- 1Department of Botany, Faculty of Science, Indira Gandhi National Tribal University, Amarkantak, India
- 2Department of Biochemistry, St. Ann’s College for Women, Hyderabad, India
- 3Department of Plant Sciences, School of Life Sciences, University of Hyderabad, Gachibowli, Hyderabad, India
Phosphorus (P) is an essential macronutrient for plants and is considered as one of the limiting nutrients to plants. The majority of P in the soil is found in the form of insoluble phosphate complexes, making the P unavailable for plants. In the present study, we report two highly efficient phosphate solubilizing bacteria isolated from the closed flower of Hedychium coronarium L., a medicinal plant commonly known as Gulbakawali. Based on morphological, biochemical, and molecular characterization, the two bacterial isolates were identified as genus Pantoea and designated as HCF6 and HCF9. Both these isolates efficiently solubilize tricalcium phosphate [Ca3(PO4)2] present in National Botanical Research Institute’s Phosphate (NBRIP) medium by releasing soluble phosphate up to 2000 μg ml-1 and also partially solubilize aluminium phosphate (AlPO4). HCF6 and HCF9 possess several plant growth promoting traits and were evaluated for plant growth promotion ability using sand as a growth medium. Wheat plants treated with the bacterial isolates had increased root and shoot length and plant dry mass and accumulated higher inorganic phosphate in the plant tissue suggesting their role in mobilizing soluble P from insoluble P-complexes. Overall, our findings suggest that both these Pantoea isolates (HCF6 and HCF9) represent promising candidates as bioinoculants.
Introduction
To meet the global food demand, farmers extensively use agrochemicals, which lead to the loss of microbial diversity in soil along with the loss of microorganisms associated with crop plants, i.e. crop microbiome. The harmful effects of agrochemicals will be worsened in the future as the global food demand is expected to increase 70% by 2050 (Cole et al., 2018). In this context, microbial inoculants hold great promise as biofertilizers. Beneficial bacteria in the rhizosphere and residing inside the plant tissues as endophytes provide several benefits to plants (Mousa et al., 2015; Papik et al., 2020; Basu et al., 2021). They can enhance plant growth by nutrient acquisition and have the potential to provide fitness advantages against biotic and abiotic stresses (Mousa et al., 2015; Singh et al., 2020). Although several plant growth promoting bacteria (PGPB) have been isolated and used as growth promoters, the development of culture techniques has led to a continued search for microorganisms from unexplored sources with novel traits (Azizoglu, 2019).
Among several beneficial traits, P acquisition in plants with the help of phosphate solubilizing bacteria (PSB) and mycorrhizae is crucial for addressing global food security (Etesami and Jeong, 2021; Tian et al., 2021). Orthophosphate or inorganic phosphate (Pi) is the major P source in soil, which is taken up by the plant for its growth and development. However, ~70% of total P in the soil exists as insoluble chemical complexes with calcium, aluminium, and iron (Sashidhar and Podile, 2010; Otieno et al., 2015). In many soils, P deficiency is a potential growth-limiting factor in agricultural systems (Daniels et al., 2009).
According to estimates, inoculating crops with phosphate solubilizing microorganisms (PSMs) can reduce up to 50% phosphate fertilizer application while maintaining crop yield (Yazdani et al., 2009). Several studies have indicated the importance of PSB in the rhizosphere and within the plants to liberate organic phosphate or solubilize insoluble Pi compounds, allowing the plant to use it. Sharon et al. (2016) isolated a Pantoea sp. Pot1 strain from potato rhizosphere that could solubilize tricalcium phosphate (Ca3(PO4)2) and boost Solanum lycopersicum L. growth in greenhouse conditions. Similarly, phosphate solubilizing bacterial endophytes such as Enterobacter sp. (J49) and Serratia sp. (S119) isolated from peanut have shown plant growth promotion in Glycine max (L.) Merr. and Zea mays L., (Lucero et al., 2021). These findings suggest that PSB could be applied to various plant species to improve the phosphate use efficiency.
The PSB convert insoluble forms of the P into soluble forms using various types of mechanisms (Kalayu, 2019). The production of organic acids is the primary mechanism for mineral phosphate solubilization. Microorganisms release organic acids that act as good chelators of the divalent cations of Ca2+ and release soluble phosphates from insoluble P compounds. Enzymes such as phytases, lyases, and phosphatases are also produced by PSB that can solubilize organic P (Illmer et al., 1995). According to many reports, the acids are produced by the direct oxidation pathway of glucose in the periplasm of Gram-negative bacteria such as Erwinia herbicola and Pseudomonas cepacia (Goldstein et al., 1993; Anthony, 2004). Gram-negative bacteria are more efficient at dissolving mineral phosphates than Gram-positive bacteria because the metabolism of sugars, mainly glucose, releases a large number of organic acids into the extracellular medium (Sashidhar and Podile, 2010).
Here we report the isolation, molecular characterization, and evaluation of PGP traits of endophytic bacteria isolated from the closed flower of Hedychium coronarium L. The study represents the identification of two highly efficient phosphate solubilizing bacteria which belong to the genus Pantoea. Using a low P containing sand medium supplemented with Ca3(PO4)2, these bacterial isolates were evaluated for P mobilization to the wheat plant. Using a pot experiment, we demonstrated that both the bacterial endophytes enhanced the Pi availability and growth promotion in wheat.
Materials and methods
Collection and surface sterilization of samples
Closed flowers from healthy H. coronarium plants were collected from the Amarkantak area of Madhya Pradesh, India. Samples were washed under running tap water to remove the dust particles. Surface sterilization was done by immersing the flower in 70% ethanol for 1 min, followed by 2% sodium hypochlorite (NaOCl) containing 0.1% Tween 20 for 30 s. Finally, the flowers were rinsed four times with sterile double distilled water for 2 min to remove any trace amounts of NaOCl and then dried under aseptic conditions in a laminar airflow (Tanco, PLT-270, India). The last rinsed water was used as a control to confirm the proper sterilization of flower samples.
Isolation of bacterial endophytes
One gram of surface-sterilized flowers was cut into small pieces and macerated with a sterile mortar and pestle in 5 ml of sterile double distilled water. One ml of flower extract was then serially diluted to 10-1 using sterile distilled water. A 0.1 to 0.2 ml of an aliquot from both undiluted and 10-1 dilution were taken and spread on Petri plates containing four different types of media: tryptic soy agar (TSA) medium (15 g tryptone, 5 g soya peptone, 5 g NaCl, 1.5% agar per 1000 ml distilled water, pH 7.3), Luria Bertani agar (LBA) medium (10 g tryptone, 5 g yeast extract, 10 g NaCl, 1.5% agar per 1000 ml distilled water, pH 7.0), nutrient agar (NA) medium (5 g peptone, 5 g NaCl, 1.5 g HM peptone B, 1.5 g yeast extract, 1.5% agar per 1000 ml distilled water, pH 7.0), and plant based media (25 g nutrient broth powder, 100 ml plant extract, 1.5% agar per 1000 ml distilled water, pH 7.0). Agar was excluded from the above mentioned media for the preparation of broth. The plates were incubated (BOD Incubator, UTS-1.12, India) at 30°C for 1–7 days for maximum recovery of bacterial colonies. Pure colonies were obtained by sub-culturing, and the isolates were preserved in 20% glycerol at -80°C.
Characterization of endophytic bacterial isolates
Identification of bacterial isolates was done according to the morphological characterization, and biochemical tests were performed using biochemical identification kit (Himedia). For molecular identification, overnight grown bacterial cultures were taken for genomic DNA isolation using the method described by Sharma and Singh (2005). The extracted genomic DNA was used as a template for PCR amplification of the 16S rRNA gene using universal primers: forward primer 27F (5’GTTTGATCCTGGCTCAG3’) and reverse primer 1492R (5’CGGTTACCTTGTTACGACTT3’). The PCR reaction mixture contained 1 μl of each primer (10 μM), 25 μl of PCR GoTaq Green Mastermix (Promega Biotech India Pvt.Ltd.), 1 μl (50 ng) of DNA template, and 22 μl of autoclaved milliQ water in a final volume of 50 μl. DNA amplification was performed in a thermocycler (Veriti™ 96-Well Fast Thermal Cycler, Applied Biosystems™) with the following thermocycler regime: initial denaturation at 95°C for 2 min followed by 30 cycles of 94°C for 1 min, 50°C for 50 s, 72°C for 1.5 min, and a final extension at 72°C for 5 min. The PCR amplified products were purified using Nucleospin® spin columns from Macherey-Nagel (Germany), following the instructions provided by the manufacturer. The purified PCR products were sequenced at Barcode Biosciences, Bangalore, India. The isolates were identified using the Nucleotide Basic Local Alignment Search Tool (BLAST N) program available at the NCBI server (www.ncbi.nlm.nih.gov/BLAST) and EZBioCloud (www.ezbiocloud.net) based on 16S rRNA gene sequences.
Plant growth promotion traits
Plant growth promotion (PGP) activities of the endophytic bacterial isolates were determined using standard protocols for indole-3-acetic acid (IAA) production, 1- aminocyclopropane-1-carboxylic acid (ACC) deaminase activity, putative N-fixing activity (Batista et al., 2021), siderophores production (Murakami et al., 2021), and hydrogen cyanide (HCN) production (Gupta and Pandey, 2019).
Screening of phosphate solubilization ability by qualitative method
The phosphate solubilization assay was done by using an NBRIP medium (10 g glucose, 5 g Ca3(PO4)2, 5 g MgCl2.6H2O, 0.25 g MgSO2.7H2O, 0.2 g KCl, 0.1 g (NH4)2SO4, 1.5% agar per 1000 ml distilled water, pH 7.0) (Batista et al., 2021). The bacterial isolates were spot inoculated on this media plates and incubated at 30°C for up to 7-10 days. The development of a clear halo zone around bacterial isolates indicated positive phosphate solubilization activity, as described by Batista et al. (2021). The diameter of each halo zone (Dh) and the diameter of its respective colony (Dc) were measured for each bacterial isolate and taken for determining the Phosphate Solubilization Index (PSI) using the formula, PSI = Dh/Dc,
Quantification of phosphate solubilization
Based on the size of halo zones, a few bacterial endophytes were further selected to quantify soluble phosphate as an indication of their ability as a phosphate solubilizer. The selected isolates were initially grown in 10 ml LB broth to ensure sufficient cell density and then pelleted down by centrifugation at 6000 rpm for 10 min. The cell pellets were washed three times with 5 ml of NBRIP medium without phosphate. The washed cells were added to 50 ml of NBRIP medium with a final OD600 of 0.1. The uninoculated medium without any bacterial isolate was served as a control. The flasks were incubated at 30°C for 5 days. Estimation of soluble phosphate was performed by taking out 5 ml of bacterial culture over a period of 24 h to 72 h, as described by Varga et al. (2020). Five ml of bacterial culture was transferred to a 15 ml falcon tube and allowed to settle for 90 min. Five μl of the supernatant from the settled cells were used to measure the soluble phosphate concentration as described by Chen et al. (1956). At 820 nm, the absorbance was measured, and the amount of solubilized phosphate was calculated using the standard equation of potassium dihydrogen phosphate (KH2PO4) in the liquid medium (Varga et al., 2020). A digital pH meter was used to determine the pH of the bacterial suspension.
Mobilization of phosphorus to wheat plants by endophytic bacterial isolates
To evaluate the effect of phosphate solubilizing bacteria on the growth of crop plants, a pot trial experiment under glasshouse conditions was conducted by selecting two endophytic bacterial isolates (HCF6 and HCF9) representing high phosphate solubilization ability. Wheat plants, variety J.W. 3173, were selected for growth evaluation using sand as the growth medium. The sand was washed with deionized water several times to remove any trace of soluble phosphate, and then left the sand to dry in the open air for 6 days. The sand was autoclaved two times at 121°C and 15 psi for 20 min. Phosphate content of the sand was estimated using a kit before it was thoroughly mixed with insoluble tricalcium phosphate in a 200:1 (wt/wt) ratio.
Plastic plant growth pots were filled with 1 kg of washed and dried sand mixed with 4 g of Ca3(PO4)2. Wheat seeds were surface sterilized by submerging for 4 min in 70% ethanol, then treating for 60 s with a 2% NaOCl solution while gently shaking them, and finally rinsing four times with sterile milliQ water. The bacterized seeds were germinated in Petri dishes (8 seeds per dish) on moist filter paper, then sealed with parafilm and kept at 22-27°C in the dark for four days. Ten-day-old seedlings were transplanted into plastic pots with eight seedlings each. One week after planting, 1 ml of bacterial inoculum was added close to the seedling roots for bacterial inoculation. Control seedling was inoculated with only sterile milliQ water. The experiment was conducted for 60 days, from February, 2022 to April, 2022, under natural light, with two replicates per treatment. Plants were watered once a week along with an inorganic phosphate-free plant nutrient solution. Plants were destructively harvested 60 days after sowing (DAS), with sand removed from the plant roots by gently brushing and washing with tap water. Root and shoot length and dry weight were determined as described by Otieno et al. (2015).
Quantification of inorganic phosphate content of treated wheat plants
Pi content in the plant materials was measured using the method of Ames (1966). Shoots and roots pulled from 3-4 wheat plants per treatment were weighed to a minimum amount of 100 mg depending on treatment condition and seedling condition, homogenized in liquid N2, extracted with 2.5 ml of 1% acetic acid, and tissue lysing by dipping the samples in liquid N2 for 30 s as described by Morcillo et al. (2020). Debris was pelleted by centrifugation at 12,000 rpm for 2 min, and 100 μl of root and shoot supernatant was mixed with 1.4 ml of (10% ascorbic acid + 0.42% ammonium molybdate 4H2O in a 1N H2SO4) reagent in the ratio of 1:6 and 500 μl of H2O (Chen et al., 1956). The reaction mixture was incubated at 45°C for 20 min, followed by absorbance at 820 nm. Pi content was calculated as 0.01 μM Pi at A820 = 0.26 Ab.
Statistical analyses
GraphPad Prism statistical software (Version 8.0.2) was used to create the graph and examine the data for significant mean differences using unpaired student t-test. Statistical significance was determined at the p<0.05.
Results
Isolation of endophytic bacteria
Bacterial endophytes have been implicated in possessing diverse beneficial roles in plants. In the present study, bacterial endophytes were isolated from unopened flowers of H. coronarium using a culture-dependent standard plating technique. Use of four different isolation media along with plant-extract amended media resulted in the isolation of 9 bacterial isolates. Out of these nine isolates, seven isolates exhibited varied morphological and biochemical characteristics. These seven endophytic isolates were designated as HCF1, HCF2, HCF3, HCF4, HCF6, HCF7, and HCF9. The isolates appeared either white or yellow in colour, and Gram staining followed by microscopic observation revealed that out of 7 bacterial isolates 5 were Gram-negative and 2 were Gram-positive.
Molecular and biochemical identification of bacterial isolates
The 16S rRNA gene sequences were used to identify the bacterial isolates. BLAST results of 16S rRNA gene sequences using EzBioCloud database revealed that out of 7 isolates, HCF1 and HCF2 showed maximum identity with genus Sphingomonas, HCF3 with Pseudomonas, HCF4 and HCF7 with Bacillus, and HCF6 and HCF9 with genus Pantoea (Table 1). All the isolates except HCF1 showed more than 97% identity with their respective matched genera. The isolates HCF6 and HCF9 revealed a maximum identity of 97.17% with the strain Pantoea sp. At-9b, and morphologically these two strains also appeared very similar. However, biochemical tests for 31 characters revealed identical results except for the utilization of mannose, sucrose, xylose, and adonitol (Table S1). The 16S rRNA gene sequences of HCF6 and HCF9 were deposited to NCBI GenBank with the accession number OP199041 and OP199042, respectively.
Plant growth promotion traits of the isolates
As shown in Table 2, the plant growth promoting traits of endophytic bacterial isolates were determined by in vitro methods. All the isolates were positive for the production of IAA, and HCF9 produced more IAA (116.67 ± 1.21 μg ml-1) in comparison to other bacterial isolates. The isolate HCF3 was the only endophyte that tested positive for HCN production. All 7 bacterial isolates tested positive for the production of ammonia, siderophores, and ACC deaminase and for the ability to fix nitrogen. The HCF3 showed more production of siderophores in comparison to other bacterial isolates. Four isolates viz. HCF3, HCF4, HCF6, and HCF9 were positive for phosphate solubilization with varied abilities as observed on the NBRIP agar media (Figure 1A).
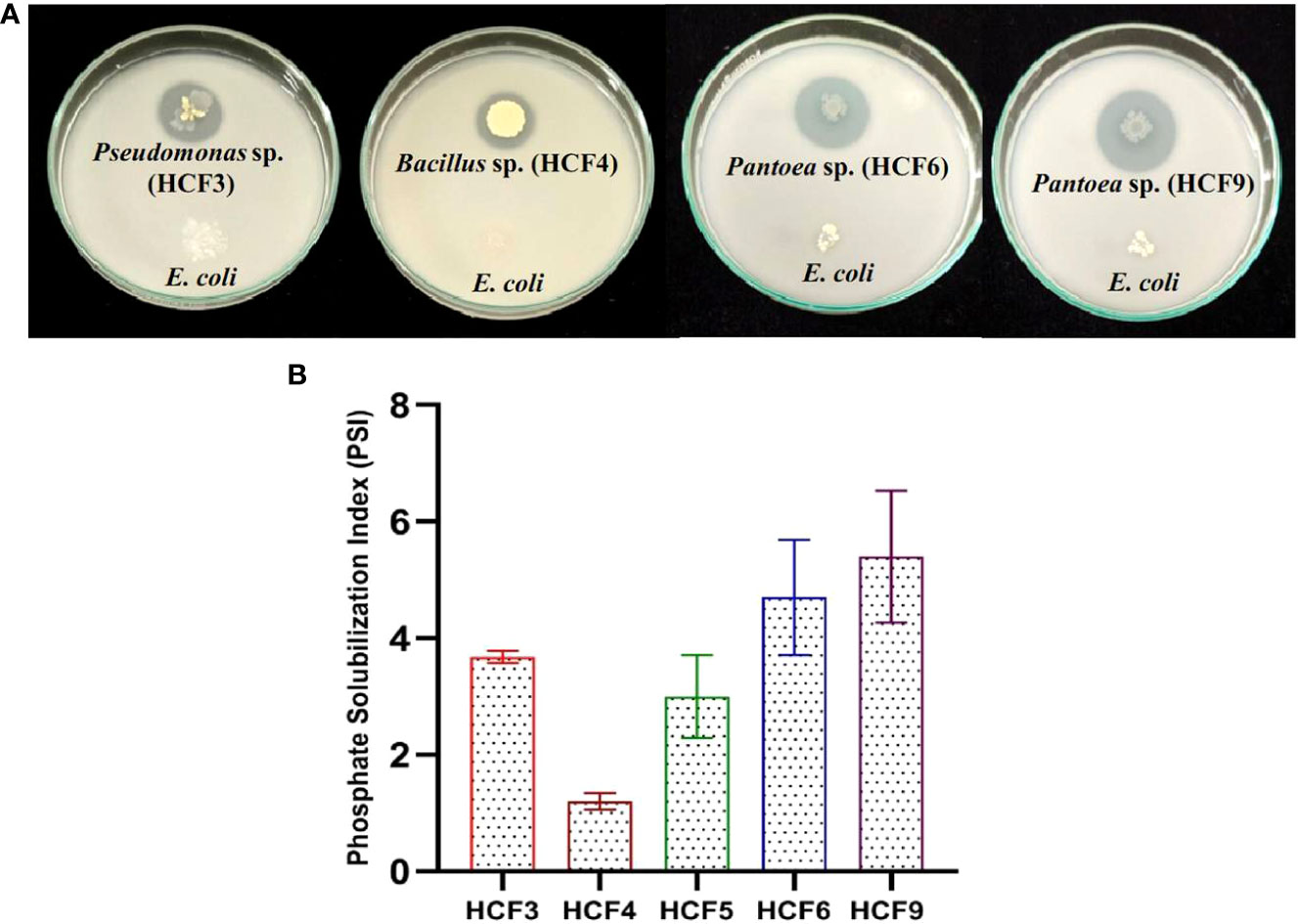
Figure 1 Phosphate solubilization by endophytic bacterial isolates on National Botanical Research Institute’s Phosphate (NBRIP) solid media. (A) Representative Petriplates showing halo zones by the test isolates along with E. coli control. (B) Phosphate solubilization index (PSI) of the bacterial isolates. Error bars represent the standard error of the means of two independent experiments.
Phosphate solubilization by the bacterial endophyte isolates
Screening of phosphate solubilization ability using the bacterial inoculum taken from solid agar media resulted in the identification of four isolates showing well-developed halo zones after 7 days of incubation in NBRIP medium. However, to have consistent and quantifiable data, 10 µl of fresh liquid cultures of four isolates with 0.1 OD600 were spot inoculated on NBRIP agar media with E. coli as a negative control. All the four positive isolates formed a clear halo zone with a diameter ranging from 1.2 to 5.4 cm (Figure 1A). PSI was higher for HCF9 (5.4 ± 0.8) followed by HCF6 (4.7 ± 0.7), HCF3 (3.67 ± 0.07) and HCF4 (1.2 ± 0.1) as shown in Figure 1B.
Bioassays on solid media indicated both HCF6 and HCF9 as efficient phosphate solubilizers. Therefore, these two bacterial isolates were further tested for their ability to solubilize Ca3(PO4)2 and AlPO4 in the liquid medium. Both isolates grew well in the medium containing Ca3(PO4)2 than AlPO4-containing medium (data not shown). Quantification of soluble phosphates by colorimetric method showed efficient solubilization of Ca3(PO4)2 by both HCF6 (2051 ± 77.74 μg ml-1) and HCF9 (2196.49 ± 218.33 μg ml-1) (Figure 2A). Both the isolates showed approximately 7-fold lesser phosphate solubilizing ability on AlPO4-containing media, and were significant when compared with the uninoculated control (Figure 2B). Additionally, the pH of the HCF6 and HCF9 inoculated media containing Ca3(PO4)2 were dropped to 3.8 and 3.5, respectively (Table S2).
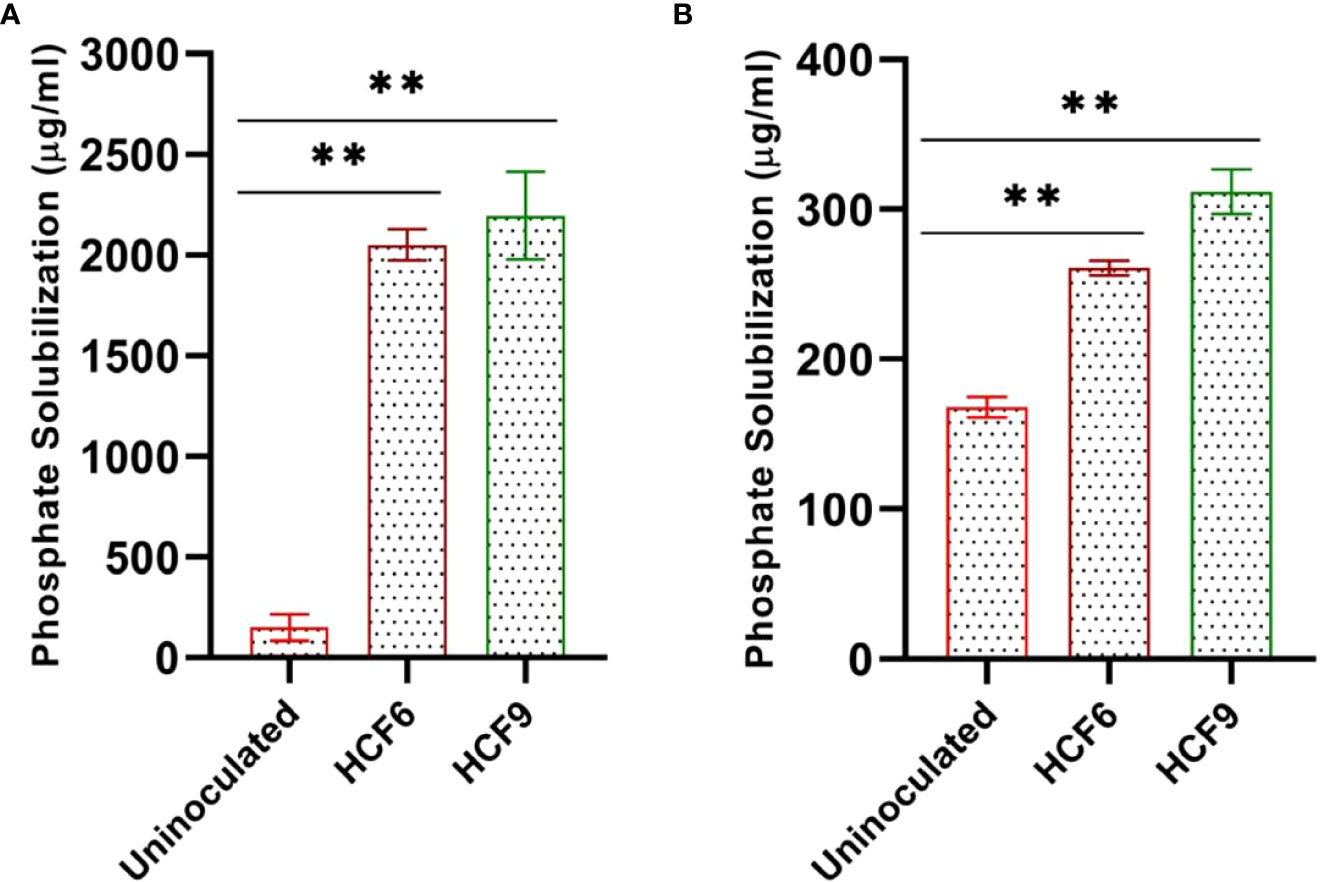
Figure 2 Quantification of soluble phosphate released from NBRIP liquid media after 3 days incubation. (A) Test bacteria inoculated with Ca3(PO4)2, and (B) with AlPO4 showing significant difference from uninoculated media. **P < 0.01.
Effect of HCF6 and HCF9 on the growth of wheat plant
To evaluate the plant growth-promoting ability, two highly efficient phosphate solubilizing isolates with several other PGP traits (Table 2) were selected for a pot experiment using sand as a medium. The P concentration of the sand used for wheat growth was estimated as low (<22 kg per hectare). HCF6 and HCF9 promoted wheat growth with slight differences in the measured growth parameters (Figure 3). Plants inoculated with HCF6 and HCF9 significantly outperformed the untreated control in terms of root and shoot length and dry weight (Figures 3B, C).
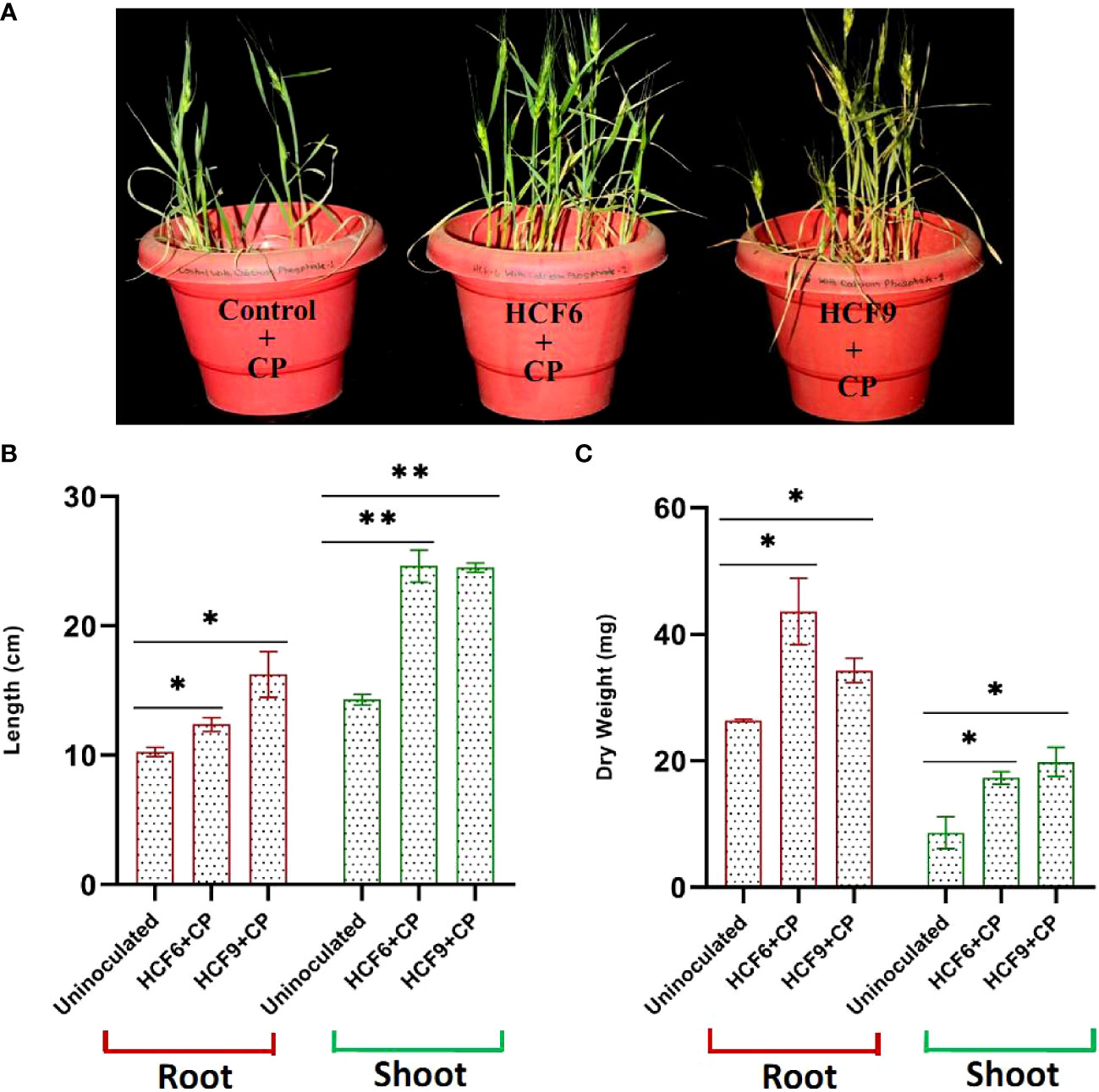
Figure 3 Effect of different bacterial inoculants when treated along with Ca3(PO4)2 (CP) on wheat plant grown in sand as growth medium. (A) Image of 45 days old wheat plant (B) Root and shoot length (cm) (C) Dry mass of root and shoot (mg). Data represent the mean values (n=6 ± SE) of two independent experiments. **P < 0.01; *P < 0.05.
Mobilization of P to wheat was assessed by measuring the Pi content in the roots and shoots of the treated plants. The Pi content in wheat root and shoot was determined using the colorimetric method. The Pi content in the root and shoot were significantly higher in HCF6- and HCF9-treated wheat plants in comparison to untreated control plants (Figure 4). The soluble Pi content in the fresh root of HCF6 was 83.58 ± 2.11 nmolPi/mg, while HCF9 showed maximum Pi content of 121.75 ± 4.49 nmolPi/mg (Figure 4A). Similarly, both HCF6 (107.23 ± 0.49 nmolPi/mg) and HCF9 (110.85 ± 1.71 nmolPi/mg) treatment had higher soluble Pi content in the fresh shoot than uninoculated (68.66 ± 1.02 nmolPi/mg) samples (Figure 4B).
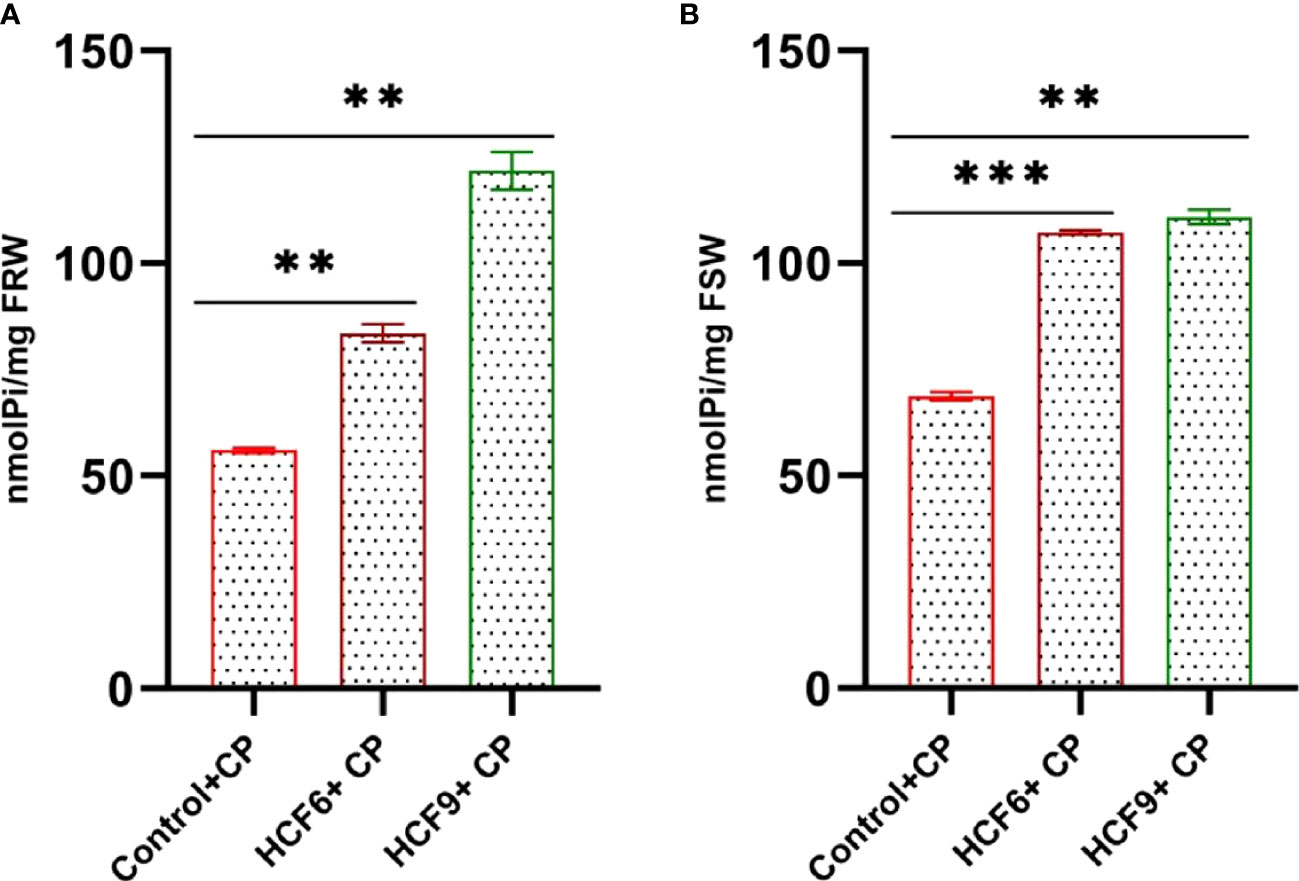
Figure 4 Quantification of inorganic phosphate (Pi) content in the root and shoot tissue of wheat plant grown in Ca3(PO4)2-mixed sand medium (CP). (A) Total soluble Pi content (nmolPi/mg) per mg of fresh root weight (FRW). (B) Total soluble Pi content (nmolPi/mg) per mg of fresh shoot weight (FSW). Data are reported as mean values (n=6 ± SE) of two independent experiments. ***P < 0.001; **P < 0.01.
Discussion
P is considered the second most limiting nutrient to the plants, next to nitrogen. Often Pi in the soil makes complexes with various cations, such as Ca2+, Al3+, and Fe3+, making the P unavailable for plants (Alori et al., 2017). Similarly, phytate is a storage form of organic phosphate, which is unavailable for plant use because it forms complexes with cations (Kalayu, 2019). In this context, phosphate solubilizing microorganisms, including fungi and bacteria, play a vital role in enhancing P acquisition in plants. In the present study, two highly efficient phosphate solubilizing bacterial endophytes (HCF6 and HCF9) belonging to the genus Pantoea were isolated from the flower of Hedychium coronarium. Both these isolates were capable of solubilizing the two most common types of metal ion complexes, i.e. Ca3(PO4)2 and AlPO4 (Figure 2). The ability of both HCF6 and HCF9 to efficiently solubilize Ca3(PO4)2 could be advantageous to crops in alkaline soil. In contrast, the capability to solubilize AlPO4 could be an advantage for crops in acidic soils. Additionally, endophyte bacteria like HCF6 and HCF9 may be advantageous as bioinoculants in solubilizing the P complexes present within the plant, similar to two reported bacterial endophytes, Rahnella sp. and Burkholderia sp. isolated from Populus trichocarpa (Varga et al., 2020).
One of the most important phosphate solubilization mechanisms is the production of low molecular weight organic acids by plant-associated bacteria, which results in soil or media acidification (Khan et al., 2014; Matos et al., 2017). In our study, both HCF6 and HCF9 fully solubilized Ca3(PO4)2 with a significant drop in pH from neutral to acidic (Table S2), suggesting secretion of organic acids into the medium could be one of the mechanisms of phosphate solubilization. Organic acids can chelate the cation bound to phosphate with their hydroxyl and carboxyl groups, thereby making the P available to plants (Kpomblekou-a and Tabatabai, 1994). A minor change in the media pH was observed in the case of AlPO4 solubilization (Table S2), suggesting a solubilization mechanism other than the involvement of organic acids. It is also conceivable that at low pH, Al3+ makes a stronger interaction with a phosphate ion, indicating the organic acid-mediated phosphate solubilization may not be suitable to solubilize AlPO4 in the soil. In addition to organic acid secretions, many bacteria in the rhizosphere secrete phytase enzyme which could release soluble phosphate from phytate, resulting in the solubilization of phosphate and making it available for plant uptake (Richardson et al., 2009).
In addition to phosphate solubilization, both the Pantoea isolates (HCF6 and HCF9) possess several PGP traits such as IAA and siderophores production, N-fixation ability, and ACC deaminase activity (Table 2). Due to these attributes, both the isolates were tested for wheat plant growth promotion under low phosphate conditions using sand as a medium for growth. Our result showed that wheat plants treated with HCF6 and HCF9 isolates had increased root and shoot length and plant dry mass (Figure 3). Increased root and shoot length could be attributed to the ability of both the isolates to produce IAA (Table 2). Production of IAA in many beneficial bacteria is considered a selection criterion as biofertilizers, and application of these bacteria has been shown to promote plant growth in wheat (Spaepen et al., 2008; Ali et al., 2009).
A greater root and shoot dry mass in the inoculated wheat plants is also suggestive of efficient mobilization of P into plants due to better solubilization of Ca3(PO4)2 provided in the sand medium. A better availability of soluble phosphate to the bacterial-treated wheat plants was further supported by enhanced Pi content in the root (33% and 54% increase for HCF6 and HCF9, respectively) and shoot (35.9% and 38.06% increase for HCF6 and HCF9, respectively), when compared with the plants that were not treated with bacteria (Figure 4). The rhizosphere and endosphere PSB belonging to genus Pantoea have been shown to increase phosphate uptake and plant growth in sugarcane (Quecine et al., 2012), tomato and pepper (Mei et al., 2021), potato (Malboobi et al., 2009), mung bean (Walpola and Yoon, 2013), and chickpea (Singh et al., 2014).
Inoculants of bacteria that promote plant growth can be used as a biofertilizer, which is a promising alternative to chemical fertilizers. Our study demonstrates that both the bacterial isolates, HCF6 and HCF9, have a tremendous ability to solubilize Ca-phosphate and Al-phosphate and enhance wheat plant growth under P starvation. However, further study is required to understand the mechanism by which both these Pantoea isolates solubilize Ca-phosphate and Al-phosphate. Additional research is also required to establish the relevance of phosphate solubilization within the plant and its implications for sustainable agriculture.
Conclusions
Using various culture media, including plant-amended media, two bacterial endophytes belonging to the genus Pantoea were isolated from the closed flowers of a medicinal plant, Hedychium coronarium. These two bacterial endophytes are bestowed with many PGP traits in addition to efficient phosphate solubilizers. Both the Pantoea isolates efficiently solubilize Ca3(PO4)2 and, to a lesser extent, solubilize AlPO4, which are highly desirable traits for phosphate solubilizing bioinoculants to be applied in both alkaline and acidic soils. Our results demonstrate that both HCF6 and HCF9 have promoted plant growth in wheat and accumulated higher Pi in plant tissues suggesting their role in mobilizing soluble P from insoluble P-complexes.
Data availability statement
The data presented in the study are deposited in NCBI GenBank, accession number OP199041 and OP199042.
Author contributions
SD, AP, and NS planned the experiments. PP and SK performed the experiments and prepare the rough draft of the article. PP, SD, and SK have analyzed the data. SD, AP, and NS revised and interpreted the data. All authors read and approved the manuscript. All authors contributed to the article and approved the submitted version.
Funding
We thank DST-FIST Level-I infrastructure support to the Department of Botany, Indira Gandhi National Tribal University. PP thanks University Grants Commission (UGC) for Non-NET fellowship. ARP thanks DST, GoI for the JC Bose Fellowship (Grant No. JCB/2017/000053).
Conflict of interest
The authors declare that the research was conducted in the absence of any commercial or financial relationships that could be construed as a potential conflict of interest.
Publisher’s note
All claims expressed in this article are solely those of the authors and do not necessarily represent those of their affiliated organizations, or those of the publisher, the editors and the reviewers. Any product that may be evaluated in this article, or claim that may be made by its manufacturer, is not guaranteed or endorsed by the publisher.
Supplementary material
The Supplementary Material for this article can be found online at: https://www.frontiersin.org/articles/10.3389/fagro.2022.990869/full#supplementary-material
References
Ali B., Sabri A. N., Ljung K., Hasnain S. (2009). Auxin production by plant associated bacteria:Impact on endogenous IAA content and growth of Triticum aestivum l. Lett. Appl. Microbiol. 48, 542–547. doi: 10.1111/j.1472-765X.2009.02565.x
Alori E. T., Glick B. R., Babalola O. O. (2017). Microbial phosphorus solubilization and its potential for use in sustainable agriculture. Front. Microbiol. 8. doi: 10.3389/fmicb.2017.0097
Ames B. N. (1966). Assay of inorganic phosphate, total phosphate and phosphatase. Meth. Enzymol. 8, 115–118. doi: 10.1016/0076-6879(66)08014-5
Anthony C. (2004). The quinoprotein dehydrogenases for methanol and glucose. Arch. Biochem. Biophys. 428 (1), 2–9. doi: 10.1016/j.abb.2004.03.038
Azizoglu U. (2019). Bacillus thuringiensis as a biofertilizer and biostimulator: a mini-review of the little-known plant growth-promoting properties of bt. Curr. Microbiol. 76 (11), 1379–1385. doi: 10.1007/s00284-019-01705-9
Basu A., Prasad P., Das S. N., Kalam S., Sayyed R. Z., Reddy M. S., et al. (2021). Plant growth promoting rhizobacteria (PGPR) as green bioinoculants: recent developments, constraints, and prospects. Sustainability. 13 (3), 1140. doi: 10.3390/su13031140
Batista B. D., Bonatelli M. L., Quecine M. C. (2021). The microbiome: Methods and protocols. Methods Mol. Biol. 2232, 61–75. doi: 10.1007/978-1-0716-1040-4
Chen P. S., Toribara T. T., Warner H. (1956). Micro determination of phosphorus. Anal. Chem. 28 (11), 1756–1758. doi: 10.1021/ac60119a033
Cole M. B., Augustin M. A., Robertson M. J., Manners J. M. (2018). The science of food security. NPJ Sci. Food. 2, 14. doi: 10.1038/s41538-018-0021-9
Daniels C., Michán C., Ramos J. L. (2009). New molecular tools for enhancing methane production, explaining thermodynamically limited lifestyles and other important biotechnological issues. Microb. Biotechnol. 2 (5), 533–536. doi: 10.1111/j.1751-7915.2009.00134.x
Etesami H., Jeong B. R. (2021). Contribution of arbuscular mycorrhizal fungi, phosphate–solubilizing bacteria, and silicon to p uptake by plant: a review. Front. Plant Sci. 12. doi: 10.3389/fpls.2021.699618
Goldstein A. H., Rogers R. D., Mead G. (1993). Mining by microbe. Biotechnology. 11 (11), 1250–1254. doi: 10.1038/nbt1193-1250
Gupta S., Pandey S. (2019). ACC deaminase producing bacteria with multifarious plant growth promoting traits alleviates salinity stress in French bean (Phaseolus vulgaris) plants. Front. Microbiol. 10, 1506. doi: 10.3389/fmicb.2019.01506
Illmer P., Barbato A., Schinner F. (1995). Solubilization of hardly-soluble AlPO4 with p-solubilizing microorganisms. Soil Biol. Biochem. 27 (3), 265–270. doi: 10.1016/0038-0717(94)00205-F
Kalayu G. (2019). Phosphate solubilizing microorganisms: promising approach as biofertilizers. Int. J. Agron. Agric. Res. 2019. doi: 10.1155/2019/4917256
Khan M. D., Zaidi A., Ahmad E. (2014). “Mechanism of phosphate solubilization and physiological functions of phosphate-solubilizing microorganisms,” in Phosphate solubilizing microorganisms (Cham: Springer), 31–62. doi: 10.1007/978-3-319-08216-5_2
Kpomblekou-a K., Tabatabai M. A. (1994). Effect of organic acids on release of phosphorus from phosphate rocks1. Soil Sci. 158 (6), 442–453. doi: 10.1097/00010694-199415860-00006
Lucero C. T., Lorda G. S., Anzuay M. S., Ludueña L. M., Taurian T. (2021). Peanut endophytic phosphate solubilizing bacteria increase growth and p content of soybean and maize plants. Curr. Microbiol. 78 (5), 1961–1972. doi: 10.1007/s00284-021-02469-x
Malboobi M. A., Behbahani M., Madani H., Owlia P., Deljou A., Yakhchali B., et al. (2009). Performance evaluation of potent phosphate solubilizing bacteria in potato rhizosphere. World J. Microbiol. Biotechnol. 25 (8), 1479–1484. doi: 10.1007/s11274-009-0038-y
Matos A. D., Gomes I. C., Nietsche S., Xavier A. A., Gomes W. S., Dos Santos J. A., et al. (2017). Phosphate solubilization by endophytic bacteria isolated from banana trees. N. Acad. Bras. Cienc. 89, 2945–2954. doi: 10.1590/0001-3765201720160111
Mei C., Chretien R. L., Amaradasa B. S., He Y., Turner A., Lowman S. (2021). Characterization of phosphate solubilizing bacterial endophytes and plant growth promotion in vitro and in greenhouse. Microorganisms. 9 (9), 1935. doi: 10.3390/microorganisms9091935
Morcillo R. J., Singh S. K., He D., An G., Vílchez J. I., Tang K., et al. (2020). Rhizobacterium-derived diacetyl modulates plant immunity in a phosphate-dependent manner. EMBO J. 39 (2), 102602. doi: 10.15252/embj.2019102602
Mousa W. K., Shearer C. R., Limay-Rios V., Zhou T., Raizada M. N. (2015). Bacterial endophytes from wild maize suppress Fusarium graminearum in modern maize and inhibit mycotoxin accumulation. Front. Plant Sci. 6. doi: 10.3389/fpls.2015.00805
Murakami C., Tanaka A. R., Sato Y., Kimura Y., Morimoto K. (2021). Easy detection of siderophore production in diluted growth media using an improved CAS reagent. J. Microbiol. Methods 189, 106310. doi: 10.1016/j.mimet.2021.106310
Otieno N., Lally R. D., Kiwanuka S., Lloyd A., Ryan D., Germaine K. J., et al. (2015). Plant growth promotion induced by phosphate solubilizing endophytic Pseudomonas isolates. Front. Microbiol. 6. doi: 10.3389/fmicb.2015.00745
Papik J., Folkmanova M., Polivkova M., Suman J., Uhlik O. (2020). The invisible life inside plants: Deciphering the riddles of endophytic bacterial diversity. Biotechnol. Adv. 44, 107614. doi: 10.1016/j.biotechadv.2020.107614
Quecine M. C., Araújo W. L., Rossetto P. B., Ferreira A., Tsui S., Lacava P. T., et al. (2012). Sugarcane growth promotion by the endophytic bacterium pantoea agglomerans 33.1. Appl. Environ. Microbiol. 78 (21), 7511–7518. doi: 10.1128/AEM.00836-12
Richardson A. E., Barea J. M., McNeill A. M., Prigent-Combaret C. (2009). Acquisition of phosphorus and nitrogen in the rhizosphere and plant growth promotion by microorganisms. Plant Soil. 321 (1), 305–339. doi: 10.1007/s11104-009-9895-2
Sashidhar B., Podile A. R. (2010). Mineral phosphate solubilization by rhizosphere bacteria and scope for manipulation of the direct oxidation pathway involving glucose dehydrogenase. J. Appl. Microbiol. 109 (1), 1–12. doi: 10.1111/j.1365-2672.2009.04654.x
Sharma A. D., Singh J. (2005). A nonenzymatic method to isolate genomic DNA from bacteria and actinomycete. Anal. Biochem. 337 (2), 354–356. doi: 10.1016/j.ab.2004.11.029
Sharon J. A., Hathwaik L. T., Glenn G. M., Imam S. H., Lee C. C. (2016). Isolation of efficient phosphate solubilizing bacteria capable of enhancing tomato plant growth. J. Soil Sci. Plant Nutr. 16 (2), 525–536. doi: 10.4067/S0718-95162016005000043
Singh O., Gupta M., Mittal V., Kiran S., Nayyar H., Gulati A., et al. (2014). Novel phosphate solubilizing bacteria ‘Pantoea cypripedii PS1’along with Enterobacter aerogenes PS16 and Rhizobium ciceri enhance the growth of chickpea (Cicer arietinum l.). Plant Growth Regul. 73 (1), 79–89. doi: 10.1007/s10725-013-9869-5
Singh B. K., Trivedi P., Egidi E., Macdonald C. A., Delgado-Baquerizo M. (2020). Crop microbiome and sustainable agriculture. Nat. Rev. Microbiol. 18 (11), 601–602. doi: 10.1038/s41579-020-00446-y
Spaepen S., Dobbelaere S., Croonenborghs A., Vanderleyden J. (2008). Effects of Azospirillum brasilense indole-3-acetic acid production on inoculated wheat plants. Plant Soil. 312, 15–23. doi: 10.1007/s11104-008-9560-1
Tian J., Ge F., Zhang D., Deng S., Liu X. (2021). Roles of phosphate solubilizing microorganisms from managing soil phosphorus deficiency to mediating biogeochemical p cycle. Biology 10 (2), 158. doi: 10.3390/biology10020158
Varga T., Hixson K. K., Ahkami A. H., Sher A. W., Barnes M. E., Chu R. K., et al. (2020). Endophyte-promoted phosphorus solubilization in populus. Front. Plant Sci. 11, 1585. doi: 10.3389/fpls.2020.567918
Walpola B. C., Yoon M. H. (2013). Phosphate solubilizing bacteria: Assessment of their effect on growth promotion and phosphorous uptake of mung bean (Vigna radiata [L.] r. wilczek). Chil. J. Agric. Res. 73 (3), 275. doi: 10.4067/S0718-58392013000300010
Keywords: Hedychium coronarium, flower endophytes, phosphate solubilization, Pantoea, plant growth promotion
Citation: Prasad P, Kalam S, Sharma NK, Podile AR and Das SN (2022) Phosphate solubilization and plant growth promotion by two Pantoea strains isolated from the flowers of Hedychium coronarium L.. Front. Agron. 4:990869. doi: 10.3389/fagro.2022.990869
Received: 10 July 2022; Accepted: 25 August 2022;
Published: 13 September 2022.
Edited by:
Shalini Tiwari, Jawaharlal Nehru University, IndiaReviewed by:
Tanvir Kaur, Eternal University, IndiaNikita Bisht, National Botanical Research Institute (CSIR), India
Copyright © 2022 Prasad, Kalam, Sharma, Podile and Das. This is an open-access article distributed under the terms of the Creative Commons Attribution License (CC BY). The use, distribution or reproduction in other forums is permitted, provided the original author(s) and the copyright owner(s) are credited and that the original publication in this journal is cited, in accordance with accepted academic practice. No use, distribution or reproduction is permitted which does not comply with these terms.
*Correspondence: Subha Narayan Das, subha.bunu@igntu.ac.in; Appa Rao Podile, arpsl@uohyd.ernet.in