- Department of Plant and Environmental Sciences, Faculty of Science, University of Copenhagen, Taastrup, Denmark
With challenges posed by chemical and mechanical weed control, there are now several research and commercial projects underway to develop autonomous vehicles equipped with lasers to control weeds in field crops. Recognition systems based on artificial intelligence have been developed to locate and identify small weed seedlings, and mirrors can direct a laser beam towards the target to kill the weed with heat. Unlike chemical and mechanical weed control, laser weeding only exposes a small area of the field for the treatment. Laser weeding leaves no chemicals in the field after the treatment or does not move the soil which may harm crop roots and non-target organisms. Yet, it is well-known that laser beams can harm living organisms; the effect on the environment and fauna should be studied before laser weeding becomes a common practice. This project aimed to study the effect of laser on some living non-target organisms. We investigated the effect of laser treatment on the mortality of two species of earthworms (Enchytraeus albidus and Enchytraeus crypticus), larvae, pupas, and beetles of yellow mealworm beetles (Tenebrio molitor) and the two-spotted lady beetle (Adalia bipunctata) for increasing dosages of laser energy. In all earthworms experiments except one, the mortality rates of the worms living in the uppermost soil layer of clay, sandy, and organic soil exposed to laser heating were not significantly different from the controls even with laser dosages up to 23.8 J mm-2. Laser doses sufficient to kill plants were lethal to the insects, and lower doses that did not kill plants, killed or harmed the insects across all life stages tested. The larger beetles survived higher doses than smaller. Laser weeding is a relatively new technology and not yet widely practiced or commercialized. Therefore, we do not discuss and compare the costs of the different weeding methods at this early stage of the development of the technology.
1 Introduction
Weed control with laser beams has achieved increasing attention as the fast development in artificial intelligence has enabled recognition of the location and identification of plant species precisely and rapidly (Rakhmatulin et al., 2021). Furthermore, laser can be guided by mirrors to target weeds (Rakhmatulin and Andreasen, 2020). When small weeds are hit correctly in the meristem, the heat from the laser can kill the plants (Heisel et al., 2001; Coleman et al., 2021). Lasers are powered by electricity, which can be supplied by batteries, charged from renewable (non-fossil) energy sources reducing CO2 emission compared to commonly used weed control methods. Suppose a laser beam has a diameter of 2 mm and there are 150 weeds m-2, then only 0.5% of the total area will be exposed to the treatment. A common practice of herbicide application and mechanical weed control exposes most of the field or the whole area to the treatments, and chemicals often stay on the soil surface or in the soil matrix for a while with the risk of affecting non-target organisms and the environment negatively (Thiour-Mauprivez et al., 2019; Mehdizadeh et al., 2021). Consequently, replacing herbicide application and mechanical weed control with laser weeding seems to be a method to reducing some of the negative environmental impact of weed control.
If the laser beam hits non-target organisms, they are likely harmed or killed. That may happen, for example, if insects or other organisms (1) are on the target plant at time of exposure, (2) move into the laser beam, or (3) if unintended platform movement results in the inaccurate position of the laser energy.
The effect of the laser beam on the weed plants depends on physical parameters (e.g., laser wavelength, beam diameter, and dose (J)) (Rakhmatulin and Andreasen, 2020) and biological factors (e.g., plant species, plant size, and developmental stage) (Heisel et al., 2001; Andreasen et al., 2022). These factors may also be crucial for the effect of laser beams on non-target organisms and should be investigated.
It is not economically feasible to study the effect of laser on all potentially exposed organisms, and therefore model organisms are often used (Eggen et al., 2004). We examined how laser treatment affected the survival rate of two earthworms and two insects.
Enchytraeids (class Oligochaeta, family Enchytraeidae) are ecologically important soil worms due to their activity in bioturbation and decomposition of organic matter in many soil types (Didden, 1993; Castro-Ferreira et al., 2012). Enchytraeids are widespread on moist soil types from the arctic to the tropics, occurring in quantities from 100s to more than 100,000 individuals m-2. Generally, they thrive within a temperature range of 8 °C–25 °C. Enchytraeids are often used as model organisms in toxicological laboratory tests (e.g., Cedergreen et al., 2013; Gomes et al., 2013; He and van Gestel, 2013). Enchytraeus albidus and E. crypticus are white worms with a long thin body with a soft skin and a fast reproduction rate. They can grow up to 3 cm long. They have been considered suitable to assess ecotoxicity in many different soil types due to their larger tolerance range to pH (4.4–8.2), clay (1–29%) and content of organic matter in the soil (1.2–42%) (Kuperman et al., 2006; Castro-Ferreira et al., 2012).
Tenebrio molitor (Coleoptera: Tenebrionidae) is a holometabolous insect (complete life cycle with egg, larva, pupa, and adult stages) and is considered a pest due to its ability to consume stored flour, grains, or animal feeds. The larvae are called mealworms. The larva is white and reaches 2−2.5 cm in length. They gradually become yellow and then darker brown. They have three pairs of legs and are active crawlers. The T. molitor beetle reach 25 mm in length and are the largest insects infesting stored products (Davidson and Lyon, 1979). Tenebrio molitor has often been used as a test insect in ecotoxicological studies as it is easy to propagate, feed, and keep indoors (e.g., McCallum et al., 2013; Lv et al. 2014; Bednarska and Świątek, 2016; Fei et al., 2022).
Adalia bipunctata (Coleoptera: Coccinellidae) is an aphidophagous beetle also called two- spotted ladybug/two-spotted ladybird/two-spotted lady beetle. It is native to North America and Western and Central Europe (Hodek et al., 2012). The larvae and adults feed on aphids and other small insects, so A. bipunctata is therefore considered a beneficial insect. It has been commercialized for aphid pest control in protected environments (Wyss et al., 1999; Khan et al., 2016). Adults reach a length of 3.5−5.2 mm (Gordon, 1985).
The aim of this study was to investigate how a fiber laser with a wavelength of 2 µm and a 2 mm diameter affected the mortality of two species of soil worms (E. albidus and E. crypticus) when the soil surface was exposed to increasing doses of laser energy. The 2 µm wavelength from the fiber laser is mainly absorbed by the water inside the target and is more beneficial for weed control than a CO2 laser, which energy is primarily absorbed on the surface of the plant (Wieliczka et al., 1989). Therefore, a thulium-doped 2 µm fiber laser has been installed in the autonomous vehicle for laser weeding developed in the EU project WeLASER (https://welaser-project.eu/). A laser energy dose of 15 J mm-2 may be used to control seedlings of weeds in agricultural and horticultural fields. Weed plants on the cotyledons and two permanent leaf stages are usually killed when they are exposed to 10 J mm-2 (Heisel et al., 2002; Andreasen et al., 2022). We also exposed larvae, pupae, and beetles of T. molitor and the A. bipunctata beetle to increasing doses of laser energy. We hypothesized that all organisms would be negatively affected if they were exposed to an energy level of 8−24 J mm-2) which may be used to control dicotyledon and monocotyledon weeds at the early stages of development (Coleman et al., 2021; Andreasen et al., 2022).
2 Materials and methods
2.1 Laser equipment
We used a thulium-doped 50 W fiber laser with a wavelength of 2 µm with a collimated beam (Ø: 2 mm) manufactured by Futonics Laser GmbH, Katlenburg-Lindau, Germany. The laser was placed within a steel box (68 cm × 68 cm × 68 cm) with a door with a metal interlock (Figure 1). On laser activation, the door locks automatically to avoid risk of laser exposure.
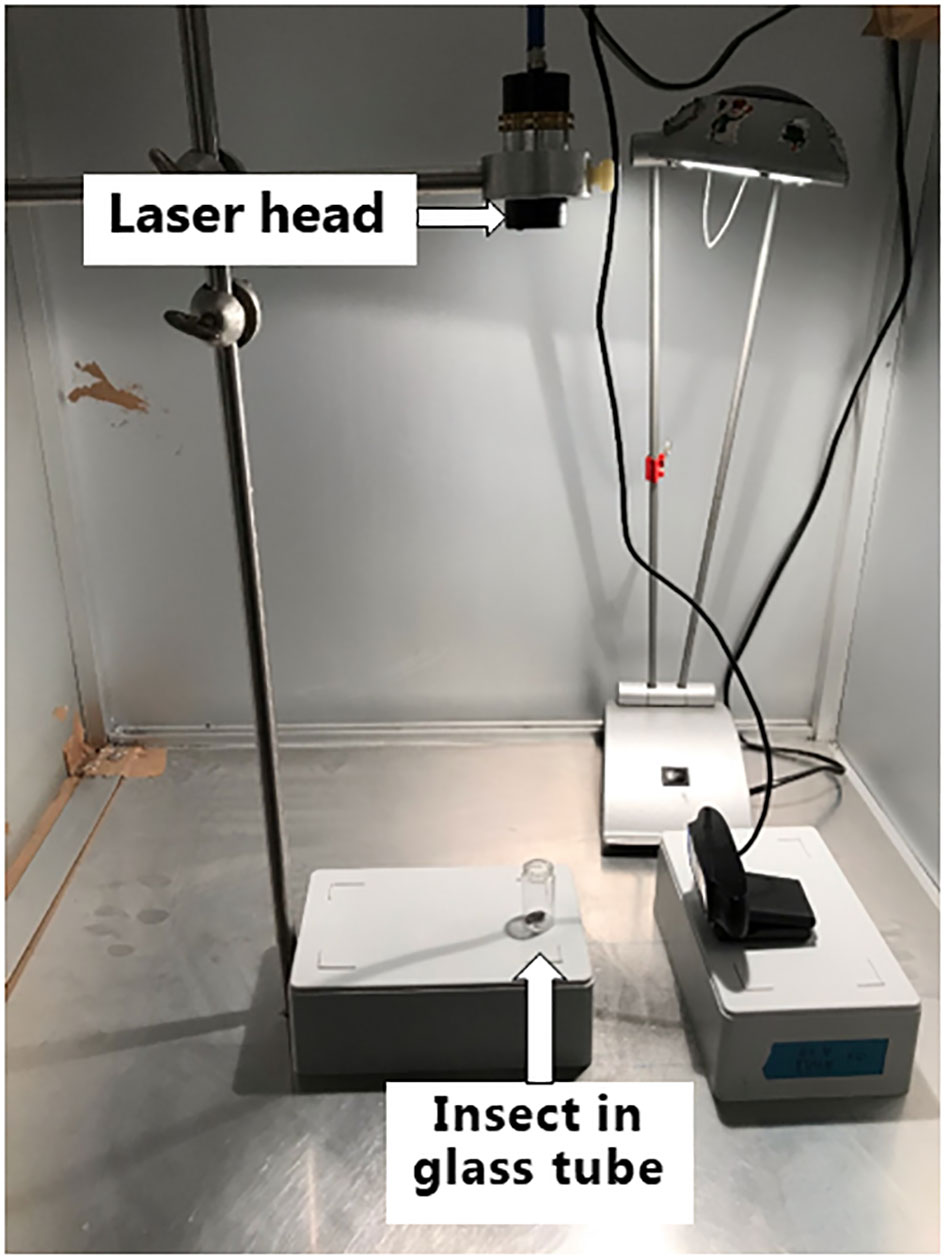
Figure 1 The laser was placed within a steel box (68 cm × 68 cm × 68 cm) with a door with a metal interlock. The organisms were placed below the laser head.
The target organisms were placed approximately 40 cm below the laser head and exposed to increasing dosages of laser energy (from 0 to 23.9 J mm-2)
2.2 Laser experiments with Enchytraeus spp.
2.2.1 Culturing conditions of Enchytraeus spp.
Enchytraeus albidus and E. crypticus were cultured in soil in plastic buckets with lids (length: 13.5 cm; Ø: 13 cm). Three soil types were used: a) sandy soil containing about 9% clay, 10% silt, 32% fine sand, 47% coarse sand, and 2% organic matter, b) clay soil containing about 28% clay, 23% silt, 10% fine sand, 36% coarse sand, and 3% organic matter, and c) an organic soil based on sphagnum (Pindstrup mixture 2 (https://www.pindstrup.dk/professionel/product-details/pindstrup-f%C3%A6rdigblanding-2), Pindstrup mosebrug a/s, Ryomgaard, Denmark). There was one bucket for each soil type and worm species (n=6). The buckets were weighed after adding soil and water, and afterward once a week to check the soil moisture. Water loss was replenished by adding an appropriate amount of deionized water. The buckets were placed in the dark in a climate cabinet at 20°C ± 2°C. The soil was kept moist but not wet corresponding to 40−60% of the water-holding capacity. Four holes (2 mm) were made in the lids of the buckets to allow adequate gaseous exchange with the atmosphere. The soil was cautiously broken up with a spatula each week to facilitate aeration. The worms were fed with rolled oats weekly. The rolled oats were ground and autoclaved (121°C, 105 min, 1200 mbar) before use to avoid infestation with flour mites (e.g., Glyzyphagus sp. and Astigmata, Acarina) or predacious mites [e.g., Hypoaspis scimitus (Cosmolaelaps) and Gamasida (Acarina)]. If the oat became contaminated with fungi, it was removed and replaced.
2.2.2 Experiments with Enchytraeus albidus and E. crypticus
To study the effect of the laser on the earthworms, 10 g of dry soil was moistened with demineralized water to achieve approximately 50% of the water-holding capacity. The moist soil was placed in a 50 ml plastic tube (length: 11.2 cm; Ø: 30 mm), with 10 holes ((Ø: 1 mm) in the lid to ensure gas exchange.
After the soil was placed in the tubes three worms were transfer to each tube and kept for one day in a climate cabinet in darkness at 20°C ± 2°C before laser treatment. For each soil type and laser doses (0 (control), 0.5, 1, and 1.5 seconds corresponding to 0, 8.0, 15.9, and 23.9 J m-2), 10 tubes with three E. albidus worms and 10 tubes with three E. crypticus worms were used. Three soil types were chosen because the heat transfer from the laser depends on the soil textures. The lid of the tubes was moved before the soil surface in the tubes was exposed to the laser beam from above. After the treatment, the tubes were placed with the lids in a climate cabinet in darkness at 20°C ± 2°C. The number of immobile, live, dead, or missing enchytraeids in all treatments were recorded by empty the tubes and searching through the soil with a spatula 7 days after the treatment. Worms could be missing because they were dead, decomposed and dissolved during the period. The experiment was repeated four times with E. albidus, using the same worms, as they were not affected by the treatment, but only done once with E. crypticus due the lack of worms.
2.3 Experiments with Tenebrio molitor
Tenebrio molitor were bought from the company InsektOrama A/S, Herning, Denmark. Adults and half of the delivered larvae were used for the first experiment and lasered two days after the delivery. The rest of the larvae were reared to produce an adequate number of new adults, larvae (to repeat the experiment) and pupae (for the first experiment and its repetition). When larvae were developed, they were moved to a round open plastic tray (10 cm high; Ø: 29 cm) and placed in a climate cabinet (at 20°C ± 2°C in darkness). The bottom of the tray was covered with rolled oats (3 cm high) necessary for the larvae’s rearing. Additional oats were added when necessary to ensure a continuous supply of feed. Four to five slices of fresh potato (Ø: 3−4 cm) were added to the tray every second day to ensure water supply. The tray was checked daily, and as soon as a pupa was observed, it was immediately separated from the larvae and placed in a new, similar tray. Adults were isolated from pupae in the same way to avoid cannibalism. Adults were producing eggs, creating a new generation of larvae required to repeat the experiment. All insect stages were kept and reared under the same conditions until they were lasered.
Ten individuals each of larvae, pupae, and adult T. molitor were exposed to the laser beam for 0, 1, 10, 20, 50, 100, and 500 ms corresponding to 0, 0.016, 0.16, 0.32, 0.80, 1.59, and 7.95 J mm-2. Ten individuals of each developmental stage of Tenebrio molitor were treated with each dose with 4 replicates (10 × 4 individuals). The larvae were retained in a groove in a small piece of wood during the treatment. The pupae were placed on a piece of paper, and the adults were placed in a glass tube (length: 40 mm; Ø: 18 mm) to prevent them escaping the treatment (Figure 1). The laser was aimed at the dorsal surface, approximately halfway along the length of the body, but because they were alive and able to move some were hit in other locations. Afterwards, the insects were carefully moved to four plastic transparent boxes with lids (12 cm × 12 cm × 4 cm) (10 individuals per box) immediately after lasering. The lids were partially (1/3 of the lid) covered by a net (holes: 1 mm × 1 mm) ensuring gas exchanges.
The bottom of the boxes was covered with approximately 1 cm of rolled oats and slices of fresh potato (Ø: 3−4 cm) was added as feed and water supply. Additional rolled oats and fresh potato was added when necessary to ensure a continuous supply of feed and water. The number of live and dead individuals were counted 8 and 15 days after the laser treatment. Damages and deformities were noted.
2.4 Experiments with Adalia bipunctata
Adalia bipunctata, produced by EWH BioProduction ApS, Tappernøje, Denmark was bought via Horticoop Scandinavia A/S, Hinnerup, 143 Denmark. The beetles were kept in transparent plastic containers (28 cm × 20 cm × 22 cm) with lids, partially (2/3) covered by a net (holes: 1 mm × 1mm) allowing gas exchange, and kept in a climate cabinet at 21°C with 12 hours light from 8 a.m. to 8 p.m. The beetles were fed with honey diluted with water (ratio 1:10) during the experimental periods. The diluted honey was supplied via pieces of filter papers (3 cm × 3 cm). Four to five new papers were placed in the box with the beetles every day. The beetles were kept and reared in the same conditions until being lasered.
For each laser treatment, ten beetles were exposed to a laser dose. Each beetle was placed in a plastic tube (length: 50 mm; Ø: 5 mm) during the irradiation preventing the beetle from escaping. We aspired to hit the beetle in the center of their dorsal surface, but they were able to move a little and therefore some were hit in other places. The laser dosages were 0, 1, 10, 20, 50, 100, and 500 ms corresponding to 0, 0.016, 0.16, 0.32, 0.80, 1.59, and 7.95 J mm-2. After exposure, each beetle was moved to a plastic container together with the nine other beetles receiving the same dose and kept exactly the same way as the pretreated adults. The mortality rate was recorded over 15 days. There were four replicates for each dose (total 4 ×10 beetles). Two independent experiments were done.
2.5 Statistical analyses
Statistical analyses were done using the statistical program R´ (R Core Team, 2021). All data sets were initially analyzed using a dose-response model. If no dose-response trend was present in the data, the lowest observed adverse effect level (LOAEL) and the no observed adverse effect level (NOAEL) were found as the lowest dose showing a significant effect compared to the control group and the highest dose with a non-significant effect compared to the control group, respectively.
2.5.1 Enchytraeus albidus and E. crypticus
Since no clear dose-response trend were observed for mortality of Enchytraeus albidus and E. crypticus, data were analyzed with a logistic regression model with laser dose as factorial explanatory variable. For E. albidus exposed in clay and sandy soil with four repetitions, a logistic mixed model was used instead. For these models, laser dose was included as a factorial fixed effect and repetition was included as random effect. Pairwise comparisons to the control group to find NOAEL and LOAEL were based on the fitted models (Hothorn et al., 2008).
2.5.2 Tenebrio molitor
A non-linear dose-response model for binary data was used to describe the association between laser dose and mortality of the individual life stages. A three-parameter log-logistic model assuming an upper limit of 100% mortality was fitted to the data from each repetition and for 8 and 15 days after treatment, individually. The effective dose (EDx) killing a percentage, x, of the individuals remaining after adjustment for background mortality was estimated from each individual model and combined in a meta-analytic linear mixed model for each ED20 and ED80, separately. Each model included the day of observation as fixed effect and repetition as random effect. For plotting, parameters from each model fit were combined in a second step using a meta-analytic linear mixed model (Jensen et al., 2020). The model included the two-way interaction of day of observation and model parameters as fixed effect, repetition as random effect with corresponding standard deviations that were assumed different for each of the three model parameters, and an unstructured variance-covariance matrix. Pairwise comparisons of parameters and ED-values between days of observation were based on the estimated meta-analytic models as post hoc pairwise comparisons (Hothorn et al., 2008).
2.5.3 Adalia bipunctata
Data for Adalia bipunctata were analyzed in a similar way as data for Tenebrio molitor but for five different time points. For day 1 and day 4, there were no background mortality and accordingly a two-parameter log-logistic model was used. For day 8, 11, and 15, a three-parameter log-logistic model was used.
3 Results
3.1 Enchytraeus albidus and E. crypticus
In all earthworms experiments except one, the mortality rates of the worms living in clay, sandy, and organic soil exposed to laser heating were not significantly different from the controls (Supplementary Table S1). Consequently, the NOAEL was the highest dose of 23.9 J mm-2 and the LOAEL could not be estimated. For E. crypticus, the highest laser dose of 23.9 J mm-2 was the only dose significantly higher than the control (p=0.0185), and accordingly the LOAEL, making 15.9 J mm-2 the NOEAL (Supplementary Table S1).
3.2 Tenebrio molitor
3.2.1 Larvae
In both experiments, the dose influenced the mortality of the larvae. A non-linear function fitted the data well (Figure 2). Model parameters and ED20, ED50, and ED80 values are shown in Supplementary Table S2.
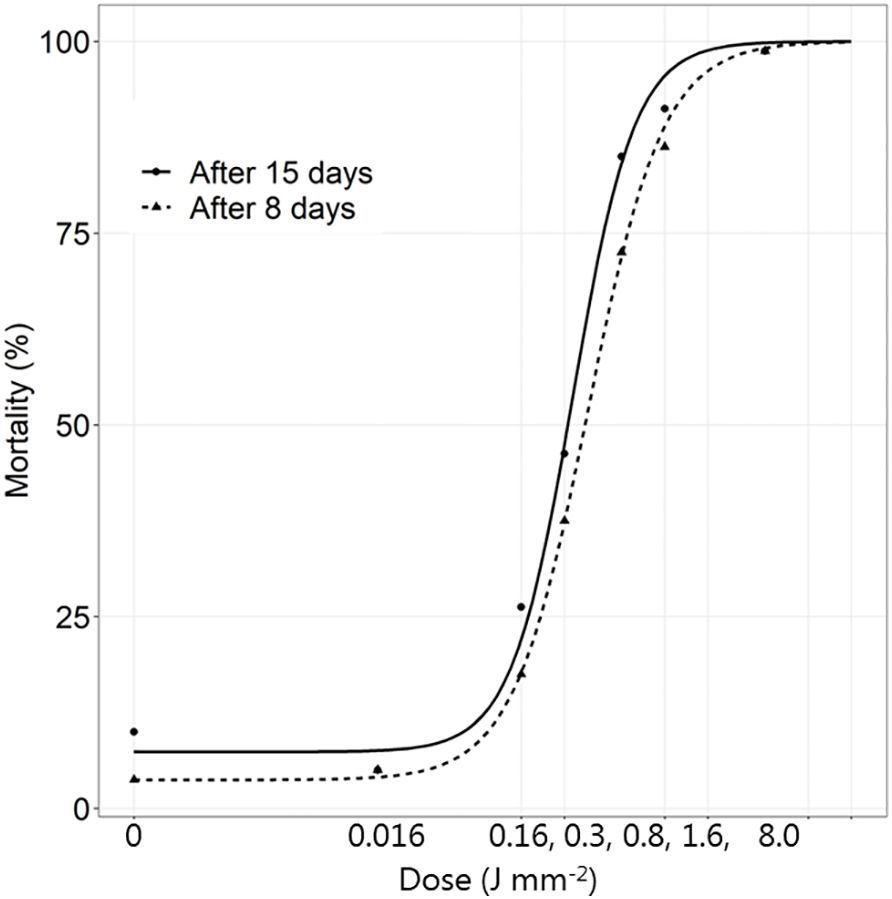
Figure 2 The mortality (%) of Tenebrio molitor larvae 8 and 15 days after exposure to increasing dosages of laser energy (J) from a thulium-doped 2 µm 50 W fiber laser with a collimated beam with a diameter of 2 mm. Points show mean values (n=20).
The mortality after 8 days did not change significantly. The larvae from the control group (0 J mm-2) developed into normal pupae and beetles. At the smallest dose (0.016 J mm-2) some larvae and pupae developed normally, but some developed into beetles with wing deformities. When the dose was increased to 0.32 J mm-2, most of the insects (all stages) were living, but the living larvae received a spot burn from the laser treatment while living adults had deformed wings. The dead insects became brown, dark or with a big dark spot from the laser. The living larvae developed into deformed beetles that almost immediately died. At a dose of 0.80 J mm-2, more than half of the larvae died. Most of the dead insects were at the larva stage and very few could complete metamorphosis resulting in severely deformed insects, and in some cases, they became half pupa and half beetle. Most of the dead larvae were dark and brown, and the few living ones received a dark spot burn from the laser (Figure 3).
Very few larvae survived a dose of 1.59 J mm-2. Most larvae died having a dark dehydrated and burned appearance. At a dose of 7.95 J mm-2, almost all the larvae died the first week after application at larva stage. Only one transformed into a pupa. The dead larvae became completely black or brown with the hemolymph running out from the burned hole in their body just after treatment (Figure 3).
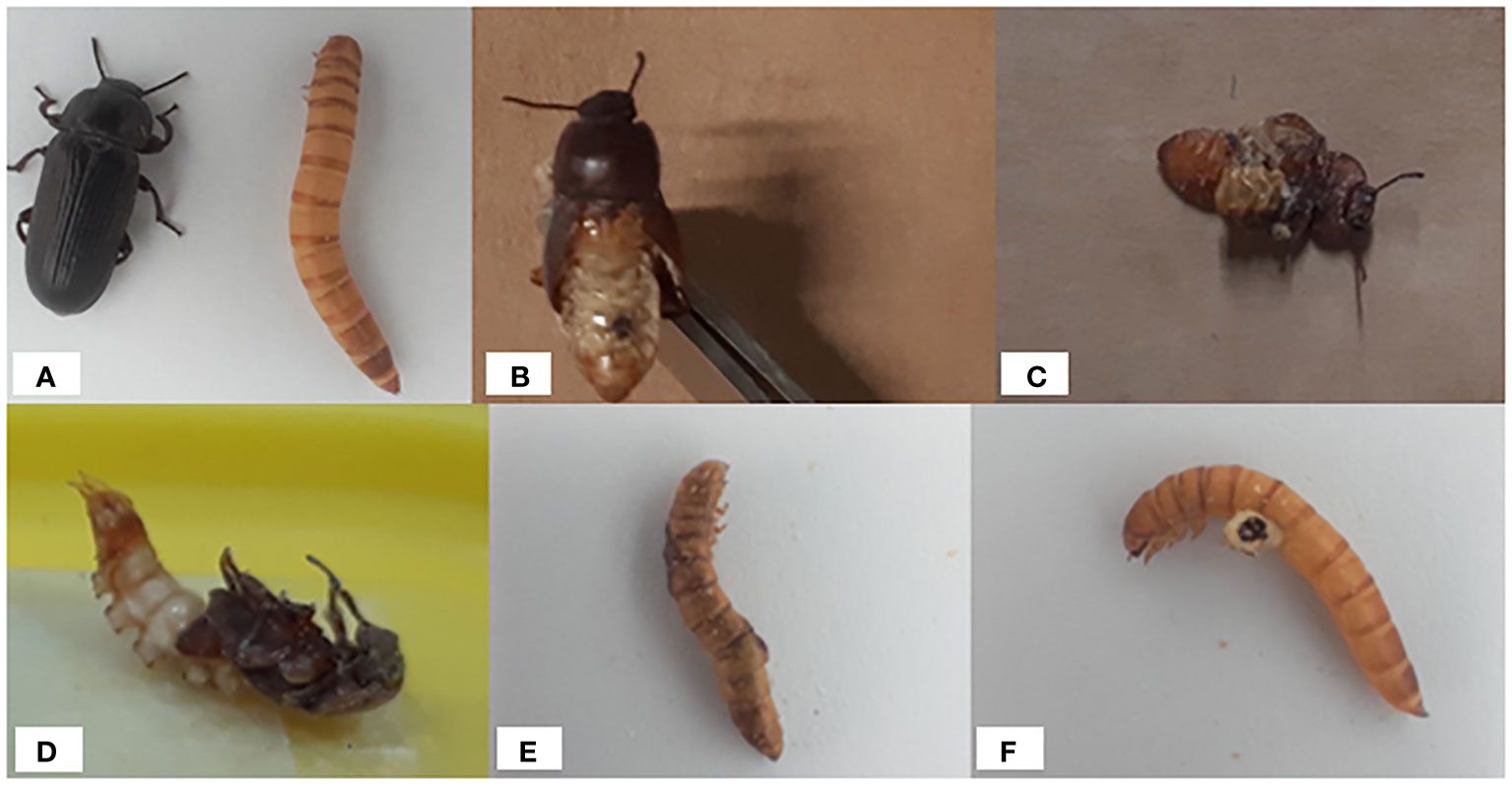
Figure 3 Deformities observed during the study of T. molitor (larvae experiment): (A) T. molitor control adult and larva. (B) 0.016 J m-2: Larva becomes a deformed alive adult. (C) 0.32 J m-2: Larva developed into a deformed adult and died. (D) 0.80 J m-2: Larva did not complete metamorphosis (severely deformed insect: half pupa, half adult). (E) 1.59 J m-2: Burned (brownish-dark) larva. (F) 7.95 J m-2: Larva were rapidly killed after exposure, and there was significant damage caused to the insects, e.g., hemolymph flowed out of the laser hole in the insects’ body.
3.2.2 Pupae
In contrast to larvae and adults, the mortality of the pupae increased between 8 and 15 days (Figure 4 and Supplementary Table S3). Fifteen days after the laser application, all pupae in the control groups developed into beetles with normal appearance and high survival rate (90%) (Figure 5A). When a dose of 0.016 J mm-2 was applied, all pupae developed into beetles with the same survival rate as the control group. However, one third developed deformed wings and/or body (Figure 5B). When the dose was increased to 0.16 J mm-2, the survival rate declined approximately 68%. The living pupae all developed into adults, which, however, had some wing and body deformities. Most of the non-living pupae and beetles were discoloured and broke into small pieces (Figure 5C). When the dose was doubled (0.32 J mm-2), the survival rate decreased even more, and the mortality rate rose to 62%. The appearance of non-living adults varied from injured (Figure 5D), cut into pieces or dead bodies without any indication of abnormal appearance. A dose of 0.80 J mm-2 increased the mortality to approximately 85%. Many pupae did not develop into adults as a high number of dead pupae had a brown dark color or completely dark and dehydrated body. A dose of 1.59 J mm-2 almost killed all insects after 15 days (mortality ~ 97.5%). Many pupae did not develop into adults due to high mortality. Dead pupae had a brown dark color or a completely dark and dehydrated body. At the highest dose (7.95 J mm-2), all pupae died within the first week after the irradiation with a burned-like appearance (Figure 5E). None of them managed to develop further to the adult stage indicating that the death happened a few days after the laser treatment.
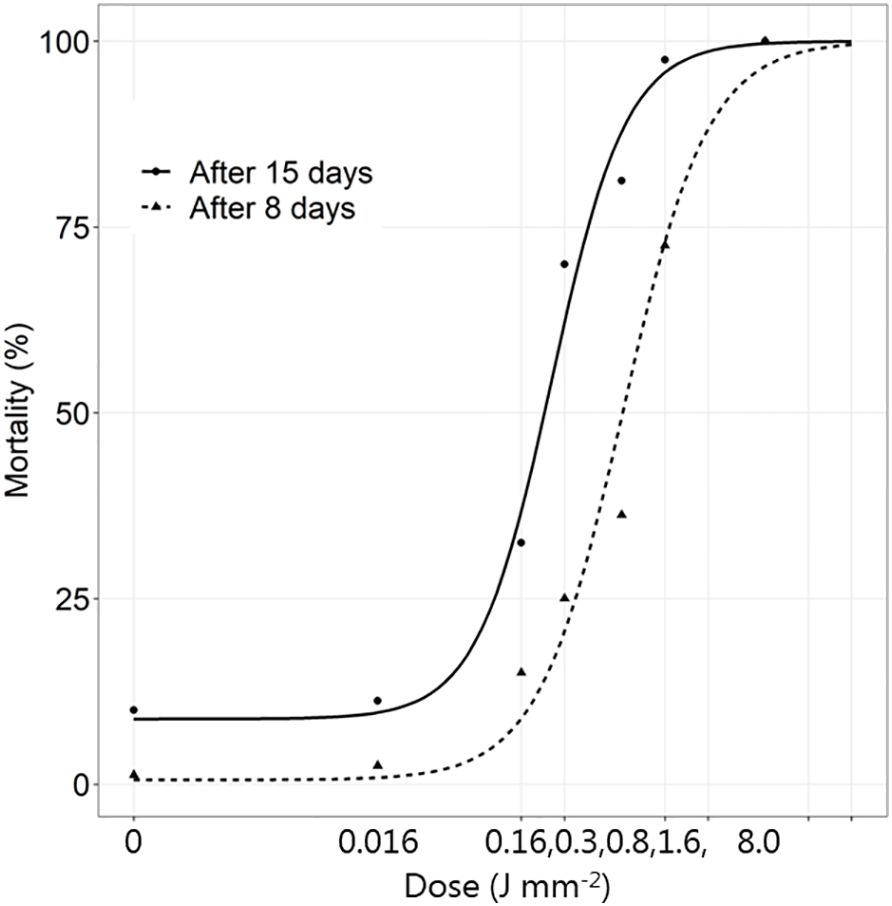
Figure 4 The mortality (%) of Tenebrio molitor pupae 8 and 15 days after exposure to increasing dosages of laser energy (J m-2) from a thulium-doped 2 µm 50 W fiber laser with a collimated beam (Ø = 2 mm). Points show mean values (n=20).
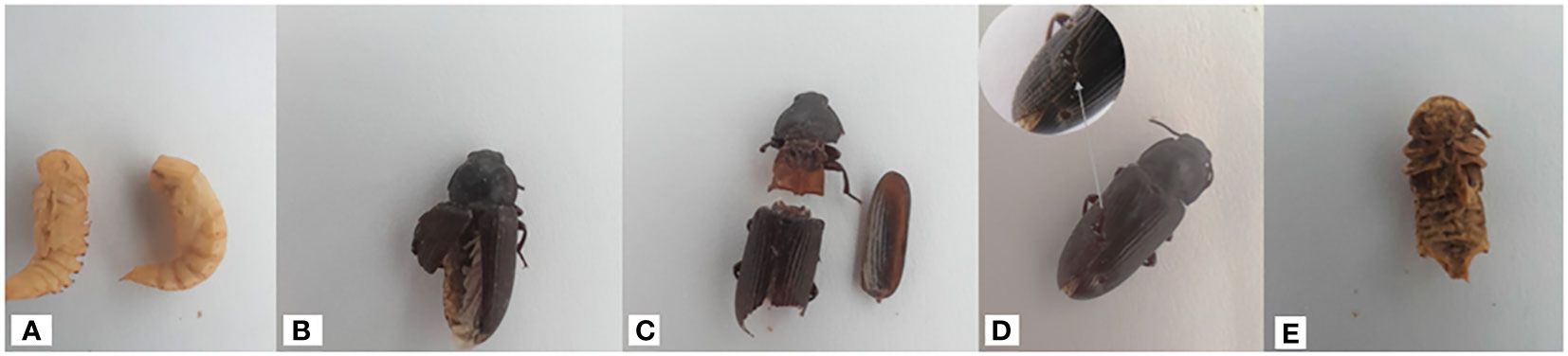
Figure 5 Typical deformities that were observed on Tenebrio molitor after laser application (pupae experiment): (A) T. molitor control pupa. (B) 0.016 J m-2 (1 ms): Pupa developed into adult with deformed wings. (C) 0.16 J m-2: Non-living adult and adults broken into pieces. (D) 0.32 J m-2: Pupa transformed to an injured adult. (E) Pupa died few days after laser application having a brownburned appearance.
3.2.3 Adults
At the lowest dose (0.016 J mm-2), the mortality did not differentiate from the controls (Figures 6, 7A and Supplementary Table 4). Treatment with 0.16 J mm-2 increased mortality. After applying a dose of 0.32 J mm-2, the mortality rate increased to ca 2.5%. Most of the living adults had a spot derived from the laser beam while most of the non-living adults had a small hole from the laser (Figure 7B). More than half of the adults died at a dose of 0.80 J mm-2 with a hole from the laser. At the highest dose, the mortality was about 92.5% and most beetles were killed immediately with hole in the body (Figure 7C).
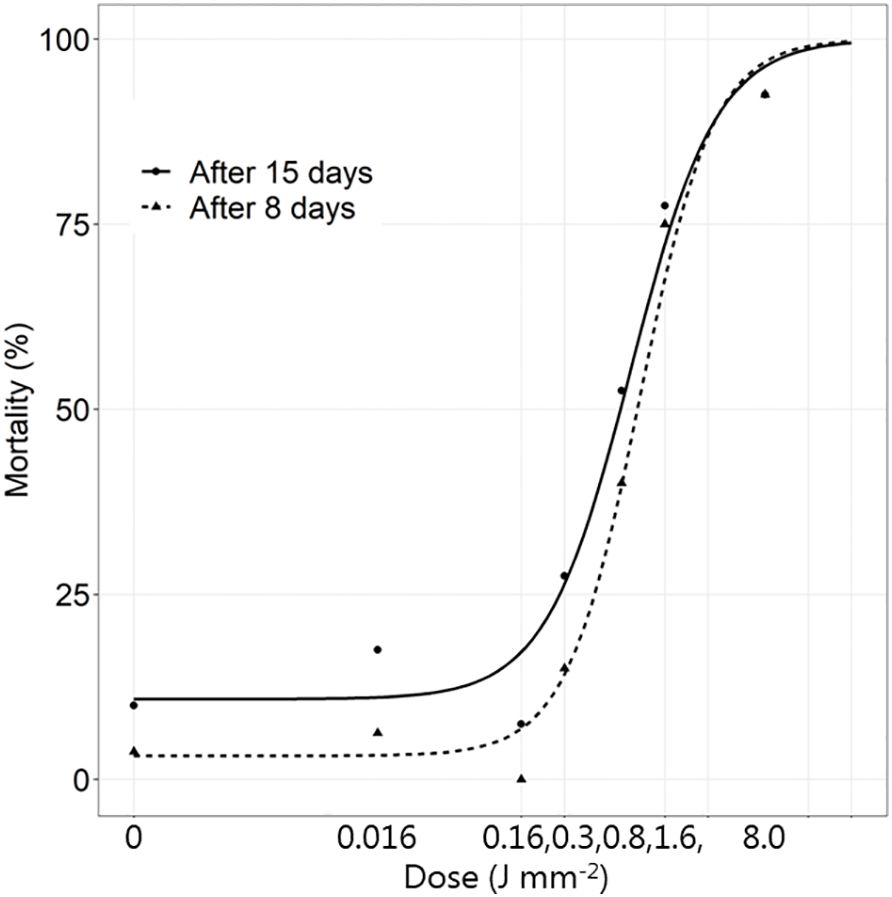
Figure 6 The mortality (%) of Tenebrio molitor beetles 8 and 15 days after exposure to increasing dosages of laser energy (J) from a thulium-doped 2 µm 50 W fiber laser with a collimated beam (Ø = 2 mm). Points show mean values (n=20).
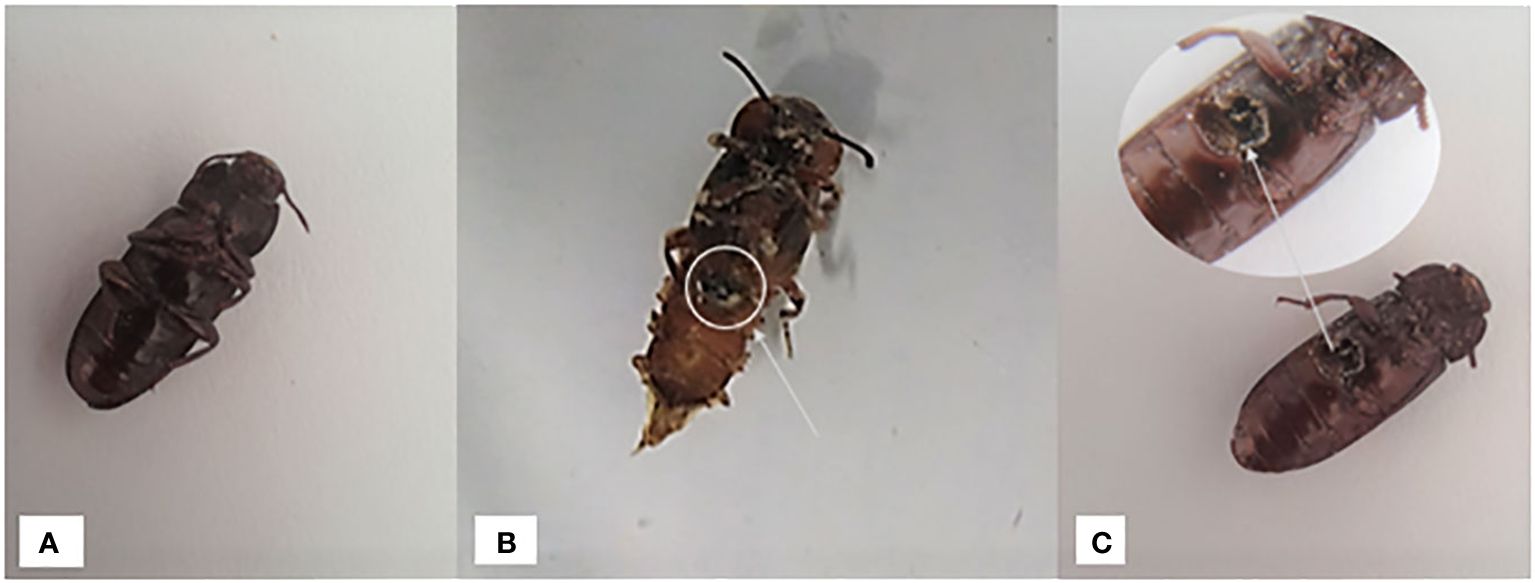
Figure 7 Typical deformities that were observed on Tenebrio molitor adults after laser application (adults’ experiment): (A) 0.016 J m-2: T. molitor adults were alive with normal appearance. (B) 0.32 J m–2-: a living adult with a spot from the laser. (C) 7.95 J m–2-: T. molitor adult died immediately with a large hole in its body.
3.3 Adalia bipunctata
In general, the non-linear model fitted the data well (Figure 8). Model parameters are shown in Supplementary Table S5. During the 15 days, some of the non-exposed beetles died. The mortality increased over time and with increasing dosages. Even 0.016 J mm-2 affected the shape of the dose-response curve, and the beetle’s elytron became brownish in color (Figure 9). A dose of 0.80 J mm-2 severely harmed the beetles, and almost all beetles died during the 15 days. A dose of 7.95 J mm-2 immediately killed all beetles burning significant holes in the beetles (Figure 9).
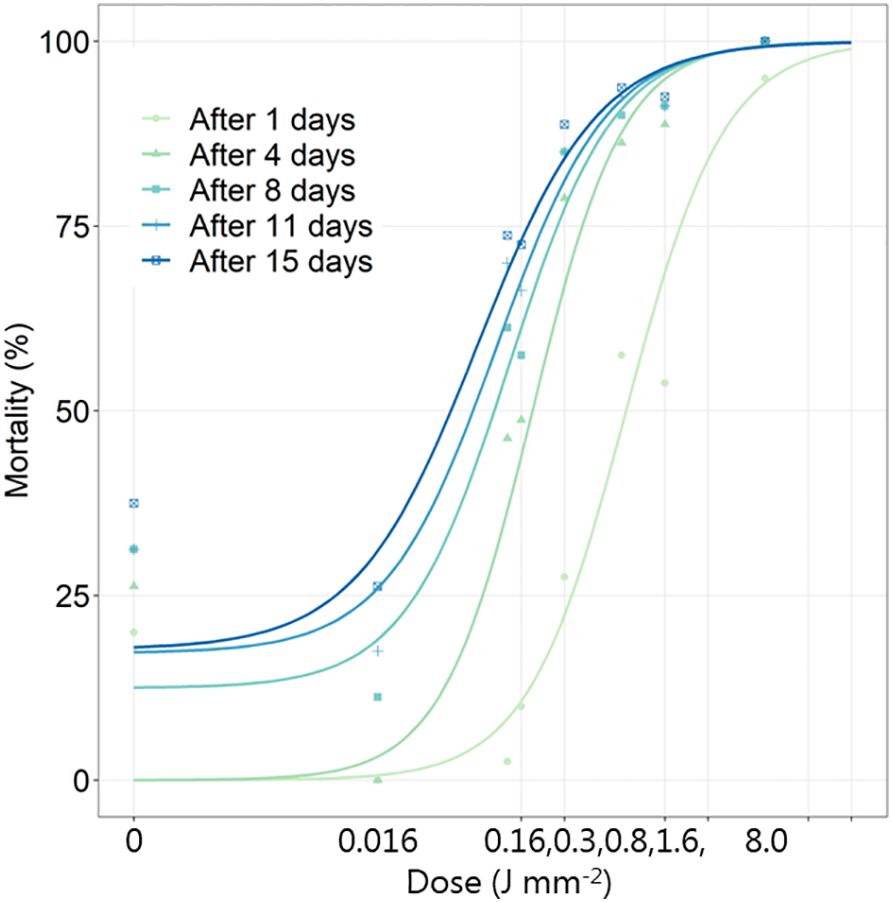
Figure 8 The mortality (%) of Adalia bipunctata beetles 1, 4, 8, 11 and 15 days after exposure to increasing dosages of laser energy (J) from a thulium-doped 2 µm 50 W fiber laser with a collimated beam (Ø = 2 mm). Points show mean values (n=20).
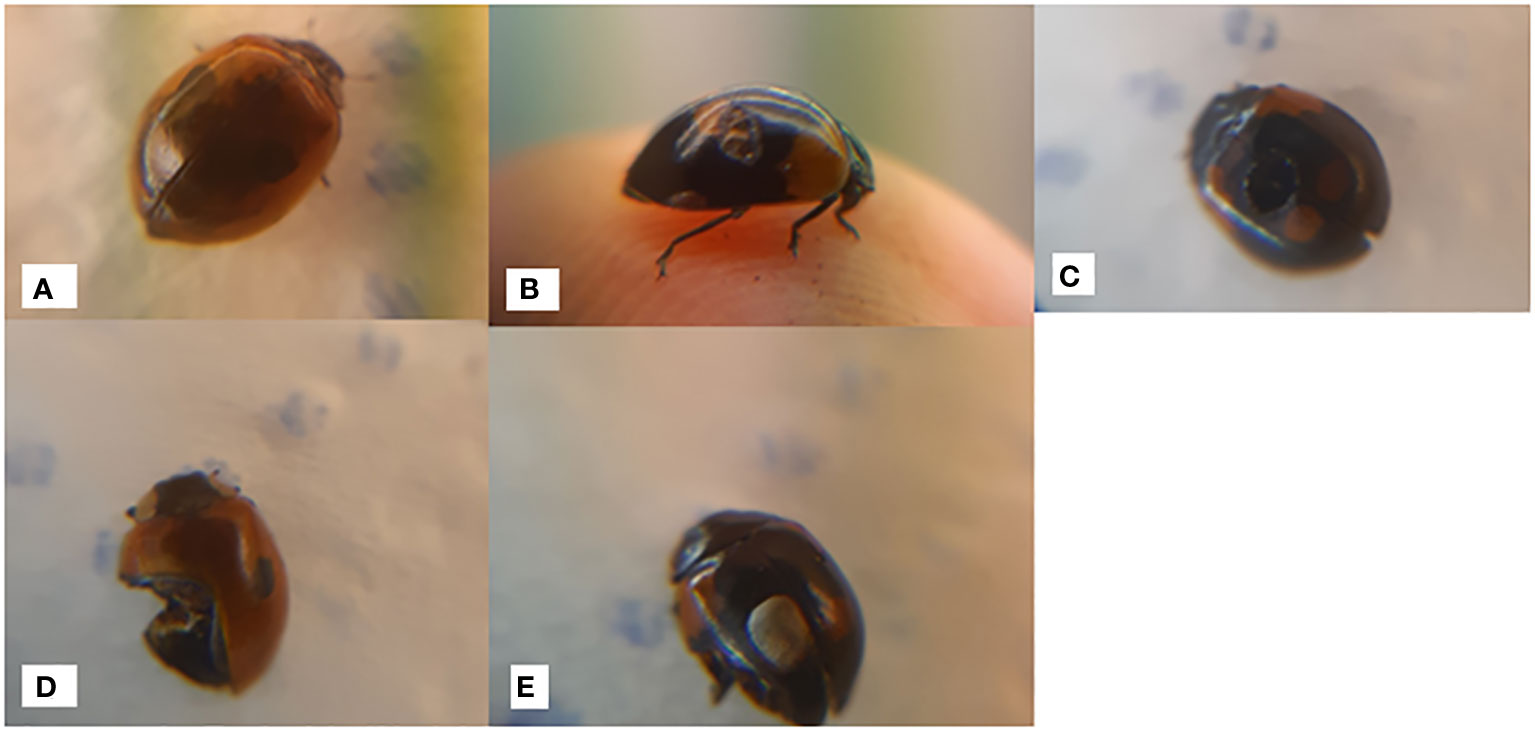
Figure 9 Malformations on the body of the adults Adalia bipunctata observed in the study. (A) 0.05 J (0.16 J mm-2): Brownish color on the outer shell. (B) 0.1 J (78 J mm-2) Small damage on an alive adult. (C) 2.5 J (50 ms J mm-2) severe damage, dead adult (D) 25 J (500 ms J mm-2) Acute damage: dead adult one day after lasering. (E) 78.57 J mm-2), fungal infection.
4 Discussion
4.1 Earthworms (Enchytraeus albidus and E. crypticus)
There were no differences in mortality between the control group and worms exposed to all laser doses, with a single exception with E. crypticus in sandy soil at a dose of 15.9 J mm-2. The spread of the heat in the soil from the laser beam depends on the water content and soil structure and composition. We cannot exclude that other conditions like a higher water content or other soil types would result in other mortalities. We consider a soil water content of 50% of the water capacity to be realistic in the early spring when weed control usually is conducted, but it depends on many factors in the field (e.g., variation in soil composition, precipitation, and evaporation). The earthworms in the tubes were living in a very small soil volume (<15 g) during the experiment mimicking the uppermost part of the soil profile. Although heat corresponding to 23.9 J mm-2 was executed on a spot with a 2 mm diameter, which easily kills weed seedling, the exposure did not warm up the soil sufficiently to affect the mortality of the worms living lose to the soil surface within the seven days.
4.2 Insects
Tenebrio molitor is a large model insect. Larger insects, such as T. molitor, appear to be more resistant to the laser than smaller insects such as A. bipunctata. In general, the insects were all killed immediately at a laser dose corresponding to what would be appropriate for killing small weed seedlings (50 J mm-2). We aimed to focus the laser on the middle of the body of the insects, but because the insects were able to move a little, there would be variations in where the laser hit. If we had focused the laser on the head or the rear part, the mortality might have been different.
4.3 Laser safety
We used a collimated beam in the experiments to precisely give the wanted dose independent of the precise distance to the target. However, if stones or other reflecting materials are hit with a collimated laser beam, the reflected beam may escape the target area, and humans or larger animals (dogs, hares, etc.) may be exposed, burned, or blinded. Therefore, the laser beam should not be collimated in laser weeding robots, but only be focused and concentrated on the meristem of the weed seedlings. If the laser then hits a reflecting material, the beam will be spread in a cone and the risk of harming the humans, animals and other plants would be significantly reduced due to the lower dose per area. That means that only insects or other organisms placed exactly in the focus point would receive the dose determined for the target plant. The further away from the focus point the lower the dose an organism would receive and the less harmful the exposure.
Some insects benefit the crop like ladybugs, spiders, and predatory beetles, as they can reduce the number of harmful insects (e.g., aphids (Aphididae) and rape beetles (Meligethes aeneus)). Some beneficial insects like ladybugs have characteristic colors and can easily be identified with recognition tools (Rakhmatulin et al., 2021). In principle, a laser-weeding robot could be programmed to recognize the different between beneficial and pest arthropods and kill the latter.
On the other hand, small dosages of laser energy could be considered to control harmful arthropods. Rakhmatulin (2021) used machine vision and showed that a low-cost device could be used to kill mosquitoes with a laser. The company Photonic Sentry (https://photonicsentry.com/) has introduced a laser mosquito neutralization technology to combating malaria. Flying insects are common vectors for the transmission of pathogens between crop plants (Heck, 2018). Mullen et al. (2016) presented proof of principle for an optical system capable of highly specific vector control using a combination of optical sources, detectors, and sophisticated software to search, detect, and identify flying insects in real-time, with the capability of eradication using a lethal laser pulse. They focused on two insect species: Diaphorina citri, a vector of the causal agent of citrus greening disease, and Anopheles stephensi, a malaria vector. There seems to be a great potential to use laser technology to protect people and crops from pestilent flying insects (Keller et al., 2020). Our experiments showed that laser dosages, which will not harm the plants, could significantly damage harmful insects.
4.4 Laser weeding compared to herbicide application and mechanical weed control
Laser weeding seems to be a promising tool to replace or supplement herbicides and mechanical weed control, with only a small treatment area in contrast to other weed control measures. Unlike herbicide spraying, laser weeding leaves no chemicals in the field that may harm non-target organisms after the treatment. Herbicides may evaporate or leach to surface and groundwater and may expose the environment to short or long-term unwanted side-effects. Laser weeding only leaves the ash from the burned weed meristem in the field after the treatment, which may be taken up by the crop plants as fertilizer.
In contrast to laser weeding, mechanical weeding impact shallow living worms negatively (and potentially other soil organisms), as the weeding implements are passing through the top layer of the soil (Doran and Zeiss, 2021). Mechanical weeding also harms beneficial organisms on the soil surface, like spiders and predatory beetles (Michalko et al., 2019; Symondson et al., 2022). Therefore, laser weeding seems to have less negative impact on the environment that other weed control measures.
5 Conclusion
The earthworms were mostly unharmed when the soil surface was exposed to laser dosages up to 23.9 J mm-2 as the soil protected them. Laser doses sufficient to kill plants were lethal to the insects, and lower doses that did not kill plants, killed or harmed the insects across all life stages tested. The larger T. monitor beetle survived higher doses than the smaller A. bipunctata beetle. The results indicate that pest control might be a possibility using laser dosages which do not harm plants. In general, the probability of harming insects with dosages used for laser-weeding is small, as only a tiny proportion of the area will be exposed for the treatment, even with a high weed density. Using another type of laser may give other results. Developing a recognition tool using artificial intelligence to differentiate between pests and beneficial arthropods would make pest control possible without harming beneficial organisms at the same time as the laser weeding takes place.
Data availability statement
The raw data supporting the conclusions of this article will be made available by the authors, without undue reservation.
Ethics statement
Ethical review and approval was not required for the study on animals in accordance with the local legislation and institutional requirements.
Author contributions
CA was responsible for funding acquisition and the design of the experiments. EV and KJ conducted the experiment with insects. SJ made the statistical analyses. CA wrote the first draft of the article, and all authors added to the manuscript, reviewed, edited, and accepted the final manuscript. All authors contributed to the article and approved the submitted version.
Funding
This work is funded by the EU–project WeLASER “Sustainable Weed Management in Agriculture with Laser-Based Autonomous Tools,” Grant agreement ID: 101000256, funded under H2020-EU.
Acknowledgments
We thank Professor Nina Cedergreen and Laboratory Technician Anja Weibel for providing earthworms, and technical support and supervision. We thank Postdoc Mahin Saberi for conducting the dose-response experiments with earthworms and Technician Mathias Mons Mørch Hansen for technical support to the insect experiments.
Conflict of interest
The authors declare that the research was conducted in the absence of any commercial or financial relationships that could be construed as a potential conflict of interest.
Publisher’s note
All claims expressed in this article are solely those of the authors and do not necessarily represent those of their affiliated organizations, or those of the publisher, the editors and the reviewers. Any product that may be evaluated in this article, or claim that may be made by its manufacturer, is not guaranteed or endorsed by the publisher.
Supplementary material
The Supplementary Material for this article can be found online at: https://www.frontiersin.org/articles/10.3389/fagro.2023.1198840/full#supplementary-material
References
Andreasen C., Scholle. K., Saberi M. (2022). Laser weeding with small autonomous vehicles: Friends or foes? Front. Agron. 4:841086. doi: 10.3389/fagro.2022.841086
Bednarska A. J., Świątek Z. (2016). Subcellular partitioning of cadmium and zinc in mealworm beetle (Tenebrio molitor) larvae exposed to metal-contaminated flour. Ecotoxicol. Environ. Saf. 133, 82–89. doi: 10.1016/j.ecoenv.2016.06.033
Castro-Ferreira M. P., Roelofs D., van Gestel C. A. M., Verweij R. A., Soares A. M. V. M., Amorim M. J. B. (2012). Enchytraeus crypticus as model species in soil ecotoxicology. Chemospere. 87 (11), 1222–1227. doi: 10.1016/j.chemosphere.2012.01.021
Cedergreen N., Nørhave N. J., Nielsen K., Johansson H. L., Marcussen H., Svendsen C., et al. (2013). Low temperatures enhance the toxicity of copper and cadmium to Enchytraeus crypticus through different mechanisms. Environ. Toxicol. Chem. 32 (10), 2274–2283. doi: 10.1002/etc.2274
Coleman G., Betters C., Squires C., Leon-Saval S., Walsh M. (2021). Low energy laser treatments control annual ryegrass (Lolium rigidum). Front. Agron. 601542. doi: 10.3389/fagro.2020.601542
Davidson R. H., Lyon W. F. (1979). Insect pests of farm, garden, and orchard. Ed. 7 (New York USA: John Wiley & Sons Inc), xi+596. doi: 10.1021/es040349c
Didden W. A. M. (1993). Ecology of terrestrial enchytraeidae. Pedobiol. 37, 2–29. Available at: https://research.wur.nl/en/publications/ecology-of-terrestrial-enchytraeidae.
Doran J. W., Zeiss M. R. (2021). Soil health and sustainability: managing the biotic components of soil quality. Appl. Soil Ecol. 15, 3–11. doi: 10.1016/S0929-1393(00)00067-6
Eggen R. I. L., Behra R., Holm P. B., Escher B. I., Schweigert N. (2004). Challenges in ecotoxicology. Environ. Sci. Technol. 59A-64A. doi: 10.1021/es040349c
Fei Q., Yang W., Yi Z., Mingsheng C., Wenjia F., Baoyuan G., et al. (2022). Toxicity and excretion of deoxynivalenol to Tenebrio molitor larvae. Asian J. Ecotoxicol. 2, 311–316. doi: 10.7524/AJE.1673-5897.20210616001
Gomes S. I. L., Soares A. M. V. M., Scott-Fordsmand J. J., Amorim M. J. B. (2013). Mechanisms of response to silver nanoparticles on(Oligochaeta): Survival, reproduction, and gene expression profile. J. Hazardous Materials 254–255), 336c344. doi: 10.1016/j.jhazmat.2013.04.005
Gordon R. D. (1985). The coccinellidae (Coleoptera) of america north of Mexico. J. New York Entomol. Soc. 93, 1. Available at: https://www.discoverlife.org/users/l/Losey,_John/JEL.html.
He E., van Gestel C. A. M. (2013). Toxicokinetics and toxicodynamics of nickel in Enchytraeus crypticus. Environ. Toxicol. Chem. 32 (8), 1835–1841. doi: 10.1002/etc.2253
Heck M. (2018). Insect transmission of plant pathogens: A systems biology perspective. mSystems. 3 (2), e00168-17. doi: 10.1128/mSystems.00168-17
Heisel T. J., Andreasen C., Christensen S. (2002). Using laser to measure stem thickness and cut weed stems. Weed Res. 42 3, 242–248. doi: 10.1046/j.1365-3180.2002.00282.x
Heisel T., Schou J., Christensen S., Andreasen C. (2001). Cutting weeds with CO2 laser. Weed Res. 41, 19–29. doi: 10.1046/j.1365-3180.2001.00212.x
Hodek I., van Emden H. F., Honěk A. (2012). Ecology and behavior of the ladybird beetles (Coccinellidae) (Chichester, UK: Blackwell Publishing Ltd.), 561. doi: 10.1002/9781118223208
Hothorn T., Bretz F., Westfall P. (2008). Simultaneous inference in general parametric models. Biometrical J. 50, 346–363. doi: 10.1002/bimj.200810425
Jensen S. M., Wolkis D., Keshtkar E., Streibig J. C., Ritz C. (2020). Improved two-step analysis of germination data from complex experimental designs. Seed Sci. Res. 30, 194–198. doi: 10.1017/S0960258520000331
Keller M. K., Norton B. J., Farrar D. J., Rutschman P., Marvit M., Makagon A. (2020). Optical tracking and laser-induced mortality of insects during fight. Sci. Rep. 10, 4795. doi: 10.1038/s41598-020-71824-y
Khan A. A., Qureshi J. A., Afzal M., Stansly P. A. (2016). Two-Spotted Ladybeetle Adalia bipunctata L. (Coleoptera: Coccinellidae): A commercially available predator to control asian citrus psyllid Diaphorina citri (Hemiptera: Liviidae). PloS One 11 (9), e0162843. doi: 10.1371/journal.pone.0162843
Kuperman R. G., Amorim M. J. B., Römbke J., Lanno R., Checkai R. T., Dodard S. G., et al. (2006). Adaptation of the enchytraeid toxicity test for use with natural soil types. Eur. J. Soil Biol. 42, 234–243. doi: 10.1016/j.ejsobi.2006.07.028
Lv X., Lui C., Li Y., Gao Y., Wang H., Li J., et al. (2014). Stereoselectivity in bioaccumulation and excretion of epoxiconazole by mealworm beetle (Tenebrio molitor) larvae. Ecotox. Environ. Safety. 107, 71–76. doi: 10.1016/j.ecoenv.2014.02.013
McCallum M. L., Matlock M., Treas J., Safi B., Sanson W., McCallum J. L. (2013). Endocrine disruption of sexual selection by an estrogenic herbicide in the mealworm beetle (Tenebrio molitor). Ecotox. 22, 1461–1466. doi: 10.1007/s10646-013-1132-3
Mehdizadeh M., Mushtaq W., Siddiqui S. A., Ayadi S., Kaur P., Yeboah S., et al. (2021). Herbicide residues in agroecosystems: Fate, detection, and effect on non-target plants. Rev. Agricul. Sci. 9, 157–167. doi: 10.7831/ras.9.0_157
Michalko R., Pekár S., Dulá M., Entling M. H. (2019) Global patterns in the biocontrol efficacy of spiders. A meta-analysis. Global Ecol. Biogeogr. 28, 1366–1378. doi: 10.1111/geb.12927
Mullen E. R., Rutschman P., Pegram N., Patt J. M., Adamczyk J. J., Johanson (2016). Laser system for identification, tracking, and control of flying insects. Opt. Express 24 (11), 11828–11838. doi: 10.1364/OE.24.011828
Rakhmatulin I. (2021). Machine vision for low-cost remote control of mosquitoes by power laser. J. Real-Time Image Process. 18, 2027–2036. doi: 10.1007/s11554-021-01079-x
Rakhmatulin I., Andreasen C. (2020). A concept of a compact and inexpensive device for controlling weeds with laser beams. Agron. 10 (1616), 1-18. doi: 10.3390/agronomy10101616
Rakhmatulin I., Kamilaris A., Andreasen C. (2021). Deep neural networks to detect and classify weeds from crops in agricultural environments in real-time: A review. Remote Sens 21), 4486. doi: 10.3390/rs13214486
R Core Team (2021). R: A language and environment for statistical computing (Vienna, Australia: R Foundation for Statistical Computing). Available at: Https://ww.R-project.org/.
Symondson W. O. C., Sunderland K. D., Greenstone M. H. (2022) Can generalist predators be effective biocontrol agents? Ann. Rev. Entomol. 47, 561–594. doi: 10.1146/annurev.ento.47.091201.145240
Thiour-Mauprivez C., Martin-Laurent F., Calvayrac C., Barthelmebs L. (2019). Effects of herbicide on non-target microorganisms: Towards a new class of biomarkers? Sci. Total Environ. 684, 314–325. doi: 10.1016/j.scitotenv.2019.05.230
Wieliczka D. M., Weng S., Querry M. R. (1989) Wedge shaped cell for highly absorbent liquids. Infrared optical constants of water. Appl. Optic. 28, 1714–1719. doi: 10.1364/AO.28001714
Wyss E., Villiger M., Hemptinne J. L., Müller-Schärer H. (1999). Effects of augmentative releases of eggs and larvae of the two-spot ladybird beetle, Adalia bipunctata, on the abundance of the rosy apple aphid, Disaphis plantaginea, in organic apple orchards. Entomol. Exp. Appl. 90, 167–173. doi: 10.1046/j.1570-7458.1999.00435.x
Keywords: integrated weed management, laser non-target, laser eco-toxicology, non-chemical weed control, site-specific weed management, thermal weed control
Citation: Andreasen C, Vlassi E, Johannsen KS and Jensen SM (2023) Side-effects of laser weeding: quantifying off-target risks to earthworms (Enchytraeids) and insects (Tenebrio molitor and Adalia bipunctata). Front. Agron. 5:1198840. doi: 10.3389/fagro.2023.1198840
Received: 02 April 2023; Accepted: 17 October 2023;
Published: 02 November 2023.
Edited by:
Mingzhi Huang, South China Normal University, ChinaReviewed by:
Liangang Mao, Chinese Academy of Agricultural Sciences, ChinaCharles Norman Merfield, The BHU Future Farming Centre, New Zealand
Copyright © 2023 Andreasen, Vlassi, Johannsen and Jensen. This is an open-access article distributed under the terms of the Creative Commons Attribution License (CC BY). The use, distribution or reproduction in other forums is permitted, provided the original author(s) and the copyright owner(s) are credited and that the original publication in this journal is cited, in accordance with accepted academic practice. No use, distribution or reproduction is permitted which does not comply with these terms.
*Correspondence: Christian Andreasen, Y2FuQHBsZW4ua3UuZGs=