- 1Extension Cropping Systems Weed Science Lab, Department of Agronomy, University of Wisconsin-Madison, Madison, WI, United States
- 2Sustainable Soil Management Lab, Department of Soil Science, University of Wisconsin-Madison, Madison, WI, United States
- 3Nutrient and Pest Management Program, Department of Horticulture, University of Wisconsin-Madison, Madison, WI, United States
- 4Department of Agronomy, Department of Agronomy, University of Wisconsin-Madison, Madison, WI, United States
Adoption of a fall established, high biomass cereal rye cover crop has potential to diversify weed management in corn and soybean production systems, reducing the selection pressure for resistance to postemergence herbicides. However, farmers and crop consultants express concern about limited weed suppression from an overwintering cover crop in areas where high biomass production is limited by cooler spring temperatures, such as in the Upper-Midwest U.S. Use of a preemergence herbicide, regardless of cover crop adoption, is a standard recommendation for improving early season weed control in corn and soybean. Field experiments were conducted at two sites in Wisconsin to assess the effects of six soil management practices (tillage, no-till, and four cereal rye cover crop termination timings/methods) with or without the use of a preemergence herbicide on weed suppression at the time of postemergence herbicide application and crop productivity. Results showed that cereal rye biomass increased > 6x between termination at the time of cash crop planting versus termination two weeks later. In corn and soybean, weed ground cover was lower for soil management with cereal rye cover crop terminated two weeks after cash crop planting (≤ 7% weed cover) compared to all other soil management practices (≥ 23% weed cover) when a preemergence herbicide was not used. Use of a preemergence herbicide resulted in low weed ground cover across treatments in corn (≤ 7% weed cover) and soybean (≤ 13% weed cover). Corn and soybean yield was not affected by preemergence herbicide treatments. Corn yield was lower at the south-central Wisconsin location for the soil management with a cereal rye cover crop terminated two weeks after cash crop planting (9.82 Mg ha-1) compared to all other soil management practices (≥ 12.07 Mg ha-1); at the southwest Wisconsin location, corn yield was greater for the conventional tillage treatment (14.28 Mg ha-1) compared to all other soil management treatments (≤ 10.89 Mg ha-1). Soil management did not affect soybean yield, although yields were different between locations with 3.44 Mg ha-1 at the south-central Wisconsin compared to 4.77 Mg ha-1 at the southwest Wisconsin location. These results indicate that in the absence of a high biomass cereal rye cover crop, preemergence herbicides are important for in-season weed control. Also, the inclusion of a late-terminated cereal rye cover crop in soybean should be considered as an effective management practice for reducing weed ground cover without affecting crop yield.
Introduction
Corn (Zea mays) and soybean (Glycine max) are the most dominant crops typically grown in rotation in the Midwest U.S. In 2019, over 80% of the harvested corn and soybean area in the U.S. was in the Midwest (USDA, 2020). Herbicides are the main method of weed control for these crops and represent a major expense for farmers. Over 92% of the area in corn production and 98% of the area in soybean production in the Midwest received a herbicide application in 2018 (USDA, 2020). Two major challenges for farmers rotating these crops are resiliency of this production system (i.e. susceptibility to erosion, drought, pests) and herbicide resistance.
Resilient cropping systems are less prone to reduced crop productivity and environmental damage imposed by natural disturbances, which are becoming more common with climate change (Bennett et al., 2014; Liang et al., 2017; Bowles et al., 2018). Natural disturbances such as heat and moisture stress during crop pollination/grain fill periods from a warmer summer climate, or delayed crop planting from increased spring precipitation can result in significant crop yield loss (Kucharik and Serbin, 2008). Resilient cropping systems are better able to withstand or recover from disturbances such as increased frequency of extreme precipitation events and abnormal temperature fluctuations (Mishra et al., 2010; Pryor et al., 2014; Gaudin et al., 2015). Practices which improve cropping systems resiliency include adding perennial crops and diversifying crop rotations to increase the duration of living cover and reduce soil disturbance (Gaudin et al., 2015; Sanford et al., 2021); however, infrastructure and demand for cropping options beyond corn and soybean are limited. Adding cover crops to corn and soybean systems can provide many benefits which can help restore ecosystem functions that are diminished by conventional production practices, making current cropping systems more resilient (Blanco-Canqui et al., 2015). Use of a fall established cover crop has been shown to benefit summer annual cropping systems by sequestering soil organic carbon (Blanco-Canqui et al., 2013), moderating soil temperature (Teasdale and Mohler, 1993), improving water infiltration (Basche and DeLonge, 2019), improving water storage (Basche et al., 2016), reducing soil erosion (Blanco-Canqui et al., 2015), and reducing nitrate leaching (Tonitto et al., 2006).
Current crop production systems rely primarily on practices that are “techno-fixes” for weed control (i.e., herbicides, herbicide-tolerant crops, and tillage), meaning they are effective at solving problems in the short term but eventually result in delaying or transforming the problem so that it continues to be a long-term issue (Scott, 2011; MacLaren et al., 2020). Herbicides have benefitted crop production systems by allowing farmers to conserve soil with no-till soil management, although the simplification of crop production systems and dependence on herbicides for weed control has led to the widespread occurrence of evolved herbicide resistance (Young, 2006; Gaines et al., 2020; Heap, 2022). The continued documentation of multiple herbicide resistance mechanisms and cross resistance mechanisms is alarming (Owen et al., 2007; Yerka et al., 2013; Shergill et al., 2018). Even more concerning are cases of metabolic resistance which can impart resistance to herbicide sites of action that a population has not previously been exposed to (Nakka et al., 2017; Shyam et al., 2021). Reducing selection pressure and preventing weeds from producing seed are essential to postpone further herbicide resistance evolution.
Integrated weed management is an approach in which farm managers use multiple practices, technologies, and/or tools in a combined effort to reduce the selection pressure for resistance to any individual control used alone (Norsworthy et al., 2012; Harker and O’Donovan, 2013), maintaining efficacy of preferred control methods, such as herbicides. Controlling early season weeds is a good strategy to reduce the selection pressure on limited postemergence (POST) herbicide options (Norsworthy et al., 2012). Two practices which have been shown to provide control of early season weeds when used separately are cover crops and preemergence (PRE) herbicides. Combining the use of PRE herbicides, a chemical weed control tool with soil residual activity, with cover crops, a physical/biological weed control tool, is one potential method to delay the selection pressure for resistance to POST herbicides. Previous research conducted in the eastern U.S. evaluating the integration of PRE herbicides and cover crops in corn and soybean has shown the potential for effective weed management when integrating these practices (Yenish et al., 1996; Bunchek et al., 2020). Additionally, research conducted in the Midwest indicates successful early-season weed control from a PRE herbicide or use of a cover crop (Ganie et al., 2017; Oliveira et al., 2017; Werle et al., 2017; Grint et al., 2022), but research studying the effects of combining these practices in a Midwest cropping system for integrated weed management to our knowledge remains limited. The effects of cover crops on crop productivity have been shown to be variable depending on geography, cropping system, and cover crop management practices used (Pantoja et al., 2015; Otte et al., 2019; Reed et al., 2019). Challenges of cover crop adoption in the U.S. Midwest include management complications (i.e. establishment and termination), infrastructure limitations (i.e. equipment availability), and uncertainty about the opportunity cost of adopting a cover crop (Roesch-McNally et al., 2018; Oliveira et al., 2019). Research in the Upper-Midwest U.S. is needed to provide farmers in Wisconsin and surrounding states with more information about management decisions and considerations for incorporating a cover crop into current cropping systems.
Field research was conducted to understand how soil management practices [tillage, no-till, and a cereal rye (Secale cereale) cover crop] and use of a PRE herbicide interact to influence weed control and crop productivity in corn-soybean systems. The addition of a cover crop in treatments without PRE herbicide use was hypothesized to improve weed control with increasing cover crop biomass accumulation when compared to soil management with tillage and no-till, and provide additional weed control when used with a PRE herbicide. Findings from this research can help farmers improve weed management and resiliency of their farms by optimizing the integration of a cereal rye cover crop and PRE herbicides into their cropping systems.
Materials and methods
Study establishment and management
Experiments were established in the fall of 2018 in Wisconsin at the University of Wisconsin-Madison Arlington Agricultural Research Station (43°18’36” N, 89°20’50” W; south-central WI) and the Lancaster Agricultural Research Station (42°49’42” N, 90°47’25” W; southwest WI) (Table 1). Soils were classified as Plano silt loam (fine-silty, mixed, superactive, mesic, Typic Argiudoll) at Arlington and Fayette silt loam (fine-silty, mixed, superactive, mesic, Typic Hapludalf) at Lancaster. Each location had two experiments, one planted to corn and one planted to soybean, during the first growing season (2019) that were managed in a corn-soybean rotation in the subsequent years, allowing for a corn and soybean experiment to be present at each location during every year of the study. Data for this study were collected from the 2019 and 2020 growing seasons. Fields were historically managed as a corn-soybean rotation under no-till soil management. Soil organic matter ranged from 2.9-3.5% at Arlington and 2.4-2.5% at Lancaster, whereas soil pH ranged from 6.1-6.5 at Arlington and 6.3-6.8 at Lancaster. Experiments for each crop at each site were established in a randomized complete block design with a six by two factorial treatment structure that included six soil management practices and two PRE herbicide treatments (inclusion or exclusion of a PRE herbicide). Soil management practices included conventional tillage (hereafter ‘tillage’), no-till (‘no-till’), and four cover crop termination timings/methods (early termination [‘early termination’] two weeks prior to crop planting, plant termination [‘plant termination’] at the time of crop planting, forage harvest [‘forage harvest’] at the time of crop planting, and late termination [‘late termination’] two weeks after crop planting). Each experiment had four replicated blocks in which experimental units (plots) were 3-m wide x 9.1-m long. The soil management practice by PRE herbicide treatment combination was maintained in each plot as crops were rotated between growing seasons in each experiment.
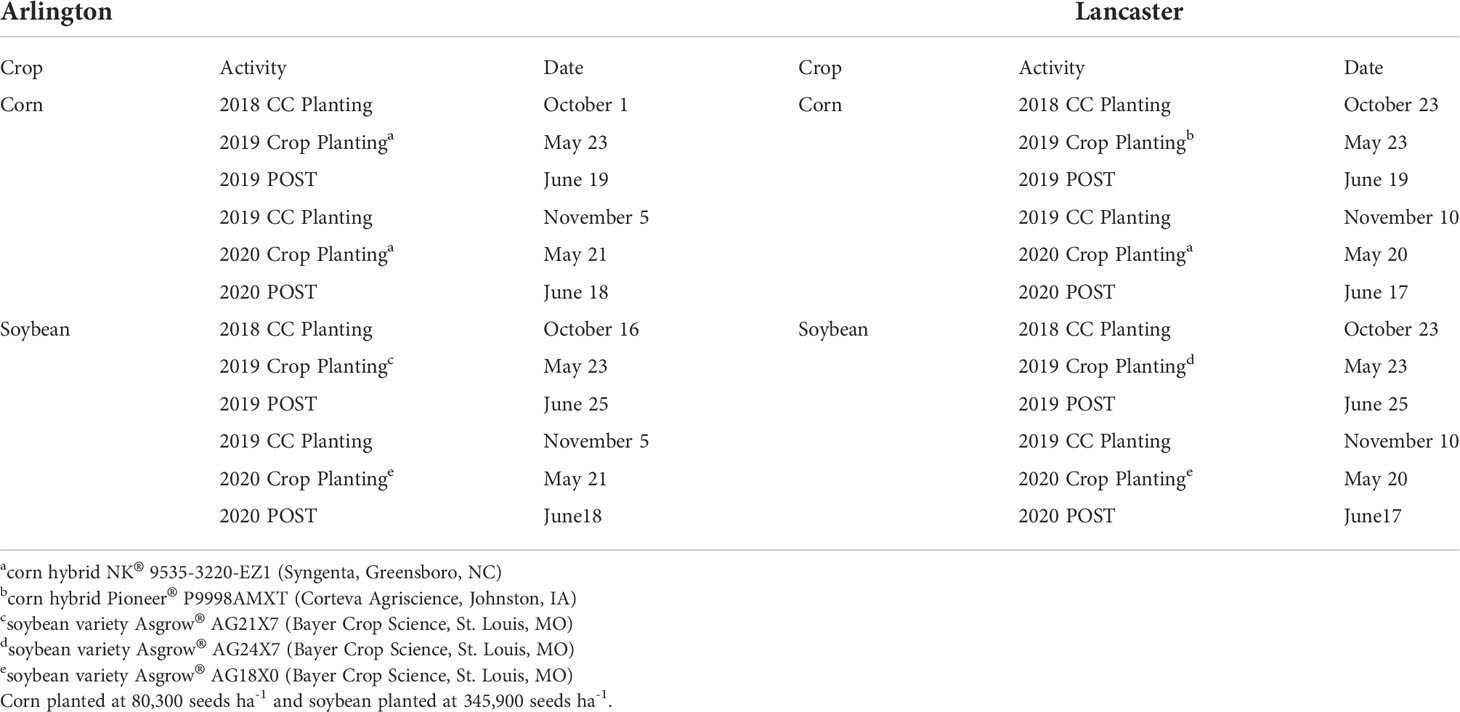
Table 1 Field activity information for corn and soybean experiments with a cereal rye cover crop (CC) conducted in 2019 and 2020 growing seasons at the Arlington Agricultural Research Station and the Lancaster Agricultural Research Station in Wisconsin.
The cereal rye (Guardian Winter Rye, La Crosse Seed, La Crosse, WI) cover crop was drilled at 67 kg ha-1 (Smith et al., 2019) in the fall following harvest of the previous crop at a 3.2 cm seeding depth and 19 cm row-spacing (Table 1). Tillage was conducted in tillage treatments using a chisel-plow in the fall and field cultivator in the spring prior to crop planting. All experiments were broadcast fertilized annually in the spring, prior to crop establishment, according to Wisconsin fertilizer recommendations for optimum nitrogen, phosphorus, and potassium (Laboski and Peters, 2012). Fertilizer rates were calculated based on the requirements of soil managed with no-till for each experiment and the same rate was used for all treatments within an experiment. Arlington corn experiments were fertilized in the spring prior to planting with 336 kg ha-1 of dry urea (46-0-0) in 2019, and 364 kg ha-1 of dry urea and 224 kg ha-1 potash (0-0-60) in 2020. The Arlington soybean experiment in 2020 was fertilized prior to planting with 280 kg ha-1 potash (0-0-60). Lancaster corn experiments were fertilized in the spring prior to planting with 292 kg ha-1 dry urea with a nitrogen stabilizer (46-0-0) and 280 kg ha-1 of a dry phosphorus/potassium fertilizer blend (4-19-38) in 2019, and 292 kg ha-1 dry urea with a nitrogen stabilizer (46-0-0) and 295 kg ha-1 of a dry phosphorus/potassium fertilizer blend (4-19-38) in 2020. Lancaster soybean experiments were fertilized with 295 kg ha-1 of a dry phosphorus/potassium fertilizer blend (4-19-38) in the spring prior to planting in 2019 and 2020. Fertilizer sources used varied due to differences in availability at each location.
Glyphosate (1,262 g a.e. ha-1, Roundup PowerMAX, Bayer Crop Science, St. Louis, MO) with ammonium sulfate (AMS) as an adjuvant (1% v/v) was used for chemical termination of all cover crop soil treatments and to control emerged weeds at the time of crop planting in no-till treatments. In the forage harvest treatment, aboveground biomass was harvested and removed from the plot area immediately before cash crops were planted. Glyphosate was applied for cover crop termination 2 weeks prior to crop planting in the early termination treatment, immediately after crop planting in the plant termination treatment, immediately after forage harvest and crop planting in the forage harvest treatment, and 2 weeks after crop planting in the late termination treatment. Glyphosate was not applied at the time of crop planting in the tillage treatments because established weeds were killed prior to crop planting with tillage. Crops were planted at 3.8 cm depth using a row-crop planter with 76-cm row-spacing (4 crop rows per plot). The PRE herbicides for each crop were applied immediately after crop planting to treatments that included a PRE herbicide. In corn, a PRE herbicide mix containing bicyclopyrone, mesotrione, and S-metolachlor (45, 179, and 1,604 g a.i. ha-1, respectively; Acuron Flexi, Syngenta, Greensboro, NC) was used for treatments that included a PRE herbicide. In soybean, a PRE herbicide mix containing sulfentrazone and metribuzin (202 and 303 g a.i. ha-1, respectively; Authority MTZ, FMC Corporation, Philadelphia, PA) was used for treatments that included a PRE herbicide. Herbicide applications for cover crop termination, no-till treatment burndown, and PRE herbicides were made using a CO2 pressurized backpack sprayer and 3-m long boom with six TTI110015 nozzles (Spraying Systems Co., Wheaton, IL) calibrated at 140 L ha-1. The POST herbicides were applied approximately 30 days after crop planting. For the POST application in corn, glyphosate (1,261.6 g a.e. ha-1, Roundup PowerMAX, Bayer Crop Science, St. Louis, MO) plus dicamba and diflufenzopyr (140.2 and 56.1 g a.e. ha-1, respectively; Status, BASF Corporation, Florham Park, NJ) with AMS (1% v/v) as an adjuvant were applied. For the POST application in soybean, glyphosate (1,261.6 g a.e. ha-1, Roundup PowerMAX, Bayer Crop Science, St. Louis, MO) plus dicamba (558.9 g a.e. ha-1, Xtendimax, Bayer Crop Science, St. Louis, MO) with a drift reducing agent (Intact, 0.5% v/v, Precision laboratories, Waukegan, IL) and a water conditioner (FS Certin, 1% v/v, GROWMARK FS, Bloomington, IL) were applied. A tractor mounted sprayer was used to apply POST herbicides for all treatments with a 6.1-m boom equipped with 12 TTI11003 nozzles (Spraying Systems Co., Wheaton, IL) calibrated to deliver 140 L ha-1 of spray solution.
Data collection
Monthly weather data (mean temperature and total precipitation) were collected from on-site weather stations. Normal (30-year) average temperature and total precipitation for each month (1988-2018) were estimated using historical daily weather data for 1 km grids at each field site using R software (daymetr package) (Thornton et al., 2016; Correndo et al., 2021).
Three subsamples of aboveground cereal rye biomass were collected at termination using a 0.09 m2 quadrat placed randomly between the center rows of each plot not treated with a PRE herbicide to assess differences in cereal rye growth among termination timings. Biomass collection for plots treated with PRE herbicide were also sampled using the same sampling strategy to determine if PRE herbicide applied at crop planting influenced cereal rye biomass in the late termination treatment. All cereal rye subsamples were combined into a composite sample per plot, and forced air dried at 50°C for two weeks until constant dry biomass was achieved before being weighed.
Weed biomass, weed density, and visual estimation of overall weed ground cover between the center rows of each plot (0-100%, with 0% = absence of weed ground cover and 100% = complete ground coverage from weeds) were collected 28 days after crop planting to assess the level of weed infestation and control prior to POST herbicide application. However, weed biomass and density data were deemed impractical for analysis due to inconsistent weed pressure based on sampling size (2 random subsamples from each plot with a 0.09 m2 quadrat). Therefore, visual estimation of weed ground cover was used to represent overall weed pressure in this study. It is suggested future researchers consider using a larger quadrat to obtain representative samples of sparse weed pressure. This sampling method, weed ground cover, was used for assessing weed pressure since populations of weed species were unevenly distributed and occurring at low frequency within studies (Teasdale et al., 2004). At Arlington, weed species present at the time of sampling included common ragweed (Ambrosia artemisiifolia L.), common lambsquarters (Chenopodium album L.), dandelion (Taraxacum officinale F.H. Wigg), yellow foxtail (Setaria pumila (Poir.) Roem. & Schult.), and giant foxtail (Setaria faberi Herrm.). At Lancaster, weed species present at the time of sampling included common lambsquarters, dandelion, waterhemp (Amaranthus tuberculatus (Moq.) Sauer), redroot pigweed (Amaranthus retroflexus L.), giant ragweed (Ambrosia trifida L.), purslane speedwell (Veronica peregrina L.), shepherd’s-purse (capsella bursa-pastoris L. Medik.), eastern black nightshade (Solanum ptychanthum Dunal), hairy galinsoga (Galinsoga quadriradiata Cav.), yellow foxtail, and giant foxtail.
Crop grain mass and moisture content were measured from the center two rows of each plot at crop physiological maturity to assess crop productivity. A Gleaner K2 (AGCO Corporation, Duluth, GA) was used to harvest corn and an ALMACO SPC40 (ALMACO, Nevada, IA) was used to harvest soybean. Grain mass was standardized to 15.5% moisture for corn and 13% moisture for soybean.
Statistical analyses
Statistical analyses were performed using R software (version 4.1.1). A linear mixed model (lme4 package) was fit to cereal rye biomass and crop yield data while a generalized linear mixed model with Template Model Builder with a beta distribution and logit link (glmmTMB package) was fit to weed ground cover data. The linear mixed model assumptions for a normal distribution and homogeneity of variance of residuals were assessed prior to analysis, and data for overall cereal rye biomass were log transformed to better meet assumptions of a normal distribution and homogeneity of variance. Data for each crop at each location were pooled between years and represented by a ‘site’ variable to test for differences between the two growing environments with distinct weed communities and soil type (Arlington versus Lancaster). Models were analyzed using ANOVA (anova function, car package for linear mixed models; Anova.glmmTMB function, glmmTMB package for generalized linear mixed models). Response variables were analyzed with separate models for each crop. In models for overall cereal rye biomass, weed ground cover, and crop yield, the experimental treatments and site were treated as fixed effects while block nested within site-year was treated as a random effect. Means were separated using the Dunn-Sidák correction (emmeans package) when interactions or fixed effects were significant (P < 0.05). When interactions involved site, separate models were fit to the data for each site with the experimental treatment that was a part of the interaction included as a fixed effect and replication nested within year as a random effect. Means were separated when the experimental treatment was significant in ANOVA (P ≤ 0.05).
A linear mixed model was also used to assess if the PRE herbicide was associated with reduced cereal rye biomass in the late termination treatment. Separate models were fit to data for each crop that included PRE herbicide treatment and site as fixed effects and block nested within site-year as a random effect. ANOVA (car package) was performed on these models and means were separated using the Dunn-Sidák correction for significant interactions and fixed effects (P ≤ 0.05).
Results
Weather
Total precipitation accumulated over the growing season (April-October) trended above the 30-year normal for Arlington 2019, Lancaster 2019, and Lancaster 2020 (Table 2). Precipitation trended lower than the 30-year normal for Arlington 2020. Average temperature across the growing season trended below the 30-year normal at both sites for 2019 and 2020.
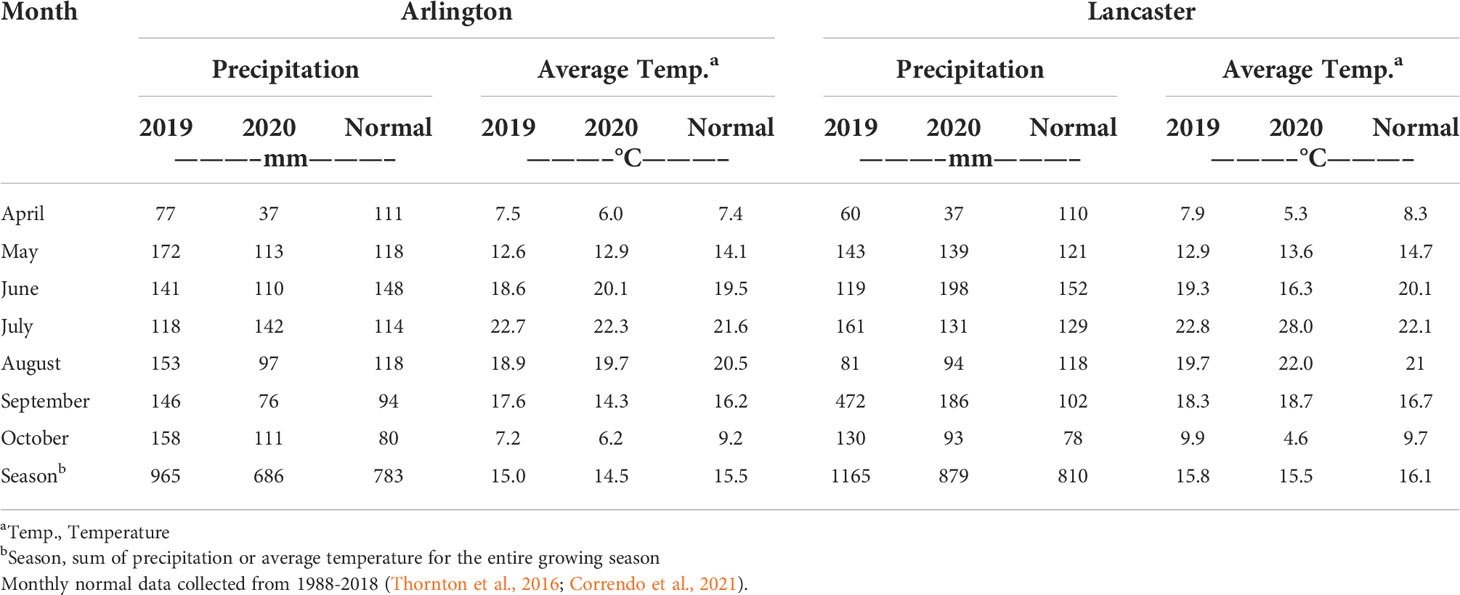
Table 2 Monthly accumulated precipitation and average temperature during the growing season at the Arlington and Lancaster Ag Research Stations in WI.
Cover crop biomass
Timing of termination was significant in the models for cereal rye planted ahead of corn (P = 2.0x10-16) and soybean (P = 2.0x10-16). Site was also significant for cereal rye planted ahead of soybean (P = 4.0x10-3) but not for corn (P = 0.14). For cereal rye planted ahead of corn, biomass increased between each termination timing, with 0.26 Mg ha-1, 0.59 Mg ha-1, and 4.08 Mg ha-1 for early termination, plant termination/forage harvest, and late termination treatment timings, respectively (Table 3). For cereal rye planted ahead of soybean, biomass increased between each termination timing, with 0.17 Mg ha-1, 0.30 Mg ha-1, and 2.59 Mg ha-1 for early termination, plant termination/forage harvest, and late termination treatment timings, respectively. Also, an average biomass accumulation of 0.40 Mg ha-1 was found at Arlington compared to 0.64 Mg ha-1 at Lancaster for cereal rye planted ahead of soybean.
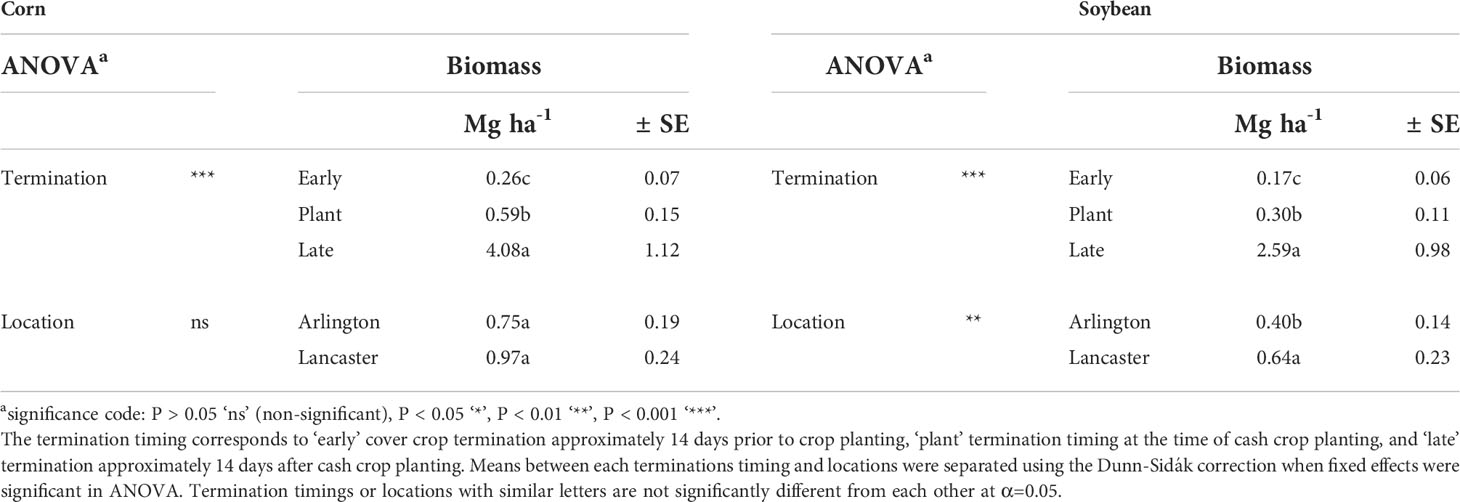
Table 3 Cereal rye cover crop aboveground biomass for corn (left) and soybean (Right) experiments conducted at Arlington and Lancaster Agricultural Research Stations in 2019 and 2020 Wisconsin growing seasons.
The PRE herbicide treatment did not impact biomass accumulation for the late termination of cereal rye in either crop (Corn P = 0.54; Soybean P = 0.37). Average biomass for the cereal rye established in corn was 4.59 Mg ha-1 (SE ± 1.13) for treatments without a PRE herbicide and 4.92 Mg ha-1 (SE ± 1.13) for treatments with a PRE herbicide. Average biomass for the cereal rye established in soybean was 2.83 Mg ha-1 (SE ± 0.69) for treatments without a PRE herbicide and 2.45 Mg ha-1 (SE ± 0.69) for treatments with a PRE herbicide.
Weed ground cover
In corn, the interaction between soil management treatment and PRE herbicide treatment was significant (P = 7.0x10-7), so means for all treatment combinations were compared. Site was not significant (P = 0.71). For treatment combinations without a PRE herbicide, the late termination (7% cover) treatment had less weed ground cover compared to all other treatments followed by the plant termination (23% cover), forage harvest (29% cover), no-till (38% cover), early termination (49% cover), and tillage (53% cover) treatments (Figure 1). Compared to the tillage treatment, weed ground cover was less in plant termination and forage harvest treatments when no PRE herbicide was used. When a PRE herbicide was used, weed ground cover was lower for all soil management treatments (except for the late termination treatment) compared to no PRE (Figure 1). When a PRE herbicide was used, weed ground cover was similar between the tillage (7% cover), no-till (7% cover), early termination (7% cover), plant termination (7% cover), forage harvest (7% cover), and late termination (6% cover) treatments.
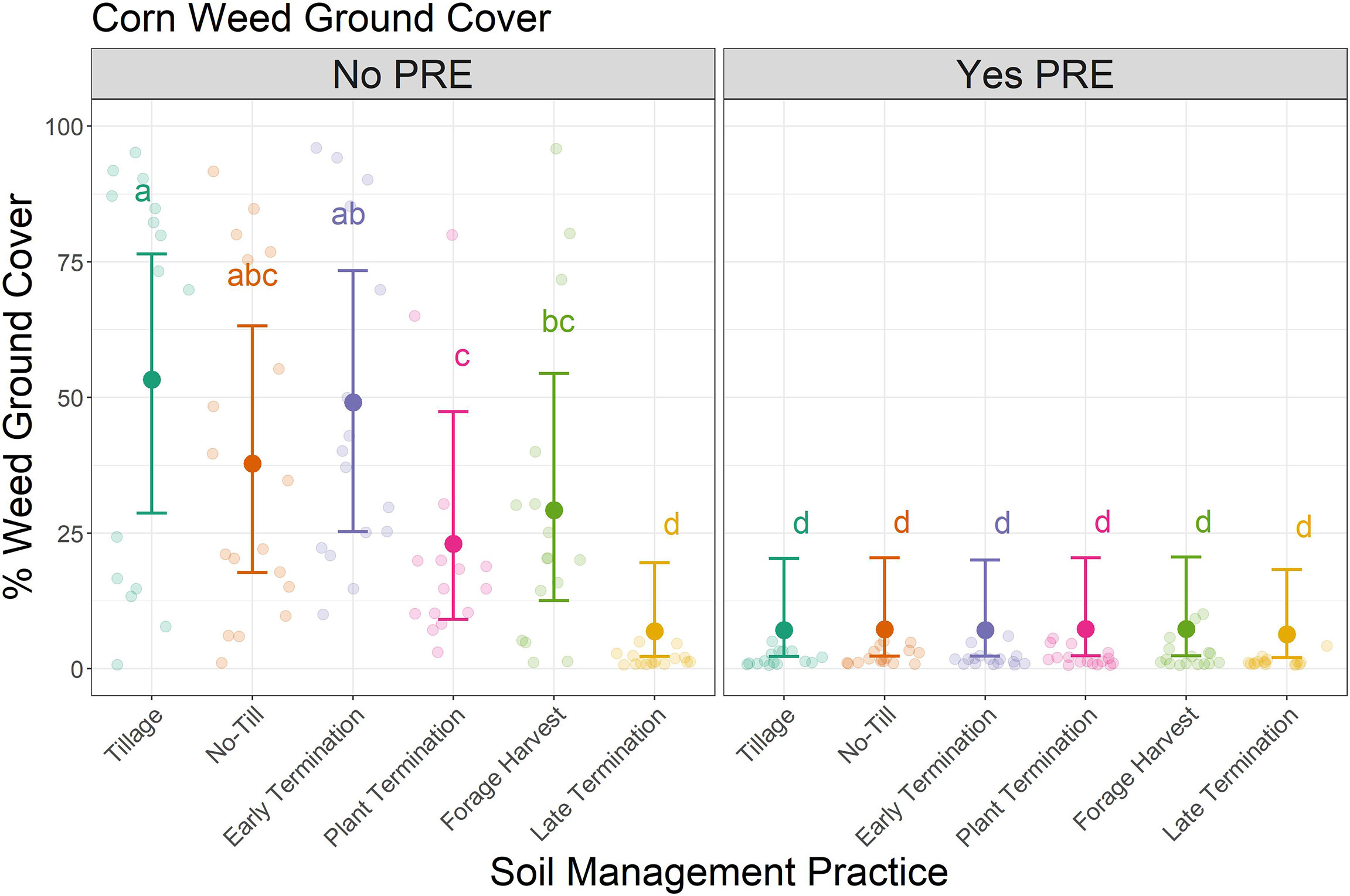
Figure 1 Visual estimation of % weed ground cover 28 days after pre-emergence herbicide (PRE) application in corn for pooled data across Arlington and Lancaster experiment locations in 2019 and 2020. Herbicide treatments included exclusion (No PRE) and inclusion (Yes PRE) of a PRE. Soil management practices include conventional tillage (‘tillage’), no-till (‘no-till’), cereal rye cover crop early termination 14 days before crop planting (‘early termination’), cereal rye plant termination (‘plant termination’), cereal rye forage harvest termination (‘forage harvest’) at the time of crop planting, and cereal rye late termination 14 days after crop planting (‘late termination’). Based on ANOVA results, means were separated using the Dunn-Sidák correction for all soil management x PRE herbicide treatments. Jittered points represent actual data, centered solid points represent means, and error bars represent 95% confidence intervals. Treatments with similar letters are not different at α=0.05.
In soybean, there was a two-way interaction between soil management treatment and location (P = 0.03), as well as a two-way interaction between soil management and PRE herbicide treatment (P = 1.9x10-4) (Figure 2). A separate model was fit to data for each location testing for differences in soil management. Soil management influenced weed cover at both Arlington (P = 0.02) and Lancaster (P = 9.9x10-3). At Arlington, soil management with the late termination treatment (9% cover) had lower weed ground cover compared to the tillage (27% cover), early termination (25% cover), and forage harvest (26% cover) treatments (Figure 2). Ground cover was similar between the tillage, no-till (21% cover), early termination, plant termination (21% cover), and forage harvest treatments. At Lancaster, weed ground cover for the late termination (8% cover) soil management treatment was lower than the no-till (22% cover) treatment, while ground cover was similar between the forage harvest (11% cover), plant termination (12% cover), early termination (16% cover), no-till, and tillage (20% cover) treatments.
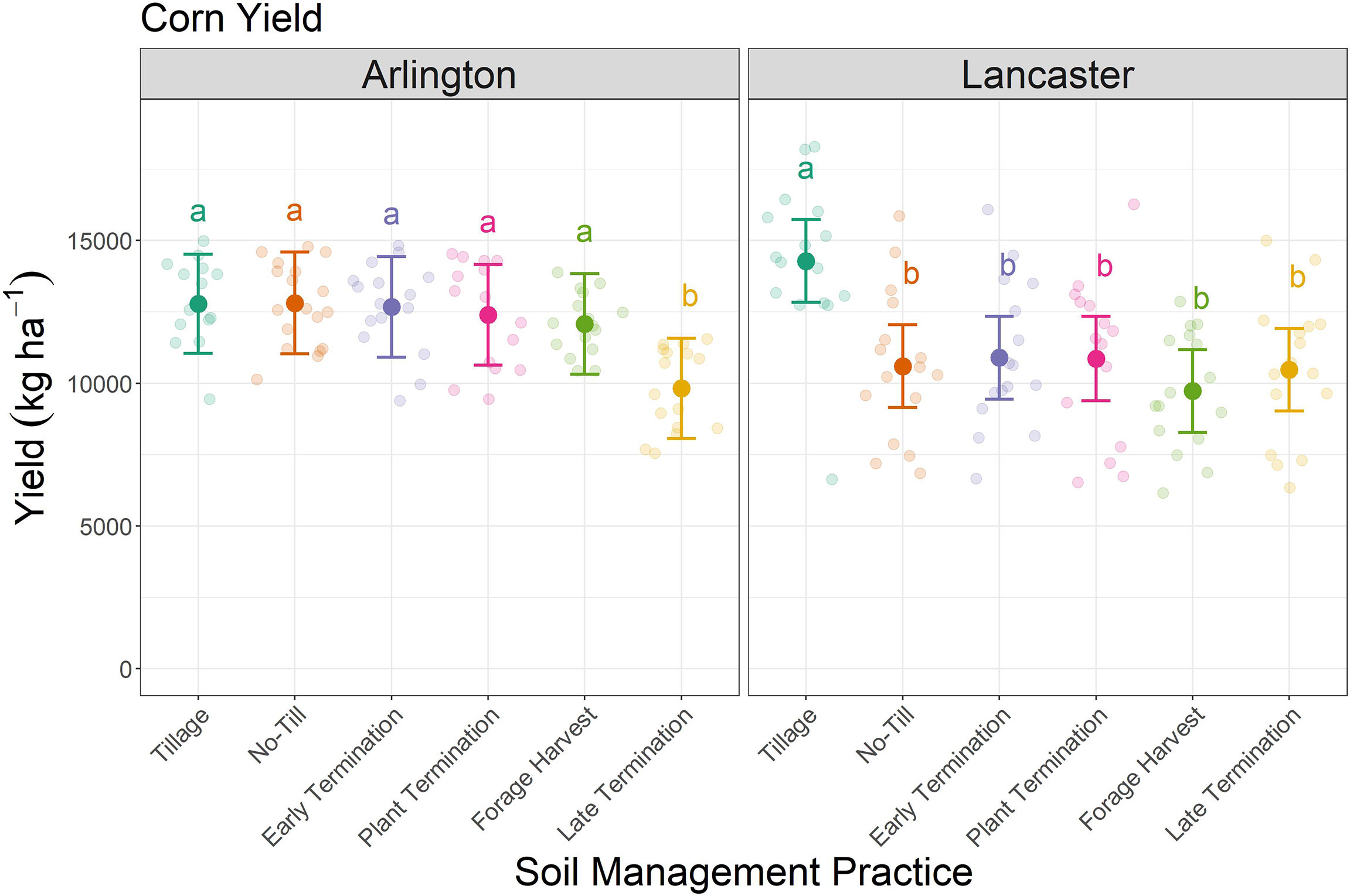
Figure 2 Visual estimation of % weed ground cover 28 days after planting in soybean for data pooled between pre-emergence herbicide treatments levels at Arlington and Lancaster experiment locations in 2019 and 2020. Soil management practices include conventional tillage (‘tillage’), no-till (‘no-till’), cereal rye cover crop early termination 14 days before crop planting (‘early termination’), cereal rye plant termination (‘plant termination’), cereal rye forage harvest termination (‘forage harvest’) at the time of crop planting, and cereal rye late termination 14 days after crop planting (‘late termination’). Based on ANOVA results, means were separated using the Dunn-Sidák correction for all soil management x PRE herbicide treatments. Jittered points represent actual data, centered solid points represent means, and error bars represent 95% confidence intervals. Treatments with similar letters are not different at α=0.05.
To explore the soil management treatment x PRE herbicide treatment interaction in soybean, means were compared across all soil management by PRE herbicide treatment combinations. When no PRE herbicide was used, weed ground cover was lower in the late termination (6% cover) soil management treatment compared to all other treatments (Figure 3). There was more weed ground cover in the tillage (43% cover) treatment compared to the plant termination (20% cover) and forage harvest (20% cover) treatments, while ground cover was similar between the no-till (25% cover), early termination (32% cover), plant termination, and forage harvest treatments with no PRE herbicide (Figure 3). When a PRE herbicide was used, weed ground cover was similar between the tillage (9% cover), no-till (12% cover), early termination (10% cover), plant termination (10% cover), forage harvest (13% cover), and late termination (5% cover) treatments.
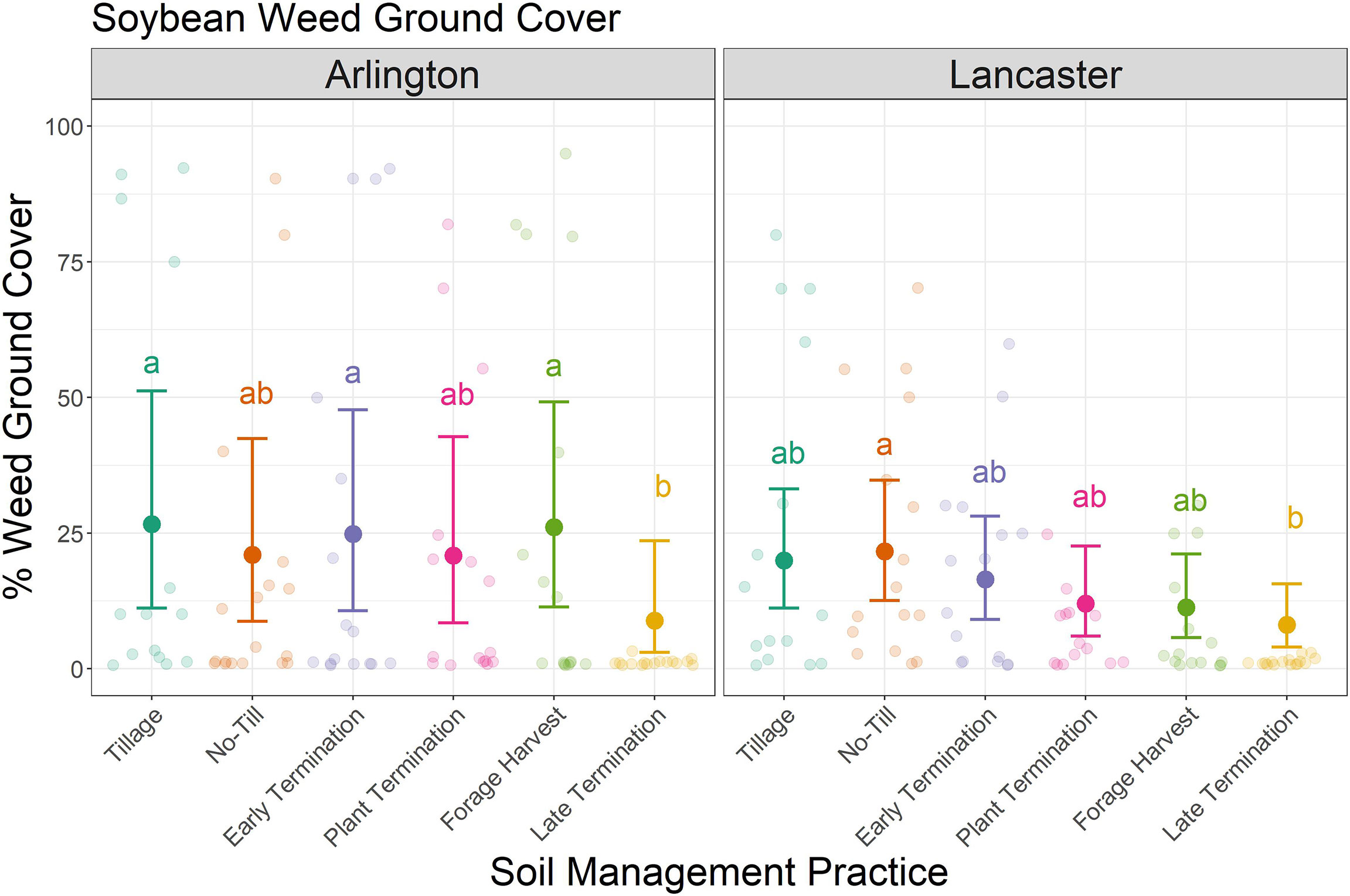
Figure 3 Visual estimation of % weed ground cover 28 days after pre-emergence herbicide (PRE) application in soybean for pooled data across Arlington and Lancaster experiment locations in 2019 and 2020. Herbicide treatments included exclusion (No PRE) and inclusion (Yes PRE) of a PRE. Soil management practices include conventional tillage (‘tillage’), no-till (‘no-till’), cereal rye cover crop early termination 14 days before crop planting (‘early termination’), cereal rye plant termination (‘plant termination’), cereal rye forage harvest termination (‘forage harvest’) at the time of crop planting, and cereal rye late termination 14 days after crop planting (‘late termination’). Based on ANOVA results, means were separated using the Dunn-Sidák correction for all soil management x PRE herbicide treatments. Jittered points represent actual data, centered solid points represent means, and error bars represent 95% confidence intervals. Treatments with similar letters are not different at α=0.05.
Crop yield
For corn yield, there was a two-way interaction between site and soil management treatment (P = 8.6x10-6) while PRE herbicide treatment had no effect on yield (P = 0.11). Individual models for each site were then fit to data to evaluate if soil management influenced yield at each site. Yield was influenced by soil management at both Arlington (P = 7.1x10-12) and Lancaster (P = 1.0x10-5). At Arlington, soil management in the late termination treatment (9.82 Mg ha-1) had reduced yield compared to the forage harvest (12.07 Mg ha-1), plant termination (12.39 Mg ha-1), early termination (12.67 Mg ha-1), no-till (12.81 Mg ha-1), and tillage (12.78 Mg ha-1) treatments (Figure 3). At Lancaster, the tillage (14.28 Mg ha-1) treatment had greater yield compared to the late termination (10.47 Mg ha-1), forage harvest (9.72 Mg ha-1), plant termination (10.86 Mg ha-1), early termination (10.89 Mg ha-1), and no-till (10.60 Mg ha-1) treatments (Figure 4).
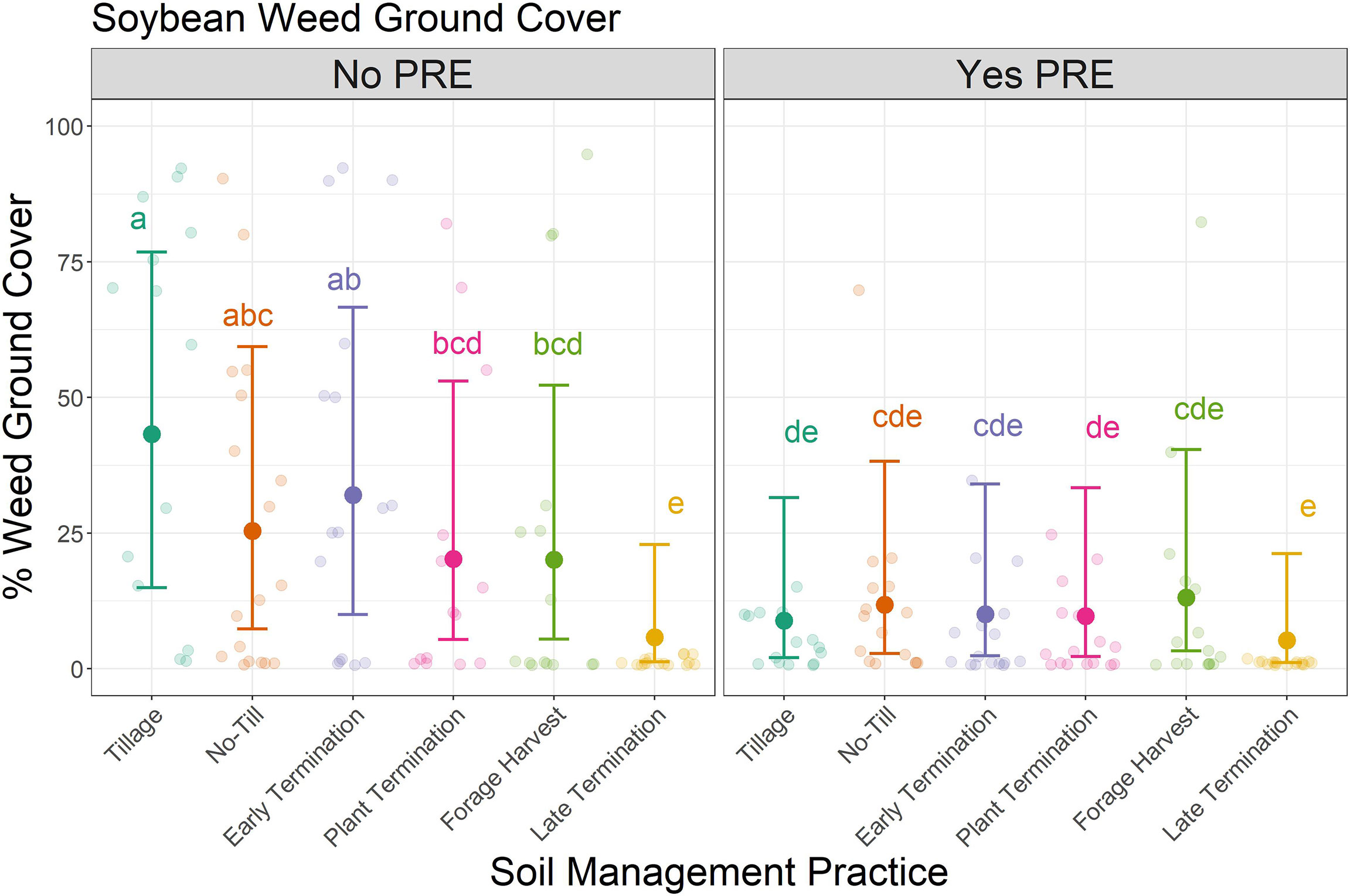
Figure 4 Corn grain yield for pooled data across pre-emergence herbicide treatment levels at Arlington and Lancaster experiment locations in 2019 and 2020. Soil management practices include conventional tillage (‘tillage’), no-till (‘no-till’), cereal rye cover crop early termination 14 days before crop planting (‘early termination’), cereal rye plant termination (‘plant termination’), cereal rye forage harvest termination (‘forage harvest’) at the time of crop planting, and cereal rye late termination 14 days after crop planting (‘late termination’). Jittered points represent actual data, centered solid points represent means, and error bars represent 95% confidence intervals. Treatments with similar letters are not different at α=0.05.
For soybean yield, the site fixed effect was significant (P = 4.7x10-7), while soil management treatment (P = 0.12) and PRE herbicide treatment (P = 0.16) were found to not influence yield. The average soybean yield was 3.44 Mg ha-1 at Arlington and 4.77 Mg ha-1 at Lancaster (Figure 5).
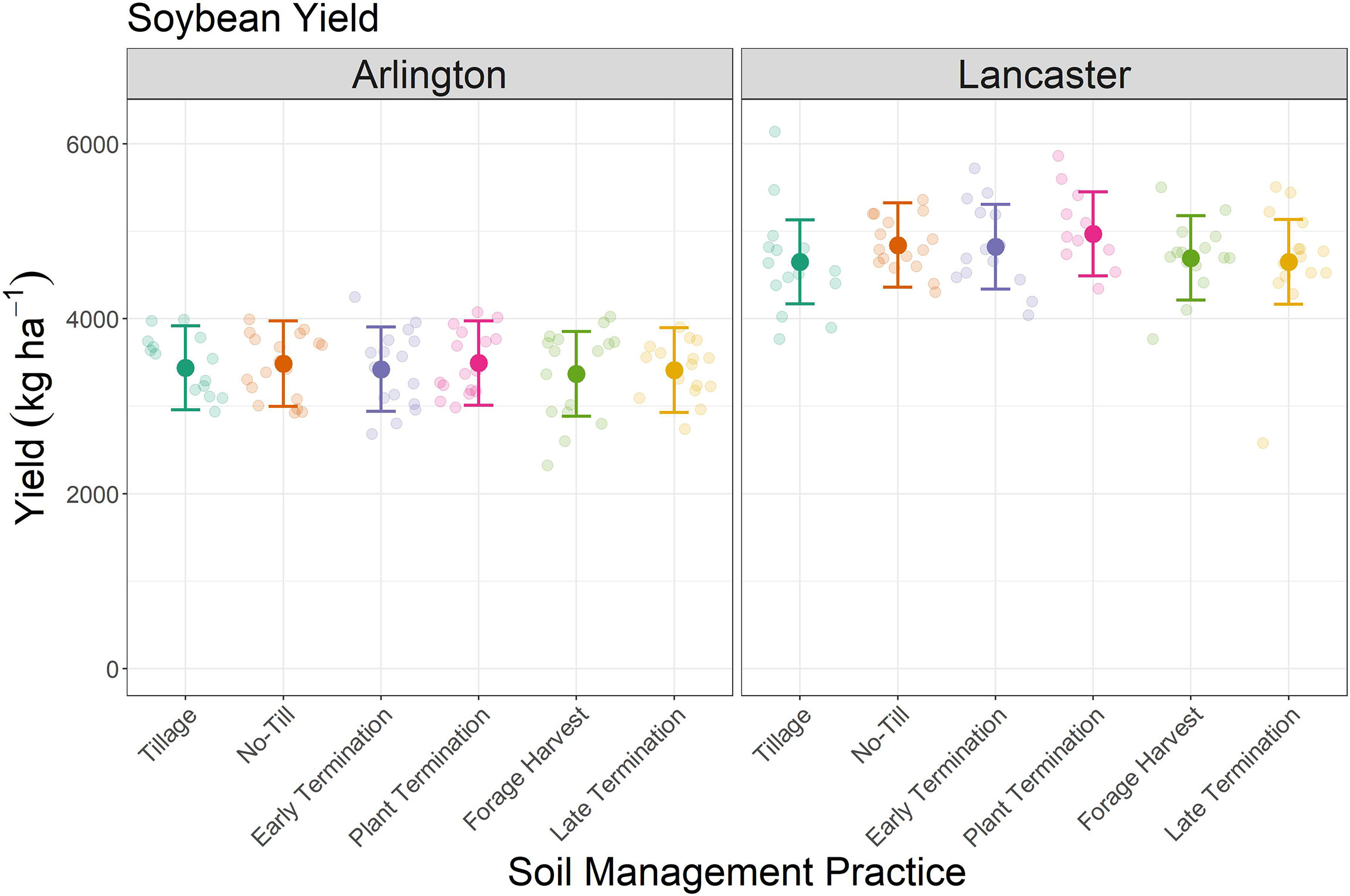
Figure 5 Soybean yield for pooled data across pre-emergence herbicide treatment levels at Arlington and Lancaster experiment locations in 2019 and 2020. Soil management practices include conventional tillage (‘tillage’), no-till (‘no-till’), cereal rye cover crop early termination 14 days before crop planting (‘early termination’), cereal rye plant termination (‘plant termination’), cereal rye forage harvest termination (‘forage harvest’) at the time of crop planting, and cereal rye late termination 14 days after crop planting (‘late termination’). Jittered points represent actual data, centered solid points represent means, and error bars represent 95% confidence intervals. Yield was similar between all treatments at each location.
Discussion
Cover crop biomass
Biomass produced by the cereal rye cover crop remained relatively low (< 0.3 Mg ha-1) for the early and at plant cover crop termination timings, with biomass accumulation increasing drastically between the plant termination/forage harvest and late termination treatments for both corn and soybean phases of the rotation (Table 3). Despite herbicide injury on cereal rye leaf foliage from the soybean PRE herbicide (Grint, personal field observation), cereal rye biomass for the late termination treatment was not affected in either crop. There was no visual injury on cereal rye leaf foliage from the corn PRE herbicide (Grint, personal field observation). Future research should be conducted to assess the effects of more PRE herbicide products on cover crop growth to support farmers in selecting PRE herbicides that have effective activity on weeds when applied to a living cover crop while still allowing for continued cover crop growth if later termination is desired to increase the amount of cover crop biomass accumulated and the likelihood of achieving subsequent cover crop benefits. Other studies conducted in Wisconsin have reported similar cereal rye biomass production to that reported here with 0.28-3.44 Mg ha-1 with termination in early May and 2.30-6.00 Mg ha-1 for termination at the rye boot growth stage (West et al., 2020). This study would have benefited from detailed data on cereal rye growth stage at the time of cover crop termination. Previous research conducted in Wisconsin organic soybean production with a greater cereal rye seeding rate and delayed cereal rye termination until after the boot growth stage, indicates that there is potential to produce cereal rye biomass of 4.30-10.80 Mg ha-1 (Bernstein et al., 2011; Vincent-Caboud et al., 2019). Greater amounts of cereal rye biomass accumulation prior to crop planting have been observed in previous studies with 2.05-3.25 Mg ha-1 in Maryland (Otte et al., 2019), 7.39 Mg ha-1 in Virginia (Pittman et al., 2020), 4.97 Mg ha-1 in South Carolina (Norsworthy, 2004), 2.20-6.10 Mg ha-1 in Illinois (Ruffo et al., 2004), and 1.15-2.89 Mg ha-1 in Missouri (Cornelius and Bradley, 2017). The warmer spring growing conditions at these locations are favorable for a cereal rye cover crop to accumulate more biomass prior to the typical time of crop planting when compared to covers grown in the Upper-Midwest U.S. (Kukal and Irmak, 2018). Years of reduced or delayed cover crop biomass production from unfavorable fall and/or spring climate are common in the Midwest U.S. (Krueger et al., 2011; Pantoja et al., 2015; West et al., 2020), and should be considered when planning for an early cereal rye termination in this region. Cover crop benefits, such as weed suppression, have been shown to be influenced by the amount of cover crop biomass produced. According to a meta-analysis from corn-soybean studies conducted in the Midwest U.S., at least 5 Mg ha-1 of cover crop biomass is needed for 75% reduction in weed biomass (Nichols et al., 2020). The average amount of cereal rye biomass produced in this study was below this threshold. Future research should be conducted to evaluate whether the amount of cereal rye cover crop biomass necessary to effectively suppress diverse weed communities at varying infestation levels change across geographies.
Weed control
Soil management impacted weed ground cover in both the corn and soybean phases of this study. When no PRE herbicide was used in corn, the lowest weed ground cover was in the late termination treatment while weed ground cover observed for the plant termination and forage harvest treatments lower than the tillage treatment (Figure 1). In soybean, lower weed ground was consistently observed in the late termination treatment compared to the tillage treatment at both locations (Figure 2). When no PRE herbicide was used in soybean, lower weed ground cover was observed for the late termination treatment compared to all other soil management treatments, while weed ground cover observed for the plant termination and forage harvest treatments was lower than tillage treatment (Figure 3). The inconsistent occurrence and variable density of weed species within experiments was limiting in this study to assess potential weed community impacts from soil management, however previous research conducted in Wisconsin observed greater weed biomass for soil management with tillage compared to a cereal rye cover crop in soybean (Bernstein et al., 2014) and greater density of common lambsquarters in chisel-plow corn compared to no-till soybean (Drewitz and Stoltenberg, 2018). Common lambsquarters was the most consistently observed species, however weed ground cover was not different between the tillage and no-till treatments in this study (Figures 1–3). Soil management that resulted in greater cereal rye biomass production such as occurred with the late, plant, and forage harvest cover crop termination timings/methods had lower weed ground cover compared to soil management with tillage in both crops (Figures 1–3). It is important to acknowledge that the action of chemical cover crop termination with glyphosate in this study likely contributed to weed control of emerged weeds for each termination timing thus impacting weed ground cover. Increased levels of cereal rye biomass accumulation have been shown to increase weed suppression from a cereal rye cover crop by delaying emergence of weeds, reducing emergence of weed seedlings, and reducing the amount of weed biomass (Mohler and Teasdale, 1993; Yenish et al., 1996; Bernstein et al., 2014; Cornelius and Bradley, 2017; Werle et al., 2017; Pittman et al., 2020). In addition, the cereal rye biomass from the late termination treatments was observed to persist longer into the growing season, and cereal rye residue from 2019 could still be found in the plots of the late termination treatments during the spring of 2020 (Grint, personal observation). As cereal rye matures, the carbon to nitrogen ratio has been shown to increase, prolonging the persistence of residue from delayed cereal rye cove crop terminations (Poffenbarger et al., 2015). The combination of increased cereal rye biomass and prolonged cereal rye residue persistence are ideal for weed suppression (Pittman et al., 2020).
Treatments containing a PRE herbicide often had lower weed ground cover in corn and soybean. In corn, there was lower weed ground cover for treatments combinations containing a PRE herbicide for all soil management treatments except for the late termination treatment, indicating that the use of a PRE herbicide did not provide additional control only when delayed termination of a high biomass cereal rye cover crop was implemented (Figure 1). In soybean, tillage and early termination treatment combinations which included a PRE herbicide had lower weed ground cover compared to tillage and early termination treatment combinations without a PRE herbicide (Figure 3). Previous research indicates the use of a PRE herbicide improves weed control when soil is managed with use of tillage, no-till, or a cover crop and can contribute to greater crop yield when weed pressure is high (Yenish et al., 1996; Reddy et al., 2003). A cereal rye cover crop has been shown to provide a similar level of weed suppression compared to use of a PRE herbicide in some cases and to reduce late season weed biomass when no PRE herbicide is used compared to soil management with tillage (Yenish et al., 1996). Previous research suggests that the use of a cover crop alone for early season weed suppression has the potential to increase selection pressure for resistance to POST herbicides by increasing the density of summer annual weeds after initial suppression (Bunchek et al., 2020). Our results do not support this suggestion, still the use of a cereal rye cover crop to reduce selection pressure for herbicide resistance should consider the influence of a cover crop on weeds prior to all potential herbicide applications. This study would have benefited from data on weed species, weed size, and weed density at the time of POST herbicide application to better assess how the interaction of PRE herbicide use with specific soil management strategies affects the risk for resistance to POST herbicides. The integration of a cover crop into a crop production system has the potential to reduce selection pressure for herbicide resistance by reducing weed size and density if long-term suppression occurs, but more research is needed to assess best management of a cereal rye cover crop used in conjunction with herbicides for weed control while reducing the selection pressure for herbicide resistance. Future research that
Crop yield
Different yield results between soil management practices were observed in corn phases between locations. At Arlington, corn yield was lower in the late termination treatment compared to all other soil management treatments (Figure 4). At Lancaster, corn yield was greater in the tillage treatment compared to all other treatments. Previous studies in Wisconsin have observed no difference in corn grain yield in a corn-soybean rotation between soil management with no-till and tillage (Pedersen and Lauer, 2003; Mourtzinis et al., 2017). No difference in corn yield between soil management with no-till and an early terminated cereal rye cover crop in this study contradicts research conducted in Iowa which reported a reduced corn yield when cereal rye was terminated approximately 7 days prior to corn planting (Pantoja et al., 2015). Similar corn yield between soil management with a cereal rye cover crop terminated 14 days before planting and cereal rye cover crop terminated at the time of crop planting in this study contradicts research in Iowa which found reduced corn yield when rye termination occurred < 10 days before crop planting (Acharya et al., 2017). Reduced corn grain yield in the late termination treatment at Arlington is supported by research conducted in Maryland, where greater amounts of cereal rye biomass production reduced corn grain yield compared to no cover crop, and Iowa, where termination 12 days after planting reduced corn grain yield (Otte et al., 2019; Acharya et al., 2022). Previous research assessing the long-term impact of using a cereal rye terminated prior to crop establishment has found no reductions in corn yield (Basche et al., 2016; Snapp and Surapur, 2018). Corn grain yield reduction from a cereal rye could be a result of less available nitrogen early in the growing season. Lower nitrogen content in corn plants has been observed with greater amounts of cereal rye biomass from delayed termination when compared to early termination timings and absence of a cover crop (Otte et al., 2019). Reduced soil nitrate at the time of corn planting with delayed cereal rye termination and nitrogen immobilization during peak timing for corn nitrogen demand have been observed (Crandall et al., 2005; Nevins et al., 2020). It is suspected that slow nitrogen release or nitrogen immobilization following late termination of cereal rye could limit available nitrogen for the corn crop, therefore reducing yield. Another potential cause of yield reduction in corn is cooler and drier soils from delayed cereal rye termination (Reed et al., 2019). Reduction of soil moisture by a cereal rye cover crop has been shown to negatively impact corn yield in semi-arid regions (Rosa et al., 2021). Unfortunately, not enough soil environmental data were collected across treatments in this study (i.e., soil moisture, soil temperature, nutrient availability) to support inferences about location differences that could have explained the lower corn yield in the late cover crop termination at Arlington versus no yield reduction in the same treatment at Lancaster when yield was compared to most of the other soil management treatments. Not enough research funds and labor were available at the time this study was established to collect such data.
Soybean yield remained consistent regardless of soil management practice being used for both locations (Figure 5). Previous research in Wisconsin comparing no-till soil management to tillage has found no yield difference (Mourtzinis et al., 2017), greater yield with tillage (Pedersen and Lauer, 2002; Arsenijevic et al., 2021), or greater yield with no-till (Pedersen and Lauer, 2003). Results from this study match previous research where no soybean yield difference was observed from a cereal rye cover crop terminated prior to crop planting compared to no-till (Pantoja et al., 2015), and no difference in soybean yield observed between a cereal rye termination after crop planting compared to termination prior to planting (Reed et al., 2019). Previous research in Nebraska also observed no difference in soybean yield between soil management with no-till, cereal rye termination prior to planting, termination at the time of planting, and termination 5-7 days after crop planting (McMechan et al., 2020, McMechan et al., 2021). Long-term research indicates soybean yield remains unaffected by use of a cereal rye cover crop when compared to no-till (Basche et al., 2016). Based on the results of this study and previous research, delayed termination of a cereal rye cover crop in soybean cropping systems can improve the potential of achieving weed suppression and other ecological benefits (i.e. reduced soil erosion, carbon sequestration, water infiltration) from cereal rye with less likelihood of reduced farmer income from yield reduction.
Integrated weed management and cropping system resiliency with conservation practices
Herbicides are currently a more efficient technology for achieving reliable weed control in cropping systems when compared to use of a cover crop alone (Yenish et al., 1996; Reddy et al., 2003; Bunchek et al., 2020). However, their effectiveness and wide-spread use results in strong selection pressure for the development of herbicide resistance (Young, 2006; Peterson et al., 2018). Novel cases of herbicide resistance are being reported at an alarming frequency (Heap, 2022), and likelihood of resistance to multiple herbicides is becoming more common with the development of metabolic resistance (Gaines et al., 2020; Han et al., 2021; Shyam et al., 2021). These are major concerns for the future use of herbicides as tools for weed control (Oliveira et al., 2020). Integrated weed management is needed to proactively manage weeds while relieving selection pressure for resistance and protecting future use of herbicides as weed management tools (Norsworthy et al., 2012; Hicks et al., 2018; MacLaren et al., 2020). The use of a cover crop has been shown to provide many environmental benefits to agricultural systems such as reduced soil erosion, reduced nitrate leaching, and improved soil water infiltration. An improved understanding of how best to use these soil management practices with herbicides can benefit integrated weed management and improve ecological sustainability of corn and soybean production systems.
Conclusion
In corn and soybean, delaying termination of a cereal rye cover crop until 14 days after crop planting to increase the amount of the cereal rye residue reduced the presence of weeds 28 days after crop planting when no PRE herbicide was used compared to all other soil management practices, and had similar weed suppression compared to when a PRE herbicide was used for all soil management practices. This finding supports our hypothesis that the addition of a cover crop in treatments without PRE herbicide use was expected to improve weed control with increasing cover biomass accumulation. Soil management with a late terminated cereal rye cover crop had lower corn yield at one site and had no effect on soybean yield at either site. These findings demonstrate delaying cereal rye cover crop termination to increase rye biomass suppressed weeds in Wisconsin and that PRE herbicides are effective for in-season weed control when a high biomass cereal rye cover crop is not present. Adoption of a late terminated cereal rye cover crop in soybean can reduce the presence of weeds with reduced risk of yield loss making this a beneficial practice for farmers to adopt in this region.
Data availability statement
The datasets presented in this study can be found in online repositories. The names of the repository/repositories and accession number(s) can be found below: https://github.com/kgrint/Cover-Crop-and-PRE-Herbicides-Publication.git.
Author contributions
RW designed the study and secured funding for the research. RD established the Arlington, WI studies. RD, KG, and NA managed the Arlington, WI studies. DHS, KG, and NA established and managed the Lancaster, WI studies. KG, MO, and RW analyzed the data. KG, RW, MO, DHS, FA, NA, and DES were involved with the interpretation of results, contributed to writing the original manuscript, and improving subsequent versions. All authors reviewed and approved the submitted article.
Funding
This work was partially funded through a North Central SARE graduate student grant awarded to KG (#GNC19-275) and by the Wisconsin Soybean Marketing Board and the Wisconsin Corn Promotion Board.
Acknowledgments
We thank the contributions of the staff at Lancaster Agricultural Research Station for monitoring and establishing the experiments. We thank the field assistance provided by the University of Wisconsin-Madison Extension Cropping Systems Weed Science lab for their technical assistance with these field experiments, and Dr. Alexandre Rosa for his assistance during the initial review of this publication.
Conflict of interest
The authors declare that the research was conducted in the absence of any commercial or financial relationships that could be construed as a potential conflict of interest.
Publisher’s note
All claims expressed in this article are solely those of the authors and do not necessarily represent those of their affiliated organizations, or those of the publisher, the editors and the reviewers. Any product that may be evaluated in this article, or claim that may be made by its manufacturer, is not guaranteed or endorsed by the publisher.
References
Acharya J., Bakker M. G., Moorman T. B., Kaspar T. C., Lenssen A. W., Robertson A. E. (2017). Time interval between cover crop termination and planting influences corn seedling disease, plant growth, and yield. Plant Dis. 101 (4), 591–600. doi: 10.1094/PDIS-07-16-0975-RE
Acharya J., Moorman T. B., Kaspar T. C., Lenssen A. W., Gailans S., Robertson A. E (2022). Effect of planting into a green winter cereal rye cover crop on growth and development, seedling disease, and yield of corn. Plant Dis. 106 (1), 114–120. doi: 10.1094/PDIS-04-21-0836-RE
Arsenijevic N., DeWerff R., Conley S., Ruark M., Werle R. (2021). Influence of integrated agronomic and weed management practices on soybean canopy development and yield. Weed Technol. 36, 1–6. doi: 10.1017/wet.2021.92
Basche A. D., DeLonge M. S. (2019). Comparing infiltration rates in soils managed with conventional and alternative farming methods: A meta-analysis. PloS One 14 (9), e0215702. doi: 10.1371/journal.pone.0215702
Basche A. D., Kaspar T. C., Archontoulis S. V., Jaynes D. B., Sauer T. J., Parkin T. B., Miguez F. E., et al. (2016). Soil water improvements with the long-term use of a winter rye cover crop. Agric. Water Manage. 172, 40–50. doi: 10.1016/j.agwat.2016.04.006
Bennett E., Carpenter S., Gordon L., Ramankutty N., Balvanera P., Campbell B., et al. (2014). Toward a more resilient agriculture. Solutions 5 (5), 65–75.
Bernstein E. R., Posner J. L., Stoltenberg D. E., Hedtcke J. L. (2011). Organically managed no-tillage rye–soybean systems: Agronomic, economic, and environmental assessment. Agron. J. 103 (4), 1169–1179. doi: 10.2134/agronj2010.0498
Bernstein E. R., Stoltenberg D. E., Posner J. L., Hedtcke J. L. (2014). Weed community dynamics and suppression in tilled and no-tillage transitional organic winter rye–soybean systems. Weed Sci. 62 (1), 125–137. doi: 10.1614/WS-D-13-00090.1
Blanco-Canqui H., Holman J. D., Schlegel A. J., Tatarko J., Shaver T. M. (2013). Replacing fallow with cover crops in a semiarid soil: Effects on soil properties. Soil Sci.Soc. Am. J. 77 (3), 1026–1034. doi: 10.2136/sssaj2013.01.0006
Blanco-Canqui H., Shaver T. M., Lindquist J. L., Shapiro C. A., Elmore R. W., Francis C. A, et al. (2015). Cover crops and ecosystem services: Insights from studies in temperate soils. Agron. J. 107 (6), 2449–2474. doi: 10.2134/agronj15.0086
Bowles T. M., Atallah S. S., Campbell E. E., Gaudin A. C. M., Wieder W. R., Grandy A. S (2018). Addressing agricultural nitrogen losses in a changing climate. Nat. Sustainability 1 (8), 399–408. doi: 10.1038/s41893-018-0106-0
Bunchek J. M., Wallace J. M., Curran W. S., Mortensen D. A., VanGessel M. J., Scott B. A (2020). Alternative performance targets for integrating cover crops as a proactive herbicide-resistance management tool. Weed Sci. 68 (5), 534–544. doi: 10.1017/wsc.2020.49
Cornelius C. D., Bradley K. W. (2017). Influence of various cover crop species on winter and summer annual weed emergence in soybean. Weed Technol. 31 (4), 503–513. doi: 10.1017/wet.2017.23
Correndo A. A., Moro Rosso L. H., Ciampitti I. A. (2021). Agrometeorological data using r-software. (Harvard Dataverse) doi: 10.7910/DVN/J9EUZU
Crandall S. M., Ruffo M. L., Bollero G. A. (2005). Cropping system and nitrogen dynamics under a cereal winter cover crop preceding corn. Plant Soil 268 (1), 209–219. doi: 10.1007/s11104-004-0272-x
Drewitz N. M., Stoltenberg D. E. (2018). Weed communities in strip-tillage corn/no-tillage soybean rotation and chisel-plow corn systems after 10 years of variable management. Weed Sci. 66 (5), 651–661. doi: 10.1017/wsc.2018.40
Gaines T. A., Duke S. O., Morran S., Rigon C. A. G., Tranel P. J., Küpper A., et al. (2020). Mechanisms of evolved herbicide resistance. J. Biol. Chem. 295 (30), 10307–10330. doi: 10.1074/jbc.REV120.013572
Ganie Z. A., Lindquist J. L., Jugulam M., Kruger G. R., Marx D. B., Jhala A. J (2017). An integrated approach to control glyphosate-resistant ambrosia trifida with tillage and herbicides in glyphosate-resistant maize. Weed Res. 57 (2), 112–122. doi: 10.1111/wre.12244
Gaudin A. C. M., Tolhurst T. N., Ker A. P., Janovicek K., Tortora C., et al. (2015). Increasing crop diversity mitigates weather variations and improves yield stability. PloS One 10 (2), e0113261. doi: 10.1371/journal.pone.0113261
Grint K. R., Arneson N. J., Oliveira M. C., Smith D. H., Werle R. (2022). Cereal rye cover crop terminated at crop planting reduces early-season weed density and biomass in Wisconsin corn–soybean production. Agrosystems Geosciences Environ. 5 (1), e20245. doi: 10.1002/agg2.20245
Han H., Yu Q., Beffa R., González S., Maiwald F., et al. (2021). Cytochrome P450 CYP81A10v7 in lolium rigidum confers metabolic resistance to herbicides across at least five modes of action. Plant J. 105 (1), 79–92. doi: 10.1111/tpj.15040
Harker K. N., O’Donovan J. T. (2013). Recent weed control, weed management, and integrated weed management. Weed Technol. 27 (1), 1–11. doi: 10.1614/WT-D-12-00109.1
Heap I. (2022) The international herbicide-resistant weed database. Available at: www.weedscience.org (Accessed 28 February 2022).
Hicks H. L., Comont D., Coutts S. R., Crook L., Hull R., et al. (2018). The factors driving evolved herbicide resistance at a national scale. Nat. Ecol. Evol. 2 (3), 529–536. doi: 10.1038/s41559-018-0470-1
Krueger E. S., Ochsner T. E., Porter P. M., Baker J. M. (2011). Winter rye cover crop management influences on soil water, soil nitrate, and corn development. Agron. J. 103 (2), 316–323. doi: 10.2134/agronj2010.0327
Kucharik C. J., Serbin S. P. (2008). Impacts of recent climate change on Wisconsin corn and soybean yield trends. Environ. Res. Lett. 3 (3), 34003. doi: 10.1088/1748-9326/3/3/034003
Kukal M. S., Irmak S. (2018). U.S. agro-climate in 20th century: Growing degree days, first and last frost, growing season length, and impacts on crop yields. Sci. Rep. 8 (1), 6977. doi: 10.1038/s41598-018-25212-2
Laboski C., Peters J. (2012). Nutrient application guidelines for field, vegetable, and fruit crops in Wisconsin (University of Wisconsin-Extension).
Liang X.-Z., Wu Y., Chambers R. G., Schmoldt D. L., Gao W., Liu C., et al. (2017). Determining climate effects on US total agricultural productivity. Proc. Natl. Acad. Sci. 114 (12), E2285–E2292. doi: 10.1073/pnas.1615922114
MacLaren C., Storkey J., Menegat A., Metcalfe H., Dehnen-Schmutz K. (2020). An ecological future for weed science to sustain crop production and the environment. A review. Agron.Sustainable Dev. 40 (4), 24. doi: 10.1007/s13593-020-00631-6
McMechan J., Hunt T., Wright R. (2020). Cover crop termination timing impact on arthropod abundance, defoliation, and soybean yield (University of Nebraska-Lincoln).
McMechan J., Hunt T., Wright R. (2021). Cover crop termination timing impact on arthropod abundance, defoliation, and soybean yield (University of Nebraska-Lincoln).
Mishra V., Cherkauer K. A., Shukla S. (2010). Assessment of drought due to historic climate variability and projected future climate change in the Midwestern United States. J. Hydrometeorol. 11 (1), 46–68. doi: 10.1175/2009JHM1156.1
Mohler C. L., Teasdale J. R. T. (1993). Response of weed emergence to rate of vicia villosa Roth and secale cereale l. residue. Weed Res. 33 (6), 487–499. doi: 10.1111/j.1365-3180.1993.tb01965.x
Mourtzinis S., Marburger D., Gaska J., Diallo T., Lauer J., Conley S (2017). Corn and soybean yield response to tillage, rotation, and nematicide seed treatment. Crop Sci. 57 (3), 1704–1712. doi: 10.2135/cropsci2016.09.0792
Nakka S., Godar A. S., Wani P. S., Thompson C. R., Peterson D. E., Roelofs J., et al. (2017). Physiological and molecular characterization of hydroxyphenylpyruvate dioxygenase (HPPD)-inhibitor resistance in palmer amaranth (Amaranthus palmeri S.Wats.). Front. Plant Sci. 8. doi: 10.3389/fpls.2017.00555
Nevins C. J., Lacey C., Armstrong S. (2020). The synchrony of cover crop decomposition, enzyme activity, and nitrogen availability in a corn agroecosystem in the Midwest united states. Soil Tillage Res. 197, 104518. doi: 10.1016/j.still.2019.104518
Nichols V., Martinez-Feria R., Weisberger D., Carlson S., Basso B., Basche A (2020). Cover crops and weed suppression in the U.S. Midwest: A meta-analysis and modeling study. Agric. Environ. Lett. 5 (1), e20022. doi: 10.1002/ael2.20022
Norsworthy J. K. (2004). Small-grain cover crop interaction with glyphosate-resistant corn (Zea mays). Weed Technol. 18 (1), 52–59. doi: 10.1614/WT-02-158
Norsworthy J. K., Ward S. M., Shaw D. R., Llewellyn R. S., Nichols R. L., Webster T. M (2012). Reducing the risks of herbicide resistance: Best management practices and recommendations. Weed Sci. 60 (SP1), 31–62. doi: 10.1614/WS-D-11-00155.1
Oliveira M. C., Butts L., Werle R. (2019). Assessment of cover crop management strategies in Nebraska, US. Agriculture 9 (6), 1–14. doi: 10.3390/agriculture9060124
Oliveira M. C., Feist D., Eskelsen S., Scott J. E., Knezevic S. Z. (2017). Weed control in soybean with preemergence- and postemergence-applied herbicides. Crop Forage Turfgrass Manage 3 (1), cftm2016.05.0040. doi: 10.2134/cftm2016.05.0040
Oliveira M. C., Osipitan O. A., Begcy K., Werle R. (2020). Cover crops, hormones and herbicides: Priming an integrated weed management strategy. Plant Sci. 301, 110550. doi: 10.1016/j.plantsci.2020.110550
Otte B., Mirsky S., Schomberg H., Davis B., Tully K. (2019). Effect of cover crop termination timing on pools and fluxes of inorganic nitrogen in no-till corn. Agron. J. 111 (6), 2832–2842. doi: 10.2134/agronj2018.10.0699
Owen M. J., Walsh M. J., Llewellyn R. S., Powles S. B. (2007). Widespread occurrence of multiple herbicide resistance in Western Australian annual ryegrass (Lolium rigidum) populations. Aust. J. Agric. Res. 58 (7), 711–718. doi: 10.1071/AR06283
Pantoja J. L., Woli K. P., Sawyer J. E., Barker D. W. (2015). Corn nitrogen fertilization requirement and corn–soybean productivity with a rye cover crop. Soil Sci. Soc Am. J. 79 (5), 1482–1495. doi: 10.2136/sssaj2015.02.0084
Pedersen P., Lauer J. G. (2002). Influence of rotation sequence on the optimum corn and soybean plant population. Agron. J. 94 (5), 968–974. doi: 10.2134/agronj2002.9680
Pedersen P., Lauer J. G. (2003). Corn and soybean response to rotation sequence, row spacing, and tillage system. Agron. J. 95 (4), 965–971. doi: 10.2134/agronj2003.9650
Peterson M. A., Collavo A., Ovejero R., Shivrain V., Walsh M. J. (2018). The challenge of herbicide resistance around the world: A current summary. Pest. Manage. Sci. 74 (10), 2246–2259. doi: 10.1002/ps.4821
Pittman K. B., Barney J. N., Flessner M. L. (2020). Cover crop residue components and their effect on summer annual weed suppression in corn and soybean. Weed Sci. 68 (3), 301–310. doi: 10.1017/wsc.2020.16
Poffenbarger H. J., Mirsky S. B., Weil R. R., Maul J. E., Kramer M., Spargo J. T., et al. (2015). Biomass and nitrogen content of hairy vetch–cereal rye cover crop mixtures as influenced by species proportions. Agron. J. 107 (6), 2069–2082. doi: 10.2134/agronj14.0462
Pryor S. C., Scavia D., Downer C., Gaden M., Iverson L., Nordstrom R., et al. (2014). “Climate change impacts in the united states: The third national climate assessment,” in U.S. global change research program. (Washington, DC: US Global Change Research Program).
Reddy K. N., Zablotowicz R. M., Locke M. A., Koger C. H. (2003). Cover crop, tillage, and herbicide effects on weeds, soil properties, microbial populations, and soybean yield. Weed Sci. 51 (6), 987–994. doi: 10.1614/P2002-169
Reed H. K., Karsten H. D., Curran W. S., Tooker J. F., Duiker S. W. (2019). Planting green effects on corn and soybean production. Agron. J. 111 (5), 2314–2325. doi: 10.2134/agronj2018.11.0711
Roesch-McNally G. E., Basche A. D., Arbuckle J. G., Tyndall J. C., Miguez F. E., Bowman T., et al. (2018). The trouble with cover crops: Farmers’ experiences with overcoming barriers to adoption. renewable agric. Food Syst. 33 (4), 322–333. doi: 10.1017/S1742170517000096
Rosa A. T., Creech C. F., Elmore R. W., Rudnick D. R., Lindquist J. L., Fudolig M., et al. (2021). Implications of cover crop planting and termination timing on rainfed maize production in semi-arid cropping systems. Field Crops Res. 271, 108251. doi: 10.1016/j.fcr.2021.108251
Ruffo M. L., Bullock D. G., Bollero G. A. (2004). Soybean yield as affected by biomass and nitrogen uptake of cereal rye in winter cover crop rotations. Agron. J. 96 (3), 1. doi: 10.2134/agronj2004.0800
Sanford G. R., Jackson R. D., Booth E. G., Hedtcke J. L., Picasso V. (2021). Perenniality and diversity drive output stability and resilience in a 26-year cropping systems experiment. Field Crops Res. 263, 108071. doi: 10.1016/j.fcr.2021.108071
Scott D. (2011). The technological fix criticisms and the agricultural biotechnology debate. J. Agric. Environ. Ethics 24 (3), 207–226. doi: 10.1007/s10806-010-9253-7
Shergill L. S., Barlow B. R., Bish M. D., Bradley K. W. (2018). Investigations of 2,4-d and multiple herbicide resistance in a missouri waterhemp (Amaranthus tuberculatus) population. Weed Sci. 66 (3), 386–394. doi: 10.1017/wsc.2017.82
Shyam C., Borgato E. A., Peterson D. E., Dille J. A., Jugulam M. (2021). Predominance of metabolic resistance in a six-way-resistant palmer amaranth (Amaranthus palmeri) population. Front. Plant Sci. 11. doi: 10.3389/fpls.2020.614618
Smith D., Broeske M., Patton J., Shelley K. B., Arriaga F. A., Jensen B., et al. (2019). Cover crops, Cover Crops 101. Madison, WI: University of Wisconsin-Madison Division of Extension.
Snapp S., Surapur S. (2018). Rye cover crop retains nitrogen and doesn’t reduce corn yields. Soil Tillage Res. 180, 107–115. doi: 10.1016/j.still.2018.02.018
Teasdale J. R., Mangum R. W., Radhakrishnan J., Cavigelli M. A. (2004). Weed seedbank dynamics in three organic farming crop rotations. Agron. J. 96 (5), 1429–1435. doi: 10.2134/agronj2004.1429
Teasdale J. R., Mohler C. L. (1993). Light transmittance, soil temperature, and soil moisture under residue of hairy vetch and rye. Agron. J. 85 (3), 673–680. doi: 10.2134/agronj1993.00021962008500030029x
Thornton P. E., Thornton M. M., Mayer B. W., Wei Y., Devarakonda R., Vose R. S., et al. (2016). Daymet: Daily surface weather data on a 1-km grid for north America, version 3 (ORNL DAAC, Oak Ridge, Tennessee, USA), 711509.8892839993 MB. doi: 10.3334/ORNLDAAC/1328
Tonitto C., David M. B., Drinkwater L. E. (2006). Replacing bare fallows with cover crops in fertilizer-intensive cropping systems: A meta-analysis of crop yield and n dynamics. Agric. Ecosyst. Environ. 112 (1), 58–72. doi: 10.1016/j.agee.2005.07.003
USDA (2020) Agricultural statistics 2020. Available at: https://www.nass.usda.gov/Publications/Ag_Statistics/2020/Ag%20Stats%202020_Complete%20Publication.pdf (Accessed 11 February 2021).
Vincent-Caboud L., Vereecke L., Silva E., Peigné J. (2019). Cover crop effectiveness baries in cover crop-based rotational tillage organic soybean systems depending on species and environment. Agronomy 9 (6), 319. doi: 10.3390/agronomy9060319
Werle R., Burr C., Blanco-Canqui H. (2017). Cereal rye cover crop suppresses winter annual weeds. Can. J. Plant Sci. 98 (2), 498–500. doi: 10.1139/cjps-2017-0267
West J. R., Ruark M. D., Shelley K. B. (2020). Sustainable intensification of corn silage cropping systems with winter rye. Agron. Sustain. Dev. 40 (2), 11. doi: 10.1007/s13593-020-00615-6
Yenish J. P., Worsham A. D., York A. C. (1996). Cover crops for herbicide replacement in no-tillage corn (Zea mays). Weed Technol. 10 (4), 815–821. doi: 10.1017/S0890037X00040859
Yerka M. K., Wiersma A. T., Lindenmayer R. B., Westra P., Johnson W. G., de Leon N., et al. (2013). Reduced translocation is associated with tolerance of common lambsquarters (Chenopodium album) to glyphosate. Weed Sci. 61 (3), 353–360. doi: 10.1614/WS-D-12-00153.1
Keywords: cereal rye, corn, soybean, soil management, preemergence herbicide, cover crop, integrated weed management, herbicide resistance management
Citation: Grint KR, Arneson NJ, Arriaga F, DeWerff R, Oliveira M, Smith DH, Stoltenberg DE and Werle R (2022) Cover crops and preemergence herbicides: An integrated approach for weed management in corn-soybean systems in the US Midwest. Front. Agron. 4:888349. doi: 10.3389/fagro.2022.888349
Received: 02 March 2022; Accepted: 27 July 2022;
Published: 16 August 2022.
Edited by:
Leslie A. Weston, Charles Sturt University, AustraliaReviewed by:
Ilias Travlos, Agricultural University of Athens, GreeceVeronica Hoyos, University of Magdalena, Colombia
Alison E. Robertson, Iowa State University, United States
Copyright © 2022 Grint, Arneson, Arriaga, DeWerff, Oliveira, Smith, Stoltenberg and Werle. This is an open-access article distributed under the terms of the Creative Commons Attribution License (CC BY). The use, distribution or reproduction in other forums is permitted, provided the original author(s) and the copyright owner(s) are credited and that the original publication in this journal is cited, in accordance with accepted academic practice. No use, distribution or reproduction is permitted which does not comply with these terms.
*Correspondence: Rodrigo Werle, cndlcmxlQHdpc2MuZWR1