- 1University of Arkansas, Department of Crop, Soil, and Environmental Sciences, Fayetteville, AR, United States
- 2Mississippi State University, Department of Plant and Soil Sciences, Starkville, MS, United States
Allelopathy and competition are components of plant-plant interactions, delimiting the level of interference. Understanding this interaction has practical applications in agriculture. Crop cultivars possessing high allelopathic ability and competitive traits are themselves tools for sustainable weed management, enabling reduced use of herbicides. Greenhouse and field experiments were conducted to assess the weed suppressive ability of selected sweetpotato (Ipomoea batatas L.) cultivars. The effect of nine cultivars on Palmer amaranth (Amaranthus palmeri S. Watson), junglerice (Echinochloa colona L.), and hemp sesbania (Sesbania hederacea P. Mill.) was first evaluated in the greenhouse. The experiment was set up in a completely randomized design with four replications and conducted twice. Sweetpotatoes were cultured in sand. The target weeds were seeded in pots filled with a 2:1 mix of field soil:potting mix and watered with 100-ml aliquot of sweetpotato root leachates once every 2 d. Weed height and shoot biomass were measured. ‘Heartogold’, ‘Centennial’, and ‘Stokes Purple’ were the most allelopathic cultivars. Junglerice was most inhibited by sweetpotato leachates. Nine cultivars were evaluated in the field. Experiments were conducted at Fayetteville and Kibler, Arkansas, USA, in a split-plot design, with weed infestation (broadleaf spp., grass spp., or weed-free) as whole plot and the cultivars as split-plot. Across locations, ‘Beauregard-14’ had the longest vines, whereas ‘Hatteras’ and ‘Heartogold’ had the tallest canopy. ‘Heartogold’ had the largest leaf area. This cultivar reduced weed biomass 2- to 4-fold in both locations. Yield was reduced on average 55 and 68% with grass and broadleaf weeds across locations, respectively. ‘Beauregard-14’ and ‘Bayou Belle-6’were the high-yielding cultivars in Kibler and Fayetteville. The highest yielding cultivars were not the most weed suppressive but did not incur the highest yield loss from weed competition, indicating the ability to withstand weed interference. Cultivar competitiveness, weed-suppressive ability, and weed tolerance are important factors that should be considered before making a cultivar selection.
Introduction
Sweetpotato (Ipomea batatas L.) belongs to the Convolvulaceae family and is the cultivated relative of the viny, wild, and weedy Ipomoea spp. The shoot architecture and prostrate growth habit of sweetpotato make this crop particularly susceptible to weed interference, especially before the vines form a closed canopy. The critical period for weed interference in sweetpotato is from 7 d to 56 d after transplanting (DAT); with the most critical time between 30 and 45 DAT (Levett, 1992). Yield losses due to weeds, particularly Palmer amaranth (Amaranthus palmeri S. Watson), could be as high as 79% with 1 to 8 Palmer amaranth plants m−1 of row (Basinger et al., 2019) and 35% to 76% at 1 to 16 large crabgrass (Digitaria sanguinalis L.) plants m−1 of row. Herbicide options are limited in sweetpotato production. Only sethoxydim, clethodim, clomazone, fluazifop, S-metolachlor, and flumioxazin are registered (Monks et al., 2019). Only selective grass herbicides (clethodim, sethoxydim, and fluazifop) can be used for postemergence weed control; all other weeds need to be removed by repeated handweeding (Kemble et al., 2022). To alleviate the cost of handweeding, the row middles can be cultivated before the vines overlap. On average, 95% of growers perform inter-row cultivation three times before vines overlap (J. Haley and J. Curtis, unpublished data). Other practices include handweeding and between-row application of postemergence herbicides, which are performed by 62% and 19% of growers, respectively (J. Haley and J. Curtis, unpublished data).
The lack of herbicide options calls for supplemental practices that provide effective weed control. The development of cultivars with superior competitive ability against weeds could complement cultural and chemical control methods. The recognition of the role of crop competitiveness in weed suppression has been explored in previous studies including corn (Zea mays L.) (Sankula et al., 2004), cotton (Gossypium hirsutum L.) (Chandler and Meredith, 1983), wheat (Triticum aestivum) (Mason et al., 2007), spring barley (Hordeum vulgare L.) (Hansen et al., 2008), and soybeans (Glycine max L. Merr.) (Trezzi et al., 2013). In the last 15 yr, the role of crop competitiveness is becoming even more important considering the widespread occurrence, and continuing evolution of, herbicide-resistant weeds (Harker and O’Donovan, 2013). Cultivar competitiveness is reflected either as: (1) ‘weed suppressive ability’ or (2) ‘tolerance’ to weed infestation, or both (Hansen et al., 2008). The first is related to the ability of a cultivar to reduce the fitness of the surrounding weeds (Christensen, 1995). In this case, competitive cultivars reduce weed emergence, growth, or weed seed production. The second outcome pertains to the ability of some cultivars to tolerate weed infestation and incur less yield loss than cultivars that are less tolerant to weed interference (Lemerle et al., 1996).
The traits contributing to crop advantage against weeds are related to morphological characteristics as being tall, rapid growth, canopy closure, and high leaf area index (Konesky et al., 1989; Balyan et al., 1991; Cudney et al., 1991). In wheat and barley, better weed suppression has been attributed to high leaf area index and wide leaf angle that promotes shading (Hoad et al., 2006; Hansen et al., 2008). In soybeans, indeterminate growth habit and faster canopy development are associated with competitive ability against weeds (Newcomer et al., 1986). Crop competitiveness could also be related to chemical interference among plants (allelopathy) (Jabran et al., 2015; Scavo and Mauromicale, 2021). Allelopathy was first described by Hans Molisch in 1937, referring to the effect of biochemical substances transferred from one plant to another. The utility of allelopathy as a viable component of weed management is well documented in crops including rice (Oryza sativa L.) (Li et al., 2015), wheat (Dadkhah, 2015), canola (Brassica napus L.) (Dadkhah, 2015), and cotton (Ma et al., 2012). Allelopathic metabolites have been found in stems, leaves, and root exudates of sweetpotato cultivars (Xuan et al., 2016). Allelochemical compounds include caffeic acid, chlorogenic acid, coumarin, trans-cinnamic acid, and hydroxy cinnamic acid (Soni et al., 2019). Several sweetpotato cultivars, including ‘Heartogold’, produce high concentrations of allelochemicals that inhibit the growth of Palmer amaranth (Soni et al., 2019). In a screening of 48 sweetpotato cultivars, three (‘Yen 36’, ‘54’, and ‘615’) suppressed cogongrass (Imperata cylindrica L.) germiantion by more than 90% (Xuan et al., 2016). A study of ten sweetpotato cultivars showed that ‘Heartogold’ and ‘529’ from Louisiana (USA) and Guatemala, respectively, had the highest concentration of allelochemicals and reduced Palmer amaranth biomass (39%) and height (≥80%) (Soni et al., 2019). In the same study, ‘Centennial’, ‘Morado’, and ‘Spokes Purple’ were classified as having intermediate allelopathic potential due to the high concentration of coumarin and caffeic acid but caused poor inhibition of Palmer amaranth biomass (≤26%). The composition and quantity of allelochemicals produced vary across cultivars; therefore, it takes great effort to find cultivars with high allelopathic potential and desirable agronomic traits. Ultimately, the differential weed suppression by sweetpotato genotypes reflects the total effect of genetic background (Xuan et al., 2016), weed-competitive morphology, the allelochemicals present, and the quantity of these compounds (Soni et al., 2019).
The objectives of this study were to (1) identify weed-suppressive sweetpotato cultivars, (2) determine the tolerance of sweetpotato cultivars to full-season weed interference, and (3) identify the crop traits contributing to its competitive advantage against weeds.
Materials and methods
Assessment of allelopathic effect in the greenhouse
Greenhouse experiments were conducted in 2020 at the Altheimer Laboratory, University of Arkansas, Fayetteville, USA (36° 5’55.213’’ N,94°10’43.038’’W). Nine sweetpotato cultivars (‘Heartogold’, ‘Centennial’, ‘Evangeline’, ‘Hatteras’, ‘Bayou Belle-2’, ‘Bayou Belle-6’, ‘Beauregard-14’, ‘Beauregard-63’, and ‘Stokes Purple’) were evaluated for allelopathic suppression of seedling growth of Palmer amaranth, junglerice (Echinochloa colona L.), and hemp sesbania (Sesbania herbacea L.) over four weeks. The experimental design was completely randomized with four replications and was conducted twice.
Sweetpotato vines (15-cm, 6 pot-1) were planted in 25-cm pots filled with 2.5 kg play sand and overlayed with 0.2 kg of commercial potting medium (Mycorrhizae®, Quebec, Canada). Each pot was placed in a plastic bucket (4.72 cm x 3.7 cm) and watered with 900 ml of tap water once every two days. The root leachates were collected and applied in 100-ml aliquots to target weeds. The control treatments received 100-ml of tap water. The target weeds were planted in square pots (11 cm x 12 cm) filled with 0.5 kg of silt loam soil (pH 6.7; with P, K, Ca, Mg, S, Na, Fe, Mn, Cu, and B contents of 84, 186, 1326, 273, 5.8, 6.4, 235, 106,4.0, 1.7, and 0.4 mg kg-1, respectively). The field soil was mixed 2:1 with commercial potting medium Mycorrhizae®, Quebec, Canada). Four seedlings were kept per pot and heights were measured once weekly. Plant height was measured from the ground level to the tip of the highest fully expanded leaf. Four weeks after planting, the plants were cut at the soil surface, oven-dried, and weighed. Biomass and height reduction were calculated as:
where the control is the mean biomass of all plants in four control pots which received water only, and the biomass of receiver species was the mean of four plants per pot treated with sweetpotato leachates. Datasets generated from the two experimental runs were pooled and analyzed together. A hierarchical clustering using Ward’s method were performed in JMP 16.1 (SAS Institute Inc., Cary, NC) to group the cultivars based on the overall allelopathic potential.
Field experiments
Field experiments were conducted in Arkansas, USA, in 2021, at the Vegetable Station (35°22’44.249’’ N, 94°13’59.506’’W), Kibler and at the Shult Agricultural Research and Extension Center (36°5’56.786’’ N, 94°10’43.9’’W), Fayetteville. The total rainfall during the growing season was 727 mm in Fayetteville and 731 mm in Kibler (Table 1). The soil in the Fayetteville site was silt loam with pH 7.1 and nutrient contents of P, K, Ca, Mg, S, Na, Fe, Mn, Zn, Cu, and B at 49, 103, 1073, 40, 7.1, 7.4, 88, 213, 2.2,1.3, and 0.4 mg kg-1, respectively. In Kibler the soil was silt loam with pH 7.1 and nutrient contents of P, K, Ca, Mg, S, Na, Fe, Mn, Zn, Cu, and B at 110,101,799, 149, 6.1, 17.3, 229,72, 2.1, 1.0, and 0.4 mg kg-1, respectively.
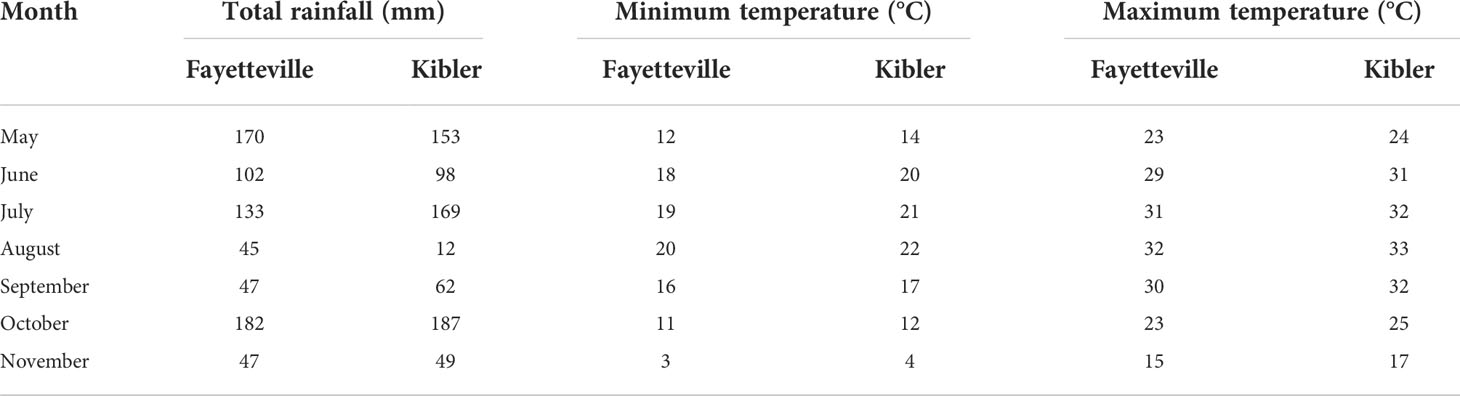
Table 1 Rainfall (mm), minimum and maximum temperature (°C) history for 2021 from May through November in Fayetteville and Kibler, AR, 2021.
The split-plot experiment consisted of (1) weed species as whole plot (broadleaf spp., grass spp., or weed-free), and (2) sweetpotato cultivars as split-plot (‘Heartogold’, ‘Centennial’, ‘Evangeline’, ‘Hatteras’, ‘Bayou Belle-2’, ‘Bayou Belle-6’, ‘Beauregard-14’, ‘Beauregard-63’, and ‘Morado’). A weed-only plot was established as check in each whole plot. The whole plot size was two rows, each 0.9 m wide and 15 m long, which were then subdivided into split-plot consisting of one row, 0.9 m wide and 3.0 m long. One week prior to transplanting the slips, complete fertilizer (13-13-13) was applied at 227 kg ha-1, and the field was bedded. Urea fertilizer (32-0-0) was applied at 45.5 kg ha-1 along the side of sweetpotato plants 8 wk after transplanting (WAT). Cuttings (20- to 30-cm long) were hand-transplanted on May 22, 2021, and June 17, 2021, in Fayetteville and Kibler, respectively. The slips were planted in a horizontal position with two nodes buried, 46 cm apart in the bed. Typically, sweetpotato cuttings are transplanted between mid-May and mid-June. Because of rainfall events, sweetpotato transplanting in Kibler was delayed by four weeks compared to Fayetteville. On the same day as sweetpotato transplanting, plots assigned to broadleaf spp. and grass spp. were broadcast-seeded with Palmer amaranth and junglerice, respectively, at a density of 20 seeds m-2. In the weedy treatments, native weeds were allowed to grow unchecked. Broadleaf weed species were manually removed from grass plots and grasses were controlled in the broadleaf plots with a postemergence application of clethodim (Select Max®, Valent U.S.A. LLC Agricultural Products, Walnut Creek, CA) at 140 g ai ha-1 plus Crop Oil Concentrate (COC) at 0.25% v v-1. Weed-free plots were hand-weeded every other week until 12 WAT.
Data were collected from the two inner plants of each plot. Weeds were counted by species at 5 and 7 WAT from 0.5- by 0.5-m quadrat in each split-plot. The canopy height and length of the longest vine were measured at 5 and 7 WAT. Canopy height was measured from soil surface to the uppermost leaf base. Sweetpotato leaves were collected from 0.13 m2 ground area 1 wk prior to harvest. Leaf area was measured using Li-cor Model 3100 leaf area meter (Li-cor Inc. Lincoln, Nebraska, USA) and then converted to leaf area index (LAI), as follows:
Shoot biomass of weeds was collected from 0.5 x 0.5 m per split-plot 2 wk before harvest. Samples were then placed in a forced-air drier for 120 h at 80°C. Dry biomass was recorded. Sweetpotato storage roots were harvested 153 and 141 d after transplanting (DAT) in Fayetteville and Kibler, respectively. Roots were graded into jumbo (8.9 cm in diameter), no. 1 (≥4.4 cm but <8.9 cm), canner (≥2.5 cm but <4.4 cm), and cull (misshapen roots) (USDA, 2005), then weighed by grade. Total marketable yield was calculated as the sum of jumbo, no. 1, and canner grades.
The phytosociological parameters relative frequency (RF), relative density (RD), relative abundance (RAb), and importance value index (IVI) of broadleaf spp. and grass spp. treatments were assessed with the following equations (Werle et al., 2021):
where RD, RF, and RAb are the number of species, their distribution, and abundance relative to other species in the sampled area, respectively. IVI indicates the most important species in the study area. Total frequency, density, and abundance were obtained from the sum of the relative number of each of the parameters.
In this study, the whole plot effect of weed species, the split-plot effect of sweetpotato cultivars, and the interaction between weed species and cultivars were considered fixed effects. The experiments were analyzed by location. The replications within location and the error associated with the whole plot and residual (split-plot) were considered as random effects. The Restricted Maximum Likelihood (REML) was used to estimate variance components. This experiment can be described with the following linear model:
where Yijk is the response variable, Bli is the random effect of blocks, Aj is the fixed effect of weed species (whole plot) on the response variable, ŋijij is the whole plot error, Bk is the fixed effect of sweetpotato cultivars (split-plot) on the response variable, ABjk is the fixed effect of the interaction between weed species and cultivars, and ϵijk is the split-plot error. Bli, ŋijij, and ϵijk are assumed to be independent of one another. Data were analyzed in JMP® Pro 16.1 (SAS Institute Inc., Cary, NC), and mean values were separated using Student’s t-test. Significant differences between the means were determined at 5% level of probability (p ≤ 0.05).
Results
Biomass and height reduction of weed species in the greenhouse
In the greenhouse, sweetpotato root leachates reduced weed growth in terms of height and shoot biomass. Weeds responded differently to root leachate of sweetpotato cultivars and the inhibitory effects on weeds declined with time, except on Palmer amaranth (Tables 2, 3). Junglerice was the most stunted regardless of sweetpotato cultivar. The maximum height reduction occurred in the first week (27%) and decreased to 16, 11, and 10% in the second, third, and fourth weeks, respectively. Root leachates from ‘Heartogold’, ‘Centennial’, ‘Evangeline’, and ‘Hatteras’ stunted junglerice the most. Height reduction of hemp sesbania was minimal at 13% in the first week and declining to 5% in the fourth week. ‘Beauregard-14’, ‘Beauregard-63’, ‘Evangeline’ and ‘Centennial’ had the highest observable effect on hemp sesbania. Palmer amaranth was the least (<10%) affected by sweetpotato leachates compared to the other weed species.
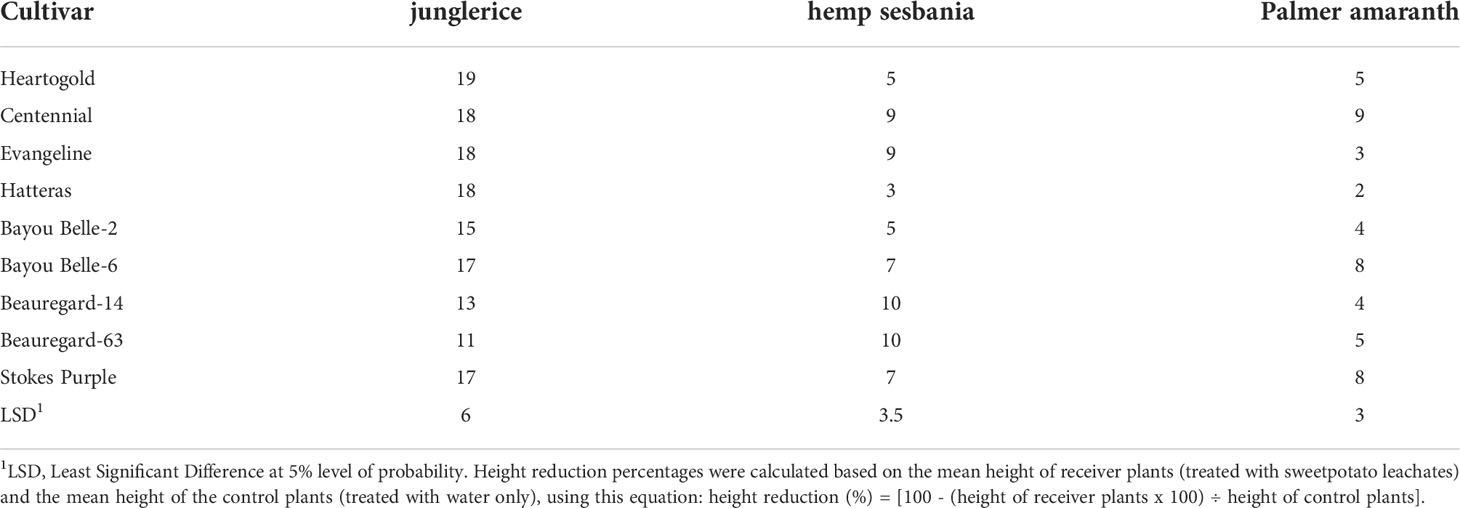
Table 2 Height reduction (%) of hemp sebania (Sesbanea herbacea), junglerice (Echinochloa colona), and Palmer amaranth (Amaranthus palmeri) seedlings when watered with root leachates of nine sweetpotato cultivars averaged over four weeks.
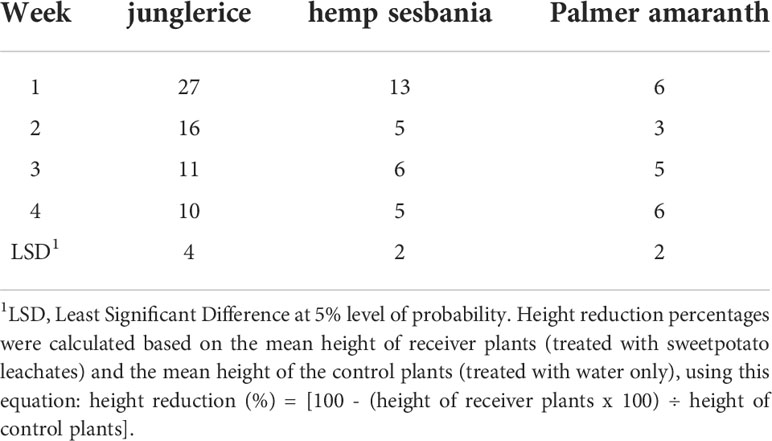
Table 3 Height reduction (%) of hemp sebania (Sesbanea herbacea), junglerice (Echinochloa colona), and Palmer amaranth (Amaranthus palmeri) seedlings when in contact with root leachates of sweetpotato over four weeks averaged across cultivars.
Junglerice biomass was most reduced by root exudates of sweetpotato cultivars compared to hemp sesbania and Palmer amaranth (Table 4). Biomass reduction of hemp sesbania ranged from 2 to 19%. ‘Centennial’ (19%) and ‘Stokes Purple’ (14%) caused the highest biomass reduction of hemp sesbania, but little difference was observed with other cultivars. ‘Stokes Purple’ and ‘Heartogold’ caused the highest numerical reduction of junglerice biomass. Palmer amaranth biomass was reduced only up to 10%.
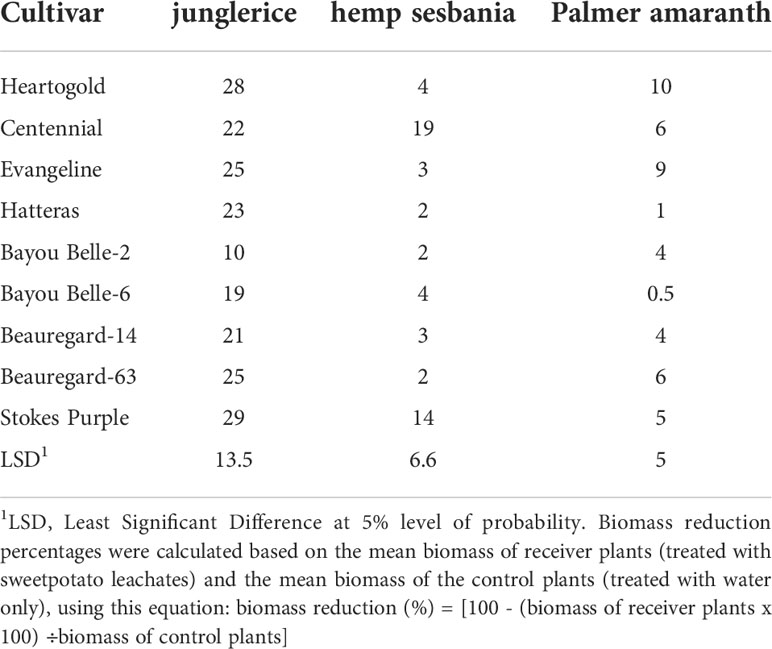
Table 4 Biomass reduction (%) of hemp sebania (Sesbanea herbacea), junglerice (Echinochloa colona), and Palmer amaranth (Amaranthus palmeri) seedlings when in contact with root leachates of nine sweetpotato cultivars at four weeks after emergence.
Allelopathic categories of sweetpotato cultivars
Three dendrograms were created to categorize the sweetpotato cultivars based on allelopathic effect on hemp sesbania, Palmer amaranth, and junglerice using height and biomass reduction data (Figure 1). ‘Centennial’, ‘Beauregard-14’, ‘Beauregard-63’, and ‘Evangeline’ composed the cluster that caused the greatest height reduction of hemp sesbania. ‘Heartogold’ and ‘Bayou Belle-6’ caused moderate height reduction and high biomass reduction of hemp sesbania. For Palmer amaranth, ‘Centennial’, ‘Stokes Purple’, and ‘Bayou Belle-6’ fell in the high height-reduction cluster, whereas ‘Evangeline’ caused the highest biomass reduction. For junglerice, ‘Heartogold’ and ‘Centennial’ caused the greatest height reduction and ‘Stokes Purple’, followed by ‘Beauregard-14’ and ‘Hatteras’ caused the highest biomass reduction.
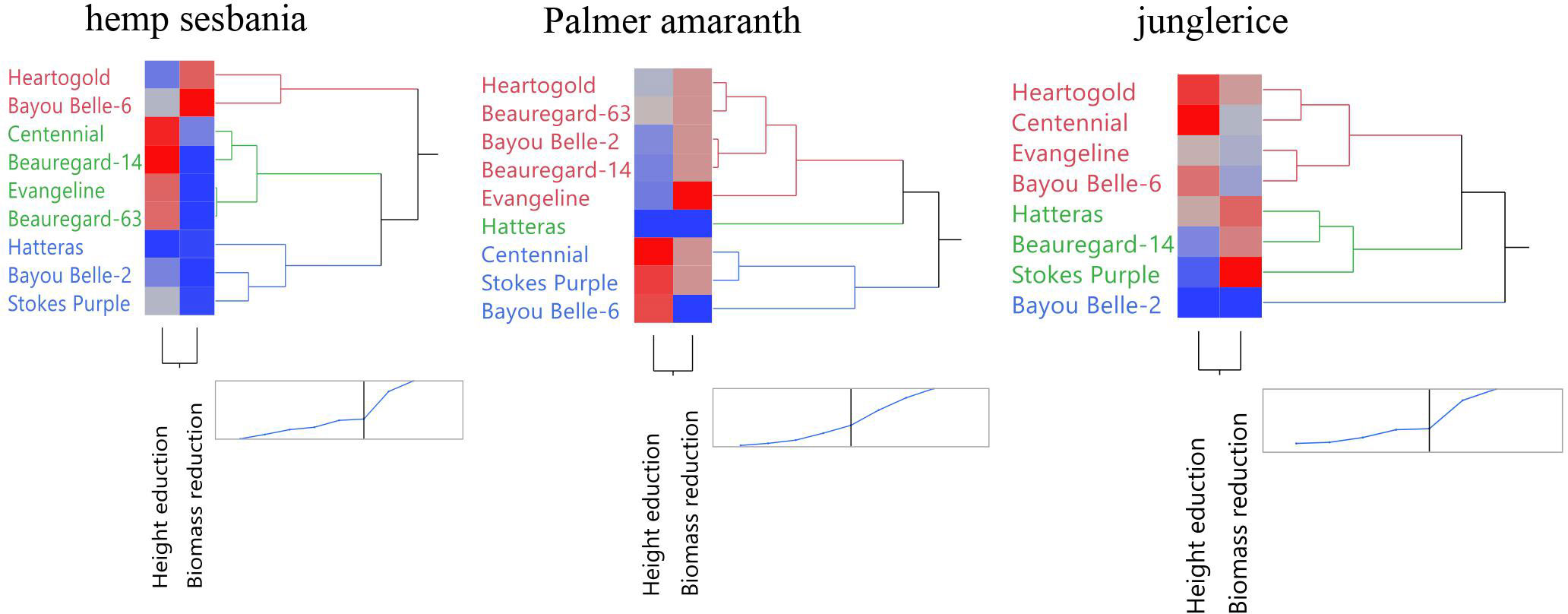
Figure 1 Clustering of sweetpotato cultivars based on height and biomass reduction of hemp sebania (Sesbanea herbacea), junglerice (Echinochloa colona), and Palmer amaranth (Amaranthus palmeri). Blue indicates a lower reduction percentage while red indicates a higher reduction percentage. Accessions grouped based on overall allelopathic potential.
Weed composition in the field
Weed composition differed between the two locations. The weed community was composed of eight broadleaf and nine grass species in Fayetteville (Table 5). The Kibler site had three broadleaf and seven grass species (Table 6). The relative weed frequency (RF), density (RD), abundance (RAb), and overall importance value index (IVI) did not differ between sweetpotato cultivars at 5 and 7 WAT.
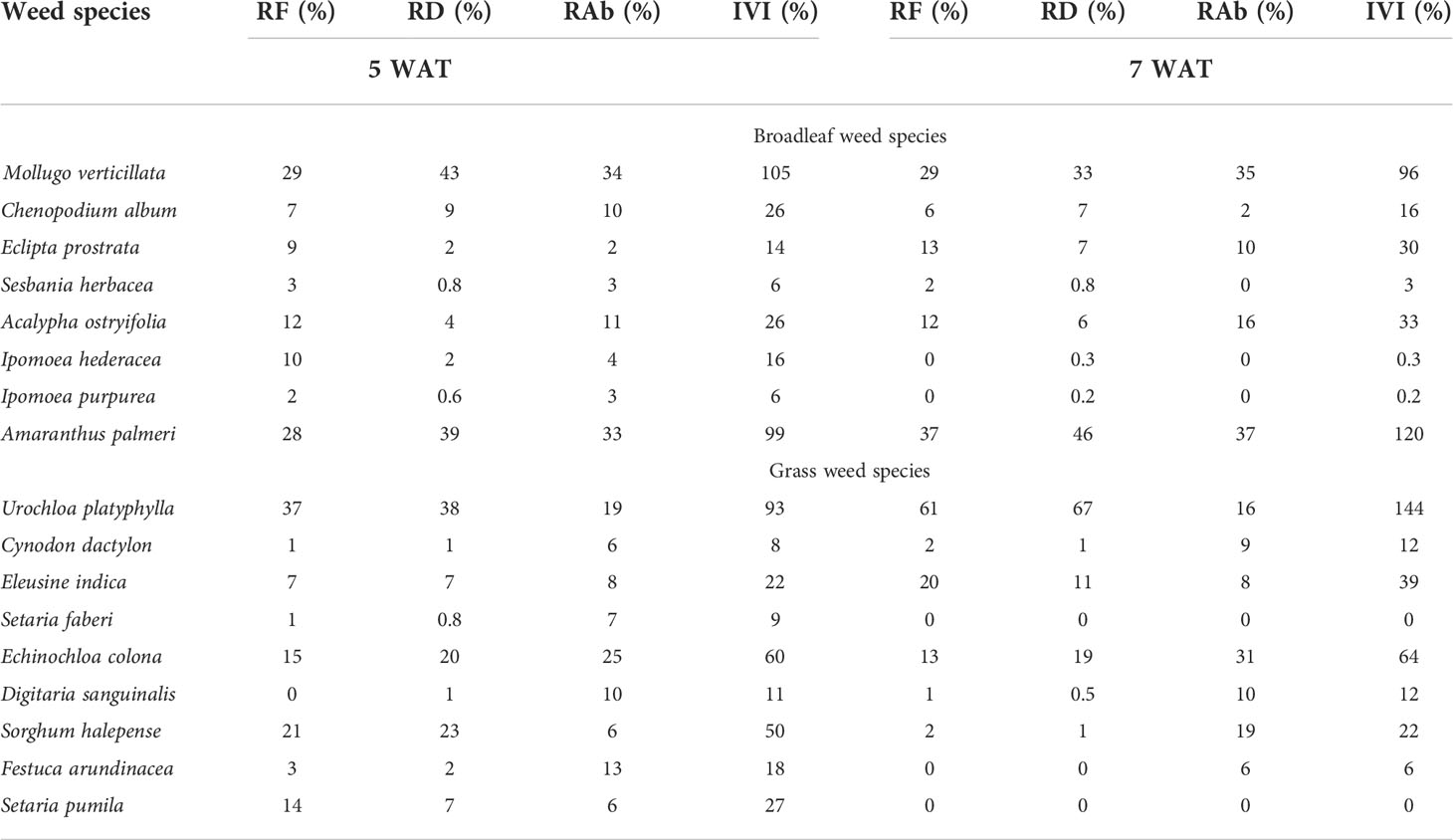
Table 5 Relative frequency (RF), relative density (RD), relative abundance (RAb), and importance value index (IVI) at 5 and 7 weeks after transplanting (WAT) in weedy plots with sweetpotato in Fayetteville, AR, 2021.
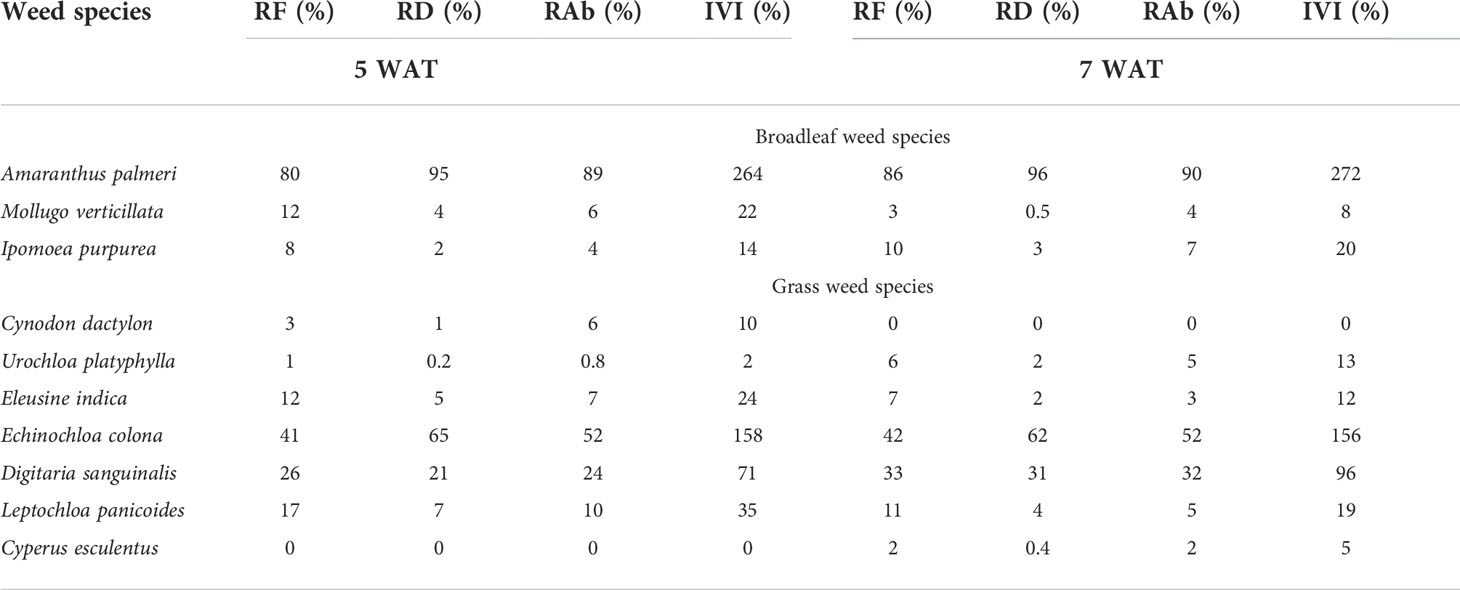
Table 6 Relative frequency (RF), relative density (RD), relative abundance (RAb), and importance value index (IVI) at 5 and 7 weeks after transplanting (WAT) in weedy plots with sweetpotato in Kibler, AR, 2021.
Among the broadleaf species in Fayetteville, carpetweed (Mollugo verticillata L.) and Palmer amaranth had the highest IVI (IVI=96-120%) at 5 WAT and 7 WAT (Table 5). Carpetweed was the most abundant (RD=43%) up to 5 WAT, but Palmer amaranth became most abundant (RD=46%) at 7 WAT. Broadleaf signalgrass [Urochloa platyphylla (Munro ex C. Wright) R.D. Webster] and junglerice were the most predominant grass species in Fayetteville. Broadleaf signalgrass had the highest RF and RD at 5 and 7 WAT and reached an IVI value of 93% and 144% at 5 and 7 WAT, respectively. Junglerice had an IVI value of 60% and 64% at 5 and 7 WAT, respectively, indicating similar importance than broadleaf signalgrass.
Palmer amaranth had the highest RF, RD, RAb, and IVI % among broadleaf weeds at 5 and 7 WAT in Kibler (Table 6). Overall, the relative densities of carpetweed and tall morningglory (Ipomoea purpurea L.) were low (RD<5%), while Palmer amaranth had RD values of approximately 95% at 5 and 7 WAT. Junglerice was the most predominant grass species in Kibler, with an IVI of 158% and 156% at 5 and 7 WAT, respectively. The RF, RD, and RAb values of junglerice remained high (42, 62, and 52%, respectively) at 7 WAT. Large crabgrass was also a dominant grass species in Kibler, showing increased importance with time (IVI =71% and 96% at 5 and 7 WAT, respectively). At 7 WAT, large crabgrass had RF, RD, and RAb values of 33, 31, and 32%, respectively.
Effect of sweetpotato on weed biomass
The cultivar by weed species interaction (p = 0.0382) was significant for dry biomass in Fayetteville (Figure 2). The cultivar by weed species interaction (p = 0.3564) was not significant in Kibler, but sweetpotato cultivars significantly reduced weed biomass (p = 0.0459) regardless of species. Grass weed biomass in weed-only plots in Fayetteville was 593 g m-2. ‘Heartogold’ had the lowest grass weed biomass (166 g m-2). The lowest and highest biomass of broadleaf species in Fayetteville was found in plots with ‘Heartogold’ (693 g m-2) and Hatteras (3,683 g m-2), respectively. In Kibler, the lowest grass spp. biomass was recorded in plots with ‘Beauregard-14’ (518 g m-2), nearly 50% lower than the weed biomass in weed-only plots (1,697 g m-2). ‘Bayou Belle-6’ and ‘Heartogold’ significantly reduced broadleaf spp. biomass to about 40% less biomass than the weedy check.
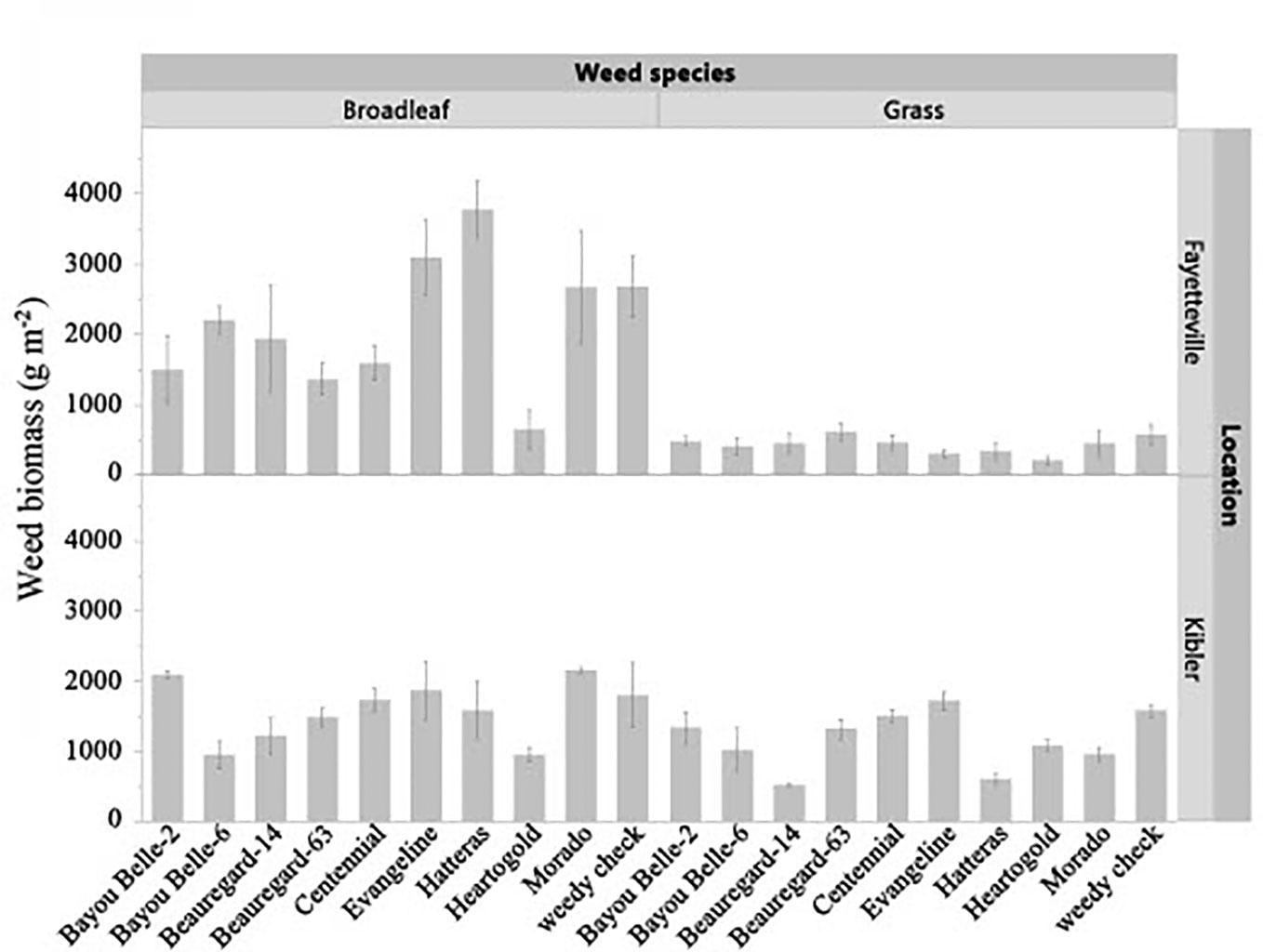
Figure 2 Effect of sweetpotato cultivars on broadleaf spp. and grass spp. biomass (g m-2) in Kibler and Fayetteville, AR, 2021. LSD to compare cultivars within location Fayetteville: 496 g m-2. LSD to compare cultivars within location Kibler: 453 g m-2. Bars represent standard error.
Sweetpotato canopy height, vine length, and leaf area
The interaction effect of cultivar and weed species on vine length and canopy height of sweetpotato was not significant in both locations, but the cultivars differed significantly (p< 0.05) in these traits regardless of the weed species in competition at both evaluation times (Figures 3, 4). The sweetpotato cultivars also differed in leaf area in both locations (p = 0.0001) and weed species (p = 0.0181) in both locations. The vine length and canopy height of sweetpotato cultivars were similar when growing weed-free. These traits also did not differ between cultivars when grown in competition with weeds (broadleaf or grasses). However, regardless of cultivar, sweetpotato vine and leaves were shorter when growing with weeds compared to growing weed-free. ‘Beauregard-14’ and ‘Beauregard-63’ had the longest vines in Fayetteville at 5 WAT, while ‘Beauregard-14’ and ‘Morado’ had the longest vines in Kibler (Figure 3). ‘Beauregard-14’ and ‘Beauregard-63’ remained the most viney in both locations at 7 WAT, although they were no longer differentiated from ‘Bayou Belle-6’ in Fayetteville and were also similar to ‘Morado’ in Kibler. At 5 WAT ‘Hatteras’, ‘Heartogold’, and ‘Centennial’ had the tallest canopy in Fayetteville (18-19 cm) and in Kibler (21-22 cm) (Figure 4). At 7 WAT, ‘Heartogold’ had the tallest canopy in Fayetteville (23 cm) and in Kibler (33 cm).
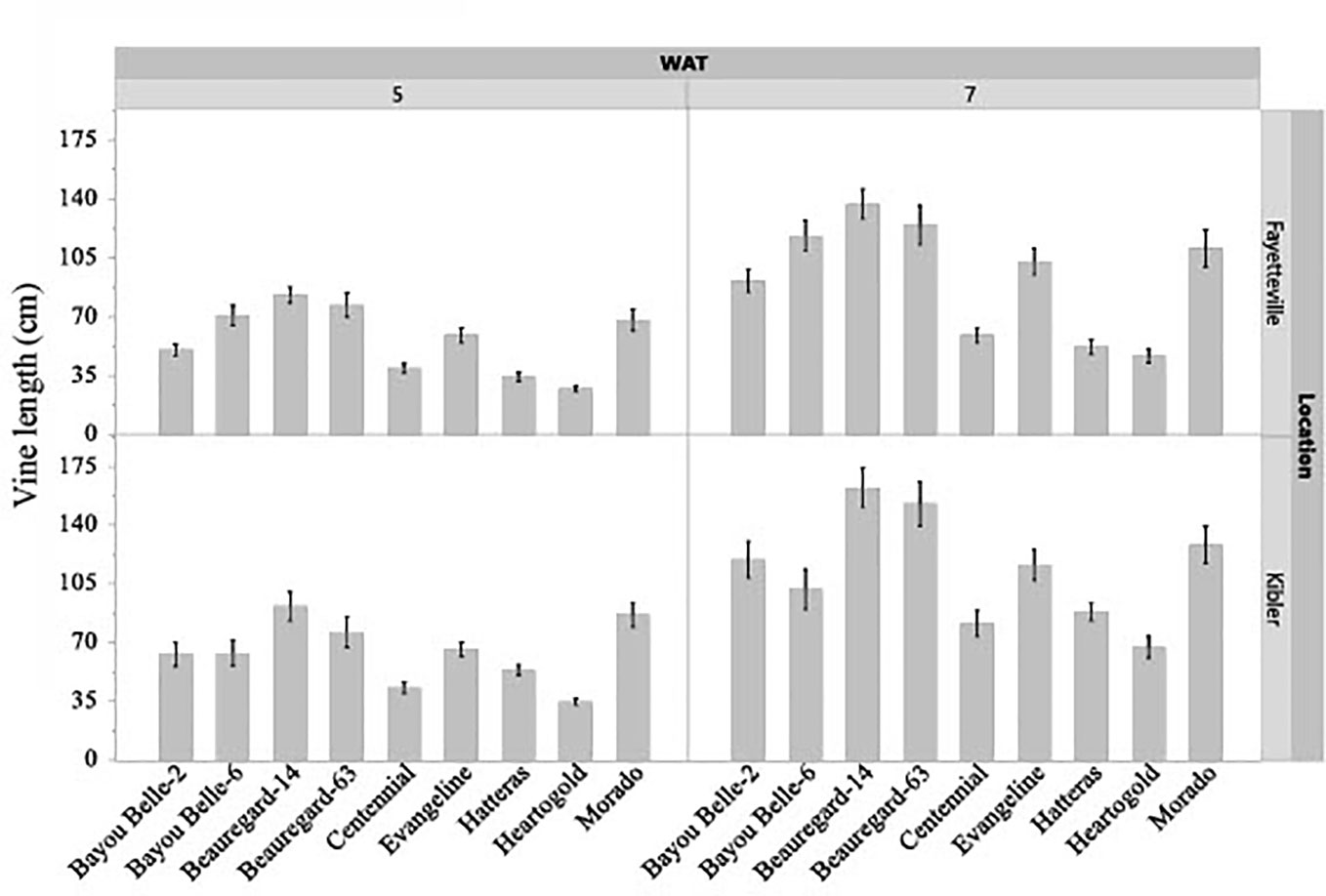
Figure 3 Sweetpotato vine length (cm) averaged across weed species at 5 and 7 weeks after transplanting (WAT) in Kibler and Fayetteville, AR, 2021. LSD to compare cultivars within location Fayetteville at 5 and 7 WAT: 7 cm; 10 cm. LSD to compare cultivars within location Kibler at 5 and 7 WAT: 9 cm; 12 cm. Bars represent standard error.
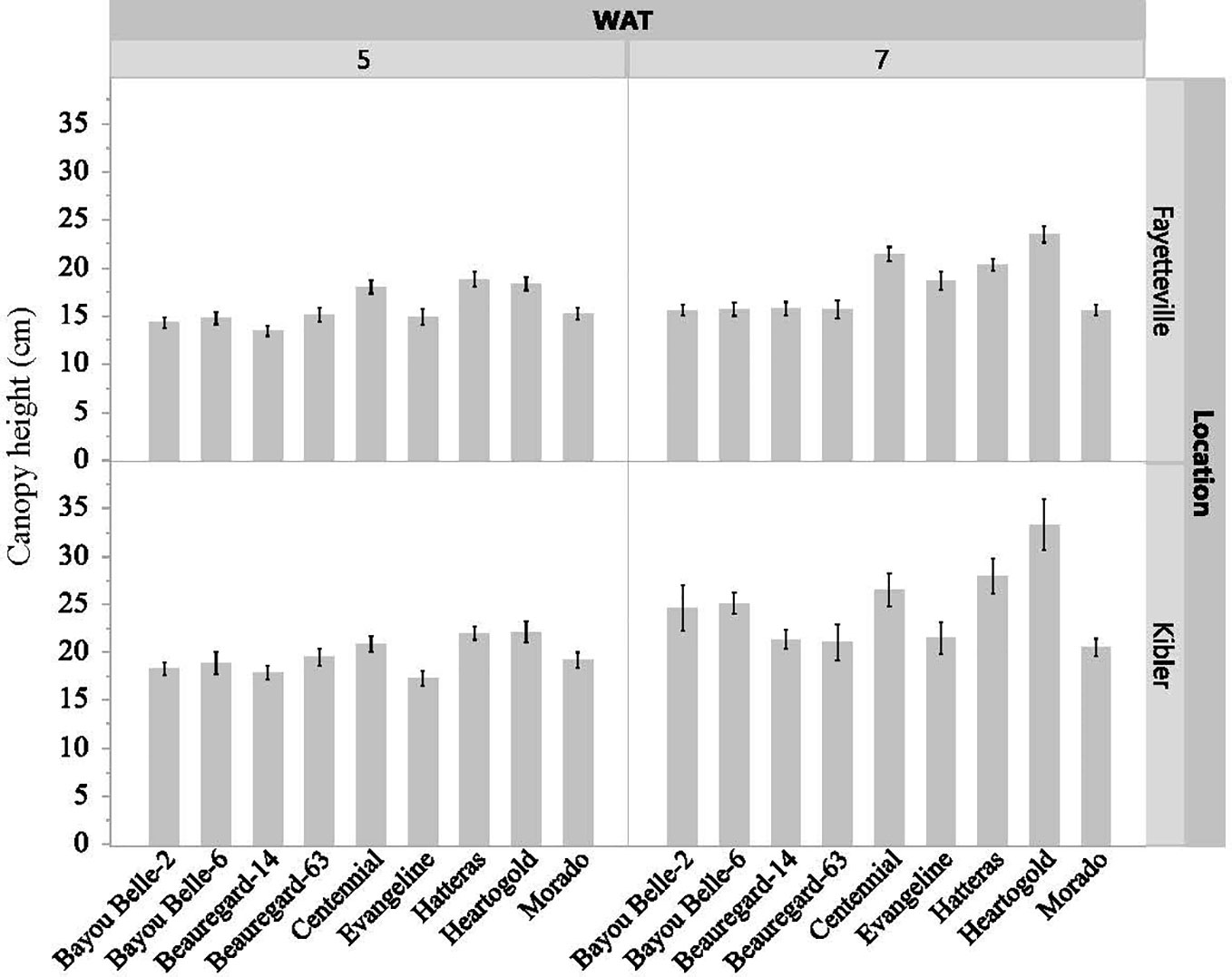
Figure 4 Sweetpotato canopy height (cm) averaged across weed species at 5 and 7 weeks after transplating (WAT) in Kibler and Fayetteville, AR, 2021. LSD to compare cultivars within location Fayetteville at 5 and 7 WAT: 1 cm; 1 cm. LSD to compare cultivars within location Kibler at 5 and 7 WAT: 1 cm; 2 cm. Bars represent standard error.
LAI was roughly 50% greater when cultivars were grown in weed-free conditions compared to plots with weeds in both locations. Averaged across cultivars, LAI in weed-free plots was approximately 2 and 1.7 in Fayetteville and Kibler, respectively (Figure 5). This was measured from a ground area of 0.13 m2. In Fayetteville, LAI across cultivars was reduced to 1.3 and 1 in plots with grasses and broadleaf weeds, respectively. In Kibler, LAI averaged 0.9 in broadleaf and grass plots. Cultivar ‘Heartogold’ had the greatest LAI (2.8) in Fayetteville (Figure 6). The greatest LAI in Kibler was also observed with ‘Heartogold’ (1.7), which was similar to that of ‘Centennial’ (1.4).
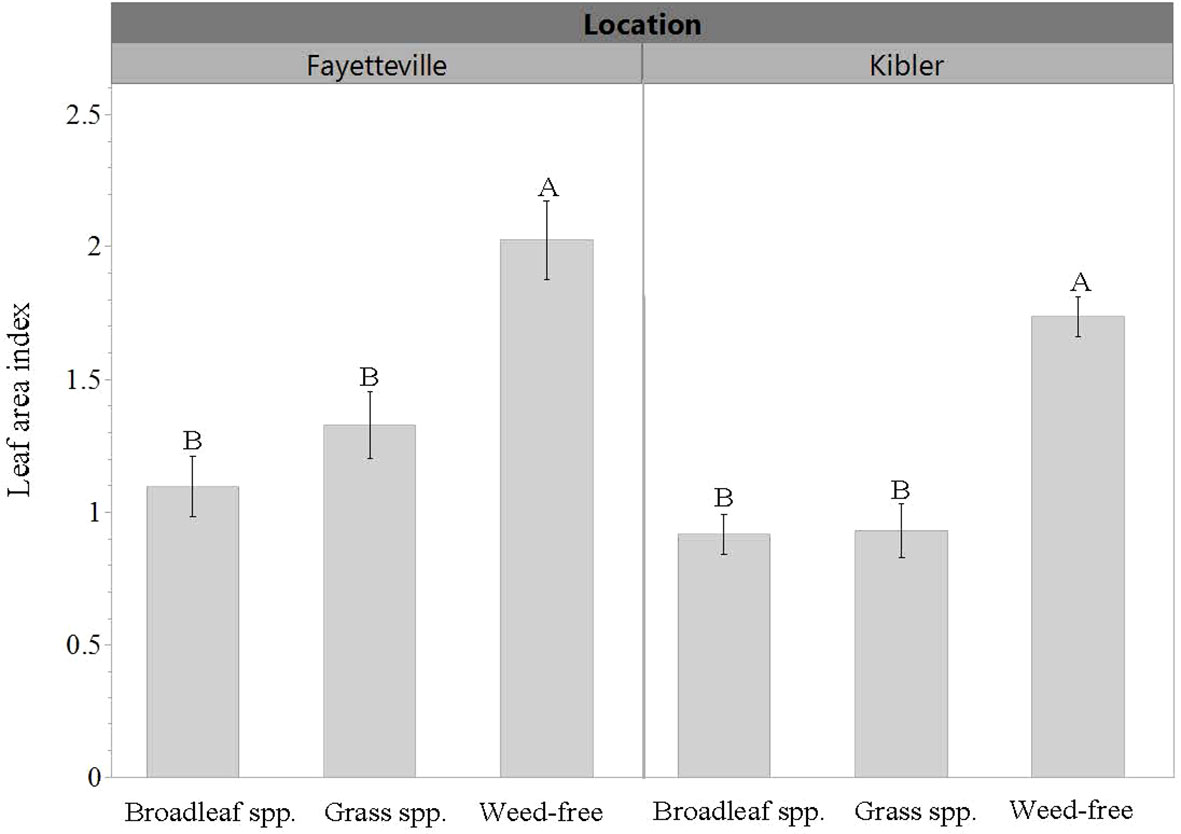
Figure 5 Leaf area index (LAI) averaged across cultivars when growing in weedy or weed-free conditions in Kibler and Fayetteville, AR, 2021. Means that do not share the same letter are significantly different from each other within a location (p ≤ 0.05). Bars represent standard error.
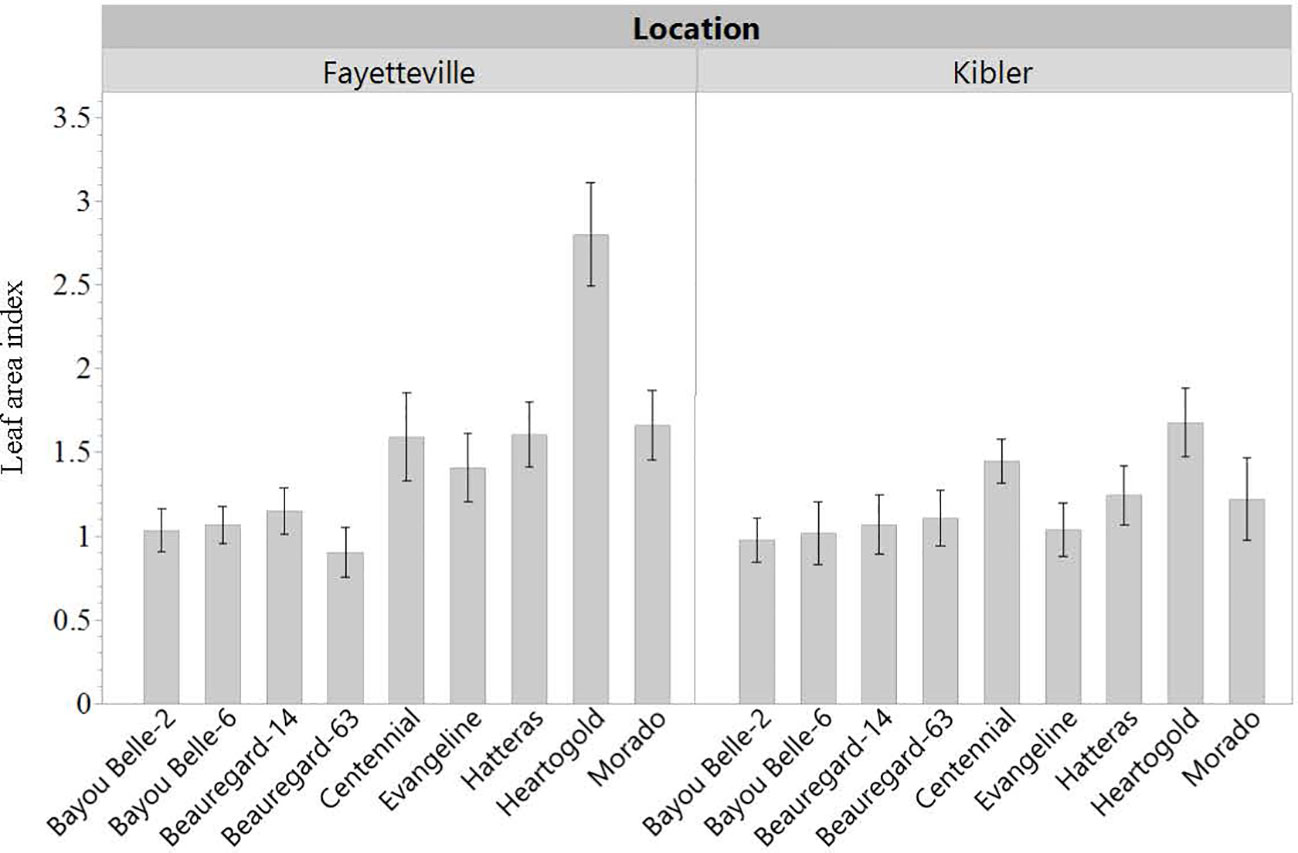
Figure 6 Leaf area index (LAI) of sweetpotato cultivars averaged across weedding treatments in Kibler and Fayetteville, AR, 2021. LSD to compare cultivars within location Fayetteville: 0.23; LSD to compare cultivars within location Kibler: 0.15. Bars represent standard error.
Sweetpotato yield by grade and yield loss
The interaction effect of weed species and cultivars on sweetpotato yield was not significant in Fayetteville and Kibler (p > 0.05); therefore, yield was averaged across cultivars within weedy treatments, and across weed species within cultivar treatments. Sweetpotato yield differed across cultivars and between weeding treatments in both locations.
Jumbo, no. 1, canner, and cull yields of the weed-free plots were 35,090; 29,500; 3,822; and 990 kg ha-1 in Fayetteville and 34,396; 34,908; 7,114; and 4,450 kg ha-1 in Kibler, respectively, averaged across cultivars (Table 7). The greatest yield reduction due to weed interference was observed in jumbo sweetpotato roots in both locations. In Fayetteville, jumbo and no.1 yield decreased to 5,019 and 10,217 kg ha-1 under broadleaf and to 7,050 and 19,041 kg ha-1 with grass infestation, respectively. In Kibler, jumbo and no. 1 yield decreased to 9,630 and 13,612 kg ha-1 and 12,041 and 16,123 kg ha-1 under broadleaf and grass infestation, respectively. In Fayetteville, canner yield was reduced to 5,844 kg ha-1 with grass infestation and 4,120 kg ha-1 under broadleaf weed infestation. Similarly, canner yield in Kibler was reduced to 5,169 and 3,450 kg ha-1 with grass and broadleaf infestation, respectively.
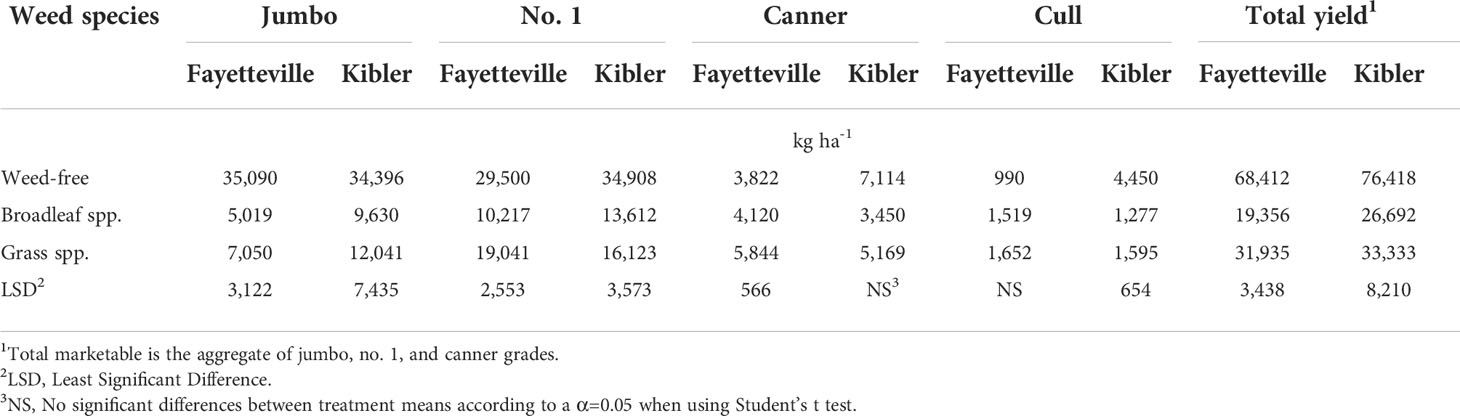
Table 7 Effect of weed infestation on yield averaged across nine sweetpotato cultivars, by grade (kg ha-1), in Fayetteville and Kibler, AR, 2021.
The greatest jumbo yield was obtained with ‘Morado’ (40,083 kg ha-1) in Fayetteville, and with Bayou Belle-6 (32,597 kg ha-1) in Kibler (Table 8). No.1 yield was greatest with ‘Bayou Belle-6’ (28,563 kg ha-1) in Fayetteville and Kibler (32,246 kg ha-1). ‘Heartogold’ had the greatest canner yield (7,406 kg ha-1) in Fayetteville, followed by ‘Centennial’ (6,961 kg ha-1), ‘Beauregard-14’ (6,541 kg ha-1), and ‘Hatteras’ (6,521 kg ha-1), whereas ‘Bayou Belle-6’ had the highest canner yield in Kibler (14,076 kg ha-1).
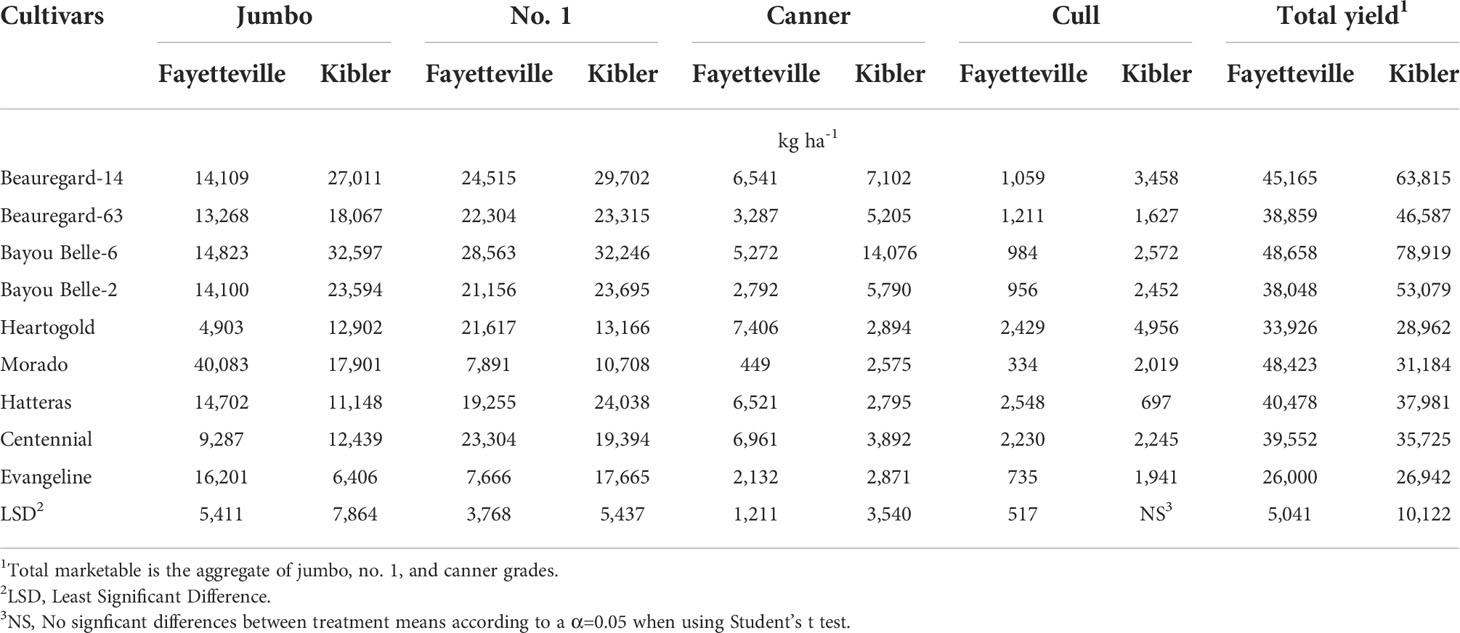
Table 8 Yield of sweetpotato cultivars by grade (kg ha-1) averaged across weedy and weed-free plots in Fayetteville and Kibler, AR, 2021.
Averaged across weedy and weed-free treatments, the highest yielding cultivars in Fayetteville were ‘Bayou Belle-6’ (48,658 kg ha-1), ‘Morado’ (48,423 kg ha-1), ‘Beauregard-14’ (45,165 kg ha-1), and ‘Hatteras’ (40,478 kg ha-1) (Table 8). ‘Bayou Belle-6’ (78,919 kg ha-1), ‘Beauregard-14’ (63,815 kg ha-1), and ‘Bayou Belle-2’ (53,079 kg ha-1) yielded the most in Kibler. ‘Evangeline’ showed the lowest yield at both locations. Overall, total sweetpotato yield was reduced by 65 and 56% in plots with broadleaf and grass species, respectively, compared to weed-free plots (76,418 kg ha-1) in Kibler (Table 7). A similar response was observed in Fayetteville, and yield was reduced by 72 and 53% broadleaf and grass species, respectively, compared to weed-free plots (68,412 kg ha-1).
Discussion
The use of weed-suppressive cultivars is gaining attention in systems where herbicide use is restricted, herbicide options are few, or in organic farms where using conventional herbicides is not allowed. Commercially desirable cultivars are those that possess enhanced weed suppressive ability coupled with superior agronomic traits such as high yield potential (Gealy and Yan, 2012). For sweetpotatoes, commercial success eventually hinges on consumer preference for eating quality. In the field, plant-plant interactions are trigged by complex chemical (allelopathy) and physical (competition) mechanisms. Competition is the consequence of plants using a limited supply of the same resources, whereas allelopathy is the inhibitory effect of chemicals released by one plant to neighboring plants (Molisch, 1937). Allelopathy and competition occur simultaneously in the field and the observable weed suppression is the total effect of these two components (Olofsdotter et al., 2002). The present study provides insight on the potential of using weed-suppressive sweetpotato cultivars for improved weed control.
Allelopathic potential is cultivar- and weed-specific. Many studies demonstrated this such as cultivar differences in allelopathy in rice (Olofsdotter et al., 1999), cucumber (Cucumis sativus L.) (Putnam and Duke, 1974), sweetpotato (Xuan et al., 2016), and wheat (Grodzinsky and Panchuck, 1974). Weed inhibition by allelochemicals decline with plant size and age. In fact, allelopathic effect is most apparent in terms of reduction of weed germination and seedling growth (Xuan et al., 2012; Xuan et al., 2016; Shen et al., 2018). In terms of seedling growth inhibition, allelopathic sweetpotato cultivars were effective only on junglerice and had minimum effect on hemp sesbania and Palmer amaranth seedlings. Nevertheless, junglerice is a major grass weed in the majority of crops, including sweetpotato. Reducing grass growth significantly improves the efficacy of herbicides in conventional production and improves weed control in organic production. In other studies, it has been documented that allelopathic effect could be more consistent within species of the same family. For instance, the correlation between allelopathic potential of rice against barnyardgrass [Echinochloa crus-galli (L.) P. Beauv.] and giant arrowhead [Sagittaria montevidensis var. spongiosa (Engelm.) B. Boivin] was 0.58, while that of grassy arrowhead (Sagittaria graminea Michx.) and water plantain (Alisma plantagoaquatica L.) was 0.93 (Seal and Pratley, 2010). Therefore, we can expect that sweetpotato cultivars which are highly allelopathic to junglerice would also be highly inhibitory to other grass weed species of similar seed size such as barnyardgrass and large crabgrass. Sweetpotato cultivars exude different quantities and quality of allelopathic compounds (Soni et al., 2019). Allelochemicals from sweetpotato include coumarin, caffeic acid, and trans-cinnamic acid (Chon and Boo, 2005). All ten cultivars analyzed by Chon and Boo (2005) produced chlorogenic and caffeic acid; a few cultivars produced hydroxycinnamic acid, trans-cinnamic acid, and coumarin. In the same study, ‘Heartogold’ and ‘529’ were classified as highly allelopathic and had higher amounts of total allelochemicals, particularly chlorogenic acid, and trans-cinnamic acid relative to other cultivars. ‘Centennial’ and ‘Stokes Purple’, on the other hand, showed intermediate allelopathic potential. These cultivars produced a high concentration of coumarin and caffeic acid (Soni et al., 2019). In our study, ‘Heartogold’, ‘Centennial’, and ‘Stokes Purple’ inhibited all the three weed species to some extent, with the highest inhibition observed on junglerice.
The weed suppressive ability of ‘Heartogold’ was also observed in the field and was consistent across locations. The inhibitory potential of this cultivar was also reported in previous studies showing 80% growth inhibition of Palmer amaranth seedlings (Soni et al., 2019). ‘Centennial’, another potentially allelopathic cultivar in our study, was among the most effective cultivars in reducing weed biomass in the field. Although these data suggest that the allelopathic potential of ‘Heartogold’ and ‘Centennial’ is advantageous against weeds, allelopathy alone cannot account for the total weed suppression observed in the field. In our field trials, sweetpotato cultivars differ widely in morphological characteristics and are therefore expected to vary in their competitive ability with weeds. For instance, ‘Heartogold’, which reduced weed biomass the most in both locations, had the greatest leaf area and canopy height among the cultivars, but had shorter vines than most cultivars. Conversely, ‘Beauregard-14’ and ‘Beauregard-63’ had the longest vines, but this characteristic had little effect on weed biomass reduction. This means that having longer vines is not as important as having large leaves and tall canopy in being able to suppress weed growth. In other crops, especially winter cereals, taller cultivars are better tolerators of weed pressure and better suppressors of weed biomass (Challaiah et al., 1986; Vandeleur and Gill, 2004). In some studies, allelopathy explained about 20% the total weed suppression ability observed in wheat (Bertholdsson, 2010), 34% in rice (Olofsdotter et al., 1999), and 58% in barley (Bertholdsson, 2010). This means that the larger component of interference is generally crop competitive ability. In our study it was noticeable that better weed suppression was achieved with cultivars that showed high allelopathic ability in the greenhouse and favorable morphological characteristics such as high leaf area index and tall canopy.
The performance of sweetpotato cultivars was similarly affected by the type of weed species present in the field. The sweetpotato leaf area, vine length, and canopy height were reduced similarly by broadleaf and grass weeds. In general, the degree of interference varies according to the species composing a weed community (Clarke, 1971). This study did not control for variation in the natural weed population nor considered the individual weed species present. Instead, a mixture of broadleaf or grass weed population was used to represent what growers would typically find in their fields. Dominant weeds within the Poaceae family in Kibler and Fayetteville were large crabgrass, junglerice, and broadleaf signalgrass. These species represent some of the common grasses that infest sweetpotatoes (Monks et al., 2019). Broadleaf weeds included Palmer amaranth, annual morningglories, and carpetweed, known to be troublesome in sweetpotato fields (Monks et al., 2019). According to Basinger et al., 2019, an individual plant of either Palmer amaranth or large crabgrass per meter of row can reduce sweetpotato yield by 50% and 35%, respectively, and the maximum yield loss due to weed density is 87% for Palmer amaranth and 83% for large crabgrass (Meyers et al., 2010). This occurs in part because of plant architecture and the ability to intercept light. In general, sweetpotato canopy reaches less than 50 cm tall. In our experiments the canopy of most sweetpotato cultivars was less than 40 cm tall. Conversely, roughly 80% of the leaves of Palmer amaranth plants are positioned about 1 m above the ground (Meyers et al., 2010; Monks et al., 2019). The fact that sweetpotato canopy is shorter than most weeds means that it is shaded by the majority of weed species, resulting in less photosynthetic activity and reduced yield. Although grasses that emerge later are vulnerable to shading by the sweetpotato canopy, in our study, broadleaf signalgrass and junglerice exceeded the height of sweetpotato canopy throughout the growing season. This indicates ample time for weeds to emerge and grow before the crop canopy approaches 100% ground copy. For several cultivars, full canopy closure was not attained at all.
Loss of jumbo and no. 1 yields was the most significant contributor to overall marketable yield reduction in weedy conditions, especially with broadleaf weeds. On average, weed interference reduced up to 85% of jumbo yield and up to 65% of no. 1 yield. Other studies predicted yield loss of jumbo and no.1 roots to be 30 to 94%, respectively for Palmer amaranth densities of 0.5 to 6.5 plants m-1 (Meyers et al., 2010). Canner grade roots, which are generally more variable and less valuable than other grades, were the least affected by weed interference in this study. Overall, allelopathic cultivars identified in our greenhouse studies, including ‘Centennial’ and ‘Heartogold’, were significantly lower yielding in the field. It is possible that high production of allelopathic compounds had diverted substantial carbon resources from storage roots. After all, allelopathy is a protection mechanism, and some protection mechanisms have trade-offs manifested in various ways such as reduced yield (McCall and Fordyce, 2010). Additionally, the autotoxicity of plants producing allelochemicals should not be ignored. The inhibitory effect of root exudates on the plant itself has been documented in cucumber, where photosynthesis process, transpiration, and stomatal functions were affected by its own root exudates (Yu et al., 2003). Other species including wheat and annual sowthistle (Sonchus oleraceus L.) produce allelochemicals that can be both phytotoxic to other species and autotoxic (Wu et al., 2007; Gomaa et al., 2014). Some derivates of benzoic and cinnamic acids, which were identified in root exudates of ‘Heartogold’, have been identified as autotoxins (Yu and Matsui, 1994).
The ability of a crop to suppress weeds and maintain yield potential under weed pressure can also be derived from different mechanisms of crop competitiveness (Lemerle et al., 2006), and may or may not be correlated (Jordan, 1993). For example, the root exudates of ‘Beauregard-14’ and ‘Bayou Belle-6’ did not affect weed growth in the greenhouse experiment and these cultivars caused little reduction in weed biomass in the field. The inferior weed suppression by these two cultivars in the field could be further attributed to the smaller leaf area and shorter canopy than that of most cultivars. Interestingly, these two cultivars were the highest yielding, with or without weed competition. These two cultivars appeared to be tolerant to weed competition, able to maintain its yield potential under weed pressure. Such trait is highly desirable.
Conclusions
Some sweetpotato cultivars including ‘Heartogold’, ‘Centennial’, and ‘Stokes purple’ are allelopathic. Junglerice seedlings are generally more affected by root leachates of these cultivars than the broadleaf species tested. Weed species differ in susceptibility to sweetpotato allelopathy, as is commonly known about allelopathic interactions. The allelopathic effects decrease with increasing plant size (or age). Cultivars with high allelopathic activity and competitive morphological characteristics cause higher and longer-lasting weed suppression. ‘Heartogold’ is strongly weed suppressive in the field regardless of weed species. This cultivar possesses superior plant architecture for weed suppression. Tall canopy and large leaf area contribute to weed suppression by this cultivar. Being viney is not important for weed suppression. ‘Beauregard-14’ and ‘Bayou Belle-6’ have superior yield performance in the absence of weeds and able to maintain their yield potential under weed pressure, despite its poor weed suppressive ability, suggesting a superior tolerance to weed competition. Effort to identify traits that can be used to improve cultivar competitiveness, yield potential, and desirable end-use characteristics must continue.
Data availability statement
All data generated or analyzed during this study are included in this published article.
Author contributions
Conceptualization, NR-B, T-MT; methodology, NR-B, MN, and IW; software, IW; validation, NR-B; formal analysis, IW; investigation, MN, SK, PC-M, KK, and IW; resources, NR-B; data curation, MN, SK, PM, KK, and IW; writing-original draft preparation, IW; writing and review, NR-B, and IW, visualization, NR-B, and IW; supervision, NR-B; project administration, NR-B, T-MT; funding acquisition, NR-B. All authors contributed to the article and approved the submitted version.
Funding
This work was supported by the Agriculture and Food Research Initiative grant no. 2019-51300-30247.
Acknowledgments
The authors would like to thank the Agriculture and Food Research Initiative for the support in funding the research. We would also like to express our gratitude for the assistance provided by Steve E. Eaton, Alden N. Hotz, Lesley M. Smith, and Gustavo B. de Lima throughout the duration of this study.
Conflict of interest
The authors declare that the research was conducted in the absence of any commercial or financial relationships that could be construed as a potential conflict of interest.
Publisher’s note
All claims expressed in this article are solely those of the authors and do not necessarily represent those of their affiliated organizations, or those of the publisher, the editors and the reviewers. Any product that may be evaluated in this article, or claim that may be made by its manufacturer, is not guaranteed or endorsed by the publisher.
References
Balyan R. S., Malik R. K., Panwar R. S., Singh S. (1991). Competitive ability of winter wheat cultivars with wild oat (Avena ludoviciana). Weed Sci. 39, 154–158. doi: 10.1017/S0043174500071393
Basinger N. T., Jennings K. M., Monks D. W., Jordan D. L., Everman W. J., Hestir E. L., et al. (2019). Interspecific and intraspecific interference of palmer amaranth (Amaranthus palmeri) and large crabgrass (Digitaria sanguinalis) in sweetpotato. Weed Sci. 67, 426–432. doi: 10.1017/wsc.2019.16
Bertholdsson N. O. (2010). Breeding for spring wheat for improved allelopathic potential. Weed Res. 50, 49–57. doi: 10.1111/j.1365-3180.2009.00754.x
Challaiah O., Burnside O. C., Wicks G. A., Johnson V. A. (1986). Competition between winter wheat (Triticum aestivum) cultivars and downy brome (Bromus tectorum). Weed Sci. 34, 689–693. doi: 10.1017/S0043174500067692
Chandler J. M., Meredith W. R. Jr (1983). Yields of three cotton (cultivars as influenced by spurred anoda competition. Weed Sci. 31 (303), 307. doi: 10.1017/S0043174500069058
Chon S. U., Boo H. O. (2005). Difference in allelopathic potential as influenced by root periderm colour of sweet potato (Ipomoea batatas). J. Agron. Crop Sci. 191, 75–80. doi: 10.1111/j.1439-037X.2004.00138.x
Christensen S. (1995). Weed suppression ability of spring barley varieties. Weed Res. 35, 241–247. doi: 10.1111/j.1365-3180.1995.tb01786.x
Cudney D. W., Jordan L. S., Hall A. E. (1991). Effect of wild oat (Avena fatua) infestations on light interception and growth rate of wheat (Triticum aestivum). Weed Sci. 39, 175–179. doi: 10.1017/S0043174500071435
Dadkhah A. (2015). Allelopathic potential of canola and wheat to control weeds in soybean (Glycine max). Russ. Agric. Sci. 41, 111–114. doi: 10.3103/S1068367415020081
Gealy D. R., Yan W. (2012). Weed suppression potential of ‘Rondo’ and other indica rice germplasm lines. Weed Technol. 26, 524–527. doi: 10.1614/WT-D-11-00141.1
Gomaa N. H., Hassan M. O., Fahmy G. M., González L., Hammouda O., Atteya A. M. (2014). Allelopathic effects of Sonchus oleraceus l. on the germination and seedling growth of crop and weed species. Acta Bot. Bras. 28, 408–416. doi: 10.1590/0102-33062014abb3433
Grodzinsky M. M., Panchuck M. A. (1974). “Allelopathic properties of crop residues of wheat-wheatgrass hybrids” in Physiological-biochemical basis of plant interactions in phytocenosis. Ed. Grodzinsky A. M. (Kiev (USSR):Naukova Dumka), 51–55.
Hansen P. K., Kristensen K., Willas J. (2008). A weed suppressive index for spring barley (Hordeum vulgare) varieties. Weed Res. 48, 225–236. doi: 10.1111/j.1365-3180.2008.00620.x
Harker N. K., O’Donovan T. J. (2013). Recent weed control, weed management, and integrated weed management. Weed Technol. 27, 1–11. doi: 10.1614/WT-D-12-00109.1
Hoad S. P., Davies D. H. K., Topp C. F. E. (2006). Designing crops for low input and organic systems: Enhancing wheat competitiveability against weeds. In proceedings of Crop Protection inNorthern Britain (Dundee, United Kingdom), 157–168.
Jabran K., Mahajan G., Sardana V., Chauhan B. S. (2015). Allelopathy for weed control in agricultural systems. Crop Prot. 72, 57–65. doi: 10.1016/j.cropro.2015.03.004
Jordan N. (1993). Prospects for weed control through crop interference. Ecol. Appl. 3, 84–91. doi: 10.2307/1941794
Kemble J. M., Meadows I., Jennings K., Rodrigues C., Walgenbach J., Wszelaki A. L., et al. (2022). 2022 vegetable crop handbook for southeastern united states (Sparta, Michigan:Auburn University), 365. Available at: https://content.ces.ncsu.edu/southeastern-us-vegetable-crop-handbook.
Konesky D. W., Siddiqi M. Y., Glass A. D. M., Hsiao A. L. (1989). Wild oat and barley interactions: varietal differences in competitiveness in relation to phosphorus supply. Can. J. Bot. 67, 3366–3371. doi: 10.1139/b89-408
Lemerle D., Blackshaw R. E., Smith A. B., Potter T. D., Marcroft S. T. (2006). Comparative survey of weeds surviving in triazine-tolerant and conventional canola crops in south-eastern Australia. Plant Prot. Q. 16, 37–40.
Lemerle D., Verbeek B., Cousens R. D., Coombes N. E. (1996). The potential for selecting wheat varieties strongly competitive against weeds. Weed Res. 36, 505–513. doi: 10.1111/j.1365-3180.1996.tb01679.x
Levett M. P. (1992). Effects of various hand-weeding programmes on yield and components of yield of sweetpotato (Ipomoea batatas) grown in the tropical lowlands of Papua new Guinea. J. Agric. Sci. 118, 63–70. doi: 10.1017/S0021859600068015
Li J. Y., Guo X. K., Zhang Q., Liu C. H., Lin Z. H., Yu Z. M., et al. (2015). A novel screening method for rice allelopathic potential: The inhibitory-circle method. Weed Res. 55, 441–448. doi: 10.1111/wre.12166
Ma Y., Lang M., Dong S., Shui J., Zhao J. (2012). Screening of some cotton varieties for allelopathic potential on clover broomrape germination. Agron. J. 104, 569–574. doi: 10.2134/agronj2011.0372
Mason H., Navabi A., Frick B., O’Donovan J., Snaper D. (2007). Cultivar and seeding rate effects on competitive ability if spring cereals are grown under organic management in northern Canada. Agron. J. 90, 1199–1207. doi: 10.2134/agronj2006.0262
McCall A. C., Fordyce J. A. (2010). Can optimal defence theory be used to predict the distribution of plant chemical defences? J. Ecol. 98, 985–992. doi: 10.1111/j.1365-2745.2010.01693.x
Meyers S. L., Jennings K. M., Schultheis J. R., Monks D. W. (2010). Interference of palmer amaranth (Amaranthus palmeri) in sweet potato. Weed Sci. 58, 119–203. doi: 10.1614/WS-D-09-00048.1
Molisch H. (1937). Der einfluss einer pflanze auf der ander – allelopathie. Jena Verlag von Gustav Fischer 106. Available at: https://scholar.google.com/scholar_lookup?title=Der+Einfluss+einer+Pflanze+auf+die+andere-Allelopathie&author=Molisch,+H.&publication_year=1937.
Monks D. W., Jennings K. M., Meyers S. L., Smith T. P., Korres N. E. (2019). “Sweet potato: Important weeds and sustainable weed management” in Weed control: Sustainability, hazards, and risks in cropping systems worldwide. Eds. Korres N. E., Roma-Burgos N., Duke S. O. (Boca Raton, FL:CRC Press), 581–596 p.
Newcomer D. T., Giraudo L. J., Banks P. A. (1986). Soybean cultivar as a factor of weed control in no-till double-cropped production following wheat (Athens, GA: Georgia Agricultural Experiment Station Research Report), Pp. 16.
Olofsdotter M., Jensen L. B., Courtois B. (2002). Improving crop competitive ability using allelopathy–an example from rice. Plant Breed. 121, 1–9. doi: 10.1046/j.1439-0523.2002.00662.x
Olofsdotter M., Navarez D., Rebulanan M., Streibig J. C. (1999). Weed-suppressing rice cultivars ± does allelopathy play a role? Weed Res. 39, 441–454.doi: 10.1046/j.1365-3180.1999.00159.x
Putnam A. R., Duke W. B. (1974). Biological suppression of weeds: evidence for allelopathy in accession of cucumber. Science 186, 370–372. doi: 10.1126/science.185.4148.370
Sankula S., VanGessel M. M., Mulford R. R. (2004). Corn leaf architecture as a tool for weed management in two production systems. Weed Sci. 52, 1026–1033. doi: 10.1614/P2001-125
Scavo A., Mauromicale G. (2021). Crop allelopathy for sustainable weed management in agroecosystems: anowing the present with a view to the future. Agronomy 11, 2104. doi: 10.3390/agronomy11112104
Seal A. N., Pratley J. E. (2010). The specificity of allelopathy in rice (Oryza sativa). Weed Res. 50, 303–311. doi: 10.1111/j.1365-3180.2010.00783.x
Shen S., Xu G., Li D., Clements D. R., Jin G., Liu S., et al. (2018). Allelopathic effects of three sweet potato cultivars (Ipomoea batatas) on the invasive plant Mikania micrantha. Paki. J. @ Biol.Sci 21, 8–15. doi: 10.3923/pjbs.2018.8.15
Soni B., Tseng T. M. P., Yue Z. M. (2019). Identification and quantification of allelochemicals from selected sweet potato (Ipomoea batatas (L.) lam.) cultivars. Am. J. Plant Sci. 10, 2354–2365. doi: 10.4236/ajps.2019.1012163
Trezzi M. M., Balbinot A. A., Benin G., Bastiani F., Patel F., Miotto E. (2013). Competitive ability of soybean cultivars with horseweed (Conyza bonariensis). Planta daninha 32, 543–550. doi: 10.1590/S0100-83582013000300006
USDA United States Department of Agriculture (2005) United states standards for grades of sweetpotatoes. Available at: https://www.ams.usda.gov/sites/default/files/media/Sweetpotato_Standard%5B1%5D.pdf.
Vandeleur R. K., Gill G. S. (2004). The impact of plant breeding on the grain yield and competitive ability of wheat in Australia. Aust. J. Agric. Res. 55, 855–861. doi: 10.1071/AR03136
Werle I. S., Zanon A. J., Streck N. A., Schaedler C. E., Barbieri G. F., Ulguim A. (2021). Technology levels in cassava cultivation alter phytosociology of weeds. HortScience 56, 787–794. doi: 10.21273/HORTSCI15643-20
Wu H., Pratley J. E., Lemerle D., An M., Liu D. L. (2007). Autotoxicity of wheat (Triticum aestivum l.) as determined by laboratory bioassays. Plant Soil 296, 85–93. doi: 10.1007/s11104-007-9292-7
Xuan T. D., Minh T. N., Trung K. H., Khanh T. D. (2016). Allelopathic potential of sweet potato varieties to control weeds: Imperata cylindrica, Bidens pilosa, and Ageratum conyzoides. Allelopathy J. 38, 41–54.
Xuan T. D., Toyama T., Khanh T. D., Tawata S., Nakagoshi N. (2012). Allelopathic interference of sweet potato with cogongrass and relevant species. Plant Ecol. 213, 1955–1961. doi: 10.1007/s11258-012-0136-z
Yu J. Q., Matsui Y. (1994). Phytotoxic substances in the root exudates of Cucumis sativus l. J. Chem. Ecol. 20, 21–31. doi: 10.1007/BF02065988
Keywords: allelopathy, crop competitiveness, cultivar selection, weed interference, weed suppression, integrated weed management (IWM)
Citation: Werle IS, Noguera MM, Karaikal SK, Carvalho-Moore P, Kouame KB-J, Tseng T-M and Roma-Burgos N (2022) Allelopathic potential and competitive traits of sweetpotato cultivars. Front. Agron. 4:990879. doi: 10.3389/fagro.2022.990879
Received: 10 July 2022; Accepted: 20 September 2022;
Published: 11 October 2022.
Edited by:
Khawar Jabran, Niğde ÖmerHalisdemir University, TurkeyReviewed by:
Naila Farooq, University of Sargodha, PakistanJamal Ragheb Qasem, The University of Jordan, Jordan
Copyright © 2022 Werle, Noguera, Karaikal, Carvalho-Moore, Kouame, Tseng and Roma-Burgos. This is an open-access article distributed under the terms of the Creative Commons Attribution License (CC BY). The use, distribution or reproduction in other forums is permitted, provided the original author(s) and the copyright owner(s) are credited and that the original publication in this journal is cited, in accordance with accepted academic practice. No use, distribution or reproduction is permitted which does not comply with these terms.
*Correspondence: Nilda Roma-Burgos, bmJ1cmdvc0B1YXJrLmVkdQ==