- 1Food Security and Safety Focus Area, Faculty of Natural and Agricultural Sciences, North-West University, Mmabatho, South Africa
- 2Laboratoire de Biologie et de Typage Moléculaire en Microbiologie, Département de Biochimie et de Biologie Cellulaire, Université d’Abomey-Calavi, Cotonou, Benin
- 3Driection Générale, Institut National des Recherches Agricoles du Bénin, Abomey-Calavi, Benin
The objective of the study was to evaluate the fertilizing potential of two arbuscular mycorrhizal fungi (Glomeraceae and Acaulosporaceae) native to the rhizosphere soils of Benin in a controlled environment (under greenhouse) and in a field environment. For this purpose, corn seeds were coated with Glomeraceae and Acaulosporaceae strains and then deposited in 5 cm deep holes. Different doses of mineral fertilizer were then applied according to the treatments.The experimental design was a split plot of ten treatments with three replicates in both conditions. Sowing was done following two seeds per pot in the greenhouse and in the field and lasted 30 and 80 days respectively. The data collected were related to growth, yield and mycorrhizal infection of the roots. The results in controlled environment (under greenhouse), show that the plants treated with Acaulosporaceae+25%NPK+Urea significantly improve the height, the diameter at the collar, and the dry subterranean biomass with respective increases of 63.74%, 61.53%, 47.79% except for the leaf area and the dry aerial biomass which were improved by Glomeraceae+25%NPK+Urea with respective increases of 42.66% and 57.2% compared to the control. The results in the field showed that the best maize yields in grain, in dry aerial biomass and dry subterranean biomass were obtained significantly with the Glomeraceae strain 25%NPK+Urea with respective increases of 65.28%, 51.26%, 136.36% compared to the control plants. Also, high values were recorded for the frequency and intensity of mycorrhization with the Glomeraceae strain under greenhouse and field conditions. Mycorrhizal inoculation of maize plants showed a beneficial effect in the field with the Glomeraceae strain in combination with 25% NPK+Urea while the Acaulosporaceae strain with 25% NPK+Urea was more expressed in the greenhouse. These endogenous strains can be used as biostimulants to increase maize productivity while considerably reducing mineral inputs in Benin.
1 Introduction
The major challenge for agriculture in the coming decades will be the sustainable production of sufficient food crops to meet the ever-increasing global demand. (Battini et al., 2017; Emmanuel and Babalola, 2020). Current agricultural systems rely heavily on the continuous application of mineral inputs including mineral fertilizers, mainly nitrogen (N), phosphorus (P) and potassium (K), which contribute to increasing yields but also lead to the decline of land-based biological fertility (Plenchette et al., 2005; Balogoun et al., 2013). This overdependence results into sevearal problems to soil, plants and human health through adverse consequences on food quality, soil health, alongside atmospheric and water systems (Yang et al., 2004; Igiehon and Babalola, 2018). As a result, in recent years there has been a focus on reducing high-input agricultural systems with more research in order to develop sustainable and eco-friendly alternatives for food production. Different approaches to reduce chemical fertilizer inputs in agrosystems include breeding varieties with efficient phosphorus acquisition (Lynch and Brown, 2009), application of natural extracts and plant growth-promoting soil microorganisms (Agbodjato et al., 2015; Assogba et al., 2017; Igiehon and Babalola, 2017; Igiehon and Babalola, 2018; Fasusi et al., 2021).
The rhizosphere is the region of the soil that includes the area immediately around plant roots and a large number of microorganisms (Babalola, 2010). It is also a region with a high turnover of nutrients and a high microbial density where biotic and abiotic factors are under strict control of each other (Enebe and Babalola, 2018; Enagbonma and Babalola, 2019). Examples of microorganisms that can be found in the rhizosphere include rhizobacteria and mycorrhizal fungi (Cruz et al., 2017).
Within the abundant microbiota associated with the root zone of the plant, Arbuscular Mycorrhizal Fungi (AMF) occupy a prominent place. They are beneficial soil microorganisms that establish mutualistic associations with a variety of food crops (Berruti et al., 2016), including maize, by improving soil characteristics and thus promoting plant growth under both normal and stressed conditions (Navarro et al., 2014; Alqarawi et al., 2014; Aguegue et al., 2020). AMF enhance nutrient uptake efficiency, suppression of soil pathogens, tolerance to water, salt and heavy metal stresses, and increase biomass through the production of phytohormones and changes in root morphology (Filho et al., 2017). AMF not only facilitates the process of decomposition of soil organic matter (Paterson et al., 2016), but also affect the plant’s fixation of atmospheric carbon dioxide through the « sink-effect » and the movement of photosynthates from aerial organ to the roots (Begum et al., 2019). Furthermore, the fungus penetrates in roots cortical cells, procedure specific haustoria like structure named as arbuscular, that functions as a mediator for the exchange of the metabolites between fungus and host cytoplasm (Oueslati, 2003). Mycorrhizae could increase the accessibility as well as transport of diffusing ions, for example, P to host plants (McArthur and Knowles, 1993; Sharda and Koide, 2010). Similarly, it shows a vital role in improving soil physical properties (Khan et al., 2020). Mycorrhizae mycelium close to soil microorganisms produces stable aggregate, therefore improve soil aggregation (Singh, 2012). This progress in soil aggregation as a result of the production of an unsolvable mass (glycoprotein) by mycorrhizae (Gadkar and Rillig, 2006), which shows its significant role in the soil firmness (Rillig et al., 2003). Arbuscular mycorrhiza hyphae flourishing in the soil (Bethlenfalvay and Linderman, 1992), supports the plant to obtain water, nutrients, from the soil, in addition, it also develops soil texture (Rillig and Mummey, 2006). It illustrates an important role in the ecosystem through nutrient cycling (Shokri and Maadi, 2009; Wu et al., 2011). Studies have revealed that about 80% of the P taken up through plants is supplied by mycorrhizal fungi (Marschner and Dell, 1994). Arbuscular mycorrhizae could also provide both macros as well as micronutrients for example; N, K, Mg, Zn and Cu mostly in the soil where it occurs in the less soluble form (Marschner and Dell, 1994; Meding and Zasoski, 2008).
Thus, many studies around the world have been carried out in recent years understand the establishment, maintenance and functioning of communities of these fungi under the influence of cultural practices. (Peyret-Guzzon, 2016; Gnamkoulamba et al., 2018a) and are now attracting much more interest in sub-Saharan African countries like Benin (Adjanohoun et al., 2011; Agbodjato et al., 2015; Assogba et al., 2017) to understand how other soil communities (bacteria and fungi) interact with plants.
Maize (Zea mays, L), a predominant crop in Benin (Adjanohoun et al., 2012), is very sensitive to low phosphorus availability (Postma and Lynch, 2011) and has been shown to be amenable to the application of symbiotic soil microorganisms (Rosas et al., 2009; Walker et al., 2011) including AMF (Aguegue et al., 2017; Koda et al., 2018; Assogba et al., 2020a). Hence the urgency of deepening and understanding the functioning, applicability and industrial-scale production of these innovative technologies for sustainable agriculture is advocated. It is within this context that this study was been initiated in order to improve maize productivity while considerably reducing mineral fertilizers through the use of AMF.
2 Materials and Methods
2.1 Materials
Maize seed of the variety 2000 SYN EE W was used during the experiment. It is an extra-early variety with a vegetative cycle of 80 days. It is resistant to breakage, streak, American rust and mildew. It is moderately drought resistant (MAEP, 2016). They are provided by the ‘‘Centre de Recherche Agricole Nord (CRA-Nord)’’ of the ‘‘Institut National de Recherches Agricoles du Bénin (INRAB)’’.
Two families of mycorrhizal fungi: Acaulosporaceae (Acaulospora capsicula, Acaulospora denticulata), Glomeraceae (Funeliformis mosseae, Funeliformis geosporum, Glomus caledonius, Glomus ambiosporum, Rhizophagus intraradices and Septoglomus contrictum), isolated and identified by Davis- INVAM key in the rhizosphere of maize from the Centre of Benin by Assogba et al. (2020a) and then stored in the “Laboratoire de Biologie et de Typage Moléculaire en Microbiologie” were used in this study.
2.2 Methods
2.2.1 Formulation of Biostimulants Based on Arbuscular Mycorrhizal Fungi
The method of Rivera et al. (2003) adapted by Assogba et al. (2020a) was used to formulate biostimulants based on arbuscular mycorrhizal fungi. It consisted of multiplying mycorrhizal fungi by trapping on the roots of a host plant, whole sorghum (Sorghum bicolor L.), grown in pots under controlled greenhouse conditions. Ten previously sterilized sorghum seeds were then sown in each pot containing the different dilution levels of sterile soil. A quantity of 600 spores of the species association of each family (Glomeraceae and Acaulosporaceae) of AMF was put into the pot immediately after sowing. Then, these pots were placed in a greenhouse at room temperature. Daily watering was done for six weeks with sterile distilled water to maintain the soil capacity approximately the same as in the field. For all strains, root biomass and soil were ground to obtain inoculum at the end of the plant’s vegetative cycle. Thus, the aerial part of the plants was cut and the soil-clay-root content was extracted from the pots in a clean container and then powdered after drying to obtain the biostimulant.
2.2.2 Evaluation of the Effect of Arbuscular Mycorrhizal Fungi on The Growth and Yield of Maize in a Controlled Environment (Greenhouse) and a Field Environment (Research Station)
2.2.2.1 Seed Coating With Mycorrhizal Fungi
The coating consisted of weighing 10 kg of seed for 1 kg of biostimulant for each group of fungi. The seeds were mixed with a quantity of water equivalent to 600 ml.kg-1 of biostimulant. The coated seeds were then left in the ambient air for 12 h to dry according to the recommendations of Fernández et al. (2000).
2.2.2.2 Location of the Experimental Site in a Controlled Environment (Greenhouse) and Field Environment
2.2.2.2.1 Controlled Environment (Greenhouse)
The controlled environment experiment was installed in the greenhouse of the Biochemistry Department at the Faculté des Science et Techniques (FAST) of the Université d’Abomey-Calavi (UAC) in Benin. The controlled environment experiment was conducted in December 2019 over a 30-day periode. A thermometer was used to detect the average temperature values inside the greenhouse. The average daytime temperature was 34.45°C and the average night-time temperature was 36.82°C during the trial period.
2.2.2.2.2 Field Environment
The field experiment was conducted in the village of Miniffi (Figure 1), in the commune of Dassa-Zoumè in Centre of Benin. It is located between 7°50.4’ North latitude and 2°10’ East longitude. The commune of Dassa-Zoumè is characterized by Guinean Sudan climate with an annual rainfall regime and wide temperature variations which an annual average of around 28°C (Assogba et al., 2020b). The field environment experiment was conducted in June 2020 over a period of 80 days. The choice was made taking into account the fact that it had hosted trials, that the decline in soil fertility was a priority constraint and that the site was an RD (Research and Development) site. Flat ploughing was carried out the day before sowing on a plot with a maximum slope of 2% and not flooded.
2.2.2.3 Experimental Design In the Greenhouse and Field Condition
In the greenhouse, the experimental design was a split plot of ten treatments with three replicates. A total of 30 pots were installed in the greenhouse
In the field (field environment), the experimental design was the same as the one installed in the greenhouse. It was a split plot of ten treatments with three replications. In total, 30 elementary plots were installed on the experimental site. Each elementary plot had an area of 12.8 m² and consisted of 4 lines of 4 m long with a spacing of 0.80 m. Sowing was done at a spacing of 0.80 m x 0.40 m with 31,250 plants/ha (Yallou et al., 2010). The distance separating the plots from each other was 1.8 m and the replications between them were 2 m, respectively. The useful plot had an area of 6.4 m2, where data were collected on the two (02) central lines.
The different treatments applied in the two conditions were: T1 = Control (no inoculation or mineral fertilizer); T2 = Glomeraceae; T3 = Acaulosporaceae; T4 = 25% NPK+ urea; T5 = Glomeraceae + 25% NPK + urea; T6 = Acaulosporaceae + 25% NPK + urea; T7 = 50% NPK + urea; T8 = Glomeraceae + 50% NPK + urea; T9 = Acaulosporaceae + 50% NPK + urea; and T10 = 100% NPK + urea. Note that the recommended dose of mineral fertilizers (NPK and urea) for maize cultivation in Benin is 200 kg/ha of NPK and 100 kg/ha of Urea (INRAB, 1995). It should also be noted that the NPK used in our study is of the formula N13P17K17. Urea contains 46% nitrogen (N).
2.2.2.4 Sowing, Inoculation and Maintenance of Pots in the Greenhouse
The substrate used was a ferruginous soil collected with a marked holland auger at a depth of 0-20 cm in Miniffi, Central Benin. The substrate was first sterilized at 120°C for 20 minutes and then the same operation was carried out 24 hours later. 2 kg of the substrate was then weighed into the pots (Gholami et al., 2009). Each pot was moistened with 2/9th of the Maximum Water Holding Capacity (MWHC) of the substrate, or 224 ml of sterile distilled water 24 hours before sowing (Eteka, 2005).
Two seeds previously coated with mycorrhizal fungi or not, depending on the treatment, were sown in the pots about 5 cm deep. Fertilizers were then applied on the same day for treatments that received different doses of NPK fertilizer, and Urea was applied on 15th day after sowing (DAS).The pots were then watered every morning with 1/9 of the MWHC, or with 112 ml. The demarcation of one plant per pot was done on the 7th day after sowing (DAS), then the data relative to different parameters to be evaluated were collected until the 30th day after sowing.
2.2.2.5 Sowing, Inoculation and Maintenance of Plots in the Field
At sowing, two (02) maize seeds, previously coated with AMF or not, were placed in a seed hole after a hole about 5 cm deep and the seed hole was immediately closed. Then, fertilizers were applied on the same day as a basal dressing for the treatments that received the different doses of NPK fertilizer and Urea was applied on the 45th day after sowing. Three weeding operations were carried out, the first one coupled with the removal of one plant per seed hole two (02) weeks after sowing. The least vigorous plant was pulled out, and the second and third weeding operations were carried out respectively six (06) and eight (08) weeks after sowing.
2.2.2.6 Chemical Analysis of the Experimental Soil
At the beginning of the experiment, a composite sample of 500 g of soil was collected from each elementary plot at a depth of 0 - 20 cm using a holande auger to determine the chemical characteristics of the experimental soil. Organic carbon was determined by the method of Walkley and Black (1934). The carbon content was determined by colorimetry at a wavelength of 650 nm. Total nitrogen was determined by the method of Kjeldahl (1883), consisting of acid digestion followed by distillation of ammonia (NH3) and its determination. Assimilable phosphorus was extracted by the Bray I method (Bray and Kurtz, 1945). Exchangeable bases (Ca2+, Mg2+, Na+, K+) were extracted by the 1N ammonium acetate extraction method at pH = 7. They were thus displaced by NH4+ ions and then measured using an atomic absorption spectrometer. The cation exchange capacity (CEC) was determined by the Metson (1957) method, which consists of saturating the humus-clay complex with ammonium acetate (1 N) at pH=7. The NH4+ ions were then displaced by a KCl (1N) solution and titrated after distillation.
2.2.3 Assessment of the Growth Parameters in Greenhouse and Field Condition
The growth parameter in the greenhouse and on station was made by measuring the variables: height, crown diameter and leaf area. The height of a corn plant is defined as the distance between the collar and the last ligulated leaf. It was measured with the use of measuring tape. The diameter of the seedlings was measured using a slide caliper and the leaf area was estimated by the affected product length and width of the sheets of coefficient 0.75 according to Ruget et al (1996).
In the greenhouse trial, both variables (plant height and collet diameter) were collected every 96 hours from 7th DAS to 30th DAS, or 7th, 11th, 15th, 19th, 20th and 30th DAS. The leaf area was estimated at the 30th DAS.
In the field condition, the two variables (plant height and collet diameter) were collected every 15 days from the 15th to the 60th day after sowing, or on the 15th, 30th, 45th and 60th DAS. The leaf area was estimated (at the 60th DAS.
2.2.4 Evaluation of Biomass Yield Parameters of Greenhouse Plants (Dry Above-Ground Biomass and Dry Below-Ground Biomass)
2.2.4.1 Greenhouse
At the 30th DAS, the harvesting of the fresh above-ground biomass consisted of cutting the plant flush with the pot using a knife. The contents of the pot (soil + root) were then poured into a container with water. The roots of the maize plants were carefully and thoroughly collected and washed thoroughly with tap water to form the fresh underground biomass.
The precision balance (Highland HCB 3001. Max 3,000 g x 0.1 g) was used to weigh the fresh weight of the different biomass treatments. These biomasses were then placed in an oven at 65°C for 72 hours until the constant weight was obtained (Yadav et al., 2010) for the determination of dry weight (DW). The dry matter (DM) thus corresponds to the ratio between the dry weight (DW) and the weight of the fresh biomass (FB) (in %).
where;
DM = dry matter in %; DW = dry biomass weight of maize plants in kg; FB = fresh biomass weight of maize plants in kg.
2.2.4.2 Field Condition
2.2.4.2.1 Determination of Plant Biomass Yield and Maize Grain Yield
The biomass produced was determined at harvest. Thus, on the two central rows of each elementary plot, 20 maize plants were carefully dug up, while taking care to separate the aerial part (without the maize cobs) from the underground part. Then, the method used in the greenhouse was the same in this case to determine the dry matter of the aerial and underground biomass.
For maize grain yield, the cobs of the previous 20 maize plants were harvested, destemmed and shelled. The percentage moisture content of the grains was determined using a moisture meter (LDS-1F). The average grain yield values of the maize plants were determined by the formula used by Valdés et al. (2013):
Where;
• R = average maize grain yield in t.ha-1; P = maize grain weight in kg.
• 10000 is the conversion from ha to m2; 1000 is the conversion from tonne (t) to kg.
• S = crop area in m²; % H = grain moisture percentage in %.
2.2.5 Estimation of Endomycorrhizal Infection Rates in Greenhouse and Field Conditions
Maize root samples were taken at harvest on 30th DAS for the greenhouse trial and 60th DAS for the field trial for the determination of endo mycorrhizal infection. For this purpose, after staining with Trypan Blue according to the method described by Phillips and Hayman (1970), arbuscular mycorrhizal fungi associated with the roots of maize plants were observed with binoculars (XSP-BM-2CEA.2013). The estimation of mycorrhizal infection of the roots was carried out using the intersection method (Giovannetti and Mosse, 1980; Trouvelot et al., 1986). The mycorrhization rate was estimated by two parameters of arbuscular mycorrhizal infections, namely:
- Mycorrhization frequency (F) determined by the following formula which reflects the degree of infection of the root system (Giovannetti and Mosse, 1980; Trouvelot et al., 1986):
Where N is the number of fragments observed and no is the number of fragments without trace of mycorrhization.
- Mycorrhization intensity: m (absolute mycorrhization intensity) which expresses the portion of the colonized cortex in relation to the whole root system (Giovannetti and Mosse, 1980; Trouvelot et al., 1986) according to the following formula:
In this formula, n5, n4, n3, n2 and n1 are the numbers of fragments respectively noted in the five (05) infection classes marking the importance of mycorrhization namely: 5 = more than 95%, 4= from 50 to 95%, 3 = 30 to 50%, 2 = 1 to 30%, 1 = 1% of cortex.
2.2.6 Statistical Analysis of the Data
The effect of the treatments on the growth and biomass parameters of the maize plants in the field was assessed using one-factor analysis of variance (ANOVA) or a non-parametric Kruskal-Wallis test. The effect of these treatments on the field maize plants was assessed by applying a linear mixed effects model (for growth parameters) and one-way analysis of variance (ANOVA) for the biomass data. In each mixed model, treatments were considered as fixed factors and time as a random factor. The Akaike Information Criterion (AIC) was used to select the best model (Burnham and Anderson, 2004).
Once the tests performed were significant, a post hoc test of pairwise comparisons using the Tuckey post hoc test (Douglas and Michael, 1991) was performed to assess statistical differences.
In order to better describe the different treatments, a Multiple Factor Analysis (MFA) was performed on a set of variables structured in groups (growth and yield).
These analyses were carried out in the R 4.0.2 software (R Core Team, 2020), and required the use of the “nlme” package for model fitting, the ggplot2 package for the creation of box-plots, the “agricolae” package for the Kruskal-Wallis test, the “car” package for the ANOVA, the “multcomp” package for the post hoc pairwise comparison test and the “FactoMineR” package for the MFA. The significance level used is 5%.
3 Results
3.1 Chemical Characteristics of the Soil in the Natural Environment
Table 1 presents the chemical characteristics of the soil at the experimentation site. The soil of the Miniffi experimental site in Centre-Benin was slightly basic (pH = 7.3) at the horizon level (0-20cm). The fertility level of the soil (table) was average and was richer in Ca2+ than in potassium K+ ions. The carbon-nitrogen ratio C/N (12.53) was relatively low at the topsoil level. The phosphorus content at topsoil level (46.73mg/kg soil) was low.1
3.2 Effects of Inoculation of Arbuscular Mycorrhizal Fungi Strains on Maize Growth in a Controlled Environment (Greenhouse)
3.2.1 Effects of AMF Inoculation on Plant Growth Parameters Under Greenhouse
Table 2 illustrates the variation in growth parameters of maize plants under greenhouse conditions at 30 days before harvest. An improvement of the different parameters was observed in the plants treated with the arbuscular mycorrhizal fungi strains combined or not with the different doses of NPK compared to the control plants. Furthermore, it was observed that the effect of the treatments on the plants varied significantly.
Indeed, the highest values of height were recorded within the plants treated with Acaulosporaceae+25%NPK+Urea (T6), followed by those treated with Glomeraceae+25%NPK+Urea (T5) with respective increases of 63.74% and 50% compared to the control plants (T1). In treatments T8 (Glomeraceae+50%NPK+Urea), T9 (Acaulosporaceae+50%NPK+Urea) and T10 (100%NPK+Urea) the plant heights were close to those of treatments T6 (Acaulosporaceae+25%NPK+Urea) and T5 (Glomeraceae+25%NPK+Urea).
In the assessment of the diameter at the collar of the plants, treatments T6 (Acaulosporaceae+25%NPK+Urea) and T5 (Glomeraceae+25%NPK+Urea) presented significantly (p <0.01) higher values, with respective increases of 61.53% and 50% compared to treatment T1 (Control).
As for leaf area, treatments T5 (Glomeraceae+25%NPK+Urea) and T7 (50%NPK+Urea) had a significant effect inducing the largest leaf areas (p<0.01). This was followed by treatments T2 (Glomeraceae) and T10 (100%NPK+Urea), which also had leaf areas closer to treatment T7 (50%NPK+Urea). These results showed the positive impact of biostimulants on the growth variables measured on maize plants in a controlled environment.
3.2.2 Effects of Inoculation of Endogenous Strains on Growth Variables
3.2.2.1 Evaluation of the Effect of Mycorrhizal Fungi on the Biomass Produced in a Controlled Environment (Greenhouse)
The dry above-ground and dry underground biomass of maize plants obtained varied significantly between treatments. The results of the different treatments revealed a highly significant difference (P < 0.001) in performance between treatments for all biomass yield parameters (Table 3). The highest values of dry above-ground biomass were obtained with treatment T5 (Glomeraceae + 25%NPK-Urea) with improvements of 78.57% compared to treatment T1 (Control) and 29.53% compared to treatment T10 (100%NPK+Urea). For dry underground biomass, treatment T6 (Acaulosporaceae + 25% NPK+ urea) with an increase of 103.17% compared to T1 (Control) and 38.83% compared to treatment T10 (100%NPK+Urea), was the most effective. Therefore, we can note that the biostimulant Glomeraceae improves the dry above-ground biomass when combined with the recommended dose of 25% NPK+Urea while the biostimulant Acaulosporaceae also combined with the recommended dose of 25% NPK+Urea improves the dry root biomass.
3.3 Effects of Inoculation of Arbuscular Mycorrhizal Fungi Strains on Maize Plant Growth and Yield Under Field Conditions
3.3.1 Effects of Mycorrhizal Fungi on Maize Plant Growth Under Field Conditions
3.3.1.1 Height (cm)
The effects of the treatments on maize plants were evaluated over a period of sixty (60) days at regular 15-day intervals (Figure 2). Thus, in the field, between the 15th and 30th day after sowing (DAS), the plants had synchronous growth with similar values for the parameters assessed. Between the 30th and the 45th day after sowing, differentiations are observed. It can be seen that treatments T5 (Glomeraceae + 25% NPK+ urea) and T8 (Glomeraceae + 50% NPK+ Urea) induce the best performance in height (p-value < 0.001). After 45 days, the variations observed between the 30th and 45th days of the season became more pronounced with the T8 treatment (Glomeraceae + 50% NPK+ urea), which induced the greatest height values with an increase of 96.26% compared to the control plants (T1). Also, a demarcation was observed between the treatment that received the biostimulant combined with half dose of NPK (T8 =Glomeraceae + 50% NPK+ urea) and the treatment that received the full dose of NPK recommended (T10 = 100% NPK+ urea) with an increase of 17.87%.
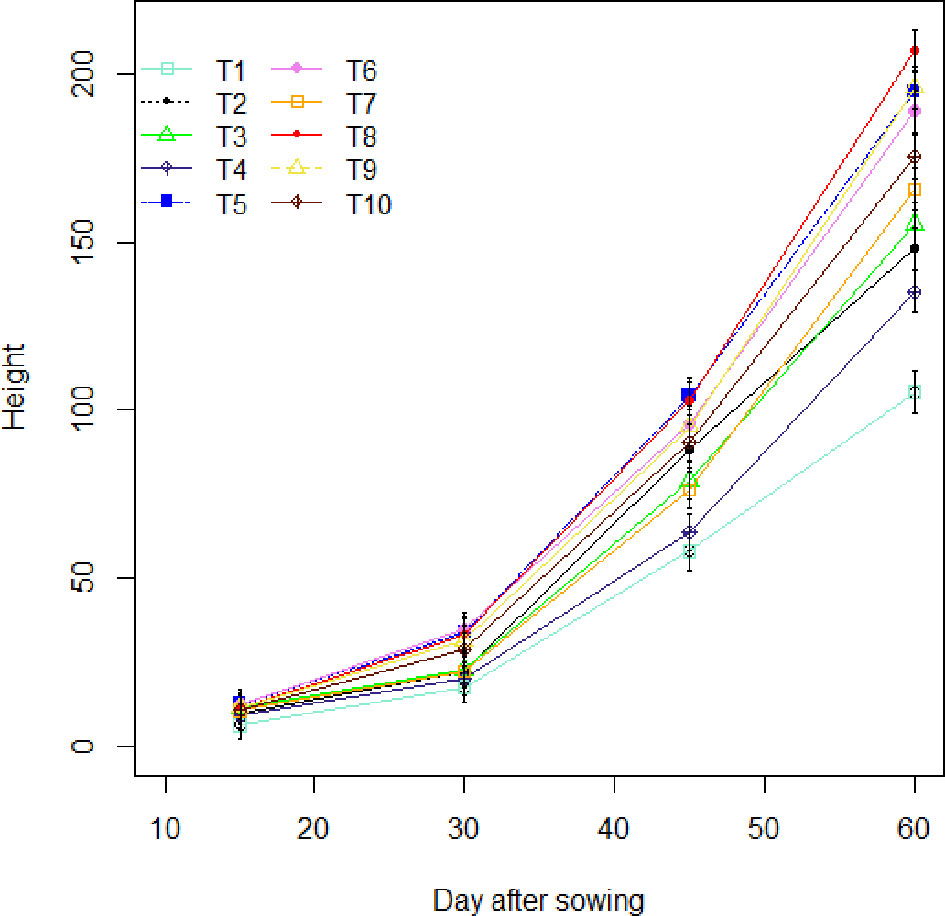
Figure 2 Height variation as a function of field-world treatments. T1, Control; T2, Glomeraceae; T3, Acaulosporaceae; T4, 25% NPK + urea; T5, Glomeraceae + 25% NPK+ urea; T6, Acaulosporaceae + 25% NPK+ urea; T7, 50% NPK + urea; T8, Glomeraceae + 50% NPK+ urea ; T9, Acaulosporaceae + 50% NPK+ urea; T10, 100% NPK+ urea.
3.3.1.2 Diameter at Collar (cm)
Figure 3 illustrates that the time-treatment interaction has a significant effect on plant diameters (p-value < 0.001). The results of the seedling analysis revealed that the highest values in diameter were recorded with treatment T5 (Glomeraceae + 25%NPK-Urea) while the lowest values in diameter were obtained with treatment T1 (Control). A difference of 70.07% was noted between treatments T5 (Glomeraceae + 25%NPK-Urea) and T1 (Control) from the 45th day of the season onwards and of 20.72% between the treatment (T5=Glomeraceae + 25%NPK-Urea) that provided plants with larger diameter values at the crown and the treatment that received the full recommended dose of NPK (T10 = 100% NPK+ urea). These results indicate an improvement in this trait following the application of the biostimulant in combination with a quarter of the recommended NPK dose compared to the application of the full NPK dose.
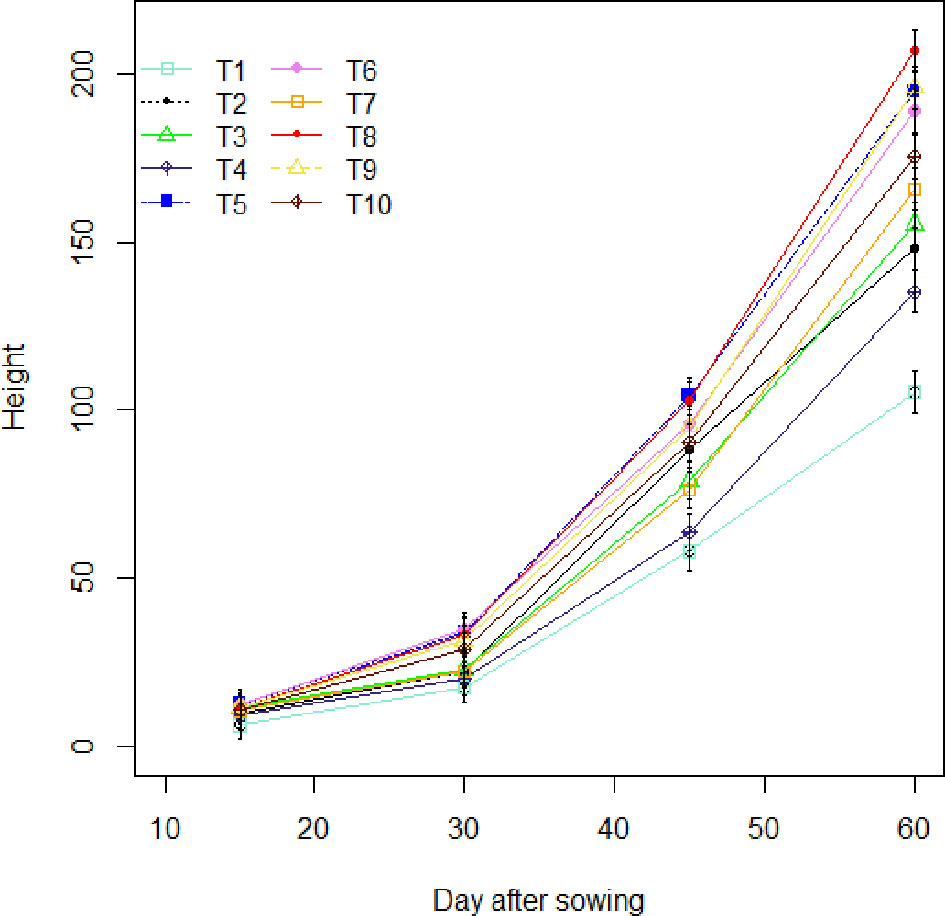
Figure 3 Variation in diameter as a function of field treatments. T1, Control; T2, Glomeraceae; T3, Acaulosporaceae; T4, 25% NPK + urea; T5, Glomeraceae + 25% NPK+ urea; T6, Acaulosporaceae + 25% NPK+ urea; T7, 50% NPK + urea; T8, Glomeraceae + 50% NPK+ urea; T9, Acaulosporaceae + 50% NPK+ urea; T10, 100% NPK+ urea.
3.3.1.3 Leaf Area (cm2)
Table 4 shows the results of leaf area measurements of maize plants inoculated or not with endogenous arbuscular mycorrhizal fungi (AMF). The results show that the leaf area of the plants varies according to the type of inoculum used. The inoculation of maize plants with Glomeraceae and Acaulosporaceae improved the leaf area of the plants. Statistical analysis showed a significant effect (p-value < 0.005) between the different treatments. The highest values in leaf area were recorded with treatment T6 (Acaulosporaceae + 25% NPK+ Urea) with an increase of 44.06% compared to treatment T1 (Control) and 14.35% compared to treatment T10 (100%NPK+Urea). We also found that contrary to the first two growth parameters (height and crown diameter), the plants with a large leaf area in the natural environment were those that received the T6 treatment (Acaulosporaceae + 25% NPK+ Urea).
3.3.2 Effects of Mycorrhizal Fungi on Maize Plant Biomass and Grain Yield in Field Conditions
Table 5 shows the mean dry above-ground biomass, dry below-ground biomass and grain yield of maize plants recorded under field conditions. A significant effect was noted on all three variables measured. The highest values (Table 5) for dry above-ground biomass, dry below-ground biomass and grain yield were obtained with the Glomeracea+25%NPK-Urea treatment compared to the control plants with increases of 51.26%, 136.36% and 65.28%, respectively. It should be noted that these different mean values per parameter vary significantly (p-value < 0.01). Table 5 shows the different treatment groups with similar effects per parameter. From the analysis of this table, it appears that treatments T5 (Glomeraceae + 25% NPK+ Urea), T6 (Acaulosporaceae + 25% NPK+ Urea) and T8 (Glomeraceae + 50% NPK+ Urea) gave the highest values in dry above-ground biomass, followed by treatments; T4 (25% NPK + Urea), T9 (Acaulosporaceae + 50% NPK+ urea) and T10 (100% NPK+ urea) have similar effects, less important than those of the first batch, followed by the group of T2 (Glomeraceae) and T3 (Acaulosporaceae) which have effects very close to the performances obtained with the T1 treatment (Control) On the other hand, treatment T5 (Glomeraceae + 25% NPK+ Urea) induced the best performance in dry below-ground biomass. A group composed of treatments T6 (Acaulosporaceae + 25% NPK+ urea), T7 (50% NPK + Urea), T8 (Glomeraceae + 50% NPK+ Urea), T9 (Acaulosporaceae + 50% NPK+ Urea), T10 (100% NPK+ Urea), produced plants with biomasses relatively close to those of the plants subjected to treatment T5 (Glomeraceae + 25% NPK+ Urea). Treatments T2 (Glomeraceae), T3 (Acaulosporaceae) and T4 (25% NPK + Urea) had relatively less effect on the below-ground biomass of the plants than the previous group. Again, treatment T1 (Control) caused the lowest values of below-ground biomass. In terms of grain yield, treatments T5 (Glomeraceae + 25% NPK+ Urea), T6 (Acaulosporaceae + 25% NPK+ Urea), T8 (Glomeraceae + 50% NPK+ Urea) and T10 (100% NPK+ Urea) had the highest yield. It was found that the group composed of treatments T4 (25% NPK+ Urea), T7 (50% NPK + Urea) and T9 (Acaulosporaceae + 50% NPK+ Urea) as well as that composed of treatments T2 (Glomeraceae) and T3 (Acaulosporaceae) were similar to each other, and the lowest values were recorded with treatment T1 (Control). The highest values for dry above-ground biomass, dry root biomass and grain yield were obtained with the Glomeraceae biostimulant when combined with the recommended dose of 25%NPK-Urea. Also, when comparing these values obtained with the best treatment (T5=Glomeraceae + 25% NPK+ Urea) and the treatment receiving the full recommended dose of NPK (T10 = 100% NPK+ Urea), we note demarcations of 7.71%, 4% and 3.23%, respectively recorded on the variables dry above-ground biomass, dry below-ground biomass and grain yield.
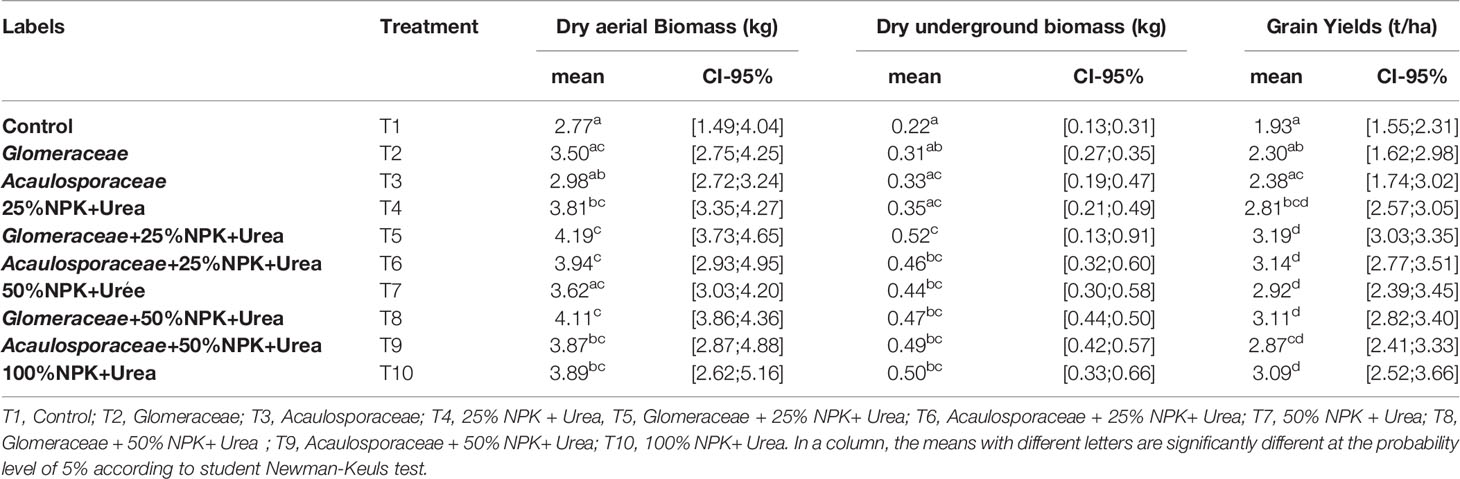
Table 5 Influence of endogenous strains on the variables of biomass produced and grain yield according to the type of treatment in the field.
3.3.3 Effect of Mycorrhizal Fungi on the Projection of Variables And Treatments in the First Two Dimensions of Multiple Factor Analysis
The proportion of variance explained by the first two dimensions (axes) is estimated at 93.2%. Figure 4 shows the association between plant growth and yield variables and treatments, as well as the correlation between variables and dimensions and between treatments and dimensions. All variables are positively correlated to the first dimension. Height, biomass and grain yield are the most correlated. The first dimension represents the high values of yield and height. The variables that are relatively correlated in the second dimension are crown diameter and leaf area.
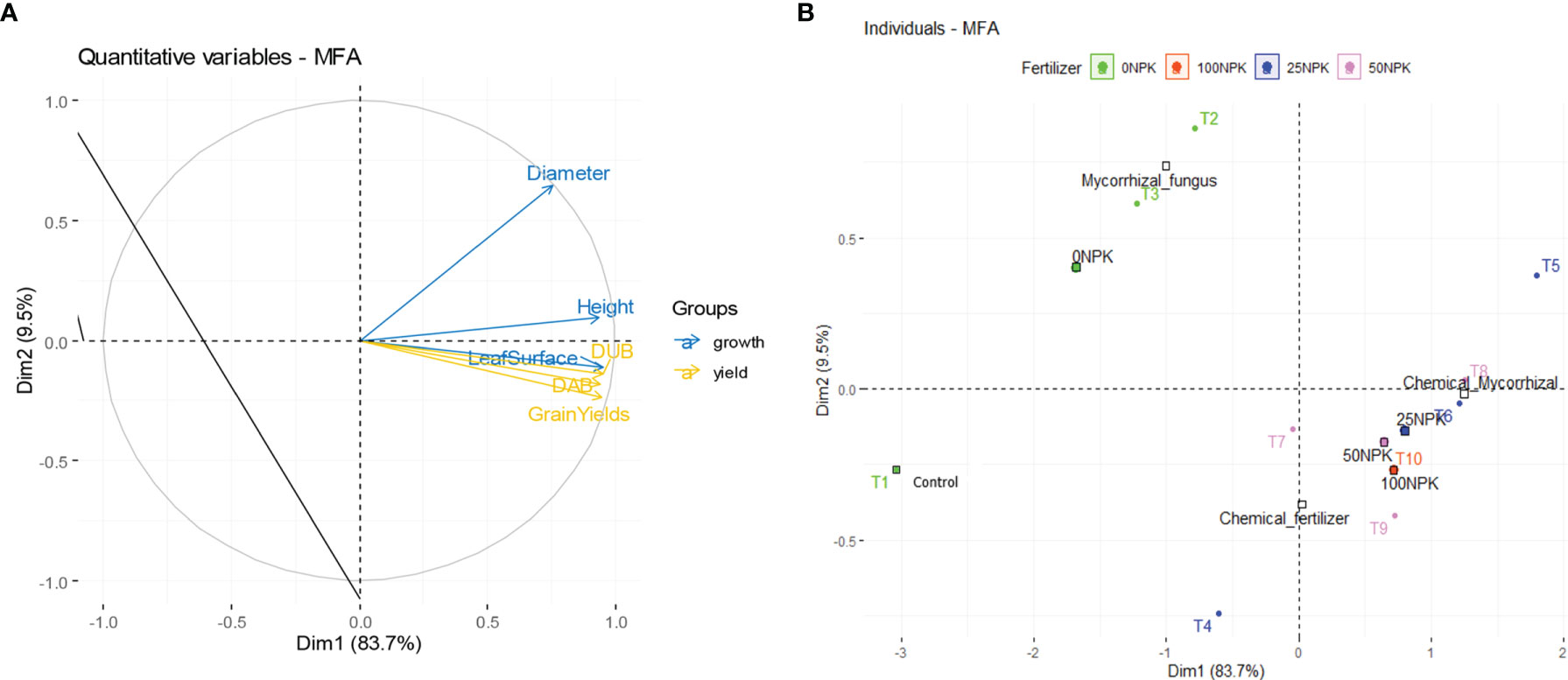
Figure 4 Projections of variables (A) and treatments (B) in the first two dimensions of the Multiple Factor Analysis (MFA).
In fact, the first dimension mainly opposes treatments T5 (Glomeraceae+25%NPK+Urea), T6 (Acaulosporaceae +25%NPK+Urea) and T8 (Glomeraceae +50%NPK+Urea) to treatment T1 (Control). Thus, it appears that the treatments based on the combination of arbuscular mycorrhizal fungi and chemical fertilizers, especially at 25% NPK, gave high values of the different growth and yield parameters, whereas the farmers’ practices produced low growth and yield values. It is deduced that arbuscular mycorrhizal fungi (especially Glomeraceae) ensure good plant performance when combined with low doses of chemical fertilizers (25%NPK+Urea).
The second axis is essentially associated with treatments T4 (25%NPK+Urea), T7 (50%NPK+Urea) and T10 (100%NPK+Urea) characterized by a high value of leaf area, which are essentially treatments based on chemical fertilizers at various doses, as opposed to treatments T2 (Glomeraceae) and T3 (Acaulosporaceae) characterized by an increase in diameters, consisting of treatments based solely on arbuscular mycorrhizal fungi. It was found that chemical fertilizers had a greater effect on the leaf development of the plants than did the fungi, whereas the arbuscular mycorrhizal fungi had a significant effect on the development of the plant diameters.
These results indicate that the arbuscular mycorrhizal fungi were very beneficial to the plants by ensuring good development and yield, and to the farmers by allowing them to reduce the use of chemical fertilizers and, in turn, to reduce economic expenses.
3.3.3.1 Impact of Mycorrhizal Fungi on Mycorrhization Parameters in the Greenhouse and in the Field
The intensity and frequency of mycorrhization varied between the different treatments (Figure 5). Analysis of the radar plot showing mycorrhization parameters by treatment shows that mycorrhization frequencies are mostly higher than mycorrhization intensities in the greenhouse and the field, as shown in the figures. Indeed, the strains without mineral fertilizers (NPK and urea) give the highest mycorrhization frequencies and intensities in the field and in the greenhouse. We noted that these frequencies and intensities decrease as soon as the quantity of mineral fertilizers increases. This is the case for the treatments ‘Glomeraceae+50%NPK+Urea’ and ‘Acaulosporaceae+50%NPK+Urea’, which give the lowest mycorrhization frequencies and intensities in the greenhouse and in the field.
Note that the Glomeraceae strain gave the highest mycorrhization frequency and intensity in the field and the highest mycorrhization frequency in the greenhouse, followed by Acaulosporaceae. The highest mycorrhization intensity in the greenhouse was obtained with the Acaulosporaceae strain.
4 Discussion
In tropical zone, the management of natural soil fertility is one of the major challenges that agricultural actors must face to transform their eùpirical and subsistence-based agriculture into sustainable, economical, more rational and income-generating agriculture (Malonda et al., 2019).
This study aims to improve the growth and grain yield of maize through the use of arbuscular mycorrhizal fungi (AMF) while reducing excessive doses of mineral fertilizers applied.
In Benin, maize grows typically under conditions of well-distributed rainfall of 400 to 600 mm, an optimum temperature of 28 to 35°C on deep ferrallitic, ferruginous or vertisol soils with pH values ranging from 5.5 to 7 (INRAB, 1995). The results of the chemical analysis of the soils revealed that the soil samples are slightly alkaline (pH = 7.3) at the horizon (0-20cm). The soil fertility (Table 1) is medium and was richer in Ca2+ than in potassium K+ ions. The carbon-nitrogen ratio C/N was relatively low at the topsoil level. Phosphorus content at the topsoil level (46.73mg/kg soil) was also low. These soils are therefore conducive for a good expression of arbuscular mycorrhizal fungi regarding their effectiveness on the different variables collected. These results are supported by the work of Gabriel and Cristina (2007).
Indeed, for adequate development of plant, it is necessary that nutrients are available in enough and balanced quantities (Yayeh and Melkamu, 2017). To achieve that plants convert light energy (from certain sunlight waves or other light sources) into chemical energy to ensure their metabolism (Ndonda, 2018). Thus, this chemical energy is stored into sugars by the plants through photosynthesis. This photosynthesis takes place within characteristic plant cell structures (chloroplasts) and gives plants some ability to convert energy from sunlight and carbon dioxide into sugars and oxygen (Ndonda, 2018). The mycorrhizal fungi in turn, use this sugar from photosynthesis for their own growth and promote better acquisition of unaccessible minerals within the soil where in one hand roots cannot reach and in other hand the proliferation of some non-symbiotic microorganisms involved in biogeochemical cycles release useful and available minerals for plants (Evelin et al., 2012).
Also, these microorganisms stimulate the root development allowing the acquisition of water in-depth for the benefit of the plant. The measured growth variables show improved mineral nutrition of the plant, indicating the effectiveness of the biostimulants in the acquisition of minerals necessary for the growth of the plant in a both controlled and field environment (Pagano, 2014).
In the greenhouse, treatments that received the biostimulant improved growth variables compared to control plants and even those that received the full recommended dose of mineral fertilizer. A significant difference (P<0.001) was noted in the growth variables (Height, Collar diameter and Leaf area). The highest height values were obtained in plants treated with Acaulosporaceae+25%NPK+Urea (T6) followed by those treated with Glomeraceae+25%NPK+Urea (T5) with respective increases of 63.74% and 50% compared to the control plants (T1).
Regarding the growth in diameter at the collar of the plants, treatments T6 (Acaulosporaceae+25%NPK+Urea) and T5 (Glomeraceae+25%NPK+Urea) presented significantly (p<0.01) the highest values, with respective increases of 61.53% and 50% compared to treatment T1 (Control).
As for the leaf area, treatments T5 (Glomeraceae+25%NPK+Urea) and T7 (50%NPK+Urea) presented the highest values (p<0.01) with respective increases of 47.99% and 32.75% compared to treatment T1 (Control). These results show the ameliorative effect of biostimulants on growth variables measured on maize plants in a controlled environment and are supported by those of many authors in Benin (Assogba et al., 2017; Bossou et al., 2019; Koda et al., 2020; Aguégué et al., 2021) and in other countries (Leye et al., 2015; Igiehon and Babalola, 2021) who have highlighted the positive effect of biostimulants on each plant organ and in turn on the plant.
Also, these biostimulants induced high values in produced biomass (dry above-ground biomass and dry below-ground biomass). The highest values in dry above-ground biomass of maize plants were obtained with the biostimulant Glomeraceae combined with 25%NPK-Urea with an increase of 78.57% compared to the control treatment and of 29.53% compared to the T10 treatment (100%NPK+Urea). Regarding dry subterranean biomass, the highest values were obtained with the biostimulant Acaulosporaceae with an increase of 103.17% compared to T1 (Control) and 38.83% compared to T10 treatment (100%NPK+Urea). These results show the ameliorative effect of biostimulants on the biomass produced and can be explained by the fact that the biostimulants fit well to their ecosystem and improve the availability of water and mineral elements to the maize plants as it is demonstrated that he weak concentrations of nutrients limit the growth of and the productivity of the crops (Shen et al., 2016). Furthermore, AMF may have produced auxin that can modulate growth and root architecture (Gamalero et al., 2004), and exopolysaccharides that may solubilize phosphate and maintain water film necessary for photosynthetic activity and plant growth (Sharma et al., 2013; Tarraf et al., 2017). We can therefore say that these biostimulants used in this study are effective in improving biomass yields while reducing the use of mineral fertilizers. The same observations were made by Koda et al. (2018); Assogba et al. (2020c) and Aguégué et al. (2021) in Benin and by Gnamkoulamba et al. (2018a) in Togo who proved the effectiveness of biostimulants on rice growth and yield.
In addition, observation of the growth of maize plants carried out in a field environment after inoculation of the plants with the two endogenous strains of arbuscular mycorrhizal fungi revealed that inoculated plants were better developed than those not inoculated.
The height values of the plants at 30 days after inoculation were similar. A significant difference was observed between the 30th and 45th day of growth with the biostimulant Glomeraceae. After 45 days, the variations observed between the 30th and the 45th day of the plant’s life became more pronounced with the T8 treatment (Glomeraceae + 50% NPK+ Urea), which induced high height values with an increase of 96.26% compared to the control plants (T1) and a rise of 17.87% compared to the treatment that received the full dose of NPK recommended (T10 = 100% NPK+ Urea)
Regarding the variables neck diameters and leaf area, they were improved respectively by treatments T5 (Glomeraceae + 25%NPK+ Urea) and T6 (Acaulosporaceae + 25% NPK+ Urea) with an increase of 44.06% compared to treatment T1 (Control) and 14.35% compared to treatment T10 (100%NPK+ Urea). These results explain the effectiveness of biostimulants (Glomeraceae and Acaulosporaceae) in improving the growth parameters measured through the good absorption of water and essential nutrients needed by maize plants for their development. We have highlighted the same observations in our studies (Assogba et al., 2017; Assogba et al., 2020b; Aguégué et al., 2021) in Benin.
These results are consistent with the work of some authors (Atul-Nayyar et al., 2009; Haro et al., 2012; Djatta et al., 2013; Haougui et al., 2013; Haro et al., 2015; Manga et al., 2017), who highlighted the improvement of mineral element uptake (phosphorus and nitrogen), nutrition and growth of plants associated with mycorrhizae.
For the yield variables of produced biomass (above-ground and dry below-ground biomass) and grain yield, we found a very highly significant difference (P < 0.001) between treatments for all measured yield variables (Table 5). The highest values for above-ground dry biomass, below-ground dry biomass and grain yield were obtained with the Glomeracea+25%NPK-Urea treatment compared to the control plants with increases of 51.26%, 136.36% and 65.28% respectively. It should be noted that these different average values per parameter vary significantly at the 5% threshold (p-value < 0.01). Gnamkoulamba et al. (2018b) made the same observations on rice. They recorded significant total dry biomass of rice and indicated an increasing trend with AMF inoculation. Moreover, Wahbi et al. (2016) found that mycorrhizal inoculation had a significantly positive effect on the shoot dry weights and total shoot N in faba bean,but not in wheat. Zhang et al. (2018) proved the involvement of MACs in improving biomass allocation to different plant organs through increased redistribution of phosphorus and nitrogen to grains. Furthermore, Haro et al. (2016) and Haro et al. (2017) demonstrated that plant growth and production are improved by mycorrhizal symbiosis even when these plants are growing on relatively mineral-poor soils and therefore exploitation of this symbiosis would be beneficial.
These results recorded at the level of growth and yield variables can be explained by a good absorption of mineral elements (Sharma et al., 2017; Aguégué et al., 2021) and a good accommodation of the strains, their resistance to observed area of drought (Aroca et al., 2007; Assogba et al., 2020c; Aguegue et al., 2020) or their resistance to specific root and leaf pathogens (Campos-Soriano et al., 2011). In terms of productivity, growth improvements may lead to a better yield (Raklami et al., 2019)
Mycorrhization rate is estimated by two arbuscular mycorrhizal infection parameters (mycorrhization frequency and mycorrhization intensity). The mycorrhization frequency (F) reflects the degree of infection of the root system, i.e., it gives us information on the number of mycorrhized root fragments observed and the number of fragments without any trace of mycorrhization on a total of one hundred (100) roots observed. The mycorrhization intensity expresses the portion of the colonized cortex in relation to the whole root system.
The results of the analyses reveal high frequencies and intensities of mycorrhization observed in the greenhouse and in the field, which explain the results obtained in the variables of growth, biomass produced and grain yield recorded on the mycorrhized plants, which present the highest values compared to the non-mycorrhized plants. These results are similar to those of Tian et al. (2013) showed 76-80% colonization of maize roots after inoculation.
Indeed, it should be noted that beyond 12% mycorrhization intensity, the benefits derived by the plant symbiont are interesting (Oliveira et al., 2006), which shows a strong accommodation between the strains and the host plant. This adaptability would be related to their endogenous origin (Benjelloun et al., 2014). Out of the two endogenous strains used in our study, Glomeraceae-induced plants gave the highest values, especially in a field environment for all variables. Therefore, this strain proved the effectiveness of AMF-based biostimulants.
5 Conclusion
The purpose of this study was to test the fertilizing potential of two endogenous AMF on the growth and grain yield parameters of maize under greenhouse and field conditions.
Results from this study showed that the arbuscular mycorrhizal fungi strains used had the ability to colonize the rhizosphere in both the greenhouse and field. It also showed a high ability to infect the roots of maize plants and improve all variables of the inoculated plants. The Acaulosporacea + 25% NPK+ Urea and Glomeracea +25%NPK-Urea treatments induced the best plant growth and corn grain yields compared to control plants under greenhouse and field conditions, respectively.
These obtained results demonstrated that mycorrhizal inoculums were able to adapt not only with chemical fertilizers but also with the native soil microflora.These results showed that the mycorrhization of maize with the different endogenous strains of Arbuscular Miccorrhizal Fungi (AMF) could improve the productivity of maize plant while discouraging excessive use of mineral fertilizers. Field experiments on larger areas need to be repeated to further establish this technology and later develop arbuscular mycorrhizal fungi-based biostimulants forsustainable agriculture in Benin.
Data Availability Statement
The original contributions presented in the study are included in the article/supplementary material. Further inquiries can be directed to the corresponding author.
Author Contributions
NA, SA, AK and RA carried out the experimental work and analysis. NA, SA, HS, GD, AA and LB-M contributed to the design, supervision, and interpretation of the results. NA, SA, and OB revised the final draft. OB reviewed and edited the final draft. All authors participated equally in the work and approved the final submission.
Conflict of Interest
The authors declare that the research was conducted in the absence of any commercial or financial relationships that could be construed as a potential conflict of interest.
Publisher’s Note
All claims expressed in this article are solely those of the authors and do not necessarily represent those of their affiliated organizations, or those of the publisher, the editors and the reviewers. Any product that may be evaluated in this article, or claim that may be made by its manufacturer, is not guaranteed or endorsed by the publisher.
Acknowledgments
The authors gratefully acknowledge the Programme d’Amelioration de la Productivité Agricole des Petits Exploitants (PAPAPE) of the Institut National des Recherches Agricoles du Bénin (INRAB), the Laboratoire de Biologie et de Typage Moléculaire en Microbiologie (LBTMM) and the National Research Foundation, South Africa Grants (UID123634) to OB. NA is grateful for North-West University Postdoctoral support.
References
Adjanohoun A., Baba-Moussa L., Glèlè kakaï R., Allagbé M., Yèhouénou B., Gotoechan-Hodonou H., et al. (2011). Caractérisation Des Rhizobactéries Potentiellement Promotrices De La Croissance Végétative Du Maïs Dans Différents Agrosystèmes Du Sud-Bénin. Int. J. Biol. Chem. Sci. 5, 433–444. doi: 10.4314/ijbcs.v5i2.72073
Adjanohoun A., Noumavo P. A., Sikirou R., Allagbé M., Gotoechan-Hodonou H., Dossa K. K., et al. (2012). Effets Des Rhizobactéries PGPR Sur Le Rendement Et Les Teneurs En Macroéléments Du Maïs Sur Sol Ferrallitique non Dégradé Au Sud-Bénin. Int. J. Biol. Chem. Sci. 6, 279–288. doi: 10.4314/ijbcs.v6i1.24
Agbodjato N. A., Noumavo P. A., Adjanohoun A., Dagbénonbakin G., Atta M., Falcon Rodriguez A., et al. (2015). Response of Maize (Zea Mays L.) Crop to Biofertilisation With Plant Growth Promoting Rhizobacteria and Chitosan Under field Conditions. JEBAS 3, 566–574. doi: 10.18006/2015.3(6).566.574
Aguégué M. R., Ahoyo Adjovi R., Agbodjato A.N., Noumavo P., Allagbé M., Chabi N.W., et al. (2020). Greenhouse Evaluation of the Growth of Zea Mays L. Inoculated by Arbuscular Mycorrhizal Fungi Strains in Native Arbuscules on Ferrous Soil. IJACS. 8, 55–63. doi:–10.33495/jacr_v8i3.20.106
Aguégué R. M., Assogba S. A., Salami H. A. A., Koda D. A., Agbodjato N. A., Amogou, et al. (2021). Organic Fertilizer Based on Rhizophagus Intraradices: Valorization in a Farming Environment for Maize in the South, Center and North of Benin. Front. Sustain. Food Syst. 5, 605610. doi: 10.3389/fsufs.2021.605610
Aguégué M. R., Noumavo A.P., Dagbénonbakin G., Agbodjato A. N., Assogba S., Koda A.D., et al. (2017). Arbuscular Mycorrhizal Fertilization of Corn (Zea Mays L.) Cultivated on Ferrous Soil in Southern Benin. JAS. 5, 99–115. doi: 10.5296/jas.v5i3.11881
Alqarawi A., Hashem A., Abd_Allah E., Alshahrani T., Huqail A. (2014). Effect of Salinity on Moisture Content, Pigment System, and Lipid Composition in Ephedra Alata Decne. Acta Biol. Hung. 65, 61–71. doi: 10.1556/abiol.65.2014.1.6
Aroca R., Porcel R., Ruiz-lozano J. M. (2007). How Does Arbuscular Mycorrhizal Symbiosis Regulate Root Hydraulic Properties and Plasma Membrane Aquaporins In Phaseolus Vulgaris Under Drought, Cold or Salinity Stresses? New Phytol. 173, 808–816. doi: 10.1111/j.1469-8137.2006.01961.x
Assogba A. S., Agbodjato A. N., Ahoyo Adjovi N., Sina H., Noumavo A. P., et al. (2020b). Effect of the Combination Rhizophagus Intraradices and 50% of NPK on Maize (Zea Mays L.) Growth and Yield in Central Benin. Int. J. Agron. Agric. R. 16, 1–15.
Assogba A. S., Ahoyo Adjovi N., Agbodjato A. N., Sina H., Adjanohoun A., Baba-moussa L. (2020a). Evaluation of the Mixed Effect of Some Indigenous Strains of Arbuscular Mycorrhizal Fungi Under Greenhouse Conditions. Eur. Sci. J. 16, 275. doi: 10.19044/esj.2020.v16n3p275
Assogba A. S., Ahoyo Adjovi N., Bello S., Noumavo A. P., Sina H., Agbodjato A. N., et al. (2020c). Inoculation of Native AMF Based Bio-Fertilizers for Improvement of Maize Productivity in the Center of Benin. Afr. J. Agric. 16, 652–660. doi: 10.5897/AJAR2019.14638
Assogba S., Noumavo A. P., Dagbenonbakin G., Agbodjato A. N., Akpode C., Koda D. A., et al. (2017). Improvement of Maize Productivity (Zea Mays L.) by Mycorrhizal Inoculation On Ferruginous Soil in Center of Benin. Int. J. Sus. Agric. 4, 63–76. doi: 10.18488/journal.70.2017.43.63.76
Atul-Nayyar A., Hamel C., Hanson K., Germida J. (2009). The Arbuscular Mycorrhizal Symbiosis Links N Mineralization to Plant Demand. Mycorrhiza 19, 239–246. doi: 10.1007/s00572-008-0215-0
Igiehon N.O., Babalola O.O. (2017). Biofertilizers and Sustainable Agriculture: Exploring Arbuscular Mycorrhizal Fungi. Appl. Microbiol. Biotechnol 101, 4871–4881. doi: 10.1007/s00253-017-8344-z
Vienna: R Foundation for Statistical Computing. Available at: www.R-project.org (Accessed January 25, 2021).
Babalola O. O. (2010). Beneficial Bacteria of Agricultural Importance. Biotechnol. Lett. 32, 1559–1570. doi: 10.1007/s10529-010-0347-0
Balogoun I., Saïdou A., Ahoton L. E., Adjanohoun A., Amadji Ezui G. L. G., Youl S., et al. (2013). “Détermination Des Formules D’engrais Minéraux Et Des Périodes De Semis Pour Une Meilleure Production Du Maïs (Zea Mays L.) Au Sud Et Au Centre Bénin,” in Bulletin De La Recherche Agronomique Du Bénin (BRAB) Numéro Spécial. Fertilité du maïs–Janvier2013 BRAB en ligne (online)surlesitewebhttp://www.slire.net Bibliothèque Nationale du Bé́nin, Bénin.
Battini F., Grønlund M., Agnolucci M., Giovannetti M., Jakobsen I. (2017). Facilitation of Phosphorus Uptake In Maize Plants by Mycorrhizosphere Bacteria. Sci. Rep. 7, 4686. doi: 10.1038/s41598-017-04959-0
Begum N., Qin C., Ahanger M. A., Raza S., Khan M. I., Ashraf M., et al. (2019). Role of Arbuscular Mycorrhizal Fungi in Plant Growth Regulation: Implications in Abiotic Stress Tolerance. Front. Plant Sci. 10. doi: 10.3389/fpls.2019.01068
Benjelloun S., El Harchli E. H., Amrani Joutei K., El Ghachtouli N., Fikri Benbrahim K., Yamani J. (2014). Etude De L’importance De La Mycorhization Dans La Synthèse Des Composés Phénoliques Chez Le Maïs (Zea Mays L.) En Condition De Stress Hydrique. Int. J. Eng. Sci. 6:1559. www.researchinventy.com.
Berruti A., Lumini E., Balestrini R., Bianciotto V. (2016). Arbuscular Mycorrhizal Fungi as Natural Biofertlizers: Let’s Benefit From Past Successes. Front. Microbiol. 6. doi: 10.3389/fmicb.2015.01559
Bethlenfalvay G., Linderman R. (1992). “Mycorrhizae in Sustainable Agriculture,” in American Society of Agronomy, vol. 54. (Madison, Wisconsin: ASA special publication).
Bossou L. D. R., Houngnandan H. B., Adandonon A., Zoundji C., Houngnandan P. (2019). Diversité Des Champignons Mycorhiziens Arbusculaires Associés a La Culture Du Maïs (Zea Mays L.) Au Bénin. Int. J. Biol. Chem. Sci. 13, 597–609. doi: 10.4314/ijbcs.v13i2.2
Burnham K. P., Anderson D. R. (2004). Multimodel Inference: Understanding AIC and BIC in Model Selection, Sociological Methods and Research, p. 261–304. doi: 10.1177%2F0049124104268644
Bray R. H., Kurtz L. T. (1945). Determination of Total Organic and Available Forms of Phosphorus in Soils. Soil Sci. 59, 39–45. doi: 10.1097/00010694-194501000-00006
Campos-Soriano L., Garcia-Martinez J., Segundo B. S. (2011). The Arbuscular Mycorrhizal Symbiosis Promotes the Systemic Induction of Regulatory Defence-Related Genes in Rice Leaves and Confers Resistance to Pathogen Infection. Mol. Plant Pathol. 13, 579–592. doi: 10.1111/j.1364-3703.2011.00773
Cruz C., Ramos A., Babalola O.O., Kamel H., Dias T., Varma A., et al (2017). Soil: Do Not Disturb, Mycorrhiza in Action. In: Varma A., Prasad R., Tuteja N. (eds) Mycorrhiza - Function, Diversity, State of the Art. Springer, Cham. doi: 10.1007/978-3-319-53064-2_3
Djatta M. B., Manzo O. L., Diouf P. R. M., Diop T. (2013). Effets De L’inoculation Mycorhizienne Sur Le Sesame (Sesamum Indicum L.) En Conditions Naturelles. Int. J. Biol. Chem. Sci. 7, 2050–2057. doi: 10.4314/ijbcs.v7i5.22
Douglas C. E., Michael F. A. (1991). On Distribution-Free Multiple Comparisons in the One-Way Analysis of Variance. Commun. Statist. Theory Methods 20, 127–139. doi: 10.1080/03610929108830487
Emmanuel O. C., Babalola O. O. (2020). Productivity and Quality of Horticultural Crops Through Co-Inoculation of Arbuscular Mycorrhizal Fungi and Plant Growth Promoting Bacteria. Microbiol. Res. 239, 126569. doi: 10.1016/j.micres.2020.126569
Enagbonma B. J., Babalola O. O. (2019). Environmental Sustainability: A Review of Termite Mound Soil Material and its Bacteria. Sustainability 11, 3847. doi: 10.3390/su11143847
Enebe M. C., Babalola O. O. (2018). The Influence of Plant Growth-Promoting Rhizobacteria in Plant Tolerance to Abiotic Stress: A Survival Strategy. Appl. Microbiol. Biotechnol. 102, 7821–7835. doi: 10.1007/s00253-018-9214-z
Eteka A. C. (2005). Contribution Des « Jachères » Manioc Dans L'amé́lioration Du Rendement Des Cultures Et Du Prélèvement Des Nutriments: Cas De La Succession Culturale Manioc-Maïs Au Centre Du Bénin. Thèse de DEA, Faculté des Sciences Agronomiques (FSA), Université d'Abomey-Calavi (UAC), Calavin, Bénin. 107 p.
Evelin H., Giri B., Kapoor R. (2012). Contribution of Glomus Intraradices Inoculation to Nutrient Acquisition and Mitigation of Ionic Imbalance in NaCl-Stressed Trigonella Foenum-Graecum. Mycorrhiza. 22, 203–217. doi: 10.1007/s00572-011-0392-0
Fasusi O., Amoo A., Babalola O. O. (2021). Propagation and Characterization of Viable Arbuscular Mycorrhizal Fungal Spores Within Maize Plant (Zea Mays L.). J. Sci. Food Agric. 101, 5834–5841. doi: 10.1002/jsfa.11235
Fernández F., Go´mez R., Vanegas L. F., Martynez M. A., de la Noval B. M., Rivera R. (2000). “Producto Inoculante Micorrizogeno,” in Oficina Nacional De Propiedad Industrial. Patente no. 22641, Cuba,
Filho J. A. C., Sobrinho R. R., Rascholati S. F. (2017). “Arbuscular Mycorrhizal Symbiosis and its Role In Plant Nutrition In Sustainable Agriculture,” in Agriculturally Important Microbes for Sustainable Agriculture. Eds. Meena V. S., Mishra P., Bisht J., Pattanayak A. (Singapore: Springer). doi: 10.1007/978-981-10-5343-6_5
Gabriel R., Cristina P. (2007). Mecanismos De Adaptación De Maíz Asociado a Glomus Spp Vol. 25 (Agronomía Colombiana: En uelos con bajo fósforo disponible), 349–356.
Gadkar V., Rillig M. C. (2006). The Arbuscular Mycorrhizal Fungal Protein Glomalin is a Putative Homolog of Heat Shock Protein 60. FEMS Microbiol. Lett. 263, 93–101. doi: 10.1111/j.1574-6968.2006.00412.x
Gamalero E., Trotta A., Massa N., Copetta A., Martinotti M. G., Berta G. (2004). Impact of Two fluorescent Pseudomonads and an Arbuscular Mycorrhizal Fungus on Tomato Plant Growth, Root Architecture and P Acquisition. Mycorrhiza 14, 185–192. doi: 10.1007/s00572-003-0256-3
Gholami A., Shahsavani S., Nezarat S. (2009). The Effect of Plant Growth Promoting Rhizobacteria (PGPR) on Germination, Seedling Growth and Yield of Maize. World Acad. Sci. Eng. Technol. 49, 19–24.
Giovannetti M., Mosse B. (1980). An Evaluation of Techniques for Measuring Vesicular–Arbuscular Infection in Roots. New Phytol. 84, 489–500. doi: 10.1111/j.1469-8137.1980.tb04556.x
Gnamkoulamba A., Tounou A.K., Tchao M., Tchabi A., Adjevi A.K. M., Batawila K., et al. (2018a). Evaluation Au Champ Du Potentiel De Croissance Et De La Production Du Riz (Oryza Sativa l.) Variété IR841 Inoculé en Pépiniére Par Quatre Souches De Champignons Mycorhiziens Á Arbuscules. Eur. Sci. J. 14 (12):452–481. doi: 10.19044/esj.2018.v14n12p452
Gnamkoulamba A., Tounou A. K., Tchao M., Tchabi A., Adjevi A., Kossi M., et al. (2018b). Prévalence Et Diversité Des Spores Des Champignons Mycorhiziens Arbusculaires En Culture De Riz Sous Les Différents Systèmes De Culture De Riz Au Togo. J. Appli. Biosci. 126, 12647–12664. doi: 10.4314/jab.v126i1.3
Haougui A., Souniabe P. S., Doumma A., Adam T. (2013). Evolution Des Populations Des Champignons Endomycorhiziens Sur Les Adventices De Quatre Sites Maraîchers De La Région De Maradi Au Niger. Int. J. Biol. Chem. Sci. 7, 554–565. doi: 10.4314/ijbcs.v7i2.12
Haro H., Sanon K. B., Blagna F., Fofana B. (2016). Effect of Native Arbuscular Mycorrhiza Fungi Inocula On The Growth Of Cowpea [Vigna Unguiculata (L.) Walp.] in Three Differents Agro Ecological Zones In Burkina Faso. J. Appli. Biosci. 108, 10553–10560. doi: 10.4314/jab.v108i1.8
Haro H., Sanon K. B., Diop I., Kane A., Dianda M., Houngnandan P., et al. (2012). Réponse a L'inoculation Mycorhizienne De Quatre Variétés De Niébé [Vigna Unguiculata (L.) Walp.] Cultivées Au Burkina Faso Et Au Sénégal. Inter. J. Biol. Chem. Sci. 6, 2097–2112. doi: 10.4314/ijbcs.v6i5.18
Haro H., Sanon K. B., Krasova-Wade T., Kane A., N’Doye I., Traoré A. S. (2015). Réponse a La Double Inoculation Mycorhizienne Et Rhizobienne Du Niébé (Variété, KVX396-4-5-2D) Cultivé Au Burkina Faso. Inter J. Biol. Chem. Sci. 9, 1485–1493. doi: 10.4314/ijbcs.v9i3.31
Haro H., Sanon K. B., Le Roux C., Duponnois R., Traoré A. S. (2018). Improvement of Cowpea Productivity by Rhizobial and Mycorrhizal Inoculation in Burkina Faso. Symbiosis, 74, 107–120. doi: 10.1007/s13199-017-0478-3
Igiehon N. O., Babalola O. O. (2018a). Rhizosphere Microbiome Modulators: Contributions of Nitrogen Fixing Bacteria Towards Sustainable Agriculture. Int. J. Environ. Res. Public. Health 15, 574. doi: 10.3390/ijerph15040574
Igiehon N.O., Babalola O.O. (2018b) Below-Ground-Above-Ground Plant-Microbial Interactions: Focusing on Soybean, Rhizobacteria and Mycorrhizal Fungi". Open Microbiol. J. 12, 261—279. doi: 10.2174/1874285801812010261
Igiehon O. N., Babalola O. O. (2021). Rhizobium and Mycorrhizal Fungal Species Improved Soybean Yield Under Drought Stress Conditions. Curr. Microbiol. 78, 1615–1627. doi: 10.1007/s00284-021-02432-w
Igiehon N. O., Babalola O. O., Cheseto X., Torto B. (2021). Effects of Rhizobia and Arbuscular Mycorrhizal Fungi on Yield, Size Distribution and Fatty Acid of Soybean Seeds Grown Under Drought Stress. Microbiol. Res. 242, 126640. doi: 10.1016/j.micres.2020.126640
INRAB (1995). Fiche Technique Sur Les Cultures Vivrières Vol. 75 (Bénin: INRAB/MAEP: Institut National des Recherches du Bénin).
Khan A., Ding Z. T., Ishaq M., Khan I., Ahmed A. A., Khan A. Q., et al. (2020). Applications of Beneficial Plant Growth Promoting Rhizobacteria and Mycorrhizae in Rhizosphere and Plant Growth: A Review. Int. J. Agric. Biol. Eng. 13 (5), 199–208. doi: 10.25165/j.ijabe.20201305.5762
Kjeldahl J. (1883). A New Method for the Determination of Nitrogen in Organic Matter. Z. Anal. Chem. 22, 366–382. doi: 10.1007/BF01338151
Koda D. A., Dagbenonbakin G., Agbodjato A. N., Akpode C., Assogba S., et al. (2018). Maize (Zea Mays L.) Response to Mycorrhizal Fertilization on Ferruginous Soil of Northern Benin. J. Exp. Biol. Agric. Sci. 6, 919–928. doi: 10.18006/2018.6(6).919.928
Koda A. D., Dagbénonbakin G., Assogba F., Agbodjato N. A., N'Tcha C., Assogba S., et al. (2020). Impact of Native Arbuscular Mycorrhizal Fungi Based Fertilizers on to Increase Maize Productivity in North Benin. African Journal of Agricultural Research, 16 (9), 1298–1306. doi: 10.5897/AJAR2020.14758
Leye E. H. M., Ndiaye M., Diouf M., Diop T. (2015). Etude Comparative De L’effet De Souches De Champignons Mycorhiziens Arbusculaires Sur La Croissance Et La Nutrition Minérale Du Sésame Cultivé Au Sénégal. Afri. Crop Sci. J. 23, 211–219.
Lynch L.P., Brown K. M. (2009). Root Strategies for Phosphorus Acquisition. In: White P.J., Hammond J.P. (eds) The Ecophysiology of Plant-Phosphorus Interactions. Plant Ecophysiology, vol 7. Springer, Dordrecht. doi: 10.1007/978-1-4020-8435-5_5
MAEP (2016) Catalogue Béninois Des Espèces Et Variétés Végétales (CaBEV), 2ème Edn. Ministère De L’Agriculture De l’Elevage Et De La Peche; INRAB/DPVPPAAO/ProCAD/MAEP & CORAF/WAAPP, Dépôt Légal N ◦ 8982 Du 21 Octobre 2016, Bibliothèque Nationale Du Bénin, 4ème Trimestre. Available at: http://inrab.org/wp-content/uploads/2018/01/CaBEV- interactif-2.pdf (Accessed January 25, 2021).
Malonda A. N., Nzola-meso T. M., Manga A. M., Yandju M. C. (2019). Effet Des Champignons Mycorhiziens Arbusculaires Sur Le Phosphore Des Sols Tropicaux Et Implication Dans La Biosynthèse Du Caroténoïde Du Manioc. J. Appli. Biosci. 135, 137. doi: 10.4314/jab.v135i1.2
Manga A., Ndiaye F., Diop T. A. (2017). Le Champignon Arbusculaire Glomus Aggregatum Améliore La Nutrition Minérale De Acacia Seyal Soumis Au Stress Salin Progressif. Int. J. Biol. Chem. Sci. 11, 2352–2365. doi: 10.4314/ijbcs.v11i5.32
Marschner H., Dell B. (1994). Nutrient Uptake in Mycorrhizal Symbiosis. Plant Soil. 159, 89–102. doi: 10.1007/BF00000098
McArthur D. A., Knowles N. R. (1993). Influence of Species of Vesicular-Arbuscular Mycorrhizal Fungi and Phosphorus Nutrition on Growth, Development, and Mineral Nutrition of Potato (Solanum Tuberosum L.). Plant Physiol. 102, 771–782. doi: 10.1104/pp.102.3.771
Meding S., Zasoski R. (2008). Hyphal-Mediated Transfer of Nitrate, Arsenic, Cesium, Rubidium, and Strontium Between Arbuscular Mycorrhizal Forbs and Grasses From a California Oak Woodland. Soil Biol. Bioch. 40, 126–134. doi: 10.1016/j.soilbio.2007.07.019
Metson A. J. (1957). Methods of Chemical Analysis for Soil Survey Samples. Soil Sci. 83, 245. doi: 10.1097/00010694-195703000-00016
Navarro J. M., Perez–Tornero O., Morte A. (2014). Alleviation of Salt Stress in Citrus Seedling Inoculated With Arbuscular Mycorrhizal Fungi Depends On The Rootstock Tolerance. J. Plant Physiol. 171, 76–85. doi: 10.1016/j.jplph.2013.06.006
Ndonda A. (2018). “Evaluations Agronomiques Des Champignons Mycorhiziens Locaux Sur La Productivité Du Manioc (Manihot Esculenta Crantz) En Sols Dégradés Des Jachères Herbeuses a Kisangani/ R.D,” in Congo. Agronomie (Français: Université de Kisangani).
Oliveira R. S., Castro P. M. L., Dodd J. C., Vosátka M. (2006). Different Native Arbuscular Mycorrhizal Fungi Influence the Coexistence of Two Plant Species in a Highly Alkaline Anthropogenic Sediment. Plant Soil 287, 209. doi: 10.1007/s11104-006-9067-6
Oueslati O. (2003). Allelopathy in Two Durum Wheat (Triticum Durum L.) Varieties. Agri. Ecosys. Environ. 96, 161–163. 7. doi: 10.1016/S0167-8809(02)00201-3
Pagano M. C. (2014). “Drought Stress and Mycorrhizal Plant,” in Use of Microbes for The Alleviation of Soil Stresses, vol. 1 . Ed. Miransari M. (New York, NY, USA: Springer), 97–110.
Paterson E., Sim A., Davidson J., Daniell T. J. (2016). Arbuscular Mycorrhizal Hyphae Promote Priming of Native Soil Organic Matter Mineralization. Plant Soil 408, 243–C254. doi: 10.1007/s11104-016-2928-8
Peyret-Guzzon M., Stockinger H., Bouffaud M-L., Farcy P., Wipf D., Redecker D., et al. (2016). Arbuscular Mycorrhizal Fungal Communities and Rhizophagus Irregularis Populations Shift in Response to Short-Term Ploughing and Fertilisation in a Buffer Strip. Mycorrhiza. 26, 33–46. doi: 10.1007/s00572-015-0644-5
Phillips J. M., Hayman D. S. (1970). Improved Procedures for Clearing Roots and Staining Parasitic and Vesicular-Arbuscular Mycorrhizal Fungi for Rapid Assessment of Infection. Trans. Br. Mycol. Soc. 55 (1), 158–IN18. doi: 10.1016/S0007-1536(70)80110-3
Plenchette C., Clermont-Dauphin C., Meynard J. M., Fortin J. A. (2005). Managing Arbuscular Mycorrhizal Fungi in Cropping Systems. Canad. J. Plant Sci. 85, 31–40. doi: 10.4141/P03-159
Postma J. A., Lynch J. P. (2011). Theoretical Evidence for the Functional Benefit of Root Cortical Aerenchyma in Soils With Low Phosphorus Availability. Ann. Bot. 107, 829–841. doi: 10.1093/aob/mcq199
Raklami A., Bechtaoui N., Tahiri A., Anli M., Meddich A., Oufdou K. (2019). Use of Rhizobacteria and Mycorrhizae Consortium in the Open Field as a Strategy for Improving Crop Nutrition, Productivity and Soil Fertility. Front. Microbiol. 10. doi: 10.3389/fmicb.2019.01106
R Core Team (2020). R: A Language and Environment for Statistical Computing. R Foundation for Statistical Computing, Vienna, Austria.
Rillig M. C., Maestre F. T., Lamit L. J. (2003). Microsite Differences in Fungal Hyphal Length, Glomalin, and Soil Aggregate Stability in Semiarid Mediterranean Steppes. Soil Biol. Bioch. 35, 1257–1260. doi: 10.1016/S0038-0717(03)00185-8
Rillig M. C., Mummey D. L. (2006). Mycorrhizas and Soil Structure. New Phytol. 171, 41–53. doi: 10.1111/j.1469-8137.2006.01750.x
Rivera R., Fernández F., Hernández-Jiménez A., Martín J. R., Fernández K. (2003). “El Manejo Efectivo De La Simbiosis Micorrízica, Una Vía Hacia La Agricultura Sostenible,” in Estudio De Caso, El Caribe. Eds. Rivera R. Y., Fernández K. (La Habana: Ediciones Instituto Nacional de Ciencias Agrícolas), 1–42.
Rosas S. B., Avanzini G., Carlier E., Pasluosta C., Pastor N., et al. (2009). Root Colonization and Growth Promotion of Wheat and Maize by Pseudomonas Aurantiaca SR1. Soil. Biol. Biochem. 41, 1802–1806. doi: 10.1016/j.soilbio.2008.10.009
Ruget F., Bonhomme R., Chartier M. (1996). Estimation Simple De La Surface Foliaire De Plantes De Maïs En Croissance. Agronomie 16, 553–562. doi: 10.1051/agro:19960903
Sharda J., Koide R. (2010). Exploring the Role of Root Anatomy in P-Mediated Control of Colonization by Arbuscular Mycorrhizal Fungi. Botany 88, 165–173. doi: 10.1139/B09-105
Sharma S., Prasad R., Varma A., Sharma A. K. (2017). Glycoprotein Associated With Funneliformis Coronatum, Gigaspora Margarita and Acaulospora Scrobiculata Suppress the Plant Pathogens In Vitro. Asian J. Plant Pathol. 11, 192–202. doi: 10.3923/ajppaj.2017.199.202
Sharma S. B., Sayyed R. Z., Trivedi M. H., Gobi T. A. (2013). Phosphate Solubilizing Microbes: Sustainable Approach for Managing Phosphorus Deficiency in Agricultural Soils. Springerplus 2, 587. doi: 10.1186/2193-18012-587
Shen H., He X., Liu Y., Chen Y., Tang J., Guo T. (2016). A Complex Inoculant of N2-fixing, P- and K-Solubilizing Bacteria From a Purple Soil Improves the Growth of Kiwifruit (Actinidia Chinensis) Plantlets. Front. Microbiol. 7. doi: 10.3389/fmicb.2016.00841
Shokri S., Maadi B. (2009). Effects of Arbuscular Mycorrhizal Fungus on the Mineral Nutrition and Yield of Trifolium Alexandrinum Plants Under Salinity Stress. J. Agron. 8, 79–83. doi: 10.3923/ja.2009.79.83
Singh P. K. (2012). Role of Glomalin Related Soil Protein Produced by Arbuscular Mycorrhizal Fungi: A Review. Agric. Sci. Res. J. 2, 119–125.
Tarraf W., Ruta C., Tagarelli A., De Cillis F., De Mastro G. (2017). Influence of Arbuscular Mycorrhizae on Plant Growth, Essential Oil Production and Phosphorus Uptake of Salvia Officinalis L. Ind. Crops Prod. 102, 144–153. doi: 10.1016/j.indcrop.2017.03.010
Tian H., Drijber R. A., Li X., Miller D. N., Wienhold B. J. (2013). Arbuscular Mycorrhizal Fungi Differ in Their Ability to Regulate the Expression of Phosphate Transporters in Maize (Zea Mays L.). Mycorrhiza 23, 507–514. doi: 10.1007/s00572-013-0491-1
Trouvelot A., Kough J. L., Gianinazzi-Pearson V. (1986). “Mesure Du Taux De Mycorhization VA D’un Système Radiculaire. Recherche De Méthode D’estimation Ayant Une Signification Fonctionnelle,” in Physiological and Genetical Aspects of Mycorrhizae: Proceedings of the 1st European Symposium on Mycorrhizae, Dijon, 217–221. INRA, Paris, France
Valdés E. M. F., González E. C., Serrano M. M., Labrada H. R., Báez E. M., Hernández F. G., et al. (2013). Experiencias Obtenidas En El Desarrollo Participativo De Híbridos Lineales Simples De Maíz (Zea Mays, L.) En Condiciónes De Bajos Insumos Agrícolas. Cult. Tropicales. 34, 61–69. Available at: http://scielo.sld.cu/pdf/ctr/v34n2/ctr10213.pdf
Wahbi S., Maghraoui T., Ha M., Sanguin H., Oufdou K., Prin Y., et al. (2016). Enhanced Transfer of Biologically fixed N From Faba Bean to Intercropped Wheat Through Mycorrhizal Symbiosis. Appl. Soil Ecol. 107, 91–98. doi: 10.1016/j.apsoil.2016.05.008
Walker V., Bertrand C., Bellvert F., Moenne-Loccoz Y., Bally R., et al. (2011). Host Plant Secondary Metabolite Profiling Shows a Complex, Strain-Dependent Response of Maize to Plant Growth-Promoting Rhizobacteria of the Genus Azospirillum. New Phytol. 189, 494–506. doi: 10.1111/j.1469-8137.2010.03484
Walkley A., Black I. A. (1934). An Examination of the Degtjareff Method for Determining Soil Organic Matter and a Proposed Modification of the Chromic Acid Titration Method. Soil Sci. 37, 29–38. doi: 10.1097/00010694-193401000-00003
Wu Q., Li G., Zou Y. (2011). Roles of Arbuscular Mycorrhizal Fungi on Growth and Nutrient Acquisition of Peach (Prunus Persica L. Batsch) Seedlings. J. Anim. Plant Sci. 21, 746–750.
Yadav Y., Verma J. P., Tiwari K. N. (2010). Effect of Plant Growth Promoting Rhizobacteria on Seed Germination and Plant Growth Chickpea (Cicer Arietinum L.) Under in Vitro Conditions,” Biological Forum, vol. 2, 15–18. Satya Prakashan, New Delhi, India.
Yallou C. G., Aïhou K., Adjanohoun A., Toukourou M., Sanni O. A., Ali D. (2010). Itinéraires Techniques de Production de Maïs au Bénin. Fiche technique. Dépôt légal N ◦ 4922 du 3 Décembre, Bibliothèque Nationale du Bénin, 18.
Yang S., Li F., Malhi S. S., Wang P., Dongrang S., Wang J. (2004). Long Term Fertilization Effects on Crop Yield and Nitrate Nitrogen Accumulation in Soil in Northwestern China. Agron. J. 96, 1039–1049. doi: 10.2134/agronj2004.1039
Yayeh B., Melkamu A. (2017). Impact of Crop Production Inputs on Soil Health-a Review. Asian J. Plant Sci. 16, 109–131. doi: 10.3923/ajps.2017.109.131
Keywords: biostimulant, productivity, Maize, mycorrhizal fungi, Benin, Sustainable Agriculture
Citation: Agbodjato NA, Assogba SA, Babalola OO, Koda AD, Aguégué RM, Sina H, Dagbénonbakin GD, Adjanohoun A and Baba-Moussa L (2022) Formulation of Biostimulants Based on Arbuscular Mycorrhizal Fungi for Maize Growth and Yield. Front. Agron. 4:894489. doi: 10.3389/fagro.2022.894489
Received: 11 March 2022; Accepted: 23 May 2022;
Published: 20 June 2022.
Edited by:
Rahul Mahadev Shelake, Gyeongsang National University, South KoreaReviewed by:
Rajesh Singhal, Indian Grassland and Fodder Research Institute (ICAR), IndiaSaisamorn Lumyong, Chiang Mai University, Thailand
Copyright © 2022 Agbodjato, Assogba, Babalola, Koda, Aguégué, Sina, Dagbénonbakin, Adjanohoun and Baba-Moussa. This is an open-access article distributed under the terms of the Creative Commons Attribution License (CC BY). The use, distribution or reproduction in other forums is permitted, provided the original author(s) and the copyright owner(s) are credited and that the original publication in this journal is cited, in accordance with accepted academic practice. No use, distribution or reproduction is permitted which does not comply with these terms.
*Correspondence: Olubukola Oluranti Babalola, b2x1YnVrb2xhLmJhYmFsb2xhQG53dS5hYy56YQ==