Erratum: Compaction, aeration, and addition of mycotoxin contaminated silage alters the fermentation profile, mycotoxin content, and aerobic stability of ryegrass (Lolium perenne) silage
- 1Department of Agriculture and Environment, Harper Adams University, Edgmond, Shropshire, United Kingdom
- 2Silage Solutions Ltd, Aberystwyth, Ceredigion, United Kingdom
- 3Alltech Bioscience Centre, Sarney, Dunboyne, Co. Meath., Ireland
This study investigated the effect of compaction, aeration and addition of a naturally mycotoxin contaminated ryegrass silage (MCS) containing 1803 µg/kg DM penicillic acid, on the nutritional value and mycotoxin content after ensiling and subsequent aerobic stability of ryegrass Lolium perenne silage (second-cut, June 2020, UK). Mini silos (30 L) were filled with differential compaction (500 kg FW/m3 and 333 kg FW/m3), aeration by injection of air (1L per 48h for 30d then 1L per 7d) and addition of MCS (1.5 g/kg FW ensiled forage) in a 2×2×2 factorial design. During ensilage, mean CO2% (kg FW) in the aerated silos increased with low compaction. Crude protein (CP) increased and ash decreased with aeration. Mean silage fermentation end products acetic (AA), lactic (LA) and propionic acid (PA) concentrations increased with MCS. PA concentration increased with aeration/low compaction. LA decreased and ethanol increased with low compaction. Mycotoxin profiles differed between the silages on opening and after 14-days incubation in aerobic conditions with disappearance of fusarenon X and penicillic acid and appearance of mycophenolic acid and roquefortine C (318 µg/kg DM and 890 µg/kg DM). Addition of MCS, increased the concentration of penicillic acid on opening with an interaction with aeration (80.6µg/kg DM MCS × aerated, 40.0 µg/kg DM in the MCS × sealed). Aerobic stability was affected by aeration and low compaction with reduced time taken to heat to +5°C and +10°C above ambient temperature, higher rate of increase in pH and higher cumulative temperatures to the first peak temperature. Higher mycotoxin concentration at opening had a similar effect increasing time to heat +5°C and +10°C above ambient temperatures in aerobic conditions. Regression analysis showed predominantly direct relationships between silage fermentation end-product concentrations and aerobic stability. This study revealed interactions between silage bacteria and fungi activity from the concentrations of fermentation end-products and mycotoxins during ensilage and subsequent aerobic spoilage. The results supported current best practice for silage making, promoting conditions for improved preservation and aerobic stability. The addition of MCS had unexpected positive effects. However, factors associated with the MCS benefiting aerobic stability were not determined.
Highlights
● Effective compaction and maintaining anaerobic conditions positively affected fermentation parameters and aerobic stability
● Fungal activity in mycotoxin contaminated silage can persist in freshly prepared silage
● Mycotoxin contamination led to unexpected benefits on aerobic stability of the silage by inhibiting yeast spoilage
Introduction
Domestic livestock agriculture benefits from the ability of the rumen microbiota to degrade plant fibre to produce energy, providing products for human consumption, such as meat and milk (McDonald et al., 2021). In temperate regions, management of livestock is subject to seasonal changes with variability in the quality and availability of pasture grassland at different times of the year (Noel et al., 2017). To provide forage when grazing is not available, forage crops that have been harvested and preserved by ensiling can be fed alone, supplemented with concentrate feeds or fed as part of a total mixed ration, formulated to meet the nutritional requirements for the expected production level of the animal (Schingoethe, 2017).
In northern European countries such as the United Kingdom and Ireland, typical forage crops include ryegrasses (Lolium perenne and Lolium multiflorum), timothy (Phleum pratense) and fescues (Festuca pratensis and F. arundinacea), which are selected for their nutritive value and sugar concentration (Wilkinson et al., 2003). Following the cutting and wilting process, ensiling involves the compaction and sealing of the cut forage either in a clamp or bale (Wilkinson and Davies, 2013). Sealing with plastic provides protection against external factors, such as rain and sunlight but most importantly maintains anaerobic conditions to promote the growth of specific species of microorganisms that generate the conditions needed to ferment and preserve the silage (Bernardes, 2016).
Poor practice during the preparation of a silage clamp, such as lack of compaction and adequate sealing can lead to aerobic spoilage during ensilage with the growth of yeast and moulds and the loss of nutrients (McEniry et al., 2008). Moreover, contamination with fungal microorganisms, such as Penicillium, Aspergillus and Fusarium species could lead to the production of mycotoxins and other secondary compounds with the potential to affect the heath and production of the animal, which may possibly be carried over into the human food chain (Yiannikouris and Jouany, 2002). Mycotoxins, such as aflatoxin, ochratoxin, zearalenone, fumonisin and other compounds found in maize silage as a result of fungal contamination have been widely studied due to their high toxicity and risk to the health of livestock and humans (Fink-Gremmels, 2008; Cogan et al., 2017; Ogunade et al., 2018; Gallo et al., 2021a). Grass silage is associated with temperate climates and has not been found to contain Fusarium or Aspergillus secondary compounds in significant quantities. However, a survey of baled grass silage found fungal contamination on 58 out of 64 bales examined after opening with Penicillium roqueforti and related species (Auerbach et al., 1998; O’Brien et al., 2005). Culture isolates revealed that these fungi were capable of producing roquefortines, andrastin, mycophenolic acid, patulin and other metabolites in quantities of up to 20 mg/kg (O’Brien et al., 2006).
Aerobic spoilage post-ensilage occurs at the silage clamp face after opening or in the event where the sealing membrane is damaged, and can lead to the growth of opportunistic spoilage fungi and bacteria (Borreani and Tabacco, 2010) with associated losses in nutritive value and dry matter (DM). After opening of the clamp, exposure to air leads to a significant increase in silage temperature, metabolism of lactic acid (LA) by Candida, Pichia, Hansenula, and Endomycopsis spp. with a subsequent increase in pH (Moon and Ely, 1979; Jonsson and Pahlow, 1984; McDonald et al., 1991). This is followed by a progressive increase in microbial diversity with a relative increase in Bacillales species and relative decrease in Lactobacillales and Enterobacteriales species (Dolci et al., 2011; Dunière et al., 2017; McAllister et al., 2018). The increase in pH also allows the opportunistic growth of fungi, such as Aspergillus, Penicillium and Fusarium species with the associated risk of mycotoxin contamination (Santos et al., 2015; Peng et al., 2018).
The hypothesis of the present study was that different conditions of forage preparation and ensiling would alter fermentation end product profiles and mycotoxin contamination as well as aerobic stability of ryegrass (Lolium perenne) silage.
Materials and methods
The experimental silage preparation was carried out using a second cut forage crop (June 2020, Shropshire, UK, 52.8° N, 2.4°W). The sward, composed predominately of Lolium perenne, was mown at approximately 7.5 cm cutting height and 3–5 cm chop length and wilted for approximately 40 h. Approximately 1.5 tonnes fresh weight (FW) of the forage crop, was loaded onto a plastic sheet placed on the ground. DM was 41% at the time of ensiling. The mini silos consisted of 40 plastic containers (30L wide neck drum with a lever lid, CJK Packaging, UK). Twenty of the mini silos were modified with an inlet at the top and pressure release valve at the bottom to allow the injection of air via a gas syringe. Ten of each type of mini silo were filled either with 15 kg FW (6.15kg DM) (high compaction ~500 kg FW/m3/205 kg DM/m3) or 10 kg FW (4.1 kg DM) (low compaction ~333 kg FW/m3/136.5 kg DM/m3) of the forage. A homogenised sample of naturally mycotoxin contaminated ryegrass silage (MCS) freshly collected within 1 h and containing 1803 µg/kg DM penicillic acid, 77.6 µg/kg DM cyclopiazonic acid 508.8 µg/kg DM fusaric acid and 30.2 µg/kg DM Fumonisin B2, was added to half of the silos at 1.5 g/kg fresh material ensiled and mixed to provide five replicates of the compaction, aeration and MCS treatments factors, respectively, in a 2×2×2 factorial design. The mini silos were left to incubate in an indoor agricultural unit at ambient conditions for 133 days (mean daily room temperature ranged from 20°C at time of ensiling to 13°C at time of opening). Air (1L) was injected using a gas syringe into each of the 20 modified mini silos (aeration treatment) every 48 h for the first 30 d and then once every seven days for the remaining incubation time. Outlet gas was collected for the first 30 days and relative quantities (% total) of CO2 measured using a portable gas analyser (Gas Data Ltd, Coventry, UK).
Samples of the silage were taken on opening of the silos and pH, LA, acetic acid (AA), propionic acid (PA), ethanol, propan‐1‐ol and propane-1,2-diol (%) were measured. Analysis was carried out at a United Kingdom Accreditation Service (UKAS) laboratory (BS EN ISO/IEC 17025:2005) (Sciantec Analytical, UK). Ammonia nitrogen concentration was measured using a method adapted from the Ministry of Agriculture Fisheries and Food (MAFF, 1986) using an auto-titrator (FOSS 1030 auto-titrator, FOSS, Warrington, UK and Buchi Labortechnik AG CH-9230, Flawil, Switzerland). Ash, and crude protein (CP) were measured using methods based on the Association of Official Agricultural Chemists (AOAC, 2000). Analysis of fungal secondary metabolites and mycotoxins was conducted using high performance liquid chromatography dual mass spectrometry (HPLC-MS/MS) (37+ Labs, Alltech, Ireland) based on the method by Jackson et al. (2012).
Subsamples from each mini silo (~2 kg) were collected at opening and mixed. Each silage subsample was further divided into three replicates of 500 g and placed into polythene lined wells of an aerobic stability vessel (ASV) consisting of a polystyrene container with 4×3 wells (diameter 9.8 cm, depth 23 cm; total volume 1,735 cm3; density 288 kg/m3). Half of the silage (250 g) was placed in each vessel with a Thermocron data logger (DS1921G) (iButton, UK) placed on top with the remaining 250 g of silage used to fill the well. The Thermocrons were set to record temperature every 15 minutes, and incubation was carried out at ambient temperatures between 18°C and 22°C. ASV replicates were destructively sampled at 14, 21 and 28 days during the aerobic spoilage period. At each time point, the well was emptied, the Thermocron retained and the silage mixed. Aerobic stability was assessed by two metrics: recording the days (d) for the silage to heat to 5°C and 10°C above the ambient based on Moran et al. (1996) and cumulative temperatures (sum of temperatures (°C) taken each hour) to the first peak (Tabacco et al., 2011).
Data were analysed using GenStat Release 19 (VSN International Ltd). Data for silage analysis parameters were tested for normality using the Kolmogorov-Smirnov test and comparison of means with SED was carried out using ANOVA in a 2×2×2 three-way factorial design with the main effects of aeration, compaction and addition of MCS. Pairwise comparisons of means were conducted using Tukey’s HSD post hoc test adjusted with Bonferroni correction.
Results
The CO2% measured from the outlet gases corrected for kg fresh weight (kg FW) was higher in the low compaction silage with no effect of MCS. (Mean 4.1% Low Compaction, 3.5% High Compaction). (Mean 3.9% No MCS and 3.7% MCS) (Figure 1).
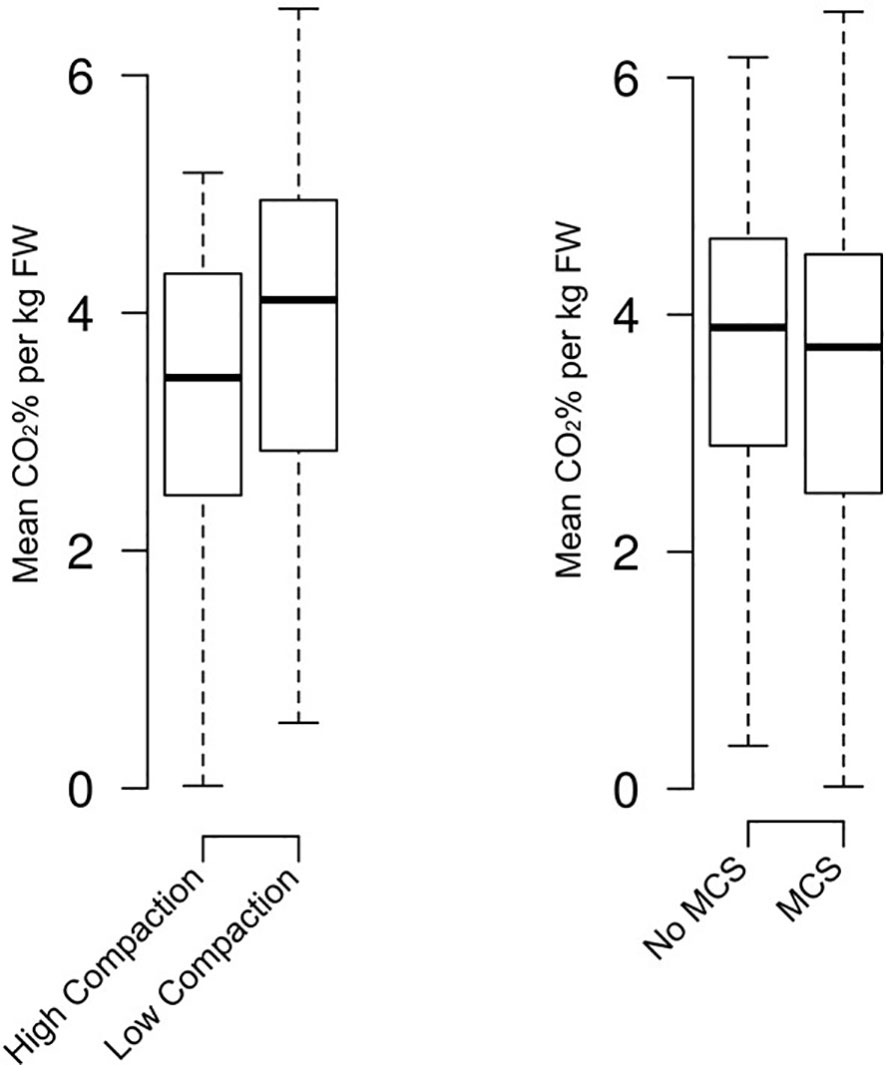
Figure 1 Aerated mini silos prepared with and without mycotoxin contaminated silage. Values correct for fresh weight (per kg FW).
Aeration, compaction and addition of MCS had no effect (P > 0.05) on the mean DM and DM loss from ensiling to opening (g/kg). CP was higher (P < 0.001) in the aerated silos, and ash was lower in the aerated relative to the sealed silos, mean: 98.8 and 100.6 g/kg DM (P = 0.024). Ash was higher with the addition of MCS, mean 100.5 and 98.8 g/kg DM, respectively (P = 0.029). All fermentation end-products were higher (P < 0.050) with the addition of MCS (Table 1). An interaction was found between aeration and compaction factors in the concentration of PA, which was higher in aerated/low compaction treatments. The concentration of PA and AA was higher in the aerated compared to non-aerated silage (P < 0.001 and 0.012 respectively) with the consequent effect of lower LA: PA ratio (P = 0.035). The concentration of ethanol was higher (P = 0.018) and LA and PA lower (P < 0.001 and 0.013 respectively) in the low compaction silage.
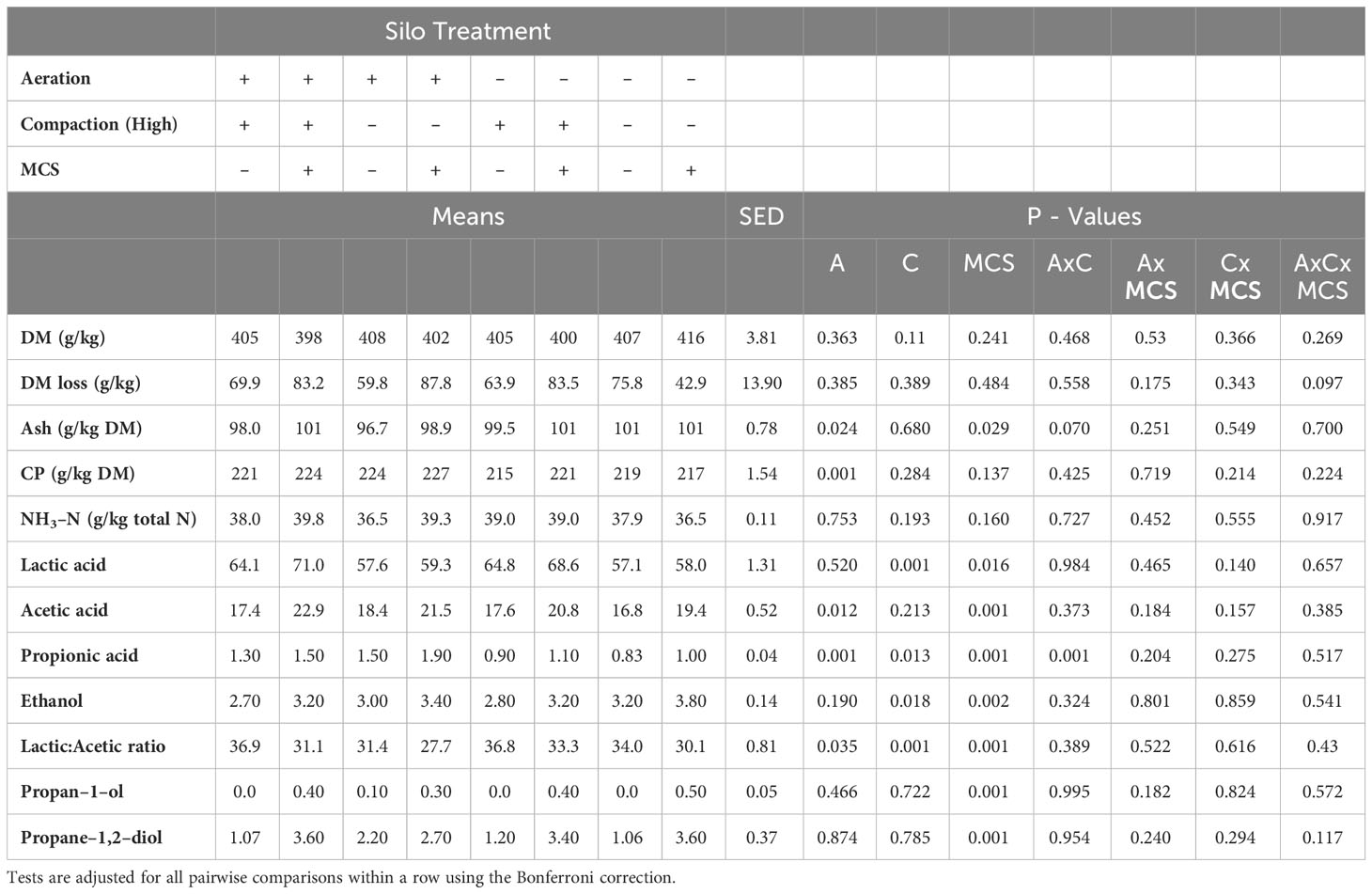
Table 1 Effect of aeration, compaction and addition of mycotoxin contaminated silage on ryegrass silage composition and fermentation end products (g/kg DM) at opening of the mini silos (Aerobic stability day 0) (A – Aeration, C – Compaction (High), MCS – Mycotoxin contaminated silage).
During the aerobic stability period, interactions between aeration, compaction and MCS were found on the concentration of fusarenon X, fusaric acid and penicillic acid on Day 0 and deoxynivalenol, fusaric acid, mycophenolic acid and roquefortine C on Day 14 (Table 2).
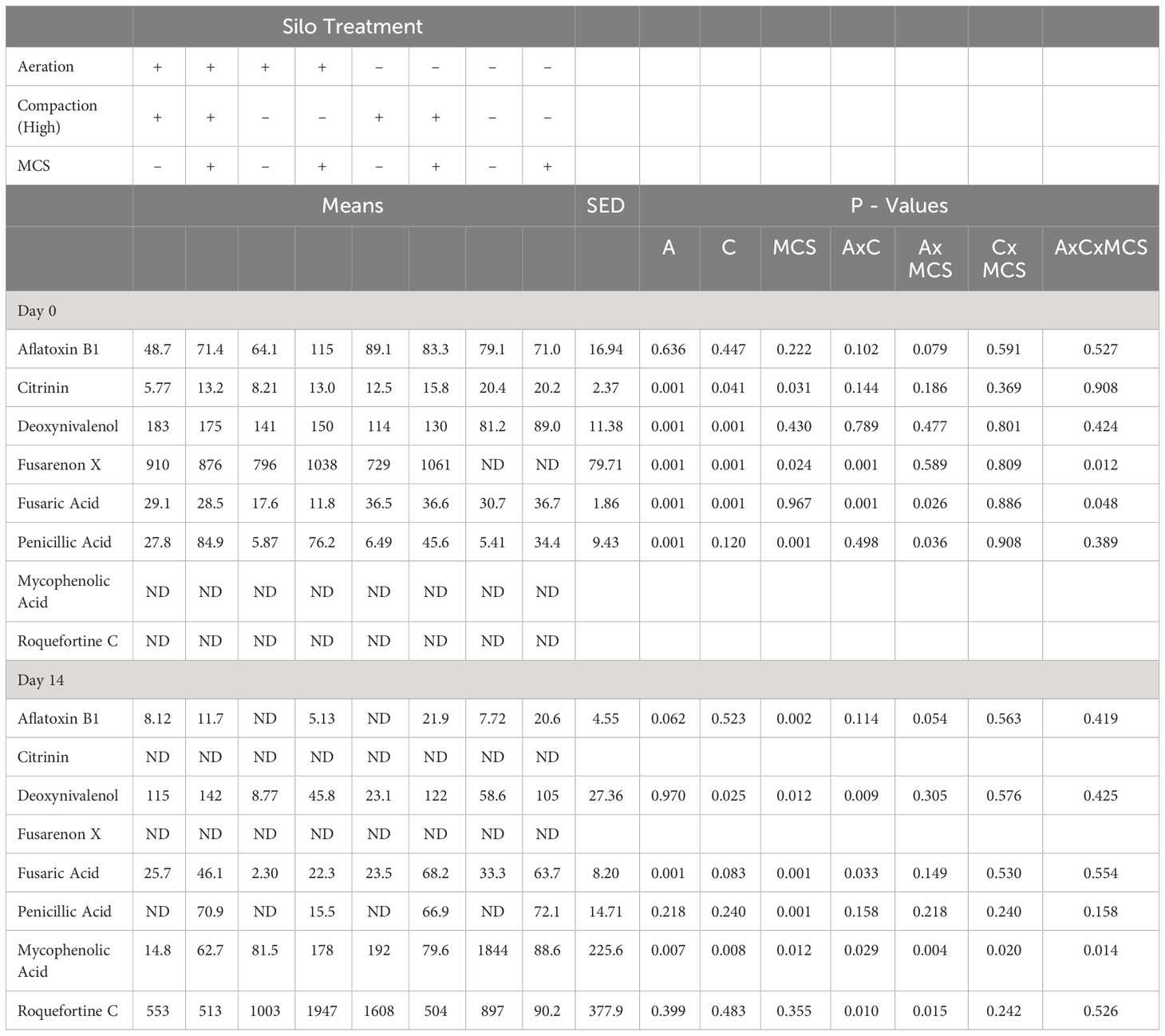
Table 2 Mean mycotoxin content of mini silo silage at the opening of the mini silos (Aerobic stability day 0 and day 14) (µg/kg DM) (A – Aeration, C – Compaction (High), MCS – Mycotoxin contaminated silage, ND – Not detected).
On Day 0 (opening), aeration of the mini silos was associated with a higher concentration of deoxynivalenol (P < 0.001) and a lower concentration of fusaric acid and citrinin (P < 0.001). Low compaction was associated with a lower concentration of deoxynivalenol (P < 0.001) and fusaric acid (P < 0.001), whilst the addition of the MCS resulted in a higher concentration of citrinin (P = 0.031), fusarenon X (P = 0.024) and penicillic acid (P < 0.001).
On Day 14, additional mycotoxins, roquefortine C and mycophenolic acid, were detected in the silage. Mycophenolic acid (MPA) was found in higher concentrations in the silage that had been prepared without aeration (P = 0.007), low compaction (P = 0.008) and without MCS (P = 0.012).
Aflatoxin B1 (P = 0.002), deoxynivalenol (P = 0.012), fusaric acid (P < 0.001) and penicillic acid (P < 0.001) concentrations all remained higher in the silage prepared with MCS.
Similarly, deoxynivalenol and fusaric acid remained in lower concentrations in the low compaction (P = 0.025) and aerated silage (P < 0.001) respectively at Day 14 of aerobic incubation, but citrinin and fusarenon X were no longer detected in any of the samples.
Interactions were found between compaction and MCS on time to 5°C above ambient and aeration, compaction, and MCS on cumulative temperature to first peak (Table 3). Aeration and low compaction at ensiling reduced the time to 5°C and 10°C above ambient temperature whereas addition of the MCS increased both aerobic stability metrics. Cumulative temperature was increased in the aerated silage, higher compaction and the addition of the MCS during preparation of the mini silos. During the four-week incubation period, mean pH was higher at Day 14 in the silage prepared with the aeration/low compaction treatments (Table 4).
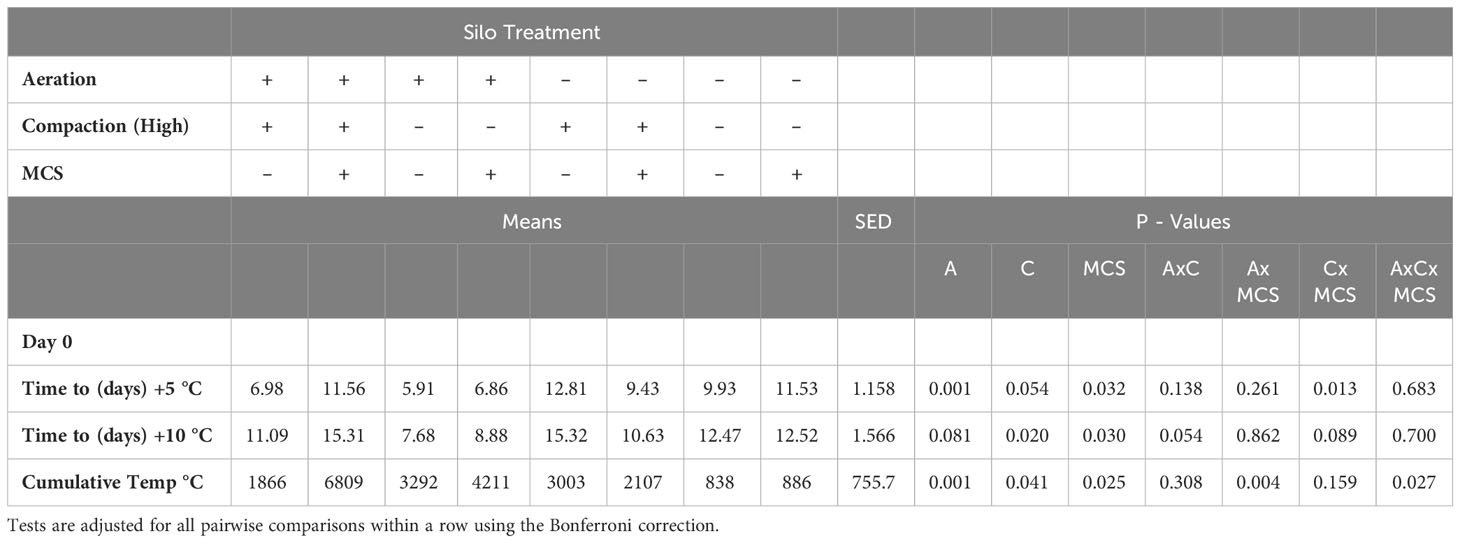
Table 3 Aerobic stability parameters: Time (Days) for the silage to heat to +5°C and +10°C above the ambient and cumulative temperatures to the first peak (A – Aeration, C – Compaction (High), MCS – Mycotoxin contaminated silage).
Regression analysis showed that higher concentrations of silage end-products measured at aerobic stability day 0 were directly related to longer times to +5°C and +10°C above ambient temperature with the exception of PA, where the relationship to time-to +5°C above ambient temperature was inverse (Table 5). The relationships to cumulative temperature were direct for LA, AA and PA. Measurements of pH at Day 14 showed a strong inverse relationship with time-to +5°C (R = –0.70, P < 0.001) and Time-to +10°C (R = –0.88, P < 0.001) above ambient. For the majority of the mycotoxins detected, higher concentrations were directly related (P < 0.05) to longer times for the silage to heat to +5°C and +10°C above ambient temperatures at both Days 0 and 14 aerobic incubation with the exception of Roquefortine C where the relationship was inverse (Day 14 only) (Table 6).
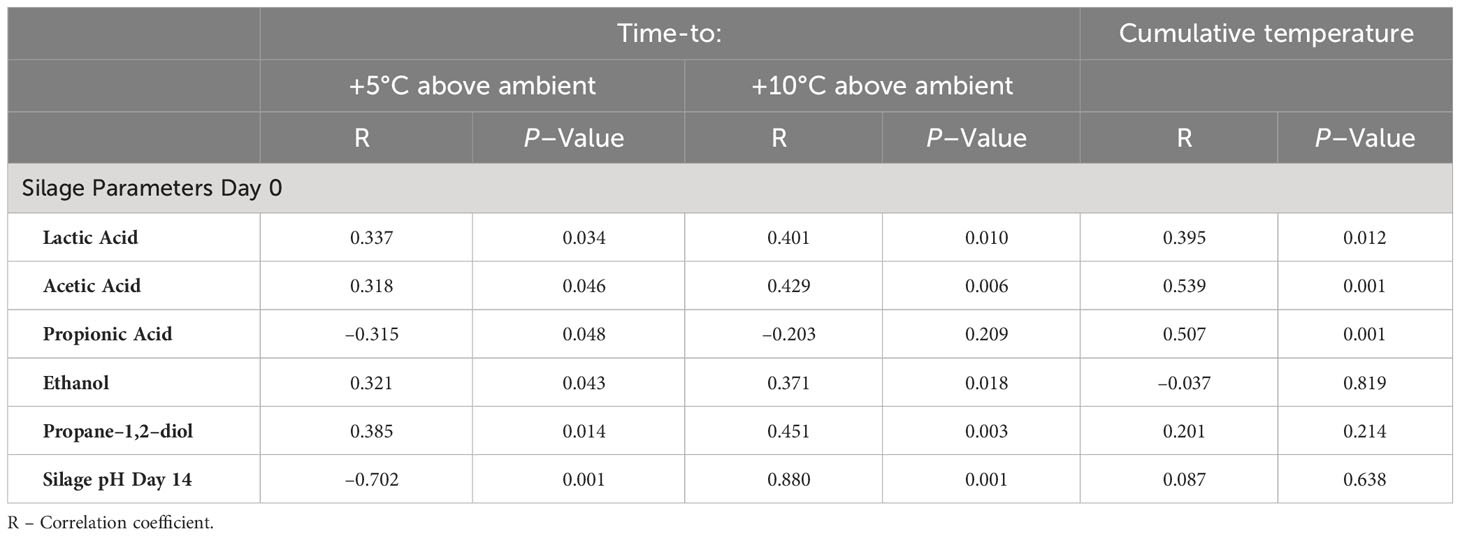
Table 5 Regression analysis of silage end-product concentration at opening of the mini silos (Aerobic stability day 0) with subsequent aerobic stability of ryegrass silage.
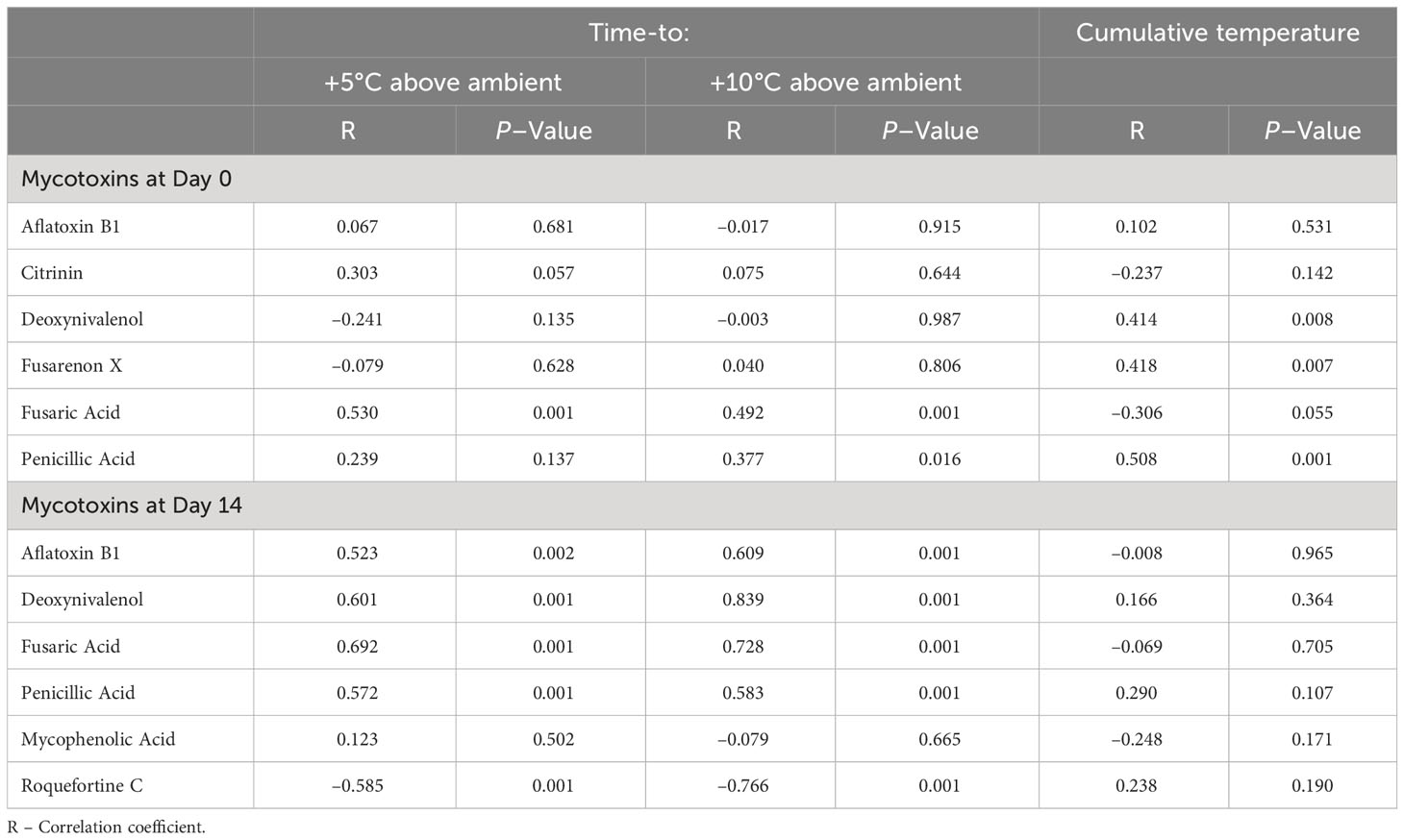
Table 6 Regression analysis of mycotoxin concentrations (Aerobic stability day 0 and day 14) with subsequent aerobic stability measurements of ryegrass silage.
Discussion
The different combinations of treatments applied during preparation led to differences in silage composition, fermentation end-products, and mycotoxin contamination, which are indicators of the nutritive value and quality of the silage and reflect the importance of the conditions during the fermentation process. The end-products could in turn have an influence on the nature of the aerobic spoilage on opening as a result of the availability of substrates that supported the growth of aerobic spoilage microorganisms.
Measurement of CO2% of the total gas during silage fermentation was only possible for the 20 aerated mini silos with the adapted inlet and outlet valves and thus the effect of compaction and MCS may have been different in the sealed mini silos. Correction of the concentration of CO2% per kg FW was undertaken to avoid the effect of higher quantities of silage in the high compaction treatment producing higher amounts of CO2%. With FW correction applied, low compaction was associated with higher concentration of CO2%, which may have been a result of increased metabolism of heterofermentative LAB or reduced production of LA due to the growth of non-LAB populations and/or a greater degradation of LA (McDonald et al., 1991). A reduced concentration of LA in the low compaction treatment with an associated higher pH supports this. This also supports recommended best practice for preparation of silage to ensure efficient compaction to promote a low pH and higher LA concentration for better silage preservation conditions (McDonald et al., 1991).
Composition of the silage on opening the aerated silos was affected by the aeration treatment, which resulted in a higher crude protein (CP) concentration. The measured CP was relatively high for the silage type (Patterson et al., 2021), which could have influenced general microbial fermentation. However, the rate of microbial breakdown of CP was not apparently affected by aeration, compaction or MCS treatments with no differences found between silage treatment and ammonia nitrogen concentrations. The mean DM losses for the silage in the aerated silos (75.2 g/kg) was higher than the sealed silos (66.5 g/kg DM) and agrees with the theory that aerobic conditions lead to greater metabolism of carbohydrates to produce CO2 and water (Borreani et al., 2018). Although not significantly different in the present study, reduced and higher DM loss (P = 0.363, P = 0.385 respectively) may explain the associated increase in CP (g/kg DM) as a result of an increased relative concentration in the aerated silage. In this respect, sample heterogeneity should also be considered as a result, of the experimental silage clamps being comparatively small.
The effect of the treatments on silage fermentation was indicated by differences in the final concentration of end-products on opening. Increased PA concentrations that were associated with aeration and low compaction, as well as the addition of MCS, indicated a slower rate of homo-lactic acid bacteria (LAB) fermentation that may have allowed the growth of bacteria including heterofermentative Lactobacillus diolivorans (Krooneman et al., 2002) and Clostridium spp. and Propionibacterium spp., which have been shown to produce PA as an end-product from the fermentation of sugars (Weinberg and Muck, 1996).
Adding MCS in the current study resulted in a significant increase in LA, AA, PA, ethanol and propane-1,2 diol concentrations. It is important to note that the addition of MCS would have introduced a variety of microorganisms and compounds, including LAB, promoting LA production, along with species of facultative anaerobe such as Enterobacteriaceae, which can produce AA as one of their main fermentation end-products (Kung et al., 2018). Increased levels of ethanol and propane-1,2-diol (propylene glycol) in the inoculated silage could also have been an effect of the activity of obligate heterofermentative LAB or Enterobacteriaceae introduced with the MCS.
Fungal secondary metabolites and mycotoxins could also have had an effect on microbial activity during the silage fermentation process (Gallo et al., 2021b). The MCS contained significant quantities of penicillic acid (1803 μg/kg) and the fungal strain producing this mycotoxin, although not isolated in the present study, was apparently still active, with MCS mini silos producing higher mean penicillic acid concentration than non MCS mini silos at aerobic stability day 0. Moreover, the significant interaction of MCS, with the aeration treatment indicate that the combined conditions may have benefitted the growth of the Penicillium strain that produced this mycotoxin. Aeration also increased concentrations of deoxynivalenol but decreased other mycotoxins, for example, citrinin and fusaric acid in the silage on aerobic stability day 0 and could also have been carried over via fungi in the MCS. The apparently contradictory results may be an effect of the interaction and competition between the different fungal species for growth and mycotoxin metabolism (Chatterjee et al., 2016). The presence of Aflatoxin B1 is of interest as this mycotoxin is typically produced by Aspergillus flavus strains and is most often associated with maize silage in southern latitudes (Wambacq et al., 2016). Previous studies at similar latitudes have not detected aflatoxins (Driehuis et al., 2008) even at the low concentrations (115 µg/kg DM) reported here. However, in recent years, warmer mean temperatures as a result of climate change could have promoted the growth of this type of fungi although the possibility of contamination from other feed materials should also be considered (Magan et al., 2011).
In the present study, incubation of the silage in aerobic conditions was conducted for 28 days. This was a longer period compared to previous studies, which have incubated for 10–14 days (Weinberg et al., 1993; Wilkinson and Davies, 2013). This was to allow additional time for the growth of spoilage microorganisms and the production of mycotoxins during the aerobic stability phase. The metrics selected (time to +5°C and +10°C above ambient, cumulative temperature to first peak and pH) are an indication of, respectively, short- and long-term aerobic stability during the aerobic incubation period (Wilkinson and Davies, 2013). Moreover, this provided insight into the effect of both the chemical composition and naturally associated microbial community of the silage associated with the aeration, compaction and MCS treatments on the aerobic spoilage process on opening.
The time-to measurements revealed reduced aerobic stability associated with the aeration and low compaction treatments, with both these factors considered poor practice during the preparation of silage (Wilkinson et al., 2003). However, the addition of the MCS had an apparent benefit on aerobic stability with increased time to +5°C and +10°C above ambient and may have been due to an inhibitory effect of the mycotoxin on aerobic spoilage microorganisms. The timescale of this phase of aerobic spoilage was during the first 15 days of aerobic incubation and thus would be relevant on farm following, for example, the exposure of a clamp face prior to mixing feed (Shan et al., 2021).
The rapid increase in temperature of the silage when exposed to air could have been an effect of the poor compaction and aeration supporting the growth of facultative aerobic spoilage microorganisms that underwent short-term rapid growth when the silage was incubated in aerobic conditions (Brüning et al., 2017). This effect was reflected in the pH measurements, which although not significant, remained consistently higher in silage from the low compaction and aerated silos during the 28-day aerobic incubation. As a measurement of good quality silage, a low pH inhibits the growth of spoilage microorganisms and is dependent on maintaining a high LA concentration. However, high LA alone can be detrimental to aerobic stability as it is a substrate for the growth of yeasts and has weak anti-fungal properties (Wilkinson and Davies, 2013). The addition of AA and PA has been proposed as a more effective suppressor of yeast spoilage (Woolford, 1975; Merry and Davies, 1999; Danner et al., 2003). Despite this, the naturally higher levels of AA in the aerated silos and AA and PA in the low compaction silos did not improve the aerobic stability in terms of the pH and time-to metrics. The addition of the MCS at ensiling was also associated with higher levels of several fermentation end-products including LA, AA, and PA, as well as alcohols and mycotoxins. Any of these individual compounds in higher quantities or combinations could have influenced the aerobic stability of the silage, which was evident from the reduced pH and increased time-to +5°C and +10°C above ambient metrics.
As a sole indicator of aerobic stability, cumulative temperature to the first peak gave results that were contrary to the pH and time-to metrics for some treatments. Higher cumulative temperature in the silage from the aerated silos indicated an increased activity of the spoilage microorganisms and lowered aerobic stability. However, silage from the MCS silos were also associated with increased cumulative temperatures, but this was opposed to the pH and time-to results.
Higher cumulative temperature may also have reflected the persistence of the aerobic spoilage over time as a result of greater availability of substrate with, for example, the higher LA found in the silage from the MCS silos. This was supported by the low compaction silage having lower LA quantities and consequently a lower cumulative temperature to first peak.
At day 14 of ASV incubation, the Penicillium mycotoxins mycophenolic acid (MPA) and roquefortine C were detected in relatively high concentrations. Conversely, aflatoxin B1 concentration was reduced and penicillic acid had dropped below detectable limit in all the silage without added MCS whilst citrinin and fusarenon X were not detected in any of the replicates. The breakdown of mycotoxins could have been a result of microbial metabolism. Bacterial taxa capable of silage fibre breakdown are similar to those found in the rumen, which are known to be effective at detoxification in this environment (Dominguez-Bello, 1996). This could have also indicated a shift in the proliferation of fungal species that were more adapted to growth in aerobic conditions outcompeting field fungi eg. Fusarium sp and storage fungi eg. Monascus sp. (Schneweis et al., 2001) in favour of other storage fungi species, such as Penicillium roquefortii (O’Brien et al., 2006). Significant interactions between the treatments indicated that the combination of aeration, compaction and addition of MCS factors would have contributed to the conditions promoting the growth of mycotoxin producing Penicillium.
In sufficiently high quantities, mycotoxins are considered problematic and a potential health risk from contaminated forage (Fink-Gremmels, 2008). However, their effect on microbial activity may benefit the aerobic stability of silage. For most of the mycotoxins detected at aerobic stability day 0 and day 14, increased concentrations were directly associated with a longer time to +5°C and +10°C above ambient temperature, indicating an inhibition of the short-term spoilage organisms that lead to the heating effect. Yeasts have the capability to detoxify mycotoxins (Hathout and Aly, 2014). However, in the present study, yeast growth and consequent aerobic spoilage of the silage was reduced in the presence of mycotoxins, either individually or in combination. The exception to this was roquefortine C concentration, which was inversely related to +5°C and +10°C above ambient temperature. Whilst being reported to have bacteriostatic properties (Kopp-Holtwiesche and Rehm, 1990) roquefortine C was apparently less effective at inhibiting spoilage yeast growth in grass silage.
Conclusions
Differential compaction, aeration and inclusion of a naturally mycotoxin contaminated ryegrass silage altered fermentation profiles, mycotoxins and aerobic stability of a ryegrass (Lolium perenne) silage. This included changes in the bacterial fermentation end product concentration of LA and other volatile fatty acids and carry over of penicillic acid. High compaction and effective sealing during preparation produced silage that was not only of a high nutritive quality but also promoted greater aerobic stability. Increased penicillic acid, as a result of the addition of MCS, highlighted the risk of contaminating silage with fungal strains capable of producing mycotoxins. Aerobic conditions were associated with the production of additional mycotoxins known to be metabolites of Penicillium fungi species, with mycophenolic acid (MPA) and roquefortine C detected in relatively high concentrations. Addition of MCS was also associated with benefits to aerobic stability. However, in the present study, it was not possible to determine the critical compounds, either individually or in combination, that may have driven this effect.
Data availability statement
The original contributions presented in the study are included in the article/supplementary material, further inquiries can be directed to the corresponding author/s.
Author contributions
Experimental design by DD and LS. Experimental work by TS and JH. Data analysis by TS, JH and LS. Manuscript written by TS, DD, JH, NA, HW, JT-P and LS. All authors contributed to the article and approved the submitted version.
Funding
This work was funded by Alltech® (Nicholasville, KY). The funding body had no role in the design of the study or collection, analysis, or interpretation of data or in writing the manuscript.
Acknowledgments
Thanks go to the HAU technicians, research project students Rosie Andrews and David Galway for their help with sample processing and data collection and Dr. Kate Le Cocq for technical expertise for data analysis. Thanks to Alltech 37+® laboratories, Dunboyne, Ireland for the silage mycotoxin analysis.
Conflict of interest
Authors NA, HW and JT-P are employed by Alltech® Nicholasville, KY. DD is the owner of Silage Solutions Ltd, Dunboyne, Co. Meath.
The remaining authors declare that the research was conducted in the absence of any commercial or financial relationships that could be construed as a potential conflict of interest.
Publisher’s note
All claims expressed in this article are solely those of the authors and do not necessarily represent those of their affiliated organizations, or those of the publisher, the editors and the reviewers. Any product that may be evaluated in this article, or claim that may be made by its manufacturer, is not guaranteed or endorsed by the publisher.
References
AOAC. (2000). Official methods of analysis of the Association of Agricultural Chemists. 17th Ed (Washington, DC: Association of Official Analytical Chemists).
Auerbach H., Oldenburg E., Weissbach F. (1998). Incidence of Penicillium roqueforti and roquefortine C in silages. J. Sci. Food Agric. 76 (4), 565–572. doi: 10.1002/(SICI)1097-0010(199804)76:4<565::AID-JSFA990>3.0.CO;2-6
Bernardes T. F. (2016). “Advances in silage sealing,” in Advances in Silage Production and Utilization, vol. Vol. 1 . Eds. Da Silva T., Santos E. M. (Rijeka, Croatia: InTech), 53–62.
Borreani G., Tabacco E. (2010). The relationship of silage temperature with the microbiological status of the face of corn silage bunkers. J. Dairy Sci. 93, 2620–2629. doi: 10.3168/jds.2009-2919
Borreani G., Tabacco E., Schmidt R. J., Holmes B. J., Muck R. E. (2018). Silage review: factors affecting dry matter and quality losses in silages. J. Dairy Sci. 101, 3952–3979. doi: 10.3168/jds.2017-13837
Brüning D., Gerlach K., Weiß K., Südekum K.-H. (2017). Effect of compaction, delayed sealing and aerobic exposure on maize silage quality and on formation of volatile organic compounds. Grass Forage Sci. 73 (1), 53–66.
Chatterjee S., Kuang Y., Splivallo R., Chatterjee P., Karlovsky P. (2016). Interactions among filamentous fungi Aspergillus Niger, Fusarium verticillioides and Clonostachys rosea: fungal biomass, diversity of secreted metabolites and fumonisin production. BMC Microbiol. 16, 83. doi: 10.1186/s12866-016-0698-3
Cogan T., Hawkey R., Higgie E., Lee M. R. F., Mee E., Parfitt D., et al. (2017). Silage and total mixed ration hygienic quality on commercial farms: implications for animal production. Grass Forage Sci. 72, 601–613. doi: 10.1111/gfs.12265
Danner H., Holzer M., Mayrhuber E., Braun R. (2003). Acetic acid increases stability of silage under aerobic conditions. Appl. Environ. Microbiol. 69 (1), 562–567. doi: 10.1128/AEM.69.1.562-567.2003
Dolci P., Tabacco E., Cocolin L., Borreani G. (2011). Microbial dynamics during aerobic exposure of corn silage stored under oxygen barrier or polyethylene films. Appl. Environ. Microbiol. 77, 7499–7507. doi: 10.1128/AEM.05050-11
Dominguez-Bello M.-G. (1996). Detoxification in the rumen. Annales Zootechnie INRA/EDP Sci. 45 (1), 323–327. doi: 10.1051/animres:19960663
Driehuis F., Spanjer M. C., Scholten J. M., Te Giffel M. C. (2008). Occurrence of mycotoxins in maize, grass and wheat silage for dairy cattle in the Netherlands. Food Addit. Contam. Part B Surveill. 1 (1), 41–50. doi: 10.1080/19393210802236927
Dunière L., Xu S., Long J., Elekwachi C., Wang Y., Turkington K., et al. (2017). Bacterial and fungal core microbiomes associated with small grain silages during ensiling and aerobic spoilage. BMC Microbiol. 17, 50. doi: 10.1186/s12866-017-0947-0
Fink-Gremmels J. (2008). Mycotoxins in cattle feeds and carry-over to dairy milk: A review. Food Addit. Contam. A Chem. Anal. Control Expo. Risk Assess. 25, 172–180. doi: 10.1080/02652030701823142
Gallo A., Fancello F., Ghilardelli F., Zara S., Froldi F., Spanghero M. (2021a). Effects of several lactic acid bacteria inoculants on fermentation and mycotoxins in corn silage. Anim. Feed Sci. Technol. 277, 114962. doi: 10.1016/j.anifeedsci.2021.114962
Gallo A., Ghilardelli F., Atzori A. S., Zara S., Novak B., Faas J., et al. (2021b). Co-occurrence of regulated and emerging mycotoxins in corn silage: Relationships with fermentation quality and bacterial communities. Toxins 13, 232. doi: 10.3390/toxins13030232
Hathout A. S., Aly S. E. (2014). Biological detoxification of mycotoxins: a review. Ann. Microbiol. 64, 905–919. doi: 10.1007/s13213-014-0899-7
Jackson L. C., Kudupoje M. B., Yiannikouris A. (2012). Simultaneous multiple mycotoxin quantification in feed samples using three isotopically labeled internal standards applied for isotopic dilution and data norMalization through ultra-performance liquid chromatography/electrospray ionization tandem mass spectrometry. Rapid Commun. Mass Spectrom. 26 (23), 2697–2713. doi: 10.1002/rcm.6405
Jonsson A., Pahlow G. (1984). Systematic classification and biochemical characterization of yeasts growing in grass silage inoculated with Lactobacillus cultures. Animal Res. Developm. 20, 7–22.
Kopp-Holtwiesche B., Rehm H. J. (1990). Antimicrobial action of roquefortine. J. Environ. Pathol. Toxicol. Oncol. 10 (1-2), 41–44.
Krooneman J., Faber F., Alderkamp A. C., Oude Elferink S. J. H. W., Driehuis F., Cleenwerck I., et al. (2002). Lactobacillus diolivorans sp. nov., a 1,2-propanediol-degrading bacterium isolated from aerobically stable maize silage. Int. J. Syst. Evol. Microbiol. 52 (2), 639–646. doi: 10.1099/00207713-52-2-639
Kung L. Jr., Shaver R. D., Grant R. J., Schmidt R. J. (2018). Silage review: Interpretation of chemical, microbial, and organoleptic components of silages. J. Dairy Sci. 101, 4020–4033. doi: 10.3168/jds.2017-13909
Magan N., Medina A., Aldred D. (2011). Possible climate-change effects on mycotoxin contamination of food crops pre- and postharvest. Plant Pathol. 60, 150–163. doi: 10.1111/j.1365-3059.2010.02412.x
McAllister T. A., Dunière L., Drouin P., Xu S., Wang Y., Munns K., et al. (2018). Silage review: Using molecular approaches to define the microbial ecology of silage. J. Dairy Sci. 101 (5), 4060–4074. doi: 10.3168/jds.2017-13704
McDonald P., Edwards R. A., Greenhalgh J. F. D., Morgan C. A., Sinclair L. A., Wilkinson R. G. (2021). Animal Nutrition (8th ed.) (UK: Pearson).
McDonald P., Henderson A. R., Heron S. J. E. (1991). The Biochemistry of Silage. 2nd edition (Marlow, Bucks, UK: Chalcombe Publications).
McEniry J., O’Kiely P., Clipson N. J. W., Forristal P. D., Doyle E. M. (2008). The microbiological and chemical composition of silage over the course of fermentation in round bales relative to that of silage made from unchopped and precision-chopped herbage in laboratory silos. Grass Forage Sci. 63, 407–420. doi: 10.1111/j.1365-2494.2008.00645.x
Merry R. J., Davies D. R. (1999). Propionibacteria and their role in the biological control of aerobic spoilage in silage. Lait 79, 149–164. doi: 10.1051/lait:1999112
Moon N. J., Ely L. O. (1979). Identification and properties of yeasts associated with the aerobic deterioration of wheat and alfalafa silages. Myco-pathologia 69, 153.
Moran J. P., Weinberg Z. G., Ashbell G., Hen Y., Owen T. R. (1996). A comparison of two methods for the evaluation of the aerobic stability of whole crop wheat silage (Aberystwyth, UK: Proc. XI Int. Silage Conf. Univ. of Wales), 162–163.
Noel S. J., Attwood G. T., Rakonjac J., Moon C. D., Waghorn G. C., Janssen P. H. (2017). Seasonal changes in the digesta-adherent rumen bacterial communities of dairy cattle grazing pasture. PloS One 12 (3), e0173819. doi: 10.1371/journal.pone.0173819
O’Brien M., Nielsen K. F., O’Kiely P., Forristal P. D., Fuller H. T., Frisvad J. C. (2006). Mycotoxins and other secondary metabolites produced in vitro by Penicillium paneum Frisvad and Penicillium roqueforti Thom isolated from baled grass silage in Ireland. J. Agric. Food Chem. 54 (24), 9268–9276. doi: 10.1021/jf0621018
O’Brien M., O’Kiely P., Forristal P. D., Fuller H. T. (2005). Fungi isolated from contaminated baled grass silage on farms in the Irish Midlands. FEMS Microbiol. Lett. 247 (2), 131–135. doi: 10.1016/j.femsle.2005.04.037
Ogunade I. M., Martinez-Tuppia C., Queiroz O. C. M., Jiang Y., Drouin P., Wu F., et al. (2018). Silage review: Mycotoxins in silage: Occurrence, effects, prevention, and mitigation. J. Dairy Sci. 101 (5), 4034–4059. doi: 10.3168/jds.2017-13788
Patterson J. D., Sahle B., Gordon A. W., Archer J. E., Yan T., Grant N., et al. (2021). Grass silage composition and nutritive value on Northern Ireland farms between 1998 and 2017. Grass Forage Sci. 76, 300–308. doi: 10.1111/gfs.12534
Peng K., Jin L., Niu Y. D., Huang Q., McAllister T. A., Yang H. E., et al. (2018). Condensed tannins affect bacterial and fungal microbiomes and mycotoxin production during ensiling and upon aerobic exposure. Appl. Environ. Microbiol. 84 (5), e02274–e02217. doi: 10.1128/AEM.02274-17
Santos M. C., Lock A. L., Mechor G. D., Kung L. (2015). Effects of a spoilage yeast from silage on in vitro ruminal fermentation. J. Dairy Sci. 98 (4), 2603–2610. doi: 10.3168/jds.2014-8683
Schingoethe D. J. (2017). A 100-year review: Total mixed ration feeding of dairy cows. J. Dairy Sci. 100 (12), 10143–10150. doi: 10.3168/jds.2017-12967
Schneweis I., Meyer K., Hörmansdorfer S., Bauer J. (2001). Metabolites of Monascus ruber in silages. J. Anim. Physiol. Anim. Nutr. (Berl). 85 (1–2), 38–44. doi: 10.1046/j.1439-0396.2001.00300.x
Shan G., Buescher W., Maack C., Lipski A., Acir I. H., Trimborn M., et al. (2021). Dual sensor measurement shows that temperature outperforms pH as an early sign of aerobic deterioration in maize silage. Sci. Rep. 22 11 (1), 8686. doi: 10.1038/s41598-021-88082-1
Tabacco E., Righi F., Quarantelli A., Borreani G. (2011). Dry matter and nutritional losses during aerobic deterioration of corn and sorghum silages as influenced by different lactic acid bacteria inocula. J. Dairy Sci. 94, 1409–1419. doi: 10.3168/jds.2010-3538
Wambacq E., Vanhoutte I., Audenaert K., De Gelder L., Haesaert G. (2016). Occurrence, prevention and remediation of toxigenic fungi and mycotoxins in silage: a review. J. Sci. Food Agric. 96 (7), 2284–2302. doi: 10.1002/jsfa.7565
Weinberg Z. G., Ashbell G., Hen Y., Azrieli A. (1993). The effect of applying lactic acid bacteria at ensiling on the aerobic stability of silages. J. Appl. Bacteriol. 75, 512–518. doi: 10.1111/j.1365-2672.1993.tb01588.x
Weinberg Z. G., Muck R. E. (1996). New trends and opportunities in the development and use of inoculants for silage. FEMS Microbiol. Rev. 19, 53–68. doi: 10.1111/j.1574-6976.1996.tb00253.x
Wilkinson J. M., Bolsen K. K., Lin C. J. (2003). History of silage Vol. 42. Eds. Buxton D. R., Muck R. E., Harrison J. H. (Madison, WI: Silage Science and Technology, Am. Soc. Agron., Crop Sci. Soc. Am., Soil Sci. Soc. Am.), 1–30.
Wilkinson J. M., Davies D. R. (2013). The aerobic stability of silage: Key findings and recent developments. Grass Forage Sci. 68, 1–19. doi: 10.1111/j.1365-2494.2012.00891.x
Woolford M. K. (1975). Microbiological screening of the straight chain fatty acids (C1–C12) as potential silage additives. J. Sci. Food Agric. 26, 219–228. doi: 10.1002/jsfa.2740260213
Keywords: aerobic, stability, compaction, mycotoxin, ryegrass, silage
Citation: Snelling TJ, Davies DR, Huntington JA, Adams N, Warren H, Taylor-Pickard J and Sinclair LA (2023) Compaction, aeration and addition of mycotoxin contaminated silage alters the fermentation profile, mycotoxin content and aerobic stability of ryegrass (Lolium perenne) silage. Front. Agron. 5:1146505. doi: 10.3389/fagro.2023.1146505
Received: 17 January 2023; Accepted: 08 August 2023;
Published: 29 August 2023.
Edited by:
Geert Jozej Willem Haesaert, Ghent University, BelgiumReviewed by:
Eva Wambacq, Hogeschool Gent, BelgiumShuai Du, Zhejiang University, China
Zhihao Dong, Nanjing Agricultural University, China
Copyright © 2023 Snelling, Davies, Huntington, Adams, Warren, Taylor-Pickard and Sinclair. This is an open-access article distributed under the terms of the Creative Commons Attribution License (CC BY). The use, distribution or reproduction in other forums is permitted, provided the original author(s) and the copyright owner(s) are credited and that the original publication in this journal is cited, in accordance with accepted academic practice. No use, distribution or reproduction is permitted which does not comply with these terms.
*Correspondence: David R. Davies, ZGF2ZS5zaWxhZ2VAZ21haWwuY29t; Liam A. Sinclair, bHNpbmNsYWlyQGhhcnBlci1hZGFtcy5hYy51aw==
†These authors share first authorship
‡ORCID: Timothy J. Snelling, orcid.org/0000-0002-8952-8826
Liam A. Sinclair, https://orcid.org//0000-0002-8543-0063