- 1Institute of Organic Farming and Sustainable Soil Use, Justus Liebig University, Giessen, Germany
- 2International Institute of Tropical Agriculture (IITA), CSIR Campus, Legon, Ghana
- 3Hans-Ruthenberg-Institute of Tropical Agricultural Sciences, University Hohenheim, Stuttgart, Germany
Low-cost but productive crop intensification options are needed to assist smallholder farmers in the tropics to move away from poverty. This study assessed the capacity of cowpea (Vigna unguiculata (L.) Walp.) to perform biological N fixation (BNF) under no-tillage practices, crop residue cover and intercropping with maize (Zea mays L.). The study was performed during the long rains of 2017 at Kiboko experimental station, located in semi-arid eastern Kenya. The research field trials had been running for three years (6 cropping seasons) by the time the sampling took place. The experimental set up was a split-plot design: main plots being tillage system (no till + maize stover retention (CA), and conventional tillage to 15 cm depth without mulch retention (CT)), sub-plot being cropping system (maize-cowpea intercrop, maize monocrop, and cowpea monocrop). Cowpea plants were sampled at 50% flowering stage and at physiological maturity to investigate biomass production and %N derived from the atmosphere (%Ndfa) through the 15N natural abundance technique, using maize as a reference control. Results showed that the number of nodules per plant was higher in CA treatments rather than in CT although not significant (p>0.05). Mean cowpea grain yield at harvest varied between 472 – 590 kg ha‐1 in intercrops whereas grain yield in monocrops was between 1465 - 1618 kg ha-1. Significant differences were however recorded between treatments with CT monocropped cowpea at flowering recording the highest mean %Ndfa (62%) and CT intercrop the lowest (52%). At harvest stage CA inter recorded the highest %Ndfa (54%) while CT intercrop the lowest (41%). The %Ndfa was higher (p<0.05) at flowering (between 57- 69%) compared with 45 - 64 % at harvest stage. Overall cowpeas in intercrops derived between 17.8 - 22.8 kg ha-1 of their total N from atmospheric dinitrogen fixation while monocrops between 54.9 - 55.2 kg ha-1. The effect of CA on BNF was positive but not significantly different from CT. These results suggest that CA has the potential to enhance the BNF process but there is a need to explore in future alternative spatial arrangement and variety choice in intercropping of cowpea and maize to optimize the BNF process.
Introduction
Maize-legume intercropping is widely used in Kenya and many countries across sub-Saharan Africa (SSA). In recent years, various options which combine use of mineral fertilizers, locally available amendments and organic matter (e.g. crop residues, compost and green manures) to improved land preparation (e.g. contour terraces, tied ridging, reduced tillage), cropping systems (e.g. mixed cropping, shifting cultivation, crop rotation) and plant management (e.g. seed germplasm, spacing densities, legume inoculation), are being explored to increase the productivity of smallholder farming systems (Sanginga and Woomer, 2009; Vanlauwe et al., 2010; Pretty et al., 2011). Among such options, conservation agriculture (CA) has been promoted for the past 20 years as a promising farm management system across vast areas of SSA. CA is characterised by three building pillars i.e., at least 30% of permanent ground cover (e.g. mulches, cover crops), minimal to no soil disturbance through tillage, and crop diversification either spatially or temporally, including a nodulating legume component (FAO, 2008). Results in literature suggest that the most pronounced benefits are derived when all these three practices are implemented and with better success on fine textured soils in the arid and semi-arid areas of the tropics and subtropics (Rusinamhodzi et al., 2011; Pittelkow et al., 2015; Steward et al., 2018). CA is designed to improve the three axes of soil health i.e., physical, biological, and chemical conditions.
In Kenya, CA was first introduced in the Laikipia County in 1997 by means of extension and training, through Farmer Field Schools (FFS) (Kaumbutho et al., 2007). It has been considered to have high potential to reduce soil degradation, sequester carbon and improve crop productivity (Van Hulst and Posthumus, 2016). While in Western parts of Kenya maize stover is more likely to be used as mulch, farmers in the East tend to maintain greater heads of livestock to which they can provide urea-treated cereal stover as feed or mix them with more digestible residues (Baudron et al., 2014). In such cases, locally available prunings from legume trees and shrubs such as Calliandra calothyrsus, Leucaena spp, Gliricidia sepium and Mucuna pruriens that persist during the dry season can be supplemented to the plot to cover the soil (Mugwe et al., 2019). Alternatively, grain legumes with a short growing cycle such as lablab, common bean, green grams, or cowpea are sown as cover crops during the short rains. Permanent ground cover through mulching contributes to increased cereal yields in seasons when soil moisture is limiting (Mupangwa et al., 2012) by acting as a buffer against large bare ground evapotranspiration due to intense solar radiation while inhibiting the conditions for weed appearance (Mgolozeli et al., 2020). Mulches also provide the initial decomposing material upon which fungi, bacteria, and abiotic processes act upon to transform complex organic material into inorganic molecules (Miki et al., 2010). Mixing of cereal mulching with more readily decomposable residues or mineral fertilizers can therefore be a viable strategy to improve stable soil organic matter (SOM) content and plant’s N availability (Gentile et al., 2008).
Nodulating grain legumes are important components of low input-high risk smallholder farming systems due to their delivery of multiple uses in food and soil fertility improvement (Giller, 2001). Intercropping grain legumes and maize is usually preferred to pure stands as an efficient means to improve land use efficiency, reduce production costs, provide a security net against single crop failures while diversifying diets and incomes (Rusinamhodzi et al., 2012). While most farmers alternate one line of maize to one of legume, enhanced competition may occur if the maize cultivar is a fast growing one and inhibits access to light to the understorey intercrop or limits belowground nutrient acquisition (Trenbath, 1986). Alternating two maize rows to two grain legume rows, also known as MBILI systems, has reduced intraspecific competition, and spread impact of environmental risks among the intercrops (Mucheru-Muna et al., 2009). Cowpea (Vigna unguiculata [L] Walp.) is an important grain legume in Kenya sowed mostly in the eastern semi-arid regions, as well as a significant and cheap source of protein for both rural and urban dwellers (Kebede et al., 2020). Both the leaves and green pods are consumed as vegetables while the dried grain is consumed as a pulse across different food preparations and is therefore referred to as a dual-purpose crop as well as being used as livestock feed (Giller, 2001). Protein content of green cowpea leaves ranges around 35% while the dry grain reports a crude protein concentration of circa 23% (Dakora and Belane, 2019). Known across the country as kunde, cowpea is an important component of cropping systems because it can contribute to enhance soil mineral N ( Nmin ) pools through its ability to establish mutualistic symbiosis with soil rhizobia leading to the conversion of elemental dinitrogen into plant available ammonia NH3, through biological nitrogen fixation (BNF) (Dakora and Belane, 2019). CA practices have been reported to enhance soil fauna, soil moisture retention and soil structure, especially under semiarid conditions (Chivenge et al., 2007; Babal et al., 2010; Ayuke et al., 2019) which could favour rhizobial nodulation to legume´s roots. Nevertheless, CA and cereal-legume intercropping with mulch retention have been reported to increase SOM content in the topsoil compared to conventional practices, which may lead to a reduction in plants´ need to acquire N from BNF. We found a dearth of information on the potential impacts of no tillage, soil cover and maize-legume intercropping on the grain legume´s BNF therefore the objective of this paper was to estimate cowpea´s %Ndfa and quantities of N returns production at flowering and at harvest stages under various management (CA and CT) and cropping systems (mono and intercrop).
Materials and methods
Site description
Kiboko experimental station is in Makueni County (37.7235’E, 2.2172’S) grouped into the lower midlands (LM5) of Eastern Kenya. The site lies at 975 m.a.s.l. surrounded by a gently hilly landscape dominated by mixed crop-livestock smallholder farming. Main cereals include maize, millet and sorghum which are cultivated in association or alternation with grain legumes such as green grams, cowpea, pigeonpea, marama beans and moth beans. Main vegetables produced in the region are pumpkins, cabbage and tomatoes and cash crops such as cotton and sunflower are also sown. The area is populated mostly by agro-pastoral Kamba and pastoral Maasai communities. The site receives on average 514 mm of rain per year, divided mostly in two seasons (short rains October-December, long rains February-May). This is a hot and semi-arid region with a mean annual temperature of 24.1°C, mean annual maximum of 31.1°C, mean annual minimum of 17°C and a mean relative humidity of 82.5% (Figure 1.). The soils at Kiboko are well drained, very deep, dark reddish brown to dark red, friable sandy clay classified as Acri-Rhodic Ferrassols developed from undifferentiated basement system rocks, predominantly banded gneisses (CIMMYT, 2013).
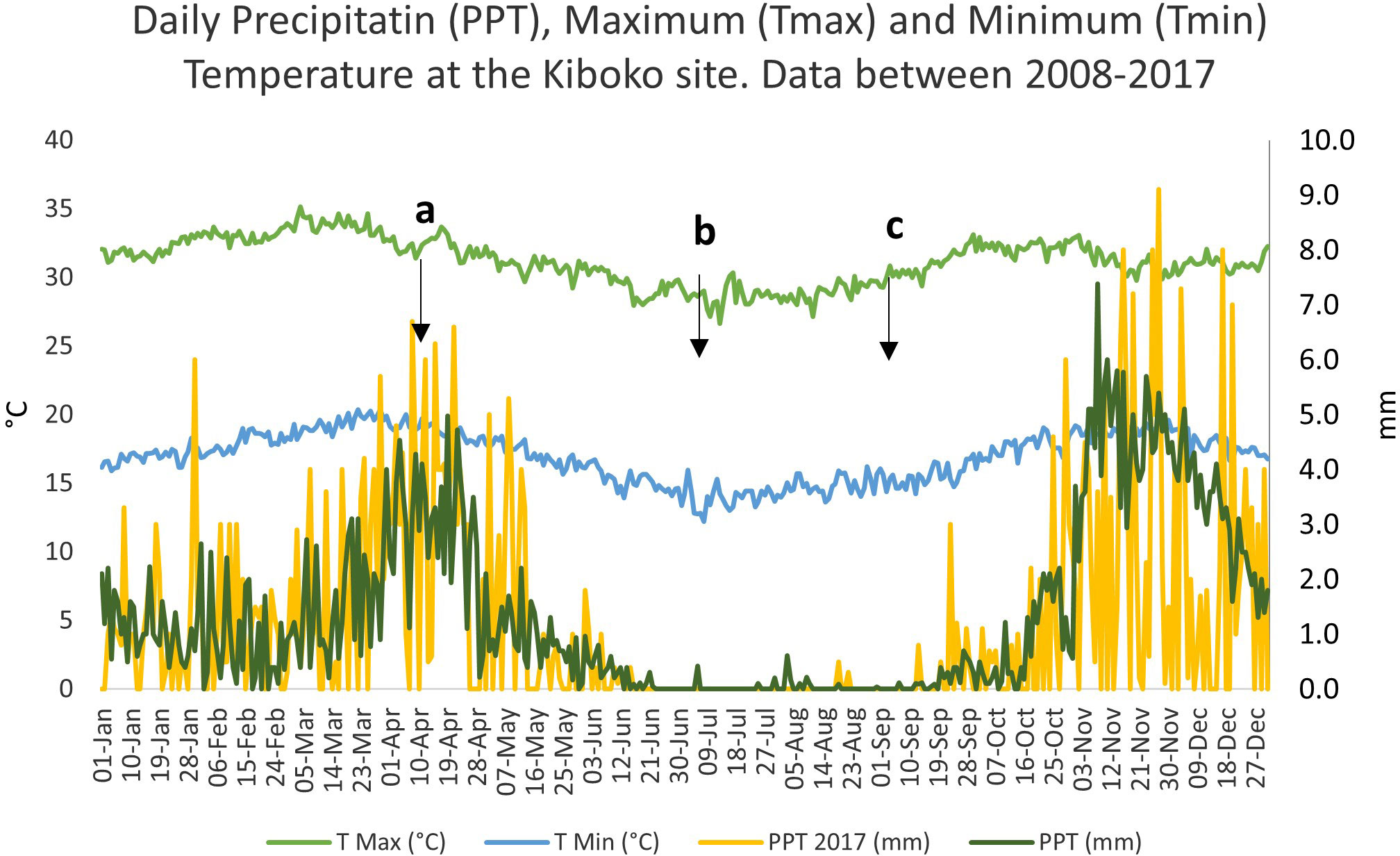
Figure 1 Daily Precipitatin (PPT), Maximum (Tmax) and Minimum (Tmin) Temperature at the Kiboko site. Data between 2008-2017. a, maize and cowpea sowing; b, cowpea harvest; c, maize harvest.
Experimental design and crop management
The experimental design was split-plot design with main plots being tillage method (conservation (CA) and conventional (CT)), subplots being cropping system (monocrop maize, monocrop cowpea and maize-cowpea intercrops) replicated in three blocks. The experimental plots had been running under the same cropping patterns since the short rains of 2014 and were in their 7th cropping season at the time the study took place (long rains of 2017), following two harvests per year. In no-till plots, maize and cowpea crop residues from the previous season were retained in situ (except for the grains and pods which were harvested) by manually uprooting stover meaning that the no tillage treatment also included crop residues retention. CA plots were aimed at being covered approximately by 70% with maize stover or ~5 Mg ha-1 of maize residues retained from previous seasons. In conventional tillage plots, stover was removed at harvest and seedbed preparation was performed manually before sowing, by using hoes to a depth of 15 cm, as per local practices to turn the soil and remove weeds. In CA plots, sowing was done after having established the row pattern, by pushing the seeds in the soil by hand to a 3 cm depth. Non-selective, pre-emergence herbicide was sprayed before sowing on all plots as well as maize systems being sprayed with duduthrine insecticide at grain filling stage and once more before harvest. Plots were watered twice between the end of the rainy season and maize harvest. Weeding was done three times during the maize growing season by manually uprooting weeds in CA and with a hoe in CT treatments. Intercrops were sowed under the MBILI system by alternating two rows of cowpea to two of maize, both in CA and CT plots. Sowing of cowpea was performed by placing two seeds per hole, later thinned to one plant per hill, while three seeds per hill were sowed for the maize, later thinned to two. In the intercrop plots intra and inter maize row spacing was 50 × 50 cm, while 33 cm between cowpea’s rows and 20 cm between cowpea’s hills within a row adding up to 53, 334 maize and 40, 675 cowpea plants ha-1. In the monocrop maize systems, maize was spaced 25 cm × 75 cm to achieve a planting density of 53, 334 plants ha-1. In the cowpea monocrop plots, these were spaced 20 × 35 cm apart resulting in 142, 587 plants ha-1. Each plot measured 36 m² while net plots for harvesting 26 m². On sowing day, starter diammonium phosphate (DAP) (N:P2O5:K2O ratio = 18:46:0) was applied to the maize plants at a rate of 100 kg ha-1 while 60 kg ha-1 of urea (N:P:K= 46:0:0) were applied as top dressing when the maize plants reached knee height. In plots used for the BNF sampling, no top dressing was applied whereas 15 kg ha-1 of triple super phosphate (TSP) (N:P2O5:K ratio = 0:45:0) were supplemented to the cowpeas 4 weeks after planting. Plots used for the BNF study were intercropped with the WE1101 maize variety, a water efficient hybrid first released in Kenya in 2013 (AATF, 2014). The cowpea variety was the M66 (Machakos 66) a local, bushy semi-spreading plant with an indeterminate growth habit, sown both for leaves and for grain, which flowers within 55-60 days and matures within 80-90 days. No inoculation was performed to the cowpea seeds as the study relied on soil’s indigenous rhizobia populations. Both the maize and cowpea plants were sown on the 8th of April, cowpea was harvested on the 3rd of July (87 days) while maize on the 1st of September (146 days) (Figure 1).
Plant sampling
Plants for biomass and N accumulation were assessed when 50% of the cowpea plants reached the 50% flowering stage, and at cowpea’s harvest stage. Flowering stage was defined as when cowpea plants had a least half of their flowers developed and open, whereas harvest stage was defined as physiological maturity, when plant pods were dried. Ten cowpeas and four maize plants per plot were harvested at each stage. Sampling was performed by measuring the total length of the cropped rows which was then divided by the number of crops which were to be taken; this resulted in the collection of one cowpea plant every 3.6 m and one maize plant every 9 m. These plants were excavated by selecting a radius of 25 cm around and below the designated plant. Digging was done both with spades and manually so to remove excess soil and carefully recover as many roots as possible. At flowering stage cowpea plants were separated into leaves, stems, flowers, pods, roots, and nodules while at harvest, cowpea plants were separated into leaves, stems, pods, grain, and roots. At both sampling stages cowpea plant parameters were recorded namely: stem length (measured from 1 cm off the ground up to the upper most part), root length, as well as number of leaves, flowers, pods, and grains. Plant parts were distinguished in the field, washed thoroughly with clean water, and placed in paper bags of known weight. Plant parts were then placed in the oven at 65°C for 48 hours and dry weights were recorded at the ICRAF soil laboratories, Nairobi. All material was then grinded to pass through a 2 mm mesh stored in a dry room in plastic bags and ball milled at the University of Hohenheim.
Litterfall collection
Plastic nets were laid on the soil to cover the area of 100 cm × 120 cm around eight adjacent cowpea plants so to recover litterfall these plants may have produced. Nets were placed 6 weeks after planting and kept until harvest. Litterfall was collected weekly starting 44 days after planting (DAP) and twice a week from 63 DAP onwards. The material was washed thoroughly, placed to dry at 65°C for 48 hours in the oven and dry weight was then recorded. Pseudo replicates within each plot were then bulked and grinded to pass through a 2mm sieve before further analysis.
Determination of soil nitrate and ammonium
Soil sampling was performed by using an auger to collect samples at 4 different depths (0-5cm, 5-15cm, 15-30 and 30-50cm). Sampling was performed at 4 locations within each plot; in intercrop plots this was done once between rows of cowpeas, once between rows of maize and twice between rows of maize and cowpea, while in monocrop plots this was done at 4 pre-established locations which were maintained constant across the plots. Extracts were immediately placed in polyethene bags and in cool boxes filled with ice to prevent denitrification. The soil filtrate was hence analysed for NH4-N and N03-N on an autoanalyzer and using the procedures described by (Anderson and Ingram, 1993).
Natural abundance of N isotopes
Determination of the 15N natural abundance was conducted at Hohenheim University, Germany using a Euro Elemental Analyzer coupled to a Finnigan Delta IRMS (Thermo Scientific, Germany). 1.5 ± 0.15 mg of cowpea plant material, 2.5mg ± 0.25 of maize plant material or 20 mg ± 2 of soil material were placed in aluminium foil caps, carefully folded prepared for analysis.
The δ15N difference is usually small but able to be measured precisely and calculated using the following equation (Shearer and Kohl, 1986):
δ15N (‰) =
where the ‘sample’ refers to the experimental sample and the ‘standard’ is atmospheric 15N2 abundance (0.3663%). R is the ratio of the concentration of 15N to the total N in the sample under investigation.
Weighted plant δ15N mean was then calculated using the following formula:
Weighted mean δ15N =
Where PNacc stands for individual plant Part N accumulation, Pd15N is the individual plant part’s δ15N and Σ in the numerator indicates the sum of the individual plant parts N accumulated*its δ15N while at the denominator indicates the sum of N accumulated by the individual plant parts.
The percentage of cowpea’s N derived from atmospheric N2 (% Ndfa) was calculated according to Unkovich et al. (2008):
% Ndfa =
Where δ15N maize is the whole maize plant δ15N weighted mean and δ 15N cowpea is the whole plant δ15N weighted mean. B is the whole cowpea δ15N value when totally dependent on biological nitrogen fixation (BNF) as source of N acquisition (Shearer and Kohl, 1991). The B value of -0.633 ‰ (Unkovich et al., 2008) for the whole plant was obtained from literature glasshouse experiments from cowpea plants entirely relying on BNF, and which was used for %Ndfa calculations at both flowering and harvest stages.
Total N accumulated by cowpea was calculated as follows:
Total N accumulated=
With total N accumulated being the sum of individual parts. Where %legume total N was the overall plant’s N content while the biomass the sum of the individual plant parts.
The amount of fixed N was calculated as follows:
N2-fixed (kg ha ¯¹ ) =
Statistical analysis
Measured data (Ndfa, nodule counts) were analysed through a generalised mixed model (GLMM) using tillage method (till or no till) and cropping system (inter or monocrop) as fixed effects while blocks (1, 2, 3) were included as random effects. Maize and cowpea grain yields were subjected to the Shapiro-Wilk normality test (Shapiro and Wilk, 1965). The data did not satisfy the assumption of normality and were thus log-transformed before analysis. The log-transformed data exhibited homogenous variance (p<0.05) as confirmed by the Bartlett’s test (Snedecor and Cochran, 1989). The generalized linear model (GLM) was fitted by REML option using the R-package ade4v. 1.7-19 in R-Studio Version 0.99.892 (RStudio, 2022). The standard error of difference between means was calculated using the procedure described by (Saville, 2003).
Results
Soil mineral nitrogen
There were significant differences in the amounts of nitrate and ammonium in the topsoil with lower NH4+ concentrations in CT monocrop systems both at the 0-5 and 5-15 layer compared to CT intercrop trails (Table 1). There were no significant differences in the amounts of nitrate and ammonium at lower soil strata (15-50 cm) among the different cropping systems Depth and nutrient concentration were inversely correlated with the following averages reported from top (0-5 cm) to bottom soil (30 – 50 cm) 4.9, 4.1, 3.2 and 3.0 mg kg-¹. Across each depth, between 57% and 61% of the Nmin was found in the form of nitrate.
At flowering stage, cowpea plants from CT intercrop plots reported significantly longer stems (99 cm) compared to CA and CT monocrop plots (72 - 71 cm) with CA intercrops being in between such values (81 cm) (Table 2). Recovered root length varied between 21 (CT monocrop) and 24 cm (CT intercrop). Peduncles number was between 3 and 5 across treatments. Number of leaves was between 47 in CA intercrop and 63 in CA monocrop plots. An average of 7 flowers per plant was reported in CT intercrop trails, 6 across the monocropped ones and 5 in CA intercrop, although these were not statistically different. At harvest stage stem length varied between 109 and 119 cm in CA intercrop and CT monocrop plants. Root length varied between 19 (CT monocrop) and 24 cm (CA intercrop). Monocrop CA and CT reported significantly greater amounts of pods compared to CT intercrop as well as CA monocrop (20 pods per plant) outperforming CA intercrop (11 pods per plant). CA monocrop also reported the greatest number of leaves (p<0.05) compared to CT treatments. Pod length varied between 16 and 17 cm (p<0.05). CA intercrops had the highest number of seeds per pod (15) while CT intercrop and CA monocrop the lowest (13) (p<0.05). Nevertheless, plants from monocrop plots reported the greatest number of seeds per plant.
At flowering, peak dry matter production across all plant parts between CA and CT intercrops was not significantly different and yielded a total of between 848.8 and 1045.1 kg ha-1 dry matter respectively (Table 3). Belowground biomass (roots + nodules) accumulated 61.2 and 78.2 kg ha-1 or 7.8 and 8.1 % of total biomass in CA and CT intercrops respectively. In monocrop plots no significant difference between CA and CT biomass accumulation was reported either. Total biomass in at flowering CA monocrop accounted for 3282 kg ha-¹ while CT monocrop 2867 kg ha-¹. Belowground biomass accounted for 6.1 and 6.8 % of total dry matter accumulation for CA and CT monocrop plants respectively. At harvest stage CT intercrop reported a significantly higher grain (590 kg ha-¹), compared with CA intercrop systems (472 kg ha-1). At harvest time, the total biomass measured was significantly less than biomass measured at flowering time due to litterfall. In monocrop systems, a significant increase in pod dry weight which led to significantly higher of 1465 kg ha-1 for CA and 1618 kg ha-1 for CT. Total biomass production was 1874.4 and 1463.9 kg ha-¹, with grains accounting for 46.3 and 50.4%, for CA and CT monocrop respectively. The total seasonal cowpea litterfall was low in intercrops with CA plot recording only 264 kg ha-¹and 408 kg ha-¹in the corresponding CT plot (Figure 2). The monocropping treatments had the largest litterfall driven by the high biomass production. The CA plot under intercropping recorded 994 kg ha-¹while the corresponding CT treatment recorded 1217 kg ha-¹.
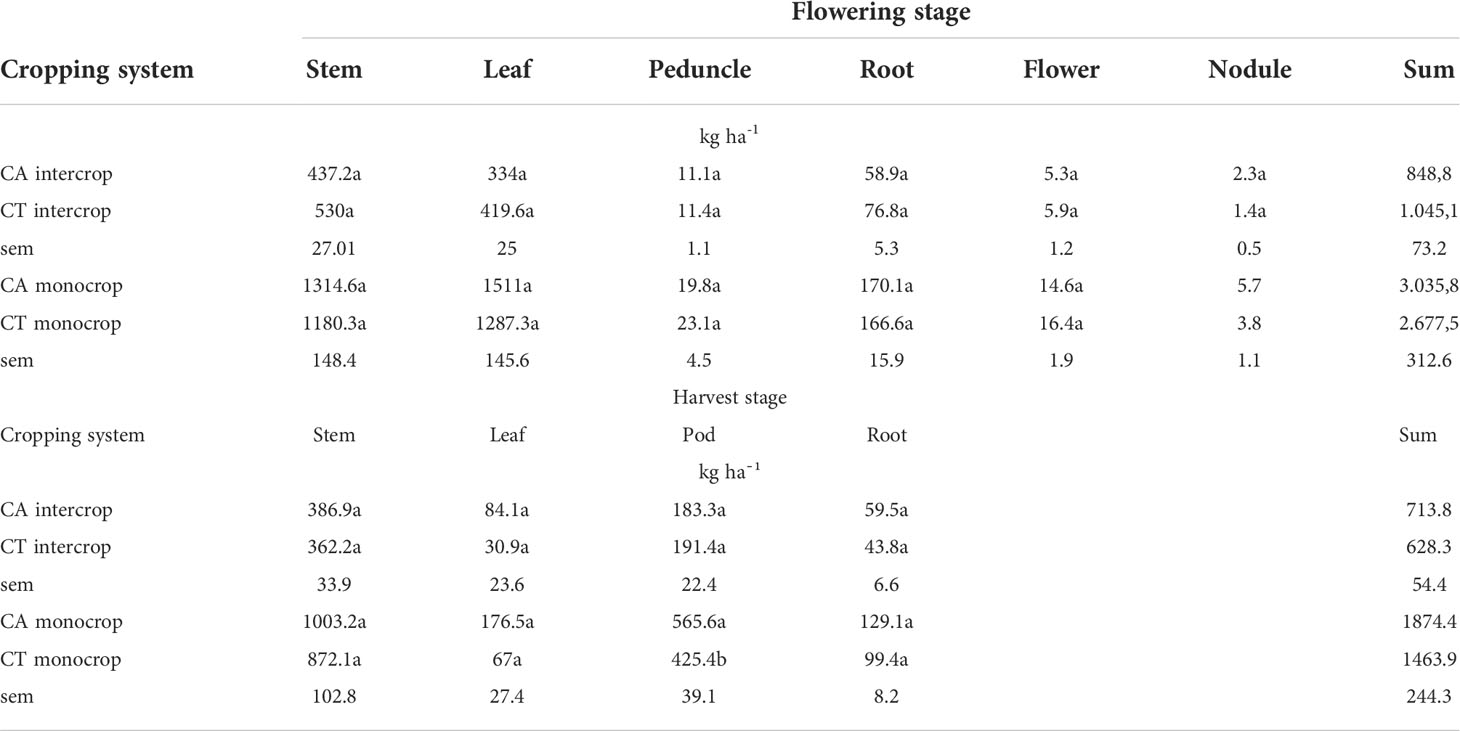
Table 3 Cowpea biomass accumulation in different plant parts at flowering and harvest stage as affected by cropping system.
Amount of N2 fixed
At harvest stage no significant differences were found between plant parts and cropping systems and the overall weighted N mean varied between 2.4 and 2.6 %. Weighted δ15N means varied between 1.5 in CT monocrop and 2.4 ‰ in CT intercrop (Table 4). Weighted means varied between 0.9 in CA intercrop and 2.9 ‰ in CT intercrop and lower (p<0.05) at flowering compared to harvest stage ). The total amount of N fixed was 94.6 kg with monocropping under CA and was lower at 83.5 kg with CT (Figures 3, 4). Intercropping significantly reduced the amounts of fixed N to 31.5 kg and 27.0 kg for CA and CT respectively (Figure 4).
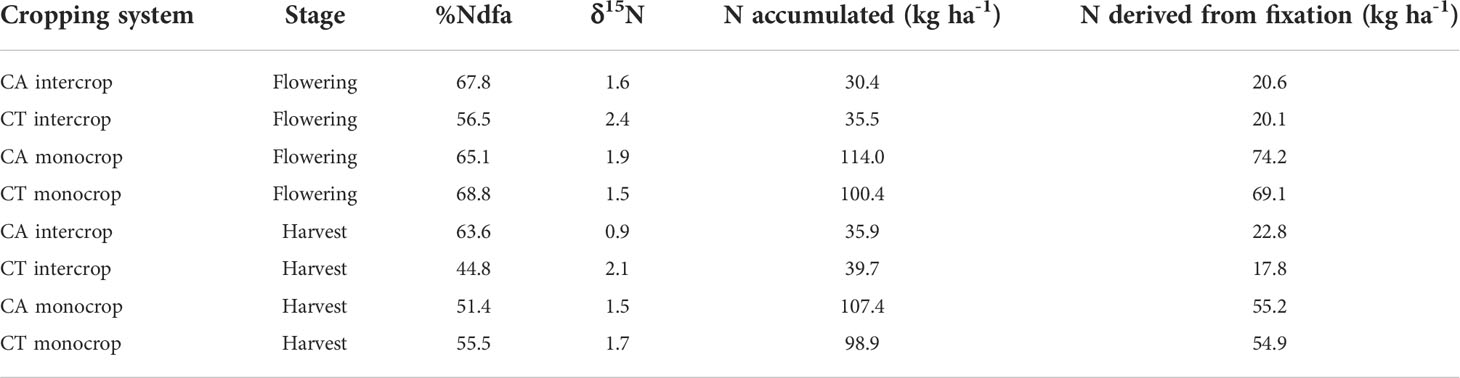
Table 4 %Ndfa, δ15N, N accumulated (kg ha‐1) and N derived from fixation (kg ha‐1) for cowpea plants under various cropping systems.
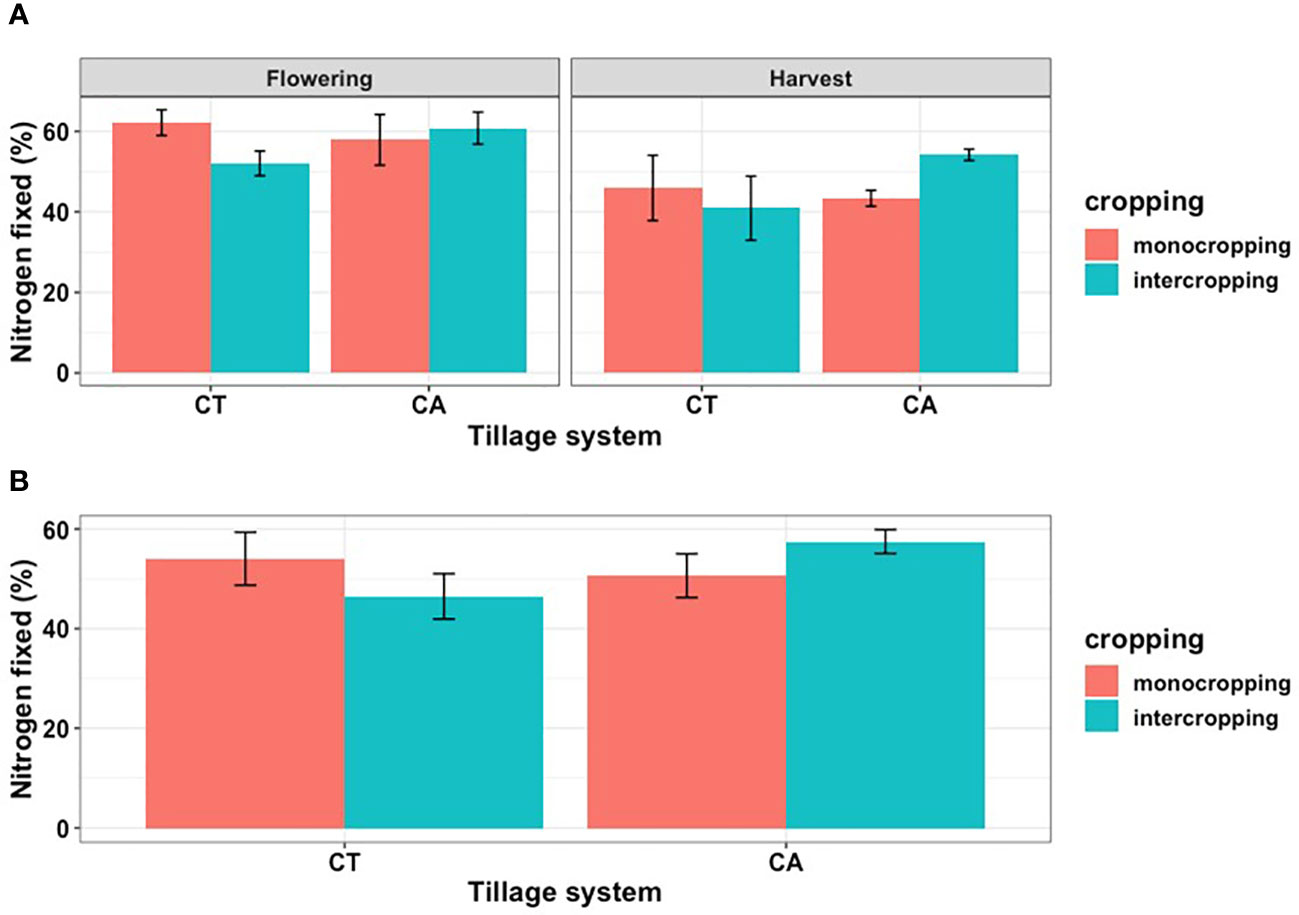
Figure 3 The percentages of N fixed as affected by cropping system, (A) at different sampling times, and (B) the overall.
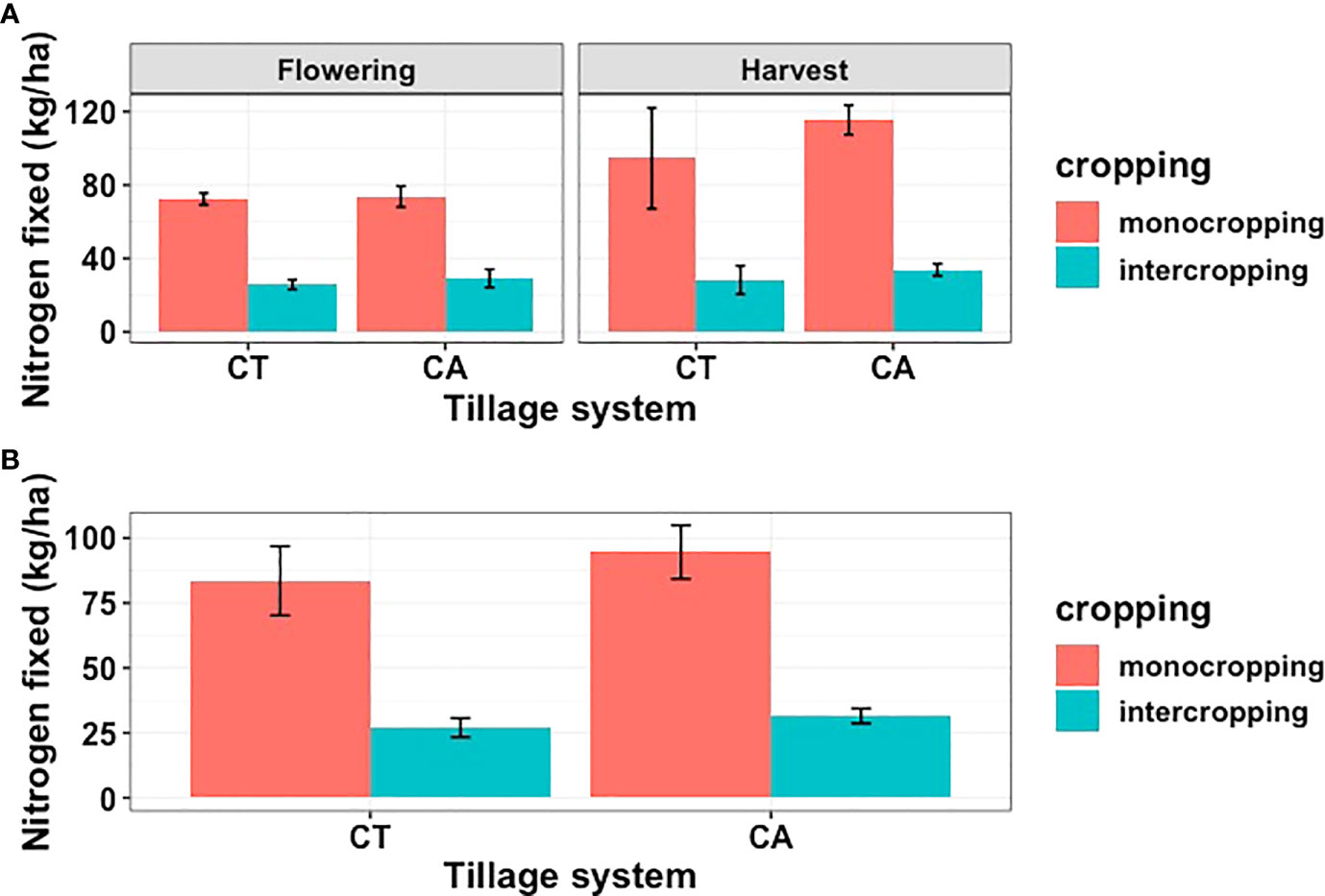
Figure 4 The amount of N fixed in kilogrammes per hectare as affected by cropping system, (A) at different sampling times, and (B) the overall.
Discussion
The study found only weak evidence that nodulation (number of nodules) was improved under CA management compared to CT as the differences were not statistically significant. Reduced tillage has been reported to enhance rhizobia populations in western Kenya (Omondi et al., 2014). In general, CA improves significantly soil microbiological activity in the long-term (Henneron et al., 2014; Habig and Swanepoel, 2015), and such outcome in combination with the promiscuous nature of cowpea meant that BNF was likely enhanced in CA plots. Additionally, improved moisture conditions, as generally observed under CA management, can also be important in enhancing BNF. For example, Guimarães et al. (2015) tested nodulation response against soil water availability and found that highest nodulation between 60 and 80% water availability, significantly reduced outside such spectra probably due to lack of aerobic conditions in clayey soils. Thus, an improve drainage could partly explain the trend to a higher nodulation under CA systems.
Nodulation occurs at early stages of plant growth since root exudates progressively lose their susceptibility to bacterial infection. In the field, small nodules can be seen starting 2-3 weeks after planting. Nodule activity will peak at peak plant biomass production to decrease as nitrogen is allocated to the grain. Belane and Dakora (2009) tested 30 cowpea genotypes and reported overall nodule numbers to be between 6 and 85, although deviations from such ranges are found in literature (Karikari et al., 2015). Our results showed that the nodulation process was successful with a as high as 74 nodules per plant in the CA treatment. Significant increases in number of nodules with increasing application of P have been widely reported (Wu and Arima, 1992; Phares et al., 2020; Mahato et al., 2021; Khan et al., 2022). While P and K were not limiting factors in Kiboko (P between 12.3 and 22.9 mg kg soil while K ranged between 261.2 and 355.9 mg kg soil) the addition of 18 kg ha‐1 of Nmin and 46 kg ha of P at soil bed preparation for maize plants might have enhanced rhizobia populations in intercrops. Also, the application of P at 6.8 kg/ha 4 weeks after planting to cowpea plants may have resulted in improving plant metabolic processes as well as the symbiosis. Nodules in intercropping systems had a significantly higher amount of N that has been related to greater N2 fixation activity. Nevertheless, the higher %N did not translate into significantly lower δ15N abundance in the nodules. Nodulation was more pronounced in plants from CA treatments, which at flowering stage reported a higher (p<0.05) % of N especially in the stem and in the root.
Cowpea is a promiscuous legume due to the large number of genera of rhizobia bacteria that can establish root nodules (Ndungu et al., 2018) as well as non-rhizobia endophytes which interact within the nodules for secondary but important chemical pathways such as phosphate solubilisation (Marciano et al., 2012). The promiscuous nature of cowpea could have led to cowpea to respond well to inoculation in the field (Giller, 2001). The advantage of this phenomena is that it can be cropped without inoculants or biofertilizers since some native bacterial strains are more adapted to the specific soil conditions than introduced ones. In this study nodulation with indigenous bacteria was successful as high numbers of between 30 and up to 100 nodules were reported in some plant without inoculation. Kyei-Boahen et al. (2017) reported that that the number of nodules in treatments without rhizobia inoculation across various Kenyan locations to be between 8 and 15 while inoculation doubled numbers and led to increased grain yields.
In this paper, we reported similar %Ndfa values in monocrops and intercrop systems like those reported Kihara et al. (2011). The same authors also did not find differences in %Ndfa between CA and CT intercrop systems with overall averages between 56.5 and 68.8%. The major assumption with mixed cropping systems is that the intercropping competition will result in an increase in BNF by stimulating the sparring effect of legumes (Franke et al., 2018) and allow the non-legume to utilize N from the soil. However, this seems not to have been the case in our experiment. We found rather a trend to have higher mineral N availability in the intercrop (Table 1), probably due to the longer-term benefits of the inputs of fixed N, which by the time we performed our measurements (year 7) reduced the need of the legume to enhance N2 fixation in competition with maize. Additionally, the MBILI system with double rows might have reduced the direct root competition or sparing effects compared to more close interactions in single row systems. This intercropping MBILI system’s reduced competition might have led to the non-significant effect of cropping systems on the cowpea’s BNF capacity across the tillage treatments. Furthermore, Vance and Heichel (1981) proved an inversely proportional relationship between nodulation and N03 availability, confirming that cowpea will allocate fewer resources to root and nodule development (and ultimately N2 fixation) when mineral N is abundant. The intercropping design of two maize to alternate with two legume rows referred to as the MBILI system (Mucheru-Muna et al., 2009), also enhances light interception by the understorey legume leading to reduced light competition and larger legume productivity. A larger legume productivity however would result in an increase in %Nfa and amount of N2 fixed (Rusinamhodzi et al., 2006; Rusinamhodzi et al., 2012).
There are several areas we identified in our study that may need improvements in the future. Our results are based on measurements carried out in one season although the field experiment had been going on for seven years. Due to many confounding factors in field trials and their subtle interactions, repeated measurements across seasons would have contributed to more robust results even though we do not assume that could have changed the major findings. Following more closely N dynamics over season in the different systems could further strengthen our arguments on the impact of the MBILI system and the recycling of fixed N on BNF in the intercropping systems.
Conclusion
The objective of this paper was to assess the effect of conservation practices including maize-cowpea intercropping on cowpea’s legume fixing capacity. The effect of CA on the biological nitrogen fixation process (BNF) positive although it was not significantly different from the conventional tillage treatment. The cropping system (sole vs intercropping) was significant, the amount of BNF was directly related to total biomass production which was larger in sole crops that intercrops. These results suggest that CA has the potential to enhance the BNF process but there is a need to explore in future alternative spatial arrangement in intercropping between short duration cowpea and maize to reduce competition, enhance cowpea productivity and optimize the BNF process.
Data availability statement
The raw data supporting the conclusions of this article will be made available by the authors, without undue reservation.
Author contributions
FB - data collection and analysis, manuscript write-up LR - designed the experiment, data visualisation, manuscript write-up GC- conceptualized the study, manuscript edits. All authors contributed to the article and approved the submitted version.
Funding
This study was made possible through the project on Sustainable Intensification of Maize-Legume Systems for Food Security in Eastern and Southern Africa - SIMLESA funded by Australian Centre for International Agricultural Research (ACIAR) through a grant to CIMMYT (CSE/2009/024 variation 3), and also by the Drought Tolerant Maize for Africa (DTMA) supported by the Bill and Melinda Gates Foundation (BMGF, grant OPPGD1390) and CGIAR Research Program on Maize (CRP MAIZE). Ferdinando Binacchi acknowledges the Fiat Panis Foundation for granting him a Dr. Hermann Eiselen Scholarship for his time abroad.
Acknowledgments
This study was made possible through the project on Sustainable Intensification of Maize-Legume Systems for Food Security in Eastern and Southern Africa - SIMLESA funded by Australian Centre for International Agricultural Research (ACIAR) through a grant to CIMMYT (CSE/2009/024 variation 3), and also by the Drought Tolerant Maize for Africa (DTMA) supported by the Bill and Melinda Gates Foundation (BMGF, grant OPPGD1390) and CGIAR Research Program on Maize (CRP MAIZE). We are grateful to George Oriyo for managing the long-term trial as well as data collection.
Conflict of interest
The authors declare that the research was conducted in the absence of any commercial or financial relationships that could be construed as a potential conflict of interest.
Publisher’s note
All claims expressed in this article are solely those of the authors and do not necessarily represent those of their affiliated organizations, or those of the publisher, the editors and the reviewers. Any product that may be evaluated in this article, or claim that may be made by its manufacturer, is not guaranteed or endorsed by the publisher.
References
Anderson J. M., Ingram J. S. I. (1993). Tropical soil biology and fertility: A handbook of methods (Wallingford, UK: CAB International).
Aryal A., Devkota A. K., Aryal K., Mahato M. (2021). Effect of different levels of phosphorus on growth and yield of Cowpea varieties in Dang, Nepal. J. Agric. Natural Resour. 4 (1), 62–78. doi: 10.3126/janr.v4i1.33228
Ayuke F. O., Kihara J., Ayaga G., Micheni A. N. (2019). Conservation agriculture enhances soil fauna richness and abundance in low input systems: Examples from Kenya. Front. Environ. Sci. 7. doi: 10.3389/fenvs.2019.00097
Babal B., Phogat V. K., Sharma M. K., Ahlawat S. (2021). Impact of long-term conservation agriculture induced changes in soil properties on persistence of pendimethalin under different cropping systems. Soil Res. 59 (3), 299–308. doi: 10.1071/SR20122
Baudron F., Jaleta M., Okitoi O., Tegegn A. (2014). Conservation agriculture in African mixed crop-livestock systems: Expanding the niche. Agriculture Ecosyst. Environ. 187 (0), 171–182. doi: 10.1016/j.agee.2013.08.020
Belane A. K., Dakora F. D. (2009). ). measurement of N2 fixation in 30 cowpea (Vigna unguiculata l. walp.) genotypes under field conditions in Ghana, using the15N natural abundance technique. Symbiosis 48 (1), 47–56. doi: 10.1007/s00374-009-0415-6
Chivenge P. P., Murwira H. K., Giller K. E., Mapfumo P., Six J. (2007). Long-term impact of reduced tillage and residue management on soil carbon stabilization: Implications for conservation agriculture on contrasting soils. Soil Tillage Res. 94, 328–337. doi: 10.1016/j.still.2006.08.006
Dakora F. D., Belane A. K. (2019). Evaluation of protein and micronutrient levels in edible cowpea (Vigna unguiculata l. walp.) leaves and seeds. Front. Sustain. Food Syst. 3. doi: 10.3389/fsufs.2019.00070
FAO (2008). Available at: http://www.fao.org/ag/ca/
Franke A. C., van den Brand G. J., Vanlauwe B., Giller K. E. (2018). Sustainable intensification through rotations with grain legumes in Sub-Saharan Africa: A review. Agriculture Ecosyst. Environ. 261, 172–185. doi: 10.1016/j.agee.2017.09.029
Gentile R., Vanlauwe B., Chivenge P., Six J. (2008). Interactive effects from combining fertilizer and organic residue inputs on nitrogen transformations. Soil Biol. Biochem. 40 (9), 2375–2384. doi: 10.1016/j.soilbio.2008.05.018
Guimarães L. S., Bonfim-Silva E. M., Fornazier Moreira J. C., Kamila Bosa C., Soares da Silva S. L., Araújo da Silva T. J. (2015). Effects of inoculation of rhizobium on nodulation and nitrogen accumulation in cowpea subjected to water availabilities. Am. J. Plant Sci. 06 (09), 1378–1384. doi: 10.4236/ajps.2015.69137
Habig J., Swanepoel C. (2015). Effects of conservation agriculture and fertilization on soil microbial diversity and activity. Environments 2 (4), 358–384. doi: 10.3390/environments2030358
Henneron L., Bernard L., Hedde M., Pelosi C., Villenave C., Chenu C., et al. (2014). Fourteen years of evidence for positive effects of conservation agriculture and organic farming on soil life. Agron. Sustain. Dev. 35 (1), 169–181. doi: 10.1007/s13593-014-0215-8
Karikari B., Arkorful E., Addy S. (2015). Growth, nodulation and yield response of cowpea to phosphorus fertilizer application in Ghana. J. Agron. 14 (4), 234–240. doi: 10.3923/ja.2015.234.240
Kaumbutho P., Kienzle J., Centre de Coopération Internationale de Recherche Agronomique pour le Développement (CIRAD), Food and Agriculture Organization of the United Nations (FAO) (2007). “Conservation agriculture as practised in Kenya: two case studies,” in Conservation agriculture in Africa (Nairobi, Paris, Rome: African Conservation Tillage Network (ACT).
Kebede E., Bekeko Z., Tejada Moral M. (2020). Expounding the production and importance of cowpea (Vigna unguiculata (L.) walp.) in Ethiopia. Cogent Food Agric. 6 (1). doi: 10.1080/23311932.2020.1769805
Khan H., Akbar W. A., Shah Z., Rahim H. U., Taj A., Alatalo J. M. (2022). Coupling phosphate-solubilizing bacteria (PSB) with inorganic phosphorus fertilizer improves mungbean (Vigna radiata) phosphorus acquisition, nitrogen fixation, and yield in alkaline-calcareous soil. Heliyon 8 (3), e09081. doi: 10.1016/j.heliyon.2022.e09081
Kihara J., Martius C., Bationo A., Vlek P. L. G. (2011). Effects of tillage and crop residue application on soybean nitrogen fixation in a tropical ferralsol. Agriculture 1 (1), 22–37. doi: 10.3390/agriculture1010022
Kyei-Boahen S., Savala C. E. N., Chikoye D., Abaidoo R. (2017). Growth and yield responses of cowpea to inoculation and phosphorus fertilization in different environments. Front. Plant Sci. 8, 646. doi: 10.3389/fpls.2017.00646
Marciano M. L., Fonsêca Sousa Soares C. R., de Oliveira S. M., Avelar Ferreira P. A., Lima Soares B., de Fráguas Carvalho R., et al. (2012). Biological nitrogen fixation and phosphate solubilization by bacteria isolated from tropical soils. Plant Soil 357 (1-2), 289–307. doi: 10.1007/s11104-012-1157-z
Mgolozeli S., Nciizah A. D., Wakindiki I. I. C., Mudau F. N. (2020). Innovative pro-smallholder farmers’ permanent mulch for better soil quality and food security under conservation agriculture. Agronomy 10, 605. doi: 10.3390/agronomy10040605
Miki T., Ushio M., Fukui S., Kondoh M. (2010). Functional diversity of microbial decomposers facilitates plant coexistence in a plant–microbe–soil feedback model. Proc. Natl. Acad. Sci. 107 (32), 14251–14256. doi: 10.1073/pnas.0914281107
Mucheru-Muna M., Pypers P., Mugendi D., Kung'u J., Mugwe J., Merckx R., et al. (2009). A staggered maize-legume intercrop arrangement robustly increases crop yields and economic returns in the highlands of central Kenya. Field Crops Res. 115 (2), 132–139. doi: 10.1016/j.fcr.2009.10.013
Mugwe J., Ngetich F., Otieno E. O. (2019). Integrated Soil Fertility Management in Sub-Saharan Africa: Evolving Paradigms Toward Integration. In: Leal Filho W., Azul A., Brandli L., Özuyar P., Wall T.(eds) Zero Hunger. Encyclopedia of the UN Sustainable Development Goals. Springer, Cham, Switzerland. doi: 10.1007/978-3-319-69626-3_71-1
Mupangwa W., Twomlow S., Walker S. (2012). Reduced tillage, mulching and rotational effects on maize (Zea mays l.), cowpea (Vigna unguiculata (Walp) l.) and sorghum (Sorghum bicolor l. (Moench)) yields under semi-arid conditions. Field Crops Res. 132 (0), 139–148. doi: 10.1016/j.fcr.2012.02.020
Ndungu S. M., Messmer M. M., Ziegler D., Gamper H. A., Mészáros al.,,É., Thuita M., et al. (2018). Cowpea (Vigna unguiculata l. walp) hosts several widespread bradyrhizobial root nodule symbionts across contrasting agro-ecological production areas in Kenya. Agriculture Ecosyst. Environ. 261, 161–171. doi: 10.1016/j.agee.2017.12.014
Omondi O. J., Mungai N. W., Ouma J. P., Baijukya F. P. (2014). Effect of tillage on biological nitrogen fixation and yield of soybean (Glycine max l. merril) varieties. Aust. J. Crop Sci. 8 (8), 1140–1146.
Phares C. A., Atiah K., Frimpong K. A., Danquah A., Asare A. T., Aggor-Woananu S. (2020). Application of biochar and inorganic phosphorus fertilizer influenced rhizosphere soil characteristics, nodule formation and phytoconstituents of cowpea grown on tropical soil. Heliyon 6 (10), e05255. doi: 10.1016/j.heliyon.2020.e05255
Pittelkow C. M., Linquist B. A., Lundy M. E., Liang X., van Groenigen K. J., Lee J., et al. (2015). When does no-till yield more? a global meta-analysis. Field Crops Res. 183, 156–168. doi: 10.1016/j.fcr.2015.07.020
Pretty J., Toulmin C., Williams S. (2011). Sustainable intensification in African agriculture. Int. J. Agric. sustainability 9 (1), 5–24. doi: 10.3763/ijas.2010.0583
RStudio Team. (2022). RStudio: Integrated Development for R. RStudio, PBC, Boston, MA. Available at: http://www.rstudio.com/.
Rusinamhodzi L., Corbeels M., Nyamangara J., Giller K. E. (2012). Maize-grain legume intercropping is an attractive option for ecological intensification that reduces climatic risk for smallholder farmers in central Mozambique. Field Crops Res. 136, 12–22. doi: 10.1016/j.fcr.2012.07.014
Rusinamhodzi L., Corbeels M., Van Wijk M. T., Rufino M. C., Nyamangara J., Giller K. E. (2011). A meta-analysis of long-term effects of conservation agriculture on maize grain yield under rain-fed conditions. Agron. Sustain. Dev. 31 (4), 657–673. doi: 10.1007/s13593-011-0040-2
Rusinamhodzi L., Murwira H. K., Nyamangara J. (2006). Cotton-cowpea intercropping and its N2 fixation capacity improves yield of a subsequent maize crop under Zimbabwean rain-fed conditions. Plant Soil 287 (1-2), 327–336. doi: 10.1007/s11104-006-9080-9
Sanginga N., Woomer P. L. (2009). Integrated soil fertility management in Africa: Principles, practices and developmental process (Nairobi, Kenya: CIAT).
Saville D. J. (2003). Basic statistics and the inconsistency of multiple comparison procedures. Can. J. Exp. Psychol. 57 (3), 167–175. doi: 10.1037/h0087423
Shapiro S. S., Wilk M. B. (1965). An analysis of variance test for normality (Complete samples). Biometrika 52(3–4), 591–611. doi: 10.1093/biomet/52.3-4.591
Shearer G. B., Kohl D. H. (1986). N2-fixation in field settings: Estimations based on natural 15N abundance. Aust. J. Plant Physiol. 13, 699–756. doi: 10.1071/PP9860699
Shearer G. B., Kohl D. H. (1990). The 15 N natural abundance method for measuring biological nitrogen fixation: Practicalities and possibilities. In Stable Isotopes in Plant Nutrition, Soil Fertility and Environmental Studies Proceedings of a International Symposium on the use of Stable Isotopes in Plant Nutrition, Soil Fertility and Environmental Studies. IAEA. pp 103–115.
Steward P. R., Dougill A. J., Thierfelder C., Pittelkow C. M., Stringer L. C., Kudzala M., et al. (2018). The adaptive capacity of maize-based conservation agriculture systems to climate stress in tropical and subtropical environments: A meta-regression of yields. Agriculture Ecosyst. Environ. 251, 194–202. doi: 10.1016/j.agee.2017.09.019
Trenbath B. R. (1986). Resource use in intercrops. In Francis C. A. (Ed.). Multiple Cropping Systems (New York, Macmillan), 57–81.
Unkovich M., Herridge D., Peoples M., Cadisch G., Boddey R., Giller K. E., et al. (2008). Measuring plant-associated nitrogen fixation in agricultural systems (Canberra, Australia: ACIAR), 258.
Vance C. P., Heichel G. H. (1981). Nitrate assimilation during vegetative regrowth of alfalfa. Plant Physiol. 68 (5), 1052–1057. doi: 10.1104/pp.68.5.1052
Van Hulst F. J., Posthumus H. (2016). Understanding (non-) adoption of conservation agriculture in Kenya using the reasoned action approach. Land Use Policy 56, 303–314. doi: 10.1016/j.landusepol.2016.03.002
Vanlauwe B., Bationo A., Chianu J., Giller K. E., Merckx R., Mokwunye U., et al. (2010). Integrated soil fertility management: Operational definition and consequences for implementation and dissemination. Outlook Agric. 39 (1), 17–24. doi: 10.5367/000000010791169998
Keywords: sustainable intensification, smallholder farmers, conservation agriculture, biological nitrogen fixation, cropping systems
Citation: Binacchi F, Rusinamhodzi L and Cadisch G (2022) The potential of conservation agriculture to improve nitrogen fixation in cowpea under the semi-arid conditions of Kenya. Front. Agron. 4:988090. doi: 10.3389/fagro.2022.988090
Received: 06 July 2022; Accepted: 20 September 2022;
Published: 07 October 2022.
Edited by:
Lydiah Gatere, FAO Silva Mediterranea, ItalyReviewed by:
Cosmas Parwada, Zimbabwe Open University, ZimbabweEsther Nyaradzo Masvaya, Marondera University of Agricultural Sciences and Technology (MUAST), Zimbabwe
Copyright © 2022 Binacchi, Rusinamhodzi and Cadisch. This is an open-access article distributed under the terms of the Creative Commons Attribution License (CC BY). The use, distribution or reproduction in other forums is permitted, provided the original author(s) and the copyright owner(s) are credited and that the original publication in this journal is cited, in accordance with accepted academic practice. No use, distribution or reproduction is permitted which does not comply with these terms.
*Correspondence: Leonard Rusinamhodzi, TC5SdXNpbmFtaG9kemlAY2dpYXIub3Jn