- Université de Lorraine, INRAE, IAM, Nancy, France
Pucciniales are fungal pathogens of plants that cause devastating rust diseases in agriculture. Chemically-synthesized pesticides help farmers to control rust epidemics, but governing bodies aim at limiting their use over the next decade. Defense peptides with antimicrobial activities may help to innovate a next generation of phytosanitary products for sustainable crop protection. This review comprehensively inventories the proteins or peptides exhibiting a biochemically-demonstrated antifungal activity toward Pucciniales (i.e., anti-rust proteins or peptides; hereafter ‘ARPs’), and also analyses the bioassays used to characterize them. In total, the review scrutinizes sixteen publications, which collectively report 35 ARPs. These studies used either in vitro or in planta bioassays, or a combination of both, to characterize ARPs; mostly by evaluating their ability to inhibit the spore germination process in vitro or to inhibit fungal growth and rust disease development in planta. Also, the manuscript shows that almost no mode of action against rust fungi was elucidated, although some might be inferred from studies performed on other fungi. This short review may serve as a knowledge and methodological basis to inform future studies addressing ARPs.
Rust Fungi Impose a Heavy Pesticide Toll on Agriculture
Rust fungi (Pucciniales) are fast-evolving plant pathogens that can infect key crops and threaten global food security (Aime et al., 2018; Figueroa et al., 2020; Duplessis et al., 2021). Farmers notably rely on the use of chemically-synthesized fungicides to control rust epidemics (Oliver and Hewitt, 2014; Cook et al., 2021). Due to the suspected or proven toxicity of those products, governing bodies aim at rapidly limiting their use in agriculture. For instance, the European Commission “Farm to Fork” (F2F) strategy aims at reducing by 50% the use of chemically-synthesized pesticides by 2030 (European Commission, 2020). Therefore, modern agriculture urgently seeks alternatives to chemically-synthesized pesticides. In this context, research and innovation actors aim at developing new tools and solutions for sustainable crop protection, notably by exploiting both our knowledge of the plant immune system and naturally-occurring antimicrobial molecules (Dangl et al., 2013; Moscou and van Esse, 2017; Schwinges et al., 2019; Chen et al., 2021).
Defense Proteins and Peptides are Effective Microbe Killers
Some proteins or peptides can directly kill microbes; mostly thank to their cationic property which leads to an interaction with negatively charged membranes of microbes (Tam et al., 2015). For instance, plants possess large protein families referred to as ‘pathogenesis-related’ (PR), whose members exhibit consistent antimicrobial activities (Van Loon and Van Strien, 1999; Van Loon et al., 2006). Such proteins and peptides may provide innovators with molecular chassis to develop active substances for the next generation of biopharmaceuticals and phytosanitary products (Haney et al., 2019). In agriculture, optimized and vectorized anti-microbial proteins or peptides may assist the development of biological pesticides (aka biopesticides) (Montesinos et al., 2012; Schwinges et al., 2019; Li et al., 2021). Such amino acid-based biopesticides would have the advantage of being residue-free and less likely to display harmful effects towards consumers and ecosystems (Kumar et al., 2021). In such a context, we need to better understand the diversity of defense proteins and peptides and how they function.
This study aimed at building a knowledge and methodological basis to assist future studies addressing proteins or peptides that exhibit an antifungal activity against rust fungi (i.e., anti-rust peptides or proteins; hereafter ARPs). To this end, we first performed a systematic analysis of the literature to build a comprehensive list of ARPs. Importantly, this analysis considered only the studies that used the exogenous application of a purified ARP on a rust fungus in vitro or in planta to evaluate its anti-rust activity (i.e., direct anti-rust evidence); it thus disregarded the studies that used non-biochemical approaches (e.g., genetic approaches using protein over-expression in planta). Then, we analyzed the bioassays used to characterize ARPs, in order to build a portfolio of methods and approaches that could be used in future studies, and surveyed the limited information available about the ARP modes of action. The review ends by discussing key peptide features that should be considered in future studies.
A Catalog of 35 ARPs with Noticeable Properties
To identify ARPs, we performed a systematic literature survey on the Web of Science by performing searches with combinations of key words such as “exogenous peptide”, “antimicrobial activity”, “Pucciniales”, “rust”, or “inhibition germination”. This survey identified sixteen papers, published between 1996 and 2021 (Table 1). Collectively, these papers explicitly reported 35 different ARPs with an antimicrobial activity towards eleven Pucciniales species (Table 1; Figure 1A). Three rust species served as models in more than three papers: the Asian soybean rust fungus Phakopsora pachyrhizi (Phakosporaceae; five papers, twelve ARPs reported) (Fang et al., 2010; Vasconcelos et al., 2011; Brand et al., 2012; Lacerda et al., 2016; Schwinges et al., 2019), the white pine blister rust fungus Cronartium ribicola (Cronartiaceae; three papers; six ARPs reported) (Jacobi et al., 2000; Zamany et al., 2011; Liu et al., 2021) and the wheat leaf rust fungus Puccinia triticina (Pucciniaceae; three papers; seven ARPs reported) (Barna et al., 2008; Alfred et al., 2013; Wang et al., 2020) (Figure 1A). The eight remaining rust species were addressed in less than two papers, and belong either to the Pucciniaceae or to the Melampsoraceae families (Corrêa et al., 1996; Mathivanan et al., 1998; Rauscher et al., 1999; Dracatos et al., 2014; Petre et al., 2016).
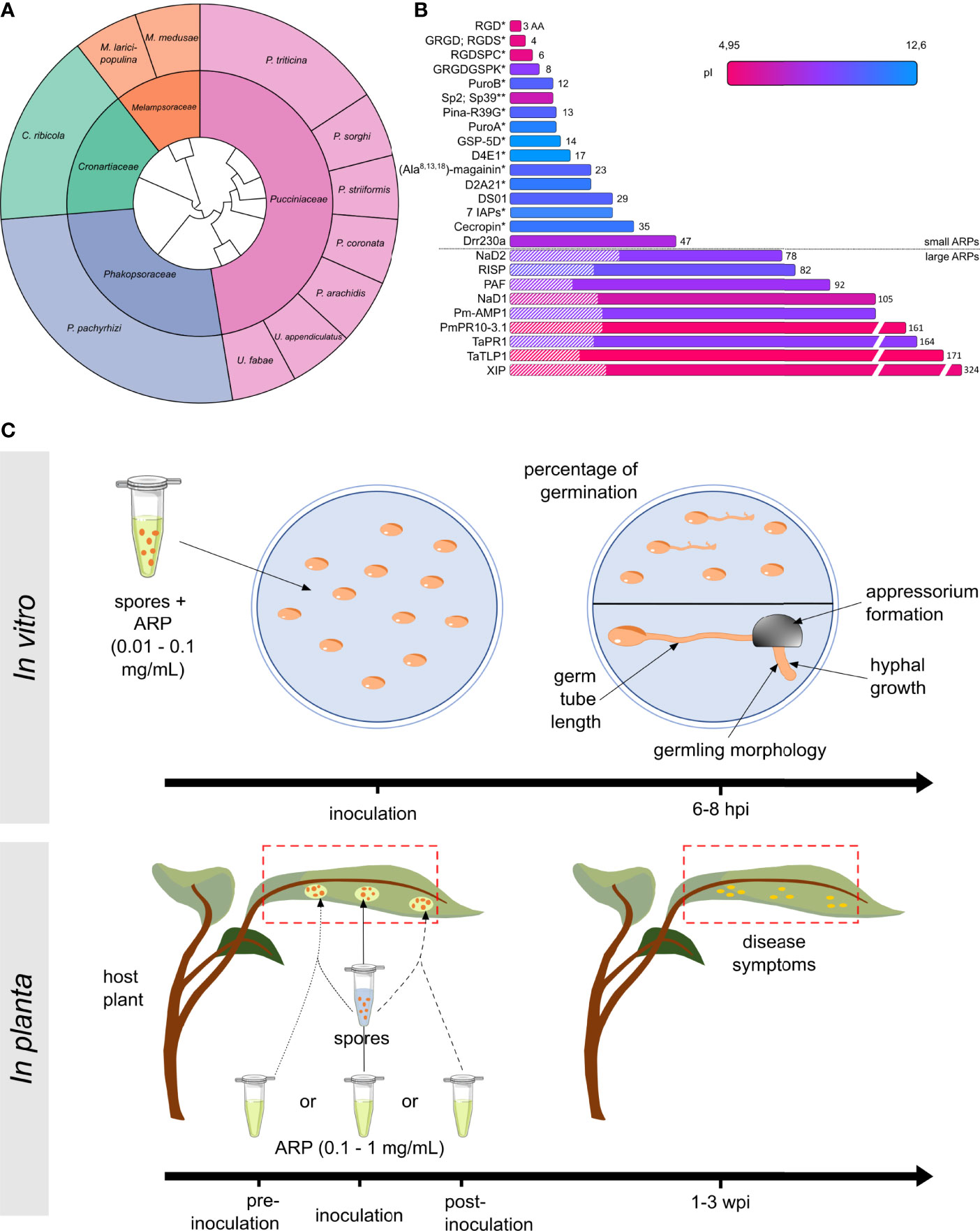
Figure 1 Overview of known anti-rust peptides (ARPs) and bioassays. (A) Sunburst phylogenetic tree of Pucciniales taxa and species used to evaluate ARPs activities. The inner and outer circles indicate Pucciniales families and species, respectively. The genus names are Phakopsora, Cronartium, Melampsora, Puccinia, or Uromyces. (B) Classification of the ARP according to their length in amino acid (aa: linear scale). Predicted isoelectric points (pI: color gradient) and known signal peptides for secretion (hatched area) are indicated. ARPs were i) purified by chromatographic purification from proteinaceous cellular extracts, ii) chemically synthesized or purchased (*), or iii) phage-displayed (**). Amino acid sequences for EC 3.2.1.14 and Pr-1a were not indicated in the original publications. (C) For in vitro bioassays, spores are treated with 0.01 to 0.1 mg/mL purified ARP and spread onto water agar media, and the percentage of germination or the germling morphology and development is assessed 6 to 8 hours post inoculation (hpi) (see Supplementary Figure S1 for more details). For in planta bioassays, host plant (whole plant, detached leaf, or leaf disc) are treated with 0.1 to 1 mg/mL purified ARP prior to inoculation (dotted line), concomitantly with inoculation (solid line), or after inoculation (dashed line). Disease symptoms are assessed using a visual scoring system 1 to 3 weeks post inoculation (wpi). Typically, the scoring system assessed uredinia size, uredinia distribution, necrosis, or chlorosis.
In total, 18 ARPs originate from plants, 10 derive from animals or fungi, and 7 are synthetic peptides (Table 1). Overall, the 35 ARPs grouped into two categories: small and large ARPs; comprising less or more than 50 amino acids, respectively (Figure 1B). ARPs globally display variable predicted isoelectric points, ranging from 4.95 to 12.6. The nine large ARPs carry an N-terminal signal peptide for secretion, and all but two belong to a well-defined plant pathogenesis related (PR) protein family (Van Loon and Van Strien, 1999). Small ARPs were obtained by chemical synthesis (performed in house or by a company to which the peptide was purchased), and large ARPs were obtained via the chromatographic purification of protein extracts from fungal, yeast, or bacterial cultures (Figure 1B).
Among the 35 ARPs, some present noticeable properties or activities that could be exploited for crop protection. For instance, RISP (Rust Induced Secreted Protein), a large ARP from poplar, showed a targeted activity that inhibits Pucciniales growth without affecting the growth of other fungi and bacteria (Petre et al., 2016). RISP could thus represent an active compound that controls rust epidemics without altering beneficial microbe communities, which may help achieve sustainable, integrated crop protection (Hacquard et al., 2017). Also, some ARPs display high stability that may be critical to withstand harsh field conditions (such as UV exposure, light and temperature variations, rain washing, and interaction with microflora and microbiota). Indeed, four ARPs are thermostable (PuroA; PuroB, RISP and chitinase EC 3.2.1.14); meaning that they remain stable and functional despite being exposed to high temperatures (Mathivanan et al., 1998; Alfred et al., 2013; Petre et al., 2016). Furthermore, some ARPs could effectively protect leaves from rust infection for weeks by stably remaining on the leaf surface (Petre et al., 2016; Schwinges et al., 2019).
ARP Studies Combine In Vitro and in Planta Approaches
To better understand the approaches used to evaluate ARP properties and activities, we analyzed the material and method sections of the sixteen studies reported in the Table 1. The studies used two main approaches: in vitro or in planta (Table 1, Figure 1C). In vitro approaches mostly evaluate the ability of purified peptides to inhibit the spore germination process, as the obligate biotrophic nature of most Pucciniales prevents the use of in vitro growth inhibition assays commonly used with cultivable fungi. In planta approaches evaluate the ability of peptide treatments to reduce the growth of the fungus on the host plant (most often on leaves). Amongst the sixteen publications, eight used only in vitro approaches, one used only an in-planta approach, and seven combined both (Table 1).
The most common in vitro assay (used in eleven studies) assesses the ability of a peptide to inhibit spore germination (‘inhibition of germination assay’). In such an assay, the experimenter classically spreads spores onto an agar medium with peptides at a concentration usually ranging from 0.01 to 0.1 mg/mL, and evaluates afterward the germination rate. In addition, ten studies used in vitro assays to evaluate the inhibition of the elongation of germ tubes or hyphae, the development of infection structures (e.g., appressoria), or the altered morphology of fungal structures. In planta assays assess the ability of a peptide to inhibit fungal growth on a leaf (‘infection assay’). In such an assay, the experimenter classically inoculates the rust fungus onto its host plant (or on detached leaves or leaf discs) before, during, or after treatment with purified peptides at a concentration usually ranging from 0.1 to 1 mg/mL, and evaluates afterward the appearance of disease symptoms by using a visual scoring system. Such scoring classically evaluates uredinia size and distribution, necrosis, and chlorosis depending on each pathosystem (Roelfs and Martens, 1988; Godoy et al., 2006). Noteworthy, for both in vitro and in planta approaches, two papers used electron microscopy to assess the alteration of fungal cellular structures (Rauscher et al., 1999; Lacerda et al., 2016). Such an approach reveals in detail the structural outcome of ARP treatment, but is arduous and costly to implement, explaining its seldom use.
ARP Modes of Actions Remain Mostly Unknown
To better understand how ARPs function, we screened the literature to identify reported modes of actions. This screen identified only two publications that reported information pertaining to the mode of action. Firstly, Fang and colleagues (2010) reported that Sp2 and Sp39 bind to a protein with an apparent size of 20 kDa from germinated urediniospores of P. pachyrhizi; though the paper did not evaluate the biological relevance of that observation (Fang et al., 2010). Secondly, Mathivanan and colleagues (1998) used light microscopy to show that treatment with a chitinase altered the cell wall appearance of P. arachidis urediniospores (Mathivanan et al., 1998). The purified peptide also displayed a chitinase activity, suggesting that it exhibits its anti-rust activity by degrading polysaccharides on the spore surface. For all the other ARPs, the literature reports no known modes of action against rust fungi. However, for some ARPs, a mode of action was proposed, or could be inferred, based on assays performed with other phytopathogenic fungi that are not Pucciniales. For instance, NaD1 binds to the cell wall of Fusarium oxysporum hyphae and permeabilizes the plasma membrane (Van Der Weerden et al., 2008; Van Der Weerden et al., 2010) (Supplementary Figure S1). To conclude, ARP modes of action remain vastly unknown, though knowledge gained via other fungi may be relevant.
Conclusion and Outlook: Key Things to Consider for Future ARP Studies
Overall, this short review inventoried sixteen papers that collectively reported 35 anti-rust peptides (ARPs) targeting in total 11 different rust species. It showed that the studies mainly used in vitro assays, sometimes complemented by in planta assays, to evaluate ARP properties and activities. The study also highlighted a clear knowledge gap regarding ARP modes of actions, since no explicit mode of action against rust fungi has been reported so far.
Defense proteins and peptides are growingly viewed as new active substances that can be leveraged to implement a next generation of sustainable biopesticides. In the case of ARPs, the research community crucially needs to better understand their mode of action in order to reach technology readiness levels aligned with phytosanitary implementation. Future studies could leverage the methods and technologies used in model fungi to decipher ARP modes of actions. Notably, assays that use fluorescent labels may help track ARPs and identify their binding sites on spores. Also, structure-function analyses that use truncated or site-directed mutagenized ARPs may help identify functional domains and residues important for anti-rust activity, stability, and specificity.
Author Contributions
Conception and design of the study (JL, BP, SD); data acquisition, analysis, and interpretation (JL, GD, EC, BP); manuscript drafting (JL, GD, EC); manuscript revision and editing (JL, GD, EC, BP, SD). All authors contributed to the article and approved the submitted version.
Funding
The authors are supported by grants overseen by the French PIA programmes Lab of Excellence ARBRE (ANR-11-LABX-0002-01), by the Pôle Scientifique A2F of the Université de Lorraine, and by the Région Grand Est (France).
Conflict of Interest
The authors declare that the research was conducted in the absence of any commercial or financial relationships that could be construed as a potential conflict of interest.
Publisher’s Note
All claims expressed in this article are solely those of the authors and do not necessarily represent those of their affiliated organizations, or those of the publisher, the editors and the reviewers. Any product that may be evaluated in this article, or claim that may be made by its manufacturer, is not guaranteed or endorsed by the publisher.
Acknowledgments
The authors acknowledge members of the UMR IAM, as well as Claire Veneault-Fourrey (INRAE, France), Sabine Fillinger (INRAE, France), Hugo Germain (UQTR, Canada) and Mathieu Gourgues (BAYER Crop Science, France) for thoughtful discussions on this topic. The authors warmly thank Linda De Bont for the critical reading of this manuscript.
Supplementary Material
The Supplementary Material for this article can be found online at: https://www.frontiersin.org/articles/10.3389/fagro.2022.966211/full#supplementary-material
Supplementary Figure 1 | Detailed effects of anti-rust peptides (ARPs). Effects of ARPs are shown on an urediniospore that germinates by differentiating a germ tube, an appressorium, and then infection hyphae. The rectangular insert on the bottom left indicates the proposed modes of actions discussed in the main text. (a) delay of Cronartium ribicola urediniospore germination; (b) particularly on chitin of nascent germ tube walls; (c) only against Puccinia coronata f. sp avenae; (d) only against Puccinia sorghi; (e) binding to a protein from Phakopsora pachyrhizi without a defined mode of action.
References
Aime M. C., Bell C. D., Wilson A. W. (2018). Deconstructing the Evolutionary Complexity Between Rust Fungi (Pucciniales) and Their Plant Hosts. Stud. Mycol. 89, 143–152. doi: 10.1016/j.simyco.2018.02.002
Alfred R. L., Palombo E. A., Panozzo J. F., Bariana H., Bhave M. (2013). Stability of Puroindoline Peptides and Effects on Wheat Rust. World J. Microbiol. Biotechnol. 29, 1409–1419. doi: 10.1007/s11274-013-1304-6
Barna B., Leiter É., Hegedus N., Bíró T., Pócsi I. (2008). Effect of the Penicillium Chrysogenum Antifungal Protein (PAF) on Barley Powdery Mildew and Wheat Leaf Rust Pathogens. J. Basic Microbiol. 48, 516–520. doi: 10.1002/jobm.200800197
Brand G. D., Magalhães M. T. Q., Tinoco M. L. P., Aragão F. J. L., Nicoli J., Kelly S. M., et al. (2012). Probing Protein Sequences as Sources for Encrypted Antimicrobial Peptides. PloS One 7, e45848. doi: 10.1371/journal.pone.0045848
Chen E. H. L., Weng C. W., Li Y. M., Wu M. C., Yang C. C., Lee K. T., et al. (2021). De Novo Design of Antimicrobial Peptides With a Special Charge Pattern and Their Application in Combating Plant Pathogens. Front. Plant Sci. 12. doi: 10.3389/fpls.2021.753217
Cook N. M., Chng S., Woodman T. L., Warren R., Oliver R. P., Saunders D. G. O. (2021). High Frequency of Fungicide Resistance-Associated Mutations in the Wheat Yellow Rust Pathogen Puccinia Striiformis F. Sp. Tritici. Pest Manag. Sci. 77, 3358–3371. doi: 10.1002/ps.6380
Corrêa A., Staples R. C., Hoch H. C. (1996). Inhibition of Thigmostimulated Cell Differentiation With RGD-Peptides in Uromyces Germlings. Protoplasma 194, 91–102. doi: 10.1007/bf01273171
Dangl J. L., Horvath D. M., Staskawicz B. J. (2013). Pivoting the Plant Immune System From Dissection to Deployment. Science 341, 746–751. doi: 10.1126/science.1236011
Dracatos P. M., van der Weerden N. L., Carroll K. T., Johnson E. D., Plummer K. M., Anderson M. A. (2014). Inhibition of Cereal Rust Fungi by Both Class I and II Defensins Derived From the Flowers of Nicotiana Alata. Mol. Plant Pathol. 15, 67–79. doi: 10.1111/mpp.12066
Duplessis S., Lorrain C., Petre B., Figueroa M., Dodds P. N., Aime M. C. (2021). Host Adaptation and Virulence in Heteroecious Rust Fungi. Annu. Rev. Phytopathol. 59, 403–422. doi: 10.1146/annurev-phyto-020620-121149
European Commission (2020) A Farm to Fork Strategy for a Fair, Healthy and Environmentally-Friendly Food System (Brussels: European Commission). Available at: https://www.eumonitor.eu/9353000/1/j4nvke1fm2yd1u0_j9vvik7m1c3gyxp/vl8tq28k4sxt/v=s7z/f=/com(2020)381_en.pdf (Accessed May 05, 2022).
Fang Z. D., Marois J. J., Stacey G., Schoelz J. E., English J. T., Schmidt F. J. (2010). Combinatorially Selected Peptides for Protection of Soybean Against Phakopsora Pachyrhizi. Phytopathology 100, 1111–1117. doi: 10.1094/PHYTO-12-09-0365
Figueroa M., Dodds P. N., Henningsen E. C. (2020). Evolution of Virulence in Rust Fungi - Multiple Solutions to One Problem. Curr. Opin. Plant Biol. 56, 20–27. doi: 10.1016/j.pbi.2020.02.007
Godoy C. V., Koga L. J., Canteri M. G. (2006). Diagrammatic Scale for Assessment of Soybean Rust Severity. Fitopatol. Bras. 31, 63–68. doi: 10.1590/S0100-41582006000100011
Hacquard S., Spaepen S., Garrido-Oter R., Schulze-Lefert P. (2017). Interplay Between Innate Immunity and the Plant Microbiota. Annu. Rev. Phytopathol. 55, 565–589. doi: 10.1146/annurev-phyto-080516-035623
Haney E. F., Straus S. K., Hancock R. E. W. (2019). Reassessing the Host Defense Peptide Landscape. Front. Chem. 7. doi: 10.3389/fchem.2019.00043
Jacobi V., Plourde A., Charest P. J., Hamelin R. C. (2000). In Vitro Toxicity of Natural and Designed Peptides to Tree Pathogens and Pollen. Can. J. Bot. 78, 455–461. doi: 10.1139/b00-025
Kumar J., Ramlal A., Mallick D., Mishra V. (2021). An Overview of Some Biopesticides and Their Importance in Plant Protection for Commercial Acceptance. Plants 10, 1185. doi: 10.3390/plants10061185
Lacerda A. F., Del Sarto R. P., Silva M. S., de Vasconcelos E., Coelho R. R., Dos Santos V., et al. (2016). The Recombinant Pea Defensin Drr230a is Active Against Impacting Soybean and Cotton Pathogenic Fungi From the Genera Fusarium, Colletotrichum and Phakopsora. 3 Biotech 6 (1), 59. doi: 10.1007/s13205-015-0320-7
Li J., Hu S., Jian W., Xie C., Yang X. (2021). Plant Antimicrobial Peptides: Structures, Functions, and Applications. Bot. Stud. 62, 1–15. doi: 10.1186/s40529-021-00312-x
Liu J. J., Fernandes H., Zamany A., Sikorski M., Jaskolski M., Sniezko R. A. (2021). In-Vitro Anti-Fungal Assay and Association Analysis Reveal a Role for the Pinus Monticola PR10 Gene (Pmpr10-3.1) in Quantitative Disease Resistance to White Pine Blister Rust. Genome 64, 693–704. doi: 10.1139/gen-2020-0080
Mathivanan N., Kabilan V., Murugesan K. (1998). Purification, Characterization, and Antifungal Activity of Chitinase From Fusarium Chlamydosporum, a Mycoparasite to Groundnut Rust, Puccinia Arachidis. Can. J. Microbiol. 44, 646–651. doi: 10.1139/w98-043
Montesinos E., Badosa E., Cabrefiga J., Planas M., Feliu L., Bardají E. (2012). “Antimicrobial Peptides for Plant Disease Control. From Discovery to Application,” in ACS Symposium Series. (Washington, USA: American Chemical Society), 235–261. doi: 10.1021/bk-2012-1095.ch012
Moscou M. J., van Esse H. P. (2017). The Quest for Durable Resistance. Science 358, 1541–1542. doi: 10.1126/science.aar4797
Oliver R. P., Hewitt H. G. (2014). “Fungicides in Crop Protection,” (Wallingford: Centre for Agriculture and Bioscience International publishing).
Petre B., Hecker A., Germain H., Tsan P., Sklenar J., Pelletier G., et al. (2016). The Poplar Rust-Induced Secreted Protein (RISP) Inhibits the Growth of the Leaf Rust Pathogen Melampsora Larici-Populina and Triggers Cell Culture Alkalinisation. Front. Plant Sci. 62. doi: 10.3389/fpls.2016.00097
Rauscher M., Ádám A. L., Wirtz S., Guggenheim R., Mendgen K., Deising H. B. (1999). PR-1 Protein Inhibits the Differentiation of Rust Infection Hyphae in Leaves of Acquired Resistant Broad Bean. Plant J. 19, 625–633. doi: 10.1046/j.1365-313X.1999.00545.x
Roelfs A. P., Martens J. W. (1988). An International System of Nomenclature for Puccinia Graminis F. Sp. Tritici. Phytopathology 78, 526–533. doi: 10.1094/PHYTO-78-526
Schwinges P., Pariyar S., Jakob F., Rahimi M., Apitius L., Hunsche M., et al. (2019). A Bifunctional Dermaseptin-Thanatin Dipeptide Functionalizes the Crop Surface for Sustainable Pest Management. Green Chem. 21, 2316–2325. doi: 10.1039/c9gc00457b
Tam J. P., Wang S., Wong K. H., Tan W. L. (2015). Antimicrobial Peptides From Plants. Pharmaceuticals 8, 711–757. doi: 10.3390/ph8040711
Van Der Weerden N. L., Hancock R. E. W., Anderson M. A. (2010). Permeabilization of Fungal Hyphae by the Plant Defensin NaD1 Occurs Through a Cell Wall-Dependent Process. J. Biol. Chem. 285, 37513–37520. doi: 10.1074/jbc.M110.134882
Van Der Weerden N. L., Lay F. T., Anderson M. A. (2008). The Plant Defensin, NaD1, Enters the Cytoplasm of Fusarium Oxysporum Hyphae. J. Biol. Chem. 283, 14445–14452. doi: 10.1074/JBC.M709867200
Van Loon L. C., Rep M., Pieterse C. M. J. (2006). Significance of Inducible Defense-Related Proteins in Infected Plants. Annu. Rev. Phytopathol. 44, 135–162. doi: 10.1146/annurev.phyto.44.070505.143425
Van Loon L. C., Van Strien E. A. (1999). The Families of Pathogenesis-Related Proteins, Their Activities, and Comparative Analysis of PR-1 Type Proteins. Physiol. Mol. Plant Pathol. 55, 85–97. doi: 10.1006/pmpp.1999.0213
Vasconcelos E. A. R., Santana C. G., Godoy C. V., Seixas C. D. S., Silva M. S., Moreira L. R. S., et al. (2011). A New Chitinase-Like Xylanase Inhibitor Protein (XIP) From Coffee (Coffea Arabica) Affects Soybean Asian Rust (Phakopsora Pachyrhizi) Spore Germination. BMC Biotechnol. 11, 11–14. doi: 10.1186/1472-6750-11-14
Wang F., Yuan S., Wu W., Yang Y., Cui Z., Wang H., et al. (2020). TaTLP1 Interacts With TaPR1 to Contribute to Wheat Defense Responses to Leaf Rust Fungus. PloS Genet. 16 (1–23), e10087. doi: 10.1371/journal.pgen.1008713
Keywords: interaction, defense protein, recombinant protein, mechanism, antifungal
Citation: Lintz J, Dubrulle G, Cawston E, Duplessis S and Petre B (2022) A Short Review of Anti-Rust Fungi Peptides: Diversity and Bioassays. Front. Agron. 4:966211. doi: 10.3389/fagro.2022.966211
Received: 10 June 2022; Accepted: 24 June 2022;
Published: 14 July 2022.
Edited by:
Pei Li, Kaili University, ChinaCopyright © 2022 Lintz, Dubrulle, Cawston, Duplessis and Petre. This is an open-access article distributed under the terms of the Creative Commons Attribution License (CC BY). The use, distribution or reproduction in other forums is permitted, provided the original author(s) and the copyright owner(s) are credited and that the original publication in this journal is cited, in accordance with accepted academic practice. No use, distribution or reproduction is permitted which does not comply with these terms.
*Correspondence: Benjamin Petre, benjamin.petre@univ-lorraine.fr