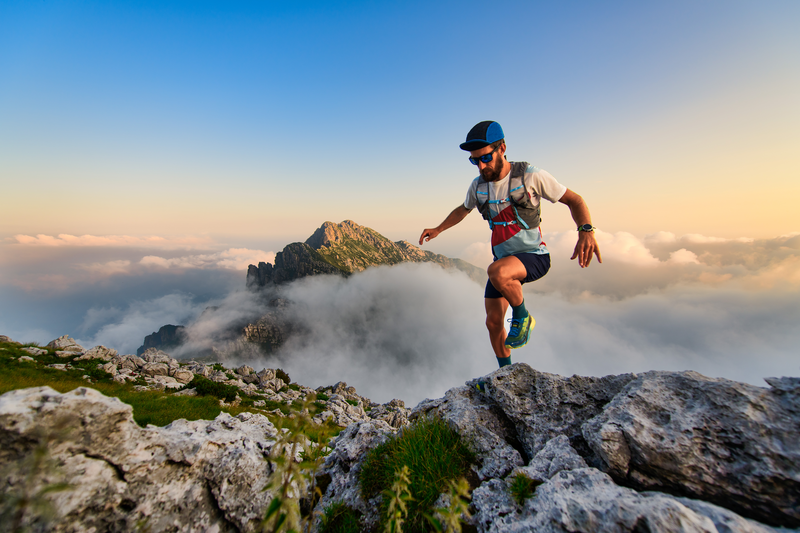
94% of researchers rate our articles as excellent or good
Learn more about the work of our research integrity team to safeguard the quality of each article we publish.
Find out more
ORIGINAL RESEARCH article
Front. Agron. , 29 July 2022
Sec. Weed Management
Volume 4 - 2022 | https://doi.org/10.3389/fagro.2022.954153
This article is part of the Research Topic Integrated Weed Management for Reduced Weed Infestations in Sustainable Cropping Systems View all 11 articles
Weed seeds can be spread by different vectors, and seed dispersal is an important mechanism for the weed to persist. Weed seeds passaging through the digestive tract of a ruminant animal is expected to result in reduced viability. Two separate experiments were conducted to determine the germinability and viability of the mature seeds of feathertop Rhodes grass (Chloris virgata Sw.) after exposure to four treatments, that is, 3 months in silage, 48 h in the rumen of steers, silage plus digestion, and passing through the digestive tract of sheep. Our results showed that three different treatments (silage, digestion, and silage plus digestion) can inhibit 90%–100% of the seed germination of feathertop Rhodes grass. Both silage and digestion reduced seed viability by 65%–90%, depending on the population. Silage followed by digestion reduced viability by 80%–97%. The sheep feeding study showed that total viable seeds from the daily recovery of feces for 12 consecutive days after ingestion was only 0.084% and 0.022% in the 2020 and 2021 experiments, respectively. In comparison with the untreated control, the seed viability of feathertop Rhodes grass was reduced by more than 99.9% after feeding through sheep, indicating that the spreading of feathertop Rhodes grass seeds via sheep feces is minimal. These results indicate that silage, digestion, silage followed by digestion, and the ingestion of mature seeds are effective non-chemical weed management options for an integrated weed management package for feathertop Rhodes grass.
Weeds are a problem in agriculture and were recently estimated to cost $3.3 billion in Australian cropping systems alone (Llewellyn et al., 2016). The primary method for weed control in most broadacre agricultural systems involves herbicide application, accounting for over 80% of weed control costs in Australia (Llewellyn et al., 2016). However, the threat to the efficacy of herbicides and to conservation tillage systems is the evolution of herbicide-resistant weeds (Heap, 2021). This is particularly the case where the repeated use of a single herbicide active ingredient (or active ingredients with the same mode of action) results in high selection pressure and accelerates the rate of herbicide resistance (Powles and Yu, 2010). To prolong the use of herbicides, future weed management will necessarily include non-chemical weed control strategies in combination with tactical herbicide use to manage herbicide resistance.
Annual weed species can frequently germinate early in the growing season and continue to germinate throughout the season, enabling them to maximize seed production and fitness across a broad range of environmental conditions (Sans and Masalles, 1997). The viable weed seeds result from weed survivors that reach maturity or can be imported into the field via other sources. Hence, the prevention of the replenishment of the weed seedbank and the reduction of viable weed seeds entering a field are critical to successful weed management. Often, annual weeds are highly palatable to grazing ruminants (Marten and Andersen, 1975) and can have high nutritive value (Moyer and Hironaka, 1993; Nashiki et al., 2005). Livestock can be used to directly consume and control weeds during fallow years and during fallow periods after harvest and before planting the next crop (Landau et al., 2006; Schoenbaum et al., 2009). Grazing livestock ingest various types of seeds when grazing mature weed plants or seeds scattered on the soil surface (Olson et al., 1997; Michael et al., 2006; Schoenbaum et al., 2009).
Passage through the digestive tract of ruminants (e.g., sheep and cattle) reduces the viability of several grass weeds seeds and legume seeds, thereby reducing the number entering the weed seed bank (Piggin, 1978; Stanton et al., 2002; Kneuper et al., 2003; Wang et al., 2017). However, some seeds are still viable after passing through the digestive tract of ruminants (Piggin, 1978; Stanton et al., 2002; Kneuper et al., 2003; Wang et al., 2017). These viable seeds can be transmitted by livestock, which is a mechanism of seed spread (endozoochory) utilized by many plant species. Weed seed spread by livestock is of most concern in regions of the world such as Australia with extensive grazing systems (Hogan and Phillips, 2011). The proportion of undamaged seeds is related to the seed coat, with hard-seeded species less affected by digestion by ruminant species and with less damage resulting from ingestion by cattle than sheep (Gardener et al., 1993a; Gardener et al., 1993b; Stanton et al., 2003; Michael et al., 2006; Haidar et al., 2010). Nevertheless, the role of the animal digestive tract on seed viability and the spread of weed seeds has not been sufficiently clarified for one of Australia’s most important weeds—feathertop Rhodes grass (Chloris virgata Sw.).
The production of silage is a common practice in many countries including Australia, providing farmers with a reserve of livestock feed that can be used strategically for production or for ameliorating seasonal feed shortages (Piltz et al., 2017). Silage is produced when forage is mechanically harvested, stored anaerobically, and fermented to produce acids that preserve the forage while anaerobic conditions are maintained (Piltz and Kaiser, 2004). Weeds and weed seeds present at the time of harvest are ensiled with the forage, and often, it is assumed that the silage renders most of these weed seeds non-viable (Kaiser et al., 2004). However, several studies reported that while most weed seeds are destroyed by silage, a small percentage remained viable (Blackshaw and Rode, 1991; Mayer et al., 2000; Piltz et al., 2017). Silage is produced for the specific purpose of feeding livestock; therefore, these viable seeds can potentially be spread by livestock in feces via ingestion and passage through the digestive tract.
Feathertop Rhodes grass is a major weed in both cropping and non-cropping situations in Australia. This annual species is very difficult to control, and no single weed management option provides adequate control. Currently, feathertop Rhodes grass control relies heavily on a few registered herbicides, particularly those with glyphosate and acetyl CoA carboxylase (ACCase) inhibitors, resulting in the rapid evolution of resistance to these key agrochemicals (Widderick, 2020; Heap, 2022). Therefore, an integrated approach is required for the effective management of this weed. Pastures and forage crops inevitably contain weed seeds, and a reduction in the number of viable seeds following ingestion or silage may present an effective control strategy. However, there are no reported data on feathertop Rhodes seed survival after silage and/or digestion. Two separate experiments were conducted to determine the effect of silage, digestion in cattle, and their combined effect on the germination and viability of different populations of feathertop Rhodes grass. Additionally, we wanted to determine the percentages of the recovery of the seed viability of feathertop Rhodes grass seeds after passing through the digestive tract of sheep.
A total of two and four populations of feathertop Rhodes grass were used for this study in 2020 and 2021, respectively (Table 1). Additionally, in 2021, annual ryegrass (Lolium rigidum) was used as a quality control and a comparison with previous studies.
Table 1 Feathertop Rhodes grass populations were tested against four different seed-damaging treatments (untreated control, silage, digestion, silage plus digestion) in 2020 and 2021.
Four treatments: (1) untreated (control), (2) silage, (3) digestion, and (4) silage plus digestion were employed in both years of the study. The seeds (n = 50) of each population were placed in bags made from white polyester monofilament (53 ± 10-µm pore size) used for in sacco digestion studies (Bar Diamond®). The pore size was small enough to contain all the seeds but still allows for the passage of water, gases, and microorganisms through the mesh (Haidar et al., 2010). Separate bags were used for each population, and the bags were ensiled in plastic bag mini-silos made of 100-µm polyethylene, each containing between 6 and 10 kg of chopped lucerne (Medicago sativa L.) forage (Wilson and Wilkins, 1972).
The forage was chopped directly from pasture with an Iseki mower with a catcher and spread in a thin (approx. 5–10-mm) layer on black plastic to wilt in order to reach the target dry matter (DM) content. The bags of seeds were layered in the chopped forage to ensure that each bag was in contact with silage. The chopped forage was physically compacted and air evacuated from the bag with a household vacuum cleaner and the bag opening tied securely to obtain an airtight seal. Then, each bag was placed inside a second bag of the same type and the vacuuming and tying process was repeated. The bags were packed into 200-L drums surrounded by damp sand with a layer of damp sand on the top to maintain weight on the bags. The bags were stored for 3 months to ensure the completion of the silage process; all silages were opened at the same time in each experiment. Two bags of each population were placed into each mini-silo, with each mini-silo representing a replicate; there were three replicates in the study. Six bags of each populations were retained and stored at room temperature (without silage).
Upon opening the silage, one bag of each population from each silo was paired with a bag of the same population that has not been ensiled. Both bags were placed in the rumen of a mature Red Poll steer for 48 h, which is the equivalent length of time as the rumen phase when determining in vitro digestibility (Tilley and Terry, 1963). All bags from each mini-silo were placed in the rumen of the same steer, and bags from different mini-silos were placed in different steers. Three steers were fed a diet consisting of lucerne hay, oaten chaff, barley grain, and oat grain at 300, 300, 200, and 200 g kg-1 of the diet, respectively, on an as-fed basis. The steers were fed daily each morning an amount calculated such that intake was approximately 1.2 times maintenance requirements. Diets were fed for 10 days prior to commencement of the digestion study to ensure that the rumen had adjusted to a standard diet and then for 48 h during which digestion degradability was determined.
After silage and digestion treatments had been imposed, the recovered seeds were placed on Whatman No. 1 filter paper moistened with 4 ml of distilled water in a 9-cm petri dish. Petri dishes were sealed with Parafilm and incubated for 11 days at 25°C/15°C day/night temperatures with a 12-h photoperiod. The number of germinated seeds was recorded, and the remaining non-germinated seeds of feathertop Rhodes grass were tested for viability using the tetrazolium test previously described by Stanton et al. (2002).
Only one population (MUTT 04/20) was used for this study due to the availability of a large number of seeds. The seeds (900 g) of this population were collected between April and May 2020 on a roadside in Gobbagombalin (146°34’201”E, 35°07’606”S), Wagga Wagga, NSW 2650. Laboratory tests showed that seed had a 65% germination rate 7 months after collection. A previous feeding trial protocol (Stanton et al., 2002) was adopted with slight modification. Briefly, before the commencement of the trial, the sheep (n = 8) were individually penned and fed a diet consisting of lucerne and oaten chaff (1:1). The sheep were fed daily each morning an amount calculated such that intake is approximately 1.2 times the maintenance requirements (average 856 g head-1.day). On 26 November 2020, the sheep were fed the standard diet mixed with 40 g of feathertop Rhodes grass seeds (approximately 135,800 seeds). Following the introduction of feathertop Rhodes grass seeds into the diet, the total feces were collected daily from day 1 to day 12 to determine the time taken from the ingestion to complete excretion of the seed. Fecal collection for each day occurred 24 h after feeding; hence, feces for day 1 were collected prior to feeding on day 2 and so on.
The feces sample was weighed and separated into three subsamples where one subsample (260 g approx.) was used to determine seed viability in a glasshouse. Other subsamples were air-dried and kept in a cool room for further testing to confirm the results. The third subsamples were used for a digestibility test (not reported here). To determine the percentages of seed viability in the feces, the first subsample was evenly spread onto a plastic seedling tray (32 cm × 28 cm × 6 cm), which was filled with a field soil (loamy soil). The feces were gently rubbed and lightly mixed with the surface soil. The trays were kept moist and monitored for 21 days. The trays were maintained under a glasshouse condition and irrigated as required. The average temperature, relative humidity, and light intensity of the glass house during the experimental period were 22–27°C, 70%–85%, and 217–363 lum/ft.2, respectively. The total emerged seedlings were counted to estimate the seed viability and the speed with which feathertop Rhodes grass seeds passed through the gut of sheep. The cumulative seedling emergence (Ec) in both years’ experiments were calculated by using a formula: Ec = e0 + ∑ (ei+1 - ei), where e0 is the number of seedlings in first count, and ei is the number of seedlings in count i. We were unable to extract the seeds from the feces through sieving or washing due to the tiny seeds (0.39 mg 100 seeds-1).
In March 2021, the above experiment was repeated with the second subsample to validate the results obtained in 2020. An additional control treatment was included where 300 seeds of feathertop Rhodes grass were spread on a seedling tray under the same glasshouse conditions in 2021. The total emerged seedlings were counted in the same manner as described above.
A randomized complete block design was employed for the germination and viability evaluation for experiment 1, where the three shelves of the incubator were considered as a blocking factor to minimize systemic errors. The R packages including agricolae (De-Mendiburu 2021) were also used on R Core Team (2022) for exploratory data analysis and data fitting in different models. The normality and distribution of data was verified by a Shapiro–Wilk normality test. The data variance was also examined by plotting the residuals to confirm the homogeneity of variance. The significant differences among treatment means were identified by Tukey’s HSD (honestly significant difference) at p<0.05.
Given that different populations were employed, and there were some variations observed between years, the data from 2020 and 2021 were analyzed separately for experiment 1. Data were analyzed using linear mixed models with the population, treatment and interaction as fixed effects and the position within the incubator as the random effect.
A completely randomized design was employed with eight replications (sheep) for experiment 2. Data were pooled from two runs, as there was no time-by-treatment interaction as determined by ANOVA. A Pearson correlation coefficient value was calculated to see if there was any significant correlation between the seedling emergence rate in 2020 and 2021 for experiment 2. The significant r-value indicated that there was consistency between the 2020 and 2021 experiments.
The silage dry matter content and pH value were 396 and 505 g kg-1 and 5.3 and 5.1 in 2020 and 2021, respectively. The germinability of untreated (control) seeds varied significantly (p<0.005) between the populations of feathertop Rhodes grass (Table 2). Silage treatments resulted in 0% germination for two and four populations tested in 2020 and 2021, respectively. Additionally, in 2020, the digestion treatment reduced seed germination and only 2%–10% of the seeds of feathertop Rhodes grass were subsequently germinated.
Table 2 Seed germination and viability of four different populations of feathertop Rhodes grass after four different seed-damaging treatments.
The repeated study in 2021 showed that the recovered seed of all 4 populations tested failed to germinate after digestion or silage plus digestion. Compared to the untreated control, the viability of the four populations was reduced by 65%–95%, 73%–90%, and 80%–97% after silage, digestion, and silage plus digestion, respectively (Table 2). The total number of viable seeds significantly (p<0.005) differed between populations after being exposed to the three different seed-damaging treatments.
There was no significant (p>0.005) difference in viability between the three treatments (silage, digestion, and silage plus digestion) (Figure 1) in 2021. On average, the seed viability of the four populations declined by 72%, 70%, and 82% after silage, digestion, and silage plus digestion, respectively.
Figure 1 Average seed viability of four populations of feathertop Rhodes grass in response to different seed treatments in 2021. Vertical bar shows mean (± standard error) of germination. Different letters show significant difference as identified by Tukey’s HSD at p<0.05.
The average amount of fresh feces of eight sheep from day 1 to day 12 is presented in Table 3. The fresh feces ranged from 695.25 to 968 g with an average of 789 g for days 1–12. During fresh feces collection, it was noticed that there were no issues with diet adaption with feathertop Rhodes grass in seven of the eight sheep. Sheep number 4 suffered from diarrhea, and the number of extracted feces was comparatively less than that of other sheep.
Table 3 Average fresh feces (g) collected, the number of seedlings emerged/tray, and cumulative emergence (%) from day 1 to day 12.
We observed that seeds in the feces emerged 4 or 5 days later than the untreated (control) seeds in 2021 (data not shown). Most of the seedling emergence (58%–62%) occurred from the feces collected during the first 3 days after seed intake in both years (Table 3). There was still limited seedling emergence from feces collected 12 days after feeding, being less than 1% and 6% of the total seeds emerged in 2020 and 2021, respectively. On average, the total number of emerged seedlings from day 1 to day 12 was 38.00 in 2020 and 9.80 in 2021, which is estimated at a survival rate of 0.08% and 0.022% in 2020 and 2021, respectively.
A correlation analysis showed (Figure 2) that there was a significant (p<0.001) correlation (r = 0.82***) in the seedling emergence pattern between the 2020 and 2021 experiments, indicating that both experiments achieved consistent results.
Figure 2 Correlation between seedling emergence (%) in sheep-feeding trial (experiment 2) in 2020 and 2021 at different days after feces collection. *** = highly significant.
In comparison, 53.4% of the seed in the control treatment germinated in the trays under the same conditions. This showed that sheep rendered more than 99.9% feathertop Rhodes grass seeds unviable after passing through the digestive system.
In our study, silage and digestion independently reduced germinability and viability compared with untreated control seeds for feathertop Rhodes grass, with the magnitude of the reduction varying with populations and in some cases between years. Our results were consistent with Piltz et al. (2017) who found that both seed damage treatments (silage and digestion) can significantly reduce the seed germinability and viability of a range of grass weed species including barley grass (Hordeum spp.), vulpia (Vulpia spp.), wild oat (Avena fauta), brome grass (Bromus diandrus), and annual ryegrass. The causes of seed death following ingestion by ruminant livestock are reasonably well understood (Hogan and Phillips, 2011). The damage of seed occurs during the digestive process in the rumen and the whole digestive tract. The extent of damage also depends on the degree of mastication, which varies between the species of an animal, with seeds being more extensively damaged by sheep and goats than by cattle (Simao Neto et al., 1987). However, the mechanism(s) operating to reduce or eliminate seed viability during silage are not yet clear. Silage is a lactic acid fermentation process that is mainly used to preserve biomass and affects the seed viability of some plant species (Piltz et al., 2017; Hahn et al., 2021; Piltz et al., 2021). Lactic acid is the strongest of silage acids, and these acids are produced by this fermentation of plant sugars. Several acids produced by the fermentation are affected by the plant parameters such as forage dry matter content, water-soluble carbohydrate, and buffering capacity during the ensiling process (Müller and Bauer, 2006; Piltz and Kaiser, 2004).
The seed viability loss of feathertop Rhodes grass under silage varied between 65% and 95% dependent on populations in our research. The differences between the studies (2020 vs. 2021) of a population could be due to the possible effects of the surrounding silage substrate and to different initial seed qualities. The seed lot quality can vary strongly depending on factors such as seed age, plant population, and storage conditions (e.g., Hay and Probert, 2013; Weller et al., 2016;). For example, the viability of our untreated seeds and the seed size differed significantly between the populations of feathertop Rhodes grass (Asaduzzaman et al., 2022). The specific mechanisms by which a small proportion of seed of feathertop Rhodes grass survived after silage are not yet clear. Our studies suggest that a significant proportion of seed viability is reduced due to silage, which can be a suitable control measure for feathertop Rhodes grass. In addition to damaging seeds, silage appeared to have weakened the seed vigor of feathertop Rhodes grass as the proportion of germinable seeds to viable seeds was reduced. We think that it is unlikely that these viable seeds will develop into true or healthy seedlings. However, this assumption was not determined in our current study. Furthermore, weed seeds ensiled from pasture or crops during commercial production would likely be less mature; therefore, the impact from silage and digestion either alone or in combination on seed viability is possibly far greater.
In our study, the effect of digestion on the seed germinability and viability of feathertop Rhodes grass was almost the same as the observed effect by silage (Figure 1). Similarly, Stanton et al. (2002) in annual ryegrass who reported that 10.8% and 32.8% of the seed ingested was excreted by sheep and cattle, respectively, with 3.9% (sheep) and 11.9% (cattle) remaining germinable. They concluded that additional strategies were required to manage the excreted viable seeds. In our study, the impacts of silage and digestion were more effective in reducing the seed viability of feathertop Rhodes grass than annual ryegrass with the exception of only one population (HOLB 01/20) tested in 2021. These data support the use of silage production and grazing as part of an integrated control program for feathertop Rhodes grass. Generally, the reduction in germinability and viability following silage plus digestion was greater than for silage or digestion alone, which is expected as each treatment will potentially reduce seed integrity independently. The seeds of feathertop Rhodes grass are small with a thin seed coat; therefore, the tiny embryo can be easily damaged. Other studies have reported that generally, the seeds of grasses were more susceptible to damage during ensiling and/or digestion than the seeds of hard-seeded species (Blackshaw and Rode, 1991; Westerman et al., 2012; Hahn et al., 2021; Piltz et al., 2021). Additionally, previous research found a longer period in silage that resulted in a higher seed-killing efficacy (Waldo et al. 1973; Mayer et al., 2000; van Eekeren et al., 2006; Trolove and Dowsett, 2015; Simard and Lambert-Beaudet, 2016). However, most studies would indicate that 3 months of ensiling rendered most seeds unviable, and this is consistent with our findings.
Similarly, damage to seed depends on the degree of mastication, which varies between the species of animal, and on the length of time the seed remains in the digestive tract. Generally, the ability of excreted seed to germinate declines with time in the tract, although the rate of decline varies between plants species and animals (Hogan and Phillips, 2011). For example, sheep and goats caused more extensive damage on seed than by cattle (Simao Neto et al., 1987). Our study indicated a clear peak in seed excretion between 1 and 12 days after ingestion, with approximately 60% of viable seed recovered during days 1–3. In the case of 2020, which had higher numbers of excreted viable seed, there was 80% collection during days 1–5. Seed viability percentages after ingestion ranged from 0.022% to 0.084%. These percentages are lower than those reported in other studies involving small ruminants. For example, seed recovery percentages after ingestion were 0%–28% (Yu et al., 2012) and 10.4%–23.0% (Manzano et al., 2005) in sheep and 7.4%–17.4% in goats (Robles et al., 2005) for annual grass species. One of the possible reasons for the relatively low viability percentage in our studies is due to the small seed size (only 0.39 mg 100 seeds-1) of feathertop Rhodes grass, which, we speculate, makes these seeds more prone to be damaged after ingestion. Additionally, the digestive tracts of smaller animals increase the likelihood of small seeds contacting the gut wall, which can damage the seed by abrasion (Razanamandranto et al., 2004). Additionally, Harvey (1981) used indirect comparisons and suggested that sheep are more effective than cattle in digesting seeds. However, we observed that some seeds were still viable at 12 days postfeeding after passing through the digestive tract. Consequently, some spread of viable seeds can still occur for a prolonged period after ingestion, and practically, farmers should monitor for the spread of feathertop Rhodes via this means into other areas.
Our study suggested that silage or digestion can significantly reduce both the seed germination and viability of feathertop Rhodes grass. However, 5%–35% seeds of feathertop Rhodes grass can remain viable after silage depending on the population. The seed viability rate can be further reduced (by up to 90%) by the combined effect of silage plus digestion. Our research also revealed that the seed viability of feathertop Rhodes grass was reduced by feeding to sheep. Feeding to sheep reduced germinable seeds from the initial 65% to less than 0.1% in both studies. Approximately 60% of the germinable seeds were excreted by the sheep during the first 3 days after feeding. The seed viability of feathertop Rhodes grass was reduced by more than 99.9% after feeding through sheep, indicating that the spreading of feathertop Rhodes grass seeds through sheep tracts is minimal. However, a low level of germinable seeds (<10%) can still be detected at 12 days after feeding. Feathertop Rhodes grass plants should not be allowed to grow to the mature stage due to the massive seed production (Widderick et al., 2014). The light-weighted awny seeds can be spread by many mechanisms such as animal hide, feet, wind, water, and machinery. The use of sheep grazing should only be performed prior to seed head emergence to reduce the risk of potential spread. If sheep are suspected of having fed on mature plants, then ideally, these sheep should be quarantined for a week, which will allow more than 90% of seeds to be excreted. Effective grazing prior to seed head emergence provides an alternative to herbicides, thereby reducing selection pressure for herbicide resistance. Grazing can also buy time for effective control. For example, advanced weed plants under moisture stress are often less responsive to herbicide application. Therefore, grazing can keep stressed plants under control and allow for the grazed plants to have an herbicide applied at a later stage when the spraying conditions may be ideal for maximum control efficacy. Ecological weed management aims to subject weeds to multiple, temporally variable stresses, for which Liebman and Gallandt (1997) coined the term “many little hammers.” Based on the results of our study, we claimed that silage and digestion are an overlooked yet effective way to reduce feathertop Rhodes grass seed loads in a sustainable manner. By reducing the number and vigor of weed seeds, silage and digestion can help exclude feathertop Rhodes grass as well as other weeds from fields. The reduction of density and delaying of emergence can reduce the interference with crop growth or reproduction. Thus, silage and digestion or a combination of both can be some of the “little hammers” in ecological weed management in an integrated farming system.
The raw data supporting the conclusions of this article will be made available by the authors, without undue reservation.
The animal study was reviewed and approved by NSW DPI. Written informed consent was obtained from the owners for the participation of their animals in this study.
MA, JP, EK, and HW contributed to the conception and the design of the study. AS, MH, MA, and JP contributed for the preparation of the silages. MA edited the manuscript, organized this project, analyzed the viability of the seeds, and performed all statistical analyses. JP, EK, and HW contributed for the revision of the manuscript. AS and MH helped for experiment set up and data collections. All authors contributed to the article and approved the submitted version.
The authors acknowledge funding from the Grain Research Development Corporation (GRDC) DAN1912-034RTX and NSW Department of Primary Industries, Australia.
We thank all the collaborators who provided seeds of two northern populations of feathertop Rhodes grass. We thank to Bernie Dominiak and Xiaocheng Zhu for reviewed an early version of the manuscript.
This study received funding from Grain Research Development Corporation (GRDC) and NSW Department of Primary Industries. The funders were not involved in the study design, collection, analysis, interpretation of data, the writing of this article.
The authors declare that the research was conducted in the absence of any commercial or financial relationships that could be construed as a potential conflict of interest.
All claims expressed in this article are solely those of the authors and do not necessarily represent those of their affiliated organizations, or those of the publisher, the editors and the reviewers. Any product that may be evaluated in this article, or claim that may be made by its manufacturer, is not guaranteed or endorsed by the publisher.
Asaduzzaman M., Wu H., Koetz E., Hopwood M., Shepherd A. (2022). Phenology and population differentiation in reproductive plasticity in feathertop rhodes grass (Chloris virgata sw.). Agronomy 12 (3), 736. doi: 10.3390/agronomy12030736
Blackshaw R. E., Rode L. M. (1991). Effect of ensiling and rumen digestion by cattle on weed seed viability. Weed Sci. 39, 104–108. doi: 10.1017/S004317450005
Gardener C. J., McIvor J. G., Jansen A. (1993a). Passage of legume and grass seed through the digestive tract of cattle and their survival in faeces. J. App. Ecol. 30, 63–74. doi: 10.2307/2404271
Gardener C. J., McIvor J. G., Jansen A. (1993b). Survival of seeds of tropical grassland species subjected to bovine digestion. J. App. Ecol. 30, 75–85. doi: 10.2307/2404272
Hahn J., deMol F., Muller J. (2021). Ensiling reduces seed viability: implications for weed management. Front. Agron. 708851. doi: 10.3389/fagro.2021.708851
Haidar M., Gharib C., Sleiman F. T. (2010). Survival of weed seeds subjected to sheep rumen digestion. Weed Res. 50, 467–471. doi: 10.1111/j.1365-3180.2010.00797.x
Harvey G. J. (1981). “Recovery and viability of prickly acacia (Acacia nilotica ssp. indica) seed ingested by cattle and sheep,” in ‘Proceedings 6th Australian weeds conference i’. Eds. Wilson B. J., Swarbrick J. T. (Clifford Gardens, Qld: Weeds Society of Queensland), 197–202.
Wilson R. F., Wilkins J. F. (1972). An evaluation of laboratory ensiling techniques. J. Scie. Fd. Agric. 23, 377–385.
Hay F. R., Probert R. J. (2013). Advances in seed conservation of wild plant species: a review of recent research. Cons. Physiol. 1, 1–11. doi: 10.1093/conphys/cot030
Heap I. (2021) The international survey of herbicide resistant weeds. Available at: www.weedscience.com (Accessed Dec 22, 2021).
Heap I. (2022). The international survey of herbicide resistant weeds. Available at: www.weedscience.com (accessed Jun 10, 2021).
Hogan J. P., Phillips C. J. C. (2011). Transmission of weed seed by livestock: a review. Anim. Prod. Sci. 51, 391–398. doi: 10.1071/AN10141
Kaiser A. G., Doonan B. M., Bowcher A. J. (2004). “In: Silage as a pasture management tool,” in Successful silage. Eds. Kaiser A. G., Piltz J. W., Hmburns, Griffiths N. W. (Orange, Australia: NSW Department of Primary Industries and Dairy Australia), 25–56.
Kneuper C. L., Scott C. B., Pinchak W. E. (2003). Consumption and dispersion of mesquite seeds by ruminants. J. Rang. Manage. 56, 255–259. doi: 10.2307/4003815
Landau S., Glasser T., Dvash L. (2006). Monitoring nutrition in small ruminants with the aid of near infrared reflectance spectroscopy (NIRS) technology: A review. Small. Rum. Res. 61, 1–11. doi: 10.1016/j.smallrumres.2004.12.012
Liebman M., Gallandt E.R. (1997). Many little hammers: Ecological management of crop–weed interactions. In: Ecology in Agriculture (ed Jackson LE), 291–343. Academic Press, San Diego, USA.
Llewellyn R., Ronning D., Clarke M., Mayfield A., Walker S., Ouzman J. (2016). Impact of weeds in Australian grain production (Canberra, ACT: Grains Research and Development Corporation).
Manzano P., Malo J. E., Peco B. (2005). Sheep gut passage and survival of Mediterranean shrub seeds. Seed Sci. Res. 15, 21–28. doi: 10.1079/SSR2004192
Marten G. C., Andersen R. N. (1975). Forage nutritive value and palatability of 12 common annual weeds. Crop Sci. 15, 821–827. doi: 10.2135/cropsci1975.0011183X001500060024x
Mayer F., Albrecht H., Pfadenhauer J. (2000). The influence of digestion and storage in silage and organic manure on the germinative ability of six weed species (Papaver argemone, P. dubium, Legousia speculum-veneris, Centaurea cyanus, Spergula arvensis, Trifolium arvense). Z. fur. Pflanzenkrankheiten und Pflanzenschutz 17, 47–54.
Michael P., Steadman K., Plummer J., Vercoe P. (2006). Sheep rumen digestion and transmission of weedy Malva parviflora seeds. Aust. J. Expt. Agr. 46, 1251–1256. doi: 10.1071/EA05285
Moyer J., Hironaka R. (1993). Digestible energy and protein content of some annual weeds, alfalfa, bromegrass, and tame oats. Cand. J. Plant Sci. 73, 1305–1308. doi: 10.4141/cjps93-169
Müller J., Bauer R. (2006). Futterkonservierung, in pflanzliche erzeugung: Grundlagen des acker- und pflanzenbaus und der guten fachlichen praxis. Eds. Munzert M., Frahm J. (München: BLV Buchverlag), 865–933.
Nashiki M., Narita H., Higashiyama Y. (2005). Herbage mass, nutritive value and palatability of five grass weeds for cattle in the northern tohoku region in Japan. Weed Biol. Mang. 5 (3), 110–117. doi: 10.1111/j.1445-6664.2005.00171.x
Olson B. E., Wallander R. T., Lacey J. R. (1997). Effects of sheep grazing on a spotted knapweed-infested Idaho fescue community. J. Range Manage. 50, 386–390. doi: 10.2307/4003305
Piggin C. M. (1978). Dispersal of Echium plantagineum l. by sheep. Weed Res. 18, 155–160. doi: 10.1111/j.1365-3180.1978.tb01152.x
Piltz J. W., Bailes K. L., Boschma S. P., Weston L. A. (2021). The impact of ensiling at different moisture contents on germinability and viability of selected weed species’ seeds. Agronomy 11, 1–10. doi: 10.3390/agronomy11081639
Piltz J. W., Kaiser A. G. (2004). “Principles of silage preservation,” in Successful silage, 2nd. Eds. Kaiser A. G., Piltz J. W., Burns H. M., Griffiths N. W. (Orange, NSW: Dairy Australia and New South Wales Department of Primary Industries), 25–56.
Piltz J. W., Stanton R. A., Wu H. (2017). Effect of ensiling and in sacco digestion on the viability of seeds of selected weed species. Weed Res. 57, 382–389. doi: 10.1111/wre.12269
Powles S. B., Yu Q. (2010). Evolution in action: plants resistant to herbicides. Ann. Rev. Plant Biol. 61, 317–347. doi: 10.1146/annurev-arplant-042809-112119
R Core Team (2022) R: A language and environment for statistical computing. R Foundation for Statistical Computing, Vienna, Austria. Available at: https://www.R-project.org/
Razanamandranto S., Tigabu M., Neya S., Oden P. C. (2004). Effects of gut treatment on recovery and germinability of bovine and ovine ingested seeds of four woody species from Sudanian savanna in West Africa. Flora 199, 389–397. doi: 10.1078/0367-2530-00167
Robles A. B., Castro J., Gonzalez Miras E., Ramos M. E. (2005). Effects of ruminal incubation and goat’s ingestion on seed germination of two legume shrubs. Options Medit. 67, 111–115.
Sans F. X., Masalles R. M. (1997). Demography of the arable weed Diplotaxis erucoides in central Catalonia, Spain. Cand. J. Bot. 75, 86–95. doi: 10.1139/b97-011
Schoenbaum I., Kigel J., Barkai D., Landau S. (2009). Weed infestation of wheat fields by sheep grazing stubble in the Mediterranean semi-arid region. Crop Past. Sci. 60, 675–683. doi: 10.1071/CP08283
Simao Neto M., Jones R. M., Ratcliff D. (1987). Recovery of pasture seed ingested by ruminants. 1. seed of six tropical pasture species fed to cattle, sheep and goats. Aust. J. Agric. Res. 27 (2), 239–246. doi: 10.1071/EA9870239
Simard M. J., Lambert-Beaudet C. (2016). Weed seed survival in experimental mini-silos of corn and alfalfa. Cand. J. Plant Sci. 96, 448–454. doi: 10.1139/cjps-2015-0261.
Stanton R., Piltz J., Pratley J., Kaiser A., Hudson D., Dill G. (2002). Annual ryegrass (Lolium rigidum) seed survival and digestibility in cattle and sheep. Aus. J. Expt. Agric. 42, 11–115. doi: 10.1071/EA01069
Stanton R., Pratley J., Hudson D. (2003). Sheep are potential vectors for the spread of canola (Brassica napus) seed. Aust. J. Expt. Agr. Res. 43, 535–538. doi: 10.1071/EA02046
Tilley J. M. A., Terry R. A. (1963). A two-stage technique for the in vitro digestion of forage crops. Grass Forage. Sci. 18, 104–111.
Trolove M. R., Dowsett C. A. (2015). Yellow bristle grass seed killed in maize silage. New Z. Plant Prot. 68, 442. doi: 10.30843/nzpp.2015.68.5847
van Eekeren N., Fehér L., Smeding F., Prins U., Jansonius P. J. (2006). “Controlling broad-leaved dock (Rumex obtusifolius) in grass clover mixtures”,” in Sustainable grassland productivity: Proceedings of 21st general meeting of the European grassland federation. Eds. Lloveras J., Gonzáles-Rodríguez A., Vázquez-Yánez O., Pineiro J., Santamaría O., Olea L., et al (European Grassland Society, Madrid, Spain), 396–398.
Waldo D. R. (1973). Extent and partition of cereal grain starch digestion in ruminants. J. Anim. Sci. 37, 1062. doi: 10.2527/jas1973.3741062x
Wang S. W., Waly N., Ma C., Zhang W., Wan C. (2017). Recovery and germination of seeds after passage through the gut of Kazakh sheep on the north slope of the tianshan mountains. Seed Sci. Res. 27, 43–49. doi: 10.1017/S0960258517000022
Weller S. L., Florentine S. K., Sillitoe J. F., Grech C. J., McLaren D. A. (2016). An investigation of the effects of stage of ensilage on Nassella neesiana seeds, for reducing seed viability and injury to livestock. Sci. Rept. 6, 22345. doi: 10.1038/srep22345
Westerman P. R., Hildebrandt F., Gerowitt B. (2012). Weed seed survival following ensiling and mesophilic anaerobic digestion in batch reactors. Weed Res. 52, 286–295. doi: 10.1111/j.1365-3180.2012.00918.x
Widderick M., Cook T., McLean A., Churchett J., Keenan M., Miller B., Davidson B., et al (2014). Improved management of key northern region weeds: Diverse Problems, Diverse Solutions. Hobart, Tasmania, Australia: Tasmanian Weed Society. pp 312–315.
Widderick M. (2020). Integrated weed management of feathertop rhodes grass, 2nd edition 2020 Update. Grain. Res. Dev. Cooperation, 1–36.
Keywords: germination, seed viability, silage, digestion, ruminant, sheep
Citation: Asaduzzaman M, Piltz J, Koetz E, Hopwood M, Shephard A and Wu H (2022) Seed viability of feathertop Rhodes grass (Chloris virgata Sw.) reduced by silage, digestion, and sheep rumen digestion. Front. Agron. 4:954153. doi: 10.3389/fagro.2022.954153
Received: 27 May 2022; Accepted: 28 June 2022;
Published: 29 July 2022.
Edited by:
Simerjeet Kaur, Punjab Agricultural University, IndiaReviewed by:
Navjyot Kaur, Punjab Agricultural University, IndiaCopyright © 2022 Asaduzzaman, Piltz, Koetz, Hopwood, Shephard and Wu. This is an open-access article distributed under the terms of the Creative Commons Attribution License (CC BY). The use, distribution or reproduction in other forums is permitted, provided the original author(s) and the copyright owner(s) are credited and that the original publication in this journal is cited, in accordance with accepted academic practice. No use, distribution or reproduction is permitted which does not comply with these terms.
*Correspondence: Md Asaduzzaman, bWQuYXNhZHV6emFtYW5AZHBpLm5zdy5nb3YuYXU=
Disclaimer: All claims expressed in this article are solely those of the authors and do not necessarily represent those of their affiliated organizations, or those of the publisher, the editors and the reviewers. Any product that may be evaluated in this article or claim that may be made by its manufacturer is not guaranteed or endorsed by the publisher.
Research integrity at Frontiers
Learn more about the work of our research integrity team to safeguard the quality of each article we publish.