- Dept. of General and Applied Biology, Biochemistry Building, Institute of Biosciences, State University of São Paulo, Rio Claro, SP, Brazil
Plant Essential Oils and their constituents are well-known for their properties as antimicrobial agents and are labeled as Generally Recognized as Safe (GRAS), prompting studies around their usage in the control of food-borne microorganisms and phytopathogens. In this study, we evaluated Oregano Essential Oil (OEO), Thymol (THY) and Carvacrol (CAR) for the control of Xanthomonas citri subsp. citri (X. citri). In vitro antibacterial assays revealed that CAR and THY inhibit X. citri growth at the concentrations of 100 µg.mL-1 and 114 µg.mL-1, lower than OEO (136 µg.mL-1). Bactericidal effects were observed at 400 µg.mL-1 for OEO and 200 ug.mL-1 for CAR and THY. Investigating potential cellular targets for the compounds showed that after 30 minutes of exposure up to 84% of the cells had their membranes disrupted, implicating the membrane as the primary target. Phytotoxicity evaluations using Lactuca sativa and Solanum lycopersicum seeds showed an acute toxic effect in all treatments above 200 µg.mL-1, except for OEO and THY in S. lycopersicum at lower concentrations. Regarding their protective effect on citrus leaves, CAR showed no effect when compared to the untreated control (0.39 and 0.50 lesions per cm2, respectively). OEO and THY were able to reduce significantly citrus canker symptoms (0.18 and 0.11 lesions per cm2, respectively). In addition, no toxic effects were observed on citrus leaves in all treatments. THY inhibits X. citri growth and the development of citrus canker lesions. These results show that THY as a viable alternative to be used in citrus canker management.
Introduction
According to the latest FAO (Food and Agriculture Organization - United Nations) report, around 143 million tons of citrus fruit were produced worldwide in 2019, with an increase of around 10 million tons from the last report, where China, Brazil and the USA are present as the leading producers (FAO, 2021). Even as one of the most widespread cultures in tropical and subtropical regions, several pathogens are known to threaten citrus production (Poveda et al., 2021; Syed-Ab-Rahman et al., 2022). Among all, the bacterium Xanthomonas citri subsp. citri, the causative agent of Citrus Canker, is known to affect all commercial citrus varieties, being responsible for significant losses in productivity and fruit quality (Behlau, 2021; Naqvi et al., 2022).
Symptoms of Citrus canker manifest initially in plants aerial parts as corky and brownish raised pustules, that can evolve, in more severe cases, to defoliation and premature fruit drop (Schubert et al., 2001; Ference et al., 2018; Martins et al., 2020; Naqvi et al., 2022). In addition to the loss of fruit quality and yield, market standards and sanitary regulations lead to further economic losses since fruit harvested in areas where citrus canker is present have commercialization restrictions (Gottwald et al., 2007; Behlau, 2021). Climatic conditions such as rainy weather and heavy winds are the major factors responsible for plant-to-plant transmission of the bacterium (Gottwald et al., 2007; Martins et al., 2020; Naqvi et al., 2022). The control of citrus canker in areas where the disease is present and/or endemic is currently done by integrated management agricultural practices (Ference et al., 2018; Behlau, 2021). These include the use of healthy nursery plants and more resistant cultivars of citrus to start orchards, windbreaks to refrain lateral spreading of the causal agent, control of the citrus leaf miner (Phyllocnistis citrella), which produces wounds in the plant tissue and facilitates infection, and the recurrent application of copper-based formulations as bactericides. Although effective, copper is a cumulative toxic metal, and resistance to it has already emerged in X. citri and many other bacterial pathogens (Canteros, 1999; Ference et al., 2018). In addition, larger markets, such as the European Union, are making efforts to reduce several kinds of pesticides, which may limit the disease control options available to the producers (Behlau et al., 2011; Jacquet et al., 2022). These concerns call for the research and development of new alternative products on par with recent regulations and trusted actions against pathogens.
Essential Oils are aromatic hydrophobic liquids with volatile and oily characteristics obtained from almost all plant organs (flowers, buds, stems, leaves, roots, fruit) or the whole plant (Pandey et al., 2017; Falleh et al., 2020). Due to their antimicrobial properties, among others, and the classification as GRAS (Generally Recognized as Safe), essential oils are excellent candidates to be explored as control agents for pathogens (Burt, 2004; Pandey et al., 2017). Oregano essential oil (OEO) (Origanum vulgare (family: Lamiaceae)) is an effective antimicrobial agent exhibiting a broad spectrum of action, besides some other properties such being an antioxidant and anti-inflammatory (Benavides et al., 2012; Rodriguez-Garcia et al., 2016; Cui et al., 2019; Leonelli Pires de Campos et al., 2022). Its main constituents are the phenolic isomers carvacrol (CAR) and thymol (THY), with concentrations up to 85%, followed by their isomeric precursors p-cymene and γ-terpinene at lower concentrations (Rodriguez-Garcia et al., 2016; Sakkas and Papadopoulou, 2017). OEO and both THY and CAR showed antibacterial properties against Gram-positive and Gram-negative bacteria with some slight variations in their microbicidal concentrations and disruptive effect on the cytoplasmatic membrane (Xu et al., 2008; Memar et al., 2017). Here, we evaluated OEO extracted from Origanum vulgare, together with THY and CAR as potential anti-X. citri agents for the control of citrus canker.
Materials and methods
Bacterial strain and growth conditions
The bacterium Xanthomonas citri subsp. citri used in all the experiments was the isolate 306 (IBSBF 1594). The bacterium was cultivated in liquid and solid NYG medium (5 g.L-1 of peptone, 3 g.L-1 of yeast extract, 2% (v/v) of glycerol) at 29 ± 1°C, with agitation of 200 rpm, when necessary.
Essential oil and its isolated constituents
The OEO was obtained from OSHADI® (lot: 2021-001), according to the company’s report, the gas chromatography (GC) analysis showed that its composition was: 71.0% CAR, 2.3% THY and 26.7% others compounds. The relationship of the two compounds in OEO was confirmed by 1H NMR analysis. The 1H NMR spectrum was performed using a Bruker DRX-400 instrument (400 MHz 1H NMR) and CDCl3 was used as solvent.
THY 98.5% and CAR 98% were purchased from Sigma-Aldrich® (Lot: SLBT5627 and MKCD478, respectively).
Bacterial growth inhibition
The inhibitory concentrations (IC) of OEO, THY, and CAR were determined through resazurin microtiter assay (REMA), described by (Silva et al., 2013). Stock solutions of 40 mg.mL-1 were prepared in absolute ethanol and diluted in NYG medium into a 96 well plate to the final concentrations ranging from 400 to 3.12 µg.mL-1 in a total volume of 100 µL per well. The negative control (NC) was NYG medium, while the positive control (PC) was NYG added with 20 µg.mL-1 kanamycin. The vehicle control (VC) was NYG containing 1% ethanol (v/v), which is the maximum concentration of this solvent present in the treatments after the dilution of the compounds. Bacterium inoculum was added to a final concentration of 105 cells per well and plates were incubated for 16 h at 29 ± 1°C. After the incubation period, aliquots of each well were transferred with a microplate replicator to a petri dish with solid NYG medium. The plate was incubated for 96 h at 29 °C. After the incubation period, plates were inspected visually to detect any bacterial growth and the bactericidal concentration was determined where the lowest concentration in which no bacterial growth was visible. To the remaining bacterial cultures on the microplates, a resazurin (Sigma-Aldrich, Darmstadt Germany) solution was added to the final concentration of 0,015 µg.mL-1/well and plates were further incubated for approximately two hours at 29 ± 1°C. The reduction of resazurin to the fluorescent compound resorufin, which takes place in the presence of live/actively respiring cells, was quantified in a fluorescent plate reader Synergy H1N1 (BioTek, Winooski, VT) set to excitation at 530 nm, and emission at 590 nm. Data were used to plot dose-response curves, and after, a curve was fitted following a dose-response mathematical model described by the equation: y = A1 + (A2-A1)/(1 + 10^((LOGx0-x)*p)), where A1 (bottom asymptote) was fixed at 0 and A2 (top asymptote) was fixed at 100. From this model, the minimal inhibitory concentration needed to inhibit 90% of cell growth (IC90) was estimated. All experiments were carried out in triplicates and repeated three times.
Cell membrane integrity
Action of OEO, THY, and CAR on the bacterial cell membrane was investigated as described by (Caccalano et al., 2021). Treatments were carried out with the compounds at their estimated IC90 values, and during 15 and 30 min of bacterial exposure. Positive control for membrane disruption was done submitting cells to thermal stress for 20 min (Savietto et al., 2018). Following treatments, cells were stained with the fluorescent dyes 4′, 6-diamidino-2-phenylindole (DAPI), that penetrates all the cells, and propidium iodide (IP), which is excluded from the cells with intact membranes. Cells were visualized under the microscope using an Olympus BX61, equipped with a monochromatic camera Orca Flash 2.8 (Hamamatsu). Images were captured with the software CellsSens Dimension (Olympus). Micrographs were analyzed using the software Fiji ImageJ (Schindelin et al., 2012) to determine the number of cells with permeabilized membranes. A total of 200 cells (n=200) were evaluated per treatment. Three independent experiments were performed and statistical analysis were conducted using the software GraphPad Prism version 6 (GraphPad Software, San Diego, CA, USA).
Plant protection assays
Experiments were conducted in a greenhouse during the period of September 21st to November 3rd, 2021. Nursery trees of Citrus sinensis (L.) Osbeck cultivar Pera (grafted on Citrus limon (L.) Osbeck), approximately 60 cm tall, were conditioned into 7 liters plastic bags containing Pinus substrate (Multiplant Citrus, Terra do Paraíso, Holambra, SP, Brazil). Automated fertigation was used with a mixed mineral fertilizer (Amazon AgroSciences Citrus 100) in a volume of approximately 200 mL per plant/day. Temperature and humidity were monitored using a digital thermo hygrometer HOBO MX2305 (Supplementary Figure 1, Supplementary Material). Young citrus leaves of approximately 4 cm in width and 10 cm in length (Cifuentes-Arenas et al., 2018) were sprayed with the compounds at their respective bactericidal concentrations until the run-off point. The positive control for infection was PBS 1X (v/v) (phosphate-buffered saline); the vehicle control was 1% ethanol/water (v/v), and the negative control for infection was the agrochemical Difere (Cu2Cl(OH)3) (Oxiquímica Agrociência - Jaboticabal - SP, Brazil) at 20 µL.mL-1, as recommended by the manufacturer. Followed 24 h of the application of the compounds, plants were spray-covered with a X. citri bacterial suspension at 108 cells.mL-1 (diluted in PBS 1X). Plants were then enclosed with transparent plastic bags for 24 h to keep high humidity and promote bacterial infection. Following 45 days post-inoculation, leaves were detached and digitalized. The number of lesions per leaf area (lesions per cm2) was measured (Cavalca et al., 2020) using the software Fiji ImageJ (Schindelin et al., 2012). Three nursery trees were used per treatment and at least 30 leaves were evaluated per treatment. The data was submitted to a nonparametric statistical analysis of Kruskal-Wallis (Dunn) with three degrees of freedom using the software GraphPad Prism version 6 (GraphPad Software, San Diego, CA, USA).
Phytotoxicity test
A seed germination assay was performed to evaluate the ecotoxicological potential of the OEO and its main components, The experiments were conducted according to Araújo and Monteiro (2005), with modifications. The toxicity of the three compounds was determined independently against seeds of two indicator plants: Lactuca sativa (ISLA®, Lot: 151297-000 S2) and Solanum lycopersicum (ISLA®, Lot: 150629-003 S2).
Treatments were composed of dilutions of the tested compounds prepared in a 1% ethanol solution in sterilized tap water to a final concentration of 400, 200, 100 and 50 µg.mL-1. The negative control (non-toxic) consisted of sterilized tap water and the positive control (toxic) was 0.05M ZnSO4. For each combination of compound/indicator plant, 20 seeds of the indicator plant were distributed evenly into Petri dishes with two layers of sterilized filter paper. Filters were soaked with 2 mL of each solution, plates were sealed with plastic film and incubated for 5 days at 25°C with a cycle of light/dark of 12 hours. Treatments were done in triplicates for each combination (n = 60). After incubation, seed roots were measured and the following equations were used to determine relative seed germination (SG%), relative root elongation (RE%) and germination index (GI):
The test was considered valid when 90% of the seeds in the negative control germinated and 90% of the seeds in the positive control did not (Pecora et al., 2018).
Results
1H NMR analysis of OEO
From the 1H NMR spectrum of OEO (Supplementary Figure 2, Supplementary Material) it is possible to verify the signal of hydrogen H7 from both CAR and THY (Figure 1), and from the integral of these two signals it was possible to determine the percentage of relation between these two compounds. In the spectrum it is possible to observe that the signal of the H7 (septet) of CAR has a chemical shift of 2.87 ppm (Quintanilla-Licea et al., 2014) and an integral equal to 1, whereas the H (septet) of THY has a chemical shift of 3.23 ppm (Wang et al., 2020) and an integral equal to 0.0328. Considering that CAR represents 71.0% of the sample and the integral of the H7 signal was equal to 1, THY with an integral value of 0.0328 shows a percentage of 2.3% of the sample, a result similar to that obtained in the company’s GC analysis.
OEO and its principal constituents inhibit X. citri growth
The ability of OEO, THY and CAR to inhibition X. citri growth was assessed by monitoring the cell respiratory activity after treatments with different concentrations of the compounds. After 16 h of compound exposure, it was observed that OEO, THY and CAR impaired X. citri growth in a dose-response manner. A more pronounced activity was observed starting at 100 µg.mL-1, where, on average, 84% of the cells had their respiratory activities affected. The IC90 of OEO was estimated at ~136 µg.mL-1, whereas CAR and THY showed lower IC90 values of ~100 µg.mL-1 and ~114 µg.mL-1, respectively (Table 1). Following the treatments, the ability of the cells to resume growth was evaluated by spreading bacterial samples onto plain NYG. Plates were incubated for up to 96 h to score for bacterial growth. Bactericidal effects were observed starting at 400 µg.mL-1 for OEO and 200 µg.mL-1 for CAR and THY (Figure 2).

Table 1 Inhibitory concentrations of oregano essential oil, carvacrol and thymol against Xanthomonas citri subsp. citri.
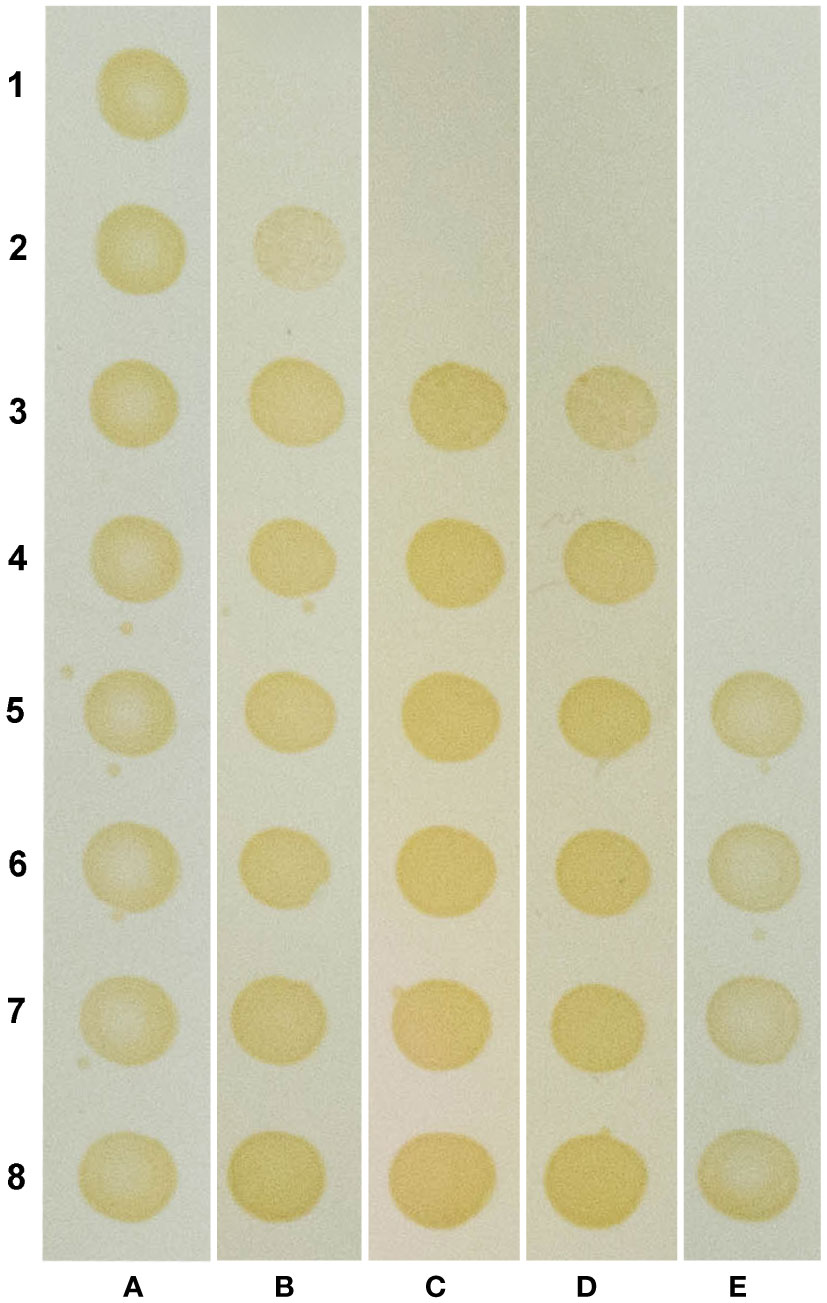
Figure 2 Compounds show a bactericidal effect against X citri. Rows 1 to 8 represent different concentrations of the compounds: 400, 200, 100, 50, 25, 12.5, 6.25 and 3.125 µg.mL -1, respectively. Columns (A–E) represent different treatments: (A) negative control (NC), untreated cells; (B) oregano essential oil (OEO); (C) Carvacrol (CAR); (D) Thymol (THY), and (E) positive control (PC), 20 µg.mL -1 kanamycin (rows E1-E4), and vehicle control (VC), 1% ethanol (rows E5-E6).
OEO, CAR and THY target the membrane of X. citri
In order to investigate some of the possible cellular targets for the compounds, X. citri was exposed to OEO, THY and CAR at their IC90s (OEO, 136 µg.mL-1; CAR, 100 µg.mL-1 and THY, 114 µg.mL-1) and after observed under the microscope to look for signs of morphological alterations and/or membrane permeability (Figure 3). Starting at 15 min of contact, we observed that OEO and THY induced membrane permeabilization in X. citri, with an average number of 94.38% (SD = 1.08) and 87.35% (SD = 0.26) cells affected, respectively (Figure 4). Cells exposed to CAR for 15 min had their membranes affected, but to a lower proportion (M= 65.88%, SD 4.38). After 30 min of exposure, we observed an increase in the average number of cells affected by CAR (M = 89.97%, SD= 5.48) (Figures 3E, F, 4). For OEO, the number of cells with disrupted membranes did not change significantly (M = 93.13% SD = 1.08), while for THY, a small increase was observed (M = 94.44%, SD = 4.07).
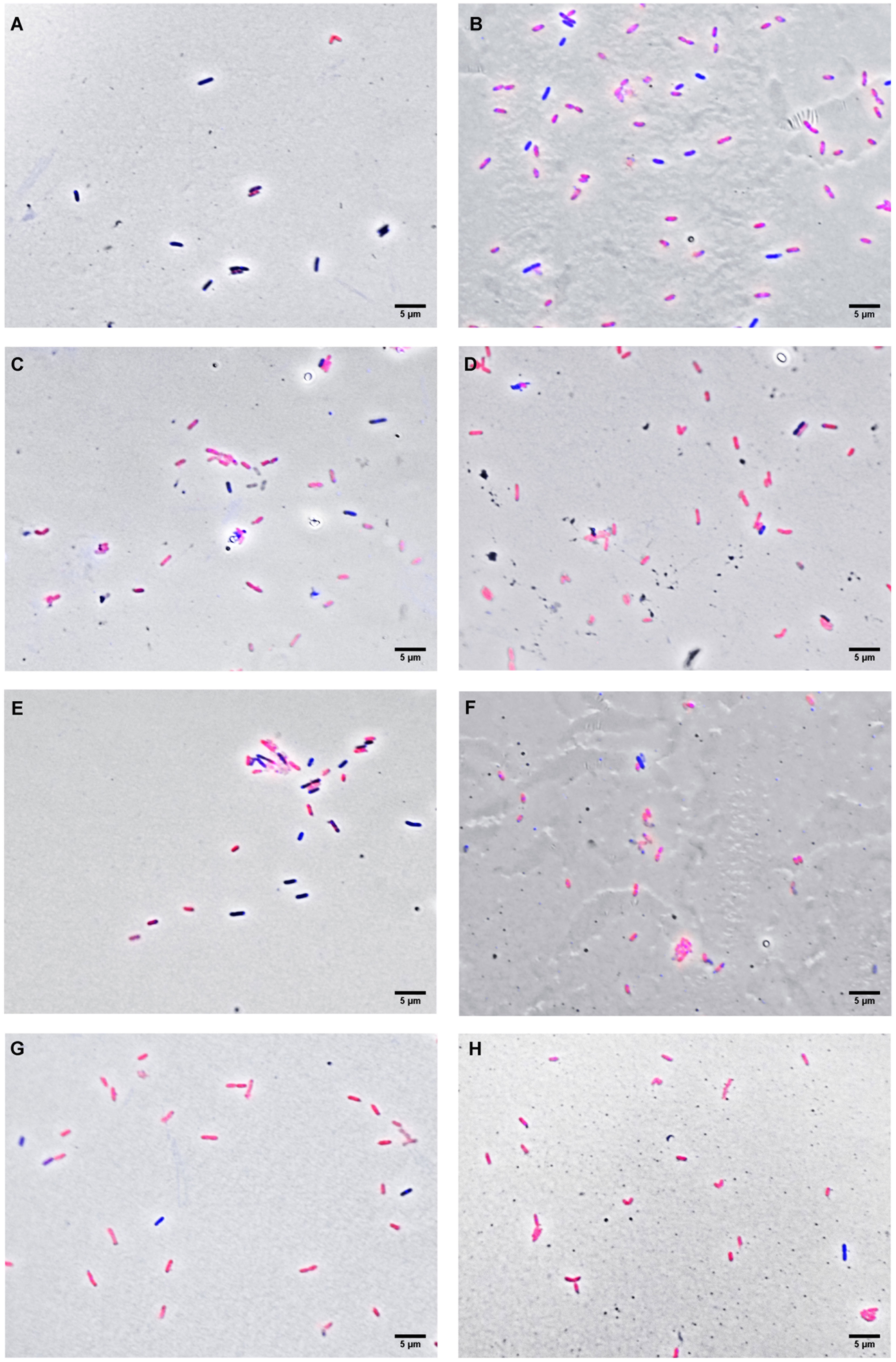
Figure 3 Fluorescence micrograph of X. citri treated with the compounds. (A) untreated cells; (B) the control for membrane disruption (cells submitted to thermal stress); (C) oregano essential oil for 15 min; (D) oregano essential oil for 30 min; (E) carvacrol for 15 min; (F) carvacrol for 30 min (G) thymol for 15 min, and (H) thymol for 30 min. Cells with corrupted membranes were stained with propidium iodide and are artificially colored in red, whereas cells with intact membranes were stained with DAPI (artificially colored in blue). Scale bar: 5 µm; magnification of 100x.
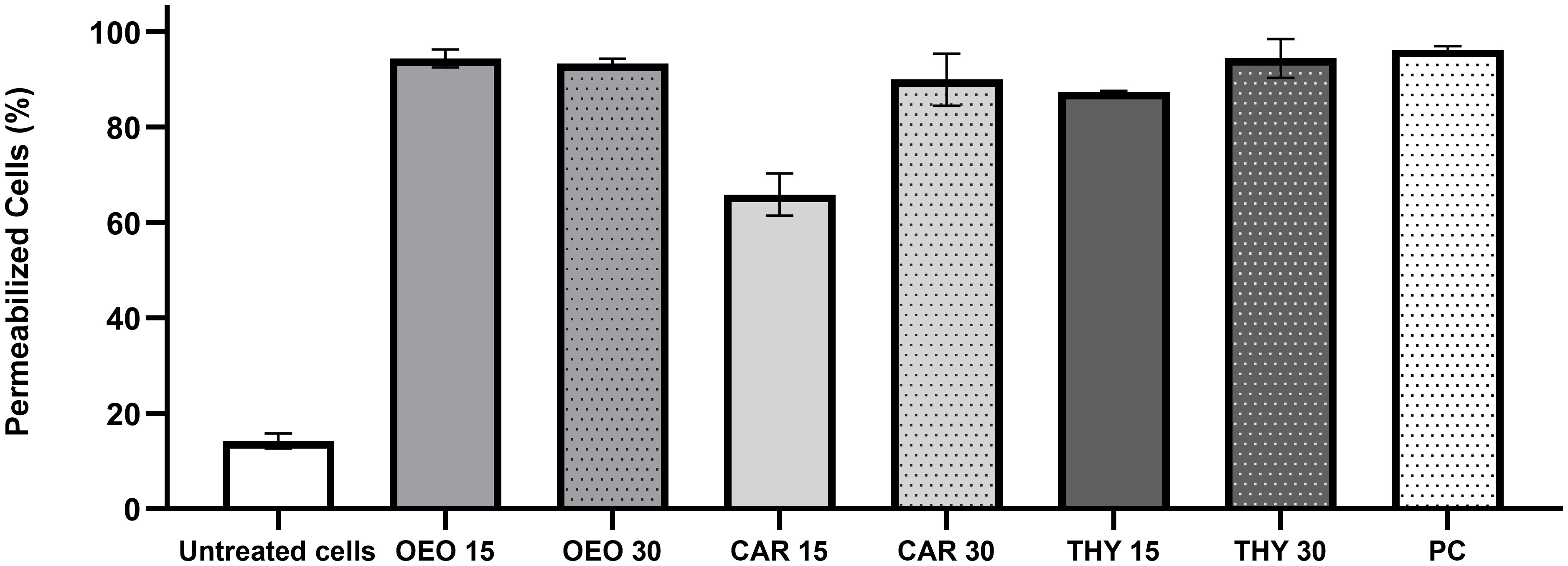
Figure 4 Percentage of X. citri cells with damaged membranes. OEO, oregano essential oil; CAR, carvacrol; THY, thymol; PC, positive control for permeability (cells submitted to thermal stress). Numbers 15 and 30 indicate the duration of the exposure period. Whiskers represent the standard deviation of the mean; 200 cells were analyzed per treatment (n=200).
Moreover, cells were inspected for morphological changes (e.g.: cell elongation, presence of cell chains, and misplaced nucleoids) that could indicate errors in cell division and/or chromosomal segregation upon exposure to the compounds. After 30 minutes of exposure, the average cell length for treated cells with OEO was 1.63 µm (SD = 0.32); CAR 1.68 µm (SD = 0.31) and THY 1.74 µm (SD = 0.31), which did not deviate significantly from untreated cells 1.68 µm (SD = 0.32) (n=200). Furthermore, no other morphological alterations were observed. Therefore, we conclude that the primary target of OEO, CAR and THY in X. citri is the bacterial membrane.
Plant protection
To evaluate if compounds could protect citrus against X. citri infection, nursery trees of a susceptible host (C. sinensis) were spray-covered with OEO, CAR and THY and 24h later challenged with the bacterium. Following 45 days of bacterial infection, we compared the number of lesions per cm2 produced among treatments and controls (Figures 5A, B). Firstly, we did not observe significant statistical differences between untreated plants (PC), plants sprayed with the vehicle (EtOH) and those treated with CAR (adjusted p>0.999). The average number of lesions per cm2 for the untreated plants was 0.5 (95% CI [0.37, 0.62]), plants treated with 1% ethanol 0.37 (95% CI [0.27, 0.46]), and the ones treated with CAR 0.39 (95% CI [0.30, 0.49]) (Figure 5B). However, for the plants treated with OEO, the average number of lesions per cm2 decreased 2.77X (0.18 lesions per cm2, 95% CI [0.12, 0.26]) when compared to the positive control (0.5, 95% CI [0.37, 0.62]). Plants treated with THY exhibited a slightly greater reduction of symptoms, with an average number of lesions per cm2 of 0.11, 95% CI [0.08, 0.14]. Both treatments with OEO and THY were significantly different from the untreated plants (p < 0.05) and similar to the negative control (Difere) (0.06 lesions per cm2, CI 95% [0.02, 0.10]).
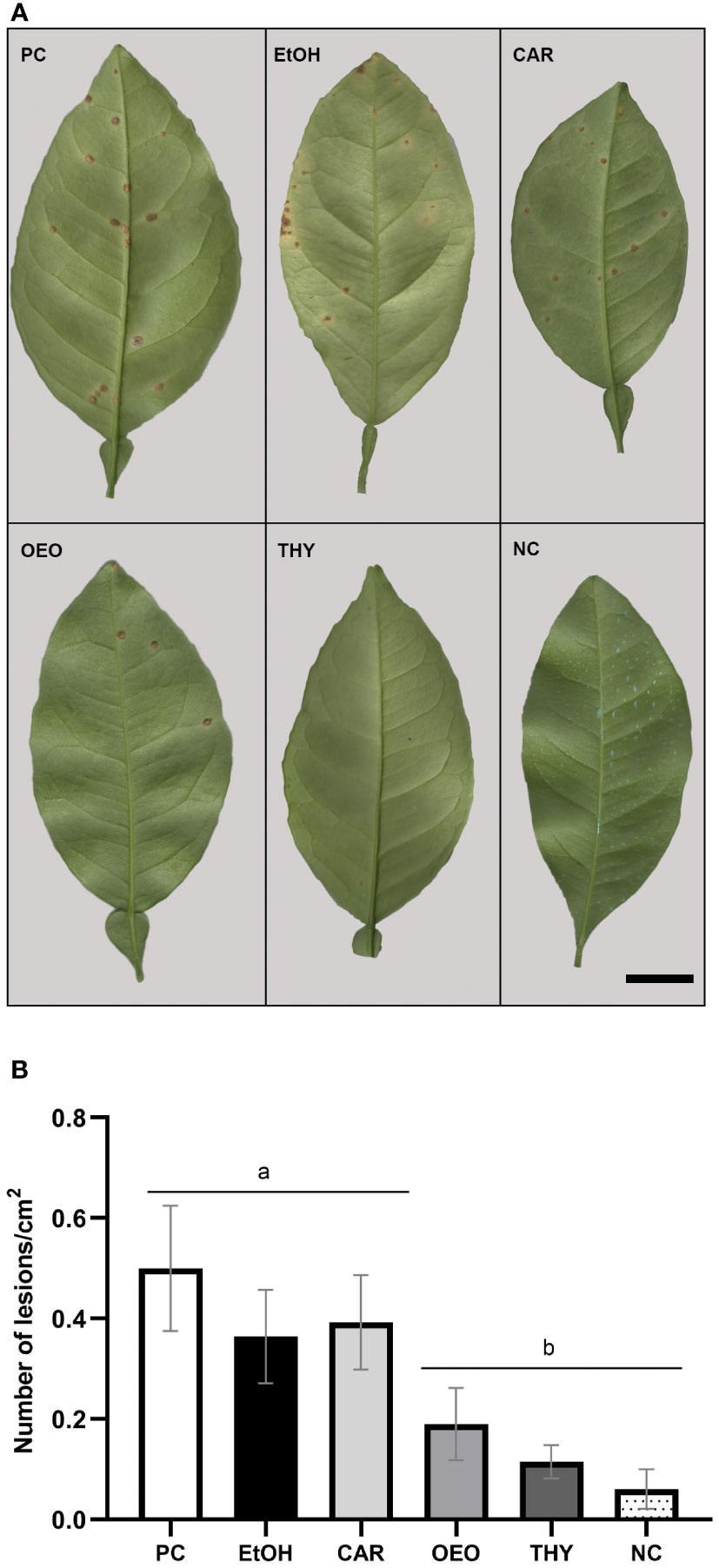
Figure 5 OEO and THY protected citrus nursery trees against X. citri infection. Nursery trees of Citrus sinensis cv. Pera were spray-treated with the compounds at their bactericidal concentrations and after, challenged with the bacterium. Leaves were detached after 45 days for documentation and analyses. (A) Representative leaves with canker lesions for each treatment. Positive control (PC) for infection, sprayed with PBS 1X; Carvacrol (CAR), oregano essential oil (OEO) and thymol (THY) treatments. Nursery trees in the negative control for infection were sprayed with the commercial formulation Difere at 20 µL.mL-1 (NC). Vehicle control (EtOH), 1% ethanol. Scale (black bar): 1 cm. (B) Canker lesion per foliar arear. Bars represent the average number of lesions/cm2 and whiskers the 95% confidence interval. Different letters (a and b) show the statistical difference between treatments (P<0.05).
Phytotoxicity assays
The phytotoxicity of the compounds was evaluated as its ability to inhibit plant development using a relation between seed germination and root elongation in two highly sensitive indicator plants. A phytotoxic effect was observed starting at the lowest concentration of all tested compounds in L. sativa, where the germination index (GI) remained below 40% (Figure 6A). A more pronounced phytotoxic effect was observed for increasing concentrations of OEO, THY and CAR, where the GI remained below 20% at 100 µg.mL-1 and dropped to zero at 200 and 400 µg.mL-1. This effect was due to a complete inhibition in seed germination occurring at the highest concentrations tested for all compounds.
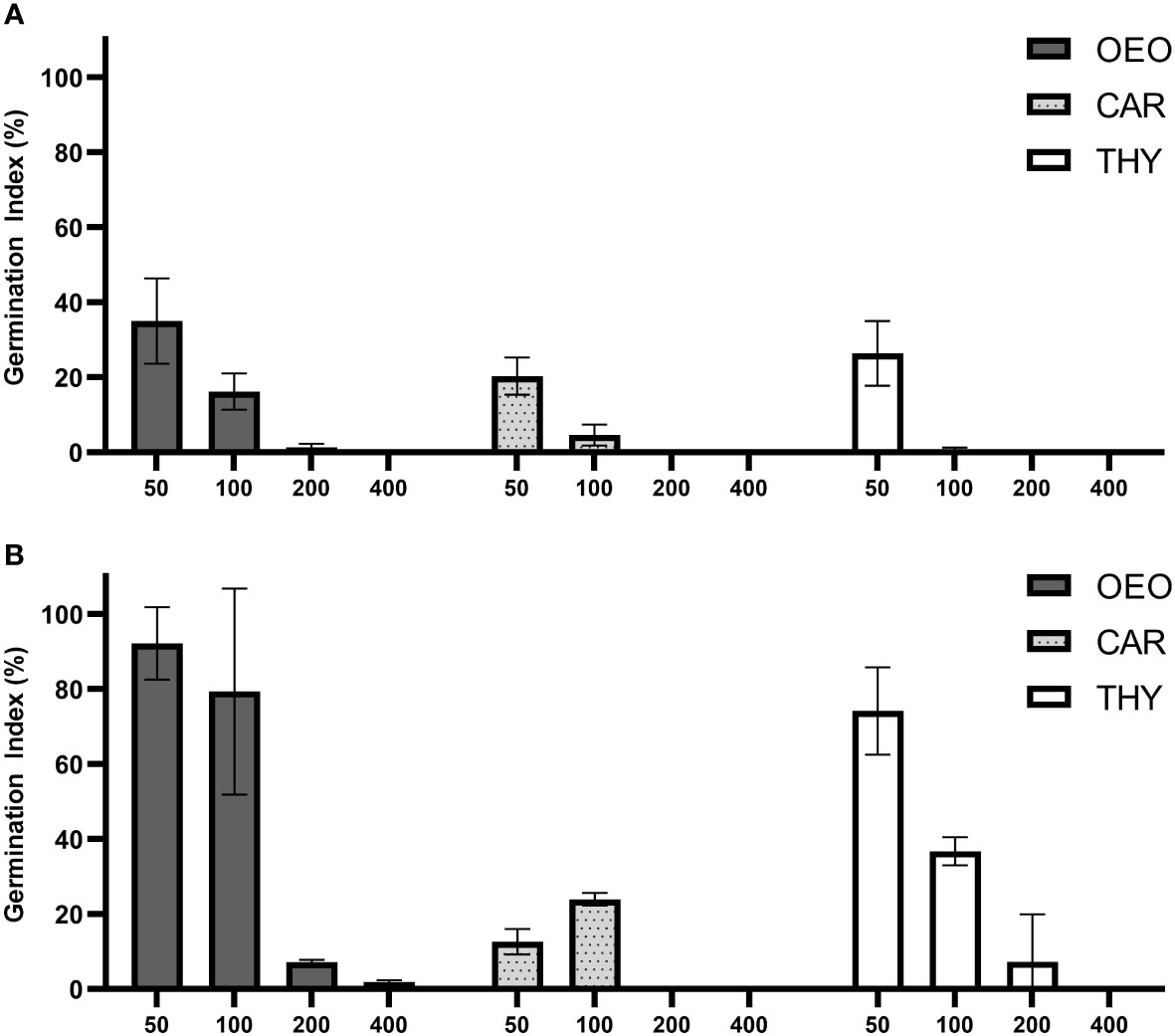
Figure 6 OEO, THY and CAR exhibited phytotoxic effect against seeds of Lactuca sativa and Solanum lycopersicum. Graph of Germination Index (%) of (A) Lactuca sativa and (B) Solanum lycopersicum when exposed to various concentrations of Oregano essential oil (OEO), carvacrol (CAR) and thymol (THY) (400; 200; 100; 50 µg.mL-1). Error bars depict standard deviation between replicates.
In comparison with L. sativa, treatments using S. lycopersicum as an indicator showed similar results for CAR, where a pronounced phytotoxic effect was observed starting at 50 µg.mL-1 (GI < 25%) (Figure 6B). For all the compounds, an acute toxic effect was observed in all treatments above 200 µg.mL-1, but with OEO and THY showing a lower phytotoxic effect in S. lycopersicum at lower concentrations. At 50 µg.mL-1 minor toxic effects in S. lycopersicum were exhibited for OEO and THY (GI > 70%).
Discussion
We showed in the present work that OEO and its two main constituents, THY and CAR, exhibited antibacterial action against X. citri, the etiological agent of citrus canker. THY and CAR showed lower IC90s than OEO, which may be due to the presence of other substances in the oil composition. Still, the antimicrobial activity of OEO is mostly attributed to its two main components THY and CAR (Nostro et al., 2007; Coccimiglio et al., 2016; Kachur and Suntres, 2020).
THY (2-isopropyl-5-methylphenol) and CAR (5-isopropyl-2-methylphenol) are phenolic monoterpene compounds (Trombetta et al., 2005; Peixoto-Neves et al., 2010). In both structures the hydroxyl group is bound to the C-1 position. In THY, the methyl group is attached to the C-5 position and the isopropyl group is attached to the C-2 position; inCAR, the methyl group is attached to the C-2 position and the isopropyl group is attached to the C-5 position. The presence of the hydroxyl group and a system of delocalized electrons is suggested to play an important role for the antimicrobial activity of these compounds (Ultee et al., 2000; Veldhuizen et al., 2006; Kachur and Suntres, 2020).
Bioassays performed with X. citri cells to investigate some of the possible cellular targets of the tested compounds showed that CAR, THY and OEO were able to permeabilize the cytoplasmic membrane of X. citri. THY and CAR are believed to have their antimicrobial action related to their ability to permeabilize and depolarize the bacterial cell membrane in other bacteria (Xu et al., 2008). Due to their hydrophobic nature, these compounds are able to integrate into the membrane, causing disruption and disturbance of normal functions (Lambert et al., 2001; Marinelli et al., 2018), and leading to increased permeability of ATP and increased release of other cellular components (Helander et al., 1998). In addition, CAR and THY have free hydroxyl groups and delocalized electrons that might be responsible for exchanging H+ ions inside and K+ ions outside the cytoplasm (Ben Arfa et al., 2006; Burt et al., 2007; Mittal et al., 2019). Furthermore, a significant difference regarding the time of action between CAR and THY was observed. After 15 minutes of contact, CAR was significantly slower in disrupting X. citri membrane when compared to THY.
In the plant protection experiments, we noticed a difference in activity among the compounds. Plants treated with OEO and THY showed a significant reduction in the number of citrus canker lesions formed when compared to the negative control. On the other hand, CAR was not efficient in protecting plants against X. citri infection. The most significant reduction in lesion formation was observed for THY, followed by OEO. Other studies describe a synergistic effect in CAR/THY mixtures (Guarda et al., 2011); in our in planta assays we suggest that other effects might be involved as well such as physicochemical characteristics that could aid the stability of the substances comprising the essential oil when in contact with leaves, since our in vitro tests did not show a more pronounced effect for OEO when compared with THY and CAR alone.
THY and CAR isomers, although with a very similar chemical structure, differ in the position of the hydroxyl group on the aromatic ring so they are classified as positional isomers. The relative position of the hydroxyl group on the aromatic ring may have influenced the difference between compounds in the rate of permeabilization of the cytoplasmic membrane of X. citri and in the protective action against bacterial infection, since the structural changes in monoterpenoids may lead to differences in biological activity (Simas et al., 2004). These differences between CAR and THY can be verified in several studies. For instance, Dorman and Deans (2000) verified that the antibacterial activity of volatile vegetable oils varied between CAR and THY against Gram-negative and Gram-positive bacteria, concluding that the relative position of the hydroxyl group exerted an influence upon the effectiveness of the components. Another work evaluated the antibacterial activity of THY, CAR and others against Staphylococcus. aureus, Escherichia. coli and Pseudmonas. aeruginosa and the results showed that among them, THY had the highest activity against all the bacteria tested (Wattanasatcha et al., 2012).
Regarding phytotoxicity, the effects observed on seed germination and root growth were rather expected, due to the allelopathic properties of essential oils and their constituents. Described as the interspecific interaction between species, where one is able to affect the growth and development of the other through chemical substances, allelopathy plays an important role both in natural system as well as in agro-ecosystems (Aslam et al., 2016; Bellache et al., 2022). Essential oils from the genus Origanum and its main constituents, THY and CAR, have already been described as phytotoxic to commercial and weed species (Synowiec et al., 2016; Ibáñez and Blázquez, 2020). It is important to notice that while capable of affecting the growth of the seeds tested, no negative effect was noticeable on C. sinensis leaves when exposed to OEO, THY and CAR in the protective assay.
Here, it was possible to verify that the IC90 and the MBC values of CAR and THY for X. citri were similar, but when applied in planta, unlike CAR, THY induced a reduction in the formation of citrus canker lesions. A similar result was observed in the work of Lu and Wu (2010), where CAR and THY displayed a similar IC and MBC against Salmonella. enterica, but in fruit sanitization tests only THY showed antibacterial potential. Although OEO was efficient in reducing canker lesion, the activity of THY in protecting plants was more pronounced. Thus, THY could be considered a novel alternative in the context of citrus plant protection, that can be used rather than copper-based bactericides or in combination with them to reduce the extent of metals applied in orchards.
Data availability statement
The original contributions presented in the study are included in the article/Supplementary Material. Further inquiries can be directed to the corresponding authors.
Author contributions
CZ: investigation, methodology, validation, formal analysis, writing original draft. VM: investigation, methodology, validation, formal analysis, writing original draft. GD: conceptualization, formal analysis. GH: investigation, formal analysis. DS: conceptualization, methodology, resources, writing/review, supervision. HF: conceptualization, writing/review, supervision, project administration, funding acquisition. All authors contributed to the article and approved the submitted version.
Funding
CZ received a MSc scholarship from Fundação de Amparo à Pesquisa do Estado de São Paulo (FAPESP no 2017/09233-9). MV received a PhD scholarship from CNPq, Conselho Nacional de Desenvolvimento Científico e Tecnológico - Brasil (Process no 142367/2019-2). This work was supported by the research grants FAPESP 2017/50454-9; 2018/21164-5 and 2021/10839-4; FAPESP no 2017/09233-9; FAPESP 2017/50454-9; 2018/21164-5 and 2021/10839-4. CNPq, Conselho Nacional de Desenvolvimento Científico e Tecnológico - Brasil (Process no 142367/2019-2.
Conflict of interest
The authors declare that the research was conducted in the absence of any commercial or financial relationships that could be construed as a potential conflict of interest.
Publisher’s note
All claims expressed in this article are solely those of the authors and do not necessarily represent those of their affiliated organizations, or those of the publisher, the editors and the reviewers. Any product that may be evaluated in this article, or claim that may be made by its manufacturer, is not guaranteed or endorsed by the publisher.
Supplementary material
The Supplementary Material for this article can be found online at: https://www.frontiersin.org/articles/10.3389/fagro.2023.1148969/full#supplementary-material
References
Araújo A. S. F., Monteiro R. T. R. (2005). Plant bioassays to assess toxicity of textile sludge compost. Scientia Agricola 62, 286–290. doi: 10.1590/s0103-90162005000300013
Aslam F., Khaliq A., Matloob A., Tanveer A., Hussain S., Zahir Z. A. (2016). Allelopathy in agro-ecosystems: A critical review of wheat allelopathy-concepts and implications. Chemoecology 27, 1–24. doi: 10.1007/s00049-016-0225-x
Behlau F. (2021). An overview of citrus canker in Brazil. Trop. Plant Pathol. 46, 1–12. doi: 10.1007/s40858-020-00377-2
Behlau F., Canteros B. I., Minsavage G. V., Jones J. B., Graham J. H. (2011). Molecular characterization of copper resistance genes from xanthomonas citri subsp. citri and xanthomonas alfalfae subsp. citrumelonis. Appl. Environ. Microbiol. 77, 4089–4096. doi: 10.1128/AEM.03043-10
Bellache M., Torres-Pagan N., Verdeguer M., Benfekih L. A., Vicente O., Sestras R. E., et al. (2022). Essential oils of three aromatic plant species as natural herbicides for environmentally friendly agriculture. Sustainability 14, 1–22. doi: 10.3390/su14063596
Ben Arfa A., Combes S., Preziosi-Belloy L., Gontard N., Chalier P. (2006). Antimicrobial activity of carvacrol related to its chemical structure. Lett. Appl. Microbiol. 43, 149–154. doi: 10.1111/j.1472-765X.2006.01938.x
Benavides S., Villalobos-Carvajal R., Reyes J. E. (2012). Physical, mechanical and antibacterial properties of alginate film: Effect of the crosslinking degree and oregano essential oil concentration. J. Food Eng. 110, 232–239. doi: 10.1016/j.jfoodeng.2011.05.023
Burt S. (2004). Essential oils: Their antibacterial properties and potential applications in foods - a review. Int. J. Food Microbiol. 94, 223–253. doi: 10.1016/j.ijfoodmicro.2004.03.022
Burt S. A., van der Zee R., Koets A. P., De Graaff A. M., Van Knapen F., Gaastra W., et al. (2007). Carvacrol induces heat shock protein 60 and inhibits synthesis of flagellin in escherichia coli O157:H7. Appl. Environ. Microbiol. 73, 4484–4490. doi: 10.1128/AEM.00340-07
Caccalano M. N., Dilarri G., Zamuner C. F. C., Domingues D. S., Ferreira H. (2021). Hexanoic acid: A new potential substitute for copper-based agrochemicals against citrus canker. J. Appl. Microbiol. 131, 2488–2499. doi: 10.1111/jam.15125
Canteros B. I. (1999). “Copper resistance in xanthomonas campestris pv. citri,” in Plant pathogenic bacteria. proceedings of the international society of bacteriology, centre for advanced study in botany. Ed. Mahadevan A. (Chennai, India: University of Madras).
Cavalca L. B., Zamuner C. F. C., Saldanha L. L., Polaquini C. R., Regasini L. O., Behlau F., et al. (2020). Hexyl gallate for the control of citrus canker caused by xanthomonas citri subsp citri. MicrobiologyOpen 9, 1–8. doi: 10.1002/mbo3.1104
Cifuentes-Arenas J. C., De Goes A., De Miranda M. P., Beattie G., Lopes S. A. (2018). Citrus flush shoot ontogeny modulates biotic potential of diaphorina citri. PLoS One 13, e0190563. doi: 10.1371/journal.pone.0190563
Coccimiglio J., Alipour M., Jiang Z. H., Gottardo C., Suntres Z. (2016). Antioxidant, antibacterial, and cytotoxic activities of the ethanolic origanum vulgare extract and its major constituents. Oxid. Med. Cell Longev 2016, 1404505. doi: 10.1155/2016/1404505
Cui H., Zhang C., Li C., Lin L. (2019). Antibacterial mechanism of oregano essential oil. Ind. Crops Products 139, 111498. doi: 10.1016/j.indcrop.2019.111498
Dorman H. J., Deans S. G. (2000). Antimicrobial agents from plants: Antibacterial activity of plant volatile oils. J. Appl. Microbiol. 88, 308–316. doi: 10.1046/j.1365-2672.2000.00969.x
Falleh H., Ben Jemaa M., Saada M., Ksouri R. (2020). Essential oils: A promising eco-friendly food preservative. Food Chem. 330, 127268. doi: 10.1016/j.foodchem.2020.127268
FAO (2021). Citrus fruit statiscal compendium 2020 (Rome: Food and Agriculture Organization of the United Nations).
Ference C. M., Gochez A. M., Behlau F., Wang N., Graham J. H., Jones J. B. (2018). Recent advances in the understanding of xanthomonas citri ssp. citri pathogenesis and citrus canker disease management. Mol. Plant Pathol. 19, 1302–1318. doi: 10.1111/mpp.12638
Gottwald T. R., Bassanezi R. B., Paulo S. (2007). Citrus huanglongbing: The pathogen and its impact plant health progress plant health progress. Plant Health Prog. 1993, 36. doi: 10.1094/PHP-2002-0812-01-RV.Increasing
Guarda A., Rubilar J. F., Miltz J., Galotto M. J. (2011). The antimicrobial activity of microencapsulated thymol and carvacrol. Int. J. Food Microbiol. 146, 144–150. doi: 10.1016/j.ijfoodmicro.2011.02.011
Helander I. M., Alakomi H.-L., Latva-Kala K., Mattila-Sandholm T., Pol I., Smid E. J., et al. (1998). Characterization of the action of selected essential oil components on gram-negative bacteria. J. Agric. Food Chem. 46, 3590–3595. doi: 10.1021/jf980154m
Ibáñez M. D., Blázquez M. A. (2020). Phytotoxic effects of commercial essential oils on selected vegetable crops: Cucumber and tomato. Sustain. Chem. Pharm. 15. doi: 10.1016/j.scp.2019.100209
Jacquet F., Jeuffroy M. H., Jouan J., Le Cadre E., Litrico I., Malausa T., et al. (2022). Pesticide-free agriculture as a new paradigm for research. Agron. Sustain. Dev. 42, 1–24. doi: 10.1007/s13593-021-00742-8
Kachur K., Suntres Z. (2020). The antibacterial properties of phenolic isomers, carvacrol and thymol. Crit. Rev. Food Sci. Nutr. 60, 3042–3053. doi: 10.1080/10408398.2019.1675585
Lambert R. J., Skandamis P. N., Coote P. J., Nychas G. J. (2001). A study of the minimum inhibitory concentration and mode of action of oregano essential oil, thymol and carvacrol. J. Appl. Microbiol. 91, 453–462. doi: 10.1046/j.1365-2672.2001.01428.x
Leonelli Pires de Campos A. C., Saldanha Nandi R. D., Scandorieiro S., Gonçalves M. C., Reis G. F., Dibo M., et al. (2022). Antimicrobial effect of origanum vulgare (L.) essential oil as an alternative for conventional additives in the minas cheese manufacture. Lwt 157, 113063. doi: 10.1016/j.lwt.2021.113063
Lu Y., Wu C. (2010). Reduction of salmonella enterica contamination on grape tomatoes by washing with thyme oil, thymol, and carvacrol as compared with chlorine treatment. J. Food Prot 73, 2270–2275. doi: 10.4315/0362-028x-73.12.2270
Marinelli L., Di Stefano A., Cacciatore I. (2018). Carvacrol and its derivatives as antibacterial agents. Phytochem. Rev. 17, 903–921. doi: 10.1007/s11101-018-9569-x
Martins P. M. M., De Oliveira Andrade M., Benedetti C. E., De Souza A. A. (2020). Xanthomonas citri subsp. citri: host interaction and control strategies. Trop. Plant Pathol. 45, 213–236. doi: 10.1007/s40858-020-00376-3
Memar M. Y., Raei P., Alizadeh N., Aghdam M. A., Kafil H. S. (2017). Carvacrol and thymol: Strong antimicrobial agents against resistant isolates. Rev. Res. Med. Microbiol. 28, 63–68. doi: 10.1097/MRM.0000000000000100
Mittal R. P., Rana A., Jaitak V. (2019). Essential oils: An impending substitute of synthetic antimicrobial agents to overcome antimicrobial resistance. Curr. Drug Targets 20, 605–624. doi: 10.2174/1389450119666181031122917
Naqvi S., Wang J., Malik M. T., Umar U. U. D., Ateeq-Ur-Rehman, Hasnain A., et al. (2022). Citrus canker–distribution, taxonomy, epidemiology, disease cycle, pathogen biology, detection, and management: A critical review and future research agenda. Agronomy 12. doi: 10.3390/agronomy12051075
Nostro A., Roccaro A. S., Bisignano G., Marino A., Cannatelli M. A., Pizzimenti F. C., et al. (2007). Effects of oregano, carvacrol and thymol on staphylococcus aureus and staphylococcus epidermidis biofilms. J. Med. Microbiol. 56, 519–523. doi: 10.1099/jmm.0.46804-0
Pandey A. K., Kumar P., Singh P., Tripathi N. N., Bajpai V. K. (2017). Essential oils: Sources of antimicrobials and food preservatives. Front. Microbiol. 7. doi: 10.3389/fmicb.2016.02161
Pecora H. B., Dilarri G., Mendes C. R., Corso C. R. (2018). Bioassays and coagulation studies using moringa oleifera seeds for the removal of textile dyes. Water Sci. Technol. 78, 1679–1692. doi: 10.2166/wst.2018.446
Peixoto-Neves D., Silva-Alves K. S., Gomes M. D., Lima F. C., Lahlou S., Magalhaes P. J., et al. (2010). Vasorelaxant effects of the monoterpenic phenol isomers, carvacrol and thymol, on rat isolated aorta. Fundam Clin. Pharmacol. 24, 341–350. doi: 10.1111/j.1472-8206.2009.00768.x
Poveda J., Roeschlin R. A., Marano M. R., Favaro M. A. (2021). Microorganisms as biocontrol agents against bacterial citrus diseases. Biol. Control 158, 104602. doi: 10.1016/j.biocontrol.2021.104602
Quintanilla-Licea R., Mata-Cardenas B. D., Vargas-Villarreal J., Bazaldua-Rodriguez A. F., Kavimngeles-Hernandez I., Garza-Gonzalez J. N., et al. (2014). Antiprotozoal activity against entamoeba histolytica of plants used in northeast Mexican traditional medicine. bioactive compounds from lippia graveolens and ruta chalepensis. Molecules 19, 21044–21065. doi: 10.3390/molecules191221044
Rodriguez-Garcia I., Silva-Espinoza B. A., Ortega-Ramirez L. A., Leyva J. M., Siddiqui M. W., Cruz-Valenzuela M. R., et al. (2016). Oregano essential oil as an antimicrobial and antioxidant additive in food products. Crit. Rev. Food Sci. Nutr. 56, 1717–1727. doi: 10.1080/10408398.2013.800832
Sakkas H., Papadopoulou C. (2017). Antimicrobial activity of basil, oregano, and thyme essential oils. J. Microbiol. Biotechnol. 27, 429–438. doi: 10.4014/jmb.1608.08024
Savietto A., Polaquini C. R., Kopacz M., Scheffers D. J., Marques B. C., Regasini L. O., et al. (2018). Antibacterial activity of monoacetylated alkyl gallates against xanthomonas citri subsp. citri. Arch. Microbiol. 200, 929–937. doi: 10.1007/s00203-018-1502-6
Schindelin J., Arganda-Carreras I., Frise E., Kaynig V., Longair M., Pietzsch T., et al. (2012). Fiji: An open-source platform for biological-image analysis. Nat. Methods 9, 676–682. doi: 10.1038/nmeth.2019
Schubert T. S., Gottwald T. R., Pierce F., Rizvi S. A., Graham J. H., Dixon W. N., et al. (2001a). 0Hhwlqj wkh &Kdoohqjh ri (Udglfdwlqj &Lwuxv &Dqnhu lq )Orulgd³$Jdlq. Plant Dis. 85.
Schubert T. S., Rizvi S. A., Sun X., Gottwald T. R., Graham J. H., Dixon W. N. (2001b). Meeting the challenge of eradicating citrus canker in Florida-again. Plant Dis. 85, 340–356. doi: 10.1094/PDIS.2001.85.4.340
Silva I. C., Regasini L. O., Petrãnio M. S., Silva D. H. S., Bolzani V. S., Belasque J., et al. (2013). Antibacterial activity of alkyl gallates against xanthomonas citri subsp. citri. J. Bacteriology 195, 85–94. doi: 10.1128/JB.01442-12
Simas N. K., Lima E. C., Conceição S. R., Kuster R. M., Lage C. L. S. (2004). Produtos naturais para o controle da transmissão da dengue: Atividade larvicida de myroxylon balsamum (óleo vermelho) e de terpenóides e fenilpropanóides. Quím. Nova 27, 46–49. doi: 10.1590/S0100-40422004000100009
Syed-Ab-Rahman S. F., Hesamian M. H., Prasad M. (2022). Citrus disease detection and classification using end-to-end anchor-based deep learning model. Appl. Intell. 52, 927–938. doi: 10.1007/s10489-021-02452-w
Synowiec A., Kalemba D., Drozdek E., Bocianowski J. (2016). Phytotoxic potential of essential oils from temperate climate plants against the germination of selected weeds and crops. J. Pest Sci. 90, 407–419. doi: 10.1007/s10340-016-0759-2
Trombetta D., Castelli F., Sarpietro M. G., Venuti V., Cristani M., Daniele C., et al. (2005). Mechanisms of antibacterial action of three monoterpenes. Antimicrob. Agents Chemother. 49, 2474–2478. doi: 10.1128/AAC.49.6.2474-2478.2005
Ultee A., Kets E. P., Alberda M., Hoekstra F. A., Smid E. J. (2000). Adaptation of the food-borne pathogen bacillus cereus to carvacrol. Arch. Microbiol. 174, 233–238. doi: 10.1007/s002030000199
Veldhuizen E. J., Tjeerdsma-Van Bokhoven J. L., Zweijtzer C., Burt S. A., Haagsman H. P. (2006). Structural requirements for the antimicrobial activity of carvacrol. J. Agric. Food Chem. 54, 1874–1879. doi: 10.1021/jf052564y
Wang J., Wang H., Ye Z., Chizaram E. P., Jiang J., Liu T., et al. (2020). Mold resistance of bamboo after laccase-catalyzed attachment of thymol and proposed mechanism of attachment. RSC Adv. 10, 7764–7770. doi: 10.1039/d0ra00315h
Wattanasatcha A., Rengpipat S., Wanichwecharungruang S. (2012). Thymol nanospheres as an effective anti-bacterial agent. Int. J. Pharm. 434, 360–365. doi: 10.1016/j.ijpharm.2012.06.017
Keywords: antibacterial, disease management, Xanthomonas citri, citrus disease, phytotoxicity
Citation: Zamuner CFC, Marin VR, Dilarri G, Hypolito GB, Sass DC and Ferreira H (2023) Oregano essential oil and its main components Thymol and Carvacrol as alternatives to control citrus canker. Front. Agron. 5:1148969. doi: 10.3389/fagro.2023.1148969
Received: 23 January 2023; Accepted: 21 March 2023;
Published: 31 March 2023.
Edited by:
Monica Höfte, Ghent University, BelgiumReviewed by:
Jian Wu, Guizhou University, ChinaAgnieszka Synowiec, University of Agriculture in Krakow, Poland
Copyright © 2023 Zamuner, Marin, Dilarri, Hypolito, Sass and Ferreira. This is an open-access article distributed under the terms of the Creative Commons Attribution License (CC BY). The use, distribution or reproduction in other forums is permitted, provided the original author(s) and the copyright owner(s) are credited and that the original publication in this journal is cited, in accordance with accepted academic practice. No use, distribution or reproduction is permitted which does not comply with these terms.
*Correspondence: Daiane Cristina Sass, daiane.sass@unesp.br; Henrique Ferreira, henrique.ferreira@linacre.oxon.org
†These authors have contributed equally to this work and share first authorship