- 1New York State Integrated Pest Management Program, Cornell University, Cornell AgriTech, Geneva, NY, United States
- 2School of Food and Agriculture, University of Maine, Orono, ME, United States
- 3Soil and Crop Sciences, School of Integrative Plant Science, Cornell University, Ithaca, NY, United States
- 4United States Department of Agriculture — Natural Resources Conservation Service (USDA-NRCS) Soil Health Division, Big Flats Plant Materials Center, Corning, NY, United States
- 5Department of Natural Resources and the Environment, University of New Hampshire, Durham, NH, United States
- 6Agroécologie, INRAE, Institut Agro, Univ. Bourgogne, Univ. Bourgogne Franche-Comté, Dijon, France
We reviewed the timing of the peak rate of emergence for 15 problematic weed species as well as ways to use this knowledge to improve control. Much of the previous literature modeled emergence based on growing-degree-days. For these models, we input average temperature data from several zones of Northeast USA. Within species, model-predicted peak emergence in the warmest and coolest zones differed by an average of 39 days. Also within species, there was some variation between models, likely reflecting different conditions in study locations and population-level differences that will need to be addressed in future modelling efforts. Summarizing both observed and modelled results, emergence typically peaked early-season for barnyardgrass, Canada thistle, common lambsquarters, common ragweed, giant foxtail, large crabgrass, perennial sowthistle, and smooth crabgrass. Emergence typically peaked mid-season for hairy galinsoga, mouseear chickweed, and red sorrel. Emergence typically peaked late-season for annual bluegrass. Several species emerged in a protracted manner, including common chickweed, quackgrass, and redroot pigweed. With this improved knowledge, farmers may target key problematic species of a particular field in several ways. Weed seedling control efforts can be timed at the highest densities or most vulnerable phenological stage. Residual herbicides and suppressive mulches can be timed to maximize effectiveness prior to their breakdown. And if management flexibility allows, crop selection and associated planting dates may be adjusted to improve crop competition or facilitate seedbank depletion through timely bare fallow periods. Such improvements to weed management based on timing of emergence will likely become even more impactful as predictive model reliability continues to improve.
Introduction
The timing of weed emergence is among the most important variables determining how species respond to management (Ryan et al., 2010; Cordeau et al., 2017c). This is especially pertinent for weeds germinating from seed, which are most vulnerable to management at the time of emergence (Mohler, 2001). The timing of emergence can also correlate with other biological traits of weeds that impact management success, such as seed weight, cotyledon type, and photosynthetic pathway (Cordeau et al., 2017b). Therefore, improved knowledge of weed emergence periodicity may be used to enhance management tactics (Bastiaans et al., 2008; Norsworthy et al., 2012; Reinhardt Piskackova et al., 2021). For example, the timing of weed control efforts (Forcella, 1999; Batlla et al., 2020), crop planting dates (Royo-Esnal et al., 2018; Sousa-Ortega et al., 2020), and seedbank depletion tactics (Nordell and Nordell, 2009) may all be adjusted to disadvantage problematic weed species.
But emergence periodicity varies greatly between species, reflecting contrasting patterns of dormancy, germination requirements, and pre-emergence growth rates, which are mediated by many abiotic factors (Batlla et al., 2020) so that even within species, empirically observed patterns of emergence can range widely (Cordeau et al., 2017b; Cordeau et al., 2017a). To improve management, modeling efforts have attempted to predict cumulative emergence for individual species, typically using Weibull or Gompertz functions (Royo-Esnal et al., 2020) with growing-degree-days (Forcella et al., 1997; Renner et al., 1999; Sousa-Ortega et al., 2020) or cooling-degree-days (Taylor et al., 2021) calculated using base temperatures required for germination of each modeled species (Masin et al., 2012). Addition of base water potential (Cao et al., 2011; Šoštarčić et al., 2021) or other edaphic parameters (Archer et al., 2006; Davis et al., 2013) may be used to further improve model accuracy.
In previous work investigating emergence periodicity at several sites in Northeast USA, Cordeau et al. (2017b) tilled new plots every two weeks and recorded weed densities by species. It was observed that some weed species exhibited prominent emergence peaks (Figure 1), which may be targeted for improved management. Emergence peaked early for most summer annual species, including common lambsquarters (Chenopodium album L.), common ragweed (Ambrosia artemisiifolia L.), giant foxtail (Setaria faberi Herrm.), large crabgrass [Digitaria sanguinalis (L.) Scop.], and smooth crabgrass (Digitaria ischaemum Schreb. ex Muhl.). But redroot pigweed (Amaranthus retroflexus L.), barnyardgrass [Echinochloa crus-galli (L.) Beauv.], and hairy galinsoga (Galinsoga quadriradiata Cav.) demonstrated a delayed emergence peak compared to the other summer annuals. Winter annuals, including common chickweed [Stellaria media (L.) Vill.] and annual bluegrass (Poa annua L.), as well as perennials, including mouseear chickweed (Cerastium fontanum Baumg.), perennial sowthistle (Sonchus arvensis L.), red sorrel (Rumex acetosella L.), and quackgrass [Elymus repens (L.) Gould] generally exhibited later or bimodal emergence peaks. The exception was the perennial, Canada thistle [Cirsium arvense (L.) Scop.], which emerged early, and for an extended period.
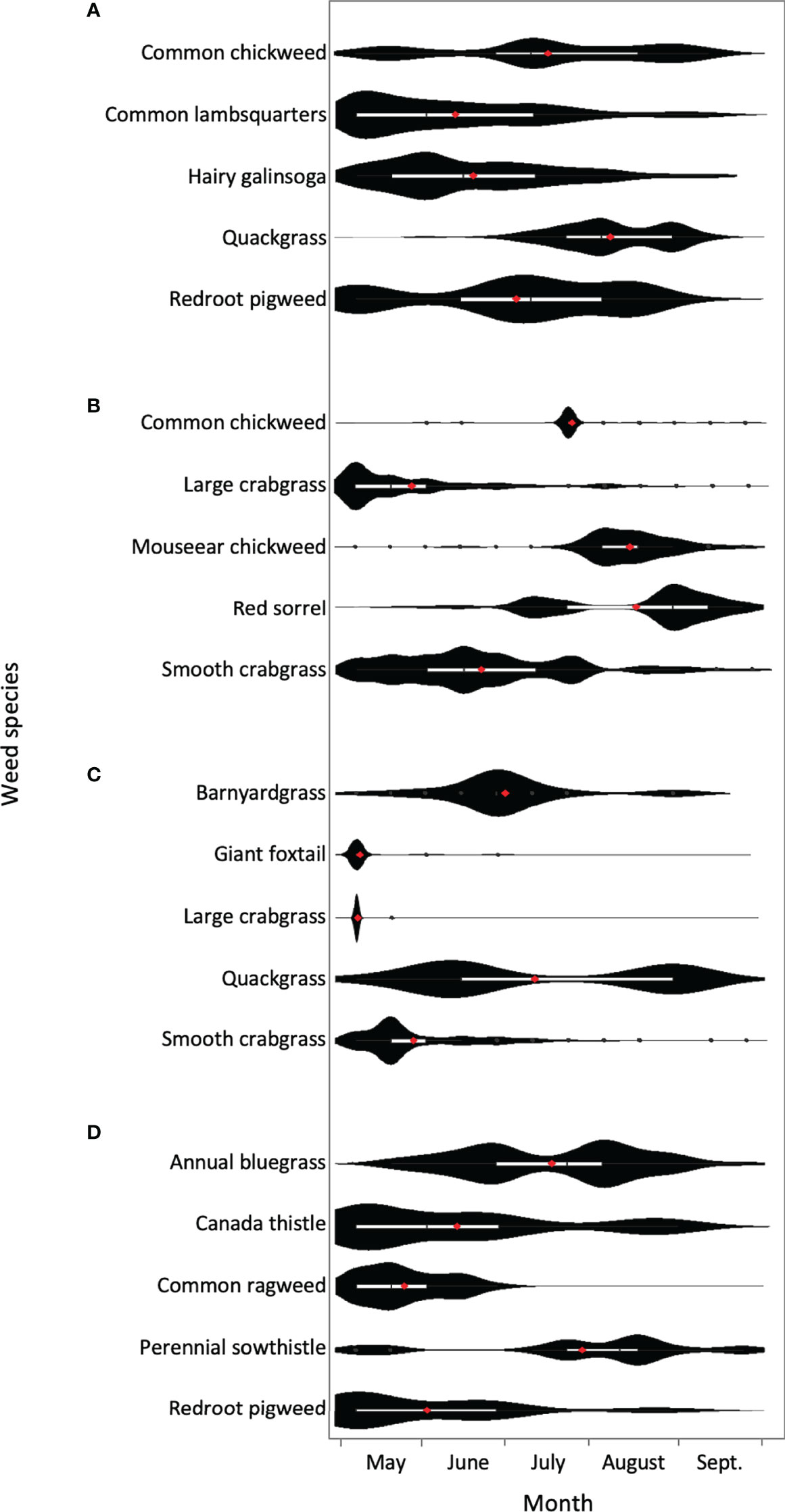
Figure 1 Violin plots of emergence of the five most abundant weeds following tillage on different dates and locations; Old Town, ME (A); Durham, NH (B), Big Flats, NY (C), and Aurora, NY (D) (adapted from Cordeau et al., 2017b). Box plots (white) are presented within violin plots (black) and means represented by red dots.
This review further examines the emergence periodicity of these 15 species – many of which have been cited by farmers as the most problematic weeds of not only Northeast USA (Jabbour et al., 2014), but also Midwest USA (Gibson et al., 2006), and Europe (Bourgeois et al., 2019). For each species, we review the empirically-observed and model-derived emergence peaks and provide an updated synthesis of emergence-based management recommendations applicable to Northeast USA and similarly temperate regions.
Review Methods
We conducted a literature search using ProQuest and Google Scholar with search terms “weed,” AND “emergence,” AND “timing,” “periodicity,” OR “phenology.” Additional searches replaced “weed” with the scientific name of each of our 15 species. Species entries in the series, The Biology of Canadian Weeds, were also reviewed. Pertinent sources cited in the resulting articles were also reviewed. No geographic limitations were imposed on our search so we could contextualize the emergence of each species over a broad range of locations. Since many of the empirically-based emergence periodicity results were qualitative, we presented them in table form (Table 1). This literature search also provided the basis for our discussion of management tactics that can be improved using knowledge of emergence periodicity.
Some results of our literature search predicted 50% cumulative emergence based on growing-degree-days. This timing typically coincides with the peak, or greatest rate of emergence (Royo-Esnal et al., 2020). To determine the timings associated with the growing-degree-days required for 50% emergence, we used weather data representing four prominent USDA Plant Hardiness Zones found in Northeast USA (USDA, 2012). Specifically, from coldest to warmest, we used weather data representing Zones 4a, 5a, 6a, and 7a, with average annual minimum temperatures of -34.4 to -31.7°C, -28.9 to -26.1°C, -23.3 to -20.6°C, and -17.8 to 15.0°C, respectively. Within each zone, we selected three representative weather station sites from the Network for Environment and Weather Applications (https://newa.cornell.edu) to encompass a wide geographic range. Zone 4a included Milan, NH, Morrisville, VT, and Saranac Lake, NY. Zone 5a included Bangor, ME, Ceres, NY, and Saratoga Springs, NY. Zone 6a included Cabot, PA, Geneva, NY, and Northborough, MA. Zone 7a included Atlantic City, NJ, Philadelphia, PA, and Riverhead, NY. Growing-degree-days were calculated in accordance with each prior study, but using mean daily maximum and minimum temperature data from 2018 to 2020 over the three locations representing each zone. If no start date was provided, we began the accumulation on January 1. One of the prediction models required additional information on the soil conditions (Archer et al., 2006). For this model we input typical conditions for Northeast USA – chisel plowed loam following corn, with wet initial conditions, and adequate rainfall. Predicted dates for 50% (peak) emergence were graphed by species and model (Figure 2).
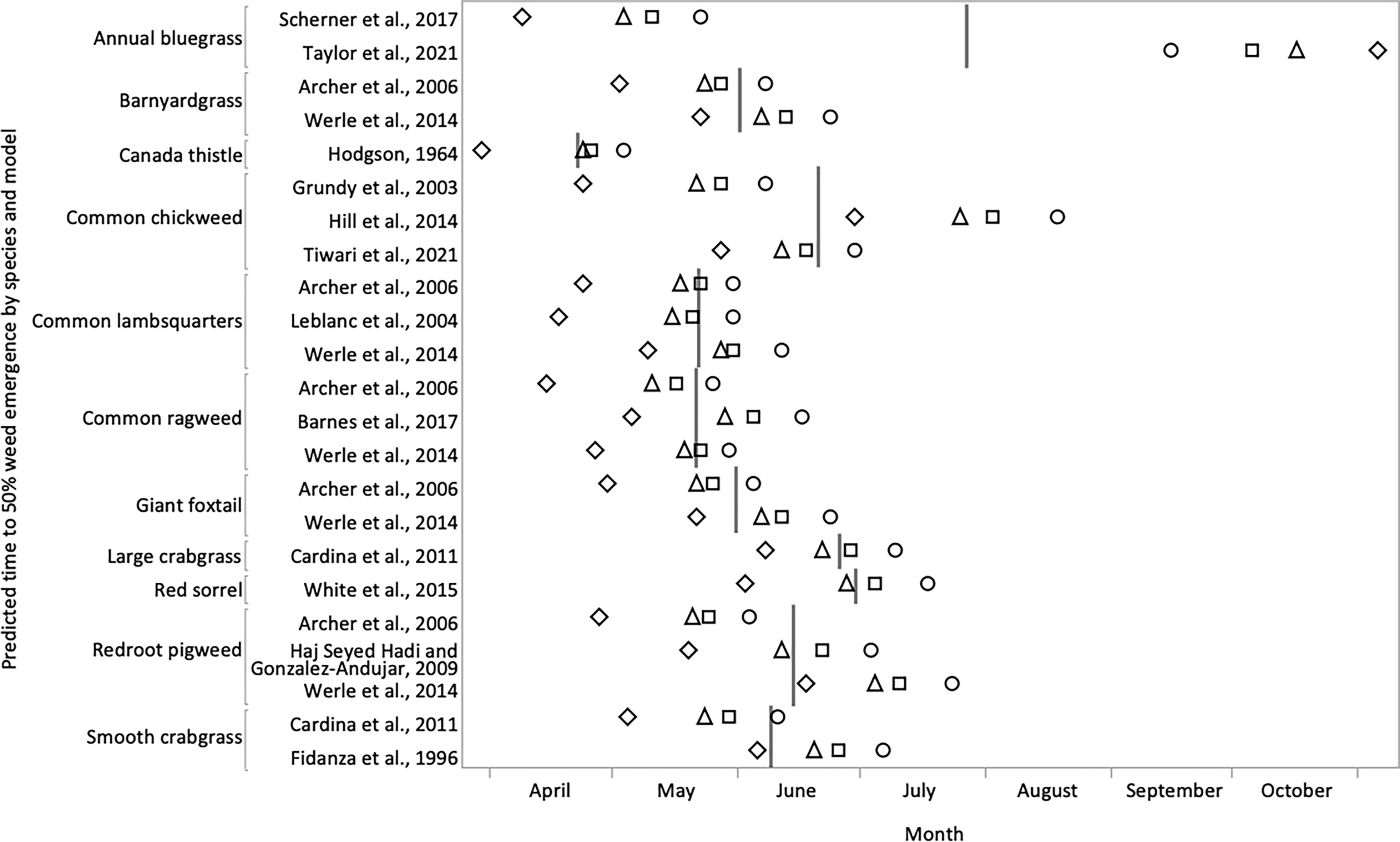
Figure 2 Predicted time to 50% (peak) weed emergence based on growing-degree-day models. Emergence was modelled from a range of USDA Plant Hardiness Zones representing most of Northeast USA. Zones include 4a (circles), 5a (squares), 6a (triangles), and 7a (diamonds). Vertical lines represent the mean predicted time to 50% emergence across presented data for each species. Models were not available for all the species we investigated.
Comparison of Emergence Peaks From Previous Research
The previous empirically-based emergence results (Table 1) were generally in agreement as to the season of peak emergence for a given species. But within such general trends, peak emergence varied by study, likely reflecting differences in growing-degree-day accumulation between sites.
Model-based predictions of peak emergence using weather data from Northeast USA (Figure 2) were in general agreement with empirically observed results. Spring-emerging species were predicted to emerge earlier in the warmer modelled zones. Within each species, the mean difference in predicted peak emergence between warmest and coolest modeled USDA Plant Hardiness Zones of Northeast USA was 39 days.
Results by Species
In this section, we will draw from empirical observations (Table 1) and model-based predictions (Figure 2) to summarize the typical emergence patterns of our 15 reviewed species. Although the climate of Northeast USA is primarily composed of USDA plant hardiness zones 4 through 7, our review found several previous results from zones 8 and 9, which we include for further context (Table 1).
Annual bluegrass generally exhibited peak emergence in autumn, but spring, summer, and even winter emergence peaks were also observed (Table 1, Figure 2). Emergence models predicted that warmer climates would experience earlier spring and later autumn emergence of annual bluegrass compared with colder climates (Figure 2). These results reflect the winter annual life cycle of annual bluegrass. Indeed, Håkansson (2003) reports that it germinates whenever dormancy has been broken and environmental conditions are adequate.
Barnyardgrass demonstrated emergence peaks in April or May from studies conducted in warm climates (Table 1). But in cooler climates, a June emergence peak was more common. For example, modelling efforts based in Minnesota and Iowa, USA showed a mean peak emergence of June 1 (Figure 2). Though emergence may occur for an extended period (Vengris, 1965; Gołębiowska and Kieloch, 2016), barnyardgrass phenological traits differ by location, and cold winter conditions likely select against late and untimely emergence (Martinková et al., 2021).
Canada thistle typically exhibited emergence peaks in early to mid-spring in studies from northern USA (Table 1, Figure 2). Emergence is mostly from overwintering root stock rather than seed (Hodgson, 1964), which likely contributes to vigorous early growth and establishment success.
Common chickweed has shown emergence peaks in spring, summer, and autumn (Table 1, Figure 2), reflecting its relatively weak dormancy (Grundy et al., 2003). Likewise, its emergence can be protracted over most of the growing season (Figure 1).
Common lambsquarters demonstrated emergence peaks mostly in mid-spring (Table 1, Figure 2). Earlier or later emergence was generally observed in warmer or colder climates, respectively. Common lambsquarters has distinct dormancy-breaking requirements (Grundy et al., 2003), but emergence can be somewhat protracted (Figure 1, Gołębiowska and Kieloch, 2016).
Common ragweed was generally the earliest emerging of the annual weeds we reviewed, with peaks mostly in early to mid-spring (Table 1, Figure 2). In several studies emergence was observed to curtail sharply in June (Table 1, Figure 1).
Giant foxtail emergence peaks were mostly in mid-spring (Table 1, Figure 2). Cordeau et al. (2017b) observed that emergence occurred over a short period, resulting in a prominent emergence peak (Figure 1).
Hairy galinsoga demonstrated emergence peaks mostly in late spring and summer (Table 1), congruent with anecdotal results from Jernigan et al. (2017). Though hairy galinsoga has no seed dormancy (Warwick and Sweet, 1983), it does require adequate soil conditions for germination, as evident in the emergence peak observed in autumn following a summer drought (Table 1).
Large crabgrass emerged in mid to late spring (Table 1, Figure 2). Cordeau et al. (2017b) found that emergence peaked sharply at one site, but was slightly protracted at another (Figure 1). In both cases, emergence was earlier than expected based on other findings from similar latitudes. This could perhaps be explained by the six-week window between tillage and sampling, which may have skewed results.
Mouseear chickweed demonstrated a mid-summer emergence peak (Table 1), but only one study was found reporting emergence periodicity.
Perennial sowthistle emerged primarily in mid spring in two of three studies (Table 1), reflecting its initiation of rhizome shoot elongation at that time (Torssell et al., 2015). But Cordeau et al. (2017b) found an emergence peak in summer, which may possibly be explained by spring field preparation with a glyphosate application that may have set back early-emerging perennial sowthistle.
Quackgrass exhibited emergence peaks in spring, spring and autumn, or summer and autumn (Table 1). But based on the experimental design of these studies, some of the summer and autumn emergence likely represents regrowth from earlier-emerged plants.
Red sorrel emergence, which is primarily from rhizomes, peaked in summer, whereas emergence from seed peaked in autumn (Table 1, Figure 2).
Redroot pigweed demonstrated a range of emergence peaks from spring to summer (Table 1, Figure 2). This protracted emergence pattern of Amaranthus species has been well-described in the literature (Jha and Norsworthy, 2009; Teasdale and Mirsky, 2015; Chahal et al., 2021; Reinhardt Piskackova et al., 2021).
Smooth crabgrass emergence peaks were primarily in May and June, similar to large crabgrass (Table 1, Figure 2).
Management Recommendations
In this section, we will provide a synthesis of management tactics that may be improved with knowledge of weed emergence. The suite of applicable management tactics depends on the season and pattern of emergence of the target weed species. For example, direct control of weed seedlings (Figure 3A), overlapping residual herbicides (Figure 3B), residue mulch (Figure 3C), and delayed planting (Figure 3D) are most applicable to early- and mid-season weeds. Early planting to improve crop competition (Figure 3E) is most applicable to mid- and late-season weeds. And feasibility of fallow or cover cropped periods (Figures 3F, G) may vary by season and cropping system (Figure 4). Therefore, we present the following management recommendations by season of weed emergence. Within each season, management tactics are organized in ascending order of management flexibility required, in accordance with Figure 3. Although many of the studies of emergence-based management tactics were conducted outside of Northeast USA, most were conducted in similarly temperate climates, and their conclusions are applicable to this review.
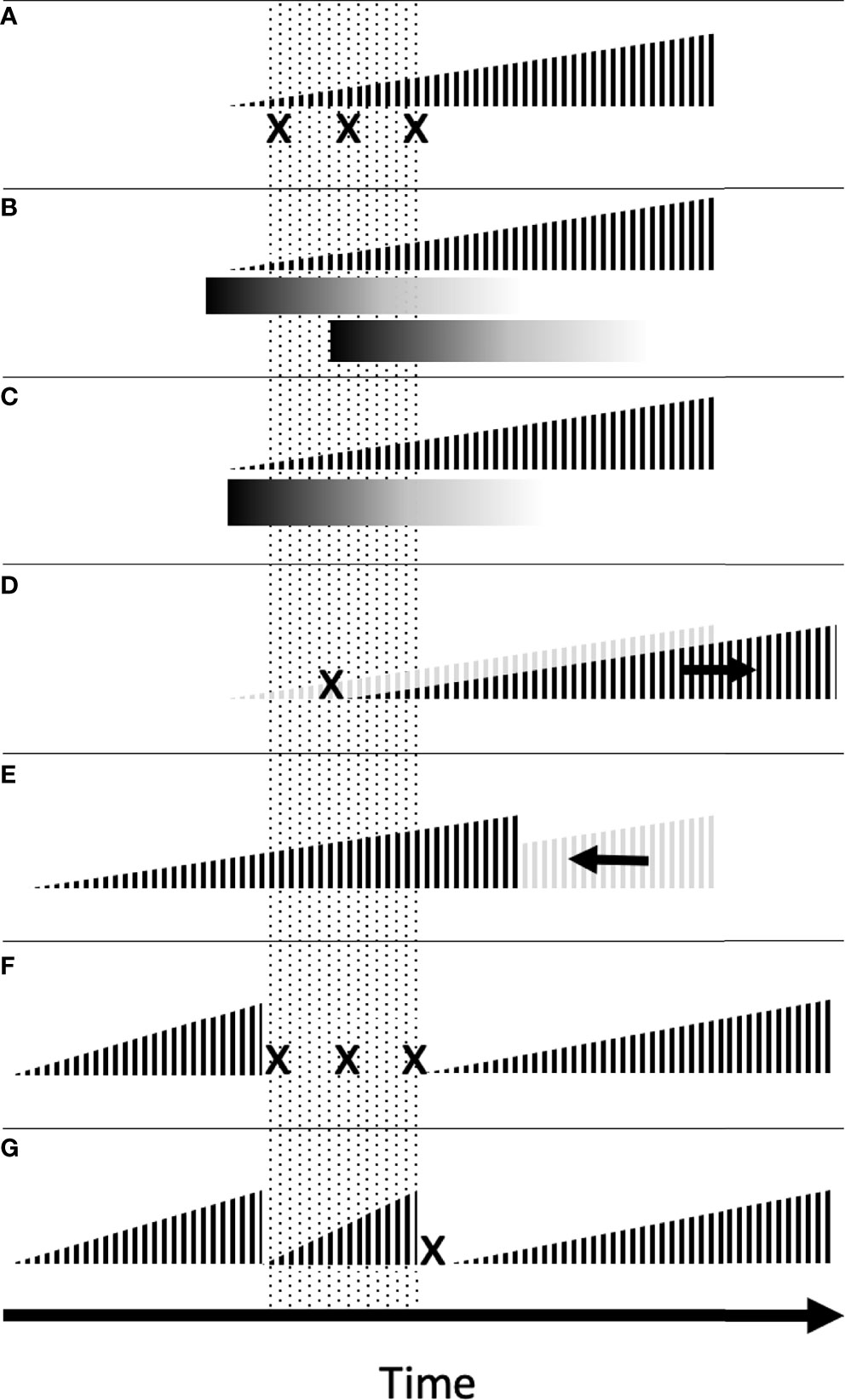
Figure 3 Depiction of tactics that may be used to target a hypothetical weed emergence peak (dotted area) occurring shortly after the typical crop planting. If the planting date or crop rotation is not flexible, frequent control of weed seedlings (A), overlapping residual herbicide applications (B), establishment of residue mulch (C), or some combination thereof may be used to target the peak emergence period. If the planting date or crop rotation are flexible, the peak emergence period may be targeted by delaying planting to allow pre-plant control of part of the flush (D), planting early at high density to expedite crop canopy closure (E), or adjusting rotation to allow for a bare fallow (F) or short-duration crop that concludes prior to weed seed production (G). Height of vertical bars represents the relative crop maturity. Physical or chemical weed seedling control actions are symbolized with an “x.” Gradients represent the breakdown of residual herbicides or residue mulch over time.
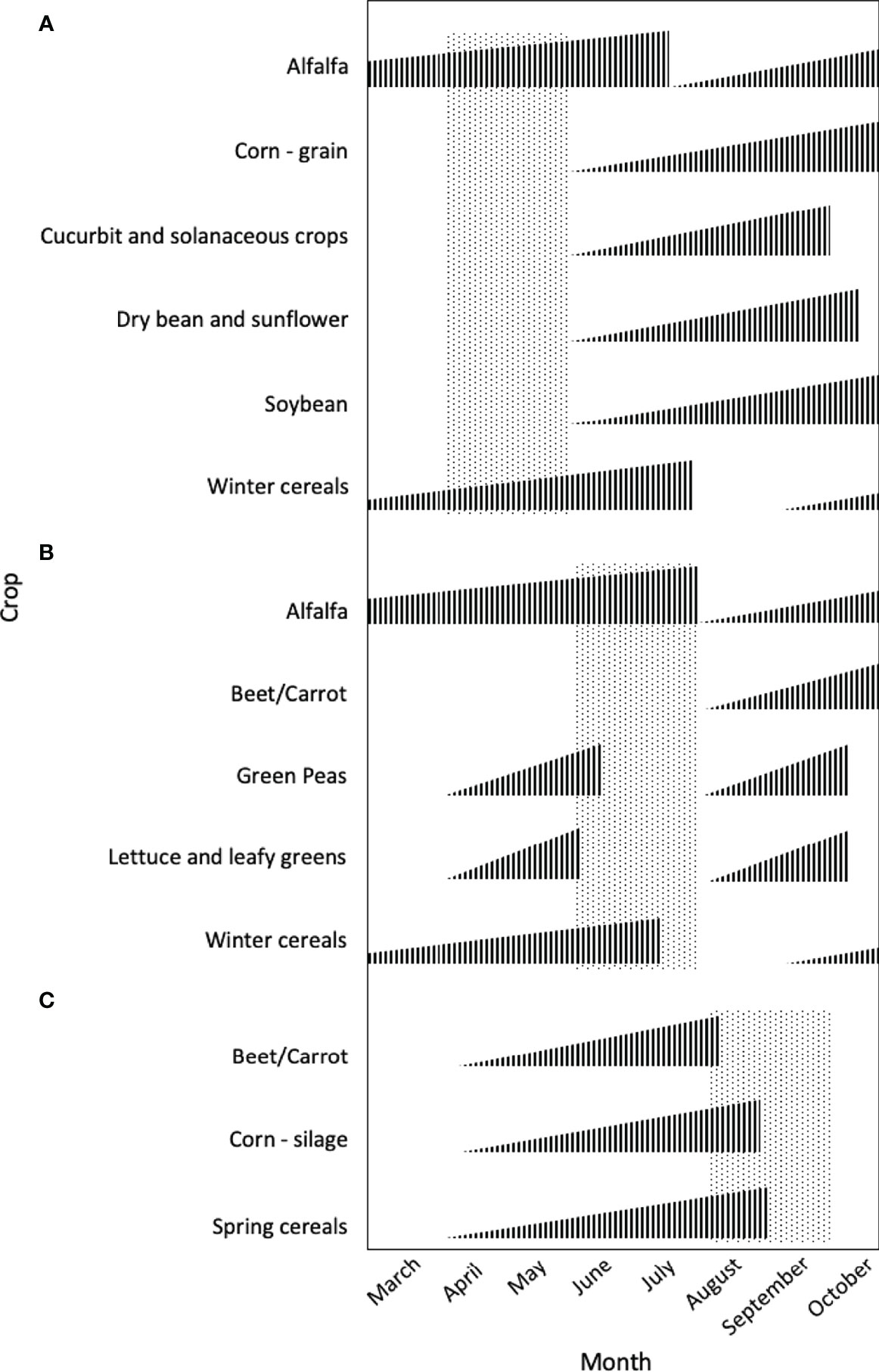
Figure 4 Examples of crops that may be shifted to avoid of peak weed emergence periods occurring in the early (A, April-May), middle (B, June-July), or late (C, August-September) growing season of the cool, temperate climate of Northeast USA. Height of vertical bars represents the relative stage of crop growth.
Weeds With Early-Season Peak Emergence
Many of the species we reviewed exhibit emergence peaks in the early part of the growing season – typically April and May (Table 1) – including barnyardgrass, Canada thistle, common lambsquarters, common ragweed, giant foxtail, large crabgrass, perennial sowthistle, and smooth crabgrass. These weeds are especially problematic since they have the potential to compete with spring-planted crops for the entire growing season.
Direct control of early-season weed seedlings with herbicides or cultivation should be guided by weed emergence to maximize effectiveness (Forcella, 1999; Zimdahl, 2013). Farmers relying on a single post-emergence herbicide application should time it well after peak weed emergence so that the greatest number of weeds are sprayed (Fidanza et al., 1996; Masin et al., 2005; Archer et al., 2006). But for early emerging summer annual weeds, like common lambsquarters, larger weeds can survive herbicide applications, so earlier timings may be more effective in such cases (DeGreeff et al., 2018). Likewise, weed seedling control using cultivation is most effective in the early “white thread” stage, especially for intra-row control (Gallandt et al., 2017), so efficiency of cultivation could be maximized if conducted around peak emergence periods of problem weeds.
Timing of early-season residual herbicide applications is also of great importance. A premature application may allow the chemical to break down prior to peak emergence, whereas a late application may not affect emerged weeds. Application and planting dates can be adjusted to optimize residual herbicide effectiveness (Culpepper et al., 2004; Webster et al., 2009; Rosario-Lebron et al., 2019). Though preplant applications of residual herbicides may not be needed if most emergence occurs prior to seedbed preparation, they may be used prior to stale or false seedbed periods to bolster seedbank depletion (Coleman et al., 2016).
Cover crop residue, or residue applied in the form of hay or straw mulch, can effectively reduce weed emergence via physical (Carrera et al., 2004) or allelopathic mechanisms (Weston and Duke, 2003). Timing of cover crop termination for creation of grown-in-place mulch may interact with many other factors to affect weed biomass following termination (Wayman et al., 2015). Teasdale and Mirsky (2015) noted that weeds emerging prior to termination have an advantage in this system, with giant foxtail becoming the dominant weed when a hairy vetch (Vicia villosa Roth.) cover crop was terminated with rolling, but in disk-killed hairy vetch, smooth pigweed (Amaranthus hybridus L.) eventually dominated, likely due to warmer soil temperatures caused by tillage, which may have stimulated germination of this later-emerging species. Roller-crimped cereal rye (Secale cereale L.) can provide effective weed control in organic soybean [Glycine max (L.) Merr.], but common ragweed often escapes suppression since it emerges before the rye is rolled into a suppressive mulch (Wallace et al., 2018). Thus, fields dominated by the earliest-emerging weeds should not rely on roller-crimped cover crops for weed control since they need to be terminated at anthesis, which is typically mid to late spring in Northeast USA. On small-scale farms, hay or straw mulch is sometimes used, and may be applied before planting robust crops or well after planting to ensure crops have achieved a sufficient height to withstand the mulching (Brown and Gallandt, 2018). When considering the best timing of mulch application relative to the peak emergence of problem weeds, farm managers should consider that it is most effective soon after application, before it decomposes (Law et al., 2006).
Delayed planting may be used to avoid peak emergence of problematic early-season weeds (Gill and Holmes, 1997). This would allow delayed usage of tillage or burndown herbicides to control most of the targeted species before the crop is planted (Chahal et al., 2021). Royo-Esnal et al. (2018) found that by delaying planting dates, they were able to avoid 50-100% of the emergence of their target weed. Common ragweed was less abundant in corn (Zea mays L.) and soybean crops when planting dates were delayed (Wallace et al., 2018). Delaying soybean planting date to June can decrease weed density at the time of post-emergence herbicide application (DeWerff et al., 2015). Delayed planting can also extend the activity and improve the control provided by pre-emergence herbicides (Rosario-Lebron et al., 2019). The timing of crop planting can also be adjusted to avoid crop losses due to the timing of weed competition (Mohler, 2001; Knezevic et al., 2002). Delaying planting may reduce the yield potential in some crops, which must be weighed against weed management benefits. Since crops differ in planting dates and associated management (Liebman and Gallandt, 1997), planting date may factor into crop or variety choice. Crops that can be planted in late-spring to avoid early-season weeds include corn, cucurbits, dry beans (Phaseolus spp.), solanaceous crops, soybeans, and sunflowers (Helianthus spp.) (Figure 4). Alternatively, alfalfa (Medicago sativa L.) and winter cereals may be planted the previous year to establish a competitive advantage over weeds with early-season peak emergence.
Bare fallow periods, including false or stale seedbeds, are some of the most powerful methods to reduce the weed seedbank (Gallandt, 2006), especially if the timing corresponds with the emergence peak of targeted weed species. Fallow periods are perhaps most feasible during the spring since more than one tillage pass or burndown herbicide application is sometimes necessary to prepare for planting, and spacing out these events allows emergence flushes between control timings. Although it is best to finish the sequence with minimal disturbance, such as with herbicides or flaming (Caldwell and Mohler, 2001), initiating with shallow tillage set to the germination depth of the target weed species can enhance the depletion of the seedbank (De Cauwer et al., 2019). But while tillage may affect the emergence of some species, the timing and magnitude of common ragweed emergence has been shown to be unaffected (Barnes et al., 2017). In such cases, it would be best to allow sufficient time for emergence rather than expecting an early peak due to tillage.
Weeds With Mid-Season Peak Emergence
Weeds with mid-season emergence peaks in June or July, included hairy galinsoga, mouseear chickweed, and red sorrel. These species may not be exposed to early season weed control efforts. Furthermore, opportunities to control these mid-season weeds may be curtailed if canopy closure or crop height restrict tractor entry into fields. Likely for these reasons, mid-season weeds can be the most abundant weeds in corn crops (Fried et al., 2020).
Direct control of mid-season weed seedlings through post-emergence herbicides or cultivation may be possible until the “layby” stage of the crop, immediately prior to canopy closure. Use of overlapping residual herbicides at this stage would further reduce emergence until full crop canopy closure (Culpepper et al., 2004).
Early planting of spring-planted crops may be used to establish a size advantage over mid-season weeds that can be used to improve control (Mohler, 2001). This size advantage is especially important for keeping weeds as small as possible for late post-emergence herbicide applications (Reinhardt Piskackova et al., 2021) and improving the selectivity of intra-row cultivation (Gallandt et al., 2017). The effect of early planting can perhaps be strengthened by additional weed suppressive tactics. Increasing crop planting density can be used to hasten canopy closure and facilitate mid-season shading (Mohler, 1996). Residue mulches (Brown and Gallandt, 2018; Wallace et al., 2018) or living mulches (Hartwig and Ammon, 2002; Westbrook et al., 2021) can provide further shading. Due to increased crop competition, late-emerging barnyardgrass and common lambsquarters had no effect on corn yield (Gołębiowska and Kieloch, 2016). Thus, planting earlier can perhaps be used to ensure mid-season weeds emerge after the critical period for weed control, thereby nullifying their threat – but only if seed production is prevented (Reinhardt Piskackova et al., 2020). For example, to reduce barnyardgrass seed production by 99%, rice crops required a 23-day advantage (Singh et al., 2017).
When planting dates were delayed, Wallace et al. (2018) found that composition of weeds with mid-season emergence periodicity increased in organic no-till corn and soybeans. They suggest integration of winter grains or perennial forages to reduce seedbanks of these species. Alternatively, in specialty crop systems, cool season crops that are established before or planted after peak emergence may avoid mid-season weeds entirely (DeVore et al., 2011). Such crops include beets, carrots, green peas, leafy greens, or lettuce (Figure 4).
Bare fallow periods targeting the seedbank of mid-season weeds may be difficult for farmers to implement in the middle of the growing season but could be integrated with cover cropping to simultaneously satisfy other pest management or soil health goals (Nordell and Nordell, 2009). Aligning the timing of cover crop seedbed preparation with the peak emergence of problem weeds would encourage weed seed germination, while termination of the cover crop prior to weed seed production would ensure a seedbank reduction (Figure 3G). Bare fallow periods can also be used in conjunction with cool season crops (Figure 4). Bare fallow periods in the mid to late season are especially important when targeting mid-season perennial weeds, such as quackgrass (Table 1), to exhaust the carbohydrate reserves in their rhizomes (Ringselle et al., 2020).
Weeds With Late-Season Peak Emergence
Weeds with peak emergence in late summer and autumn included the winter annual species, annual bluegrass. Weeds emerging at this timing provide minimal competition with established spring-planted crops (Knezevic et al., 2002; Cordeau et al., 2015; Gołębiowska and Kieloch, 2016). In interviews about problematic weeds, organic vegetable farmers made almost no mentions of winter annuals (Jabbour et al., 2014), reflecting the control of winter annuals by spring tillage. But late-season weeds can be problematic in winter cereals (Royo-Esnal et al., 2018). And in no-till systems, autumn-established winter annuals can grow too large the following spring to control with post-emergence herbicides (Wallace et al., 2019).
Autumn-applied burndown herbicides can reduce overwintering weed densities the following spring (Hasty et al., 2004). And autumn-applied residual herbicides in conjunction with winter cover crops can reduce the emergence of winter annual weeds (Walters et al., 2007) and keep them at a manageable height the following spring (Wallace et al., 2019). Interseeding may be used to expedite cover crop establishment and improve late season weed suppression, but compatibility with other post-harvest weed control tactics may be limited.
As grain corn or soybean crops mature and leaves wither, the open canopy can allow successful establishment of weeds with late-season emergence peaks. Harvest of these crops often occurs in late autumn, forcing farm managers to decide whether to terminate the weeds in autumn, or use the weeds as winter soil cover (Jabbour et al., 2014) but risk their escape in spring (Wallace et al., 2018).
Shifting spring-planted crops earlier (Figure 4) can allow for a late-season bare fallow period, which would target the seedbank of winter annuals, and help exhaust carbohydrate reserves of perennial weeds to minimize their survival over winter (Andersson et al., 2013). Late fallow periods may also necessitate a delay in autumn-planted crops. This could perhaps be offset with denser seeding rates. Royo-Esnal et al. (2018) found that by delaying winter cereal planting dates, they were able to avoid most emergence of their target weed. But in a dry year, weed emergence was delayed, and an earlier planting date would have been preferable.
Weeds With Protracted Emergence
Rather than emerging in a short, easily manageable cohort, several species exhibited a protracted emergence pattern. These included common chickweed, quackgrass, and redroot pigweed. The protracted emergence of Amaranthus species is well-known (Jha and Norsworthy, 2009; Chahal et al., 2021). Common lambsquarters (Gołębiowska and Kieloch, 2016) and hairy galinsoga (Jernigan et al., 2017) may also sometimes display protracted emergence. This emergence pattern allows these species to avoid early-season control efforts. Although late emerging weeds may not affect crop yield (Knezevic et al., 2002), they should still be controlled from a seedbank management standpoint (Norris, 1999; Brown and Gallandt, 2018) and because they are subject to sublethal herbicide doses, which can select for resistance (Norsworthy et al., 2012). Perennial weeds may also appear to have a protracted emergence due to their survival and resprouting after successive control efforts (Ringselle et al., 2020).
Control of weeds with protracted emergence requires an extended effort. We suggest a multifaceted strategy that includes as many of the following tactics as is feasible. In chronological order: densely planting crops that quickly close canopy (Mohler, 1996); overlapping residual herbicides to extend control through canopy closure (Chahal et al., 2018); ensuring seedling control is as effective as possible by timing control efforts while weeds are less than 10 cm tall (Reinhardt Piskackova et al., 2020) and using a final pass as late as possible (Crow et al., 2015); minimizing seed production of escapes using hand removal, mowing, rescue herbicide applications (Hill et al., 2016), electrical discharge systems, or artificial pollination (Lidor-Nili and Noivirt-Brik, 2017); using harvest weed seed control if the escapes have already produced seed (Walsh et al., 2012); avoiding post-harvest tillage to allow for seed predation (Birthisel et al., 2015); and moldboard plowing to bury remaining seeds below germinable depth (Mohler, 1996).
Management of Multiple Problem Weeds
As seen in Figure 1, farms often contain species with different weed emergence patterns. Therefore, farmers may need to prioritize targeting the emergence peaks of the most problematic species. Ideally, the most competitive or difficult-to-control weeds would be avoided entirely by adjusting planting dates or crop selection to allow for a bare fallow (Figure 3F) or short-term cover crop (Figure 3G). Lower priority weeds could be addressed with adjusted in-crop weed control (Figures 3A–C) combined with tactics that improve the competitive advantage of the crop (Figures 3D, E). Fields with profuse weed emergence over the entire growing season may need to be taken out of production for a full year to allow for extended bare fallow periods (Nordell and Nordell, 2009) or rapid succession cover crops that are incorporated before weeds have time to set seed (Sarrantonio and Gallandt, 2003; Gallandt, 2006; Mirsky et al., 2010).
Conversely, fields may have weed communities dominated by species with similar phenology, reflecting the history of management (Ryan et al., 2010). For example, an organic vegetable rotation and a conventional corn and alfalfa rotation resulted in two different weed communities with very different phenology (Mohler et al., 2018). Likewise, corn and soybean are more conducive to summer annual broadleaf weed emergence than wheat and alfalfa (Goplen et al., 2017). Therefore, in some cases it may be possible to target all of the problematic weeds by temporarily switching to a cropping system that allows for new management timings – such as the occasional rotation of spring versus autumn-planted crops.
Outlook
Variation in Peak Emergence Within Each Species
From our review of previous research, the peak emergence of each species varied by study (Figure 1, Table 1). Indeed, for a given weed species, emergence timing is expected to differ by location due to different weather conditions (Royo-Esnal et al., 2020). But there was also variation between modelling results of a given species when we imposed identical weather conditions (Figure 2). This may be due to other differences between study sites where models were validated, such as soil texture (Leblanc et al., 2004), surface residue (Oreja et al., 2020), crop canopy (Cardina et al., 2011), or type of tillage employed (Mohler, 2001). Additionally, depth of burial can influence not only the time required by a seedling to reach the soil surface (Gonzalez-Andujar et al., 2016), but also the accumulation of conditions required to break seed or bud dormancy (Cao et al., 2011). Furthermore, within a species there may be genetic-based differences in emergence between populations resulting from varying selection pressure in different microclimates (Dorado et al., 2009; Papiernik et al., 2020; Liu et al., 2021). For example, barnyardgrass emergence differed based on the elevation of the seed collection site (Martinková et al., 2021) and common lambsquarters emergence varied by the slope of the landscape where seeds developed (Papiernik et al., 2020).
While Grundy et al. (2003) used a single thermal time model to sufficiently predict cumulative emergence of common chickweed from different sites brought to a single location, similar common garden experiments have found differences in emergence periodicity between populations (Papiernik et al., 2020; Martinková et al., 2021). Likewise, for large crabgrass (Dorado et al., 2009) and annual ryegrass (Lolium rigidum Gaud., Sousa-Ortega et al., 2019), single models could accurately predict emergence at single but not multiple locations, suggesting that different models should be developed for different regions.
But models may only require minor adjustments to accurately predict emergence in new regions. For example, the Italian emergence model, AlertInf (Masin et al., 2012), was modified with the base temperature and water potential required for germination of barnyardgrass in Croatia, to effectively predict emergence in Croatian corn (Šoštarčić et al., 2021). To further improve emergence predictions, other site-specific factors may need to be incorporated into models to maximize their accuracy and utility to farmers (Royo-Esnal et al., 2020). Inclusion of real-time in-situ information may be useful as well, such as phenological indicators (Cardina et al., 2007; Cardina et al., 2011), degree of crust formation, shading provided by the crop, and weed emergence scouting data. Models may also need to incorporate an adjustment factor to account for genetic tendencies of some populations within a species to emerge earlier or later than predicted. Farmers may achieve a simpler version of such a process by comparing their observed emergence to model predicted emergence and adjusting the prediction accordingly in subsequent years.
Considerations for Future Modelling Efforts
Other improvements to emergence models may be gained by improving the parameter estimates through statistically independent sampling and reducing the time between sampling events (Gonzalez-Andujar et al., 2016). For example, work of Cordeau et al. (2017b) was based on statistically independent samples as each sample through the season was from a new plot. But sampling occurred six weeks after each plot was tilled, which was ideal for investigating cohort effects resulting from different tillage timings, but was too imprecise for emergence modeling.
For this review, we focused on peak emergence or 50% cumulative emergence. The two terms are similar since the rate of emergence is theoretically highest at 50% cumulative emergence (Royo-Esnal et al., 2020). But the terms are not interchangeable since the greatest empirically observed emergence peak may occur before or after 50% cumulative emergence if the distribution of emergence is skewed by drought or other random variables. In cumulative emergence models, emergence peaks would be represented by large, predicted flushes of emergence resulting from favorable weather, which may or may not bring the cumulative total above 50%. Such predictions of large flushes may be just as useful for planning purposes as cumulative emergence because weed seedling control will need to be prioritized while the cohort remains small. Thus, farmer-oriented emergence models should allow for incorporation of weather forecasts to provide corresponding weed emergence forecasts.
We have made the case for managing weeds based on their emergence peaks, but accurate predictions of the start, end, or any other point on the emergence continuum can also be useful for management purposes. For example, to maximize efficiency, weed seedling controls with nearly 100% efficacy should not be conducted until most of the weeds have emerged (Fidanza et al., 1996; Masin et al., 2005; Archer et al., 2006), whereas controls that are only effective on small weeds should be initiated when relatively few have emerged (Oriade and Forcella, 1999).
Feasibility of Adjusting Management Based on Weed Emergence
We have presented a range of techniques to improve weed management based on knowledge of peak emergence (Figure 3). Certainly, slight adjustments to the timing of seedling control may be more feasible for many farmers than making drastic changes to the cropping system. But farmers are very responsive to adjusting their management based on the biology and timing of their most problematic weeds (Jabbour et al., 2014) and will sometimes go to extreme efforts to regain control. Since most of the tactics we present embody a “weed smarter, not harder” approach, they may be easily adopted by farmers.
For farmers unable to shift the timing of their planting, it may still be possible to alter the edaphic environment to shift weed emergence earlier or later. Prior to planting, weed emergence may be expedited through practices that increase soil warming, such as tillage (Travlos et al., 2020) or solarization. Timing of irrigation may also be used to alter weed emergence (Kanatas et al., 2021). Conversely, delaying the emergence of problematic weeds may be achieved through limiting absorption of sunlight via surface residue management (Oreja et al., 2020) or crop canopy establishment.
The feasibility of emergence-based management may be impacted by other factors warranting future research. Such as the weed community shifts that may occur after long-term emergence-based management. Or changing weather patterns that will likely lengthen the growing season to the benefit of many weed species (Peters and Gerowitt, 2015). The increased rainfall expected in Northeast USA may decrease the number of days when soils are dry enough to support tractor-drawn equipment, thereby increasing the importance of access to ample equipment and labor to conduct timely weed control efforts (Birthisel et al., 2021). Likewise, timely control may be challenging on large-scale farms since it may require several days to cover the entire acreage. But perhaps fields or microclimatic areas with consistently early weed emergence could be managed first, and so forth, to allow control timing to mirror emergence trends.
Overall, previous studies were generally in agreement as to the season of peak emergence for each species. Variation in observed results likely reflected the climatic conditions of each study site. But our use of identical weather data in previous models also showed variation in emergence trends within species, highlighting the importance of local edaphic conditions and population-level differences. Nonetheless, prediction accuracy will improve as models become more advanced (Royo-Esnal et al., 2020) and farmers can further improve prediction reliability based on their own ground truthing and adjustments to model predictions in subsequent years. Furthermore, even our broad characterizations of peak emergence for each species (i.e., “late spring”) may be used to improve management. Depending on management flexibility, knowledge of the season and pattern of weed emergence be used to improve the timing of control efforts, enhance crop competition, or expedite weed seedbank depletion.
Author Contributions
BB conducted the literature search. All authors were involved in the interpretation of the results. BB, EG, and SC wrote the initial draft. All authors were involved in improving the subsequent drafts. All authors contributed to the article and approved the submitted version.
Funding
This work was partially supported by a joint research and extension project funded by the Cornell University Agricultural Experiment Station (Hatch funds) and Cornell Cooperative Extension (Smith Lever funds) received from the National Institutes for Food and Agriculture (NIFA) U.S. Department of Agriculture (Project: 2013-14-425). This work was financially supported by the Maine Agriculture and Forestry Experiment Station, French ANR COSAC project (ANR-14-CE18-0007), and the Burgundy Region in France (FABER project Couv’Herbi). Additional funding was provided by the French program Investissements d’Avenir ANR PPR SPECIFICS project (ANR-20-PCPA-0008) and the Ecophyto Dephy EXPE ABC (Agroécologie en Bourgogne et Région centre). Additional funding was provided by the New York State Department of Agriculture and Markets.
Conflict of Interest
The authors declare that the research was conducted in the absence of any commercial or financial relationships that could be construed as a potential conflict of interest.
Publisher’s Note
All claims expressed in this article are solely those of the authors and do not necessarily represent those of their affiliated organizations, or those of the publisher, the editors and the reviewers. Any product that may be evaluated in this article, or claim that may be made by its manufacturer, is not guaranteed or endorsed by the publisher.
Acknowledgments
The authors acknowledge field assistance from Joseph Cannon, Paul Stachowski, Scott Morris, Meredith Ward, Christopher Pelzer, Jeff Liebert, Kristine Averill, and Nicholas Warren.
Abbreviations
annual bluegrass, Poa annua L.; barnyardgrass, Echinochloa crus-galli (L.) Beauv.; Canada thistle, Cirsium arvense (L.) Scop.; common chickweed, Stellaria media (L.) Vill.; common lambsquarters, Chenopodium album L.; common ragweed, Ambrosia artemisiifolia L.; giant foxtail, Setaria faberi Herrm.; hairy galinsoga, Galinsoga quadriradiata Cav.; large crabgrass, Digitaria sanguinalis (L.) Scop.; mouseear chickweed, Cerastium fontanum Baumg.; perennial sowthistle, Sonchus arvensis L.; quackgrass, Elymus repens (L.) Gould; redroot pigweed, Amaranthus retroflexus L.; red sorrel, Rumex acetosella L.; smooth crabgrass, Digitaria ischaemum Schreb. ex Muhl.
References
Amor R. (1985). Seasonal Emergence of Weeds Typically Occurring in the Victorian Cereal Belt. Plant Prot. Q. 1, 18–20.
Andersson L., Boström U., Forkman J., Hakman I., Liew J., Magnuski E. (2013). Sprouting Capacity From Intact Root Systems of Cirsium arvense and Sonchus arvensis Decrease in Autumn. Weed Res. 53, 183–191. doi: 10.1111/wre.12013
Archer D., Forcella F., Korth A., Kuhn A., Eklund J., Spokas K. (2006). WeedCast Version 4.0 (Morris, MN: USDA ARS).
Bagavathiannan M. V., Norsworthy J. K., Smith K. L., Burgos N. (2011). Seedbank Size and Emergence Pattern of Barnyardgrass (Echinochloa crus-galli) in Arkansas. Weed Sci. 59, 359–365. doi: 10.1614/WS-D-10-00149.1
Barnes E., Werle R., Sandell L., Lindquist J., Knezevic S., Sikkema P., et al. (2017). Influence of Tillage on Common Ragweed (Ambrosia artemisiifolia) Emergence Pattern in Nebraska. Weed Technol. 31, 623–631. doi: 10.1017/wet.2017.38
Bassett I. J., Crompton C. W. (1975). The Biology of Canadian Weeds: 11. Ambrosia artemisiifolia L. And A. psilostachya DC. Can. J. of Plant Sci. 55, 463–476. doi: 10.4141/cjps75-072
Bastiaans L., Paolini R., Baumann D. T. (2008). Focus on Ecological Weed Management: What is Hindering Adoption? Weed Res. 48, 481–491. doi: 10.1111/j.1365-3180.2008.00662.x
Batlla D., Malavert C., Farnocchia R. B. F., Benech-Arnold R. (2020). “Modelling Weed Seedbank Dormancy and Germination,” in Decision Support Systems for Weed Management. Eds. Chantre G. R., González-Andújar J. L. New York. Springer. doi: 10.1007/978-3-030-44402-0_4
Birthisel S. K., Clements R. S., Gallandt E. R. (2021). Review: How Will Climate Change Impact the ‘Many Little Hammers’ of Ecological Weed Management? Weed Res. 61, 327—341. doi: 10.1111/wre.12497
Birthisel S. K., Gallandt E. R., Jabbour R., Drummond F. A. (2015). Habitat and Time are More Important Predictors of Weed Seed Predation Than Space on a Diversified Vegetable Farm in Maine, USA. Weed Sci. 63, 916–927. doi: 10.1614/WS-D-15-00057.1
Bourgeois B., Munoz F., Fried G., Mahaut L., Armengot L., Denelle P., et al. (2019). What Makes a Weed a Weed? A Large-Scale Evaluation of Arable Weeds Through a Functional Lens. Am. J. Bot. 106, 90–100. doi: 10.1002/ajb2.1213
Brown B., Gallandt E. (2018). A Systems Comparison of Contrasting Organic Weed Management Strategies. Weed Sci. 66, 109–120. doi: 10.1017/wsc.2017.34
Buhler D., Mester T., Kohler K. (1996). The Effect of Maize Residues and Tillage on Emergence of Setaria faberi, Abutilon theophrasti, Amaranthus retroflexus and Chenopodium album. Weed Res. 36, 153–165. doi: 10.1111/j.1365-3180.1996.tb01811.x
Caldwell B., Mohler C. L. (2001). Stale Seedbed Practices for Vegetable Production. HortScience 36, 703–705. doi: 10.21273/HORTSCI.36.4.703
Cao R., Francisco-Fernandez M., Anand A., Bastida F., Gonzalez-Andujar J. L. (2011). Computing Statistical Indices for Hydrothermal Times Using Weed Emergence Data. J. Agric. Sci. 149, 701–712. doi: 10.1017/S002185961100030X
Cardina J., Herms C. P., Herms D. A. (2011). Phenological Indicators for Emergence of Large and Smooth Crabgrass (Digitaria sanguinalis and D. ischaemum). Weed Technol. 25, 141–150. doi: 10.1614/WT-D-10-00034.1
Cardina J., Herms C. P., Herms D. A., Forcella F. (2007). Evaluating Phenological Indicators for Predicting Giant Foxtail (Setaria faberi) Emergence. Weed Sci. 55, 455–464. doi: 10.1614/WS-07-005.1
Carrera L. M., Abdul-Baki A. A., Teasdale J. R. (2004). Cover Crop Management and Weed Suppression in No-Tillage Sweet Corn Production. HortScience 39, 1262–1266. doi: 10.21273/HORTSCI.39.6.1262
Chahal P., Barnes E., Jhala A. (2021). Emergence Pattern of Palmer Amaranth (Amaranthus palmeri) Influenced by Tillage Timings and Residual Herbicides. Weed Tech. 35, 433–439. doi: 10.1017/wet.2020.136
Chahal P. S., Ganie Z. A., Jhala A. J. (2018). Overlapping Residual Herbicides for Control of Photosystem II and 4-Hydroxyphenylpyruvate Dioxygenase (HPPD) Inhibitor-Resistant Palmer Amaranth (Amaranthus palmeri S. Watson) in Glyphosate-Resistant Maize. Front. Plant Sci 8. doi: 10.3389/fpls.2017.02231
Coleman L. B., Chaudhari S., Jennings K. M., Schultheis J. R., Meyers S. L., Monks D. W. (2016). Evaluation of Herbicide Timings for Palmer Amaranth Control in a Stale Seedbed Sweetpotato Production System. Weed Technol. 30, 725–732. doi: 10.1614/WT-D-15-00133.1
Cordeau S., Guillemin J.-P., Reibel C., Chauvel B. (2015). Weed Species Differ in Their Ability to Emerge in No-Till Systems That Include Cover Crops. Ann. Appl. Biol. 166, 444–455. doi: 10.1111/aab.12195
Cordeau S., Smith R. G., Gallandt E. R., Brown B., Salon P., Ditommaso A., et al. (2017a). Disentangling the Effects of Tillage Timing and Weather on Weed Community Assembly. Agriculture 7, 66. doi: 10.3390/agriculture7080066
Cordeau S., Smith R. G., Gallandt E. R., Brown B., Salon P., Ditommaso A., et al. (2017b). How do Weeds Differ in Their Response to the Timing of Tillage? A Study of 61 Species Across the Northeastern United States. Ann. Appl. Biol. 171, 340–352. doi: 10.1111/aab.12377
Cordeau S., Smith R. G., Gallandt E. R., Brown B., Salon P., Ditommaso A., et al. (2017c). Timing of Tillage as a Driver of Weed Communities. Weed Sci. 65, 504–514. doi: 10.1017/wsc.2017.26
Crow W. D., Steckel L. E., Hayes R. M., Mueller T. C. (2015). Evaluation of POST-Harvest Herbicide Applications for Seed Prevention of Glyphosate-Resistant Palmer Amaranth (Amaranthus palmeri). Weed Technol. 29, 405–411. doi: 10.1614/WT-D-14-00146.1
Culpepper A. S., Flanders J., York A. C., Webster T. M. (2004). Tropical Spiderwort (Commelina benghalensis) Control in Glyphosate-Resistant Cotton. Weed Technol. 18, 432–436. doi: 10.1614/WT-03-175R
Davis A. S., Clay S., Cardina J., Dille A., Forcella F., Lindquist J., et al. (2013). Seed Burial Physical Environment Explains Departures From Regional Hydrothermal Model of Giant Ragweed (Ambrosia trifida) Seedling Emergence in US Midwest. Weed Sci. 61, 415–421. doi: 10.1614/WS-D-12-00139.1
De Cauwer B., De Cuypere T., De Ryck S., Delanote L., Dewaele K., Willekens K., et al. (2019). Reduction in Field Emergence and Seedbank Density of Galinsoga quadriradiata and Other Weeds After Contrasting False Seedbed Strategies in Organic Vegetable Fields. Weed Res. 59, 265–278. doi: 10.1111/wre.12363
DeGreeff R. D., Varanasi A. V., Dille J. A., Peterson D. E., Jugulam M. (2018). Influence of Plant Growth Stage and Temperature on Glyphosate Efficacy in Common Lambsquarters (Chenopodium album). Weed Technol. 32, 448–453. doi: 10.1017/wet.2018.38
DeVore J., Norsworthy J., Johnson D., Starkey C., Wilson M., Griffith G. (2011). “Palmer Amaranth Emergence as Influenced by Soybean Production System and Deep Tillage,” in 2011 Proceedings, Southern Weed Science Society, San Juan, PR. 239.
DeWerff R. P., Conley S. P., Colquhoun J. B., Davis V. M. (2015). Weed Control in Soybean as Influenced by Residual Herbicide Use and Glyphosate-Application Timing Following Different Planting Dates. Weed Technol. 29, 71–81. doi: 10.1614/WT-D-14-00040.1
Dickerson C. T. J. (1968). Studies on the Germination, Growth, Development, and Control of Common Ragweed (Ambrosia artemisiifolia L.) (Ithaca (NY: Cornell University).
DiTommaso A. (2016) Weed Emergence Patterns in Response to Disturbance on Two Soil Types (New York State Integrated Pest Management). Available at: https://hdl.handle.net/1813/52046 (Accessed 2 November 2021).
Donald W. W. (2000). A Degree-Day Model of Cirsium arvense Shoot Emergence From Adventitious Root Buds in Spring. Weed Sci. 48, 333–341. doi: 10.1614/0043-1745(2000)048[0333:ADDMOC]2.0.CO;2
Dorado J., Sousa E., Calha I., Gonzalez-Andujar J. L., Fernandez-Quintanilla C. (2009). Predicting Weed Emergence in Maize Crops Under Two Contrasting Climatic Conditions. Weed Res. 49, 251–260. doi: 10.1111/j.1365-3180.2008.00690.x
Fidanza M., Dernoeden P., Zhang M. (1996). Degree-Days for Predicting Smooth Crabgrass Emergence in Cool-Season Turfgrasses. Crop Sci. 36, 990–996. doi: 10.2135/cropsci1996.0011183X0036000400029x
Forcella F. (1999). Estimating the Timing of Weed Emergence. Site Specific Management Guidelines (Atlanta: Potash & Phosphate Institute).
Forcella F., Wilson R. G., Dekker J., Kremer R. J., John C., Randy L. A., et al. (1997). Weed Seed Bank Emergence Across the Corn Belt. Weed Sci. 45, 67–76. doi: 10.1017/S0043174500092493
Fried G., Chauvel B., Munoz F., Reboud X. (2020). Which Traits Make Weeds More Successful in Maize Crops? Insights From a Three-Decade Monitoring in France. Plants 9, 40. doi: 10.3390/plants9010040
Gallandt E. R. (2006). How Can We Target the Weed Seedbank? Weed Sci. 54, 588–596. doi: 10.1614/WS-05-063R.1
Gallandt E. R., Brainard D. C., Brown B. (2017). “Developments in Physical Weed Control,” in Integrated Weed Management for Sustainable Agriculture. Ed. Zimdahl R. L. (Sawston, UK: Burleigh Dodds Science Publishing), 261–283.
Gibson K., Johnson W., Hillger D. (2006). Farmer Perceptions of Weed Problems in Corn and Soybean Rotation Systems. Weed Technol. 20, 751–755. doi: 10.1614/WT-05-089R1.1
Gill G. S., Holmes J. E. (1997). Efficacy of Cultural Control Methods for Combating Herbicide-Resistant Lolium rigidum. Pest Manage. Sci. 51, 352–358. doi: 10.1002/(SICI)1096-9063(199711)51:3<352::AID-PS648>3.0.CO;2-M
Gołębiowska H., Kieloch R. (2016). The Competitive Ability of Chenopodium album and Echinochloa crus-galli in Maize Crops Depending on the Time of Their Occurrence or Removal. Acta Agrobot. 69, 1688. doi: 10.5586/aa.1688
Gonzalez-Andujar J. L., Chantre G. R., Morvillo C., Blanco A. M., Forcella F. (2016). Predicting Field Weed Emergence With Empirical Models and Soft Computing Techniques. Weed Res. 56, 415–423. doi: 10.1111/wre.12223
Goplen J. J., Sheaffer C. C., Becker R. L., Coulter J. A., Breitenbach F. R., Behnken L. M., et al. (2017). Seedbank Depletion and Emergence Patterns of Giant Ragweed (Ambrosia trifida) in Minnesota Cropping Systems. Weed Sci. 65, 52–60. doi: 10.1614/WS-D-16-00084.1
Grundy A., Peters N., Rasmussen I. A., Hartmann K., Sattin M., Andersson L., et al. (2003). Emergence of Chenopodium album and Stellaria media of Different Origins Under Different Climatic Conditions. Weed Res. 43, 163–176. doi: 10.1046/j.1365-3180.2003.00330.x
Haj Seyed Hadi M. R., Petersonzalez-Andujar J. L. (2009). Comparison of FittingWeed Seedling Emergence ModelsWith Nonlinear Regression and Genetic Algorithm. Comput. Electr. Agric. 65, 19–25. doi: 10.1016/j.compag.2008.07.005
Hakansson S. (1969). Experiments With Sonchus Arvensis L.: I. Development and Growth, and the Response to Burial and Defoliation in Different Developmental Stages. Uppsala Lantbrukshogsk Ann. 35, 989–1030.
Håkansson S. (2003). Weeds and Weed Management on Arable Land: An Ecological Approach (Wallingford, UK: CABI).
Hartwig N. L., Ammon H. U. (2002). Cover Crops and Living Mulches. Weed Sci. 50, 688–699. doi: 10.1614/0043-1745(2002)050[0688:AIACCA]2.0.CO;2
Harvey S., Forcella F. (1993). Vernal Seedling Emergence Model for Common Lambsquarters (Chenopodium album). Weed Sci. 41, 309–316. doi: 10.1017/S0043174500076220
Hasty R. F., Sprague C. L., Hager A. G. (2004). Weed Control With Fall and Early-Preplant Herbicide Applications in No-Till Soybean. Weed Technol. 18, 887–892. doi: 10.1614/WT-03-041R3
Hill E. C., Renner K. A., Sprague C. L. (2014). Henbit (Lamium amplexicaule), Common Chickweed (Stellaria media), Shepherd's-Purse (Capsella bursa-pastoris), and Field Pennycress (Thlaspi arvense): Fecundity, Seed Dispersal, Dormancy, and Emergence. Weed Sci. 62, 97–106. doi: 10.1614/WS-D-13-00074.1
Hill E., Renner K., VanGessel M., Bellinder R., Scott B. (2016). Late-Season Weed Management to Stop Viable Weed Seed Production. Weed Sci. 64, 112–118. doi: 10.1614/WS-D-15-00096.1
Hodgson J. (1955). Whitetop and its Control (Moscow, ID: Idaho Agricultural Extension Service Experiment), p.1–p19.
Hodgson J. M. (1964). Variations in Ecotypes of Canada Thistle. Weeds 13, 167–171. doi: 10.2307/4040720
Jabbour R., Zwickle S., Gallandt E. R., Mcphee K. E., Wilson R. S., Doohan D. (2014). Mental Models of Organic Weed Management: Comparison of New England US Farmer and Expert Models. Renew. Agric. Food Syst. 29, 319–333. doi: 10.1017/S1742170513000185
Jernigan A., Caldwell B., Cordeau S., DiTommaso A., Drinkwater L., Mohler C., et al. (2017). Weed Abundance and Community Composition Following a Long-Term Organic Vegetable Cropping Systems Experiment. Weed Sci. 65, 639–649. doi: 10.1017/wsc.2017.33
Jha P., Norsworthy J. K. (2009). Soybean Canopy and Tillage Effects on Emergence of Palmer Amaranth (Amaranthus palmeri) From a Natural Seed Bank. Weed Sci. 57, 644–651. doi: 10.1614/WS-09-074.1
Jursík M., Holec J., Soukup J. (2014). Field Emergence of Weeds During the Growing Season of Sugar Beet. Listy Cukrovarnicke a Reparske 130, 166–172.
Kaminski J. E., Dernoeden P. H. (2007). Seasonal Poa annua L. Seedling Emergence Patterns in Maryland. Crop Sci. 47, 775–779. doi: 10.2135/cropsci2006.03.0191
Kanatas P., Gazoulis I., Travlos I. (2021). Irrigation Timing as a Practice of Effective Weed Management in Established Alfalfa (Medicago sativa L.) Crop. Agronomy 11, 550. doi: 10.3390/agronomy11030550
King C. A., Oliver L. R. (1994). A Model for Predicting Large Crabgrass (Digitaria sanguinalis) Emergence as Influenced by Temperature and Water Potential. Weed Sci. 42, 561–567. doi: 10.1017/S0043174500076955
Knezevic S. Z., Evans S. P., Blankenship E. E., Van Acker R. C., Lindquist J. L. (2002). Critical Period for Weed Control: The Concept and Data Analysis. Weed Sci. 50, 773–786. doi: 10.1614/0043-1745(2002)050[0773:CPFWCT]2.0.CO;2
Law D. M., Rowell A. B., Snyder J. C., Williams M. A. (2006). Weed Control Efficacy of Organic Mulches in Two Organically Managed Bell Pepper Production Systems. Horttechnology 16, 225–232. doi: 10.21273/HORTTECH.16.2.0225
Lawson H. M., Waister P. D., Stephens R. J. (1974). Patterns of Emergence of Several Important Arable Weed Species. Br. Crop Pr. 9, 121–135.
Leblanc M. L., Cloutier D. C., Stewart K. A., Hamel C. (2004). Calibration and Validation of a Common Lambsquarters (Chenopodium album) Seedling Emergence Model. Weed Sci. 52, 61–66. doi: 10.1614/P2002-109
Lemna W. K., Messersmith C. G. (1990). The Biology of Canadian Weeds. 94. Sonchus arvensis L. Can. J. Plant Sci. 70, 509–532. doi: 10.4141/cjps90-060
Lidor-Nili E., Noivirt-Brik O. (2017). Composition, Kits and Methods for Weed Control. US Patent 5,925,808 (Washington, DC: U.S. Patent and Trademark Office).
Liebman M., Gallandt E. (1997). “Many Little Hammers: Ecological Management of Crop-Weed Interactions,” in Ecology in Agriculture. Ed. Jackson L. (New York: Academic Press), 291–343.
Liu R., Kumar V., Jha P., Stahlman P. W. (2021). Emergence Pattern and Periodicity of Palmer Amaranth (Amaranthus palmeri) Populations From Southcentral Great Plains. Weed Technol 36:110–117. doi: 10.1017/wet.2021.81
Magarey R. D., Borchert D. M., Schlegel J. W. (2008). Global Plant Hardiness Zones for Phytosanitary Risk Analysis. Sci. Agric. 65, 54–59. doi: 10.1590/S0103-90162008000700009
Martinková Z., Honěk A., Pekár S., Leišova-Svobodová L. (2021). Geographic Differentiation of Adaptive Phenological Traits of Barnyardgrass (Echinochloa crus-galli) Populations. Weed Sci. 69, 353–361. doi: 10.1017/wsc.2021.11
Masin R., Loddo D., Benvenuti S., Otto S., Zanin G. (2012). Modeling Weed Emergence in Italian Maize Fields. Weed Sci. 60, 254–259. doi: 10.1614/WS-D-11-00124.1
Masin R., Macolino S. (2016). Seedling Emergence and Establishment of Annual Bluegrass (Poa annua) in Turfgrasses of Traditional and Creeping Perennial Ryegrass Cultivars. Weed Technol. 30, 238–245. doi: 10.1614/WT-D-15-00070.1
Masin R., Zuin M. C., Archer D. W., Forcella F., Zanin G. (2005). WeedTurf: A Predictive Model to Aid Control of Annual Summer Weeds in Turf. Weed Sci. 53, 193–201. doi: 10.1614/WS-04-066R1
Maun M. A., Barrett S. C. H. (1986). The Biology of Canadian Weeds. 77. Echinochloa crus-galli (L.) Beauv. Can. J. Plant Sci. 66, 739–759. doi: 10.4141/cjps86-093
Mirsky S. B., Gallandt E. R., Mortensen D. A., Curran W. S., Shumway D. L. (2010). Reducing the Germinable Weed Seedbank With Soil Disturbance and Cover Crops. Weed Res. 50, 341–352. doi: 10.1111/j.1365-3180.2010.00792.x
Mohler C. L. “Weed Life History: Identifying Vulnerabilities.” in Ecological Management of Agricultural Weeds. Eds. Liebman M., Mohler C. L., Staver C. P. (2001) (Cambridge, UK: Cambridge University Press), 40–98.
Mohler C. L. (1996). Ecological Bases for the Cultural Control of Annual Weeds. J. Prod. Agric. 9, 468–474. doi: 10.2134/jpa1996.0468
Mohler C. L., Caldwell B. A., Marschner C. A., Cordeau S., Maqsood Q., Ryan M. R., et al. (2018). Weed Seedbank and Weed Biomass Dynamics in a Long-Term Organic Vegetable Cropping Systems Experiment. Weed Sci. 66, 611–626. doi: 10.1017/wsc.2018.52
Myers M. W., Curran W. S., Vangessel M. J., Calvin D. D., Mortensen D. A., Majek B. A., et al. (2004). Predicting Weed Emergence for Eight Annual Species in the Northeastern United States. Weed Sci. 52, 913–919. doi: 10.1614/WS-04-025R
Norris R. F. (1999). Ecological Implications of Using Thresholds for Weed Management. J. Crop Prod. 2, 31–58. doi: 10.1300/J144v02n01_03
Norsworthy J. K., Ward S. M., Shaw D. R., Llewellyn R. S., Nichols R. L., Webster T. M., et al. (2012). Reducing the Risks of Herbicide Resistance: Best Management Practices and Recommendations. Weed Sci. 60, 31–62. doi: 10.1614/WS-D-11-00155.1
Oreja F. H., Batlla D., de la Fuente E. B. (2020). Digitaria sanguinalis Seed Dormancy Release and Seedling Emergence are Affected by Crop Canopy and Stubble. Weed Res. 60, 111–120. doi: 10.1111/wre.12392
Oriade C., Forcella F. (1999). Maximizing Efficacy and Economics of Mechanical Weed Control in Row Crops Through Forecasts of Weed Emergence. J. Crop Prod. 2, 189–205. doi: 10.1300/9785537
Papiernik S. K., Forcella F., Amundson G. B. (2020). Emergence of Common Lambsquarters (Chenopodium album L.) is Influenced by the Landscape Position in Which Seeds Developed. Agric. Environ. Lett. 5, e20000. doi: 10.1002/ael2.20000
Peters K., Gerowitt B. (2015). Weed Growth Properties of Amaranthus retroflexus, Echinochloa crus-galli and Setaria viridis as Influenced by Shifts in the Maize Cropping Season. J. Plant Dis. Prot. 122, 49–55. doi: 10.1007/BF03356530
Reinhardt Piskackova T. A., Reberg-Horton S. C., Richardson R. J., Jennings K. M., Franca L., Young B. G., et al. (2021). Windows of Action for Controlling Palmer Amaranth (Amaranthus palmeri) Using Emergence and Phenology Models. Weed Res. 61, 188–198. doi: 10.1111/wre.12470
Reinhardt Piskackova T. A., Reberg-Horton C., Richardson R. J., Jennings K. M., Leon R. G. (2020). Integrating Emergence and Phenology Models to Determine Windows of Action for Weed Control: A Case Study Using Senna obtusifolia. Field Crops Res 258, e107959. doi: 10.1016/j.fcr.2020.107959
Renner K. A., Swinton S. M., Kells J. J. (1999). Adaptation and Evaluation of the WEEDSIM Weed Management Model for Michigan. Weed Sci. 47, 338–348. doi: 10.1017/S0043174500091876
Ringselle B., De Cauwer B., Salonen J., Soukup J. (2020). A Review of non-Chemical Management of Couch Grass (Elymus repens). Agronomy 10, e1178. doi: 10.3390/agronomy10081178
Roberts H. A., Dawkins P. A. (1967). Effect of Cultivation on the Numbers of Viable Weed Seeds in Soil. Weed Res. 7, 290–301. doi: 10.1111/j.1365-3180.1967.tb01384.x
Roman E. S., Murphy S. D., Swanton C. J. (2000). Simulation of Chenopodium album Seedling Emergence. Weed Sci. 48, 217–224. doi: 10.1614/0043-1745(2000)048[0217:SOCASE]2.0.CO;2
Rosario-Lebron A., Leslie A. W., Yurchak V. L., Chen G., Hooks C. R. R. (2019). Can Winter Cover Crop Termination Practices Impact Weed Suppression, Soil Moisture, and Yield in No-Till Soybean [Glycine max (L.) Merr.]? Crop Prot. 116, 132–141. doi: 10.1016/j.cropro.2018.10.020
Royo-Esnal A., Recasens J., Garrido J., Torra J. (2018). Rigput Brome (Bromus diandrus Roth.) Management in a No-Till Field in Spain. Agronomy 8, e251. doi: 10.3390/agronomy8110251
Royo-Esnal A., Torra J., Chantre G. R. (2020). ““Weed Emergence Models,”,” in Decision Support Systems for Weed Management. Eds. Chantre G. R., González-Andújar J. L. (Cham, Switzerland: Springer), 85–116.
Ryan M. R., Smith R. G., Mirsky S. B., Mortensen D. A., Seidel R. (2010). Management Filters and Species Traits: Weed Community Assembly in Long-Term Organic and Conventional Systems. Weed Sci. 58, 265–277. doi: 10.1614/WS-D-09-00054.1
Sarrantonio M., Gallandt E. (2003). The Role of Cover Crops in North American Cropping Systems. J. Crop Prod. 8, 53–74. doi: 10.1300/J144v08n01_04
Scherner A., Melander B., Jensen P. K., Kudsk P., Avila L. A. (2017). Germination of Winter Annual Grass Weeds Under a Range of Temperatures and Water Potentials. Weed Sci. 65, 468–478. doi: 10.1017/wsc.2017.7
Shem-Tov S., Fennimore S. A. (2003). Seasonal Changes in Annual Bluegrass (Poa annua) Germinability and Emergence. Weed Sci. 51, 690–695. doi: 10.1614/0043-1745(2003)051[0690:SCIABP]2.0.CO;2
Singh M., Bhullar M. S., Chauhan B. S. (2017). Relative Time of Weed and Crop Emergence is Crucial for Managing Weed Seed Production: A Study Under an Aerobic Rice System. Crop Prot. 99, 33–38. doi: 10.1016/j.cropro.2017.05.013
Šoštarčić V., Masin R., Loddo D., Svečnjak Z., Rubinić V., Šćepanović M. (2021). Predicting the Emergence of Echinochloa crus-galli (L.) P. Beauv. In Maize Crop in Croatia With Hydrothermal Model. Agronomy 11, e2072. doi: 10.3390/agronomy11102072
Sousa-Ortega C., Chamber E., Urbano J. M., Izquierdo J., Loureiro I., Marí A. I., et al. (2019). Should Emergence Models for Lolium rigidum be Changed Throughout Climatic Conditions? The Case of Spain. Crop Prot 68, 268–277. doi: 10.1016/j.cropro.2019.105012
Sousa-Ortega C., Royo-Esnal A., DiTommaso A., Izquierdo J., Loureiro I., Marí A. I., et al. (2020). Modeling the Emergence of North African Knapweed (Centaurea diluta), an Increasingly Troublesome Weed in Spain. Weed Sci. 68, 268–277. doi: 10.1017/wsc.2020.22
Stoller E. W., Wax L. M. (1973). Periodicity of Germination and Emergence of Some Annual Weeds. Weed Sci. 21, 574–580. doi: 10.1017/S0043174500032513
Taylor D. R., Prorock M., Horvath B. J., Brosnan J. T. (2021). Modeling Seasonal Emergence of Poa annua in Urban Greenspace. Sci. Rep. 11, e18960. doi: 10.1038/s41598-021-98525-4
Teasdale J., Mirsky S. (2015). Tillage and Planting Date Effects on Weed Dormancy, Emergence, and Early Growth in Organic Corn. Weed Sci. 63, 477–490. doi: 10.1614/WS-D-14-00112.1
Tiwari R., Reinhardt Piskackova T. A., Devkota P., Mulvaney M. J., Ferrell J. A., Leon R. G. (2021). Emergence Patterns of Winter and Summer Annual Weeds in Ethiopian Mustard (Brassica carinata) Cropping System. Weed Sci. 69, 446–453. doi: 10.1017/wsc.2021.20
Torssell B., Eckersten H., Anbari S., Lundkvist A., Verwijst T. (2015). Modelling Below-Ground Shoot Elongation and Emergence Time of Sonchus Arvensis Shoots. Acta Agriculturae. Scandinavica. 65, 582–588. doi: 10.1080/09064710.2015.1044463
Travlos I., Gazoulis I., Kanatas P., Tsekoura A., Zannopoulos S., Papastylianou P. (2020). Key Factors Affecting Weed Seeds’ Germination, Weed Emergence, and Their Possible Role for the Efficacy of False Seedbed Technique as Weed Management Practice. Front. Agron. 2. doi: 10.3389/fagro.2020.00001
USDA (2012) USDA Plant Hardiness Zone Map (Agricultural Research Service, U.S. Department of Agriculture). Available at: http://planthardiness.ars.usda.gov (Accessed 10 November 2021).
Vasileiadis V., Froud-Williams R., Loddo D., Eleftherohorinos I. (2016). Emergence Dynamics of Barnyardgrass and Jimsonweed From Two Depths When Switching From Conventional to Reduced and No-Till Conditions. Span. J. Agric. Res. 14, e1002. doi: 10.5424/sjar/2016141-8752
Vengris J. (1965). Seasonal Occurrence of Barnyardgrass in Potato Fields in Massachusetts. Weeds 13, 374–375. doi: 10.2307/4040902
Wallace J., Curran W., Mortensen D. (2019). Cover Crop Effects on Horseweed (Erigeron canadensis) Density and Size Inequality at the Time of Herbicide Exposure. Weed Sci. 67, 327–338. doi: 10.1017/wsc.2019.3
Wallace J. M., Keene C. L., Curran W., Mirsky S., Ryan M. R., VanGessel M. J. (2018). Integrated Weed Management Strategies in Cover Crop–Based, Organic Rotational No-Till Corn and Soybean in the Mid-Atlantic Region. Weed Sci. 66, 94–108. doi: 10.1017/wsc.2017.53
Walsh M. J., Harrington R. B., Powles S. B. (2012). Harrington Seed Destructor: A New Nonchemical Weed Control Tool for Global Grain Crops. Crop Sci. 52, 1343–1347. doi: 10.2135/cropsci2011.11.0608
Walters S. A., Young B. G., Nolte S. A. (2007). Cover Crop and Pre-Emergence Herbicide Combinations in No-Tillage Fresh Market Cucumber Production. J. Sustain. Agric. 30, 5–19. doi: 10.1300/J064v30n03_03
Warwick S. I., Sweet R. D. (1983). The Biology of Canadian Weeds. 58. Galinsoga parviflora and Galinsoga quadriradiata (=G. Ciliata). Can. J. Plant Sci. 63, 695–709. doi: 10.4141/cjps83-087
Wayman S., Cogger C., Benedict C., Burke I., Collins D., Bary A. (2015). The Influence of Cover Crop Variety, Termination Timing and Termination Method on Mulch, Weed Cover and Soil Nitrate in Reduced-Tillage Organic Systems. Renew. Agric. Food Syst. 30, 450–460. doi: 10.1017/S1742170514000246
Webster T. M., Grey T. L., Flanders J. T., Culpepper A. S. (2009). Cotton Planting Date Affects the Critical Period of Benghal Dayflower (Commelina benghalensis) Control. Weed Sci. 57, 81–86. doi: 10.1614/WS-08-118.1
Werle R., Sandell L. D., Buhler D. D., Hartzler R. G., Lindquist J. L. (2014). Predicting Emergence of 23 Summer Annual Weed Species. Weed Sci. 62, 267–279. doi: 10.1614/WS-D-13-00116.1
Werner P. A., Rioux R. (1977). The Biology of Canadian Weeds. 24. Agropyron repens (L.) Beauv. Can. J. Plant Sci. 57, 905–919. doi: 10.4141/cjps77-130
Westbrook A. S., Bhaskar V., DiTommaso A. (2021). Weed Control and Community Composition in Living Mulch Systems. Weed Res 62, 12–23. doi: 10.1111/wre.12511
Weston L. A., Duke S. O. (2003). Weed and Crop Allelopathy. Crit. Rev. Plant Sci. 22, 367–389. doi: 10.1080/713610861
White S. N., Boyd N. S., Van Acker R. C. (2015). Temperature Thresholds and Growing-Degree-Day Models for Red Sorrel (Rumex acetosella) Ramet Sprouting, Emergence, and Flowering in Wild Blueberry. Weed Sci. 63, 254–263. doi: 10.1614/WS-D-14-00048.1
Williams E. (1971). Germination of Seeds and Emergence of Seedlings of Agropyron repens (L.) Beauv. Weed Res. 11, 171–181. doi: 10.1111/j.1365-3180.1971.tb00993.x
Keywords: emergence periodicity, seedbank, tillage, annual weed, ecologically-based management, stale seedbed
Citation: Brown B, Gallandt ER, DiTommaso A, Salon P, Smith RG, Ryan MR and Cordeau S (2022) Improving Weed Management Based on the Timing of Emergence Peaks: A Case Study of Problematic Weeds in Northeast USA. Front. Agron. 4:888664. doi: 10.3389/fagro.2022.888664
Received: 03 March 2022; Accepted: 13 May 2022;
Published: 21 June 2022.
Edited by:
Karla Leigh Gage, Southern Illinois University Carbondale, United StatesReviewed by:
Vipan Kumar, Kansas State University, United StatesPanagiotis Kanatas, University of Patras, Greece
Virginia Nichols, Arizona State University, United States
Copyright © 2022 Brown, Gallandt, DiTommaso, Salon, Smith, Ryan and Cordeau. This is an open-access article distributed under the terms of the Creative Commons Attribution License (CC BY). The use, distribution or reproduction in other forums is permitted, provided the original author(s) and the copyright owner(s) are credited and that the original publication in this journal is cited, in accordance with accepted academic practice. No use, distribution or reproduction is permitted which does not comply with these terms.
*Correspondence: Stéphane Cordeau, c3RlcGhhbmUuY29yZGVhdUBpbnJhZS5mcg==
†ORCID: Bryan Brown, orcid.org/0000-0002-7666-2209
Eric R. Gallandt, orcid.org/0000-0001-6684-6250
Antonio DiTommaso, orcid.org/0000-0001-8215-2777
Richard G. Smith, orcid.org/0000-0002-3571-5888
Matthew R. Ryan, orcid.org/0000-0001-5667-9452
Stéphane Cordeau, orcid.org/0000-0003-1069-8388