- Department of Entomology, Rutgers University, New Brunswick, NJ, United States
Over the past three decades, an increasing body of entomological research has been published on integrated pest management (IPM) in cranberries (Vaccinium macrocarpon Aiton). However, no paper has been published that synthesizes the existing literature. This paper fills this gap by analyzing 139 peer- and editor-reviewed articles that were data driven and had direct relevance to the subject of insect pests or insect pest management of V. macrocarpon. Results show that the top three studied insect pests of cranberries have been Sparganothis fruitworm (Sparganothis sulfureana Clemens), blackheaded fireworm (Rhopobota naevana Hübner), and cranberry fruitworm (Acrobasis vaccinii Riley). The regions with the most published entomological papers on cranberry IPM research have been New Jersey, Massachusetts, and Wisconsin in the United States, followed by British Columbia in Canada. Among IPM tactics, published research on chemical control, as well as on host-plant resistance, has increased likely due to recent advances on newer, reduced-risk insecticides and high-yielding cultivars; while published research focusing on behavioral control has declined likely due to the cost of these tactics. There are no consistent trends in published research on natural and biological control or cultural control. These historical research trends are important when considering regulatory changes on insecticide use, such as the Food Quality Protection Act of 1996 in the United States, which resulted in the banning and restrictions of certain broad-spectrum insecticides. As more insecticides are banned or restricted and global and organic cranberry production increases, we anticipate further advances in research related to sustainable IPM tactics.
Introduction
The concept of Integrated Pest Management (IPM) was first used in the late 1950s by Stern et al. (1959) in response to the overuse of insecticides for pest control in agriculture. Since then, IPM programs have been developed and implemented in various urban, medical and veterinary, forest, and agricultural settings. These IPM programs are, however, constantly evolving due to regulatory, environmental, economic, and societal factors (Dara, 2019; Magarey et al., 2019; Hu, 2020). Thus, knowledge of historical trends in IPM research can help us identify past priorities and existing gaps that may guide future activities related to insect pest management in agroecosystems.
The American cranberry (Vaccinium macrocarpon Aiton) (Figure 1) is an ericaceous, woody, perennial crop native to North America that is grown commercially on acidic peat, loamy sand, and mineral sandy soils (Eck, 1990). This crop, which has only been domesticated for around 200 years, is grown using a unique method of agriculture, being farmed in beds (Figure 1A), also known as bogs or marshes, which are flooded in the autumn for harvest (Kennedy et al., 2016). These beds frequently remain underwater over winter to protect the dormant plants from frost (Averill et al., 1997; Kennedy et al., 2016), and thus require copious amounts of water, often utilizing nearby surface and ground water (Kennedy et al., 2016).
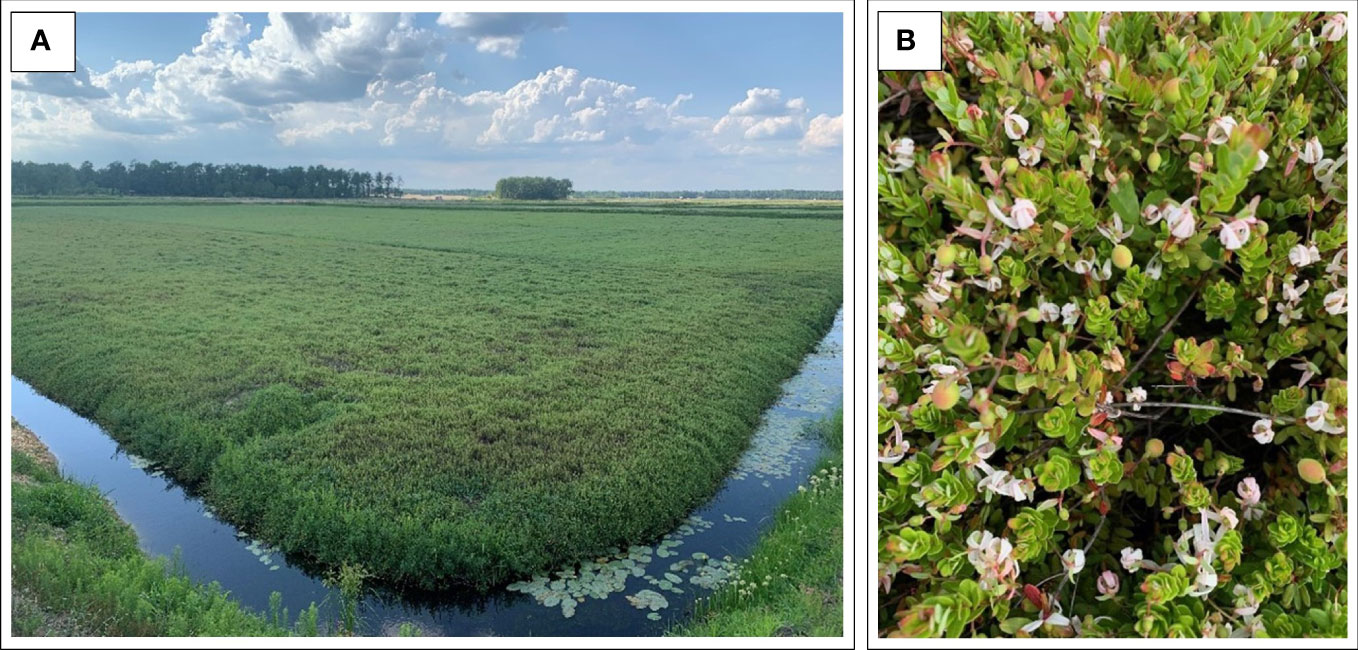
Figure 1 The American cranberry (Vaccinium macrocarpon Aiton). A cranberry bed in a commercial farm (A), and a close-up of the cranberry plant during bloom and early fruit set (B).
Cranberries have the highest world production in their native North American range, particularly in certain regions of the United States of America (USA) and Canada, such as Wisconsin, Massachusetts, New Jersey, Oregon, British Columbia, and Québec (USDA NASS, 2022) (Figure 2A). In recent years, however, worldwide production has been increasing, with this crop now being grown in countries like Chile and Turkey (FAOSTAT, 2022) (Figure 2B). In their native region, cranberries often face injury from several insect pests both aboveground and belowground that can be economically devastating to growers (Averill and Sylvia, 1998; Fitzpatrick, 2009). Along with the global expansion of cranberry production, there has been increasing research devoted to IPM to prevent injury from insect pests, especially over the last three decades. Notably, there have been major regulatory changes such as the Food Quality Protection Act of 1996 (Wheeler, 2002; Rajabi, 2014) and new emerging insect pest problems (Steffan et al., 2013; Jaffe et al., 2021a; Jaffe et al., 2021b) that have affected cranberry IPM in a direction towards more sustainable or environmentally friendly methods of pest control. Moreover, there has been a push to practice IPM and a desire from cranberry growers to learn more about possible methods and approaches (Blake et al., 2007).
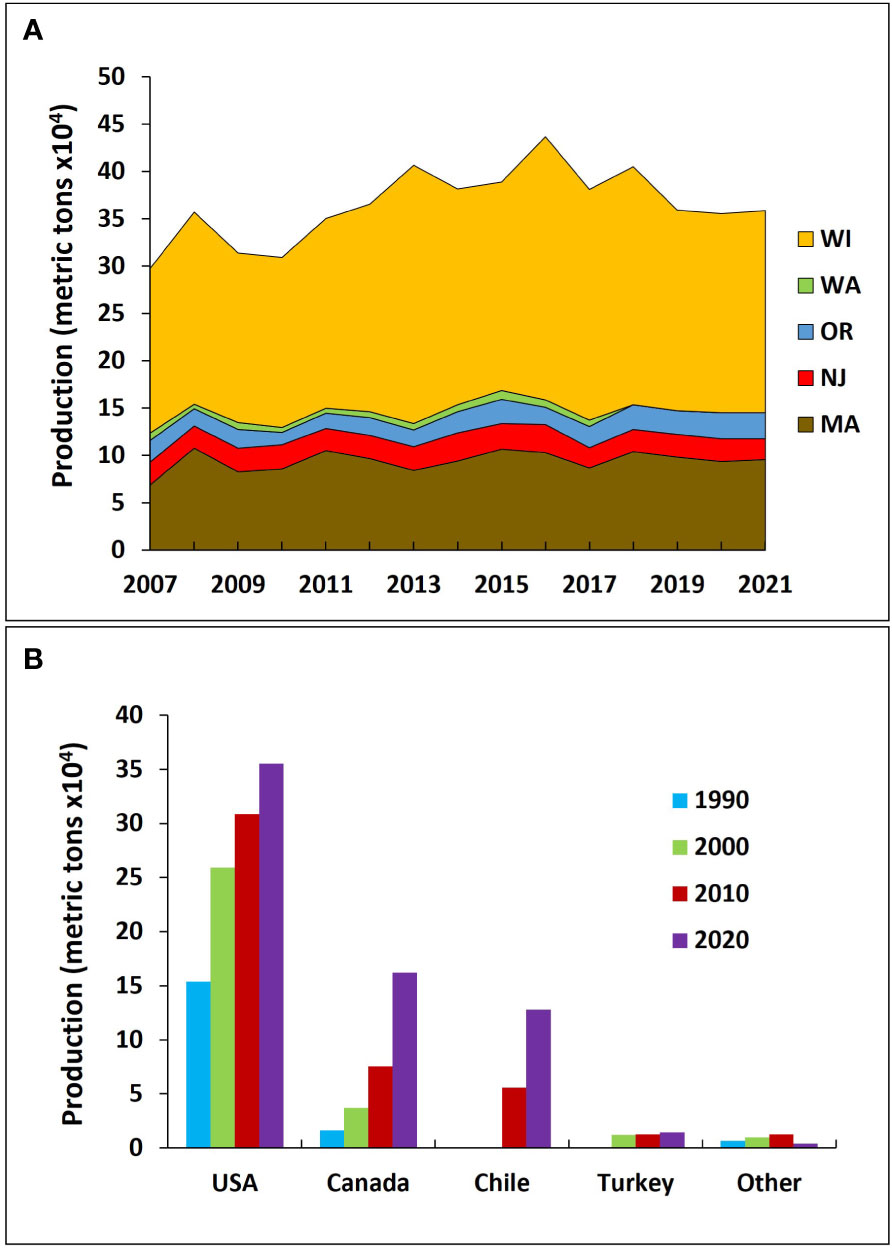
Figure 2 Trends in cranberry production (in metric tons) within the United States of America (USA) (A) and global cranberry production (B). US states: WI, Wisconsin; MA, Massachusetts; NJ, New Jersey; OR, Oregon; WA, Washington. Data obtained from USDA NASS (2022) and FAOSTAT (2022).
In this literature review, we provide a synthesis of cranberry insect IPM research over the past three decades. In particular, we aim to identify knowledge gaps and research accomplishments, provide a framework to guide future research programs, and provide information that can be immediately applied in IPM.
Global cranberry production
Cranberries are a unique crop because of their farming practices and the fact that they grow in acidic beds that utilize a lot of water for various management purposes (Averill et al., 1997; Kennedy et al., 2016). As such, there are likely limited places around the world that are capable of mass producing this crop partly due to environmental conditions (Gareau et al., 2018). The primary areas of production are found in the crop’s native ranges of the USA and Canada, which have generated on average around 376,000 and 139,000 metric tons of cranberries annually in the past 10 years, respectively (FAOSTAT, 2022) (Figure 2). Within the USA, Wiscons in is the largest producer of cranberries, producing on average ~229,000 metric tons of cranberries every year for the past decade (USDA NASS, 2022) (Figure 2A). In the USA, after Wisconsin, Massachusetts on average produces ~96,000 metric tons annually (USDA NASS, 2022) (Figure 2A). Over the past 10 years, New Jersey and Oregon produced an annual average of around 25,000 and 21,000 metric tons, respectively; although Oregon very recently overtook New Jersey in annual production (USDA NASS, 2022) (Figure 2A). In Canada, Québec and British Columbia account for ~94% of the national cranberry production, producing a respective average of around 89,500 and 40,000 metric tons in the past decade (Agriculture and Agri-Food Canada, 2021; Statistics Canada, 2022). Other US states and Canadian provinces that produce cranberries include Washington, Maine, New Brunswick, and Newfoundland and Labrador.
The largest cranberry producer outside of North America has been Chile, producing an average of 97,000 metric tons over the past 10 years (FAOSTAT, 2022) (Figure 2B). The southern regions of Chile have acidic soils from redistributed volcanic ash and similar temperatures to the northwestern USA, which are suitable conditions for cranberry production, as well as minimal insect pest pressures (Stang, 1997). The country began growing cranberries in the 1990s, experiencing a significant increase in production in the early 2000s, reaching 19% of the global market in 2020 (FAOSTAT, 2022) (Figure 2B). After Chile, Turkey is the fourth biggest producer of cranberries, responsible for an average of 11,500 metrics tons annually in the past decade (Figure 2B), followed by a range of countries mostly across Europe (FAOSTAT, 2022).
Brief history of cranberry IPM
The first cultivation of cranberries from the wild began in the early 1800s in Massachusetts (Figure 3), resulting in varieties like ‘Early Black,’ although wild fruit was still being collected and sold (Eck, 1990; Vorsa and Johnson-Cicalese, 2012). The first major breeding program took place ~100 years later in 1929 (Figure 3), intended to select for higher resistance against the blunt-nosed leafhopper, Limotettix vaccinii Van Duzee (Hemiptera: Cicadellidae) (Vorsa and Johnson-Cicalese, 2012). Limotettix vaccinii is a native pest that vectors a phytoplasma that causes cranberry false blossom disease, which is characterized by the malformation of the flowers, resulting in reduced yields (Beckwith and Hutton, 1929; Dobroscky, 1931; Pradit et al., 2020). Around a decade later, shortly after the successful breeding of resistant varieties like ‘Stevens,’ the popularization of broad-spectrum insecticides, that were inexpensive, fast-acting, and effective, reduced the necessity for these breeding programs (Vorsa and Johnson-Cicalese, 2012). Insecticide classes such as organochlorines, like DDT, as well as organophosphates and carbamates, which are acetylcholinesterase inhibitors, were being developed and sold (Figure 3), all of which were effective against many insect pests, including leafhoppers (Wheeler, 2002). These chemicals had, however, negative side effects, killing nontarget organisms and harming the environment, which led to a ban on organochlorines in the 1970s and the implementation of the Food Quality Protection Act of 1996 (FQPA) by the Environmental Protection Agency (EPA) (Figure 3) (Wheeler, 2002; Rajabi, 2014). The EPA FQPA eliminated and restricted the usage of certain broad-spectrum insecticides (organophosphates and carbamates) and led to a reevaluation of these insecticides, many of which were important in cranberry production (Wheeler, 2002; Rajabi, 2014). As a result, new methods of pest control needed to be developed, such as target-specific and more environmentally-sustainable chemical control classes with different modes of action (Rodriguez-Saona et al., 2016) (Figure 3). Additionally, behavioral control methods, such as mating disruption, have been evaluated (Figure 3), with newer technologies allowing for better identification of pheromones of cranberry insect pests (Rodriguez-Saona et al., 2020a). Furthermore, there has been increasing research on biological and natural control, particularly on the use of entomopathogenic nematodes for soil insect pests (Hayes et al., 1999; Koppenhöfer et al., 2008; Ye et al., 2018; Foye and Steffan, 2019).
Because of increased insecticide restrictions since the passing of the EPA FQPA, cranberry growers have been incorporating new tools that are more compatible with IPM to manage insect pests, such as more selective, reduced-risk insecticides and natural and biological or cultural controls (Blake et al., 2007). Despite a societal demand for fewer pesticides, there is still only a relatively small market for organic cranberry farming (Zeldin, 2005). This may be at least in part because most cranberries are used for processing (e.g., juices, sauces) instead of sold fresh, potentially influencing market price and making organic cranberries less profitable. Growing cranberries organically is also challenging because of high pest pressure, particularly from fungal pathogens that cause fruit rot, especially in humid regions (Zeldin, 2005). Due to market demand and suitable environmental conditions, the majority of organic cranberry production occurs in Quebec, followed by Wisconsin (Zeldin, 2005; Rioux, 2018; Drolet et al., 2019). Since cranberries are considered a specialty crop that are not widely grown, there is limited federal support and support from the private industry for research on new pest management strategies (Alston and Pardey, 2008).
Literature search
Our literature search focused on data-driven entomological research on IPM tactics for cranberries over the last 30 years (1990–2021). To meet our selection criteria, and thus be included in this review, the paper had to be published in a peer- or editor-reviewed journal and needs to have direct relevance to the subject of insect pests or insect pest management of the American cranberry, V. macrocarpon. For this, the Web of Science Core Collection Database was used because it is the oldest and most widely used and authoritative database of research publications and citations (Birkle et al., 2020). To do so, various keyword combinations were searched in the database. These keyword combinations were: cranberr* (the asterisk accounts for the potential plurality or different forms of the word) and one of the following: insect*, bug*, pest*, IPM, integrated pest management, and monitor*. Other than Web of Science, another database we used was Arthropod Management Tests, which gave editor-reviewed (instead of peer-reviewed) records, and that represent data-driven entomological publications largely focusing on pesticide trials. The keyword used in the Arthropod Management Tests search was: cranberr*. Because our paper focuses on research trends, we only included peer- and editor-reviewed publications and excluded extension publications, such as abstracts, proceedings, newsletter articles, and fact sheets, that are for the most part not data-driven and not easily accessible to search in an open source database.
We created a PRISMA flow chart diagram, following Page et al. (2021), to document the steps taken for the inclusion of publications in our literature review (Figure 4). Our initial search resulted in 897 publications, and after removing duplicates from the search process, we began screening the records. In addition to the records from our database search, five studies were discovered through the citations of the utilized papers that were not present on either Web of Science or Arthropod Management Tests. In the screening process, we removed the records that were out of scope and irrelevant to cranberry insect IPM, as well as those that did not meet our criteria of being a peer- or editor-reviewed papers published in the years 1990 to 2021, yielding a total of 139 papers used in our literature review (Supplemental Table 1). Because we were interested in examining trends and gaps in the existing literature on entomological IPM research in cranberries over the past three decades, the data extracted from these selected papers included the year of publication, location of the study, the insect pest(s) studied, and the IPM strategy used. It should be noted that only 25 relevant studies were indexed in the databases from prior to 1990, ranging in publication date from 1909 to 1989, and were not included in our data set. Since there were such few publications before 1990, we believe that omitting these records would not affect the findings of our analyses. Moreover, our focus was on the last three decades because this period has the most consistent IPM research and it captures research before and after the implementation of the EPA FQPA, a key regulatory event that impacted pesticide use and insect IPM in cranberries.
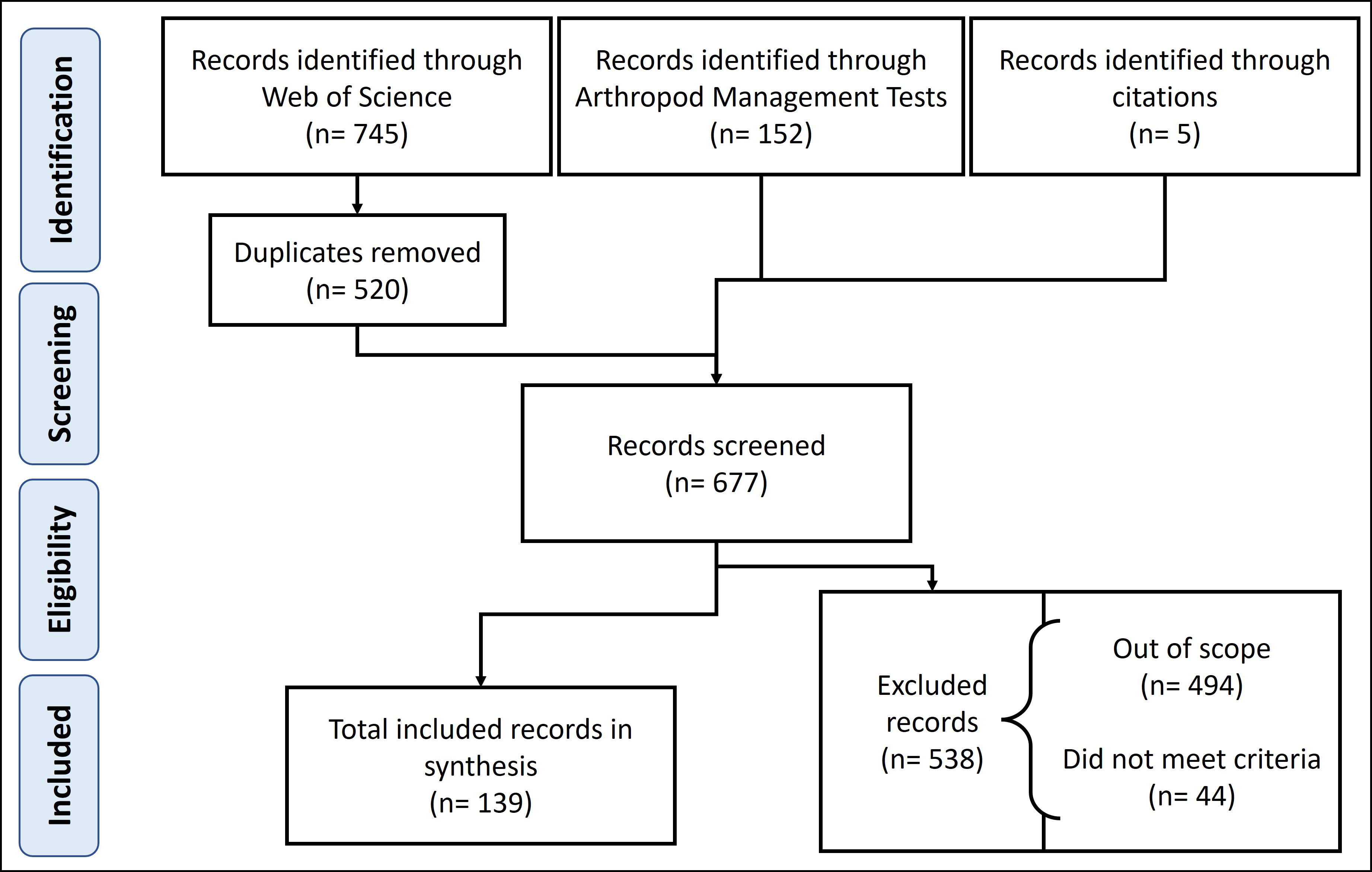
Figure 4 PRISMA flow diagram depicting the steps to the literature review. The scope of our paper was on cranberry insect IPM, and to meet our criteria for inclusion the article had to be published in a peer- or editor-reviewed journal and in the years 1990 to 2021.
Geographical region
Almost the totality of our current knowledge on cranberry insect IPM comes from studies conducted in the USA and Canada (138 of 139 publications within the past three decades) (Figure 5A). The USA accounts for about 84% of the world’s publications, with most of the research coming from three states (New Jersey, Massachusetts, and Wisconsin) (Figure 5B). Since 1990, Canada accounts for ~16% of the total research on cranberry insect IPM, with the majority (75%) coming from British Columbia (Figure 5B). Other North American regions with published cranberry IPM research include Québec, Washington, Oregon, and to a lesser extent, Newfoundland and Labrador and Maine (Figure 5B). Across North American regions, the number of publications tends to match the cranberry production (Figure 5C), indicating that funding for research likely depends on the financial support from regional stakeholders such as cranberry grower organizations; except for New Jersey, where the number of publications is much greater than the state’s crop production, and Quebec, where production acreage is much greater than the number of publications. Outside North America, the only publication on IPM of cranberry insects came from New Zealand, describing the endemic and polyphagous manuka moth, Declana floccosa Walker (Lepidoptera: Geometridae) (Miller et al., 2006). Although insect pest pressures might not be as strong in the newer regions of cranberry production, like Chile (Stang, 1997), as compared to the crop’s native regions, we expect research on the subject to increase as insect pests adapt to the introduced crop.
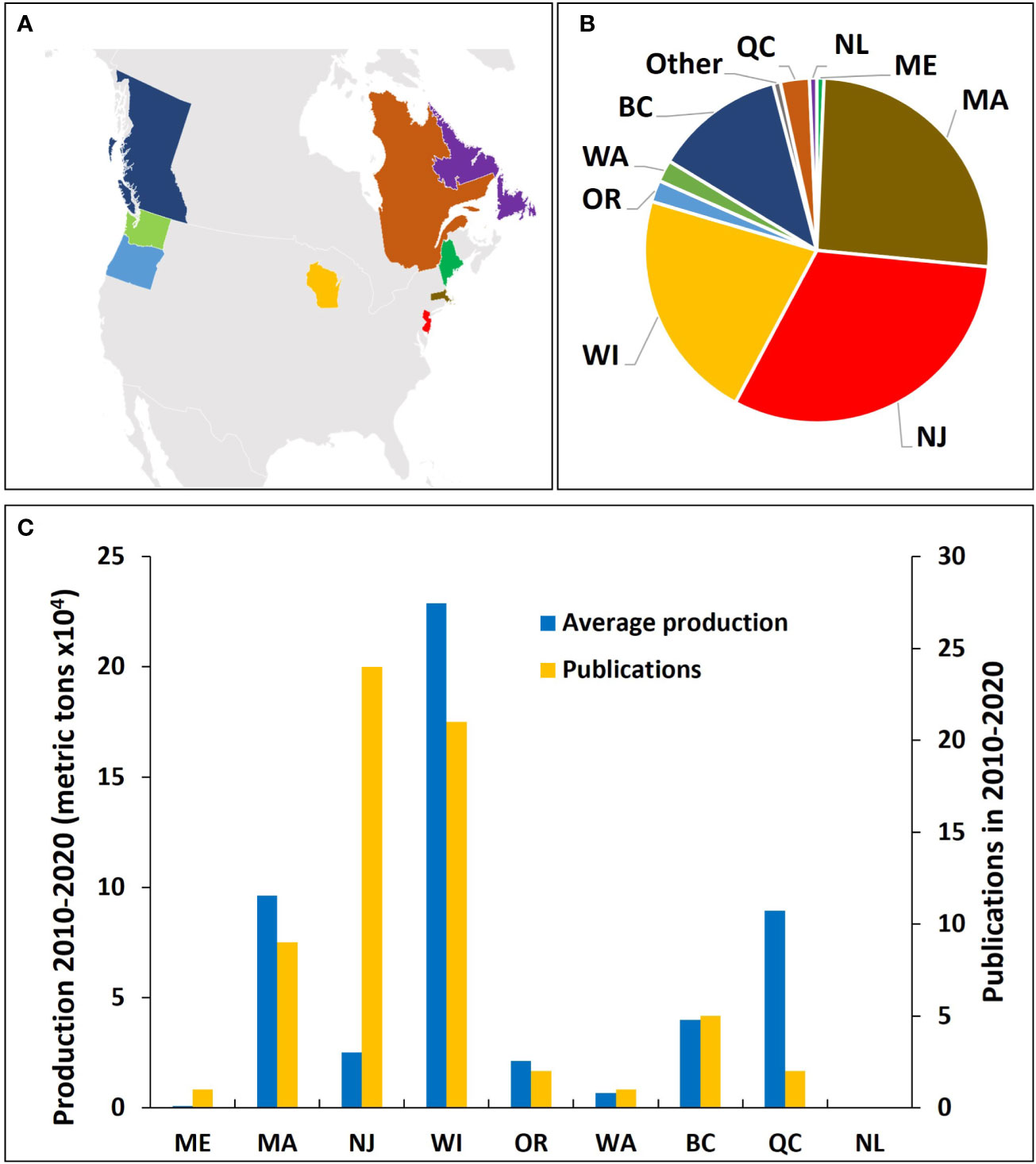
Figure 5 Regions in North America where most scientific publications about cranberry entomology topics have been conducted (A), and proportion of scientific publications by region (B) from 1990 to 2021. Publication counts compared to average production in the years 2010 to 2020 (C). US states: WI, Wisconsin; MA, Massachusetts; NJ, New Jersey; OR, Oregon; WA, Washington; ME, Maine. Canadian provinces: BC, British Columbia; QC, Québec; NL, Newfoundland and Labrador. Data obtained from USDA NASS (2022) and Statistics Canada (2022).
Cranberry insect pests
In the past three decades, there were 31 insect pests or potential pests with varying degrees of importance in cranberries that were researched; out of which 15 were Lepidoptera, 8 Coleoptera, 4 Hemiptera, 2 Diptera, 1 Hymenoptera, and 1 Orthoptera (Supplemental Table 1). The three most researched insect pests of cranberry have been Sparganothis fruitworm (Sparganothis sulfureana Clemens; Lepidoptera: Tortricidae), cranberry fruitworm (Acrobasis vaccinii Riley; Lepidoptera: Pyralidae), and blackheaded fireworm (Rhopobota naevana Hübner; Lepidoptera: Tortricidae)—all of which are native pests of Vaccinium spp. All together, these three species account for almost 40% of the studies on cranberry insect IPM (Figure 6A); although the research focus on them has fluctuated over time (Figure 6B).
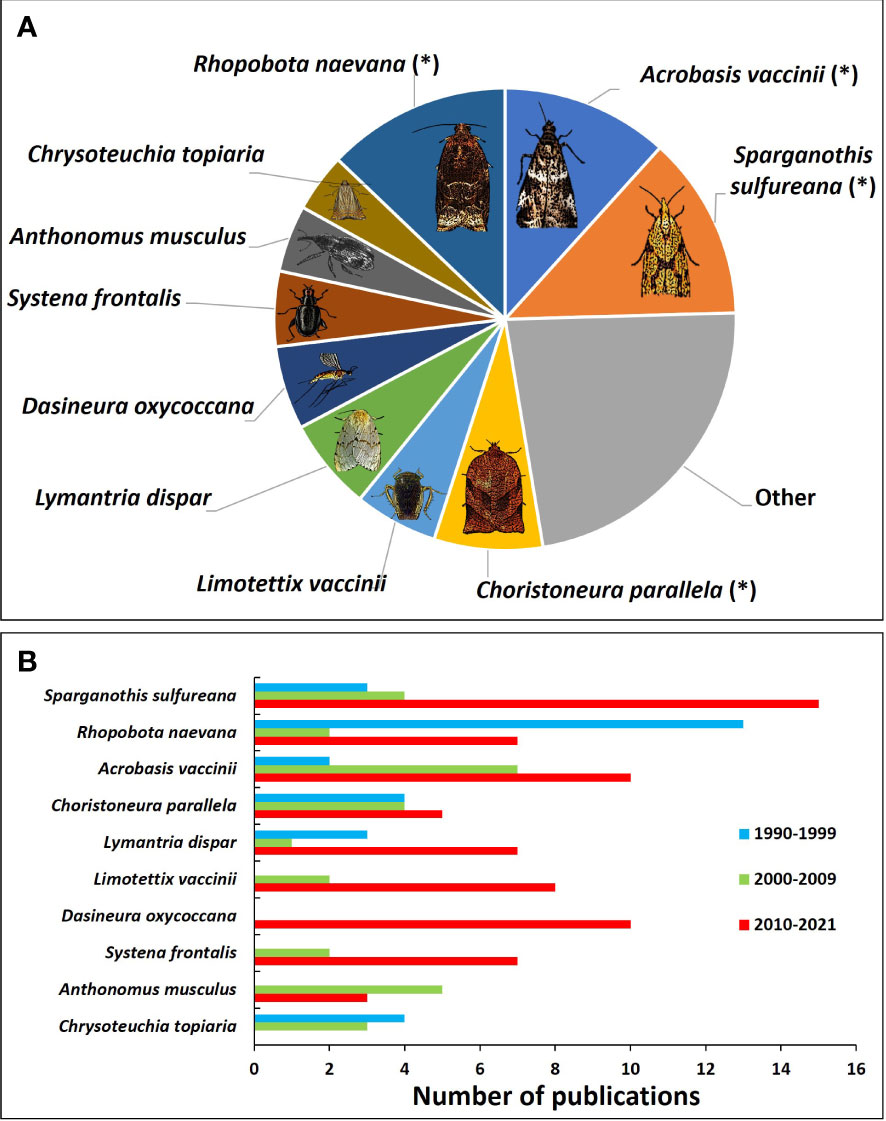
Figure 6 Proportion of scientific publications about cranberry insect IPM published by insect species (A) and the amount of papers about insects over each of the past three decades (B). Of the most studied insect pests, blackheaded fireworm (Rhopobota naevana), Sparganothis fruitworm (Sparganothis sulfureana), and cranberry fruitworm (Acrobasis vaccinii) are the top three, at 12.9%, 12.9%, and 11.7% respectively. (*) denotes a direct pest, which injures the marketable fruit.
The other top studied insect pests are spongy moth (formerly known as gypsy moth) (Lymantria dispar Linnaeus; Lepidoptera: Erebidae), cranberry tipworm (Dasineura oxycoccana Johnson; Diptera: Cecidomyiidae), spotted fireworm (Choristoneura parallela Robinson; Lepidoptera: Tortricidae), cranberry girdler (Chrysoteuchia topiaria Zeller; Lepidoptera: Crambidae), cranberry weevil (Anthonomus musculus Say; Coleoptera: Curculionidae), blunt-nosed leafhopper (Limotettix vaccinii Van Duzee; Hemiptera: Cicadellidae), and red-headed flea beetle (Systena frontalis Fabricius; Coleoptera: Chrysomelidae) (Figures 6A, B).
Most of these cranberry pests are native to North America, with only a few notable invasive species, such as L. dispar and the black vine weevil (Otiorhyncus sulcatus Fabricius; Coleoptera: Curculionidae) from Europe, and the oriental beetle (Anomala orientalis Waterhouse; Coleoptera: Scarabaeidae) from Asia. These invasive pests are generalist herbivores that can likely cope with a variety of hosts with different chemistries, allowing them to survive on plants with high phenolics, such as cranberries (Rodriguez-Saona et al., 2011b). Furthermore, these invasives feed on the less defended parts of cranberries, particularly O. sulcatus and A. orientalis that feed on the roots, which are likely less apparent than the rest of the plant due to being underground. Although L. dispar feeds on cranberry leaves, cranberries are not a preferred host (Rodriguez-Saona et al., 2011b).
Of these top studied cranberry pests, larvae of S. sulfureana, A. vaccinii, and C. parallela are direct pests, causing damage to the berries. Sparganothis sulfureana larvae, R. naevana larvae, and adult S. frontalis preferentially feed on new plant growth and foliage, whereas D. oxycoccana is a gall midge that destroys the apical meristems of the cranberries. Limotettix vaccinii is a phloem feeder that vectors a phytoplasma that causes a disease known as cranberry false blossom. Larvae of C. topiaria and S. frontalis are crown and root pests, which can kill the plant early in the season.
Considering that many of the cranberry-producing regions in North America are widely separated geographically, they probably experience different insect pest complexes and pressures, which is evident when looking at regional research. For instance, published research coming from Wisconsin, British Columbia, Washington, and Oregon often pertains to R. naevana and O. sulcatus, whereas all the literature on A. musculus and L. vaccinii came from Massachusetts and New Jersey, respectively (Supplemental Table 1). By contrast, S. sulfureana tends to be a major pest that is widely distributed across North America (Deutsch et al., 2014; Deutsch et al., 2015), and has thus been studied in many regions.
IPM tactics
We divided the published papers into monitoring and decision-making, and five control tactics: chemical control, natural and biological control, behavioral control, host-plant resistance, and cultural control. If a paper mentioned multiple IPM approaches, then it would count for every tactic it discussed. Articles on monitoring and decision-making were analyzed separately because they are IPM tactics used to assist in the pest management decision process but not for directly controlling pests as with the other tactics. Of the control tactics, chemical control has been researched the most, followed by natural and biological control and then behavioral control (Figure 7A). However, there have been slightly different trends in the focus of IPM research over the past three decades (Figure 7B), with natural and biological control being highly studied in the 1990s, then experiencing a drop in the 2000s, and increasing again in the 2010s. Chemical control has consistently been the most studied IPM approach every decade, continuously increasing with every decade. Behavioral control has also been consistently studied; however, in the 2010s, papers on behavioral control have almost halved. On the other hand, research on host-plant resistance received little attention until the 2010s, when it surpassed research on behavior and cultural control. Cultural control has received little research attention throughout the past three decades. These control tactics together with monitoring and decision-making are the foundation of an effective IPM program for cranberries (Figure 8).
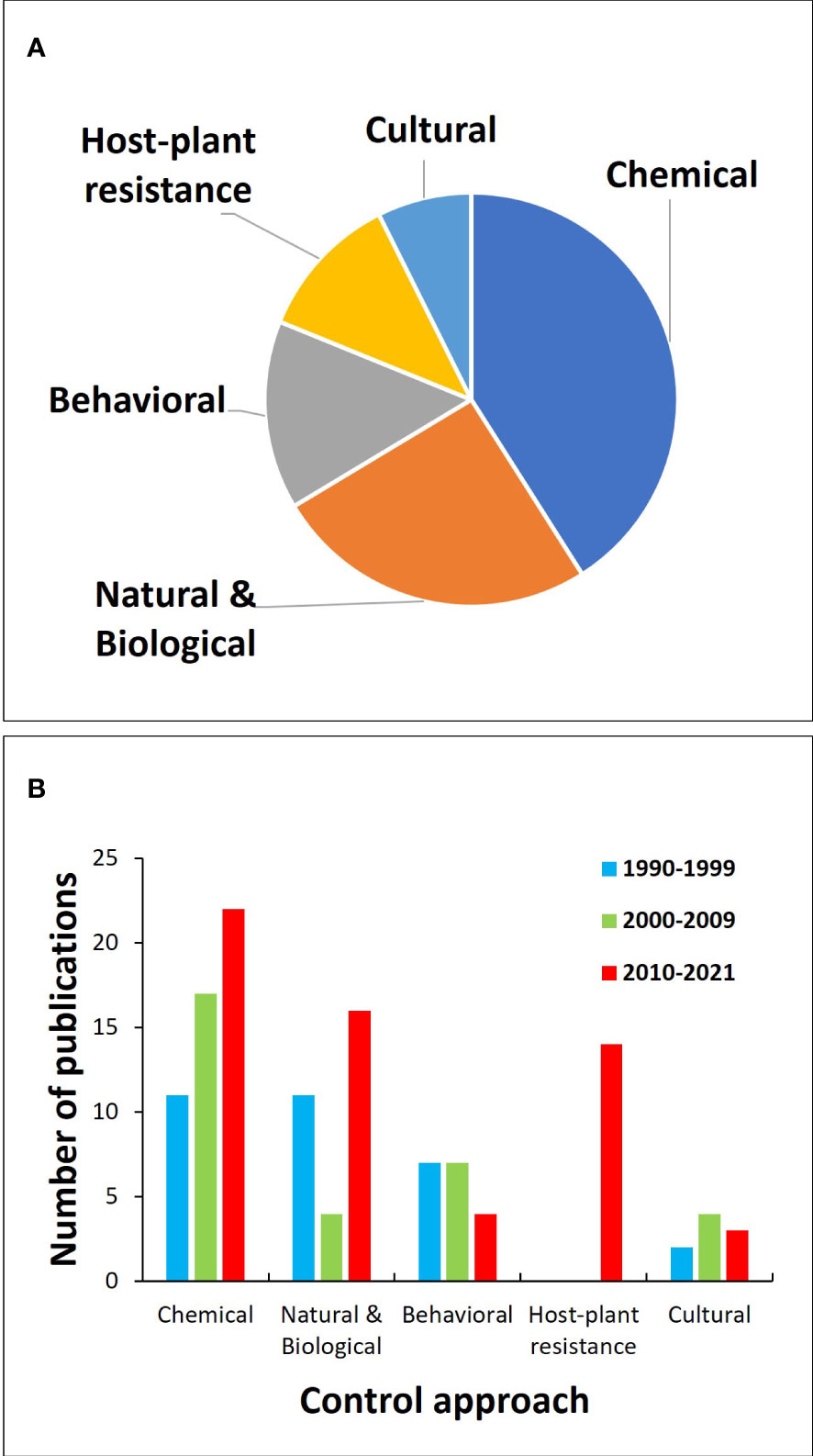
Figure 7 Proportion of scientific publications about different cranberry insect IPM tactics (A) and the number of scientific articles about IPM published by decade from 1990 to 2021 by IPM approach (B).
Monitoring and decision-making
Knowledge of the presence of pestiferous and beneficial insects in the agroecosystem allows farmers and researchers to implement further pest control measures. Thus, there have been many studies in cranberries that focussed on specific techniques of monitoring or decision-making. The most common methods of monitoring for cranberry insect pests involve sweep netting and the use of traps baited with sex pheromones. Sweep netting is a scouting technique that uses a 12-inch diameter net swung along the canopy of the cranberry plant, with around 25 sweeps per sweep set being done for every one to two acres (Averill and Sylvia, 1998; Armstrong, 2016). As proficiency in chemical ecology techniques improved, new insect pheromones have been identified. The major sex pheromone components have been identified and used in Delta traps for R. naevana (Fitzpatrick and Troubridge, 1992; Cockfield et al., 1994c; Dixon and Hillier, 2005; Steffan et al., 2017b), A. vaccinii (Marchand and McNeil, 2004b; Dixon and Hillier, 2005; Medina et al., 2013; Steffan et al., 2017b) (Figure 3), and S. sulfureana (Polavarapu et al., 2001; Deutsch et al., 2014; Steffan et al., 2017b; Pradit et al., 2019), which are important direct pests, widely distributed across cranberry-producing regions.
Other known insect pheromones, but not widely used, are those for A. musculus (Szendrei et al., 2011; Rodriguez-Saona et al., 2020a), C. parallela (Polavarapu and Lonergan, 1998), C. topiaria (Dixon and Hillier, 2005), D. oxycoccana (Fitzpatrick et al., 2000), the cranberry blossomworm, Epiglaea apiata Grote (Lepidoptera: Noctuidae) (Zhang and Polavarapu, 2003), Hoplia equina LeConte (Coleoptera: Scarabaeidae) (Zhang et al., 2003; Weber et al., 2005), the white grub Phyllophaga georgiana Horn (Coleoptera: Scarabaeidae) (Robbins et al., 2009), and the cranberry root grub, Lichnanthe vulpina Hentz (Coleoptera: Scarabaeidae) (Robbins et al., 2006). There are many potential reasons for their limited use, including their cost, lack of efficacy, and limited availability due to the narrow distribution of the pest. For example, the major components of the A. musculus aggregation pheromone have been identified and evaluated using yellow sticky cards in cranberries (Szendrei et al., 2011; Rodriguez-Saona et al., 2020a); yet, the pheromone blend remains inefficient and needs improvement, likely because additional components might be missing. Populations of D. oxycoccana that live on blueberries (Vaccinium corymbosum L.) are theorized to have a different sex pheromone than those that subsist on cranberries (Cook et al., 2011; Fitzpatrick et al., 2013), which might mean that the two populations are cryptic species (Cook et al., 2011; Cook et al., 2012); however, the high cost of pheromone production might challenge their use for monitoring D. oxycoccana populations (Fitzpatrick et al., 2013).
Traps and baits that are under evaluation for use in cranberry IPM include sticky traps of varying colors that can be baited with the plant volatile methyl salicylate to attract natural enemies of herbivores (Rodriguez-Saona et al., 2011a; Rodriguez-Saona et al., 2012; Salamanca et al., 2017; Salamanca et al., 2019; Rodriguez-Saona et al., 2020c). Sticky traps catch a wide variety of insects, making them good for monitoring local diversity, but a lack of specificity can make them ineffective when looking for one specific pest. Other types tested include bucket traps for D. oxycoccana and H. equina (Weber et al., 2005; Fitzpatrick et al., 2018) and cross vane traps for P. georgiana (Robbins et al., 2009). Visual inspection of cranberry plants and surrounding weeds can also be used as a monitoring tool. For instance, A. vaccinii tends to prefer larger cranberries (Marchand and McNeil, 2006) and can induce early fruit reddening (Marchand and McNeil, 2004a). Also, some cranberry pests, like C. parallela (Stuart and Polavarapu, 1998) and C. topiaria (Roland, 1990), show oviposition and feeding preferences for different weeds, with the latter sometimes preferring to feed on the weeds over the cranberries (Roland, 1990). Thus, assessment of oviposition and feeding damage on weeds in cranberry beds can be indicative of the presence of some insect pests. The two invasive pests L. dispar (Rodriguez-Saona et al., 2011b) and O. sulcatus (Miller et al., 2012) are highly polyphagous and are thus likely to be present on, and sometimes even prefer, certain weed species.
In addition to monitoring for insect pests, decision-making and risk assessment are critical aspects of IPM. This is commonly achieved using degree-day models which set temperature-mediated thresholds based on insect phenology. Degree-day models have been developed to predict phenological events, such as the onset of adult activity, peak adult flight, and time of egg hatch, to better time insecticide applications for S. sulfureana (Deutsch et al., 2014; Deutsch et al., 2015), A. vaccinii (Medina et al., 2013; Chasen and Steffan, 2016), C. topiaria (Cockfield and Mahr, 1994), and R. naevana (Cockfield et al., 1994b). Studies have also been conducted to characterize the injury caused by insect pests like D. oxycoccana (Tewari et al., 2012; Tewari et al., 2013), A. musculus (Long and Averill, 2003), S. frontalis (Jaffe et al., 2021a), the toad bug Phylloscelis rubra Ball (Hemiptera: Dictyopharidae) (Rodriguez-Saona et al., 2020b), and the broad-winged bush katydid Scudderia pistillata Brunner von Wattenwyl (Orthoptera: Tettigoniidae) (Labarre et al., 2020). For example, plants that were infested with D. oxycoccana or mechanically girdled had more than a 55% decrease in fruit size relative to uninfested, ungirdled plants (Tewari et al., 2014), and simulated A. musculus damage led to a 30% decrease in fruit number and weight (Long and Averill, 2003). These studies can help with the establishment of economically-relevant damage levels and economic thresholds. For example, knowledge on the pest’s phenology and life history with the use of economic thresholds can reduce crop damage caused by A. musculus (Long and Averill, 2003). Unfortunately, many important cranberry pests currently do not have economic thresholds, such as L. vaccinii (de Lange and Rodriguez-Saona, 2015a), D. oxycoccana (Mahr, 2005), and C. topiaria (Madore, 2010). Other pests have combined thresholds, such as all noctuid lepidopterans with L. dispar at 4.5 larvae per sweep set (Averill and Sylvia, 1998; Le Duc et al., 2004), or 4 larvae per sweep set for C. parallela (de Lange and Rodriguez-Saona, 2015b). There are also pests that have different thresholds depending on the location; for instance, S. sulfureana has an economic threshold of 5 larvae per sweep set in eastern Canada (Le Duc et al., 2004; Madore, 2010), but 2 larvae per sweep set in Massachusetts and Wisconsin (Sylvia and Averill, 2013; Guédot and McMahan, 2015). Establishing better and more up-to-date models on insects and their injury characterization can contribute to the economic threshold calculations, which is needed for several insect pests of cranberries.
Chemical control
There have been several changes in chemical control in cranberries in the last 30 years. In 1996, the EPA FQPA was passed in the USA, restricting certain broad-spectrum insecticides, such as the organophosphates azinphos-methyl (Guthion®) and chlorpyrifos (Lorsban®), ultimately leading to the implementation of newer reduced-risk insecticides (Figure 3). As such, chemical control has been the highest studied control approach, comprising 41% of all research on cranberry insect IPM (Figure 7A). Decades before the EPA FQPA, cranberry growers had used DDT to control R. naevana and L. vaccinii. But these insects developed resistance to this insecticide, necessitating a switch to pyrethrum, malathion, dieldrin, and parathion (Brody et al., 2002). In the 1990s, parathion was utilized via chemigation, aerial, or boom sprays to control various insect pests (Clark et al., 1994), whereas azinphos-methyl, in conjunction with parathion, was also used to control R. naevana (Wan et al., 1995). Since then, these two organophosphates have been banned for use in cranberries because of environmental and human health concerns.
In 2021, the organophosphate chlorpyrifos was also banned for use in cranberries (Figure 3). This insecticide was widely used to control several cranberry insect pests, including S. sulfureana, C. parallela (Putnam et al., 2002; Rodriguez-Saona et al., 2016), A. musculus (Putnam et al., 2002), and L. vaccinii (Rodriguez-Saona et al., 2019). However, residues could be detected as long as 2 months after application (Putnam et al., 2002) and the insecticide has significant negative effects on beneficial insects (Rodriguez-Saona et al., 2016). Sparganothis sulfureana has already developed resistance to organophosphates in Massachusetts (Averill and Sylvia, 1998). As a response to the EPA FQPA, farmers soon began switching to newer insecticides with different modes of action, such as insect growth regulators (IGRs), spinosyns, and diamides, which are more environmentally friendly and have greater compatibility with biological control methods than organophosphates (Rodriguez-Saona et al., 2016). The past two decades saw the registration of IGRs like methoxyfenozide (Intrepid®), spinosyns like spinetoram (Delegate®), diamides like chlorantraniliprole (Altacor®), and pyrethroids like fenpropathrin (Danitol®) and bifenthrin (Fanfare®) (Figure 3). IGRs, spinosyns, and diamides are more selective than the broad-spectrum insecticides (i.e., organophosphates, carbamates, and pyrethroids) targeting mainly lepidopteran pests such as S. sulfureana, A. vaccinii, R. naevana, C. parallela, and L. dispar (Rodriguez-Saona et al., 2016). Due to the highly permeable sandy soils and connection to water in the regions where cranberries are grown, there are few registered insecticides, namely neonicotinoids like imidacloprid, to control root insect pests; however, neonicotinoids can have severe nontarget effects especially on pollinators (Lu et al., 2020). For organic farming, pyrethrins (like Pyganic®), the spinosad Entrust®, and neem oil products (like Trilogy®) are currently available for insect pest control (Armstrong, 2017).
Natural and biological control
A little more than one-fourth of the published research on insect IPM in cranberries dealt with natural and biological control (Figure 7A), which utilizes predators, parasitoids, and pathogens to reduce pest abundance. The most popular predators used in cranberry agriculture are entomopathogenic nematodes that can be sprayed to control root pests, such as Steinernema carpocapsae Weiser (Rhabditida: Steinernematidae) for the control of O. sulcatus (Hayes et al., 1999), Heterorhabditis bacteriophora Poinar (Rhabditida: Heterorhabditidae) for the cranberry rootworm, Rhabdopterus picipes Oliver (Coleoptera: Chrysomelidae) (Polavarapu et al., 2000), and Steinernema scarabaei Stock and Koppenhöfer (Rhabditida: Steinernematidae) for P. georgiana (Koppenhöfer et al., 2008). The recently discovered nematode Oscheius onirici Torrini et al. (Rhabditida: Rhabditidae) can kill S. sulfureana (Ye et al., 2018; Foye and Steffan, 2019) and A. vaccinii (Ye et al., 2018), as well as suppress S. frontalis (Foye and Steffan, 2019). Other predators that are commonly present in cranberry ecosystems include spiders (Bardwell and Averill, 1996; van Zoeren et al., 2018; de Lange et al., 2019b), staphylinid beetles (Haase-Statz, 1997), coccinellid beetles (Rodriguez-Saona et al., 2011a; Salamanca et al., 2017; de Lange et al., 2019b; Salamanca et al., 2019), chrysopid lacewings (Rodriguez-Saona et al., 2011a; de Lange et al., 2019b; Salamanca et al., 2019), and syrphid flies (Rodriguez-Saona et al., 2011a; Salamanca et al., 2017; de Lange et al., 2019b; Rodriguez-Saona et al., 2020c).
Among parasitoids, native trichogrammatid wasps have been found to occur naturally, and shown effective on R. naevana (Li et al., 1993; McGregor and Henderson, 1998), A. vaccinii (Simser, 1995), and C. parallela (Stuart and Polavarapu, 2000). In addition, several naturally occurring braconid and ichneumonid wasps, as well as tachinid flies, were found to parasitize the lepidopterous pests R. naevana, S. sulfureana, the false armyworm Xylena nupera Lintner (Lepidoptera: Noctuidae), the green spanworm Macaria sulphurea Packard (Lepidoptera: Geometridae), and the Rannoch looper Macaria brunneata Thunberg (Lepidoptera: Geometridae) (Drolet et al., 2019). Native eulophid and platygastrid wasps were found to attack D. oxycoccana (Peach et al., 2012).
In terms of pathogens, it has been demonstrated that a fungal formulation of indigenous Metarhizium robertsii Metchnikoff Sorokin (Hypocreales: Clavicepitaceae) (formerly known as Metarhizium anisopliae) can be used against O. sulcatus and C. topiaria (Booth and Shanks, 1998; Booth et al., 2000). There has also been a bacterium, Chromobacterium subtsugae MWU12-2387 (Neisseriales: Neisseriaceae), discovered in the rhizosphere of cranberry plants that has biocontrol potential (Vöing et al., 2017). Another strain of C. subtsugae, PRAA4-1T, is actually used as a bioinsecticide (Grandevo®), which can be useful to control S. sulfureana, A. vaccinii, R. naevana, the common eupithecia Eupithecia miserulata Grote (Lepidoptera: Geometridae), and occasionally D. oxycoccana (Guédot and Perry, 2015; Guédot and Perry, 2016a; Guédot and Perry, 2016b). Burkholderia rinojensis A396 (Burkholderiales: Burkholderiaceae) is also a bacterium that has been marketed (Venerate®) for pest control in cranberries, showing efficacy against S. sulfureana, A. vaccinii, R. naevana, and E. miserulata (Guédot and Perry, 2015a; Guédot and Perry, 2016b). Another bacterium that has been widely utilized as a biocontrol agent, particularly in organic farming, is Bacillus thuringiensis Berliner (Bacillales: Bacillaceae) (Dipel®, Biobit®, and Xentari®) in the form of aerial sprays and occasionally chemigation to target lepidopterous pests such as L. dispar, E. apiata, X. nupera, M. sulphurea, and the brown spanworm Ematurga amitaria Guenée (Lepidoptera: Geometridae) (Sandler and Mason, 1997; Armstrong, 2017). There has been an unnamed granulosis virus discovered that is associated with R. naevana mortality (Theilmann et al., 1995), although there have been few studies on this virus and its use as a biological control agent.
Behavioral control
Research on behavioral control comprises 14.8% of the published literature (Figure 7A). Mating disruption functions by releasing components of each pest’s sex pheromone which can reduce mating and fecundity (Fitzpatrick, 2006), and is the main behavioral control tactic tested to manage cranberry insect pests like S. sulfureana (Cockfield et al., 1994a; Polavarapu et al., 2001) (Figure 3), R. naevana (Cockfield et al., 1994c; Fitzpatrick et al., 1995; Baker et al., 1997; Fadamiro et al., 1998; Fitzpatrick, 2006) (Figure 3), and C. parallela (Polavarapu and Lonergan, 1998). Mating disruption for A. orientalis has also been evaluated in cranberries (Wenninger and Averill, 2006), although it has yet to be implemented likely because other grub species are more important pests. However, the efficacy of mating disruption can be inconsistent, like in the case of A. vaccinii (Steffan et al., 2017a). Other times they have not even been attempted because of sufficient tolerance to the pest, as in the case of H. equina, which has an effective pheromone lure (Zhang et al., 2003; Weber et al., 2005). Various methods to deploy pheromones for mating disruption have been assessed including drones (Luck et al., 2021), flowable emulsions for A. vaccinii and R. naevana (Steffan et al., 2017b), a sprayable microencapsulated formulation of S. sulfureana pheromones (Polavarapu et al., 2001), as well as a meter semiochemical timed release system for R. naevana (Baker et al., 1997; Fadamiro et al., 1998). Mating disruption can be used in organic cranberry farms; however, there were no commercially available products at the time of the submission of this paper.
Host-plant resistance
Most of the insect pests of cranberries are native to North America and have probably co-evolved with the crop. Cranberries have a high phenolic content (Rodriguez-Saona et al., 2011b; Vorsa and Johnson-Cicalese, 2012), which may limit feeding by non-adapted insect herbivores. However, recent releases of high-yielding cranberry cultivars have led to an increase in research on host-plant resistance from 0 to 11.5% in the last decade (Figure 7A). Cranberry cultivars have been bred for different purposes. In the beginning of cultivation and during the 19th century, farmers grew cranberries that were propagated from wild plants, some of which are still actively cultivated varieties, such as ‘Early Black,’ ‘Ben Lear,’ ‘Howes,’ ‘McFarlin,’ and ‘Potter’ (Vorsa and Johnson-Cicalese, 2012). There have been two major breeding programs since the cultivation of cranberries, one starting in the 1920s and the other in the 1980s (Vorsa and Johnson-Cicalese, 2012). The first breeding program crossed wild cultivars and was directed at an ultimate goal of resistance to L. vaccinii, the vector of a phytoplasma that causes cranberry false blossom disease (Hancock et al., 2008; Vorsa and Johnson-Cicalese, 2012) (Figure 3). This resulted in the release of the popular cultivars ‘Stevens’ and ‘Franklin’ in the 1950s (Vorsa and Johnson-Cicalese, 2012). However, in the 1940s and 1950s, reliable broad-spectrum insecticides, such as organophosphates, organochlorines, and carbamates, were increasingly utilized to control L. vaccinii (Wheeler, 2002), reducing the need for developing insect-resistant cultivars. Therefore, the second breeding program for cranberries was aimed at developing higher-yielding cultivars, with bigger fruit that ripens earlier and with more intense color, producing cultivars such as ‘Crimson Queen,’ ‘Mullica Queen,’ ‘Demoranville,’ ‘GH1,’ and ‘HyRed’ (Hancock et al., 2008; Vorsa and Johnson-Cicalese, 2012; McMahan et al., 2016).
Because of the history of breeding in cranberries, cultivars are expected to differ in resistance against insect herbivores. For instance, Franklin was bred for high productivity and resistance to L. vaccinii, whereas Demoranville and Crimson Queen were bred for large fruit size, high yield, and earlier ripening seasons (de Lange et al., 2019a; de Lange et al., 2019b). In terms of insects, Mullica Queen and Ben Lear suffered more A. vaccinii larval damage than did Stevens or GH1 (McMahan et al., 2016). On the other hand, S. sulfureana performance did not differ among Mullica Queen, Ben Lear, Stevens, GH1, and HyRed (McMahan and Guédot, 2018). Stevens appears to be more tolerant to O. sulcatus than McFarlin (Miller et al., 2012). Two high-yielding cultivars, Crimson Queen and its parent Ben Lear (Rodriguez-Saona et al., 2011b), along with Howes and Franklin (de Lange et al., 2019a), were shown to be more susceptible to L. dispar than McFarlin (Rodriguez-Saona et al., 2011b), Stevens (Rodriguez-Saona et al., 2011b; de Lange et al., 2019a), or the very resistant cultivar Potter (Rodriguez-Saona et al., 2011b; de Lange et al., 2019a). Stevens, Howes, Franklin, Crimson Queen, Early Black, and Potter all showed equal resistance to S. sulfureana and C. parallela (de Lange et al., 2019a). Lymantria dispar also showed a preference for Howes over Early Black, although A. musculus did not show any preference, and S. frontalis had an insignificant trend toward preferring Howes (Neto et al., 2010). Lymantria dispar damage on Stevens, Mullica Queen, and Howes did not affect the cranberry’s induced response (Tjiurutue et al., 2017), despite the three cultivars having different phenolic and volatile profiles (Tjiurutue et al., 2017; de Lange et al., 2019b). In general, there seems to be an inverse relationship between growth and defense such that cranberry cultivars that were bred for high yield tend to be less defended against insect pests (de Lange et al., 2019a).
In some cases, plant diseases, such as cranberry false blossom, can interfere with the levels of nutrients and defenses in cranberry plants (Pradit et al., 2019; Pradit et al., 2020; Rodriguez-Saona et al., 2021), which can affect L. vaccinii development (Pradit et al., 2019), as well as benefit non-vector pests, like S. sulfureana, C. parallela, and L. dispar (Pradit et al., 2020). Moreover, commercial elicitors of plant defenses, such as activators of the salicylic acid (SA) and jasmonic acid (JA) defense pathways, failed to increase cranberry resistance against insect herbivores as well as the disease-causing phytoplasma (Rodriguez-Saona et al., 2021). However, the application of elicitors of the JA defense pathway can lead to higher volatile production that can potentially attract natural enemies of insect pests (de Lange et al., 2019b; Rodriguez-Saona et al., 2021) or repel pests, such as S. sulfureana (Rodriguez-Saona et al., 2013).
Cultural control
Cultural control tactics account for 7.4% of the published research on cranberry insect IPM (Figure 7A). These are methods in which the grower changes the environment of the cranberry bed to combat pests, such as flooding, sanding, and weeding. Flooding is normally implemented in the fall for harvest, with the crop remaining underwater for the duration of the winter for protection from frost (Averill et al., 1997), although the timing of fall flooding (Demoranville et al., 2005) or spring draining (Averill et al., 1997) can be altered for insect pest management. For example, A. musculus adults emerge from non-crop habitats around farms in early spring and start moving to the cranberry beds to lay eggs on the flower buds (Szendrei et al., 2011). In New Jersey, where spring draining of cranberry beds typically begins in mid to late April, a discontinuity between insect and plant phenology may serve as an agent to reduce A. musculus incidence; although A. musculus is not a top pest of cranberries in New Jersey (Mechaber, 1992; Szendrei et al., 2011). Flooding can be used to drown many insect pests but has been used particularly to control R. naevana (Cockfield and Mahr, 1992; Teixeira and Averill, 2006), A. vaccinii (Averill et al., 1997; Demoranville et al., 2005; Teixeira and Averill, 2006), and C. topiaria (Teixeira and Averill, 2006; Fitzpatrick, 2007), and it has the potential to work against S. sulfureana (Teixeira and Averill, 2006). Flooding also has been prescribed throughout the year to control E. apiata, L. dispar, X. nupera, the white grub, Phyllophaga anxia LeConte (Coleoptera: Scarabaeidae), and the southern red mite, Oligonychus ilicis McGregor (Trombidiformes: Tetranychidae) (Averill et al., 1997). Additionally, early season flooding has been shown to increase natural enemy abundance, such as spiders and parasitoids (van Zoeren et al., 2018). Flooding is also used in IPM against certain weeds (Demoranville et al., 2005) and fungi (Averill et al., 1997). It prevents the crop from being exposed to pests and low temperatures when not in production (Averill et al., 1997; Demoranville et al., 2005). However, since flooding requires a lot of water and also has the potential to lead to fungal rot, there has been a decrease in its usage for pest control with the increased popularity of insecticides (Cockfield and Mahr, 1992; Averill et al., 1997; Demoranville et al., 2005). Other than flooding, sanding has been shown to control C. topiaria and C. parallela without reducing cranberry yields (Davenport and Schiffhauer, 2000). Mowing, pruning, and burning of weeds are additional cultural control methods for some cranberry insect pests, like C. parallela (Stuart and Polavarapu, 1998) and C. topiaria (Roland, 1990). Still, flooding remains as the most effective cultural control practice that cranberry growers utilize against insect pests (Armstrong, 2016).
Conclusions and future perspectives
Figure 8 provides a summary of the advances in IPM strategies for cranberry insect pests. By looking at the research conducted over the past 30 years on cranberry insect IPM, there are some evident trends. Research on chemical control has consistently increased, and research on host-plant resistance has gained increased attention in the past decade. This is likely due to the recent focus on testing the efficacy of new, reduced-risk insecticides and on breeding for high-yielding cultivars with no prior knowledge of their resistance to pests. Other control tactics, such as natural and biological, behavioral, and cultural control, have remained relatively stable across the decades, with the research focus ranked as follows: natural and biological control > behavioral control > cultural control (Figure 7B).
We expect future IPM research in cranberries to likely result in better monitoring techniques and thresholds for insect pests. As of now, there are economic thresholds for only a few key insect pests (Averill and Sylvia, 1998), and we lack thresholds for new emerging pests, such as L. vaccinii. Coordinating economic thresholds with degree-day models of insect growth can be an important next step in IPM, especially considering how insect populations may be affected by ongoing climate change. The discovery of new pheromones and other attractants is likely, and the subsequent development and employment of more efficacious traps and lures, either for monitoring or as a method of behavioral control. Furthermore, there is a need for more research on monitoring and conserving beneficial insects, such as the natural enemies of pests and pollinators (Blake et al., 2007).
As new high-yielding cranberry cultivars are developed, there will be an increased need to study their susceptibility to insect pests. For instance, cultivars with higher yields often have reduced resistance to pests (Rodriguez-Saona et al., 2011b), and will likely experience more pest pressure and require more insecticide applications. These newer high-yielding cultivars can be more susceptible to not only direct pests but also to vectors of diseases, such as L. vaccinii. Since the reduced insect resistance of the newer cultivars could result in increased pest pressure, we expect the future focus of breeding programs to shift to resistance against pests, as well as understanding the mechanisms of resistance in wild populations. An IPM tactic that has remained relatively unexplored is genetic control. With newer molecular technologies, it is possible to investigate the genetic modification of either the cranberry crop (to make it more protected against pests) or the insect pests, such as sterile male technologies. However, caution needs to be taken because of the risk of modified genes being transferred to wild cranberry populations in their native range.
More than two decades after the EPA FQPA, many broad-spectrum insecticides are still being banned or are limited in use. These broad-spectrum insecticides are being replaced by more selective, environmentally-friendly, and reduced-risk insecticides. However, the adoption of these reduced-risk insecticides can lead to a resurgence of old insect pests, such as has been the case for the blunt-nosed leafhopper, L. vaccinii, and the toad bug, P. rubra. As farmers adopted new reduced-risk insecticides against lepidoptera pests in the past two decades, such as IGRs, spinosyns, and diamides, populations of pests that are not affected by these insecticides, like L. vaccinii, have been on the rise (de Lange and Rodriguez-Saona, 2015a). This, in conjunction with a shift in cranberry breeding from insect resistance to fruit quality (Vorsa and Zalapa, 2020), has resulted in the increased incidence of false blossom disease in New Jersey, namely because the only effective control of the disease is to target the vector (Rodriguez-Saona et al., 2021). This pest resurgence has been exacerbated by the recent banning of the organophosphate chlorpyrifos (Lorsban®) that was widely used to control L. vaccinii. Recently, however, two pyrethroids, a class of synthetic insecticides not previously available to farmers, were registered for use in cranberries, i.e., fenpropathrin (Danitol®) and bifenthrin (Fanfare®) (Figure 3), which are effective against the pest. As cranberry production continues to globalize, there is a rising threat of invasive insect pests, which will likely require adaptation of the existing pest management programs. Moreover, public concerns about pesticide use have in general increased the interest in organic farming; however, there are few effective tools currently available for organic pest control in cranberries (Armstrong, 2017; Rioux, 2018; Drolet et al., 2019). With the increase in organic cranberry production, we hope to see an increased registration of behavior-based strategies, such as mating disruption, that can provide an alternative tool for insect pest control.
As restrictions in insecticide use expand, research on nonchemical management tactics is expected to increase. Farmer adoption of these tactics could help conserve beneficial insects and thus improve biological control and pollination in cranberry agroecosystems (Rodriguez-Saona et al., 2011a). Moving forward, the role of non-crop habitats surrounding cranberry farms, i.e., landscape composition, as a potential source of insect pests and beneficial insects (natural enemies and pollinators) warrants more research.
In summary, this literature review provides a synthesis of cranberry insect IPM research over the past three decades, which aims at providing knowlegde on recent trends and at existing knowledge gaps. With an increasing global production of cranberries and the threat of invasive pests, we expect changes in IPM tactics to be reflected in future research. Also, as more insecticides are being banned or their use restricted, we anticipate further research related to sustainable IPM tactics.
Author contributions
YB-Z wrote the manuscript. CR-S edited the manuscript. All authors contributed to the article and approved the submitted version.
Funding
YB-Z and CR-S are supported by the New Jersey Cranberry Research Council, the Cranberry Institute, Ocean Spray, and the Cape Cod Cranberry Growers’ Association, and CR-S by the Hatch project NJ08140.
Acknowledgments
We thank Julia Wooby for revising early drafts and providing photos of the cranberry plant and bed, and Robert Holdcraft for providing images of the cranberry pests. We also thank the reviewers for providing helpful comments on an earlier draft of the manuscript.
Conflict of interest
The authors declare that the research was conducted in the absence of any commercial or financial relationships that could be construed as a potential conflict of interest.
Publisher’s note
All claims expressed in this article are solely those of the authors and do not necessarily represent those of their affiliated organizations, or those of the publisher, the editors and the reviewers. Any product that may be evaluated in this article, or claim that may be made by its manufacturer, is not guaranteed or endorsed by the publisher.
Supplementary material
The Supplementary Material for this article can be found online at: https://www.frontiersin.org/articles/10.3389/fagro.2023.1006106/full#supplementary-material
Supplementary Table 1 | The 139 publications reviewed.
References
Agriculture and Agri-Food Canada (2021). “Statistical overview of the Canadian fruit industry 2020”, Canada: Government of Canada, Horticulture Section, Crops and Horticulture Division. Available at: https://agriculture.canada.ca/sites/default/files/documents/2021-08/fruit_report_2020-eng.pdf.
Alston J. M., Pardey P. G. (2008). Public funding for research into specialty crops. HortScience 43 (5), 1461–1470. doi: 10.21273/HORTSCI.43.5.1461
Armstrong C. (2016). “Integrated pest management in cranberries: An overview,” Orono, Maine: The University of Maine Cooperative Extension. Available at: https://extension.umaine.edu/cranberries/wp-content/uploads/sites/40/2020/04/Cranberry-IPM-Overview-opt.pdf.
Armstrong C. (2017). “Organic control for cranberry insect management,” Orono, Maine: The University of Maine Cooperative Extension. Available at: https://extension.umaine.edu/cranberries/wp-content/uploads/sites/40/2017/04/MaineCranberryInsects2017-organic.pdf.
Averill A. L., Sylvia M. M. (1998). Cranberry insects of the northeast, a guide to identification, biology and management (East Wareham, MA: University of Massachusetts Cranberry Station).
Averill A., Sylvia M., Kusek C., DeMoranville C. (1997). Flooding in cranberry to minimize insecticide and fungicide inputs. Am. J. Altern. Agric. 12 (2), 50–54. doi: 10.1017/s0889189300007220
Baker T. C., Dittl T., Mafra-Neto A. (1997). Disruption of sex pheromone communication in the blackheaded fireworm in Wisconsin cranberry marshes by using MSTRS devices. J. Agric. Entomology 14 (4), 449–457. Available at: https://ento.psu.edu/files/108bakeretal1997.pdf.
Bardwell C., Averill A. (1996). Effectiveness of larval defenses against spider predation in cranberry ecosystems. Environ. Entomology 25 (5), 1083–1091. doi: 10.1093/ee/25.5.1083
Beckwith C. S., Hutton S. B. (1929). Cranberry false blossom and the blunt-nosed leafhopper. New Jersey Agric. Experiment Station Bull. 491, 1–16.
Birkle C., Pendlebury D. A., Schnell J., Adams J. (2020). Web of science as a data source for research on scientific and scholarly activity. Quantitative Sci. Stud. 1 (1), 363–376. doi: 10.1162/qss_a_00018
Blake G., Sandler H., Coli W., Pober D., Coggins C. (2007). An assessment of grower perceptions and factors influencing adoption of IPM in commercial cranberry production. Renewable Agric. Food Syst. 22 (2), 134–144. doi: 10.1017/s1742170507001664
Booth S., Shanks C. (1998). Potential of a dried rice/mycelium formulation of entomopathogenic fungi to suppress subterranean pests in small fruits. Biocontrol Sci. Technol. 8 (2), 197–206. doi: 10.1080/09583159830261
Booth S., Tanigoshi L., Dewes I. (2000). Potential of a dried mycelium formulation of an indigenous strain of Metarhizium anisopliae against subterranean pests of cranberry. Biocontrol Sci. Technol. 10 (5), 659–668. doi: 10.1080/095831500750016451
Brody J., Vorhees D., Melly S., Swedis S., Drivas P., Rudel R. (2002). Using GIS and historical records to reconstruct residential exposure to large-scale pesticide application. J. Exposure Sci. Environ. Epidemiol. 12 (1), 64–80. doi: 10.1038/sj.jea.7500205
Chasen E., Steffan S. (2016). Temperature-mediated growth thresholds of Acrobasis vaccinii (Lepidoptera: Pyralidae). Environ. Entomology 45 (3), 732–736. doi: 10.1093/ee/nvw053
Clark J., Tessier D., Marion J. (1994). Mitigation of airborne parathion residues from treated cranberry bog environments bordering suburban areas by a spray adjuvant. J. Environ. Sci. Health Part A 29 (1), 215–236. doi: 10.1080/10934529409376030
Cockfield S. D., Butkewich S., Samoil K., Mahr D. L. (1994a). Forecasting flight activity of Sparganothis sulfureana (Lepidoptera: Tortricidae) in cranberries. J. Economic Entomology 87 (1), 193–196. doi: 10.1093/jee/87.1.193
Cockfield S. D., Fitzpatrick S., Giles K., Mahr D. L. (1994b). Hatch of blackheaded fireworm (Lepidoptera: Tortricidae) eggs and prediction with temperature-driven models. Environ. Entomology 23 (1), 101–107. doi: 10.1093/ee/23.1.101
Cockfield S. D., Fitzpatrick S., Patten K., Henderson D., Dittl T., Poole A., et al. (1994c). Modeling of blackheaded fireworm (Lepidoptera: Tortricidae) oviposition and pheromone-trap catches. J. Economic Entomology 87 (3), 787–792. doi: 10.1093/jee/87.3.787
Cockfield S. D., Mahr D. L. (1992). Flooding cranberry beds to control blackheaded fireworm (Lepidoptera: Tortricidae). J. Economic Entomology 85 (6), 2383–2388. doi: 10.1093/jee/85.6.2383
Cockfield S. D., Mahr D. L. (1994). Prediction models for flight activity of the cranberry girdler (Lepidoptera: Pyralidae) in Wisconsin. Great Lakes Entomologist 27 (2), 107–112.
Cook M., Fitzpatrick S., Roitberg B. (2012). Phenology of Dasineura oxycoccana (Diptera: Cecidomyiidae) on cranberry and blueberry indicates potential for gene flow. J. Economic Entomology 105 (4), 1205–1213. doi: 10.1603/ec11418
Cook M., Ozeroff S., Fitzpatrick S., Roitberg B. (2011). Host-associated differentiation in reproductive behaviour of cecidomyiid midges on cranberry and blueberry. Entomologia Experimentalis Applicata 141 (1), 8–14. doi: 10.1111/j.1570-7458.2011.01166.x
Dara S. K. (2019). The new integrated pest management paradigm for the modern age. J. Integrated Pest Manage. 10 (1), 12, 1–12, 9. doi: 10.1093/jipm/pmz010
Davenport J., Schiffhauer D. (2000). Cultivar influences cranberry response to surface sanding. Hortscience 35 (1), 53–54. doi: 10.21273/hortsci.35.1.53
de Lange E., Kyryczenko-Roth V., Johnson-Cicalese J., Davenport J., Vorsa N., Rodriguez-Saona C. (2019a). Increased nutrient availability decreases insect resistance in cranberry. Agric. For. Entomology 21 (3), 326–335. doi: 10.1111/afe.12335
de Lange E., Rodriguez-Saona C. (2015a). “Blunt-nosed leafhopper: a vector of cranberry false blossom disease,” New Brunswick, New Jersey: Rutgers New Jersey Agricultural Experiment Station. Available at: https://njaes.rutgers.edu/fs1248/.
de Lange E., Rodriguez-Saona C. (2015b). “Spotted fireworm: a cranberry pest in new Jersey,” New Brunswick, New Jersey: Rutgers New Jersey Agricultural Experiment Station. Available at: https://njaes.rutgers.edu/fs1247/.
de Lange E., Salamanca J., Polashock J., Rodriguez-Saona C. (2019b). Genotypic variation and phenotypic plasticity in gene expression and emissions of herbivore-induced volatiles, and their potential tritrophic implications, in cranberries. J. Chem. Ecol. 45 (3), 298–312. doi: 10.1007/s10886-018-1043-0
Demoranville C., Sandler H., Shumaker D., Averill A., Caruso F., Sylvia M., et al. (2005). Fall flooding for management of cranberry fruitworm (Acrobasis vaccinii) and dewberry (Rubus hispidus) in Massachusetts cranberry production. Crop Prot. 24 (11), 999–1006. doi: 10.1016/j.cropro.2005.01.024
Deutsch A., Rodriguez-Saona C., Kyryczenko-Roth V., Sojka J., Zalapa J., Steffan S. (2014). Degree-day benchmarks for Sparganothis sulfureana (Lepidoptera: Tortricidae) development in cranberries. J. Economic Entomology 107 (6), 2130–2136. doi: 10.1603/ec14261
Deutsch A., Rodriguez-Saona C., Zalapa J., Steffan S. (2015). Temperature-mediated development thresholds of Sparganothis sulfureana (Lepidoptera: Tortricidae) in cranberries. Environ. Entomology 44 (2), 400–405. doi: 10.1093/ee/nvu062
Dixon P., Hillier N. (2005). Insect pests of wild cranberry, Vaccinium macrocarpon, in Newfoundland and Labrador. Phytoprotection 83 (3), 139–145. doi: 10.7202/706237ar
Dobroscky I. D. (1931). Studies on cranberry false blossom disease and its insect vector. Contributions Boyce Thompson Institute 3, 59–83.
Drolet I., Guay J., Fournier V., Cloutier C. (2019). Biodiversity of lepidopteran pests and their parasitoids in organic and conventional cranberry crop. Biol. Control 129, 24–36. doi: 10.1016/j.biocontrol.2018.11.003
Fadamiro H. Y., Cosse A. A., Dittl T., Baker T. C. (1998). Suppression of mating by blackheaded fireworm (Lepidoptera: Tortricidae) in Wisconsin cranberry marshes by using MSTRS devices. J. Agric. Entomology 15 (4), 377–386.
FAOSTAT (2022) Cranberries. food and agriculture organization of the United Nations. Available at: https://www.fao.org/faostat/en/#data/QCL/visualize.
Fitzpatrick S. (2006). Delayed mating reduces fecundity of blackheaded fireworm, Rhopobota naevana, on cranberry. Entomologia Experimentalis Applicata 120 (3), 245–250. doi: 10.1111/j.1570-7458.2006.00452.x
Fitzpatrick S. (2007). Survival of submerged larvae of cranberry girdler, Chrysoteuchia topiaria, in the laboratory. Crop Prot. 26 (12), 1810–1816. doi: 10.1016/j.cropro.2007.03.016
Fitzpatrick S. (2009). Insect life histories in fruit, shoot and root environments of cranberry and blueberry. Acta Hortic. 810, 231–250. doi: 10.17660/ActaHortic.2009.810.31
Fitzpatrick S., Gries R., Khaskin G., Peach D., Iwanski J., Gries G. (2013). Populations of the gall midge Dasineura oxycoccana on cranberry and blueberry produce and respond to different sex pheromones. J. Chem. Ecol. 39 (1), 37–49. doi: 10.1007/s10886-012-0230-7
Fitzpatrick S., Troubridge J. (1992). Relative efficacies of two commercial pheromone blends for monitoring the blackheaded fireworm (Lepidoptera: Tortricidae) of cranberries. J. Economic Entomology 85 (3), 947–949. doi: 10.1093/jee/85.3.947
Fitzpatrick S., Troubridge J., Henderson D. (2000). Ochropleura implecta (Lepidoptera: Noctuidae), a new cutworm pest of cranberries. Can. Entomologist 132 (3), 365–367. doi: 10.4039/ent132365-3
Fitzpatrick S., Troubridge J., Maurice C., White J. (1995). Initial studies of mating disruption of the blackheaded fireworm of cranberries (Lepidoptera: Tortricidae). J. Economic Entomology 88 (4), 1017–1023. doi: 10.1093/jee/88.4.1017
Fitzpatrick S., Wong W., Matthews K., Mathur S., Elsby M., Schurmann K., et al. (2018). A bucket-type emergence trap for detecting overwintered Dasineura oxycoccana (Diptera: Cecidomyiidae) and its parasitoids in cranberry. Florida Entomologist 101 (4), 695. doi: 10.1653/024.101.0410
Foye S., Steffan S. (2019). Two native Wisconsin nematodes represent virulent biocontrol agents in cranberries. Biol. Control 138, 104042. doi: 10.1016/j.biocontrol.2019.104042
Gareau B. J., Huang X., Gareau T. P. (2018). Social and ecological conditions of cranberry production and climate change attitudes in New England. PloS One 13 (12), e0207237. doi: 10.1371/journal.pone.0207237
Guédot C., McMahan E. (2015). Sparganothis fruitworm. university of Wisconsin – Extension. Cranberry Crop Manage. J. 28 (11), 8–10.
Guédot C., Perry R. S. (2015). Evaluation of foliar insecticides for the control of cranberry fruitworm and Sparganothis fruitworm in cranberry, 2014. Arthropod Manage. Tests 40 (1), 1–2. doi: 10.1093/amt/tsv017
Guédot C., Perry R. S. (2016a). Evaluation of foliar insecticides for the control of tipworm in cranberry, 2015. Arthropod Manage. Tests 40 (1), 1–2. doi: 10.1093/amt/tsv020
Guédot C., Perry R. S. (2016b). Evaluation of foliar insecticides for the control of Rhopobota naevana and Eupithecia miserulata in cranberry, 2015. Arthropod Manage. Tests 41 (1), 1–2. doi: 10.1093/amt/tsw112
Haase-Statz C. (1997). Evaluation of Paederus littorarius (Coleoptera: Staphylinidae) as an egg predator of Chrysoteuchia topiaria (Lepidoptera: Pyralidae) in Wisconsin cranberry bogs. Great Lakes Entomologist 30 (2), 93–98.
Hancock J. F., Lyrene P., Finn C. E., Vorsa N., Lobos G. A. (2008). “Blueberries and cranberries,” in Temperate fruit crop breeding. Ed. Hancock J. F. (Dordrecht, The Netherlands: Springer), 115–150.
Hayes A., Fitzpatrick S., Webster J. (1999). Infectivity, distribution, and persistence of the entomopathogenic nematode Steinernema carpocapsae all strain (Rhabditida: Steinernematidae) applied by sprinklers or boom sprayer to dry-pick cranberries. J. Economic Entomology 92 (3), 539–546. doi: 10.1093/jee/92.3.539
Hu Z. (2020). What socio-economic and political factors lead to global pesticide dependence? a critical review from a social science perspective. Int. J. Environ. Res. Public Health 17, 8119. doi: 10.3390/ijerph17218119
Jaffe B., Rink S., Guédot C. (2021a). Life history and damage by Systena frontalis F. (Coleoptera: Chrysomelidae) on Vaccinium macrocarpon Ait. J. Insect Sci. 21 (1), 1–8. doi: 10.1093/jisesa/ieab004
Jaffe B., Wallin M., Fox M., Guédot C. (2021b). Cranberry (Vaccinium macrocarpon) is a marginal host for brown marmorated stink bug. J. Economic Entomology 114 (3), 1401–1405. doi: 10.1093/jee/toab032
Kennedy C. D., Jeranyama P., Alverson N. (2016). Agricultural water requirements for commercial production of cranberries. Can. J. Soil Sci. 97 (1), 38–45. doi: 10.1139/cjss-2015-0095
Koppenhöfer A., Rodriguez-Saona C., Polavarapu S., Holdcraft R. (2008). Entomopathogenic nematodes for control of Phyllophaga georgiana (Coleoptera: Scarabaeidae) in cranberries. Biocontrol Sci. Technol. 18 (1), 21–31. doi: 10.1080/09583150701721705
Labarre D., Piché-Luneau S., Gervais F., Légaré J., Cormier D., Lucas E. (2020). Fruit damages caused by the broad-winged bush katydid, Scudderia pistillata (Orthoptera: Tettigoniidae) in commercial cranberry bog. J. Appl. Entomology 144 (9), 830–833. doi: 10.1111/jen.12817
Le Duc I., Turcotte C., Allard F. (2004). “Eastern Canada Cranberry IPM manual,” Canada: Health Canada Department of the Government of Canada.
Li S., Sirois G., Luczynski A., Henderson D. (1993). Indigenous Trichogramma (Hymenoptera: Trichogrammatidae) parasitizing eggs of Rhopobota naevana (Lepidoptera: Tortricidae) on cranberries in British Columbia. Entomophaga 38 (3), 313–315. doi: 10.1007/bf02374447
Long B., Averill A. (2003). Compensatory response of cranberry to simulated damage by cranberry weevil (Anthonomus musculus say) (Coleoptera: Curculionidae). J. Economic Entomology 96 (2), 407–412. doi: 10.1603/0022-0493-96.2.407
Lu C., Hung Y. T., Cheng Q. (2020). A review of sub-lethal neonicotinoid insecticides exposure and effects on pollinators. Curr. pollut. Rep. 6, 137–151. doi: 10.1007/s40726-020-00142-8
Luck B., Chasen E., Williams P., Steffan S. (2021). Drones that deliver: Pheromone-based mating disruption deployed via uncrewed aerial vehicles in U.S. cranberries. J. Economic Entomology 114 (5), 1910–1916. doi: 10.1093/jee/toab068
Madore L. (2010). Cranberry integrated pest management final report (Canada: Forestry and Agrifoods Agency). Available at: https://www.gov.nl.ca/ffa/files/publications-pdf-cranberry-pest-10.pdf.
Magarey R. D., Chappell T. M., Trexler C. M., Pallipparambil G. R., Hain E. F. (2019). Social ecological system tools for improving crop pest management. J. Integrated Pest Manage. 10 (1), 2, 1–2, 6. doi: 10.1093/jipm/pmz004
Mahr D. L. (2005). Cranberry tipworm (Madison, Wisconsin: University of Wisconsin – Madison). Available at: https://s3.wp.wsu.edu/uploads/sites/2166/2018/01/Cranberry-Tipworm.pdf.
Marchand D., McNeil J. (2004a). Avoidance of intraspecific competition via host modification in a grazing, fruit-eating insect. Anim. Behav. 67 (3), 397–402. doi: 10.1016/j.anbehav.2003.03.017
Marchand D., McNeil J. (2004b). The importance of behavioral plasticity for maximizing foraging efficiency in frugivorous lepidopteran larvae. J. Insect Behav. 17 (5), 673–684. doi: 10.1023/b:joir.0000042548.24435.d0
Marchand D., McNeil J. (2006). Is fruit size important in the selection of oviposition sites by cranberry fruitworm, Acrobasis vaccinii? Entomologia Experimentalis et Applicata 119 (3), 213–219. doi: 10.1111/j.1570-7458.2006.00424.x
McGregor R., Henderson D. (1998). The influence of oviposition experience on response to host pheromone in Trichogramma sibericum (Hymenoptera: Trichogrammatidae). J. Insect Behav. 11 (5), 621–632. doi: 10.1023/a:1022390608064
McMahan E., Guédot C. (2018). Development of Sparganothis sulfureana (Lepidoptera: Tortricidae) on cranberry cultivars. Insects 9 (1), 4. doi: 10.3390/insects9010004
McMahan E., Steffan S., Guédot C. (2016). Population densities of lepidopteran pests in selected cranberry cultivars in Wisconsin. J. Economic Entomology 110 (3), 1113–1119. doi: 10.1093/jee/tow274
Mechaber W. L. (1992). Ecology of Anthonomus musculus: Hostplant finding and oviposition by cranberry weevil (Medford, Massachusetts: Tufts University, Medford, MA).
Medina R., Szendrei Z., Harrison K., Isaacs R., Averill A., Malo E., et al. (2013). Exploring host-associated differentiation in the north American native cranberry fruitworm, Acrobasis vaccinii, from blueberries and cranberries. Entomologia Experimentalis Applicata 150 (2), 136–148. doi: 10.1111/eea.12143
Miller B., Bruck D., Walton V. (2012). Relationship of black vine weevil egg density and damage to two cranberry cultivars. Hortscience 47 (6), 755–761. doi: 10.21273/hortsci.47.6.755
Miller S., Patel N., Stanley C. (2006). Cranberry pests and diseases in new Zealand. Acta Hortic. 715), 509–512. doi: 10.17660/actahortic.2006.715.78
Neto C., Dao C., Salvas M., Autio W., Vanden Heuvel J. (2010). Variation in concentration of phenolic acid derivatives and quercetin glycosides in foliage of cranberry that may play a role in pest deterrence. J. Am. Soc. Hortic. Sci. 135 (6), 494–500. doi: 10.21273/jashs.135.6.494
Page M. J., McKenzie J. E., Bossuyt P. M., Boutron I., Hoffmann T. C., Mulrow C. D., et al. (2021). The PRISMA 2020 statement: An updated guideline for reporting systematic reviews. Systematic Rev. 10, 89. doi: 10.1186/s13643-021-01626-4
Peach D., Huber J., Fitzpatrick S. (2012). Hymenopterous parasitoids of cranberry tipworm (Diptera: Cecidomyiidae) in British Columbia, Canada. Can. Entomologist 144 (3), 487–490. doi: 10.4039/tce.2012.37
Polavarapu S., Lonergan G. (1998). Sex pheromone of Choristoneura parallela (Lepidoptera: Tortricidae): Components and development of a pheromone lure for population monitoring. Environ. Entomology 27 (5), 1242–1249. doi: 10.1093/ee/27.5.1242
Polavarapu S., Lonergan G., Peng H., Neilsen K. (2001). Potential for mating disruption of Sparganothis sulfureana (Lepidoptera: Tortricidae) in cranberries. J. Economic Entomology 94 (3), 658–665. doi: 10.1603/0022-0493-94.3.658
Polavarapu S., Stuart R. J., Schiffauer D. (2000). Evaluation of insecticides against cranberry rootwor. Arthropod Manage. Tests 21 (1), 1. doi: 10.1093/amt/25.1.C9
Pradit N., Mescher M., De Moraes C., Rodriguez-Saona C. (2020). Phytoplasma infection of cranberry affects development and oviposition, but not host-plant selection, of the insect vector Limotettix vaccinii. J. Chem. Ecol 46 (8), 722–734. doi: 10.1007/s10886-019-01137-6
Pradit N., Mescher M., Wang Y., Vorsa N., Rodriguez-Saona C. (2019). Phytoplasma infection of cranberries benefits non-vector phytophagous insects. Front. Ecol. Evol 7, 181. doi: 10.3389/fevo.2019.00181
Putnam R., Nelson J., Clark J. (2002). The persistence and degradation of chlorothalonil and chlorpyrifos in a cranberry bog. J. Agric. Food Chem 51 (1), 170–176. doi: 10.1021/jf020744r
Rajabi M. (2014). “Food quality protection act,” in Encyclopedia of toxicology, 3rd Edn, vol. 2. Ed. Wexler P. (San Diego, CA, USA: Elsevier), pp. 637–638.
Rioux M.-C. (2018). Portrait-diagnostic sectoriel de la canneberge au québec, Québec, Canada: Ministère de l’Agriculture of the Government of Canada. Available at: http://collections.banq.qc.ca/ark:/52327/3448642.
Robbins P. S., Nojima S., Polavarapu S., Koppenhöfer A., Rodriguez-Saona C., Holdcraft R., et al. (2009). Sex pheromone of the scarab beetle Phyllophaga (Phytalus) georgiana (Horn). J. Chem. Ecol. 35, 336–341. doi: 10.1007/s10886-009-9593-9
Robbins P. S., Zhang A., Averill A., Linn C., Roelofs W., Sylvia M., et al. (2006). Sex pheromone of the cranberry root grub Lichnanthe vulpina. J. Chem. Ecol. 32 (8), 1663–1672. doi: 10.1007/s10886-006-9100-5
Rodriguez-Saona C., Alborn H., Oehlschlager C., Calvo C., Kyryczenko-Roth V., Tewari S., et al. (2020a). Fine-tuning the composition of the cranberry weevil (Coleoptera: Curculionidae) aggregation pheromone. J. Appl. Entomology 144 (5), 417–421. doi: 10.1111/jen.12752
Rodriguez-Saona C., Byers J., Schiffhauer D. (2012). Effect of trap color and height on captures of blunt-nosed and sharp-nosed leafhoppers (Hemiptera: Cicadellidae) and non-target arthropods in cranberry bogs. Crop Prot. 40, 132–144. doi: 10.1016/j.cropro.2012.05.005
Rodriguez-Saona C., Holdcraft R., Kyryczenko-Roth V. (2019). Blunt-nosed leafhopper control on cranberries 2018. Arthopod Manage. Tests 44 (1), 1–2. doi: 10.1093/amt/tsz009
Rodriguez-Saona C., Kaplan I., Braasch J., Chinnasamy D., Williams L. (2011a). Field responses of predaceous arthropods to methyl salicylate: A meta-analysis and case study in cranberries. Biol. Control 59 (2), 294–303. doi: 10.1016/j.biocontrol.2011.06.017
Rodriguez-Saona C., Kyryczenko-Roth V., Schiffhauer D., Firbas N. (2020b). Characterizing the feeding injury caused by Phylloscelis rubra (Hemiptera: Dictyopharidae) to cranberries. J. Insect Sci. 20 (6), 1–9. doi: 10.1093/jisesa/ieaa143
Rodriguez-Saona C., Polashock J., Kyryczenko-Roth V., Holdcraft R., Jimenez-Gonzalez G., De Moraes C., et al. (2021). Application of plant defense elicitors fails to enhance herbivore resistance or mitigate phytoplasma infection in cranberries. Front. Plant Sci. 12. doi: 10.3389/fpls.2021.700242
Rodriguez-Saona C., Polashock J., Malo E. (2013). Jasmonate-mediated induced volatiles in the American cranberry, Vaccinium macrocarpon: From gene expression to organismal interactions. Front. Plant Sci. 4. doi: 10.3389/fpls.2013.00115
Rodriguez-Saona C., Urbaneja-Bernat P., Salamanca J., Garzón-Tovar V. (2020c). Interactive effects of an herbivore-induced plant volatile and color on an insect community in cranberry. Insects 11 (8), 524. doi: 10.3390/insects11080524
Rodriguez-Saona C., Vorsa N., Singh A., Johnson-Cicalese J., Szendrei Z., Mescher M., et al. (2011b). Tracing the history of plant traits under domestication in cranberries: potential consequences on anti-herbivore defences. J. Exp. Bot. 62 (8), 2633–2644. doi: 10.1093/jxb/erq466
Rodriguez-Saona C., Wanumen A., Salamanca J., Holdcraft R., Kyryczenko-Roth V. (2016). Toxicity of insecticides on various life stages of two tortricid pests of cranberries and on a non-target predator. Insects 7 (2), 15. doi: 10.3390/insects7020015
Roland J. (1990). Use of alternative plant species as a monitoring tool for the cranberry girdler (Lepidoptera: Pyralidae). Environ. Entomology 19 (3), 721–724. doi: 10.1093/ee/19.3.721
Salamanca J., Souza B., Kyryczenko-Roth V., Rodriguez-Saona C. (2019). Methyl salicylate increases attraction and function of beneficial arthropods in cranberries. Insects 10 (12), 423. doi: 10.3390/insects10120423
Salamanca J., Souza B., Lundgren J., Rodriguez-Saona C. (2017). From laboratory to field: electro-antennographic and behavioral responsiveness of two insect predators to methyl salicylate. Chemoecology 27 (2), 51–63. doi: 10.1007/s00049-017-0230-8
Sandler H., Mason J. (1997). Evaluation of three bioinsecticides for control of lepidopteran pests in cranberries. Acta Hortic. 446, 447–456. doi: 10.17660/actahortic.1997.446.65
Simser D. (1995). Parasitism of cranberry fruitworm (Acrobasis vaccinii; Lepidoptera: Pyralidae) by endemic or released Trichogramma pretiosum (Hymenoptera: Trichogrammatidae). Great Lakes Entomologist 27 (4), , 189–196.
Stang E. J. (1997). The emerging cranberry industry in Chile. Acta Hortic. 146, 159–164. doi: 10.17660/ActaHortic.1997.446.23
Statistics Canada (2022). Table 32-10-0364-01 area, production and farm gate value of marketed fruits. Canada: Statistics Canada Department of the Government of Canada. doi: 10.25318/3210036401-eng
Steffan S., Chasen E., Deutsch A., Mafra-Neto A. (2017a). Multi-species mating disruption in cranberries (Ericales: Ericaceae): Early evidence using a flowable emulsion. J. Insect Sci. 17 (2), 1–6. doi: 10.1093/jisesa/iex025
Steffan S., Lee J., Singleton M., Vilaire A., Walsh D., Lavine L., et al. (2013). Susceptibility of cranberries to Drosophila suzukii (Diptera: Drosophilidae). J. Economic Entomology 106 (6), 2424–2427. doi: 10.1603/ec13331
Steffan S., Singleton M., Sojka J., Chasen E., Deutsch A., Zalapa J., et al. (2017b). Flight synchrony among the major moth pests of cranberries in the upper Midwest, USA. Insects 8 (1), 26. doi: 10.3390/insects8010026
Stern V. M., Smith R. F., van den Bosch R., Hagen K. S. (1959). The integration of chemical and biological control of the spotted alfalfa aphid: The integrated control concept. Hilgardia 29 (2), 81–101. doi: 10.3733/hilg.v29n02p081
Stuart R., Polavarapu S. (1998). Oviposition preferences of the polyphagous moth Choristoneura parallela (Lepidoptera: Tortricidae): Effects of plant species, leaf size, and experimental design. Environ. Entomology 27 (1), 102–109. doi: 10.1093/ee/27.1.102
Stuart R., Polavarapu S. (2000). Egg-mass variability and differential parasitism of Choristoneura parallela (Lepidoptera: Tortricidae) by endemic Trichogramma minutum (Hymenoptera: Trichogrammatidae). Ann. Entomological Soc. America 93 (5), 1076–1084. doi: 10.1603/0013-8746(2000)093[1076:EMVADP]2.0.CO;2
Sylvia M. M., Averill A. L. (2013). “Managing sparganothis fruitworm,” University of Massachusetts Cranberry Experiment Station, East Wareham, MA. East Wareham, Massachusetts: UMass Extension. Available at: http://www.umass.edu/cranberry/downloads/Spag2013%20updated%202014.pdf.
Szendrei Z., Averill A., Alborn H., Rodriguez-Saona C. (2011). Identification and field evaluation of attractants for the cranberry weevil, Anthonomus musculus say. J. Chem. Ecol. 37 (4), 387–397. doi: 10.1007/s10886-011-9938-z
Teixeira L., Averill A. (2006). Evaluation of flooding for cultural control of Sparganothis sulfureana (Lepidoptera: Tortricidae) in cranberry bogs. Environ. Entomology 35 (3), 670–675. doi: 10.1603/0046-225x-35.3.670
Tewari S., Buonaccorsi J., Averill A. (2012). Injury to apical meristem of cranberry by Dasineura oxycoccana (Diptera: Cecidomyiidae) reduces production of floral-units in the next growing season. J. Economic Entomology 105 (4), 1366–1378. doi: 10.1603/ec11395
Tewari S., Buonaccorsi J., Averill A. (2013). Impact of early season apical meristem injury by gall inducing tipworm (Diptera: Cecidomyiidae) on reproductive and vegetative growth of cranberry. J. Economic Entomology 106 (3), 1339–1348. doi: 10.1603/ec12269
Tewari S., Buonaccorsi J., Averill A. (2014). Physiological integration plays key role in cranberry (Ericales: Ericaceae) for tolerance of damage by Dasineura oxycoccana (Diptera: Cecidomyiidae). Environ. Entomology 43 (1), 75–82. doi: 10.1603/en13231
Theilmann D. A., Fitzpatrick S. M., Skelton F. (1995). Identification of a granulosis virus associated with mortality in the blackheaded fireworm, Rhopobota naevana (Hübner) (Lepidoptera, tortricidae). J. Invertebrate Pathol. 66 (2), 209–211. doi: 10.1006/jipa.1995.1089
Tjiurutue M., Sandler H., Kersch-Becker M., Theis N., Adler L. (2017). Gypsy moth herbivory induced volatiles and reduced parasite attachment to cranberry hosts. Oecologia 185 (1), 133–145. doi: 10.1007/s00442-017-3915-3
USDA NASS (2022). “Cranberries,” United states department of agriculture, national agricultural statistics service. United States of America: The United States Department of Agriculture. Available at: https://quickstats.nass.usda.gov/results/0E92F055-AC10-3E0D-9B49-68AFFE026EB5.
van Zoeren J., Guédot C., Steffan S. (2018). Conserving carnivorous arthropods: an example from early-season cranberry (Ericaceae) flooding. Can. Entomologist 150 (2), 265–273. doi: 10.4039/tce.2017.66
Vöing K., Harrison A., Soby S. (2017). Draft genome sequence of Chromobacterium subtsugae MWU12-2387 isolated from a wild cranberry bog in Truro, Massachusetts. Genome Announcements 5 (12), e01633–16. doi: 10.1128/genomea.01633-16
Vorsa N., Johnson-Cicalese J. (2012). “American Cranberry,” in Fruit breeding: handbook of plant breeding, vol. 8 . Eds. Badenes M. L., Byrne D. H. (Boston, MA: Springer US), 191–223.
Vorsa N., Zalapa J. (2020). “Domestication, genetics, and genomics of the American cranberry,” in Plant breeding reviews, 1st Edn, vol. 43 . Ed. Goldman I. (Hoboken, New Jersey, USA: John Wiley & Sons, Inc), 279–315.
Wan M., Szeto S., Price P. (1995). Distribution and persistence of azinphos‐methyl and parathion in chemigated cranberry bogs. J. Environ. Qual. 24 (4), 589–596. doi: 10.2134/jeq1995.00472425002400040006x
Weber D., Robbins P., Averill A. (2005). Hoplia equina (Coleoptera: Scarabaeidae) and nontarget capture using 2-tetradecanone–baited traps. Environ. Entomology 34 (1), 158–163. doi: 10.1603/0046-225x-34.1.158
Wenninger E., Averill A. (2006). Mating disruption of oriental beetle (Coleoptera: Scarabaeidae) in cranberry using retrievable, point-source dispensers of sex pheromone. Environ. Entomology 35 (2), 458–464. doi: 10.1603/0046-225x-35.2.458
Wheeler W. B. (2002). Role of research and regulation in 50 years of pest management in agriculture. J. Agric. Food Chem. 50, 4151–4155. doi: 10.1021/jf0256438
Ye W., Foye S., MacGuidwin A., Steffan S. (2018). Incidence of Oscheius onirici (Nematoda: Rhabditidae), a potentially entomopathogenic nematode from the marshlands of Wisconsin, USA. J. Nematol. 50 (1), 9–26. doi: 10.21307/jofnem-2018-004
Zeldin E. (2005). “Overview of organic cranberry production,” Madison, Wisconsin: University of Wisconsin -Madison Center for Integrated Agricultural Systems. Available at: https://cias.wisc.edu/wp-content/uploads/sites/194/2008/07/orgcran1.pdf.
Zhang A., Polavarapu S. (2003). Sex pheromone of the cranberry blossom worm, Epiglaea apiata. J. Chem. Ecol. 29 (9), 2153–2164. doi: 10.1023/a:1025646804797
Keywords: Vaccinium macrocarpon, IPM, entomology, literature trends, globalization
Citation: Ben-Zvi Y and Rodriguez-Saona C (2023) Advances in cranberry insect pest management: A literature synthesis. Front. Agron. 5:1006106. doi: 10.3389/fagro.2023.1006106
Received: 28 July 2022; Accepted: 16 February 2023;
Published: 06 March 2023.
Edited by:
Maryke T. Labuschagne, University of the Free State, South AfricaReviewed by:
Giovanni Tamburini, University of Bari Aldo Moro, ItalyGiuseppe Eros Massimino Cocuzza, University of Catania, Italy
Copyright © 2023 Ben-Zvi and Rodriguez-Saona. This is an open-access article distributed under the terms of the Creative Commons Attribution License (CC BY). The use, distribution or reproduction in other forums is permitted, provided the original author(s) and the copyright owner(s) are credited and that the original publication in this journal is cited, in accordance with accepted academic practice. No use, distribution or reproduction is permitted which does not comply with these terms.
*Correspondence: Yahel Ben-Zvi, eS5iekBydXRnZXJzLmVkdQ==
‡ORCID: Yahel Ben-Zvi, orcid.org/0000-0003-3533-3473
Cesar Rodriguez-Saona, orcid.org/0000-0001-5888-1769