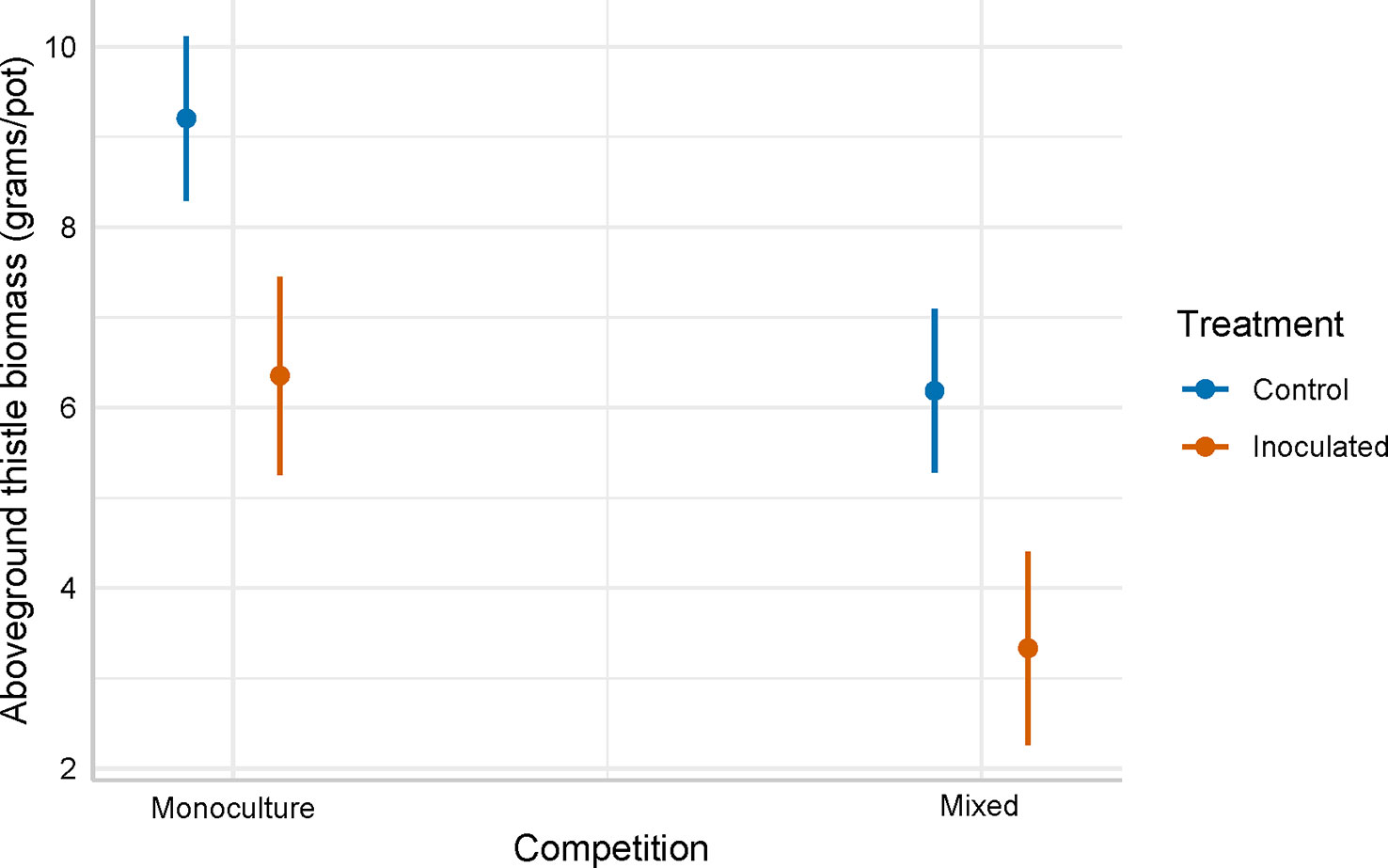
Figure 2 Model predicted aboveground C. arvense biomass (grams/pot) between the inoculated and non-inoculated (control). Inoculated and non-inoculated (control) C. arvense was either grown in a monoculture or grown with interspecific competition where C. arvense was mixed with a sequence of annual crops.

Table 1 ANOVA results for the C. arvense aboveground biomass response to P. punctiformis inoculation treatments (inoculated/non-inoculated) and competition treatments (monoculture/mixed).
C. arvense rhizome biomass was 6.9 grams/pot in the P. punctiformis inoculated treatment and 12.5 grams/pot in the non-inoculated (control) treatment, after an average of 12.9 months of growth. Rhizome biomass in the P. punctiformis inoculated treatment was less than rhizome biomass in the non-inoculated (control) treatment (F = 25.791; p< 0.001). The estimated biomass of C. arvense rhizome in the inoculated treatment was ( ± SE) 5.6 ± 1.1 grams/pot less than in the control treatment. Cirsium arvense grown with crop competition produced ( ± SE) 2.7 ± 1.1 grams/pot less rhizome biomass than C. arvense grown in monoculture (F = 6.211; p-value = 0.0141). Rhizome biomass in the integrated P. punctiformis inoculated and crop competition treatment was ( ± SE) 8.3 ± 1.6 grams/pot less than rhizome biomass in the monoculture, non-inoculated (control) treatment (t = 5.353; p< 0.001; Figure 3, Table 2).
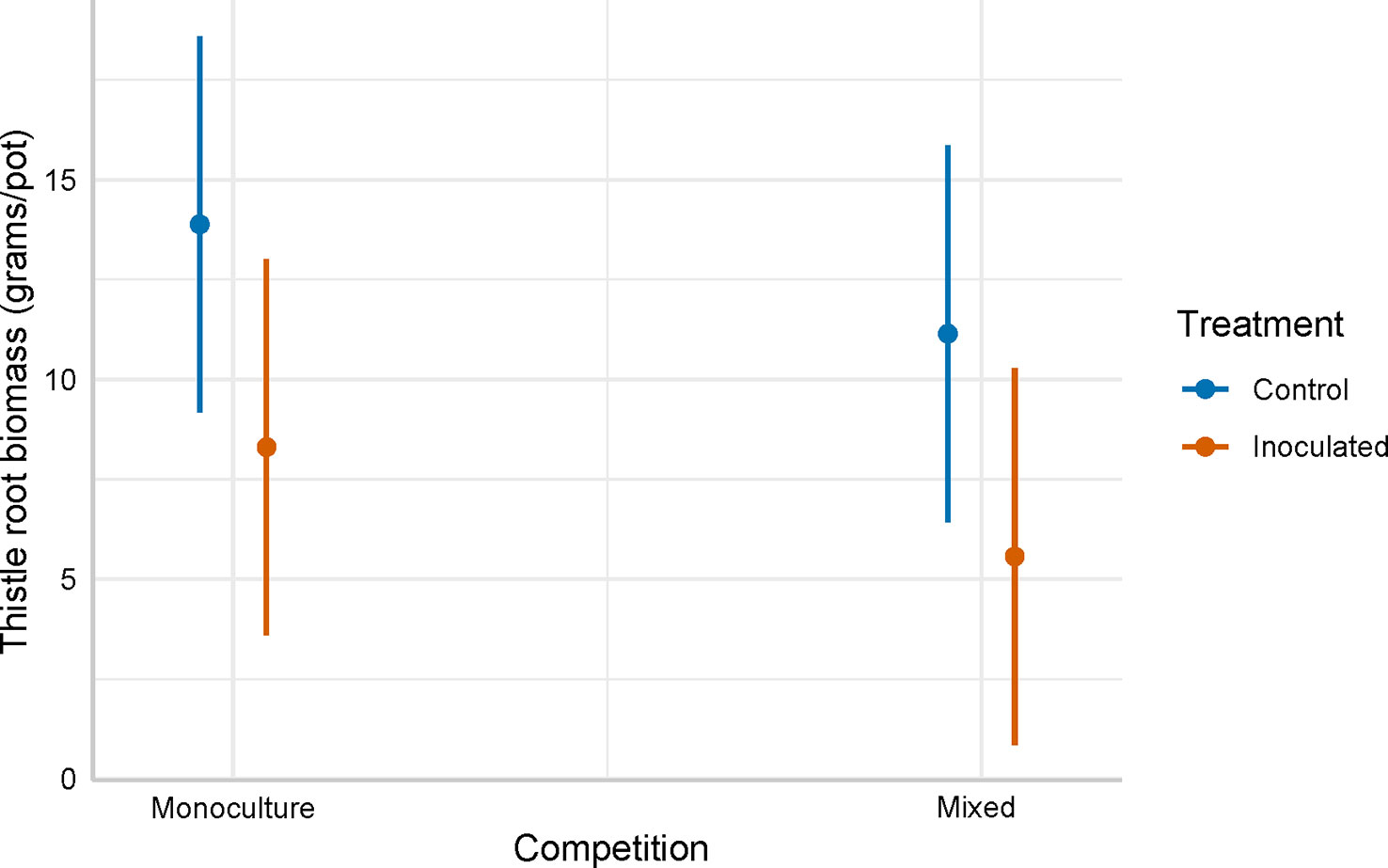
Figure 3 Model predicted belowground C. arvense biomass (grams/pot) between the inoculated and non-inoculated (control). Inoculated and non-inoculated (control) C. arvense was either grown in a monoculture or grown with interspecific competition where C. arvense was mixed with a sequence of annual crops.

Table 2 ANOVA results for the C. arvense root biomass response to P. punctiformis inoculation treatments (inoculated/non-inoculated) and competition treatments (monoculture/mixed).
3.3 Puccinia punctiformis impact on Cirsium arvense competition
Crop competition reduced aboveground biomass, with ( ± SE) 49.2% ± 5.9 biomass loss in the inoculated treatment, and ( ± SE) 39.2% ± 5.9 biomass loss in the non-inoculated (control) treatment, when compared against the monoculture index for growth in the non-inoculated (control) treatment. There was some evidence for a difference in RCI between the inoculated treatment and the non-inoculated (control) (F = 2.816, p-value = 0.0987). The relative competition of C. arvense varied between crop phases (wheat, pea, and safflower) in both the inoculated and control treatments (F = 63.669; p< 0.001). Crop competition reduced aboveground biomass by ( ± SE) 48% ± 5.9 in the wheat phase, ( ± SE) 71% ± 5.9 in the pea phase, and ( ± SE) 14% ± 5.9 in the safflower phase, when compared against the monoculture index for growth in the non-inoculated (control) treatment. Additionally, there was an interaction between the inoculation treatments and crop phases (F = 3.329; p = 0.0393). The RCI between the inoculation treatments increasingly separated as the crop sequence progressed, where the inoculated treatment lost ( ± SE) 24% ± 8.3 more biomass than the non-inoculated (control) treatment by the final safflower phase in the crop sequence (Figure 4, Table 3).
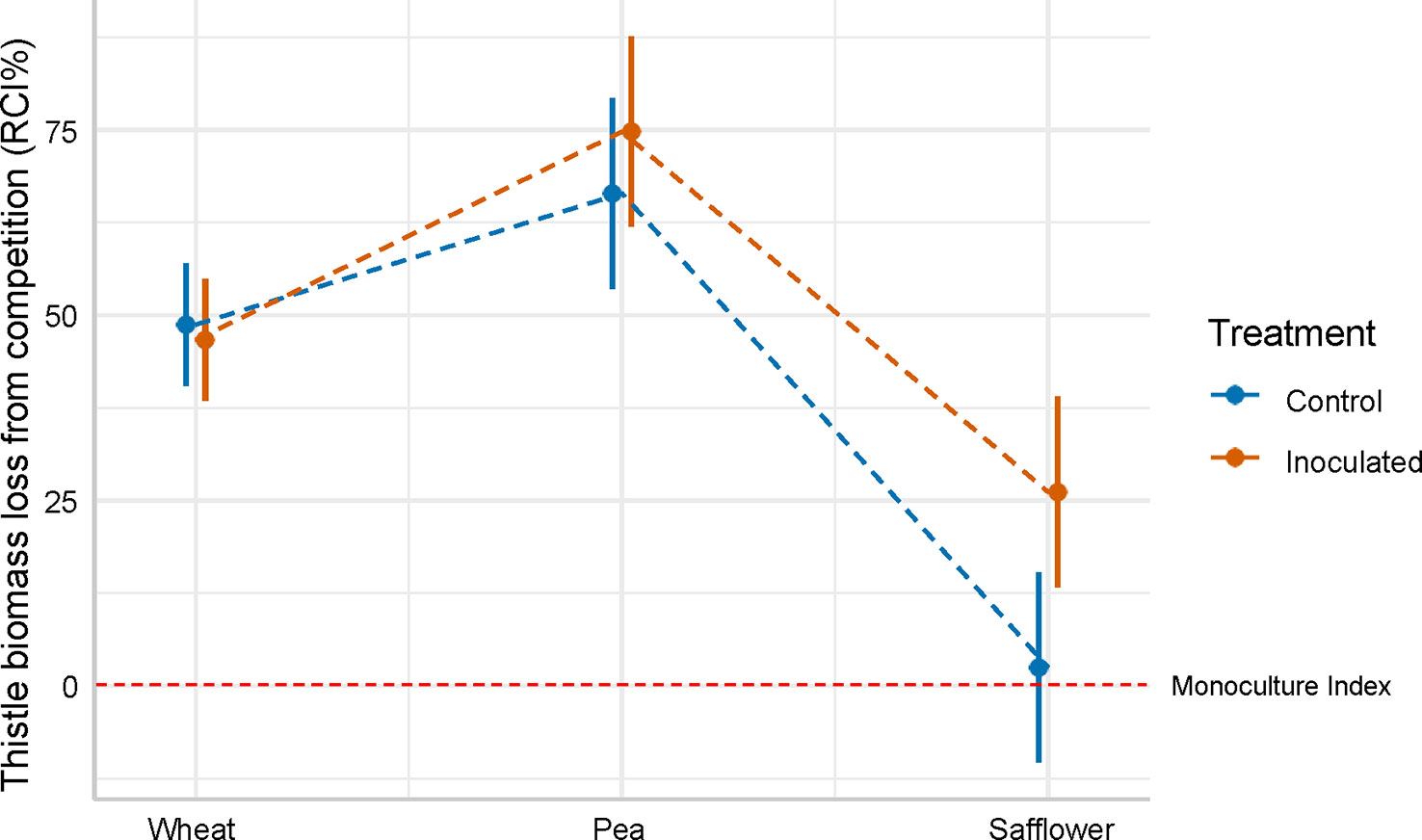
Figure 4 The relationship in aboveground C. arvense biomass loss in competition (RCI%) between the P. punctiformis inoculated and non-inoculated (control) treatments for the three crop phases for all three trials. There was no difference in RCI between the treatments or the crop phases. An RCI value ≤ 0 indicates that C. arvense grown in mixed pots produced as much or more aboveground biomass compared to C. arvense grown in a monoculture. In contrast, RCI > 0 indicates that aboveground biomass of C. arvense was reduced when grown in mixed pots, and RCI = 100 indicates that no aboveground C. arvense biomass was produced in the mixed treatment.

Table 3 ANOVA results for the relative competition intensity (RCI) of C. arvense aboveground biomass response to P. punctiformis inoculation treatments (inoculated/non-inoculated), competitive crop phases (wheat/pea/safflower), and the interaction between inoculation treatments and crop phases.
4 Discussion
Sustainable C. arvense management in organic cropping systems is a primary challenge in temperate regions around the globe. Integrated weed management strategies are needed to reduce the abundance, slow the spread, and minimize the impact of C. arvense in cropping systems over a long term (Liebman et al., 2001; Liebman and Davis, 2009; Davis et al., 2018; Orloff et al., 2018). In this study, we found that the integration of P. punctiformis and crop competition interacted to impact C. arvense biomass and competitive ability. Integrated weed management of C. arvense that combines the P. punctiformis biocontrol with crop competition can reduce C. arvense vigor but requires careful consideration for effective use within complex cropping systems.
Repeated inoculations of C. arvense rosettes with P. punctiformis yielded systemically infected C. arvense stems in all phases of the crop sequence. Inoculation of rosettes resulted in few systemically infected C. arvense stems in the first phase (3-4 months of growth) of the crop sequence, but incidence of infection increased over time. The slow development of systemically infected stems is consistent with the general development of plant pathogens, which often require an incubation period before infected plants develop symptoms (Agrios, 2005). Our findings are also consistent with literature that suggests that P. punctiformis mostly resides asymptomatically within C. arvense rhizomes (Bailiss and Wilson, 1969), especially during the initial stages of infection. In a study testing asymptomatic C. arvense rosettes in proximity to P. punctiformis inoculations, Berner et al. (2015) discovered that up to 60% of asymptomatic rosettes were positive hosts for P. punctiformis. Therefore, the success of our inoculations was likely greater than what was observed aboveground.
While systemically infected stems were observed in most inoculated greenhouse pots, the majority of stems produced were asymptomatic. This supports the conclusion that P. punctiformis is primarily a root pathogen (Berner et al., 2015; Kentjens et al., 2023) that remains latent until adequate resources are gathered from the host and environmental conditions are suitable for the emergence of spore bearing C. arvense stems (Mendgen and Hahn, 2002). The stabilization of infected C. arvense stems after the fallow phase reflects the host’s capacity to support P. punctiformis, given the limitations of plant growth in greenhouse pots. Berner et al. (2015) and Watson and Koegh (1980) suggested that the robustness of infected C. arvense can be a factor that influences the development of systemically infected C. arvense stems, where a robust host is more likely to produce a relatively high abundance of infected stems, and systemic infection in a weaker host could produce fewer infected stems. It was concluded that systemic infection in a less robust host remains mostly asymptomatic and caused death more quickly than systemic infection in a robust host.
Cirsium arvense that was inoculated with the P. punctiformis biocontrol produced less belowground biomass compared to C. arvense that was not inoculated. Our results agree with the findings of Thomas et al.’s (1994) greenhouse experiment, where P. punctiformis inoculated C. arvense produced less root biomass than non-inoculated C. arvense. A weakened root system can directly impact aboveground biomass production, where root resources that would otherwise promote stem growth, are instead allocated to costly defense compounds, or become parasitized by P. punctiformis (Herms and Mattson, 1992; Thomas et al., 1994; Monson et al., 2022). This was demonstrated in our findings, where P. punctiformis inoculations yielded less aboveground biomass compared to C. arvense that was not inoculated, confirming that P. punctiformis inoculations can effectively impact the overall growth of C. arvense.
Competition with annual crops affected C. arvense aboveground growth, although the effects differed between crop species. Unexpectedly, peas were the most competitive annual crop species in the sequence, despite their relatively slow germination, shallow rooting depth, and open canopy (McKay et al., 2003). It is possible that wheat, a moderately competitive cereal species (Mason and Spaner, 2006), had a lasting impact on C. arvense vigor that wasn’t evident until the following pea phase. The weak competitive qualities of peas may have facilitated a recovery in C. arvense vigor, becoming evident in the following phase, where safflower had the lowest relative competition intensity. However, safflower, known to be a weak competitor in the early stages of growth (Emongor and Oagile, 2017), was disadvantaged as the last crop in the sequence. It is possible that greenhouse pots with fully developed roots gave C. arvense a strong competitive advantage by the final phase of the crop sequence; seeding safflower directly into a dense and confined C. arvense root network likely impacted optimal safflower development.
When inoculated C. arvense was grown in mixed pots with interspecific crop competition, the biocontrol interacted additively with crop competition to further reduce above-and belowground biomass, more than individual impacts from the biocontrol or crop competition alone. Although C. arvense was never eradicated by the combination of P. punctiformis and crop competition, there was an interaction between the crop phases and the inoculation treatments, where the difference between the P. punctiformis inoculated and the non-inoculated (control) relative competition intensities gradually increased as the crop sequence progressed. As P. punctiformis inoculations did not immediately affect C. arvense’s competitive ability, but increased through time, the effects appear to be associated with the establishment of infected C. arvense stems. The greatest impact on C. arvense competition emerged after aboveground disease incidence stabilized and persisted through time.
Although we didn’t evaluate physiological responses of C. arvense, there is potential to accelerate disease establishment and increase the severity of P. punctiformis infection by stimulating hormonal responses (Clark et al., 2020), thus enhancing future integrations of the biocontrol. Overall, these results support our hypothesis and provide evidence in favor of integrated weed management as an effective strategy for C. arvense control (Demers et al., 2006; Liebman and Davis, 2009; Sciegienka et al., 2011; Davis et al., 2018; Orloff et al., 2018).
While crop competition is already a common integrated weed management practice (Pavlychenko and Harrington, 1934; Bullock, 1992; Liebman and Dyck, 1993; Liebman and Davis, 2009), there remain practical challenges to the integration of the P. punctiformis biocontrol in field settings. Inoculum sourcing and mass production is limited by the inability to culture transmissible teliospores (Kentjens et al., 2023), creating a reliance on the harvest of teliospore bearing C. arvense. Limitations in inoculum ultimately reduce the scalability of the biocontrol under current sourcing methods. Most natural transmissions of P. punctiformis are limited to 12 meters from the source plant, with no transmissions occurring beyond 17 meters (Berner et al., 2015). Insect vectors or mowing have shown potential to transmit P. punctiformis and increase infection levels across fields (Demers et al., 2006; Wandeler and Bacher, 2006), however, careful cropping system management is required to facilitate effective spore distributions. The greenhouse environment simplifies biocontrol manipulations, but successful integration of P. punctiformis in a field setting will be dependent on variable environmental conditions and cropping system management that can influence survivability and germination of the biocontrol (French and Lightfield, 1990; Berner et al., 2013; Kentjens et al., 2023). Additionally, Thomas et al. (1994) found that P. punctiformis inoculations did not impact aboveground biomass production compared to non-inoculated C. arvense, suggesting inconsistent performance of the pathogen. Inconsistencies in the biocontrol’s impact on C. arvense aboveground growth may be an indication of genetic variability within the host and pathogen populations, where disease severity can be determined by a range of resistance mechanisms in C. arvense or virulence factors in P. punctiformis. Despite inconsistencies and challenges, P. punctiformis has shown potential to increase C. arvense’s vulnerability to integrated weed management tactics, making the biocontrol a viable management option.
5 Conclusion
The fungal biocontrol, P. punctiformis can be successfully integrated with crop competition as a C. arvense management tool. In this greenhouse study, inoculation of C. arvense rosettes with P. punctiformis teliospores caused an increase of symptomatically infected C. arvense stems over time, impacting above- and belowground C. arvense biomass production. Furthermore, P. punctiformis intensified the effects of crop competition when the biocontrol was integrated into a simulated crop sequence. While the use of P. punctiformis is possible in a greenhouse, successful integration of the biocontrol into a field setting will be dependent on a combination of environmental factors and deliberate cropping system management. Puccinia punctiformis is not a singular management solution for C. arvense, however it has potential to be integrated as a low-cost, and low-input biocontrol agent that can improve sustainable management of C. arvense.
Data availability statement
The raw data supporting the conclusions of this article will be made available by the authors, without undue reservation.
Author contributions
DC was the primary author, who conducted the data collection, statistical analysis, and writing. CL assisted with experimental design, statistical analysis, and editing. JE wrote the initial grant proposal and edited the manuscript. FM guided the writing and edited the manuscript. TS supervised data collection, assisted with statistical analysis, guided the writing, and edited the manuscript. All authors contributed to the article and approved the submitted version.
Funding
This work was funded by Western Sustainable Agriculture Research and Agriculture (Grant ID: GW21-218), the USDA National Institute of Food and Agriculture, Organic Agriculture Research and Extension Initiative (Grant ID: 2018-51300-28432), the United States Forest Service Biological Control of Invasive Forest Pests (R1-2021-4), and the Montana Noxious Weed TrustFund (2021-005).
Acknowledgments
We would like to acknowledge Dr Li Huang, professor of genetics and plant pathology at Montana State University, for offering expertise on rust pathogens and for reviewing this manuscript.
Conflict of interest
The authors declare that the research was conducted in the absence of any commercial or financial relationships that could be construed as a potential conflict of interest.
Publisher’s note
All claims expressed in this article are solely those of the authors and do not necessarily represent those of their affiliated organizations, or those of the publisher, the editors and the reviewers. Any product that may be evaluated in this article, or claim that may be made by its manufacturer, is not guaranteed or endorsed by the publisher.
Supplementary material
The Supplementary Material for this article can be found online at: https://www.frontiersin.org/articles/10.3389/fagro.2023.1201600/full#supplementary-material
Supplementary Figure 1 | Canada thistle growth was assessed within three levels of a competition treatment (crop monoculture, thistle monoculture, thistle & crop polyculture) that were nested into two levels of an inoculation treatment (control & thistle rust inoculated). Canada thistle was grown for 16 months in greenhouse pots, and evaluated for density and biomass within a 4-phase diversified crop rotation.
References
Bailiss K. W., Wilson I. M.. (1967). Growth Hormones and the Creeping Thistle Rust. Ann. Bot. 31, 195–211.
Berner D., Smallwood E., Cavin C., Lagopodi A., Kashefi J., Kolomiets T., et al. (2013). Successful establishment of epiphytotics of puccinia punctiformis for biological control of cirsium arvense. Biol. Control 67, 350–360. doi: 10.1016/j.biocontrol.2013.09.010
Berner D. K., Smallwood E. L., Cavin C. A., McMahon M. B., Thomas K. M., Luster D. G., et al. (2015). Asymptomatic systemic disease of Canada thistle (Cirsium arvense) caused by puccinia punctiformis and changes in shoot density following inoculation. Biol. Control 86, 28–35. doi: 10.1016/j.biocontrol.2015.02.006
Buller A. H. R. (1950). Researches on fungi, vol. VII: the sexual process in the uredinales. (Toronto: University of Toronto Press).
Clark A. L., Jahn C. E., Norton A. P. (2020). Initiating plant herbivory response increases impact of fungal pathogens on a clonal thistle. Biol. Control 143, 104207. doi: 10.1016/j.biocontrol.2020.104207
Cripps M., Bourdôt G., Saville D. J., Berner D. K. (2014). “Success with the rust pathogen, Puccinia punctiformis, for biological control of Cirsium arvense,” in XIV International Symposium on Biological Control of Weeds. ed. Impson F. A. C., Kleinjan C. A., Hoffman J. H. (South Africa: University of Cape Town), 2–7.
Davis S., Mangold J., Menalled F., Orloff N., Miller Z., Lehnhoff E. (2018). A meta-analysis of Canada thistle (Cirsium arvense) management. Weed Sci. 66, 548–557. doi: 10.1017/wsc.2018.6
Demers A. M., Berner D. K., Backman P. A. (2006). Enhancing incidence of puccinia punctiformis, through mowing, to improve management of Canada thistle (Cirsium arvense). Biol. Control 39, 481–488. doi: 10.1016/j.biocontrol.2006.06.014
Emongor V., Oagile O. (2017). Safflower production (Gaborone: Botswana University of Agriculture and Natural ResourcesI).
French R. C. (1990). Stimulation of germination of teliospores of puccinia punctiformis by nonyl, decyl, and dodecyl isothiocyanates and related volatile compounds. J. Agric. Food Chem. 38, 1604–1607. doi: 10.1021/jf00097a037
French R. C., Lightfield A. R. (1990). Induction of systemic aecial infection in Canada thistle (Cirsium arvense) by teliospores of puccinia punctiformis. Phytopathology 80, 872–877. doi: 10.1094/Phyto-80-872
Herms D. A., Mattson W. J. (1992). The dilemma of plants: to grow or defend. Quarterly Rev. Bio. 67, 283–335.
Kentjens W., Casonato S., Kaiser C. (2023). Californian Thistle (Cirsium arvense): endophytes and puccinia punctiformis. Pest Manage. Sci. doi: 10.1002/ps.7387
Kuznetsova A., Brockhoff P. B., Christensen R. H. B. (2017). lmerTest package: tests in linear mixed effects models. J. Stat. Soft 82, 1–26. doi: 10.18637/jss.v082.i13
Lehnhoff E., Miller Z., Miller P., Johnson S., Scott T., Hatfield P., et al (2017). Organic agriculture and the quest for the Holy Grail in water-limited ecosystems: managing weeds and reducing tillage intensity. Agriculture 7, 33.
Liebman M., Davis A. S. (2009). “Managing weeds in organic farming systems: an ecological approach,” in Organic farming: the ecological system agronomy monographs ed. Francis C. (USA: American Sopciety of Agronomy, Inc., Crop Science Society of America, Inc., Soil Society of America, Inc.), 173–195. doi: 10.2134/agronmonogr54.c8
Liebman M., Dyck E. (1993). Crop rotation and intercropping strategies for weed management. Ecol. Appl. 3, 92–122. doi: 10.2307/1941795
Liebman M., Mohler C. L., Staver C. P. (2001). Ecological management of agricultural weeds (Cambridge: Cambridge University Press). doi: 10.1017/CBO9780511541810
Mason H. E., Spaner D. (2006). Competitive ability of wheat in conventional and organic management systems: a review of the literature. Can. J. Plant Sci. 86, 333–343. doi: 10.4141/P05-051
Mendgen K., Hahn M. (2002). Plant infection and the establishment of fungal biotrophy. Trends Plant Sci. 7, 352–356. doi: 10.1016/S1360-1385(02)02297-5
Menzies B. P. (1953). Studies on the systemic fungus, puccinia suaveolens. Ann. Bot. 17, 551–568. doi: 10.1093/oxfordjournals.aob.a083369
Monson R. K., Trowbridge A. M., Lindroth R. L., Lerdau M. T. (2022). Coordinated resource allocation to plant growth–defense tradeoffs. New Phytol. 233, 1051–1066. doi: 10.1111/nph.17773
Moore R. J. (1975). The biology of Canadian weeds.: 13. cirsium arvense (L.) scop. Can. J. Plant Sci. 55, 1033–1048. doi: 10.4141/cjps75-163
Orloff N., Mangold J., Miller Z., Menalled F. (2018). A meta-analysis of field bindweed (Convolvulus arvensis l.) and Canada thistle (Cirsium arvense l.) management in organic agricultural systems. Agriculture Ecosyst. Environ. 254, 264–272. doi: 10.1016/j.agee.2017.11.024
Pavlychenko T. K., Harrington J. B. (1934). Competitive efficiency of weeds and cereal crops. Can. J. Res. 10, 77–94. doi: 10.1139/cjr34-006
Ramsey F., Schafer D. (2012) The statistical sleuth: a course in methods of data analysis (Accessed February 1, 2023).
Sciegienka J. K., Keren E. N., Menalled F. D. (2011). Interactions between two biological control agents and an herbicide for Canada thistle (Cirsium arvense) suppression. Invasive Plant Sci. Manage. 4, 151–158. doi: 10.1614/IPSM-D-10-00061.1
Tautges N. E., Goldberger J. R., Burke I. C. (2016). A survey of weed management in organic small grains and forage systems in the Northwest united states. Weed Sci. 64, 513–522. doi: 10.1614/WS-D-15-00186.1
Thomas R. F., Tworkoski T. J., French R. C., Leather G. R. (1994). Puccinia punctiformis affects growth and reproduction of Canada thistle (Cirsium arvense). Weed Technol. 8, 488–493. doi: 10.1017/S0890037X00039567
Tiley G. E. D. (2010). Biological flora of the British isles: cirsium arvense (L.) scop. J. Ecol. 98, 938–983. doi: 10.1111/j.1365-2745.2010.01678.x
Wandeler H., Bacher S. (2006). Insect-transmitted urediniospores of the rust puccinia punctiformis cause systemic infections in established cirsium arvense plants. Phytopathology® 96, 813–818. doi: 10.1094/PHYTO-96-0813
Watson A. K., Koegh W. J. (1980). “Mortality of Canada thistle due to puccinia punctiformis,” in International Symposium on Biological Control of Weeds. (Brisbane, Australia: Commonwealth Scientific and Industrial Research Organization), 325–332.
Weigelt A., Jolliffe P. (2003). Indices of plant competition. J. Ecol. 91, 707–720. doi: 10.1046/j.1365-2745.2003.00805.x
Keywords: crop competition, Puccinia punctiformis, agriculture, integrated weed management (IWM), Canada thistle, thistle rust, biocontrol
Citation: Chichinsky D, Larson C, Eberly J, Menalled FD and Seipel T (2023) Impact of Puccinia punctiformis on Cirsium arvense performance in a simulated crop sequence. Front. Agron. 5:1201600. doi: 10.3389/fagro.2023.1201600
Received: 06 April 2023; Accepted: 28 April 2023;
Published: 12 May 2023.
Edited by:
Simerjeet Kaur, Punjab Agricultural University, IndiaReviewed by:
Ioannis Roussis, Agricultural University of Athens, GreeceHossein Ghanizadeh, Massey University, New Zealand
Copyright © 2023 Chichinsky, Larson, Eberly, Menalled and Seipel. This is an open-access article distributed under the terms of the Creative Commons Attribution License (CC BY). The use, distribution or reproduction in other forums is permitted, provided the original author(s) and the copyright owner(s) are credited and that the original publication in this journal is cited, in accordance with accepted academic practice. No use, distribution or reproduction is permitted which does not comply with these terms.
*Correspondence: Daniel Chichinsky, ZGNoaWNoaW5za3lAZ21haWwuY29t