- Department of Plant Agriculture, University of Guelph, Guelph, ON, Canada
One billion people globally suffer from protein (amino acid) malnutrition. Grain legumes represent a solution. They recruit symbiotic rhizobia bacteria from soil into root nodules, where the rhizobia convert atmospheric nitrogen gas (N2) into ammonia (NH3) which serves as a building block for chlorophyll and protein. However, when a legume species is newly introduced to a region, yields can be low due to incompatible soil rhizobia. Millions of subsistence legume farmers can benefit from inoculation with exotic rhizobia bacteria, but many subsistence farmers especially in Africa do not benefit from commercial inoculants due to real-world constraints. Here, in a sequential series of indoor and outdoor experiments, we show that root nodules (rhizobia habitats) can be harvested and crushed onto legume seeds, ultimately improving nodulation and chlorophyll under field conditions. 16S rRNA metagenomic sequencing confirmed that nodule crushing onto seeds effectively transferred rhizobia to next-generation nodules. Therefore, nodule crushing represents a simple method to diffuse elite rhizobia strains. However, exotic rhizobia come with risks and limitations. Therefore, in addition to diffusing elite rhizobia, we propose that this simple, decentralized technology can also empower smallholders to improve indigenous strains or indigenize exotic strains by repeated nodule crushing from healthy plants.
1 Introduction
One billion people worldwide suffer from protein (amino acid) deficiencies, primarily in Africa and South Asia (Wu et al., 2014). During crop production, nitrogen is required to synthesize amino acids, as well as chlorophyll, which is essential for good yields (Lal, 2021). When fuel prices increase, synthetic fertilizer prices also increase (Brunelle et al., 2015), making fertilizers unaffordable for the world’s 400 million subsistence farming families (Samberg et al., 2016), triggering leaps in malnutrition (Food Security Information Network, 2022). Grain legumes (including soybeans, common bean, lentil, cowpea) represent a solution. They recruit symbiotic rhizobia bacteria from soil into specialized root organs called nodules, where the rhizobia convert atmospheric nitrogen gas (N2) into ammonia (NH3), a process termed biological nitrogen fixation (BNF) (Mendoza-Suárez et al., 2021).
When a legume species is introduced to a region, including the current introduction of soybeans across Africa, yields can be low due to incompatible rhizobia in the soil (Mendoza-Suárez et al., 2021; Thilakarathna and Raizada, 2016). Fortunately, exotic rhizobia can be inoculated onto seeds (Thilakarathna and Raizada, 2016; Chibeba et al., 2018; van Heerwaarden et al., 2018; Nyaga & Njeru, 2020). Despite their promise, many subsistence farmers especially in Africa do not benefit from commercial inoculants due to poor distribution systems, lack of refrigeration, pathogen contamination during shipping and a short shelf life (Vanlauwe et al., 2019; Raimi et al., 2021). Overcoming this problem may raise African legume yields which averaged 1.1 t/ha compared to 2.6 t/ha in North America in 2020 (Food and Agriculture Organization of United Nations, 2022). A new approach is needed to replace the existing paradigm of shipping elite rhizobia strains from a manufacturing source, through a distribution network, to subsistence farmers. At the same time, exotic rhizobia suffer from risks and limitations (e.g. competition from indigenous rhizobia, poor adaptation to local environments, pathogen contamination) (Thilakarathna and Raizada, 2016; Vanlauwe et al., 2019; Mendoza-Suárez et al., 2021), hence a new strategy to improve elite strains and/or scale up indigenous rhizobia is also needed.
Rhizobia can remain viable in mature nodules both in determinate (e.g. soybean, cowpea) and indeterminate (e.g. lentil, peas) types (Mendoza-Suárez et al., 2021). Laboratory scientists routinely recover rhizobia by crushing nodules into microbiological growth media (Guan et al., 2013; Youseif et al., 2014). Here we hypothesized that nodulation of legume plants growing in virgin soils could be improved under real-world field conditions simply by crushing harvested nodules directly onto legume seeds under conditions that mimic the resources available to subsistence farmers. We also demonstrate that field-derived nodules also improve nodulation after crushing onto seeds, suggesting this strategy holds potential for improving exotic or indigenous rhizobia.
2 Materials and methods
2.1 Biological materials
Seeds of soybean (Glycine max variety Colby) were acquired from the Soybean Breeding Laboratory (Prof. Istvan Rajcan), University of Guelph, while the remaining seeds were acquired from William Dam Seeds, Ontario, Canada as follows: pea (Pisum sativum, #2941C), lentil (Lens culinaris, #4884A) and cowpea (Vigna unguiculata, #4886A). Rhizobia strains were as follows: for soybean (Bradyrhizobium japonicum USDA110); for green pea/lentil (Rhizobium leguminosarum bv viciae Rlv3841) and for cowpea (Bradyrhizobium yuanmingense TTC9).
2.2 Seed inoculation methods
2.2.1 Inoculation using pure rhizobia
Seeds were inoculated with each rhizobia strain grown in liquid YM at 30˚C for 3-6 days (OD600 = 0.5). Subsequently, 500 µl inoculum was mixed with 10 ml of 10% (v/v) sterile molasses, to which 25 seeds were added and incubated for 1 h at room temperature before planting.
2.2.2 Inoculation using nodule crushing
Nodules of six-week-old plants were harvested, washed, sterilized and crushed using methods adapted from a prior study (Senthilkumar et al., 2021). Briefly, roots with nodules (Figure 1A) were rinsed with sterile ddH2O, then nodules detached (by hand or using sterilized blades), and surface sterilized using 2% sodium hypochlorite for 5 min, followed by 5 washes with sterile ddH2O. Nodules were placed in sterile tubes, mixed with 1 ml sterile ddH2O, then left in the fridge overnight. The next day, under sterile conditions, nodules were crushed using sterile forceps (Figure 1B), then 500 µl liquid was removed and mixed with 10 ml of 10% (v/v) sterile molasses, to which 25 seeds were added (Figure 1C) and incubated for 1 h at room temperature before planting.
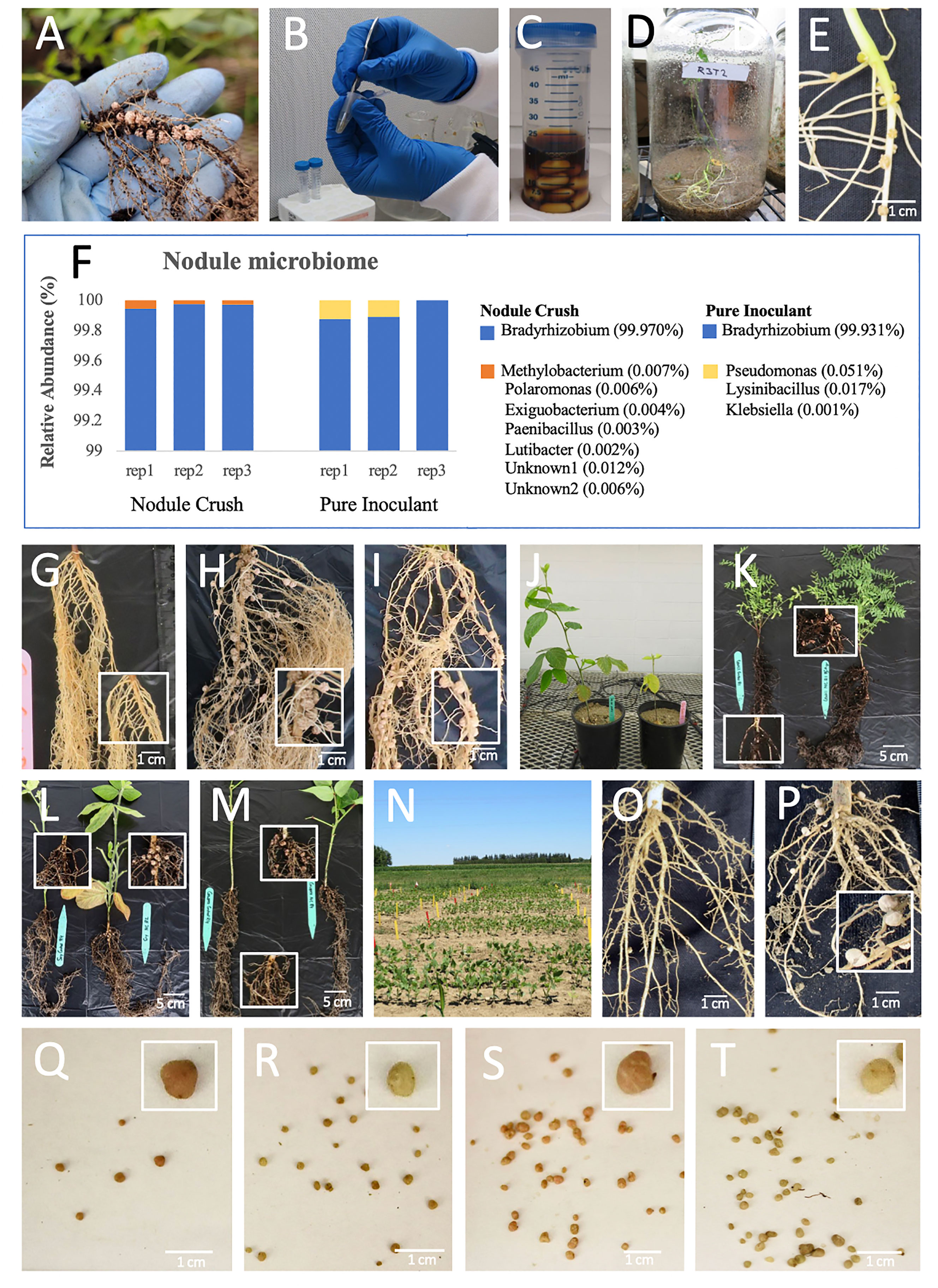
Figure 1 Effectiveness of nodule crushing onto seeds to transfer nitrogen-fixing rhizobia. (A-E) The nodule crushing technique: (A) Example of donor root nodules (cowpea). (B) Crushing soy nodules. (C) Incubating crushed nodule extract with seeds using a sticky agent (e.g. molasses). (D) Closed, sterile jar to demonstrate that future nodules solely originate from crushed nodules. (E) Resulting soy nodules in a closed jar following nodule crushing. (F) Soybean nodule microbiome, showing transfer of rhizobia by nodule crushing is equally effective as pure inoculant. (G–J) Soybean roots grown on sterilized sand, after seeds were treated with: (G) buffer (negative control), (H) nodule crushing, (I) pure inoculant (positive control). (J) Plants derived from seeds treated with nodule-crushing (left) versus buffer (right) on sand. (K–M) Whole plants grown on commercial soil in pots derived from seeds treated with buffer coating (left) versus nodule crushing (right) for: (K) lentil, (L) soybean and (M) cowpea. (N–T) Nodule crushing experiments in field-grown cowpea (Elora, Canada): (N) plots; (O, P) roots of plants derived from (O) buffer-coated seeds showing rare nodules and (P) nodule crushing-treated seeds showing increased nodulation; (Q, R) nodules derived from buffer-inoculated seeds showing (Q) few pink nodules (active) compared to (R) non-pink nodules (inactive); (S,T) nodules derived from nodule crushing-treated seeds showing (S) increased pink nodules versus (T) non-pink nodules.
2.3 Indoor plant trials
2.3.1 Indoor sterile jar trials (closed system)
To obtain first-generation donor nodules, soybean seeds were germinated in 4 L pre-sterilized glass jars (height, 25 cm; diameter, 15 cm) containing 500 ml of sterile Phytagel containing 1/2 strength Hoagland’s Modified Basal Salt Mixture without nitrogen (Catalog HOP03, Caisson Labs, USA) at pH 6.8. All work was undertaken under sterile conditions in a biosafety hood. Soybean seeds were surface-sterilized by submersing them for 3 min in 2% bleach followed by 4 min in 70% ethanol. The seeds were then soaked in autoclaved water overnight before the day of planting. Three seeds were added per jar, then sealed. Jars were kept in darkness until germination then moved under full-spectrum LED lights with an intensity of ~150 μmol/m2/sec at canopy level (90 μmol/m2/sec at pot level) with a 16:8 hour day:night photoperiod at room temperature. Nodules were harvested at 42 days after germination.
To test whether crushed nodules could directly transfer rhizobia to the next generation, while ensuring no foreign source of rhizobia, the nodules were crushed, mixed with molasses and coated onto new soybean seeds (see Inoculation Methods, above), which were planted into new sealed jars, but this time containing sterilized sand. Sand was used to mimic many subtropical African soils. The same procedure was used as the Phytagel experiment, except per jar, 500 g of sterile pure sand was mixed with the nutrient solution as a single starter dose (225 ml of 1/2 strength Hoagland’s Modified Basal Salt Mixture without nitrogen, pH 6.8) and irrigated twice over the entire experiment with sterile ddH2O. The negative control was the molasses buffer coated onto seeds. The positive control was pure rhizobia (Bradyrhizobium japonicum USDA110; see Inoculation Methods, above). The pure rhizobia was mixed with molasses and coated onto seeds; to prevent cross-contamination with the other treatments, the positive control treatment was undertaken one day later. There were 4 replicates per treatment (4 jars, each with 3 plants). Jars were randomized and regularly rotated. Nodules were again harvested at 42 days after germination, and plants were phenotyped.
2.3.2 Indoor sterile sand pot trial (open system)
We then tested whether crushed nodules could transfer rhizobia effectively to seeds in an open environment, using soybean and field pea. Treated seeds were sown into open pots (2.5 L) containing autoclaved sand, and placed in an indoor growth room. The seed treatments were: crushed nodules in molasses, molasses buffer (negative control), and pure rhizobia (positive control: for soybean, Bradyrhizobium japonicum USDA110; for field pea, Rhizobium leguminosarum bv viciae Rlv3841). The nodule donors had been surface sterilized (see Inoculation Methods, above) and obtained from soybean or pea plants growing in non-sterile commercial soil (BM6-HP, Berger Canada) in pots. There were 3 replicates per treatment (3 pots, each with 1 plant). Pots were randomized and regularly rotated. Pots were placed under mixed fluorescent and incandescent light at 320 μmol/m2/s at canopy level (280 μmol/m2/sec at pot level), with a 16:8 hour day:night photoperiod, with 25˚C day/20˚C night temperatures. Per day, 180 ml/pot of water was supplied through drip irrigation. Fertilizers were supplied through a stock solution of NPK (0:40:20) with added MgSO4 and micronutrients mixed with irrigation water at a 1:100 ratio, 2 days/week. Nodules were harvested at 42 days after germination, and plants were phenotyped.
2.3.3 Indoor non-sterile commercial soil trial
We then tested whether crushed nodules could transfer rhizobia effectively to seeds planted in living soil, using soybean, lentil and cowpea. Treated seeds were sown into open pots (2.5 L) containing non-autoclaved commercial soil (BM6-HP, Berger Canada), and placed in an indoor growth room. The seed treatments again were: crushed nodules in molasses (obtained from soybean, lentil or cowpea plants growing in non-sterile BM6-HP soil), molasses buffer (negative control), and pure rhizobia (positive control: for soybean, Bradyrhizobium japonicum USDA110; for lentil, Rhizobium leguminosarum bv viciae Rlv3841 and for cowpea, Bradyrhizobium yuanmingense TTC9). There were 3 replicates per treatment (3 pots, each with 1 plant). Pots were randomized and regularly rotated. All remaining conditions were the same as the sand pot trial above, except for water and fertilizer rates: water was applied at a rate of 120 ml/day, and fertilizer was applied 1 day/week. Nodules were harvested at 42 days after germination, and plants were phenotyped.
2.4 Field trials
We then tested whether nodule crushing based inoculation of seeds could improve nodulation under field conditions. The experiment occurred at the Elora Research Station, Elora, Canada in 2022, at a site with a long history of soybean cultivation and prior inoculation with compatible rhizobia. The seed treatments were again: crushed nodules in molasses (obtained from soybean, lentil or cowpea plants growing in non-sterile BM6-HP soil), molasses buffer (negative control), and pure rhizobia (positive control: for soybean, Bradyrhizobium japonicum USDA110; for lentil, Rhizobium leguminosarum bv viciae Rlv3841 and for cowpea, Bradyrhizobium yuanmingense TTC9). Treated seeds were manually sown from July 14-22, 2022. Each treatment plot was 2 m x 2 m containing 4 rows, with 20 plants/row. Per crop, treatments were arranged in a randomized complete block design (RCBD) with 4 blocks. At harvest, soil pH was 7.5 and available NPK were 16, 9 and 60 ppm respectively. NPK (0:40:20) with micronutrients were applied 20 days after planting as a single-dose topdress. Harvesting occurred 62, 63, and 71 days after germination for cowpea, lentil and soy, respectively.
2.5 Field-derived nodule efficacy trial
Finally, we tested whether field-derived nodules could be a viable source of rhizobia via the nodule crushing procedure. The experiment was conducted in soybean. Field soil was collected from the Elora Research Station, Elora, Canada and mixed with pure sand in a ratio of 1:1 to enable subsequent nodule collection and phenotyping. For the nodule crush treatment, fresh nodules were harvested from soybean plants grown under field conditions in Elora (see Field Trial method above) at 65 days after germination. The seed treatments were: crushed field-derived nodules in molasses, molasses buffer (negative control), and pure rhizobia (positive control: Bradyrhizobium japonicum USDA110). There were 3 replicates per treatment (3 pots, each with 1 plant). Pots were randomized and regularly rotated. All remaining conditions were the same as the sand pot trial above, except for water and fertilizer rates: water was applied at a rate of 120 ml/day, and fertilizer was applied 1 day/week. Nodules were harvested at 42 days after germination, and plants were phenotyped.
2.6 Relative chlorophyll measurements
Relative chlorophyll was estimated using a SPAD-502Plus meter (KonicaMinolta, Japan) by measuring the 3rd fully emerged leaf from the top of each branch. An average of 3 branches/plant were measured at 35 and/or 50 days after emergence as noted. For indoor pot trials, there were 3 replicates (each replicate was 1 plant per pot). For the field trial, there were 4 replicates (4 blocks) per treatment per time point, with each replicate representing the average of 5 plants.
2.7 Nodule trait measurements
The following nodule parameters were measured at harvest (harvest times noted in each trial above): nodule number, fresh weight, colour, and diameter using ImageJ tracing. For indoor trials, there were 4 replicates per treatment for the jar trials (each replicate was the average of 3 plants per jar) or 3 replicates for the pot trials (each replicate was 1 plant per pot). For the field trial, there were 4 replicates (4 blocks) per treatment, with each replicate representing the average of 15 plants for cowpea and lentil, and 20 plants for soybean. To prevent differential nodule drying, for indoor trials, nodules were phenotyped immediately after harvesting. For outdoor trials, immediately after the harvest, nodules were placed in airtight containers (Falcon tubes) in a styrofoam box containing coolant blocks until they were phenotyped.
2.8 Vegetative biomass and tissue nitrogen measurements
Shoot and root dry biomass were measured at harvest (harvest times noted in each trial above). For indoor pot trials, there were 3 replicates (each replicate was 1 plant per pot). For the field trial, there were 4 replicates (4 blocks) per treatment, with each replicate representing the average of 5 plants for cowpea, and 10 plants for soybean and lentil. At harvest, shoot-combustible nitrogen content was measured from field-grown plants as noted by harvesting the entire shoot; shoots of 5 plants were then pooled per replicate, ground, and then 10 g of a dried subsample was submitted for analysis (AFL, University of Guelph).
2.9 Statistical analysis
Data were analyzed using a generalized linear mixed model (GLMM) using PROC GLIMMIX, then analyzed using One-Way ANOVA, and means were compared using Tukey’s pairwise comparison in SAS 9.4 (SAS Institute, Cary, North Carolina, USA) with a significance level of P ≤ 0.05 or P ≤ 0.10 as noted.
2.10 16S RNA metagenome profiling
To confirm whether nodule crushing onto soybean seeds transfers the expected rhizobia to subsequent nodules, their microbiomes were analyzed using 16S RNA Illumina MiSeq metagenome analysis as previously described (Khalaf et al., 2021). Seeds were treated with crushed nodules in molasses or pure rhizobia (positive control: Bradyrhizobium japonicum USDA110). The nodule donors had been surface sterilized (see Inoculation Methods, above) and obtained from soybean plants growing in non-sterile commercial soil (BM6-HP, Berger Canada) in pots. Treated seeds were then sown in open pots (2.5 L) containing autoclaved sand, and placed in an indoor growth room. The growth conditions were those noted in the indoor sterile sand pot trial above. There were 3 replicates per treatment (3 pots, each with 1 plant). Pots were randomized and regularly rotated. At 42 days after germination, approximately 20 nodules per replicate per treatment were harvested. Under sterile conditions, the nodules were surface sterilized using 2% sodium hypochlorite for 5 min, followed by 5 washes with sterile ddH2O. Nodules were then crushed with a mortar and pestle in liquid nitrogen, and then genomic DNA was isolated using the ZymoBIOMICS DNA Miniprep Kit (Zymo Research, USA). The DNA was PCR amplified and sequenced using V4-V5 16S rRNA primers (Illumina, MetagenomBio, Canada) with data processing, as previously described (Khalaf et al., 2021). Fastq files of obtained sequences were deposited in Genbank under the BioProject accession number PRJNA930900.
3 Results
The nodule crushing procedure as a source of rhizobia inoculant involved harvesting nodules (Figure 1A), surface sterilizing and crushing them in sterile water (Figure 1B), followed by mixing with a sticky agent (molasses, Figure 1C), and coating onto legume seeds. Subsequently, plants were grown (Figure 1D) and nodulation was examined (Figure 1E).
The first experiment was to confirm whether nodule crushing onto seeds could transfer rhizobia. A compatible Bradyrhizobium starter inoculant was coated onto soybean seeds to generate donor nodules. When these nodules were crushed onto seeds and germinated indoors on sterilized sand, 16S RNA based microbiome analysis of the next generation nodules showed that >99.9% of the bacterial community was Bradyrhizobium, similar to seeds inoculated with the pure strain (positive control) (Figure 1F). Buffer-inoculated seeds (negative control) produced no nodules. This result verified that the nodule crushing procedure could transfer viable rhizobia onto seeds.
Thereafter, the efficacy of nodule crushing was eventually tested using four legumes possessing determinate nodules (soybean, cowpea) and indeterminate nodules (pea, lentil) (Howieson and Dilworth, 2016) in a sequential series of experiments across two years, from a closed sterile system, to open pots with sterilized sand followed by non-sterile soil, and finally under real world field conditions:
3.1 Indoor nodulation trials
Initial measurements of nodulation involved a sealed jar with sterilized sand. In this system, soybean seeds inoculated with crushed nodules were found to produce nodules, whereas buffer-inoculated roots were bare (Figure 1E; Table 1a). Next, nodules were harvested from soy or pea grown on commercial soil, then crushed onto seeds planted in sterilized sand indoors but in open pots; the germinated plants were observed to have dramatically and significantly (p ≤ 0.05) improved nodulation, leaf chlorophyll and plant biomass compared to buffer-inoculated seeds (Figures 1G–J and Tables 1b, c; Figure S1A). Similar results were obtained under non-sterile conditions, wherein crushed nodules from indoor plants (lentil, soy, cowpea) grown in commercial soil were used as inoculum for seeds also sown indoors in commercial soil (Figures 1K–M and Tables 1d–f; Figure S1B).
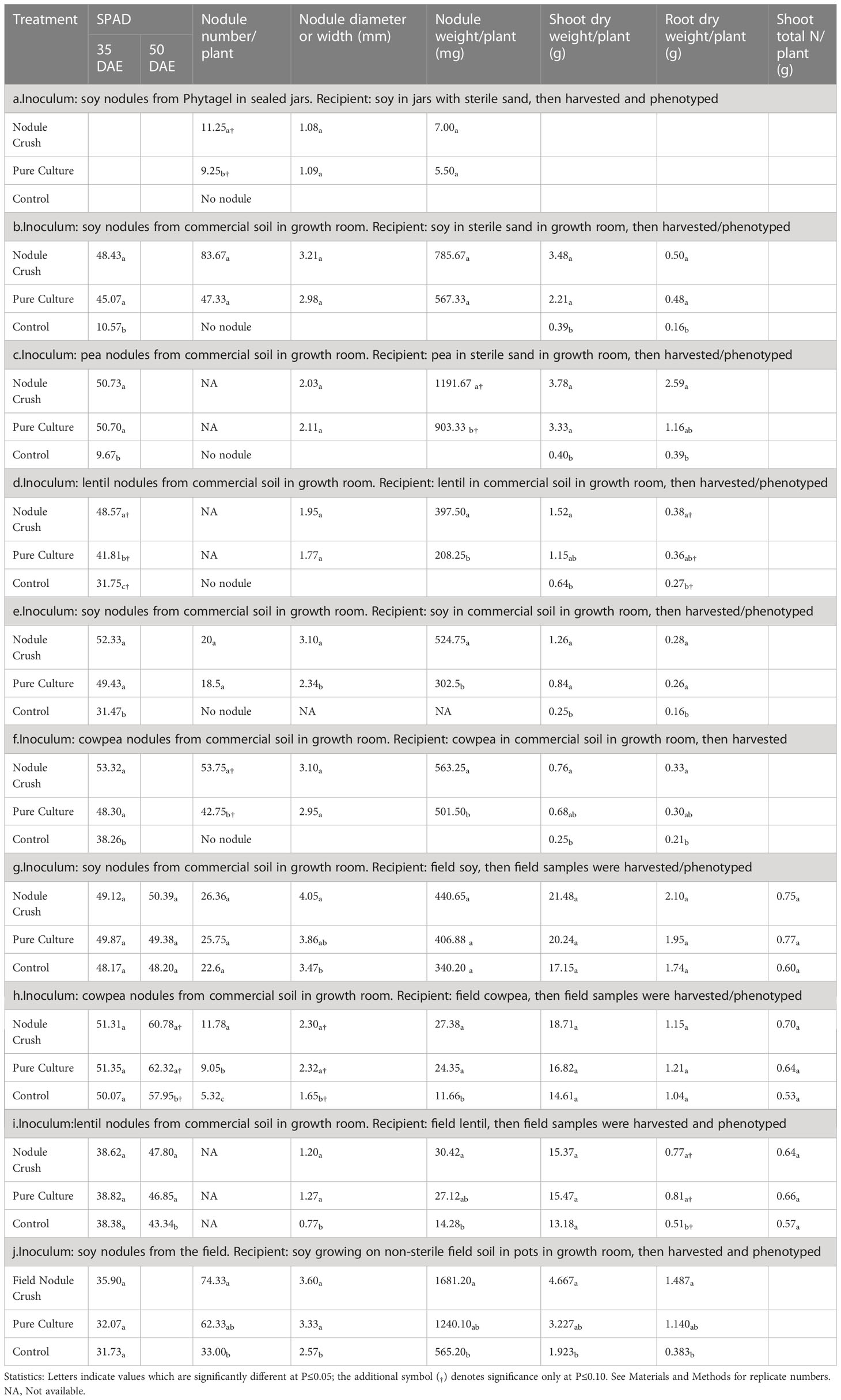
Table 1 Effectiveness of crushing legume root nodules onto seeds to transfer rhizobia compared to pure rhizobia inoculant (positive control) or buffer (negative control).
3.2 Field Trials
Critically, nodules from lentil, soy and cowpea grown indoors on non-sterile commercial soil were crushed onto seeds, then sown in a field which historically had soybean (and soybean-compatible rhizobia) but not lentil or cowpea (Figure 1N). The nodule crush treated plants showed dramatic increases in nodule weight compared to buffer-inoculated controls in lentil and cowpea (Tables 1g–i; cowpea in Figures 1O, P). This field experiment mimicked a scenario of a farmer introducing a new legume species (i.e. cowpea) to his/her farm. Interestingly, the field-grown cowpea showed a significantly higher percentage (p ≤ 0.05) of pink nodules when inoculated with crushed nodules (42%; Figures 1S, T) compared to the buffer inoculation control (12%; Figures 1Q, R), indicative of active leghaemoglobin and nitrogen fixation (Mendoza-Suárez et al., 2021). This colour difference was not observed in lentil or soy (data not shown). Nodule-crushing was equally effective (p ≤ 0.05) as pure-inoculant (42% versus 37%, respectively) in terms of achieving pink nodules in field-grown cowpea.
3.3 Viability of field-derived nodules
Finally, we tested whether field-derived nodules could be a viable source of rhizobia for the nodule crushing procedure. Soybean nodules were harvested from the field and crushed onto soybean seeds indoors. Compared to the buffer inoculation control, nodule crushing from field-derived nodules significantly (p ≤ 0.05) increased nodule number and weight, and root and shoot biomass (Table 1j; Figures S1C, D). Furthermore, these plants were healthy and showed no visible disease symptoms above ground or below ground (Figures S1C, D).
4 Discussion
Effective rhizobia bacteria have the potential to improve the yield and protein content of grain legumes (Murphy-Brokern et al., 2017; Allito et al., 2020), critical for the nearly one billion people worldwide who suffer from deficiencies in amino acids such as lysine, methionine and tryptophan, primarily in SubSaharan Africa and South Asia (Schönfeldt and Hall, 2012; Food Security Information Network, 2022). By enhancing biological nitrogen fixation, effective rhizobia can also reduce the usage of synthetic nitrogen fertilizer which consumes natural gas and contributes to climate change (Guo et al., 2022). Rhizobia can deliver fixed nitrogen directly inside plants, bypassing leaching associated with chemical fertilizers, which is especially problematic on the sandy soils prevalent in the African subtropic (Syers et al., 1996). Despite their promise, however, effective and viable rhizobia are not reaching tens of millions of small scale farmers who need them (Bala et al., 2011; Vanlauwe et al., 2019). Here, we demonstrated in 10 independent experiments, including under field conditions, that the nodulation and chlorophyll content of diverse legume crops can be improved simply by crushing nodules containing effective rhizobia onto seeds. Critically, results from cowpea field experiments demonstrated that nodule crushing can deliver functional rhizobia, i.e. resulting in pink nodules, to a crop that is newly introduced to a region and hence may lack such rhizobia in local soils (Vanlauwe et al., 2019; Mendoza-Suárez et al., 2021). The loamy soil used in our field trial contained much more nitrogen than exists on the depleted sandy soils of many low-input subsistence farmers (Hamarashid et al., 2010), and thus the potential benefit of nodule crushing for them would likely be greater than shown here. Based on these results, when a legume is introduced to subsistence farmers in a region lacking compatible rhizobia, we propose they can now scale up effective rhizobia, including elite, exotic strains, simply by crushing and smearing nodules of healthy legume plants onto seeds.
4.1 The potential of nodule crushing to help small scale farmers
In this study, we successfully used Bradyrhizobium japonicum strain USDA 110 to transfer rhizobia onto soybean seeds using nodule crushing. Studies have shown that inoculation with USDA 110, which is considered an elite, exotic rhizobia, can improve yields of soybean varieties in Africa (Chibeba et al., 2018; van Heerwaarden et al., 2018). Today, soybean is starting to spread across Africa (Vanlauwe et al., 2019; Food and Agriculture Organization of United Nations, 2022; Foyer et al., 2019) and compatible rhizobia inoculants are needed to allow the high yield and protein potential of this crop to be achieved. Nodules as a local commodity reduce some of the challenges associated with the distribution of bacteria which are invisible, require specialized training and infrastructure (Bala et al., 2011; Raimi et al., 2021). In some legume crops including soybean, nodules are large (>3 mm in diameter) and hence visible to the naked eye; a low cost magnifying glass/sheet may help to detect smaller nodules. A single legume plant can produce >100 nodules (under low nitrogen), with only a single soybean nodule required to inoculate 50 seeds in our experience.
Nodule crushing should be an easily teachable and scalable technique; indeed, picture-based lessons already exist to train low-literacy subsistence farmers how to excavate and select active nodules (Raizada and Smith, 2016; Devkota et al., 2020). After introduction of an elite/exotic strain and basic training (extension) to early adopting farmers, the farmers themselves could train and make their nodules available to family and friends, who could then spread subsequent nodules to their networks, thus diffusing inoculants in a geographic region. Small scale farmers could also trade or sell nodules as for-profit microenterprises, similar to the long-held practice by indigenous peoples of sharing and selling seeds (Vernooy et al., 2015).
The other materials required for the nodule crushing technique are all available in many villages and inexpensive. These materials include local alcohols as nodule surface disinfectants, boiled water as buffer for crushing nodules, and Gum Arabic, molasses or other local sticky agents to adhere inoculants to seeds (Somasegaran and Hoben, 1994).
4.2 Challenges of using nodule crushing to help farmers especially pertaining to exotic rhizobia
Despite the promise of nodule crushing to help small scale farmers, there may be constraints especially pertaining to elite, exotic rhizobia. First, nodules may not possess viable rhizobia or the rhizobia may not retain viability after harvesting. Our preliminary experiments show that crushed soybean (determinate) and pea (indeterminate) nodules can grow abundant, viable rhizobia, respectively, after 30 days and 15 days of nodule storage at room temperature (Figures S1E–N), which is sufficient time to harvest and distribute/sell nodules locally. However, longer-term storage trials are needed, perhaps with dried nodules, and under warm temperatures. This is important, because legume production is seasonal, and hence nodule bacteria need to remain viable between seasons. Alternatively, we propose that small homestead nurseries could serve as living sources of nodules to mitigate storage challenges and for pre-season scaling-up.
The second challenge is the risk that nodule crushing will accidentally spread pathogens, nodule-occupying “cheater” bacteria, and/or simply a diversity of less beneficial rhizobia strains (Hartman et al., 2017; Sharaf et al., 2019). A rich carbon source (e.g. molasses) used to adhere rhizobia to seeds may also promote pathogens (Talbot, 2010). These represent significant concerns which will require farmers to be trained to be vigilant and to take precautions (e.g. using boiled water to sterilize containers), concomitment with the development of effective yet simple extension materials. After multiple experiments, we have never observed disease symptoms on plants after nodule crushing, including following the use of field-derived nodules (e.g. Figures S1C, D). Whether stored nodules can become subsequently contaminated with pathogens needs to be investigated under real world conditions. However, inherent to the nodule crushing strategy is training farmers to select the healthiest, greenest plants and pinkest nodules, as nodule donors, which should empower farmers to select against pathogens at each crop generation. Critically, the alternative to nodule crushing is the current paradigm of shipping pure rhizobia to small scale farmers across long distances without refrigeration on solid carriers such as peat – often resulting in contaminated inoculants (Herridge et al., 2014). In terms of molasses (e.g. jaggery), it promotes rhizobia growth and is already used in current inoculant strategies (Singh et al., 2011; Singh et al., 2014) and in compost teas (Scheuerell and Mahaffee, 2002), but as already noted, there are less carbon-rich sticky agents available locally such as Gum Arabic in Africa which may prove to be safer alternatives.
A third challenge is that inoculation with elite rhizobia may not necessarily always improve biological nitrogen fixation (Thilakarathna and Raizada, 2016). Exotic strains are sometimes genetically incompatible with local legume landraces/varieties, not adapted to local soils or environmental conditions (e.g. temperature, pH), and/or are outcompeted by local strains (Deaker et al., 2004; Thilakarathna and Raizada, 2016; Mendoza-Suárez et al., 2021).
4.3 Potential of nodule crushing to improve indigenous rhizobia
Given the above challenges, the greatest potential of nodule crushing might be to catalyze the improvement of indigenous rhizobia. As noted above, indigenous rhizobia strains are already adapted to the local environment and sometimes highly competitive compared to exotic strains (Thilakarathna and Raizada, 2016; Nyaga and Njeru, 2020; Mendoza-Suárez et al., 2021). In some cases, indigenous rhizobia, if compatible with the crop, have been shown to result in greater yield than exotic elite strains under field conditions when delivered as an inoculant (Hungria et al., 2006; Thilakarathna and Raizada, 2016; Thilakarathna et al., 2019; Nyaga and Njeru, 2020). We propose that smallholders can now improve their indigenous rhizobia, possibly regardless of their initial efficacy, by repeated cycles of nodule crushing from the healthiest plants and their pinkest nodules available in their fields. Previously, a Ralstonia pathogen was evolved into a nodule-forming Mimosa symbiont, initially by synthetic introduction of a symbiosis plasmid, followed by sequential rounds of nodule crushing/selection under laboratory conditions (Guan et al., 2013; Remigi et al., 2014; Marchetti et al., 2017). Here, we demonstrated that field-derived soybean nodules from healthy plants resulted in healthy green plants following nodule crushing onto seeds; the next-generation plants possessed healthy nodules (Table 1j; Figures S1C, D). This result infers the viability of farmers practicing repeated cycles of nodule crushing to improve indigenous rhizobia. Over centuries, subsistence farmers have domesticated and improved indigenous crops (Hufford et al., 2019); but since seeds do not transfer rhizobia inoculant (Chartrel et al., 2021; Moroenyane et al., 2021), the traditional crop selection could not simultaneously improve such rhizobia directly. Nodule crushing may now enable farmers to directly select and breed beneficial indigenous rhizobia for the first time. Farmers could potentially also use nodule crushing to adapt promising exotic rhizobia to local soils, environments and crops, thus indigenizing elite strains.
4.4 Future Perspectives
Moving forward, nodule crushing has the potential to decentralize rhizobia inoculants, catalyze farmer experimentation, improve the yields of traditional crop landraces which often receive little attention from breeders (Li et al., 2020; Popoola et al., 2022), improve farmer access to indigenous microbial biodiversity (Vanlauwe et al., 2019), and empower farmers by enabling microenterprises. Ultimately this technology can reduce the need for fossil fuel requiring synthetic nitrogen fertilizers (Kebede, 2021), while improving the nutrition, income and resilience of subsistence farmers globally.
Data availability statement
The datasets presented in this study can be found in online repositories. The names of the repository/repositories and accession number(s) can be found below: https://www.ncbi.nlm.nih.gov/genbank/, PRJNA930900.
Author contributions
RP designed and conducted all experiments, analyzed the data and wrote the manuscript. OH assisted with the indoor experiments. MR conceived the study and edited the manuscript. All authors contributed to the article and approved the submitted version.
Funding
Funding was provided by grants to MR from the Ontario Ministry of Agriculture, Food and Rural Affairs (OMAFRA) and the Natural Sciences and Engineering Research Council (NSERC) of Canada.
Acknowledgments
We thank Dylan Brettingham, Erik Glemser, Jodi Miles, Jake Gregory, Travis Goron, Kamal Khadka, Manar Al-Janabi and Eman Khalaf for technical assistance (University of Guelph, Canada). We thank Prof. Istvan Rajcan (University of Guelph, Canada) for the gift of soybean seeds. RP is a recipient of the Arrell Graduate Scholarship (University of Guelph).
Conflict of interest
The authors declare that the research was conducted in the absence of any commercial or financial relationships that could be construed as a potential conflict of interest.
Publisher’s note
All claims expressed in this article are solely those of the authors and do not necessarily represent those of their affiliated organizations, or those of the publisher, the editors and the reviewers. Any product that may be evaluated in this article, or claim that may be made by its manufacturer, is not guaranteed or endorsed by the publisher.
Supplementary material
The Supplementary Material for this article can be found online at: https://www.frontiersin.org/articles/10.3389/fagro.2023.1161978/full#supplementary-material
References
Allito B. B., Ewusi-Mensah N., Logah V. (2020). Legume-rhizobium strain specificity enhances nutrition and nitrogen fixation in faba bean (Vicia faba l.). Agron. 10, 10060826. doi: 10.3390/agronomy10060826
Bala A., Karanja N., Murwira M., Lwimbi L., Abaidoo R., Giller K. (2011). Production and use of rhizobial inoculants in Africa. N2Africa Project. 21pp. Available at: https://www.n2africa.org/sites/default/files/N2Africa_Production%20and%20use%20of%20rhizobial%20inoculants%20in%20Africa_0.pdf.
Brunelle T., Dumas P., Souty F., Dorin B., Nadaud F. (2015). Evaluating the impact of rising fertilizer prices on crop yields. Agric. Econ. 46, 653–666. doi: 10.1111/agec.12161
Chartrel V., Dugat-Bony E., Sarthou A. S., Huchette S., Bonnarme P., Irlinger F. (2021). The microbial community associated with pea seeds (Pisum sativum) of different geographical origins. Plant Soil. 462, 405–427. doi: 10.1007/s11104-021-04856-6
Chibeba A. M., Kyei-Boahen S., Guimarães M., de F., Nogueira M. A., Hungria M. (2018). Feasibility of transference of inoculation-related technologies: A case study of evaluation of soybean rhizobial strains under the agro-climatic conditions of Brazil and Mozambique. Agric. Ecosys. Environ. 261, 230–240. doi: 10.1016/j.agee.2017.06.037
Deaker R., Roughley R. J., Kennedy I. R. (2004). Legume seed inoculation technology - a review. Soil Biol. Biochem. 36, 1275–1288. doi: 10.1016/j.soilbio.2004.04.009
Devkota R., Odame H. H., Fitzsimons J., Pudasaini R., Raizada M. N. (2020). Evaluating the effectiveness of picture-based agricultural extension lessons developed using participatory testing and editing with smallholder women farmers in Nepal. Sustainability. 12, 9699. doi: 10.3390/su12229699
Food and Agriculture Organization of United Nations. (2022). FAOSTAT statistical database. Available at: https://www.fao.org/faostat/en/#data (Accessed November 20, 2022).
Food Security Information Network. (2022). Global report on food crises 2022. Available at: https://www.fao.org/3/cb9997en/cb9997en.pdf.
Foyer C. H., Siddique K. H. M., Tai A. P. K., Anders S., Fodor N., Wong F. L., et al. (2019). Modelling predicts that soybean is poised to dominate crop production across Africa. Plant Cell Environ. 42, 373–385. doi: 10.1111/pce.13466
Guan S. H., Gris C., Cruveiller S., Pouzet C., Tasse L., Leru A., et al. (2013). Experimental evolution of nodule intracellular infection in legume symbionts. ISME J. 7, 1367–1377. doi: 10.1038/ismej.2013.24
Guo K., Yang J., Yu N., Luo L., Wang E. (2022). Biological nitrogen fixation in cereal crops: progress, strategies, and perspectives. Plant Commun. 4, 100499. doi: 10.1016/j.xplc.2022.100499
Hamarashid N. H., Othman M. A., Hussain M. H. (2010). Effects of soil texture on chemical compositions, microbial populations and carbon mineralization in soil. Egypt. J. Exp. Biol. (Bot.). 6, 59–64.
Hartman K., van der Heijden M. G. A., Roussely-Provent V., Walser J. C., Schlaeppi K. (2017). Deciphering composition and function of the root microbiome of a legume plant. Microbiome. 5, 2. doi: 10.1186/s40168-016-0220-z
Herridge D. F., Hartley E., Gemell L. G. (2014). Rhizobial counts in peat inoculants vary amongst legume inoculant groups at manufacture and with storage: implications for quality standards. Plant Soil. 380, 327–336.
Howieson J. G., Dilworth M. J. (2016). “Working with rhizobia,” in Australian Centre for International Agricultural Research (Canberra: ACIAR Monograph), 173.
Hufford M. B., Berny Mier Y Teran J. C., Gepts P. (2019). Crop biodiversity: an unfinished magnum opus of nature. Annu. Rev. Plant Biol. 70, 727–751. doi: 10.1146/annurev-arplant-042817
Hungria M., Franchini J. C., Campo R. J., Crispino C. C., Moraes J. Z., Sibaldelli R. N. R., et al. (2006). Nitrogen nutrition of soybean in Brazil: Contributions of biological N2 fixation and n fertilizer to grain yield. Can. J. Plant Sci. 86, 927–939. doi: 10.4141/P05-098
Kebede E. (2021). Contribution, utilization, and improvement of legumes-driven biological nitrogen fixation in agricultural systems. Front. Sustain. Food Syst. 5, 767998. doi: 10.3389/fsufs.2021.767998
Khalaf E. M., Shrestha A., Rinne J., Lynch M. D. J., Shearer C. R., Limay-Rios V., et al. (2021). Transmitting silks of maize have a complex and dynamic microbiome. Sci. Rep. 11, 13215. doi: 10.1038/s41598-021-92648-4
Lal R. (2021). “Soil health and human nutrition,” in The soil-human health nexus. Ed. Lal R. (Boca Raton, FL: CRC Press), 315–326.
Li X., Yadav R., Siddique K. H. M. (2020). Neglected and underutilized crop species: the key to improving dietary diversity and fighting hunger and malnutrition in Asia and the pacific. Front. Nutr. 7, 593711. doi: 10.3389/fnut.2020.593711
Marchetti M., Clerissi C., Yousfi Y., Gris C., Bouchez O., Rocha E., et al. (2017). Experimental evolution of rhizobia may lead to either extra- or intracellular symbiotic adaptation depending on the selection regime. Mol. Ecol. 26, 1818–1831. doi: 10.1111/mec.13895
Mendoza-Suárez M., Andersen S. U., Poole P. S., Sánchez-Cañizares C. (2021). Competition, nodule occupancy, and persistence of inoculant strains: key factors in the rhizobium-legume symbioses. Front. Plant Sci. 12, 690567. doi: 10.3389/fpls.2021.690567
Moroenyane I., Tremblay J., Yergeau E. (2021). Soybean microbiome recovery after disruption is modulated by the seed and not the soil microbiome. Phytobiomes J. 5, 418–431. doi: 10.1094/PBIOMES-01-21-0008-R
Murphy-Brokern D., Peeters A., Westhoek H. (2017). “The role of legumes in bringing protein to the table,” in Legumes in cropping systems. Eds. Murphy-Bokern D., Stoddard F. L., Watson C. A. (Wallingford: CAB International), 18–36.
Nyaga J. W., Njeru E. M. (2020). Potential of native rhizobia to improve cowpea growth and production in semiarid regions of Kenya. Front. Agron. 2, 606293. doi: 10.3389/fagro.2020.606293
Popoola J. O., Aworunse O. S., Ojuederie O. B., Adewale B. D., Ajani O. C., Oyatomi O. A., et al. (2022). The exploitation of orphan legumes for food, income, and nutrition security in Sub-Saharan Africa. Front. Plant Sci. 13, 782140. doi: 10.3389/fpls.2022.782140
Raimi A., Roopnarain A., Adeleke R. (2021). Biofertilizer production in Africa: Current status, factors impeding adoption and strategies for success. Sci. Afric. 11, e00694. doi: 10.1016/j.sciaf.2021.e00694
Raizada M. N., Smith L. (2016). A picture book of best practices for subsistence farmers: Edition: Sub Saharan Africa/Carribbean (Guelph, Canada: University of Guelph Sustainable Agriculture Kit (SAK) Project). Available at: www.SAKBooks.com.
Remigi P., Capela D., Clerissi C., Tasse L., Torchet R., Bouchez O., et al. (2014). Transient hypermutagenesis accelerates the evolution of legume endosymbionts following horizontal gene transfer. PloS Biol. 12, e1001942. doi: 10.1371/journal.pbio.1001942
Samberg L. H., Gerber J. S., Ramankutty N., Herrero M., West P. C. (2016). Subnational distribution of average farm size and smallholder contributions to global food production. Environ. Res. Lett. 11, 124010. doi: 10.1088/1748-9326/11/12/124010
Scheuerell S., Mahaffee W. (2002). Compost tea: Principles and prospects for plant disease control. Compost Sci. Util. 10, 313–338. doi: 10.1080/1065657X.2002.10702095
Schönfeldt H. C., Hall N. G. (2012). Dietary protein quality and malnutrition in Africa. Br. J. Nutr. 108, 69–76. doi: 10.1017/S0007114512002553
Senthilkumar M., Amaresan N., Sankaranarayanan A. (2021). “Isolation, characterization, and preservation of rhizobia from root nodules of legumes,” in Plant-microbe interactions: Springer Protocols Handbooks (LLC: Springer Science Business Media), 3–8. doi: 10.1007/978-1-0716-1080-0_1
Sharaf H., Rodrigues R. R., Moon J., Zhang B., Mills K., Williams M. A. (2019). Unprecedented bacterial community richness in soybean nodules vary with cultivar and water status. Microbiome. 7, 63. doi: 10.1186/s40168-019-0676-8
Singh S., Gupta G., Khare E., Behal K. K., Arora N. K. (2014). Effect of enrichment material on the shelf life and field efficiency of bioformulation of rhizobium sp. and p-solubilizing Pseudomonas fluorescens. Sci. Res. Rep. 4, 44–50.
Singh A. K., Singh G., Bhatt R. P., Pant S., Naglot A., Singh L. (2011). Sugars waste, an alternative growth and complete medium for fast growing rhizobium cells. Afr. J. Microbiol. Res. 5, 3289–3295. doi: 10.5897/ajmr11.408
Somasegaran P., Hoben H. J. (1994). “Testing the survival of rhizobia on inoculated seeds,” in Handbook for rhizobia (New York: Springer). doi: 10.1007/978-1-4613-8375-8_29
Syers J. K., Lingard J., Pieri C., Ezcurra E., Faure G. (1996). Sustainable land management for the semiarid and subhumid tropics. Ambio. 25, 484–491.
Talbot N. J. (2010). Living the sweet life: How does a plant pathogenic fungus acquire sugar from plants? PloS Biol. 8, 2. doi: 10.1371/journal.pbio.1000308
Thilakarathna M. S., Chapagain T., Ghimire B., Pudasaini R., Tamang B. B., Gurung K., et al. (2019). Evaluating the effectiveness of rhizobium inoculants and micronutrients as technologies for Nepalese common bean smallholder farmers in the real-world context of highly variable hillside environments and indigenous farming practices. Agric. 9, 20. doi: 10.3390/agriculture9010020
Thilakarathna M. S., Raizada M. N. (2016). A meta-analysis of the effectiveness of diverse rhizobia inoculants on soybean traits under field conditions. Soil Biol. Biochem. 105, 177–196. doi: 10.1016/j.soilbio.2016.11.022
van Heerwaarden J., Baijukya F., Kyei-Boahen S., Adjei-Nsiah S., Ebanyat P., Kamai N., et al. (2018). Soyabean response to rhizobium inoculation across sub-Saharan Africa: Patterns of variation and the role of promiscuity. Agric. Ecosys. Environ. 261, 211–218. doi: 10.1016/j.agee.2017.08.016
Vanlauwe B., Hungria M., Kanampiu F., Giller K. E. (2019). The role of legumes in the sustainable intensification of African smallholder agriculture: Lessons learnt and challenges for the future. Agric. Ecosys. Environ. 284, 106583. doi: 10.1016/j.agee.2019.106583
Vernooy R., Shrestha P., Sthapit B. (2015). Community seed banks: origins, evolution and prospects (New York: Routledge). Available at: www.bioversityinternational.org.
Wu G., Bazer F. W., Cross H. R. (2014). Land-based production of animal protein: Impacts, efficiency, and sustainability. Ann. N. Y. Acad. Sci. 1328, 18–28. doi: 10.1111/nyas.12566
Keywords: Africa, cowpea, inoculant, lentil, biological nitrogen fixation, pea, rhizobia, soybean
Citation: Pudasaini R, Hewedy OA and Raizada MN (2023) Improving field legume nodulation by crushing nodules onto seeds: implications for small-scale farmers. Front. Agron. 5:1161978. doi: 10.3389/fagro.2023.1161978
Received: 09 February 2023; Accepted: 31 March 2023;
Published: 14 April 2023.
Edited by:
Maria J. Delgado, Spanish National Research Council (CSIC), SpainReviewed by:
Jean-jacques Drevon, DEVA Domaine Experimental du Val d Ainan, FranceJohn M. Maingi, Kenyatta University, Kenya
Copyright © 2023 Pudasaini, Hewedy and Raizada. This is an open-access article distributed under the terms of the Creative Commons Attribution License (CC BY). The use, distribution or reproduction in other forums is permitted, provided the original author(s) and the copyright owner(s) are credited and that the original publication in this journal is cited, in accordance with accepted academic practice. No use, distribution or reproduction is permitted which does not comply with these terms.
*Correspondence: Manish N. Raizada, cmFpemFkYUB1b2d1ZWxwaC5jYQ==