- 1International Maize and Wheat Improvement Center (CIMMYT), Sustainable Agrifood Systems (SAS) Program, Dhaka, Bangladesh
- 2Global Evergreening Alliance, Melbourne, VIC, Australia
- 3International Maize and Wheat Improvement Center (CIMMYT), Sustainable Agrifood Systems (SAS), Ciudad de México, Mexico
- 4The World Bank, Dhaka, Bangladesh
- 5Independent Consultant, Dhaka, Bangladesh
- 6Winrock International, Dhaka, Bangladesh
- 7SNV Netherlands Development Organization, Dhaka, Bangladesh
- 8Soil and Crop Sciences, School of Integrative Plant Sciences, Cornell University, NY, United States
While numerous studies have documented the benefits of conservation agriculture (CA) in South Asia, most focus on favorable environments where farmers have reliable access to energy supporting irrigation and inputs. The performance of CA in South Asia’s under-developed coastal environments is comparatively understudied. In these environments, farmers are increasingly interested in growing a second crop to meet food security and income generation objectives in rotation following the predominant monsoon season rice crop, though labor, energy costs, and investment constraints limit their ability to do so. We hypothesized that rotating rice (Oryza sativa) with maize (Zea mays) using conservation agriculture, or CA (i.e., strip-tilled maize followed by unpuddled transplanted rice), or seasonally alternating tillage (SAT, i.e., strip-tilled maize followed by fully-tilled, puddled rice with residues retained across rotations) would reduce costs and energy use, increase energy-use efficiency, and reduce yield-scaled CO2-eq emissions (YSE) and total global warming potential (GWP), compared to farmers’ own practices (FP) and conventional full-tillage (CT) under the same rotation in Bangladesh’s coastal region. Starting with winter maize followed by summer rice, we evaluated four tillage and crop establishment treatments in farmer-managed experiments in partially irrigated and rainfed environments over three years in 35 farmer’s fields across Bangladesh’s coastal districts. Treatments included FP, CT, complete CA, and SAT under a rice-maize rotation. Across years, the full suite of CA practices and SAT were significantly more energy-efficient and energy-productive than FP or CT. The order of YSE in rice was CA< CT or FP < SAT while in maize, it was CA or SAT < FP < CT. Across environments, CA and SAT resulted in 15-18% higher yield at the cropping systems level (maize and rice yields combined) and 26-40% less manual labor than CT or FP. CA and SAT also reduced by 1-12% and 33-35% total production costs respective to CT and FP. This was associated with 13-17% greater grain energy output in CA and SAT, and 2-18% lower YSE, compared to CT or FP. While our data suggest that both CA and SAT can result in a range of positive agronomic, economic, and environmental outcomes compared to FP or CT, post-trial surveys and discussions with farmers revealed a strong practical aversion to use of the full suite of CA practices and preference for adapted practices due to logistical constraints in negotiating the hire of laborers for unpuddled manual transplanting.
Highlights
1. We facilitated farmer-managed rice-maize trials of conservation agriculture (CA) and alternative tillage and crop management techniques.
2. CA or seasonally alternating tillage (SAT) increased energy productivity and use efficiency, reducing yield-scaled greenhouse gas emissions (YSE).
3. Rice YSE was lowest in CA and highest in SAT. Maize YSE was lowest in CA and SAT and highest in full-till.
4. CA or SAT had grain energy output 13-17% greater and YSE 2-18% lower compared to full-till or farmers’ practices.
5. While both CA and SAT can increase systems yield and energy productivity and reduce YSE, farmers prefer SAT
Introduction
Efficient use of resources and mitigation of greenhouse gas (GHG) emissions are key milestones towards the goal of sustainability in intensive cropping systems. Achieving these outcomes may however clash with the increased use of agrochemicals, water, and energy associated with high-yielding double cropping systems. Intensive rice (Oryza sativa)-based cropping systems such as the rice-wheat (Triticum aestivum) or rice-maize (Zea mays) rotational crop sequences that are common in South Asia are crucial for food and income security in this densely populated region (Ali et al., 2009; Timsina et al., 2010; 2018). In these systems, while over-use of inputs can lower production efficiency, under-use can also compromise farmers’ food production and economic objectives (FAO, 2011a; FAO, 2011a; FAO, 2011b). Of the resources used in crop production in South Asia, non-renewable energy sources such as diesel are widely used in pumping from aquifers or canals. Diesel is also used for land preparation, with tillage usually requiring high amounts of energy (Pimentel, 2009; Woods et al., 2010). Agriculture accounts for approximately 20% of all energy use in South Asia (Rasul, 2014), and while energy inputs can aid in increasing yield, their inefficient use can also be associated with GHG emissions (Woods et al., 2010). This can compromise the dual objectives of economic development and environmental stewardship in agriculture (Pathak et al., 2011; Alam et al., 2016; Alam et al., 2019b). More appropriate crop production practices are therefore needed to address trade-offs with actions that support improved environmental quality.
Alternative tillage and crop establishment practices, which require no or reduced tillage operations for land preparation, are likely to result in lower energy use and reduced GHG emissions (Gathala et al., 2013; Laik et al., 2014; Alam et al., 2015; Gathala et al., 2015; Gathala et al., 2016; Hossen et al., 2018; Islam et al., 2019; Gathala et al., 2020). One such practice is conservation agriculture (CA), which is based on the principles of reduced or zero-tillage, full or partial residue retention, and profitable crop rotations (Hobbs et al., 2008; Derpsch et al., 2014; FAO, 2018). A range of studies in South Asia have reported that CA can accrue improved production efficiencies and result in environmental gains (Hobbs et al., 2008; Jat et al., 2014; Dixon et al., 2020; Gathala et al., 2020). Studies have also demonstrated that reductions of GHG emissions from CA compared to conventional tillage (CT) (Govaerts et al., 2009; Aryal et al., 2016; Alam et al., 2019a; Alam et al., 2019b). Haque et al. (2016); Bell et al. (2019), and Islam et al. (2013) reported that compared to conventional repetitive tillage, fuel consumption could be reduced 2-3 fold where farmers use strip tillage as a CA practice in northwestern Bangladesh. Haque et al. (2016); Bell et al. (2019), and Hossen et al. (2018) also suggested that unpuddled transplanted rice, in which fields are not wet tilled prior to crop establishment, can also decrease time and fuel consumption by 50-70%, while also boosting energy productivity (EP) by 8-12%, relative to CT. Gathala et al. (2016; 2020) demonstrated that energy use was significantly lower and energy-use efficiency (EUE) higher for maize planted with strip tillage compared to CT in a range of rice-based cropping sequences across India, Nepal, and in northwestern Bangladesh. In addition, Alam et al. (2015) and Laik et al. (2014) reported that intensive tillage practices in central Bangladesh and northeastern India, respectively, used greater amounts of total energy compared to production under unpuddled rice transplanting in which fields were not wet tilled.
As a result of these and numerous other studies, CA is increasingly popularized as a strategy for efficient energy use in agriculture, as well as a means to adapt to and mitigate climate change (Harvey et al., Harvey et al., 2013; Pretty and Bharucha, 2014). Yet while CA has performed well in terms of increasing or maintaining yields, increasing profits, and reducing systems-level energy use and GHG emissions (Gathala et al., 2016; Gathala et al., 2020), farmers’ adoption of the full suite of CA practices has tended to be very low (Pannell et al., 2014; Ward et al., 2017). This comes despite considerable investment in agricultural development projects that have worked to popularize CA (Jat et al., 2013; Pannell et al., 2014). In South Asia, while there is some adoption of zero tillage and residue retention that has been enabled by specialized planting machinery, the adoption of the full suite of CA practices is rare, and farmers routinely till at least one crop – most commonly prior to rice establishment – during rotations (Keil et al., 2017; Akter et al., 2021).
Conservation agriculture and zero-tillage practices have also been most widely adopted in relatively favorable and irrigated environments in South Asia’s western Indo-Gangetic Plains (Keil et al., 2017). In these locations, farmers tend to be somewhat better-off from an economic standpoint and tend to have relatively good access to irrigation and other inputs (Erenstein and Thorpe, 2011). Conversely, comparatively little work in South Asia has addressed CA under rainfed production practices, or in environments where freshwater resources are scarce, and where farmers may have significant constraints to their economic resources, as is the case in the region’s coastal areas (Krupnik et al., 2017). In Bangladesh, both government and international donors have increased focus on agricultural development in these environmentally-risk prone coastal regions, with emphasis on profitable crop diversification (Aravindakshan et al., 2021). Although rice-rice or rice-fallow sequences are common (Krupnik et al., 2017), a range of organizations have placed emphasis on the cultivation of alternative crops such as maize (Katalyst and Swiss Contact, 2017). Primarily grown in sequence after monsoon season rice, the winter season maize is cultivated to produce feed for a rapidly expanding poultry industry as an income-generating cash crop among smallholder farmers (Rahman, 2012; Katalyst and Swiss Contact, 2017). Systematic, multi-locational and multi-year efforts to study CA and adaptations of CA practices are however scarce in these environments.
To date, there has been no integrated evaluation of the agronomic, economic, energetic, and GHG mitigation potential of CA in the context of rice-maize rotations in coastal environments compared to systems in which farmers may apply tillage seasonally to the rice crop. Such studies are crucial, given that most of the area under which zero-tillage has been adopted in South Asia includes tillage prior to the monsoon season rice crop, while the subsequent crop is established without tillage (Aryal et al., 2014; Keil et al., 2020). As such, farmers engaged in adapting CA to rice-maize systems in coastal environments may also prefer to make use of similar practices given the long history of wet tillage applied to rice in Asia (Timsina and Connor, 2001). Moreover, there is a lack of knowledge on the performance of CA under rainfed conditions and/or with limited application of irrigation, both of which are likely to be logical adaptations to these practices given the slow pace of reliable irrigation development in Bangladesh’s coastal zones (Krupnik et al., 2017).
In response to these knowledge gaps, we test the hypothesis that seasonally alternating tillage (SAT) practices that alternate between strip-tillage in the winter season for maize and CT prior to rice can reduce energy use, increase energy productivity, and reduce yield-scaled emissions while increasing or maintaining yield and profit, even under these challenging conditions. We consequently compared the full suite of CA to SAT practices against CT and farmers’ own practices in 35 fields in experiments managed by farmers across partially irrigated and rainfed environments in southern coastal Bangladesh over a period of three consecutive years. Multiple indicators were examined to quantify the agronomic, environmental, and economic impacts of these systems in these relatively under-studied environments, with the implications of SAT considered in the context of regional agricultural development efforts.
Materials and Methods
Site Description
Researcher-designed but farmer-managed field trials were conducted in rainfed and partially irrigated environments for three years from the 2011-2012 winter ‘rabi’ crop season to the 2014 monsoon ‘aman’ season across Bangladesh’s central and western coastal areas. In the latter, constraints to the availability of quality freshwater meant that farmers could apply at most two irrigations in the beginning of the season before surface water supplies became unavailable due to a lack of surface water recharge in canals, or before irrigation with shallow groundwater extraction became unviable due to seasonally increasing salinity and/or farmers’ inability to afford additional irrigation. The rainfed sites included farms in Bhatia Ghata (89°31’38.617”E 22°40’51.422”N), Babuganj (90°19’52.828”E 22°47’37.573”N), and Kalapara (90°10’40.552”E 21°56’18.812”N) upazilas (sub-districts), while the partially irrigated sites included locations in Kaliganj (89°1’26.064”E 22°28’29.126”N), Babuganj, and Kalapara upazilas (Figure 1). In Babuganj, while rotations began with rabi maize in the winter 2011-12 season, prolonged tidal flooding during the late grain-filling stage resulted in rot and near total crop losses. As such, rainfed maize data from the first year were not included from this location, although rice data are presented for the purposes of examining tillage and varietal performance. Year 1 analyses at the rice-maize cropping systems level in rainfed locations however do not include this location due to the reasons described above.
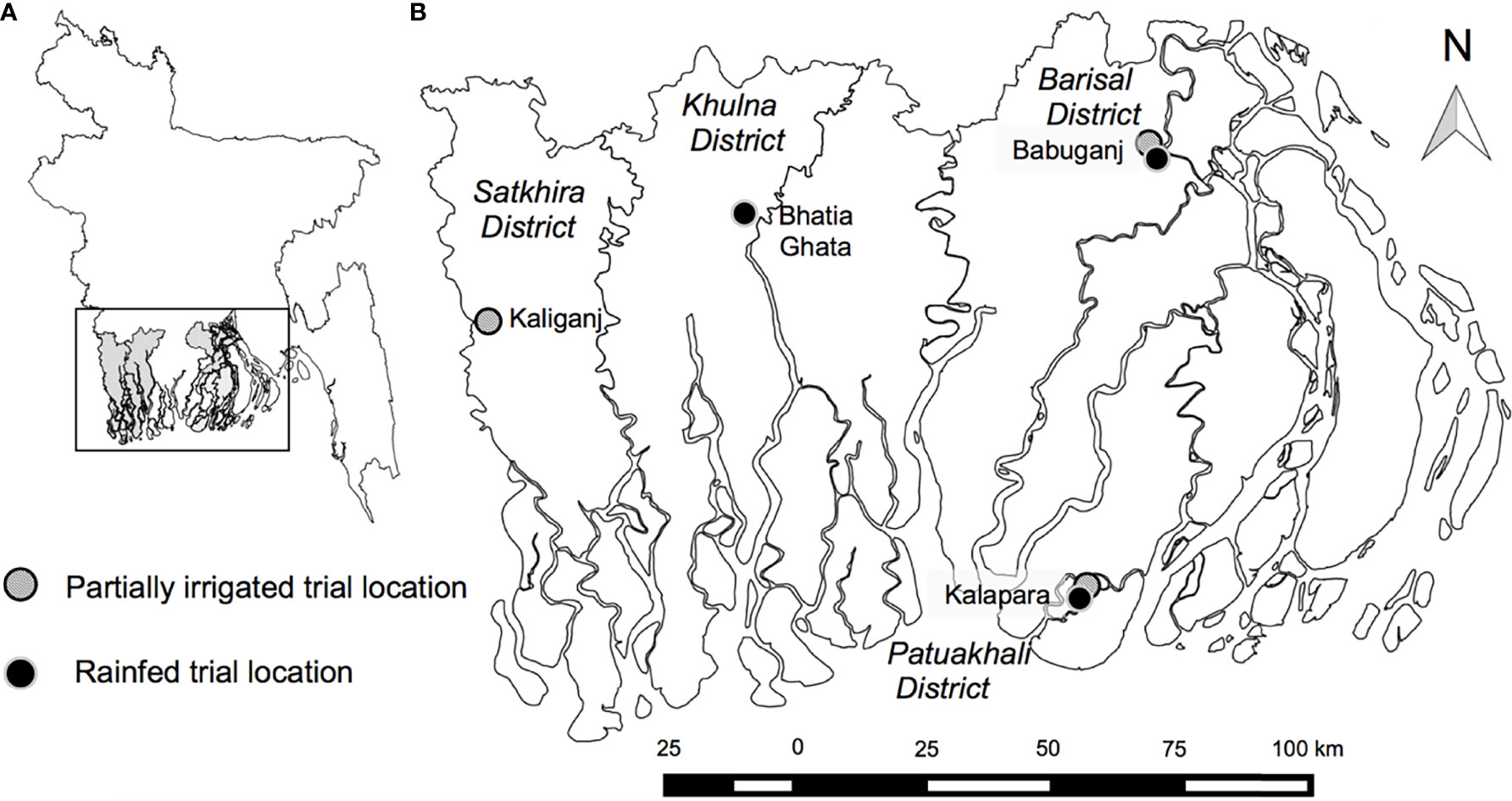
Figure 1 (A) Four districts in southern coastal Bangladesh with (B) detail of trial locations in each district.
Weather data were collected from automatic weather stations and the Bangladesh Meteorological Department within 30 km of experimental sites. The mean monthly daily minimum temperature during the maize-growing seasons ranged from 6.5 to 22.4°C, while maximum temperature ranged from 27.2 to 40.7°C. Temperature across years and environments followed similar trends, with highest maximum and minimum temperatures in March-June and lowest in December-January. Precipitation during winter was unevenly distributed, ranging from 1 to 325 mm across three years. Rainfall during the monsoon season was also variable, with cumulative rainfall ranging from 514 to 1,587 mm across three years (data not shown). During the rice phase of their rotation, the days in which plots had standing floodwater or lacked floodwater were noted. Soil qualities are shown in Table 1.
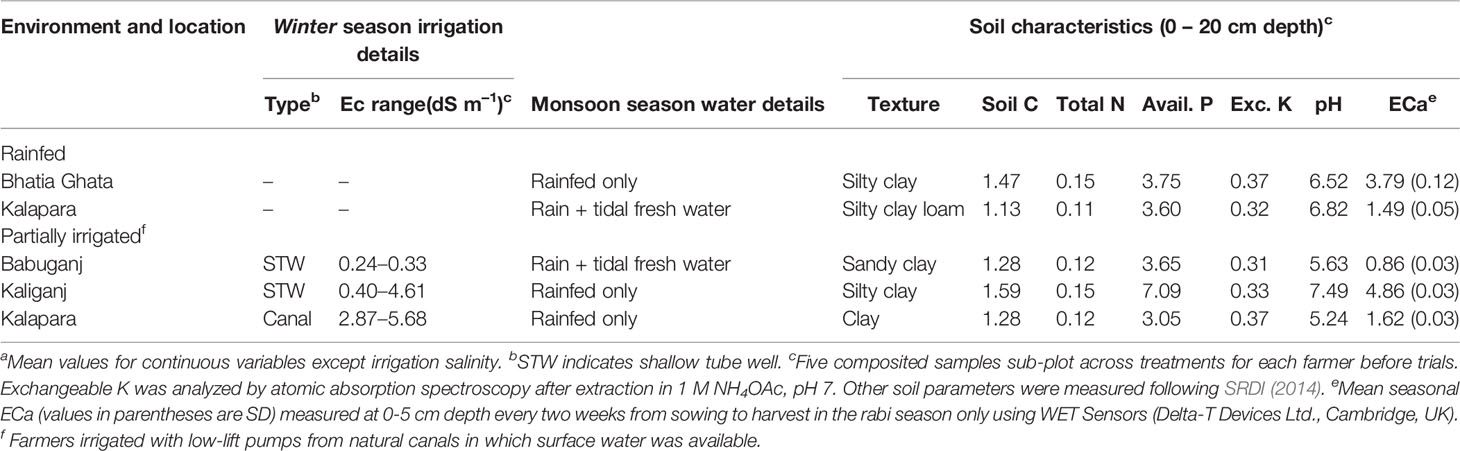
Table 1 Description of the environments and soils [soil C (%), total N (%), available P (mg kg–1), exchangeable K (meq 100 g–1), pH and ECa (dS m–1)] for each study location in coastal Bangladesha.
Participant Farmer Selection and Treatment Description
Prior to experiments, researchers engaged with farmers in community meetings to introduce the research questions associated with CA and to engage with farmers in experimental design. During these meetings many farmers expressed an aversion to implementation of the full suite of CA principles, instead indicating their preference to till their fields prior to the rice phase of the rotation. As a result of these interactions, four tillage and crop establishment (TCE) treatments identified by researchers and farmers that were applied to main plots in all locations: (1) CA in both crops (CA), (2) seasonally alternating tillage (SAT) in which maize was grown without tillage and with rice residues from the previous season retained as mulch, but rice was fully tilled and wet puddled with maize residues retained, (3) conventional tillage (CT) in which soils were fully tilled prior to crop establishment in both crops with all residues exported, and (4) farmers’ practices (FP) in which each farmer was requested to grow each crop using their own management practices and input rates as they would typically manage these crops in the absence of researcher or experimental intervention. Supplementary Table 1 provides additional treatment details, including information on how tillage and residue management was implemented.
Farmers were chosen to participate who (a) had land tenure to maintain trials over multiple years, (b) who had attended Department of Agricultural Extension led trainings on CA and maize crop management, (c) were able and willing to use their own labor and/or hire their own labor to manage treatments, thereby simulating real farm conditions as much as possible. Finally, (d) in case of partially irrigated locations, farmers were selected who were able to supply at least one irrigation. Fifteen farmers in rainfed environments (five in Kalapara and 10 in Bhatia Ghata) and 20 farmers in partially irrigated environments (five each in Babuganj and Kalapara and 10 in Kaliganj) were subsequently selected.
Experiments in all locations were laid out in a split-plot design during the winter season in 2011-2012 with maize hybrid NK40 planted to 28.1 m2 sub-plots and a 50 cm alley provided between each sub-plot and 30 cm wide bunds surrounding main plots. During the 2012 monsoon season, sub-sub-plots were established with two high-yielding and stress-tolerant rice genotypes (salinity-tolerant BRRI Dhan 41, or BRRI-41, and submergence-tolerant BRRI Dhan 52, or BRRI-52; Ismail et al., 2013) in a split-split plot design. NK40 was planted subsequently to all sub-plots in the ensuing winter season. Farmers were considered as dispersed replicates.
Crop Management
The planting date of winter maize across years and trials ranged from 10 December to 12 February, with maize in CA and SAT sown into residue of the preceding non-experimental rice crop in 2011, while rice seedbed establishment took place from 20 June to 30 July, with transplanting from 22 July to 6 September. In all locations and across treatments, farmers were encouraged to establish their plots as early as possible. During the winter rabi season, variability in the sowing dates of maize was a result of the different times at which farmers were able to traffic their fields with two-wheeled tractors following rice harvest and the recession of monsoon season floodwater and subsequent soil drying within experimental plots. Sowing dates varied between years, although they tended to be latest in Babuganj and Kalapara under partially irrigated conditions, and in Babuganj which also had rainfed trial locations. Plot drainage challenges and heavy soils in these sites contributed to this delay.
Maize was directly drilled using a power tiller operated seeder (PTOS) for strip tillage by skilled machinery service providers. The PTOS is a 1200 mm wide single-pass shallow tillage implement with a seed and fertilizer drill. It is compatible with two-wheeled tractors made by Dongfeng company, Wuhan, China. Fluted rollers were used for seed and fertilizer metering. The PTOS can be modified for strip tillage by removing selected rotary blades (Krupnik et al., 2013). Strip tilled furrows are usually < 5 cm width, and therefore disturb < 10% of the soil surface and conform to CA recommendations (cf. Derpsch et al., 2014). Seeds were sown at 6-7 cm depth by the same operator in each site. 35-40 cm standing rice residue height was retained on the soil surface. Because the CA and SAT treatments eliminated repetitive tillage for maize, farmers were able to establish these treatments 2-9 and 3-9 (average of 4 and 6) days earlier, respectively, compared to the CT and FP treatments.
Fertilizers to both crops were applied at recommended rates provided by government agricultural institutes. In all treatments, fertilizer rates for rabi maize were held constant, though they differed for partially irrigated and rainfed locations. In rainfed environments, N, P and K were applied at 150, 25 and 85 kg ha-1, respectively, while in partially irrigated environments, 200, 35 and 130 kg N, P and K ha-1 were applied. In rainfed environments, half of N was applied basally and the remaining when eight to ten fully developed leaf collars were visible (V8-V10 stage), coinciding with precipitation. In partially irrigated environments, 30% of N was applied basally, with the remaining applied equally at V6 (when six leaf collars visible) and V10, with a light irrigation (~5 cm depth) to incorporate fertilizer into the soil. All P fertilizer was applied basally. In rainfed environments, all K was applied basally while in partially irrigated environments, 50% was applied basally and 50% at V8-V10 stage. In CA and SAT maize, all basal fertilizers were drilled using a PTOS, with splits broadcast. Rice in all locations was rainfed. In Babuganj and Kalapara, fields also experienced freshwater tidal inflow and outflow movement in the monsoon season. Nitrogen, P, K and S to rice was applied at 90, 24, 41 and 60 kg ha-1, respectively, with same rates in all treatments. One-third N to rice was applied basally, with the remaining two-thirds applied equally by broadcasting at 20-25 days after transplanting and at panicle initiation, at times farmers deemed appropriate to minimize losses due to water movement. All P, K and S were applied basally in all locations. Rates were the same across locations, exempting Babuganj and Kalapara, where Zn (5 kg ha-1) was also applied basally to overcome known soil Zn deficiency in these sites. For both crops, N, P, K, S, and Zn were applied through urea, TSP, MOP, gypsum, and ZnSO4 heptahydrate, respectively.
Water is a scarce resource during the winter season in coastal areas. In both environments, maize was therefore established with residual soil moisture following rice. In the partially irrigated locations, a light irrigation (approx. 50 mm) was applied after urea applications at V6 and V10. Rainfed trials did not receive any irrigation. During the monsoon, rice was entirely rainfed and/or received water from tidally mediated land inundation.
Input Use, Yield, and Profitability
Input costs and labor use data (e.g., tillage, transplanting, irrigation, fertilizer and pesticide applications, hand weeding, harvesting, and threshing) were collected from farmers through surveys 3-4 times season–1 and after harvest per each treatment. Prices for inputs and outputs for each season were monitored from local markets. Fuel use for land preparation and seeding, as well as irrigation, were measured as described by Gathala et al. (2016).
Maize was harvested from 10.08 m2 in the center of each plot to determine grain yield (15.5% moisture content) after air drying to a constant weight. Stover yields were obtained by drying 20 plants the same way, with ~350 g fresh sub-samples used to determine moisture content gravimetrically after oven drying (70° C for 72 h). Rice grain yield (14% moisture content) was measured from 10 m2 after the same drying process. Straw yield was recorded similarly from a 1.8 m2 surface in each harvest plot. In CA and SAT, residues retained as mulch or incorporated were measured separately from those exported. Cropping systems level yields were accounted for as the sum of rice and maize yield (kg ha–1) grown in rotation within a single year.
Economic and Energy Analysis
An inventory of all inputs (fertilizers, crop seeds, irrigation water, herbicides and insecticides, diesel, and human labor) and outputs (grain and straw/stover) from maize, rice and rice-maize cropping systems was prepared, from which the energy inputs to each TCE treatment was calculated. Following these measurements, farmers’ profits from each treatment was calculated by dividing all variable costs from gross returns from grain and exported stover or straw. Crop inputs and outputs were also converted to energy equivalents using published conversion coefficients (Table 2). Direct measurements of diesel use were taken in each treatment for tillage and irrigation operations following Gathala et al. (2016). We assumed the same energy conversion for human labor for both men and women. Total energy use through all energy sources (EU; Mj ha-1) was calculated as:
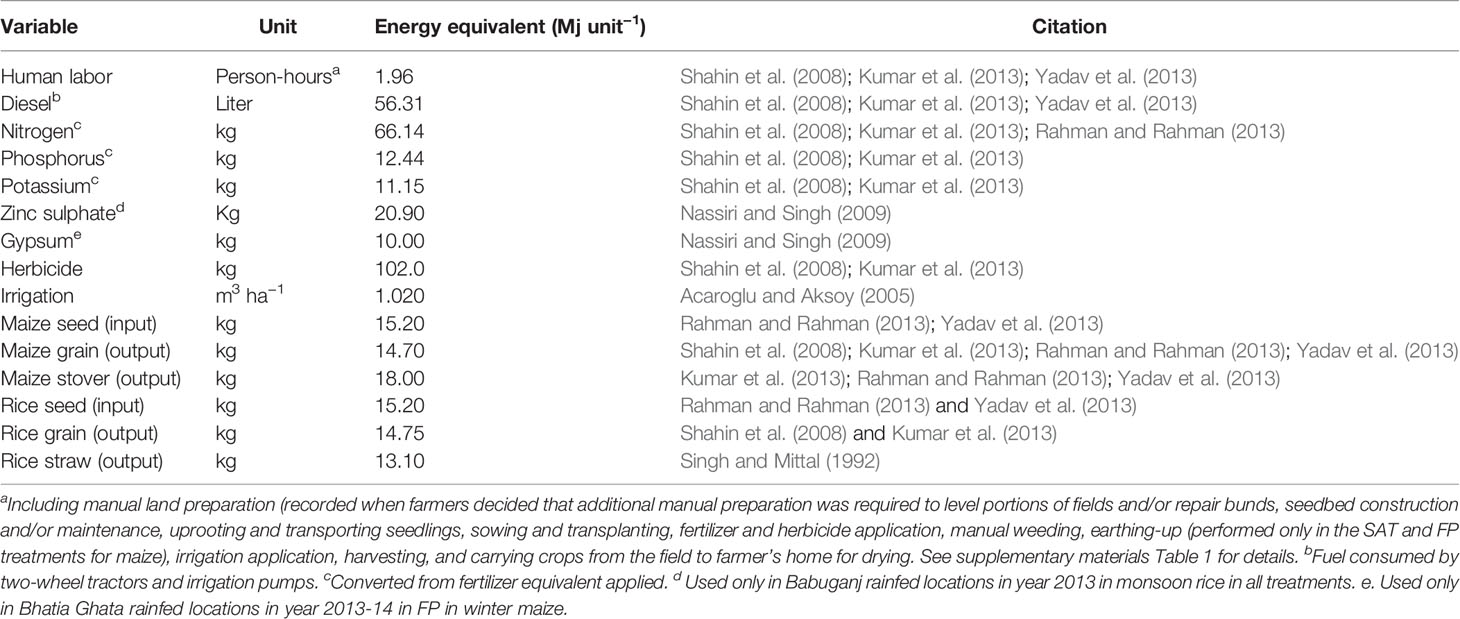
Table 2 Production system energy conversion units used to calculate the inputs and outputs for maize, rice and rice-maize systems treatments in southern coastal Bangladesh.
where El is manual labor (in person-hours) converted to energy use, Ed is the energy used for diesel, and Ei is the energy derived from all other inputs or outputs. The total energy produced in grain and straw/stover yields (Kg ha-1), or total output energy (EO, Mj ha-1), was subsequently calculated as:
where the energy coefficient is the specific conversion factor for grain or straw yield (Mj kg-1). Energy-use efficiency (EUE) is a dimensionless term; it considers returns on investment in energy inputs with the objective of maximizing energy returns (Woods et al., 2010). It was calculated following Equation 3:
Finally, energy productivity (EP; Kg ha-1 grain yield/Mj ha-1 energy input) measures the level of economic crop production relative to energy used and was computed according to Equation 4.
Global Warming Potential and Yield-Scaled GHG Emissions
We applied the CCAFS’ Mitigation Options Tool (CCAFS-MOT) (Feliciano et al., 2017) which includes set of empirical models to estimate GHG emissions associated with crop production system until the farm-gate level. This tool uses plot-level information on input and crop management from the trails and corresponding soil and climatic information to estimate GHG emissions. We used a version of the CCAFS-MOT scripted in R software (R Core Team, 2020). Emissions from rice included N2O, CO2 and CH4. These were estimated from Yan et al. (2005), which calculates CH4 emissions from under different floodwater and irrigation conditions as a function of soil pH, climate, and the use of organic amendments or residue. N2O emissions from fertilizer were based on Stehfest and Bouwman (2006). The Ecoinvent Center (2007) database was used to quantify emission fertilizer production and transport. Modeled soil C from residue management were based on Ogle et al. (2005) and Smith et al. (1997). Soil C responses from tillage management were based on Powlson et al. (2016). CO2 emissions from nutrient and irrigation were estimated from the IPCC (2006). For maize, the model produced estimates of N2O and CO2 only, as there was no prolonged flooding of maize plots observed, and no manures were applied nor were crop residues burned. All GHGs were converted into CO2-equivalent (CO2eq) using 100-year global warming potentials (GWPs) of 34 and 298 for CH4 and N2O, respectively (IPCC, 2013). Yield-scaled emissions (YSE) for each treatment was determined as in Equation 5:
Farmer Surveys
At the conclusion of experiments, each participating farmer was surveyed and asked to rank their preferences among treatments and interest in adopting complete CA, SAT, or CT relative to their own practices. This was followed by open discussion regarding the reasons for farmers’ preferences.
Data Analysis
Following confirmation of the normality assumptions necessary for ANOVA, data were analyzed separately for each year for partially irrigated and rainfed environments employing a split-split plot design. Location, tillage, and rice genotype plots were considered the main, sub-, and sub-sub sources of variation. Farmer replicates were considered as a random effect. The fundamentals of split-split plot design and its use in on-farm experimentation with sources of variation as observed in these experiments are widely cited and have been provided by Gomez and Gomez (1984). Analyses were performed using the restricted maximum likelihood (REML) option in JMP 14 (SAS Institute Inc., San Francisco). Multi-indicator performance was examined conducted using radar diagrams to visually examine the relative trade-offs among productivity, profitability, energetics, and GWP and YSE parameters for the four TCE treatments under both environments and across years, although due to failure of the maize crop in Babuganj under rainfed conditions, radar diagrams do not consider the first year rainfed rice-maize rotation in this location. Use of radar diagrams considering trade-offs among various indicators in analysis of CA and alternative management practices are common in the literature (Gathala et al., 2015; Gathala et al., 2016; Magar et al., 2022a; Magar et al., 2022b). Farmer survey and subsequent discussion information were analyzed descriptively and qualitatively.
Results and Discussion
Crop and Cropping Systems Energy Analysis
Variability in external and recycled energetic inputs to maize, the latter largely in residue recycling in the CA and SAT treatments, was observed (Tables 3, 4). In both rainfed and partially irrigated environments, differences in energy inputs to maize were primarily due to differences in human labor and tillage number (under CT and FP and across years, the ranges of tillage events for maize in partially irrigated and rainfed environments, were 2-3 and 3, respectively). Significant differences (P<0.001) were observed in maize in both environments, with CA and SAT utilizing 1-5% and 1-4% fewer energy inputs than CT across years. While significant, these small differences were due to the relatively limited number of tillage passes in CT, and the energy embodied in herbicides (on average 803 Mj ha–1) used in strip tilled maize.
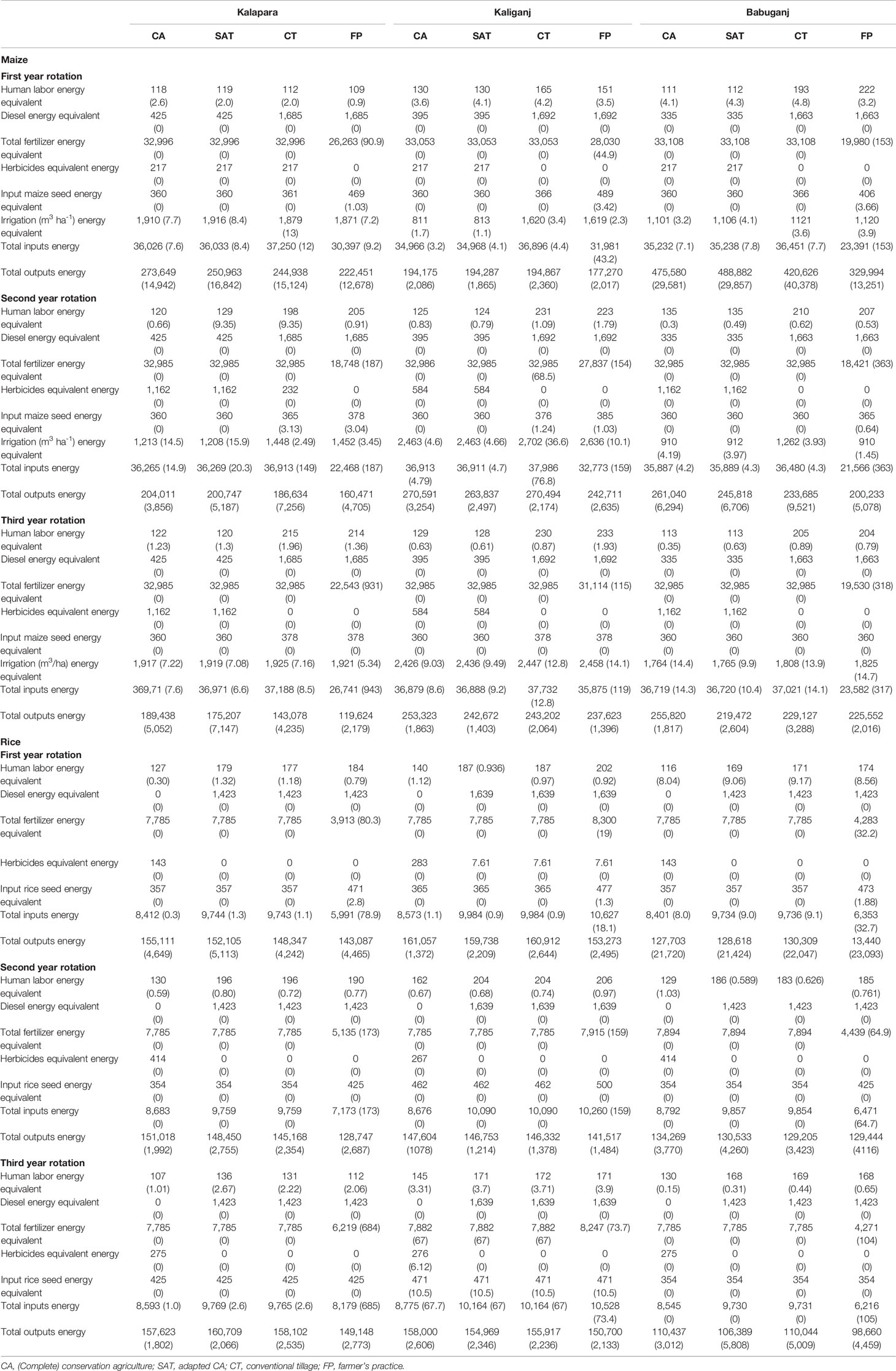
Table 3 Details for mean energy inputs and outputs (Mj ha-1) for dry winter season maize and monsoon season rice over three years of rotation in partially-irrigated environments in coastal Bangladesh (numbers in parentheses are the standard error of the mean).
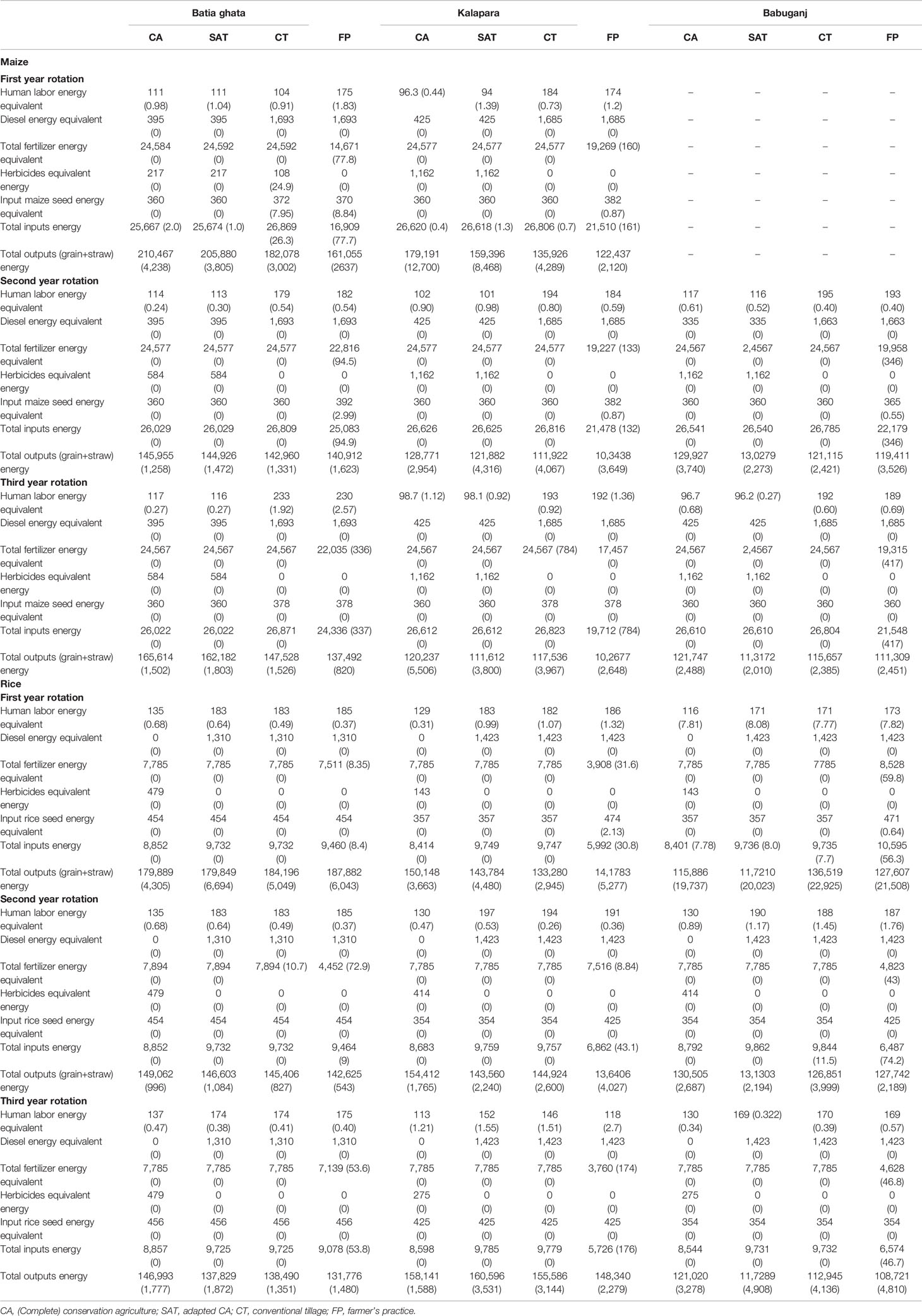
Table 4 Details for mean nergy inputs and outputs (Mj ha-1) for dry winter season maize and monsoon season rice over three years of rotation in rainfed environments in coastal Bangladesh (numbers in parentheses are the standard error of the mean).
In rice, CA required significantly (P<0.001) lower energy than SAT and CT (10-12% less energy for both treatments) in rainfed environments across years, but FP entailed the least energy inputs (7,978 Mj ha–1 on average) followed by CA (8,606 Mj ha–1 on average) in partially irrigated environments across years. Variability in energy input was small (Tables 2, 3), a consequence of a trade-off in herbicide use in CA relative to lower fuel consumption, and lower fertilizer use (25% less than the other treatments) in FP relative to greater fuel consumption. Differences observed resulted mainly from reduced tillage in SAT and FP (2-3 and 3-4 tillage events across years, respectively) relative to CT (2-4 tillage events across years). These results are broadly consistent with research in northwestern Bangladesh including Islam et al. (2013); Hossen et al. (2018), and Gathala et al. (2020) which reported that unpuddled transplanted rice reduced the time and fuel required for tillage by two- to three-fold and by 50-70%, respectively, compared to CT. Alam et al. (2015); Gathala et al. (2016); Gathala et al. (2020), and Laik et al. (2014) also reported that full tillage in Bihar in northeastern India and in central and northwestern Bangladesh used more energy than unpuddled transplanting. Conversely, interest in rice transplanters in Bangladesh is growing, a consequence of governmental subsidies offsetting 50-70% of their cost (Rahman et al., 2021). While efforts are underway to assess the use of mechanical transplanters under unpuddled conditions cf. Ashik-E-Rabbani et al, (2018); Basir et al, (2019), most research has considered rice in isolation rather than part of an integrated crop rotation, therefore representing an important research gap.
Our analysis of EUE and EP highlighted significant effects of location, tillage and crop establishment, and location × tillage and crop establishment interactions, but no differences were observed for the other two-way and three-way interactions, regardless of environment (Tables 3, 4). In rainfed environments, crop and systems-level EUE was highest (P<0.001) in FP followed by CA, while EP was similar in CA and FP. Observed differences resulted from comparatively lower fertilizer-based energetic input rates (25% less than the other treatments) in FP (Table 2). At the cropping systems level, and across locations and rice varieties, CA was on average 6% and 11% more energy-efficient than SAT and CT (both P<0.001) and 5% and 13% more energy-productive than these treatments, respectively (both P<0.001). In partially irrigated environments, across three years, systems-level EUE ranged 8.9-11.1 and 7.3-9.3, while systems-level EP ranged 0.25-0.30 and 0.21-0.23 under FP and CT, respectively (Table 5). In rainfed environments, though systems-level energy parameters were comparable, the lack of energy consumed for irrigation rendered them lower (Table 6). Across environments, use of the submergent tolerant BRRI-52 resulted in significantly higher (P<0.001) systems-level EUE (8.0-18.2) and EP (0.25-0.35) compared to BRRI-41 (EUE, 7.8-10.0; EP, 0.23-0.30). These differences likely resulted from this cultivar’s ability to withstand prolonged inundation (Kamruzzaman and Shaw, 2018), particularly in Babuganj and Kalapara where monsoon season tidal water movement and inundation was observed, whereas a marginal loss (6% on average across treatments) of rice hills was observed following transplanting during extended flooding in the first and third year of rotation, respectively, when BRRI-41 was cultivated (data not shown). Our observations support Hossen et al. (2018) who reported increases in EP by 8-12% and energy output-input ratios by 22-24% for unpuddled rice transplanting on raised beds or with strip tillage in Bangladesh.
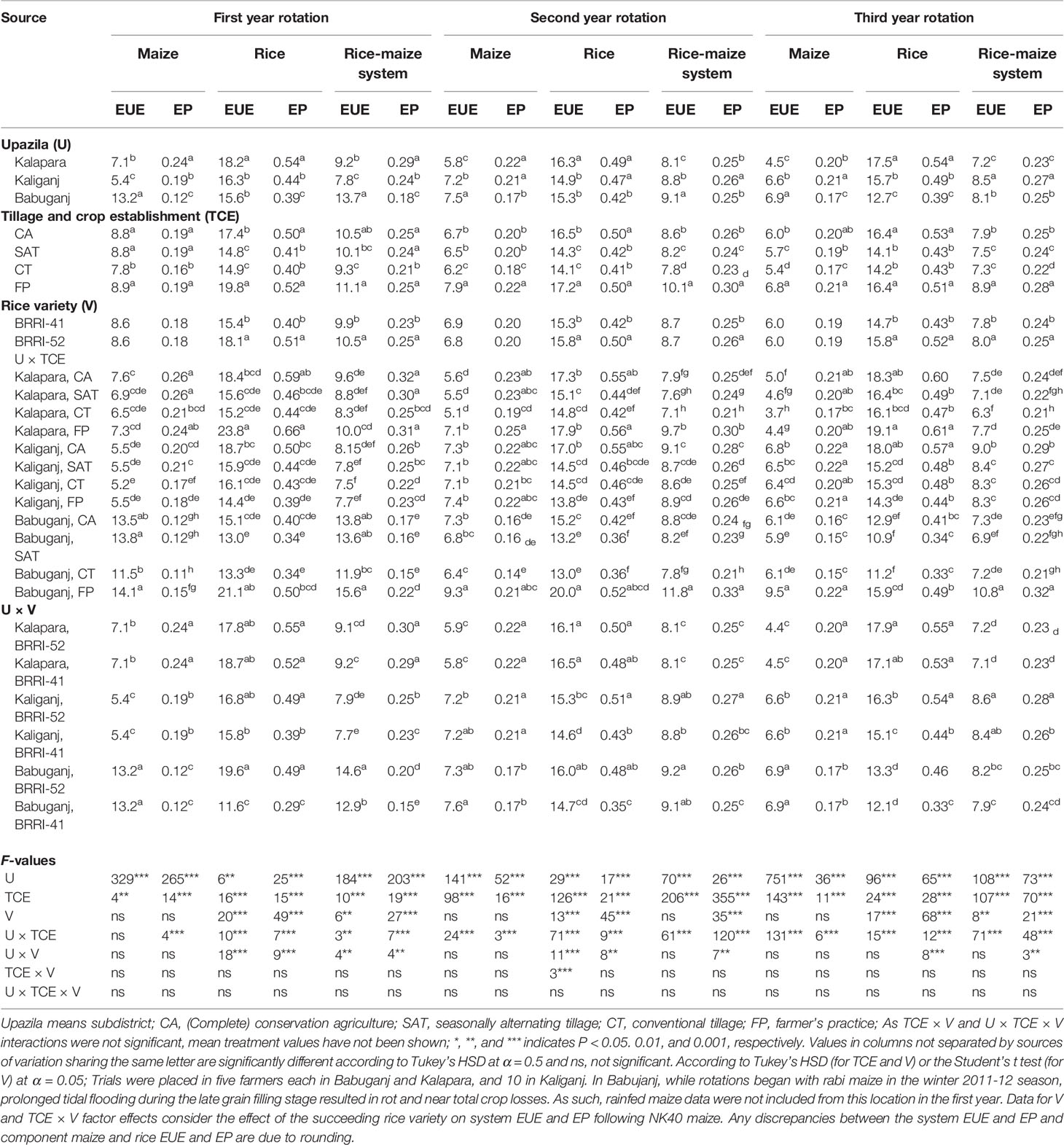
Table 5 Energy-use efficiency (EUE; Mj ha-1 output/Mj ha-1 input) and energy productivity (EP; Kg ha-1 grain yield/Mj ha-1 input) for dry winter season maize, monsoon season rice, and systems (maize + rice) over three years of rotation in partially irrigated environments in southern coastal Bangladesh.
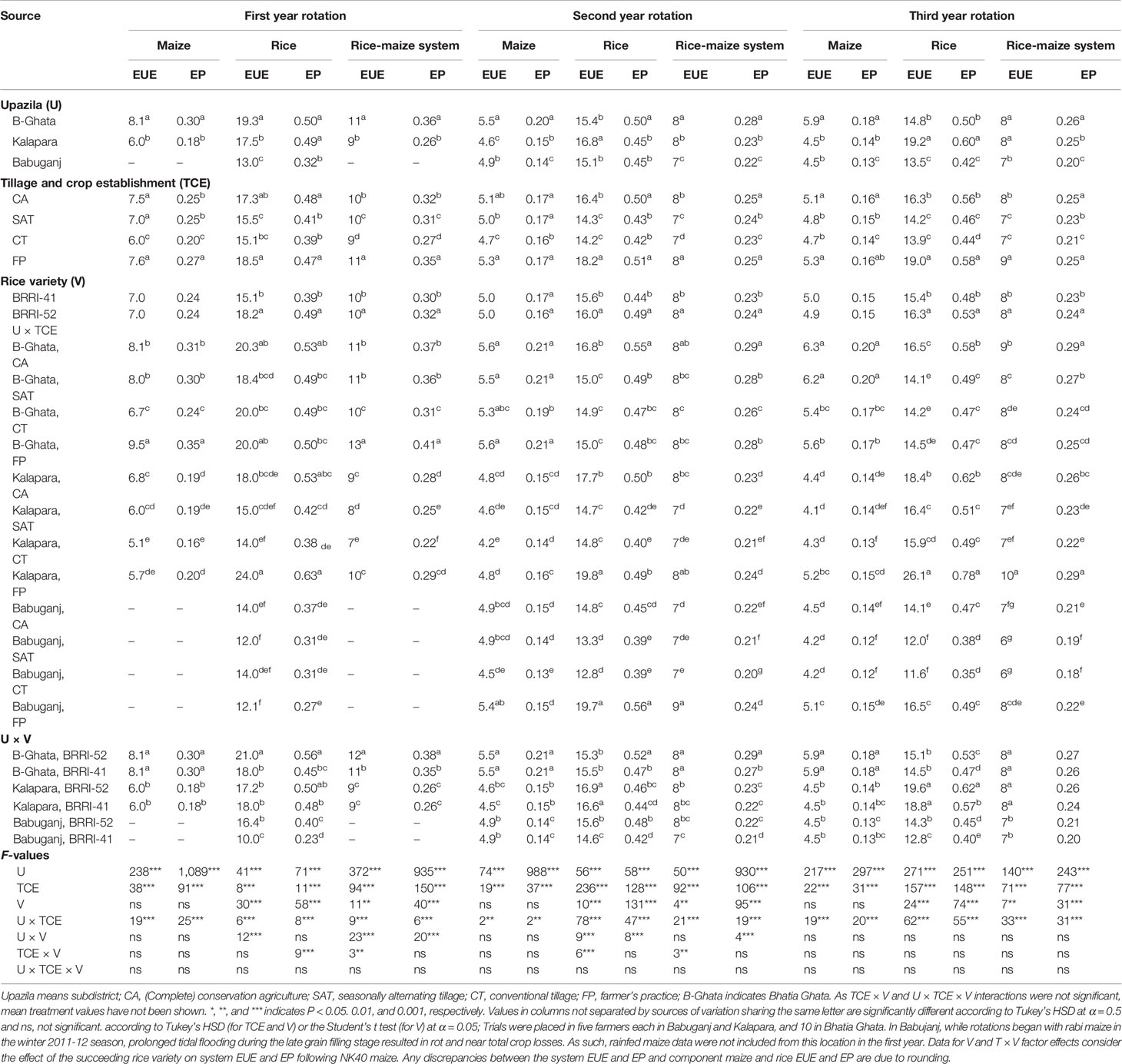
Table 6 Energy use efficiency (EUE; Mj ha-1 output/Mj ha-1 input) and energy productivity (EP; Kg ha-1 grain yield/Mj ha-1 input) for dry winter season maize, monsoon season rice, and systems (maize + rice) over three years of rotation in rainfed environments in southern coastal Bangladesh.
Global Warming Potential
Though the soil carbon sequestration potential of CA has been debated (Powlson et al., 2016), reduced tillage can lower GHG emissions through reductions in fuel use (Alam et al., 2015; Govaerts et al., 2009; Alam et al., 2019a). We observed significant (P<0.001) locational differences in total GWP within partially irrigated and rainfed environments for both crops and cropping systems across years (Tables 7, 8). Comparing tillage treatments separately in those environments, but across locations and rice varieties within them, significant differences (P<0.001) were found for rice and maize individually, and at the cropping systems level. Use of BRRI-52 or BRRI-41 however had no observable carry-over effect on total GWP (Kg CO2eq ha–1) in maize, although rice varieties influenced GWP at the cropping systems-level. This resulted from greater productivity with BRRI-52, particularly in rainfed locations prone to flooding in the monsoon. For rice in partially irrigated environments, FP had the lowest total GWP across years (averaging 4,176 kg CO2eq ha–1) due to lower input use, followed by CT (mean of 4,319 kg CO2eq ha–1), CA (mean of 4,586, kg CO2eq ha–1) and SAT (mean of 5,195, kg CO2eq ha–1) (Table 7). These perhaps counter-intuitive results come from higher reactive CH4 emissions when maize residue was retained or incorporated in CA and SAT as computed using the CCAFS-MOT. This highlights the trade-offs associated with residue retention and yield, profitability, and energetics with total GWP as described in Section 3.3. It should however be noted that emissions arising from farmers’ post-harvest use of residues taken off the field and stored for later use as feed (in rice) or fuel (in maize) are not accounted for in the crop field-based CCAFS-MOT; as such, these results should be taken conservatively. Conversely, compared to partially irrigated environments, GWP from rice was comparatively lower in CA (with a mean of 3,022 kg CO2eq ha–1 across years) compared to FP or CT (means of 3,041 and 3,192 kg CO2eq ha–1, respectively) in rainfed environments (Table 8).
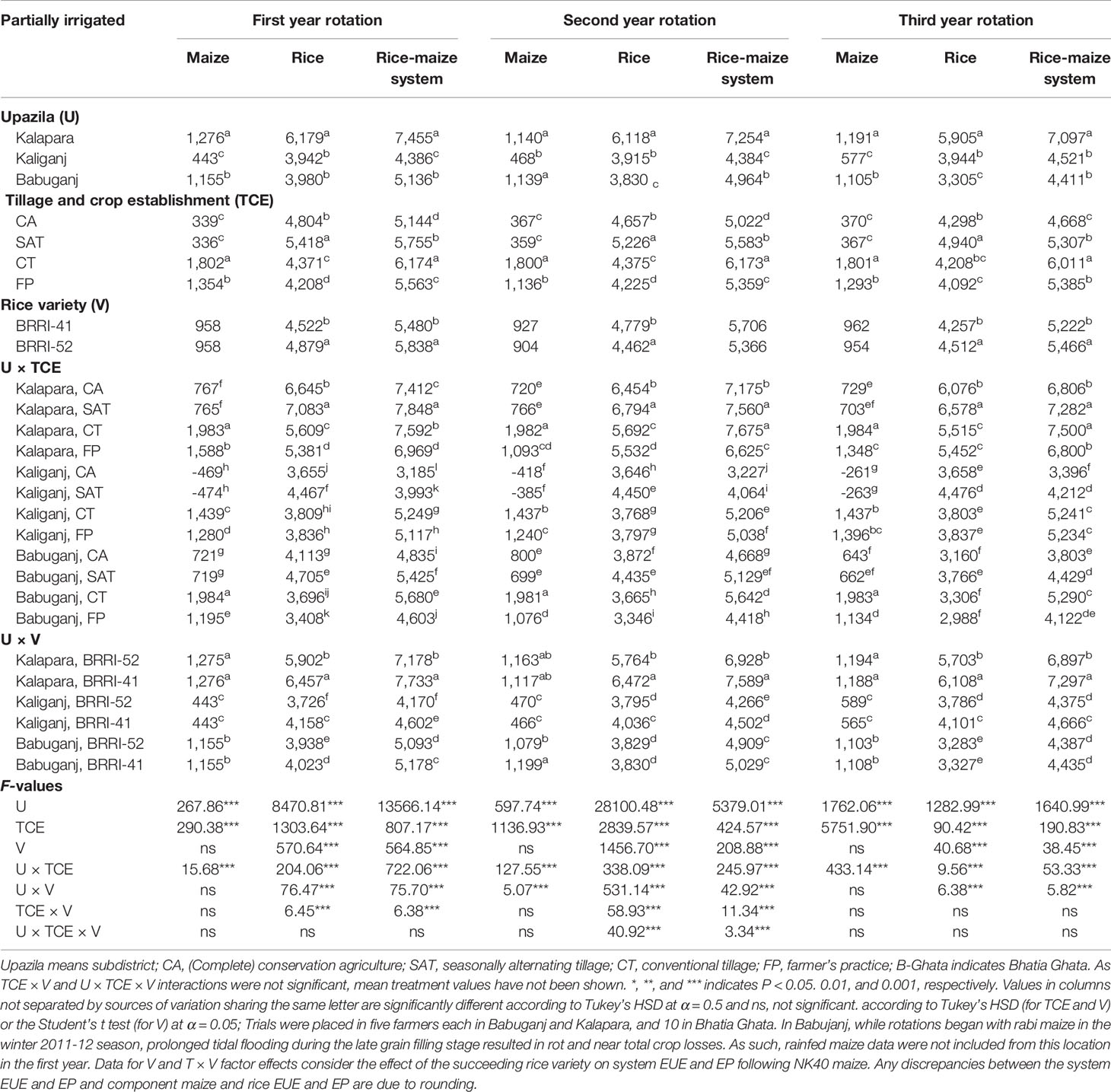
Table 7 Components of global warming potential (Mt CO2eq ha-1) by dry winter season maize and monsoon season rice over three years of rotation in partially irrigated environments in southern coastal Bangladesh.
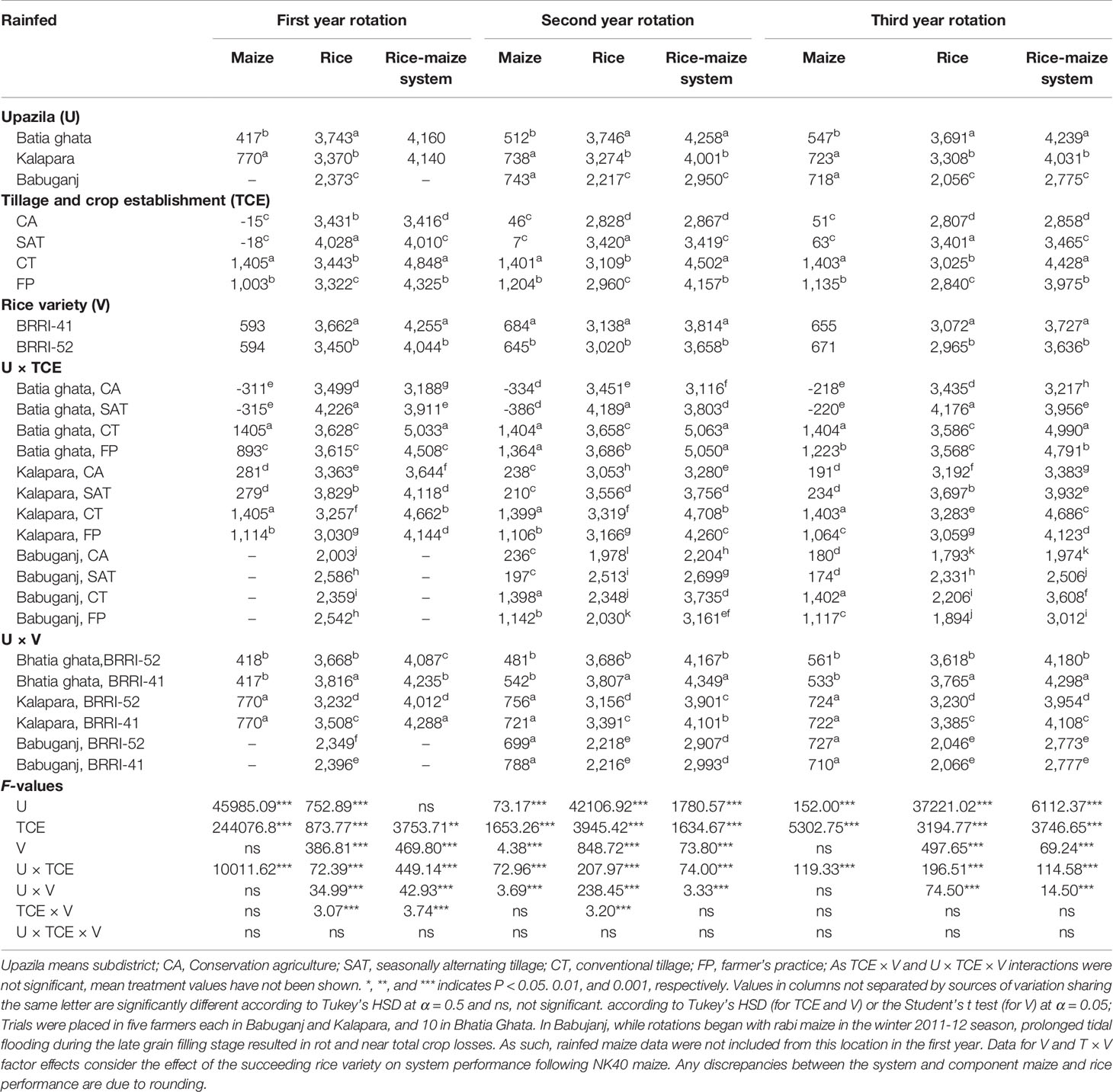
Table 8 Components of global warming potential (Mt CO2eq ha-1) by dry winter season maize and monsoon season rice over three years of rotation in rainfed environments in southern coastal Bangladesh.
Considering maize across three years in partially irrigated environments (Table 7), CA and SAT had an average GWP of 902 and 907 kg CO2eq ha–1 less than FP, and 1,442 and 1,447 kg CO2eq ha–1 less than CT, respectively. Trends in maize were similar in rainfed environments, although the lack of energy consumed for irrigation and minimization of tractor fuel requirements under strip tillage resulted in more emissions under CA (27 kg more CO2eq ha–1) and SAT (17 kg more CO2eq ha–1), respectively, relative to CT (Table 8). Conversely, although all rice residues were exported prior to maize establishment under FP and CT in rainfed environments, much higher GWP (means of 1114 and 1,403 Kg CO2eq ha–1, respectively) was observed, representative of increasing fuel use for tillage. At the cropping systems level, GWP varied considerably (P<0.001) in both environments. Considering both maize and rice in rotation, GWP followed the trend CA < SAT < FP < CT in rainfed and CA < FP < SAT < CT in partially irrigated environments.
Yield-Scaled Emissions
Yield-scaled emissions provide a measure of agronomic performance by integrating production with mitigation goals (Mosier et al., 2006; Pittelkow et al., 2014; Sainju, 2016). In both partially irrigated (Table 9) and rainfed (Table 10) environments, we observed significant effects (P<0.001) of location, tillage and crop establishment, and location × tillage and crop establishment interaction on YSE by both rice and maize and at the cropping systems level. In all years, there were also significant effects (P<0.001) of rice variety and location × variety interaction on YSE by rice and at the cropping systems level, but not by maize (Tables 7, 8). In partially irrigated environments, the 3-year average YSE at the systems-level was lowest in CA (1,085 kg CO2eq Mt-1 grain), followed by SAT (1,285 kg CO2eq Mt-1 grain), FP (1,316 kg CO2eq Mt-1 grain) and CT (CT 1,338 kg CO2eq Mt-1 grain), respectively (Table 9). Similar patterns were observed in rainfed environments, though YSE tended to be higher than in partially irrigated environments (Table 10), due to lower yields (data not shown). In both environments, the order of YSE by rice was CA < CT or FP < SAT, by maize CA or SAT < FP < CT, and at the cropping systems level was CA < SAT < FP < CT. The decreases in YSE under CA and SAT compared to FP and CT consequently also tended to be larger in rainfed than the partially irrigated environments. These results are consistent with Zeroes et al. (2017) for maize and Islam et al. (2020) for rice, and with other studies reporting reduced GHG emissions from CA or ZT compared to CT globally (Govaerts et al., 2009; Alam et al., 2019a; Alam et al., 2019b), although comparatively less research has considered adaptation of these practices, as included in this study.
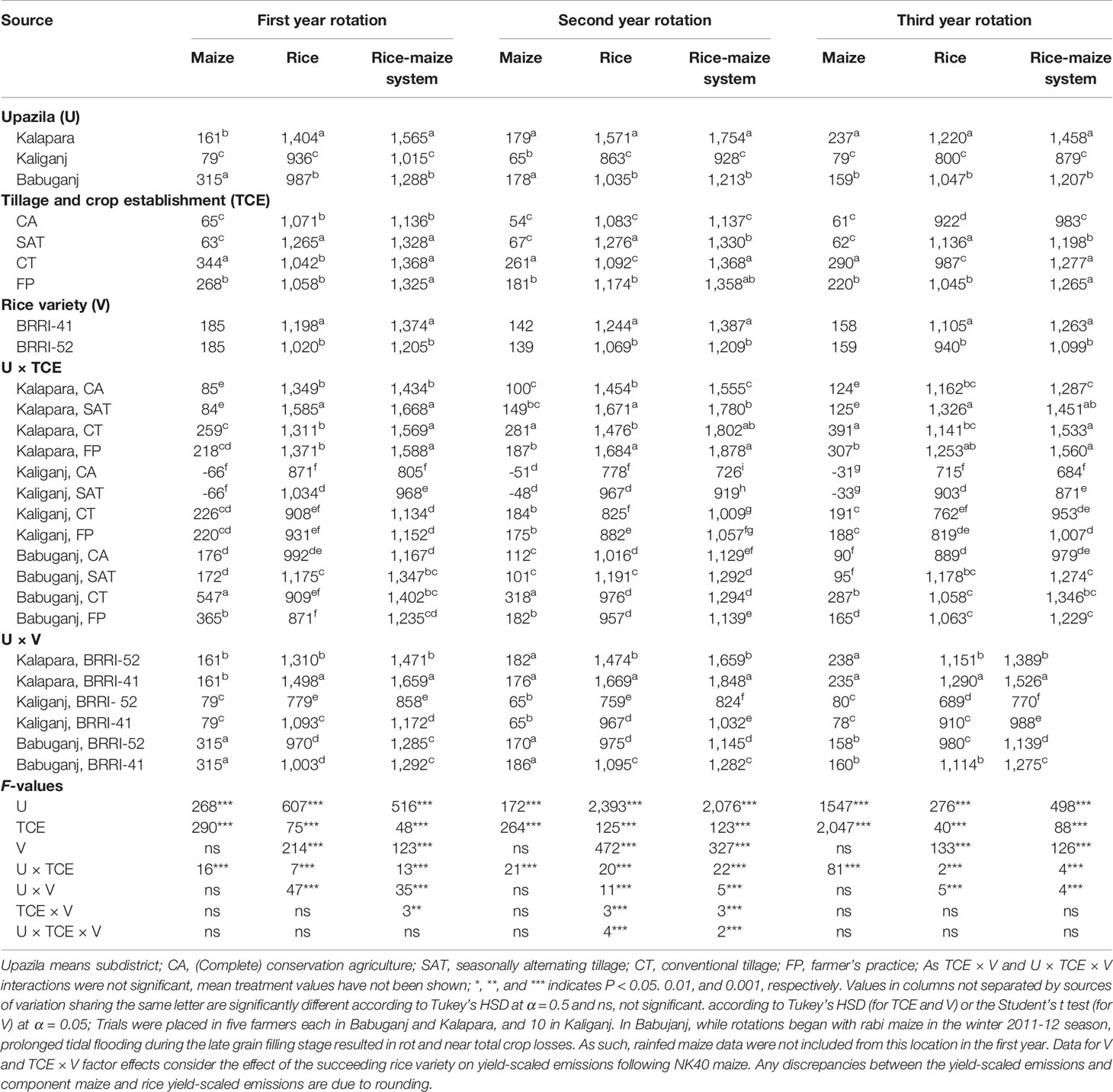
Table 9 Yield-scaled emissions (Kg CO2eq Mt-1 grain) by dry winter season maize, monsoon season rice, and systems (maize + rice) over three years of rotation in partially irrigated environments in southern coastal Bangladesh.
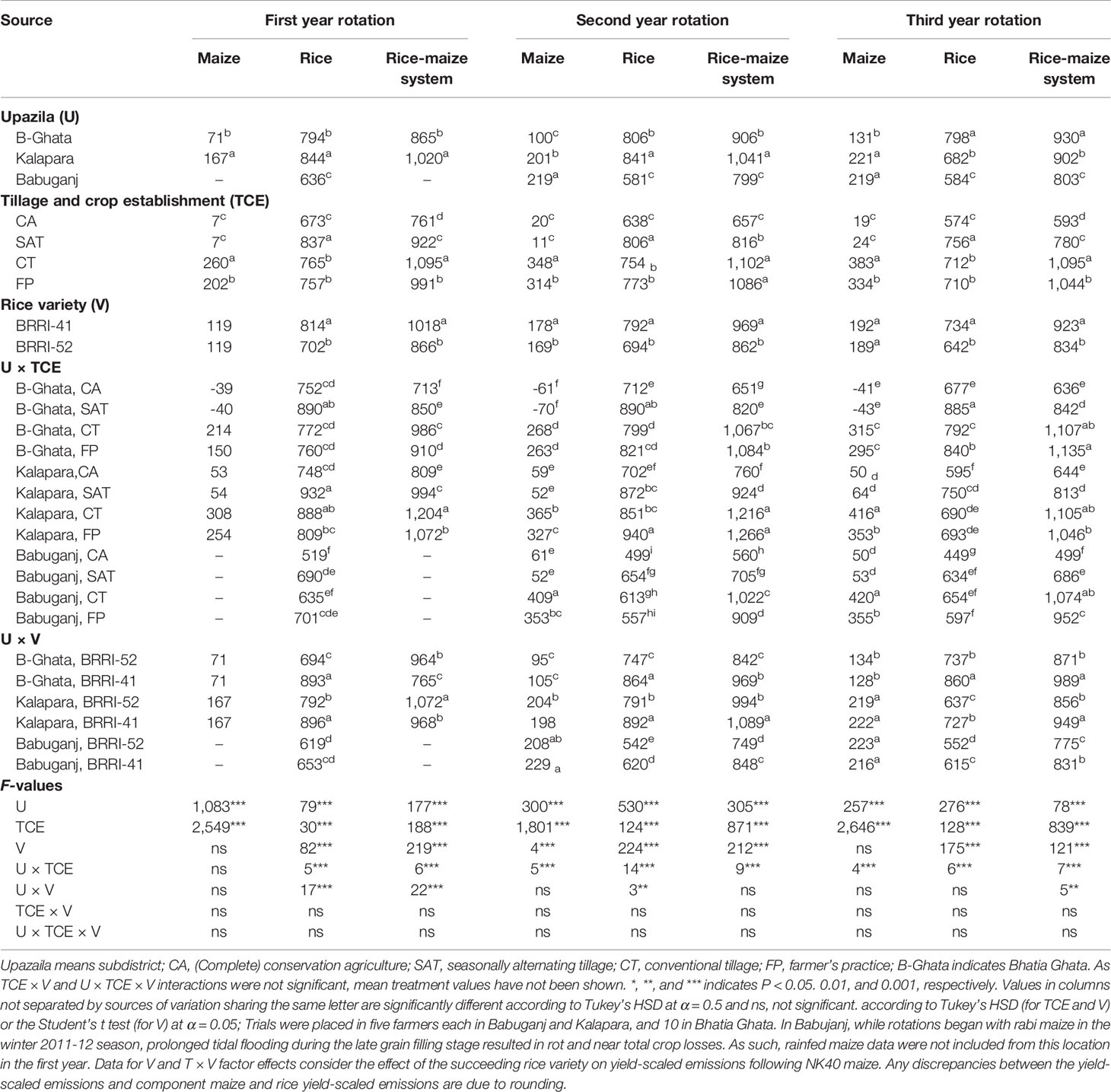
Table 10 Yield-scaled emissions (Kg CO2eq Mt-1 grain) by dry winter season maize, monsoon season rice, and systems (maize + rice) over three years of rotation in rainfed environments in coastal Bangladesh.
We do however suggest that these results should be considered conservatively. While our modeled results are indicative of the likely pattern of GWP and YSE across environments and treatments observed, in-situ measurements may to some extent differ (Richards et al., 2016). Such measurements are challenging, if not logistically infeasible in dispersed on-farm trials such as those described in this paper. The CCAFS-MOT also considers only the period within the cropping season (Feliciano et al., 2016); as such, we were also unable to model the GWP of fallow periods between the end of monsoon and start of the winter season, nor were we able to assess the transition from winter into monsoon season. Yet where researchers are unable to secure sufficient funding equipment and logistics to conduct repetitive in-situ measurements from many dispersed farmer-managed trials over multiple environments and years, modeling approaches such as those afforded by the CCAFS-MOT can be a secondary and useful indicator.
Additionally, Mei et al. (2018) concluded that N2O emission from conservation tillage is influenced by increased N rates, frequent wetting and drying cycles from irrigation, and the retention of residues, especially in warm tropical climates. In our study, however, N rates were not different between CA, SAT and CT for rice or maize in either rainfed or partially irrigated environments, though they were lower than FP. Farmers were also only able to apply a maximum of two irrigations in the partially irrigated locations, limiting wetting and drying cycles that couple nitrification with denitrification. Retention of residue conversely provides a substrate for nitrifier and denitrifier microbial populations that could accelerate emissions under lower soil O2 conditions that can also result from residue decomposition (Chen et al., 2013), and this was accounted for in the CCAFS-MOT. Yet while the model does account for N2O in rice, its ability to model N2O emissions that result from different tillage operations for non-rice crops is limited. Further research should therefore be conducted to improve tools such as the CCAFS-MOT for N2O under non-flooded conditions.
Integrated Analysis of Cropping Systems Performance
As evidenced from our results, complex changes in crop and cropping systems management can result in multi-dimensional trade-offs among agronomic, socio-economic, energetic, and environmental objectives. Complexity increases when additional objectives – for example reductions in labor and/or total input costs, and/or increased profitability, which may be a key objective for many farmers – are included. Careful assessment therefore needed to quantify trade-offs and offer solutions to resolve conflict among various criteria.
From the three years of researcher-designed but farmer-managed rice-maize rotational trials comparing different tillage and crop establishment methods, we observed that CA and SAT resulted in higher cropping (rice-maize) systems-level yields (by 15-18%), lower manual labor requirements (by 26-40%), and lower total production and tillage and crop establishment costs (by 1-12 and 33-55% respectively). As a consequence, these treatments also tended towards greater labor productivity (by 71-152%), produced greater grain energy output (by 13-17%), had higher total benefits and value-cost ratio (by 26-51% and 27-72% respectively), and had lower GWP (up to 9% lower) and YSE (2-18% less) compared to CT and FP in partially irrigated environments and with almost similar advantages in rainfed environments of southern coastal Bangladesh (Figure 2). CA also tended to perform slightly better across these criteria compared to SAT. Numerous studies have also reported multiple benefits of CA over CT in one or more of these indicators (Hobbs et al., 2008; Govaerts et al., 2009; Jat et al., 2014; Sapkota et al., 2015; Aryal et al., 2016; Alam et al., 2019a; Alam et al., 2019b; Dixon et al., 2020; Gathala et al., 2020). We are however unaware of studies reporting the benefits of adapted CA in the form of rotational tillage in rice-maize cropping systems, nor are we aware of studies on these topics in coastal environments.
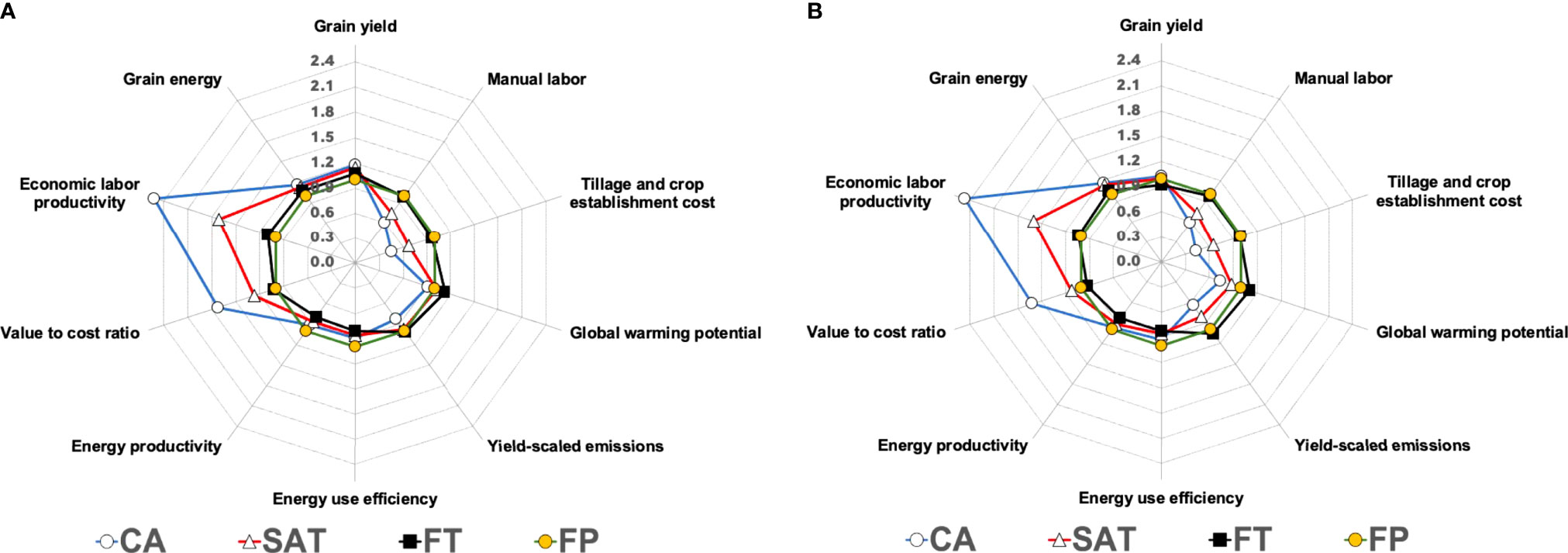
Figure 2 Radar diagrams representing multi-indicator assessment showing relative values (percent change relative to farmer’s practice) for grain yield (Mt ha-1), manual labor requirements (person-days ha-1), tillage and crop establishment cost (USD ha-1), value-cost ratio, energy-use efficiency (Mj ha-1 output/Mj ha-1 input), energy productivity (Kg ha-1 grain yield/Mj ha-1 input energy), economic labor productivity (net profits/labor use), global warming potential ((Mt CO2eq ha-1) and yield-scaled emissions (Kg CO2eq Mt-1 grain), for CA (open circle), adapted CA, i.e., SAT (open triangle), conventional tillage, i.e., CT (filled square), and farmer’s practice, i.e., FP (filled circle) treatments. Data presented show the average rice-maize rotational systems performance across all farmers within (A) partially irrigated or (B) rainfed environments. Note that in Babuganj, while rotations began with rabi maize in the winter 2011-12 season, prolonged tidal flooding during the late grain-filling stage resulted in rot and near total crop losses. As such, radar diagrams for rainfed locations do not consider the first year rice-maize rotation in this location.
Both CA and SAT involved considerable changes in the ways in which farmers typically grow rice and maize in rotation. Weed management under reduced tillage can be challenging (Somasundaram et al., 2020), and as such both the CA and SAT treatments used in this study relied on herbicides for weed control under strip-till conditions. This contrasts with the use of human labor for weed control in CT and FP, contributing to the 26-39% and 28-41% reduction in the person-days ha–1 required to grow these crops under CA and SAT, respectively. However, given the general low-degree of understanding among farmers of the potentially detrimental ecotoxicological effects of pesticide use (Shammi et al., 2018; Ahmed et al., 2021), as well as the potential for shifts in the community composition of weed species under reduced tillage and rice-maize rotations in Bangladesh (Hossain et al., 2020), caution should be applied when considering the introduction of herbicides.
When establishing maize, participating farmers in this study made use of pre-plant glyphosate followed by a post-emergence application of pendimethaline under CA for both rice and maize and for SAT. In rice, glyphosate was used followed by pretilachlor. The implications of glyphosate for human and environmental health remain a subject of intense debate and are reviewed by Van Bruggen et al. (2018) and Meftaul et al. (2020). Vighi et al. (2017) conversely discuss the human and ecological toxicity risks of pendimethaline, while Kaur et al. (2017) address pretilachlor. Although these molecules are commercially available in Bangladesh, extension services an organizations advising farmers should be aware of the evidence and implications of each product on human and environmental health, as well as of research on alternative weed management techniques for conservation agriculture (Sims et al., 2018; Somasundaram et al., 2020). In particular, the evolution of glyphosate resistance has become a challenge in a number of cropping systems where active ingredients are insufficiently rotated (Heap and Duke, 2018; Meftaul et al., 2020). Considering these issues, additional research should assess the viability of alternative and integrated management techniques for weed control under strip-tillage in Bangladesh.
Relative to FP, and comparing the multi-year mean performance of yield of rice and maize and at the cropping systems level to net profit, EP and yield-scaled CO2eq emissions across all treatments in both environments showed that all three performance indicators tended to be lower with YSE at the cropping systems level (Figures 2A, B). Relative differences were however smaller in rainfed locations, likely to lower maize yields and greater yield variability than in partially irrigated locations. These results – which are consistent across environments – suggest that high cereal cropping systems yields, high net profits, and high EP are possible with reduced YSE and investment costs where farmers apply complete CA. This backs Gathala et al. (2020) and Kumar et al. (2013), who demonstrated that CA can produce higher crop yields and profits with lower GWP than CT. Conversely, sustained adoption of the full suite of CA practices by farmers is exceedingly rare in South Asia, with most farmers modifying at least one component during rotations (Jat et al., 2020). Major challenges to CA adoption in South Asia include small land holdings (<1 ha), farmers’ low-risk bearing and investment capacity, a low level of technological reach to farmers, issues with weed management, low availability of appropriate seed drills, and the perception that well-tilled fields are an indicator of good agricultural practices (Somasundaram et al., 2020).
Farmers’ Preferences for Alternative Treatments
At the cropping systems level, although the full application of CA tended to exhibit the most favorable multi-indicator performance outcomes, farmers’ own preferences for treatments conversely indicated an aversion to CA. Many manual tasks performed on farms in Bangladesh are completed by hired laborers (Depenbusch et al., 2021). In our experiments, participant farmers bore the costs for all land preparation and crop establishment operations. While direct seeding machinery service providers were available and could be afforded to establish the maize crop under CA and SAT, farmers faced significant difficulties convincing hired laborers to manually transplant unpuddled rice plots under the CA treatment. Laborers were averse to this practice, which they complained increased drudgery and could injure their fingers.
While interest in mechanical and even unpuddled mechanical transplanting in response to increasing labor costs is growing in Bangladesh (Ashik-E-Rabbani et al., 2018; Basir et al., 2019), efforts are needed to assess these practices under on-farm conditions with farmers’ involvement in technology evaluation, as well as in the context of rotational cropping systems. In Bangladesh’s coastal region, in which control of floodwater prior to, during, and after the rice season can be p (Krupnik et al., 2017), use of mechanical transplanting – which requires shallow water during machine operation – may be challenging because of a lack of control over floodwater depth in the early monsoon season. Conversely, our study did demonstrate the superior performance of the submergent tolerant BRRI-52, which appears to be a reasonable option to manage rice in these coastal, monsoon season flood-prone environments.
As a consequence of these challenges, 72% of the farmers participating in experiments in partially irrigated locations indicated that they would not consider application of the full suite of CA practices as something they would be willing to apply in their own fields. In comparison, 94% indicated their dislike for the CT treatment, as they felt that tillage could be eliminated so long as power-tiller operated seeders were available that could be used to establish maize with strip-tillage in the winter season. Only 28% of participating farmers found the SAT treatment infeasible and suggested their preference for it over other treatments. A similar pattern was observed in the rainfed locations, in which 10 and 22% more farmers, respectively, preferred the CA and SAT treatments compared to CT. Importantly, however, farmers did clearly stress that their interest in approaches aligned with the SAT treatment were contingent on the continued affordability and availability of machinery service providers who could reliably offer quality strip till seeding services for maize on an affordable fee-for-services basis.
Additionally, while we were unable to quantify the transactions costs that farmers may have accrued when negotiating with laborers to establish rice in the CA treatment, many farmers discussed the time and effort it took to mobilize labor to be willing to manually transplant into unpuddled fields. Although costs may have been limited due to the relatively small size of our experimental plots, farmers expressed concern that convincing laborers to manually transplant larger, non-experimental fields that are not puddled may present significant challenges. The importance of considering farmer-workforce relations and laborers’ agreement to support technology changes and adoption are discussed by Cofre-Bravo et al. (2018). After three years of participation in the management of experimental treatments, feedback provided by farmers during the post-trial discussions provide support for this observation, emphasizing the need to consider measurements of farmers’ preferences for and satisfaction with experimental treatments, as well as quantification of transaction costs and logistical challenges associated with alternative management practices.
These observations are important, as they provide some inference as to why the adoption of the full suite of CA practices has remained low in South Asia (cf. Somasundaram et al., 2020; Akter et al., 2021). In particular, the practice of wet-tillage of rice fields, which in part is conducted to aid in making transplanting easier, is a thousands of years old practice (Greenland, 1997). Discussions with farmers both prior to and after three years of experiments confirmed a strong aversion to the elimination of tillage for rice, although farmers found strip tillage for maize more to be a viable management practice.
Conclusions
Through multi-year and multi-location farmer-managed trials, we studied the multi-indicator performance of tillage and crop establishment performance of rice-maize systems in terms of energy use, energy-use efficiency and energy productivity, GWP and yield-scaled CO2-eq emissions, in addition to farmers’ preferences. This was accomplished using multiple agronomic, energetic, environmental, and economic criteria in relatively under-studied partially irrigated and rainfed environments in southern coastal Bangladesh. At the cropping systems level, CA followed by SAT, tended to have significantly lower energy use and higher energy productivity, and reduced GWP and yield-scaled CO2-eq emissions, compared to CT and FP. Multi-indicator assessment revealed that CA and SAT practices result in improved energetic performance and higher rice-maize cropping systems yields, while entailing reductions in manual labor, and crop establishment and total production costs. This resulted in higher profitability levels than alternative treatments. CA and SAT also tended to produce greater grain energy output and resulted in lower yield-scaled GHG emissions compared to CT and FP across environments.
While numerous agricultural development programs have worked to popularize CA in South Asia, our data suggest that adapted CA still results in many of the benefits of complete CA. We conclude that in these coastal environments, both CA and SAT practices have the potential to increase cereal yields and energy productivity while reducing yield-scaled emissions, thereby enabling farmers even in challenging coastal environments to produce more while reducing energy use and mitigating GHG emissions. However, in consideration of farmers’ aversion to the elimination of tillage in rice, our study suggests that adaptations in CA practices and seasonal tillage prior to rice may be a more practical fit for rice-maize systems managed by smallholders reluctant to eliminate tillage for rice in coastal Bangladesh. Future research and development efforts should consequently concentrate on raising awareness of the advantages of these practices – not only among farmers, but also among agricultural development organizations that have focused more strongly on popularizing the full suite of CA practices without adequately considering farmers’ preferences or the trade-offs that may result from the significant change to CA management in otherwise fully tilled systems. Lastly, although our data provide support for the adaptation of CA or SAT practices, the establishment of maize under strip-tillage outside of experimental settings will require integrated development efforts that focus not only on agronomic management, but also on building supportive value chains to improve availability and affordability of the inputs and farm machinery required to successfully establish crops with such practices.
Data Availability Statement
The original contributions presented in the study are included in the article/Supplementary Material. Further inquiries can be directed to the corresponding author.
Ethics Statement
The studies involving human participants were reviewed and approved by CIMMYT Internal Research Ethics Committee. Written informed consent for participation was not required for this study in accordance with the national legislation and the institutional requirements.
Author Contributions
TJK, MKG, SY, MS, FH, AK, SR, and AJM contributed to the conception and design of the study. TJK, SY, MS, KH, FH, AK, BR, and AJM, organized the database. TJK, MH, and TBS performed the statistical analysis and interpretation of data. TS and JT wrote the first draft of the manuscript. TJK, JT, MKG, and AJM wrote sections of the manuscript. All authors contributed to manuscript revision, read, and approved the submitted version.
Author Disclaimer
The contents and opinion expressed herein are those of the author(s) and do not necessarily reflect the views of USAID, the United States Government, CGIAR or its donors, and shall not be used for advertising or product endorsement purposes.
Conflict of Interest
The authors declare that the research was conducted in the absence of any commercial or financial relationships that could be construed as a potential conflict of interest.
Publisher’s Note
All claims expressed in this article are solely those of the authors and do not necessarily represent those of their affiliated organizations, or those of the publisher, the editors and the reviewers. Any product that may be evaluated in this article, or claim that may be made by its manufacturer, is not guaranteed or endorsed by the publisher.
Acknowledgments
This research was conducted under the USAID and Bill and Melinda Gates Foundation supported Cereal Systems Initiative for South Asia (CSISA; http://csisa.org/). Analytical and written work was also implemented as part of the CGIAR Regional Integrated Initiative Transforming Agrifood Systems in South Asia, or TAFSSA (https://www.cgiar.org/initiative/20-transforming-agrifood-systems-in-south-asia-tafssa/) and Securing the Food Systems of Asian Mega-Deltas for Climate and Livelihood Resilience (https://www.cgiar.org/initiative/18-securing-the-asian-mega-deltas-from-sea-level-rise-flooding-salinization-and-water-insecurity). We thank Ken Sayer for proposing the research design, and Sreejith Aravindakshan for analytical advice. This paper is dedicated to the memory of Dr. Md. Murshedul Alam, whose untimely passing is a significant blow to Bangladesh’s agronomic community.
Supplementary Material
The Supplementary Material for this article can be found online at: https://www.frontiersin.org/articles/10.3389/fagro.2022.829737/full#supplementary-material
References
Acaroglu M., Aksoy A. S. (2005). The Cultivation and Energy Balance of Miscanthus Giganteus Production in Turkey. Biomass Bioenergy 29, 42–48. doi: 10.1016/j.biombioe.2005.01.002
Ahmed S., Kumar V., Alam M., Dewan M. R., Bhuiyan K. A., Miajy A. A., et al. (2021). Integrated Weed Management in Transplanted Rice: Options for Addressing Labor Constraints and Improving Farmers’ Income in Bangladesh. Weed. Tech. 35, 697–709. doi: 10.1017/wet.2021.50
Akter S., Gathala M. K., Timsina J., Islam S., Rahman M., Ghosh A. K. (2021). Adoption of Conservation Agriculture-Based Tillage Practices in the Rice-Maize Systems in Bangladesh. World Dev. Perspec. 21, 100297. doi: 10.1016/j.wdp.2021.100297
Alam M. K., Bell R. W., Biswas W. K. (2019b). Decreasing the Carbon Footprint of an Intensive Rice-Based Cropping System Using Conservation Agriculture on the Eastern Gangetic Plains. J. Clean. Prod. 218, 259–272. doi: 10.1016/j.jclepro.2019.01.328
Alam M. K., Biswas W. K., Bell R. W. (2016). Greenhouse Gas Implications of Novel and Conventional Rice Production Technologies in the Eastern-Gangetic Plains. J. Clean. Prod. 112, 3977–3987. doi: 10.1016/j.jclepro.2015.09.071
Alam M. K., Biswas W. K., Bell R. W. (2019a). Increases in Soil Sequestered Carbon Under Conservation Agriculture Cropping Decrease the Estimated Greenhouse Gas Emissions of Wetland Rice Using Life Cycle Assessment. J. Clean. Prod. 224, 72–87. doi: 10.1016/j.jclepro.2019.03.215
Alam M. M., Ladha J. K., Faisal M. W., Sharma S., Saha A., Noor S., et al. (2015). Improvement of Cereal-Based Cropping Systems Following the Principles of Conservation Agriculture Under Changing Agricultural Scenarios in Bangladesh. Field Crops Res. 175, 1–15. doi: 10.1016/j.fcr.2014.12.015
Ali M. Y., Waddington S. R., Timsina J., Hodson D., Dixon J. (2009). Maize-Rice Cropping Systems in Bangladesh- Status and Research Need. J. @ Agric. Sci. Techn. 3, 35–53. doi: 10.22004/ag.econ.56106
Aravindakshan S., Krupnik T. J., Amjath-Babu T. S., Speelman E. N., Tur-Cardona J., Tittonell P., et al. (2021). Quantifying Farmers’ Preferences for Cropping Systems Intensification: A Choice Experiment Approach Applied in Coastal Bangladesh’s Risk Prone Farming Systems. Agric. Syst. 189, 103069. doi: 10.1016/j.agsy.2021.103069
Aryal J. P., Sapkota T. B., Jat M. L., Bishnoi D. K. (2014). On-Farm Economic and Environmental Impact of Zero-Tillage Wheat: A Case of Northwest India. Experim. Agric. 51, 1–16. doi: 10.1017/S001447971400012X
Aryal J. P., Sapkota T. B., Stirling C. M., Jat M. L., Jat H. S., Rai M., et al. (2016). Conservation Agriculture-Based Wheat Production Better Copes With Extreme Climate Events Than Conventional Tillage-Based Systems: A Case of Untimely Excess Rainfall in Haryana, India. Agric. Ecosyst. Environ. 233, 325–335. doi: 10.1016/j.agee.2016.09.013
Ashik-E-Rabbani M., Hossain M. M., Sarkar S., Saha C. K., Alam M. M., Kalita P. K., et al. (2018). “Cost Saving Rice Transplanting Technology for Puddled and Unpuddled Soil Condition,” in 2018 ASABE Annual International Meeting, 1 St. Joseph, MI: ASABE. (Cobo Center, Detroit, Michigan USA: American Society of Agricultural and Biological Engineers).
Basir S., Ashik-E-Rabbani Md., Sarkar S., Alam M., Saha C. K. (2019). Suitability of Mechanical Transplanting of Hybrid Variety Rice in Unpuddled Soil Condition. J. Agroeco. Nat. Res. Mgt. 6, 186–191.
Bell R. W., Haque M. E., Jahiruddin M., Rahman M. M., Begum M., Miah M. A. M., et al. (2019). Conservation Agriculture for Rice-Based Intensive Cropping by Smallholders in the Eastern Gangetic Plain. Agriculture 9, 5. doi: 10.3390/agriculture9010005
Chen H., Li X., Hu F., Shi W. (2013). Soil Nitrous Oxide Emissions Following Crop Residue Addition: A Meta-Analysis. Glob. Change Biol. 19, 2956–2964. doi: 10.1111/gcb.12274
Cofre-Bravo G., Engler A., Klerkx L., Leiva-Bianchi M., Adasme-Berrios C., Caceres C. (2018). Considering the Farm Workforce as Part of Farmers’ Innovative Behaviour: A Key Factor in Inclusive on-Farm Processes of Technology and Practice Adoption. Exp. Agric. 55, 723–737. doi: 10.1017/S0014479718000315
Depenbusch L., Farnworth C. R., Schreinemachers P., Myint T., Islam M. M., Kundu N. D., et al. (2021). When Machines Take the Beans: Ex-Ante Socioeconomic Impact Evaluation of Mechanized Harvesting of Mungbean in Bangladesh and Myanmar. Agronomy 11, 925. doi: 10.3390/agronomy11050925
Derpsch R., Franzluebbers A. J., Duiker S. W., Reicosky D. C., Koeller K., Friedrich T., et al. (2014). Why do We Need to Standardize No-Tillage Research? Soil Till. Res. 137, 16–22. doi: 10.1016/j.still.2013.10.002
Dixon J., Rola-Rubzen M. F., Timsina J., Cummins J., Tiwari T. P. (2020). “Socioeconomic Impacts of Conservation Agriculture Based Sustainable Intensification (CASI) With Particular Reference to South Asia,” in No-Till Farming Systems for Sustainable Agriculture: Challenges and Opportunities. Eds. Dang Y., Dalal R., Menzies N. W. (Springer Nature Switzerland AG 2020: Springer publication).
Ecoinvent Center (2007). “Ecoinvent Data V2.0. Ecoinvent Report No. 1-25,” in Swiss Centre of Life Cycle Inventories (Dubendorf, Switzerland: Swiss Centre for Life Cycle Inventories, Dübendorf, 2007).
Erenstein O., Thorpe W. (2011). Livelihoods and Agro-Ecological Gradients: A Meso-Level Analysis in the Indo-Gangetic Plains, India. Agric. Syst. 104, 42–53. doi: 10.1016/j.agsy.2010.09.004
FAO (2011a). The State of the World’s Land and Water Resources for Food and Agriculture (SOLAW) – Managing Systems at Risk (Rome and Earthscan, London: Food and Agriculture Organization of the United Nations).
FAO (2011b). “Biodiversity for Food and Agriculture Contributing to Food Security and Sustainability in a Changing World,” in Outcomes of an Expert Workshop Held by FAO and the Platform on Agrobiodiversity Research. (Rome, Italy: FAO/ Platform for Agrobiodiversity Research).
FAO (2018)Conservation Agriculture Land Area in Afghanistan, Bangladesh, India, Nepal, Pakistan, Sri Lanka in 2013. In: Aquastat. Available at: http://www.fao.org/nr/water/aquastat/main/index.stm (Accessed 30 August 2018).
Feliciano D., Nayak D., Vetter S. H., Hillier J. (2016) CCAFS Mitigation Options Tool. Available at: www.ccafs.cgiar.org (Accessed 30 August 2018).
Feliciano D., Nayak D. R., Vetter S. H., Hillier J. (2017). CCAFS-MOT - A Tool for Farmers, Extension Services and Policy-Advisors to Identify Mitigation Options for Agriculture. Agric. Syst. 154, 100–111. doi: 10.1016/j.agsy.2017.03.006
Gathala M. K., Kumar V., Sharma P. C., Saharawat Y. S., Jat H. S., Singh M., et al. (2013). Optimizing Intensive Cereal-Based Cropping Systems Addressing Current and Future Drivers of Agricultural Change in the North-Western Indo-Gangetic Plains of India. Agric. Ecosyst. Environ. 177, 85–97. doi: 10.1016/j.agee.2013.06.002
Gathala M. K., Laing A. M., Tiwari T. P., Timsina J., Islam S., Bhattacharya P. M., et al. (2020). Energy-Efficient, Sustainable Crop Production Practices Benefit Smallholder Farmers and the Environment Across Three Countries in the Eastern Gangetic Plains, South Asia. J. @ Clean. Prod. 246, 118982. doi: 10.1016/j.jclepro.2019.118982
Gathala M. K., Timsina J., Islam M. S., Krupnik T. J., Bose T. R., Islam N., et al. (2016). Productivity, Profitability, and Energetics: A Multi-Criteria Assessment of Farmers’ Tillage and Crop Establishment Options for Maize in Intensively Cultivated Environments of South Asia. Field Crop Res. 186, 32–46. doi: 10.1016/j.fcr.2015.11.008
Gathala M. K., Timsina J., Islam M. S., Rahman M. M., Hossain M. I., Harun-Ar-Rashid M., et al. (2015). Conservation Agriculture Based Tillage and Crop Establishment Options can Maintain Farmers’ Yields and Increase Profits in South Asia’s Rice–Maize Systems: Evidence From Bangladesh. Field Crop Res. 172, 85–98. doi: 10.1016/j.fcr.2014.12.003
Gomez K. A., Gomez A. A. (1984). Statistical Procedures for Agricultural Research. 2nd ed (New York: John Wiley and Sons), 680.
Govaerts B., Verhulst N., Castellanos-Navarrete A., Sayre K. D., Dixon J., Dendooven L. (2009). Conservation Agriculture and Soil Carbon Sequestration: Between Myth and Farmer Reality Crit. Rev. Plant Sci. 28, 97–122. doi: 10.1080/07352680902776358
Greenland D. J. (1997). The Sustainability of Rice Farming (Wallingford, Oxon, UK; New York Manila: Cab International, International Rice Research Institute).
Haque M. E., Bell R. W., Islam M. A., Rahman M. A. (2016). Minimum Tillage Unpuddled Transplanting: An Alternative Crop Establishment Strategy for Rice in Conservation Agriculture Cropping Systems. Field Crops Res. 185, 31–39. doi: 10.1016/j.fcr.2015.10.018
Harvey C. A., Chacón M., Donatti C. I., Garen E., Hannah L., Andrade A. (2013). Climate-Smart Landscapes: Opportunities and Challenges for Integrating Adaptation and Mitigation in Tropical Agriculture. Cons. Lett. 7, 77–90. doi: 10.1111/conl.12066
Heap I., Duke S. O. (2018). Overview of Glyphosate-Resistant Weeds Worldwide. Pest Mgt. Sci. 74, 1040–1049. doi: 10.1002/ps.4760
Hobbs P. R., Sayre K., Gupta R. (2008). The Role of Conservation Agriculture in Sustainable Agriculture. Philos. T. R. Soc B. 363, 543–555. doi: 10.1098/rstb.2007.2169
Hossain K., Timsina J., Johnson D. E., Gathala M. K., Krupnik T. J. (2020). Multi-Year Weed Community Dynamics and Rice Yields as Influenced by Tillage, Crop Establishment, and Weed Control: Implications for Rice-Maize Rotations in the Eastern Gangetic Plains. Crop Prot. 138, 105334. doi: 10.1016/j.cropro.2020.105334
Hossen M. A., Hossain M. M., Haque M. E., Bell R. W. (2018). Transplanting Into non-Puddled Soils With a Small-Scale Mechanical Transplanter Reduced Fuel, Labour and Irrigation Water Requirements for Rice (Oryza Sativa L.) Establishment and Increased Yield. Field Crops Res. 225, 141–151. doi: 10.1016/j.fcr.2018.06.009
IPCC (2006). “IPCC Guidelines for National Greenhouse Gas Inventories,” in IPCC Guidelines for National Greenhouse Gas Inventories. Eds. Eggleston S., Buendia L., Miwa K., Ngara T., Tanabe K. (Japan: Institute for Global Environmental Strategies).
IPCC (2013). Climate Change: The Physical Science Basis. Working Group I Contribution to the FiCTh Assessment Report of the Intergovernmental Panel on Climate Change. Chapter 8: Anthropogenic and Natural Radiative Forcing. Intergovern. Panel. Climate Change.
Islam A.K.M.S., Hossain M.M., Saleque M.A., Rabbani M.A., Sarker R.I. (2013). Energy Consumption in Unpuddled Transplanting of Wet Season Rice Cultivation in North West Region of Bangladesh. Prog. Agric. 24, 229–237. doi: 10.3329/pa.v24i1-2.19176
Islam S., Gathala M. K., Tiwari T. P., Timsina J., Laing A. M., Maharjan S., et al. (2019). Conservation Agriculture for Sustainable Intensification: Increasing Yields and Water Productivity for Smallholders of the Eastern Gangetic Plains. Field Crops Res. 238, 1–17. doi: 10.1016/j.fcr.2019.04.005
Islam S. F., Sander B. O., Quilty J., de Neergaard A., Groenigen J., Jensen L. (2020). Mitigation of Greenhouse Gas Emissions and Reduced Irrigation Water Use in Rice Production Through Water-Saving Irrigation Scheduling, Reduced Tillage and Fertiliser Application Strategies. Sci. Total. Environment. 739, 140215. doi: 10.1016/j.scitotenv.2020.140215
Ismail A. M., Singh U., Singh S., Dar M. H., Mackill D. J. (2013). The Contribution of Submergence-Tolerant (Sub1) Rice Varieties to Food Security in Flood-Prone Rainfed Lowland Areas in Asia. Field Crop Res. 152, 83–93. doi: 10.1016/j.fcr.2013.01.007
Jat M. L., Chakraborty D., Ladha J. K., Rana D. S., Gathala M. K., McDonald A., et al. (2020). Conservation Agriculture for Sustainable Intensification in South Asia. Nat. Sustainab. 3, 336–343. doi: 10.1038/s41893-020-0500-2
Jat R. A., Sahrawat K. L., Kassam A. H. (2013). Conservation Agriculture: Global Prospects and Challenges (Boston: CAB International).
Jat R. K., Sapkota T. B., Singh R. G., Jat M. L., Kumar M., Gupta R. K. (2014). Seven Years of Conservation Agriculture in a Rice-Wheat Rotation of Eastern Gangetic Plains of South Asia: Yield Trends and Economic Profitability. Field Crop Res. 164, 199–210. doi: 10.1016/j.fcr.2014.04.015
Kamruzzaman M., Shaw R. (2018). Lood and Sustainable Agriculture in the Haor Basin of Bangladesh: A Review Paper. Univ. J. Agr. Res. 6, 40–49.
Katalyst and Swiss Contact (2017) Maize Cultivation Improves Income in Bangladesh: A Mini Case Study (Katalyst, House 20, Road 6 Baridhara, Dhaka 1212, Bangladesh). Available at: www.swisscontact.org (Accessed 23 April 2019).
Kaur P., Kaur P., Duhan A., Bhullar M. S. (2017). Effect of Long-Term Application of Pretilachlor on its Persistence and Residues in Paddy Crop. Environ. Tech. 38, 2410–2415. doi: 10.1080/09593330.2016.1263684
Keil A., D’Souza A., McDonald A. (2017). Zero Tillage is a Proven Technology for Sustainable Wheat Intensification in the Eastern Indo-Gangetic Plains: What Determines Farmer Awareness and Adoption? Food Secur. 9, 723–743. doi: 10.1007/s12571-017-0707-x
Keil A., Mitra A., McDonald A., Malik R. K. (2020). Zero-Tillage Wheat Provides Stable Yield and Economic Benefits Under Diverse Growing Season Climates in the Eastern Indo-Gangetic Plains. Int. J. @ Agric. Sustainab. 18 (6), 567–593. doi: 10.1080/14735903.2020.1794490
Krupnik T. J., Santos Valle S., Hossain I., Gathala M. K., Justice S., Gathala M. K., et al. (2013). Made in Bangladesh: Scale-Appropriate Machinery for Agricultural Resource Conservation (Mexico, D.F: International Maize and Wheat Improvement Center).
Krupnik T. J., Schulthess U., Ahmed Z. U., McDonald A. J. (2017). Sustainable Crop Intensification Through Surface Water Irrigation in Bangladesh? A Geospatial Assessment of Landscape-Scale Production Potential. Land. Use Policy 60, 206–222. doi: 10.1016/j.landusepol.2016.10.001
Kumar V., Saharawat Y. S., Gathala M. K., Jat A. S., Singh S. K., Chaudhary N., et al. (2013). Effect of Different Tillage and Seeding Methods on Energy Use Efficiency and Productivity of Wheat in the Indo-Gangetic Plains. Field Crops Res. 142, 1–8. doi: 10.1016/j.fcr.2012.11.013
Laik R., Sharma S., Idris M., Singh A. K., Singh S. S., Bhatt B. P., et al. (2014). Integration of Conservation Agriculture With Best Management Practices for Improving System Performance of the Rice–Wheat Rotation in the Eastern Indo-Gangetic Plains of India. Agric. Ecosyst. Environ. 195, 68–82. doi: 10.1016/j.agee.2014.06.001
Magar S. T., Timsina J., Devkota K., Liang W. (2022b). Energy and Greenhouse Gas Footprint Analysis of Conventional and Reduced Tillage Practices in Rainfed and Irrigated Rice-Wheat Systems. Paddy. Water Environ. (In press) 7. doi: 10.1007/s10333-022-00902-w
Magar S. T., Timsina J., Devkota K., Liang W., Neeranjan R. (2022a). Conservation Agriculture for Increasing Productivity, Profitability and Water Productivity in Rice-Wheat System of the Eastern Gangetic Plains. Environ. Challen. 7, 100468. doi: 10.1016/j.envc.2022.100468
Meftaul I. M., Venkateswarlu K., Dharmarajan R., Annamalai P., Asaduzzaman M., Parven A., et al. (2020). Controversies Over Human Health and Ecological Impacts of Glyphosate: Is it to be Banned in Modern Agriculture? Env. pollut. 263, 114372. doi: 10.1016/j.envpol.2020.114372
Mei K., Wang Z., Huang H., Zhang C., Shang X., Dahlgren R. A., et al. (2018). Stimulation of N2O Emission by Conservation Tillage Management in Agricultural Lands: A Meta-Analysis. Soil Till. Res. 182, 86–93. doi: 10.1016/j.still.2018.05.006
Mosier A. R., Halvorson A. D., Reule C. A., Liu X. J. (2006). Net Global Warming Potential and Greenhouse Gas Intensity in Irrigated Cropping Systems in North-Eastern Colorado. J. Environ. Qual. 35, 1584–1598. doi: 10.2134/jeq2005.0232
Nassiri S. M., Singh S. (2009). Study on Energy Use Efficiency for Paddy Crop Using Data Envelopment Analysis (DEA) Technique. App. Energy 86, 1320–1325. doi: 10.1016/j.apenergy.2008.10.007
Ogle S., Breidt F. J., Paustian K. (2005). Agricultural Management Impacts on Soil Organic Carbon Storage Under Moist and Dry Climatic Conditions of Temperate and Tropical Regions. Biogeochem 72, 87–121. doi: 10.1007/s10533-004-0360-2
Pannell D. J., Llewellyn R. S., Corbeels M. (2014). The Farm-Level Economics of Conservation Agriculture for Resource-Poor Farmers. Agriculture, Ecosystems & Environment187, 1, 52–64. doi: 10.1016/j.agee.2013.10.014
Pathak H., Saharawat Y. S., Gathala M., Ladha J. K. (2011). Impact of Resource-Conserving Technologies on Productivity and Greenhouse Gas Emission in Rice-Wheat System. Greenh. Gas.: Sci. Tech. 1, 261–277. doi: 10.1002/ghg.27
Pimentel D. (2009). Crop Production in Developing and Developed Nations. Energies. 2, 1–24. doi: 10.3390/en20100001
Pittelkow C. M., Adviento-Borbe M. A., van Kessel C., Hill J. E., Linquist B. A. (2014). Optimizing Rice Yields While Minimizing Yield-Scaled Global Warming Potential. Global Change Biol. 20, 1382–1393. doi: 10.1111/gcb.12413
Powlson D. S., Stirling C. M., Thierfelder C., White R. P., Jat M. L. (2016). Does Conservation Agriculture Deliver Climate Change Mitigation Through Soil Carbon Sequestration in Tropical Agro-Ecosystems? Agric. Ecosyst. Environ. 220, 164–174. doi: 10.1016/j.agee.2016.01.005
Pretty J., Bharucha Z. P. (2014). Sustainable Intensification in Agricultural Systems. Ann. Bot. 114, 1571–1596. doi: 10.1093/aob/mcu205
Rahman M. M. (2012). Enhancement of Resilience of Coastal Community in Bangladesh Through Crop Diversification in Adaptation to Climate Change Impacts. M. Sc. Thesis Vol. 82 (Dhaka, Bangladesh: BRAC University).
Rahman M. M., Ali M. R., Oliver M. M. H., Hanif M. A., Uddin M. Z., Tamim Ul H., et al. (2021). Farm Mechanization in Bangladesh: A Review of the Status, Roles, Policy, and Potentials. Food Res. 6, 100225. doi: 10.1016/j.jafr.2021.100225
Rahman S., Rahman S. (2013). Energy Productivity and Efficiency of Maize Accounting for the Choice of Season and Environmental Factors: An Empirical Analysis From Bangladesh. Energy 49, 339–346. doi: 10.1016/j.energy.2012.10.042
Rasul G. (2014). Food, Water, and Energy Security in South Asia: A Nexus Perspective From the Hindu Kush Himalayan Region. Env. Sci. Pol. 39, 35–48. doi: 10.1016/j.envsci.2014.01.010
R Core Team (2020). “R: A Language and Environment for Statistical Computing,” in R Foundation for Statistical Computing(Vienna, Austria). Available at: www.R-project.org.
Richards M., Metzel R., Chirinda N., Ly P., Nayamadzawo G., Duong Q., et al. (2016). Limits of Agricultural Greenhouse Gas Calculators to Predict Soil N2O and CH4 Fluxes in Tropical Agriculture. Sci. Rep. 6. doi: 10.1038/srep26279
Sainju U. M. (2016). A Global Meta-Analysis on the Impact of Management Practices on Net Global Warming Potential and Greenhouse Gas Intensity From Cropland Soils. PloS One 11 (2), e0148527. doi: 10.1371/journal.pone.0148527
Sapkota T. B., Jat M. L., Aryal J., Jat R., Khatri-Chhetri A. (2015). Climate Change Adaptation, Greenhouse Gas Mitigation and Economic Profitability of Conservation Agriculture: Some Examples From Cereal Systems of Indo-Gangetic Plains. J. @ Integr. Agric. 14, 1524–1533. doi: 10.1016/S2095-3119(15)61093-0
Shahin S., Jafari A., Mobli H., Rafiee S., Karini M. (2008). Effect of Farm Size on Energy Ratio on Wheat Production: A Case Study From Arbadil Province of Iran. Am. Euras. J. Agric. Environ. Sci. 3, 604–608.
Shammi M., Sultana A., Hasan N., Mostafizur Rahman M., Saiful Islam M., Bodrud-Doza M., et al. (2018). Pesticide Exposures Towards Health and Environmental Hazard in Bangladesh: A Case Study on Farmers’ Perception. J. Saudi. Soc Ag. Sci. 37, 243–260. doi: 10.1007/s10669-017-9628-7
Sims B., Corsi S., Gbehounou G., Kienzle J., Taguchi M., Friedrich T. (2018). Sustainable Weed Management for Conservation Agriculture: Options for Smallholder Farmers. Agriculture 8, 118. doi: 10.3390/agriculture8080118
Singh S., Mittal J. P. (1992). Energy in Production Agriculture. New Delhi. India.: Mittal. Publ. New Delhi. India. p, 158.
Smith P., Powlson D., Glendining M., Smith J. O. (1997). Potential for Carbon Sequestration in European Soils: Preliminary Estimates for Five Scenarios Using Results From Long-Term Experiments. Global Change Biol. 3, 67–79. doi: 10.1046/j.1365-2486.1997.00055.x
Somasundaram J., Sinha N. K., Dalal R. C., Lal R., Mohanty M., Naorem A. K., et al. (2020). No-Till Farming and Conservation Agriculture in South Asia – Issues, Challenges, Prospects and Benefits, Crit. Rev. Plant Sci. 39, 236–279. doi: 10.1080/07352689.2020.1782069
SRDI (2014). Land and Soil Resources Utilization (in Begali) (Dhaka, Bangladesh: Soil Resources Development Institute).
Stehfest E., Bouwman L. (2006). N2O and NO Emission From Agricultural Fields and Soils Under Natural Vegetation: Summarizing Available Measurement Data and Modeling of Global Annual Emissions. Nutr. Cycl. Agroecosyst. 74, 207–228. doi: 10.1007/s10705-006-9000-7
Timsina J., Connor D. J. (2001). The Productivity and Management of Rice-Wheat Cropping Systems: Issues and Challenges. Field Crops Res. 69, 93–132. doi: 10.1016/S0378-4290(00)00143-X
Timsina J., Jat M. L., Majumdar K. (2010). Rice-Maize Systems of South Asia: Current Status, Future Prospects and Research Priorities for Nutrient Management. Plant Soil 335, 65–82. doi: 10.1007/s11104-010-0418-y
Timsina J., Wolf J., Guilpart N., van Bussel L., Grassini P., van Wart J., et al. (2018). Can Bangladesh Produce Enough Cereals to Meet Future Demand? Agric. Syst. 163, 36–44. doi: 10.1016/j.agsy.2016.11.003
Van Bruggen A. H. C., He M. M., Shin K., Mai V., Jeong K. C., Finckh M. R., et al. (2018). Environmental and Health Effects of the Herbicide Glyphosate. Sci. Total. Env. 616-617, 255–268. doi: 10.1016/j.scitotenv.2017.10.309
Vighi M., Matthies M., Solomon K. R. (2017). Critical Assessment of Pendimethalin in Terms of Persistence, Bioaccumulation, Toxicity, and Potential for Long-Range Transport. J. Toxicol. Environ. Health B. Crit. Rev. 20, 1–21. doi: 10.1080/10937404.2016.1222320
Ward P., Bell A., Droppelmann K., Benton T. (2017). Early Adoption of Conservation Agriculture Practices: Understanding Partial Compliance in Programs With Multiple Adoption Decisions. Land Use Policy 70. doi: 10.1016/j.landusepol.2017.10.001.
Woods J., Williams A., Hughes J. K., Black M., et al. (2010). Energy and the Food System. Phil. Trans. R. Soc 365, 2991–3006. doi: 10.1098/rstb.2010.0172
Yadav S. N., Chandra R., Khura T. K., Chuhan N. S. (2013). Energy Input–Output Analysis and Mechanization Status for Cultivation of Rice and Maize Crops in Sikkim. Agric. Eng. Int. CIGR J. 15, 108–116.
Keywords: energy productivity, energy-use efficiency, global warming potential, yield-scaled emissions, multi-criteria assessment, on-farm experiment
Citation: Krupnik TJ, Hossain MK, Timsina J, Gathala MK, Sapkota TB, Yasmin S, Shahjahan M, Hossain F, Kurishi A, Miah AA, Rahman BMS and McDonald AJ (2022) Adapted Conservation Agriculture Practices Can Increase Energy Productivity and Lower Yield-Scaled Greenhouse Gas Emissions in Coastal Bangladesh. Front. Agron. 4:829737. doi: 10.3389/fagro.2022.829737
Received: 06 December 2021; Accepted: 07 June 2022;
Published: 13 July 2022.
Edited by:
Stéphane Cordeau, INRA UMR1347 Agroécologie, FranceReviewed by:
Mina Devkota, International Center for Agricultural Research in the Dry Areas (ICARDA), MoroccoDinesh Panday, The University of Tennessee, Knoxville, United States
Nicolas Munier-Jolain, Institut National de recherche pour l’agriculture, l’alimentation et l’environnement (INRAE), France
Copyright © 2022 Krupnik, Hossain, Timsina, Gathala, Sapkota, Yasmin, Shahjahan, Hossain, Kurishi, Miah, Rahman and McDonald. This is an open-access article distributed under the terms of the Creative Commons Attribution License (CC BY). The use, distribution or reproduction in other forums is permitted, provided the original author(s) and the copyright owner(s) are credited and that the original publication in this journal is cited, in accordance with accepted academic practice. No use, distribution or reproduction is permitted which does not comply with these terms.
*Correspondence: Timothy J. Krupnik, t.krupnik@cgiar.org