- 1Laboratory of Biotechnology & Sustainable Development of Natural Resources, Polydisciplinary Faculty, Sultan Moulay Slimane University, Beni-Mellal, Morocco
- 2Unit of Biotechnology and Plant Physiology, Faculty of Sciences, Mohamed V University, Rabat, Morocco
- 3ERP Research Laboratory, Faculty of Natural and Life Sciences and Earth Sciences, University of Khemis Miliana, Khemis Miliana, Algeria
Low phosphorus (P) availability is a major limiting factor facing current agriculture in several agricultural areas. Many P fertilizers are applied to enhance P availability; however, the major part is likely to lose due to various processes related to P cycle. Silicon (Si) treatment and P-solubilizing bacteria inoculation have been emerged as a promising way to improve plant P nutrition. This study aimed to investigate the synergistic effects of Si treatment and Pseudomonas alkylphenolica PF9 strain inoculation on plant growth, P nutrition, acid phosphatase (APase) activity, oxidative stress markers, and antioxidant metabolism in the Moroccan alfalfa population Oued Lmalah (OL) under low P availability. Results revealed a significant reduction in dry biomass, plant height, leaf number, and area under low P conditions. P deficiency also altered P nutrition and chlorophyll (Chl) content. However, P-deficient alfalfa plants treated with Si or inoculated with PF9 strain displayed higher plant growth, Chl content, and remarkably the effect was much higher when Si was applied together with PF9 strain. Moreover, the simultaneous application of Si and PF9 strain to the P-deficient alfalfa plants improved APase activity and as a result, P contents in both the shoots and roots. Results also showed that the application of both the Si and PF9 counteracted the low P availability stress-induced oxidative damage by lowering the accumulation of reactive oxygen species (ROS), electrolyte leakage, and lipid peroxidation. This seems to be related to the ability of both the Si and PF9 strain to modulate both the enzymatic and non-enzymatic antioxidant molecules including superoxide dismutase activity, total polyphenols, flavonoids, and proline contents. Our findings suggest that the combined application of Si and P. alkylphenolica PF9 strain could be a promising way for improving growth of alfalfa under low-P availability.
Introduction
As a perennial forage legume, alfalfa [Medicago sativa L. (M. sativa)] is the most cultivated forage crop in Mediterranean area (Farissi et al., 2011, 2013; Bouizgaren et al., 2013). It has a great importance for agronomy because of its impressive nutritional value, biomass production, and atmospheric nitrogen (N2) fixation (Carlsson and Huss-Danell, 2003; Radovic et al., 2009). Furthermore, alfalfa's pivoting root system gives it the ability to absorb water by up to 3 m in depth (El-Ramady et al., 2020), as a result of adaptation to some extreme abiotic stresses such as drought and salinity. However, its growth is highly susceptible to soil nutrient deficiency and, especially, phosphorus (P) deficiency (Farssi et al., 2021).
Phosphorus is a vital macronutrient required for plant growth and development. It plays important roles in energy transfer, respiration, enzyme activation/inactivation, photosynthesis, and root elongation (Elhaissoufi et al., 2020). Moreover, P has also been known to play a vital role during biological nitrogen fixation (BNF) in legumes, since 20% of total plant P being allocated to nodules (Bargaz et al., 2021; Farssi et al., 2021). P also represents the main constituent of plants cell including nucleic acids, membrane phospholipids, etc. (Vance et al., 2003). However, although P concentration in most soils is typically high, the available P in the soil solution is less than 10 μM, which is below the plant requirements (Bargaz et al., 2021). Thus, in the most agricultural soils, farmers use chemical P fertilizers to increase the available P levels (Ekardt, 2016). However, the major parts of the applied P are likely to lose due to various biochemical processes related to P cycle including sorption, fixation, and immobilization (Penn and Camberato, 2019). On the another hand, at high concentration, P may cause significant environmental risk from leaching, runoff, and erosion (Bargaz et al., 2021). Therefore, there is a need for developing strategies that can improve P use efficiency by plants without having any risks on the environment.
The use of plant growth-promoting rhizobacteria (PGPR) including P-solubilizing bacteria (PSB) has been shown to improve P use efficiency by plants (Suleman et al., 2018; Bargaz et al., 2021; Fatima et al., 2021). PSB are a large group of soil microorganisms that have the ability to mobilize directly or indirectly P from both the organic and inorganic sources and make it available for plant use. Among the PSB, Pseudomonas alkylphenolica (P. alkylphenolica) has been recently considered as one of the most effective PSB, which continues to be a key research priority (Farssi et al., 2021). Thus, when P-stressed alfalfa plants were inoculated with P. alkylphenolica PF9 strain, they exhibited higher biomass production, photosynthesis activity, and P content (Farssi et al., 2021). Another interesting study conducted by Shi et al. (2019) showed that P. alkylphenolica strain was able to remove mercury (Hg) in the solution, immobilize Hg in soil, promote growth, decrease Hg accumulation, and improve photosynthesis of Brassica campestris exposed to Hg stress.
Silicon (Si), second most important element in terms of abundance in the earth crust, has been considered as a beneficial element that promotes plants tolerance to the various biotic and abiotic stresses including P deficiency (El Moukhtari et al., 2021b; Etesami et al., 2021; Pavlovic et al., 2021). According to Greger et al. (2018), Si supplementation could increase the soil-P availability by up to 50%. Thus, Si-mediated P deficiency tolerance in Solanum lycopersicum is associated with increased photosynthesis, membrane stability, osmoregulation, and nutrient use efficiency including P (Zhang et al., 2019). Another interesting study indicated that Si supply to the soil solution of Triticum aestivum (T. aestivum) plants improved nutrient use efficiency and changed C:N:P stoichiometry (Neu et al., 2017). Decrease in P sorption in soil (Owino-Gerroh and Gascho, 2005), increasing root exudation of some organic acids such as malate and citrate (Kostic et al., 2017), increasing soil P availability by augmenting soil pH (Owino-Gerroh and Gascho, 2005) and upregulating some plant genes involved in P uptake (Kostic et al., 2017) are all evoked by Si to improve soil-P availability and, consequently, its uptake by plants, particularly during P limitation.
The interactive effects of PGPR and Si has been reported on Vigna radiata and Zea mays (Z. mays) under salt stress (Mahmood et al., 2016; Kubi et al., 2021), Coriandrum sativum (C. sativum) under lead (Pb) (Fatemi et al., 2020), and T. aestivum and Sorghum bicolor (S. bicolor) under P deficiency (Rezakhani et al., 2019, 2020), which continue to be a key research priority. However, to date, there is no study on the interactive effects of P. alkylphenolica and Si on alfalfa tolerance to P deficiency. Therefore, the aim of this study was to assess the effect of individual and combined use of Si and P. alkylphenolica PF9 strain on growth, photosynthesis, membrane stability, antioxidant responses, and P nutrition of alfalfa under low P availability. We found that Si treatment and P. alkylphenolica PF9 strain inoculation were effective in alleviating low P availability on alfalfa plants and that the application of both the Si and P. alkylphenolica PF9 strain together had a greater effect than their single application. These findings could provide a new approach for improving growth and productivity of alfalfa when grown under low P availability.
Materials and Methods
Experimental Design
To study the individual and combined application of exogenous silicon (Si) and Pseudomonas alkylphenolica PF9 strain on the tolerance of Moroccan alfalfa (Medicago sativa L.) population to low P availability, a 2 × 2 × 2 factorial experiment in a completely randomized design was laid out in sterilized perlite in five replications was conducted. Sterilized perlite was placed in the experimental plastic pots (10 cm diameter and 15 cm tall). The research was carried out in a growth chamber at the Polydisciplinary Faculty of Beni Mellal (Sultan Moulay Slimane University, Morocco) using the Oued Lmalah (OL) population. The OL population was chosen according to our previous study (Farssi et al., 2021).
The experimental treatments included: (i) P factor at two levels, plants fertilized with soluble P (250 μmol P in the form of KH2PO4) and the plants fertilized with insoluble P (250 μmol P in the form of Ca3HPO4); (ii) Si factor at two levels, plants non-treated with Si (0 Mm CaSiO3) and plants treated with Si (3 mM CaSiO3); and (iii) PF9 strain factor at two levels, plants non-inoculated with PF9 strain and plants inoculated with PF9 strain. A total of 8 different treatments (2 × 2 × 2) were obtained from combination of different factors mentioned. A total of 40 pots (8 × 5) were used in this experiment. The concentrations of Si and P were chosen according to our previous studies (El Moukhtari et al., 2021a; Farssi et al., 2021).
Bacterial Strain
The strain used as inoculum was isolated from the nodules of M. sativa plants grown in the Beni-Mellal region in Morocco and identified as P. alkylphenolica strain PF9 using the housekeeping gene rpoD with the accession number of KY950274.1 (Farssi et al., 2021). The strain was evaluated in vitro for its P-solubilizing activity (92.42 ± 0.32 μg P ml−1) and acid phosphatase (APase) production (43.81 ± 0.67 μmol pNPP ml−1 min−1) following the methods described previously (Araújo et al., 2008; Elhaissoufi et al., 2020).
Plant Growth Conditions and Treatments
Sterilized seeds, which were immersed in sodium hypochlorite (6%) for 5 min and rinsed thoroughly with sterilized distilled water, were allocated to germinate in pots with 16 h photoperiod (75–80 μmol photons s−1 m−2) at 25 ± 1°C and 60–80% relative humidity. After emergence, 5 seedlings with uniform length were maintained per pot. After 2 weeks of sowing, pots were split into 2 plots: (i) plants watered with Hoagland nutrient solution containing soluble P form (KH2PO4) (control) and (ii) plants watered with Hoagland nutrient solution containing insoluble P form (Ca3HPO4) (stressed).
The composition of the nutrient solution (Hoagland and Arnon, 1950) used was as follows: [KNO3 (600 μmol), MgSO4 (1,000 μmol), K2SO4 (750 μmol), CaCl2 (1,650 μmol), Fe-ethylenediaminetetraacetic acid (EDTA) (16 μmol), MnSO4 (6 μmol), H3BO3 (4 μmol), ZnSO4 (1 μmol), NaMoO4 (0.1 μmol), and CuSO4 (1 μmol)]. By using 0.1 M HCl or 0.1 M NaOH, the pH of the nutrient solution was, respectively, reduced or raised to reach 7 before use. Stress was applied for 1 month and then some parameters related to growth, photosynthesis, membrane stability, oxidative stress, antioxidant metabolism, and nutrient contents were assessed.
Plant Growth Attributes Determination
Just before the harvest, plant height (PH) and number of leaves were determined in three random plants from each treatment. At harvest, the shoots were cut off from the roots, rinsed three times with deionized water, and placed in a ventilated oven at 80°C. The root parts were separated from the soil by handpicking, washed thoroughly with deionized water, and dried afterward at 80°C for 48 h. Shoots and roots dry weights were determined in three random plants from each treatment.
Leaf area (LA) was determined on three leaves of three random plants from each treatment as described previously (Farssi et al., 2021). For this purpose, leaves were cut and laid out on a white sheet containing a scale and then scanned using a digital scanner. The images were then analyzed using Mesurum software version 3.4.4.0.
Total Chlorophyll (Chl) Content
Total Chl was determined according to Arnon (1949). 100 mg of fresh leaf were homogenized in a mortar using diluted acetone (80%). The resulted homogenate was centrifuged for 10 min at 10,000 xg and the obtained supernatant was used to detect the absorbance at 645 and 663 nm using a SP-UV1000 Spectrophotometer. The concentration of total Chl was calculated using formula below:
Acid Phosphatase Activity Assessment
Acid phosphatase activity was determined in alfalfa roots for three random plants per treatment using the method of Araújo et al. (2008) with slight modification. Fresh root samples (100 mg) were homogenized in 1 ml of sodium acetate buffer (0.1 M, pH 5.6) containing 2.2% polyvinylpyrrolidone (PVP). After 15 min of centrifugation at 12,000 × g at 4°C, 125 μl of the obtained supernatant was added to 500 μl of sodium acetate buffer and 125 μl of 10 mM p-nitrophenyl phosphate (pNPP) and the mixture was incubated for 1 h at 37°C. Reaction was stopped by adding 125 μl of 0.5 M CaCl2 and 500 μl of 0.5 M NaOH. The optical density (OD) was recorded at 405 nm. APase activity was defined as the amount of protein hydrolyzing 1 mol of p-NPP min−1 g−1 protein and the results are expressed as the mean of three replicates per treatment.
Oxidative Stress Markers and Histochemical Staining of Hydrogen Peroxide (H2O2) and Superoxide ()
Malondialdehyde (MDA) was determined in the second fully developed leaves following the method described by Heath and Packer (1968) in three replicates per treatment. 100 mg of frozen fresh leaf was homogenized in 1 ml of 0.5% thiobarbituric acid (TBA) prepared in 20% trichloroacetic acid (TCA). The homogenate was heated at 95°C for 30 min and after cooling down, samples were centrifuged at 4°C for 10 min at 10,000 × g. Supernatant was recuperated and its OD was measured at 532 and 600 nm. MDA concentration was determined using its extinction coefficient of 155 mM−1 cm−1 and expressed as μmol MDA g−1 FW.
According to Ghoulam et al. (2002), electrolyte leakage (EL) was determined by comparing the electrical conductivity (EC) before and after autoclaving the sample. For this purpose, fresh leaf samples were rinsed thoroughly with deionized water to remove surface minerals and placed in polyethylene tubes containing 10 ml of deionized water. Samples were then shaken for 24 h at 25°C and their initial EC1 was determined at 25°C using a DDS-12DW, Benchtop Conductivity Meter. Samples were then autoclaved for 20 min at 120°C and after cooling down, their final EC2 was carried out at 25°C. The EL was determined in three replicates per treatment using below formula:
Histochemical staining of H2O2 in alfalfa leaves was determined according to Kumar et al. (2014) using 3,3′-diaminobenzidine (DAB) method. Well-developed leaves from different treatments were immersed in DAB solution (1 mg ml−1) prepared in water (pH 3.8). After an overnight shaken at 200 rpm, DAB solution was drained off and the Chl was removed by immersing the leaves in absolute ethanol and heating. Samples were transferred on a paper towel saturated with 60% glycerol and photographed using a digital camera.
For histochemical detection of , nitroblue tetrazolium (NBT) staining method was adopted (Kumar et al., 2014). Well-developed alfalfa leaf samples were immersed in 50 mM sodium phosphate buffer (pH 7.5) containing 0.2% (W/V) NBT and shaken for an overnight. For proper visualization of the stain, Chl was removed by immersing the leaves in absolute ethanol and heated. Leaves were transferred on a paper towel saturated with 60% glycerol and photographed using a digital camera.
Determination of Non-enzymatic Antioxidant Contents
For total polyphenols, the Folin–Ciocalteu (FC) method was adopted (Skotti et al., 2014). 100 mg of fresh plant material were homogenized in 1 ml of 80% methanol at 4°C. The obtained homogenate was centrifuged at 4°C for 20 min at 12,000 × g and the supernatant was used to determine total polyphenols content. For this purpose, 250 μl of FC reagent was added to 50 μl of supernatant and the volume was then adjusted to 5 ml with distilled water. The mixture was incubated at room temperature for 3 min and then 1.5 ml of Na2CO3 (20%) was added. The OD was recorded at 725 nm after 1 h of incubation in the dark and the total polyphenols content was calculated using calibration curves established with gallic acid. The results were expressed as milligrams of gallic acid equivalent per g−1 FW. For each treatment, three replicates were considered.
Flavonoids content were determined in the same extract used in total polyphenols following the method described in El Moukhtari et al. (2021a), which was adopted from Chang et al. (2002). To 100 μl of supernatant, 300 μl of methanol (95%), 20 μl of 10% aluminum chloride, 20 μl of potassium acetate (1 M), and 560 μl of distilled water were added. The mixture was incubated at room temperature for 30 min and then the OD was determined at 415 nm. Flavonoids content was estimated using calibration curves established with known concentration of quercetin.
Proline content was estimated according to Bates et al. (1973). Fresh leaf material (100 mg) was ground in 3 ml of aqueous sulfosalicylic acid (3% w/v) in prechilled pestle and mortar under cold condition and then centrifuged at 14,000 × g for 10 min at 4°C. To 400 μl of supernatant, equal volume of ninhydrin (3%) and glacial acetic acid were added. The mixture was vortexed and incubated in a boiling water bath for 1 h. After cooling down, 2 ml of toluene was added and the OD of the pink phase was measured at 520 nm. Proline content was determined using calibration curves prepared with known L-proline concentration and expressed as μmol proline g−1 FW. The results are expressed as a mean of three replicates per treatment.
Superoxide Dismutase (SOD) Activity Determination
Fresh leaves (0.5 g) were ground at 4°C in 5 ml of 50 mM phosphate buffer (pH 7.8) containing 0.1 mM EDTA and 1% PVP. The homogenate was centrifuged for 20 min at 20,000 × g at 4°C and the supernatant was used for SOD (EC 1.15.1.1) assay. SOD activity was assayed according to the method described by Beyer and Fridovich (1987). 30 μl of enzyme extract was added to 1 ml of 50 mM phosphate buffer (pH 7.8) containing NBT (65 μM), L-methionine (13.3 μM), and riboflavin (1.33 μM). The mixture was exposed to a fluorescence lamp for 5 min and then the OD was measured at 560 nm. One enzymatic unit (EU) of SOD was defined as the amount required for the inhibition of 50% NBT. The activity was expressed as EU min−1 mg−1 protein.
P and Si Contents
For P content, 0.5 g of dry material (shoot or root) was incinerated for 6 h at 600°C. Ashes were recovered in 3 ml HCl (10 N) and the volume was adjusted to 50 ml using deionized water. After filtration, P content was determined using the sodium molybdate method (Farssi et al., 2021; Oukaltouma et al., 2021). Thus, to 1 ml of the obtained solution, 4 ml of distilled water and 5 ml of a mixture of 2.5% (w/v) sodium molybdate and 0.15% (w/v) hydrazine sulfate were added and the mixture was heated in a water bath (95°C) for 10 min. Samples were then left to cool and the OD was determined at 825 nm. P concentration was calculated based on a standard curve established with KH2PO4 solutions and the results were expressed as a mean of three independent replicates.
For Si, dry plant material was incinerated at 600°C for 6 h and the ashes formed was recovered in a mixture of concentrated HCl/nitric oxide (2/1, v/v) and heated for 1 h at 200°C. After filtration, the solution was adjusted with distilled water to 100 ml. Si concentration was determined using inductively coupled plasma optical emission spectroscopy (Optima 8000 ICP-OES) as described in our previous study (El Moukhtari et al., 2021a).
Data Analysis
Statistical analysis was performed using SPSS software version 22. It concerned a ANOVA III, where phosphorus, silicon, and the PF9 strain were the independent variables. Means were compared using Tukey's test. The difference was considered significant at P ≤ 0.05 and indicated by different letters.
Results
Effect of Si Treatment and PF9 Strain Inoculation on Plant Growth Attributes
P deficiency conditions reduced shoot dry weight (SDW) of alfalfa plants by 18% as compared to nonstressed plants (Table 1). However, under low P conditions, treatment of alfalfa plants with Si, their inoculation with BF9 strain, or their combination significantly (p <0.001; F = 47.04) increased SDW whatever the form of P with the highest increment rate observed for plants inoculated with PF9 strain alone. Indeed, inoculation with the PF9 strain increased the SDW by 135 and 189% in comparison to the uninoculated plants grown, respectively, under nonstressed or stressed conditions.
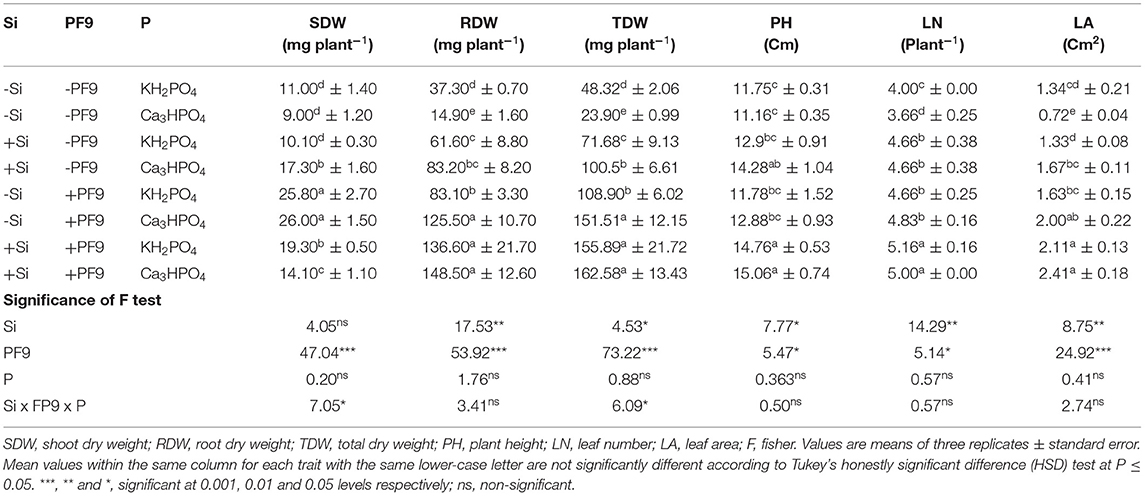
Table 1. Effect of 3 mM Si treatment, PF9 strain inoculation, and their combination on SDW, RDW, TDW, PH, LN, and LA of Medicago sativa (OL population) plants grown under 250 μmol of soluble (KH2PO4) or insoluble (Ca3HPO4) phosphorus conditions.
Low P availability significantly reduced root dry weight (RDW) by 60% as compared to the non-stressed plants (Table 1). However, Si application or PF9 strain inoculation significantly (p <0.001; F = 53.92) improved RDW and the effect was interestingly more obvious under the simultaneous application of Si and PF9 strain in comparison to untreated stressed plants. Indeed, the recorded RDW in P-deficient alfalfa plants was 9.96-fold higher under the combined application of Si and PF9 strain in comparison to the untreated and stressed plants. Under P-sufficient conditions, as compared to control, the single application of either Si or PF9 strain significantly (p <0.001) increased the RDW and the increase was much furthered under their combined application.
Results given in Table 1 shows that the exposure of alfalfa plants to low P availability significantly (p <0.001) reduced total dry weight (TDW) by 51% as compared to control. However, Si treatment or PF9 strain inoculation led to a significant increase (p ≤ 0.001) in TDW and interestingly the effect was further increased under their combined application. Under P-deficient conditions, TDW of alfalfa plants treated with Si and inoculated with PF9 strain was 6.8-fold higher as compared to the untreated plants. Under P-sufficient conditions, Si treatment or PF9 strain inoculation significantly (p <0.001) increased TDW and the increase was much furthered under their combined application.
For leaf number (LN), PH, and LA, the obtained results (Table 1) showed that P deficiency reduced them by 9, 5, and 46%, respectively, relative to control. However, Si treatment or PF9 strain inoculation significantly improved these parameters in P-deficient alfalfa plants and the positive impact was especially much higher when alfalfa plants were treated with 3 mM Si and inoculated with PF9 strain. Indeed, as compared to the untreated P-deficient alfalfa plants, the simultaneous application of Si and PF9 strain improved LN, PH, and LA by 37, 35, and 235%, respectively. Under P-sufficient conditions, Si treatment or PF9 strain inoculation significantly (p <0.05; F = 5.47) increased LN, PH, and LA and the increase was much important under the combined application of both the treatments.
Effect of Si Treatment and PF9 Strain Inoculation on Total Chl
Results illustrated in Figure 1 indicates that total Chl was significantly (p <0.001; F = 19.37) reduced under P-deficient conditions. However, Si treatment, PF9 strain inoculation, or their simultaneous application significantly increased the total Chl content with no significant difference between the applied treatments as compared to the untreated stressed alfalfa plants. Under normal conditions, Si applied alone or in combination with PF9 strain increased significantly total Chl of alfalfa plants by 20 and 17%, respectively, as compared to the untreated control plants.
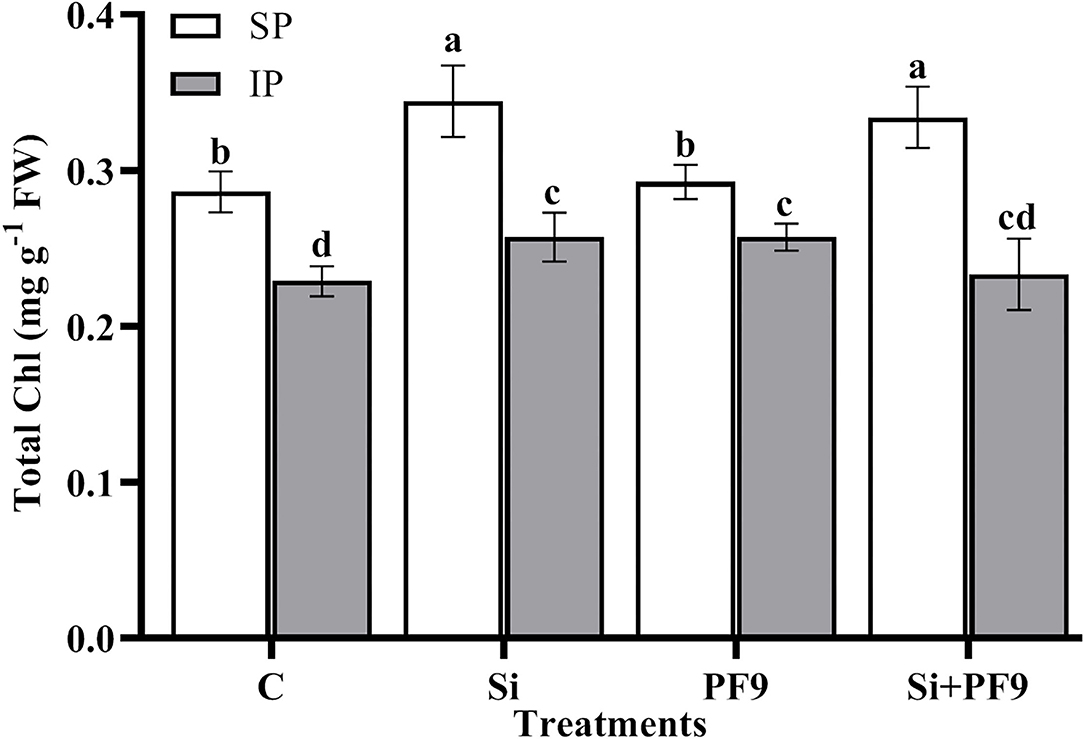
Figure 1. Effect of 3 mM Si treatment and PF9 strain inoculation on total chlorophyll (Chl) of Medicago sativa (OL population) plants grown under 250 μmol of either soluble (KH2PO4) (SP) or insoluble (Ca3HPO4) (IP) phosphorus conditions. Values are means of three replicates and error bars are SD. Different letters indicate significant difference at p <0.05.
Effect of Si Treatment and PF9 Strain Inoculation on Shoot and Root P Content and APase Activity
For the shoot and root P content (Table 2), the results indicated that the treatment of alfalfa plants with insoluble P form reduced shoot and root P content by 39 and 86%, respectively, when compared to those treated with soluble P form. However, Si incorporation to the growth medium of P-deficient alfalfa plants or their inoculation with the PF9 strain significantly (p <0.001; F = 109.5) improved the P content and the effect was much higher under the combined treatment. Indeed, under P-deficient conditions, the highest amount of P in both the shoot (3.68 mg P g−1 DW) and root (3.59 mg P g−1 DW) was observed in alfalfa plants treated with 3 mM Si and inoculated with PF9 strain. The simultaneous application of Si and PF9 strain under normal conditions increased significantly shoot P content.
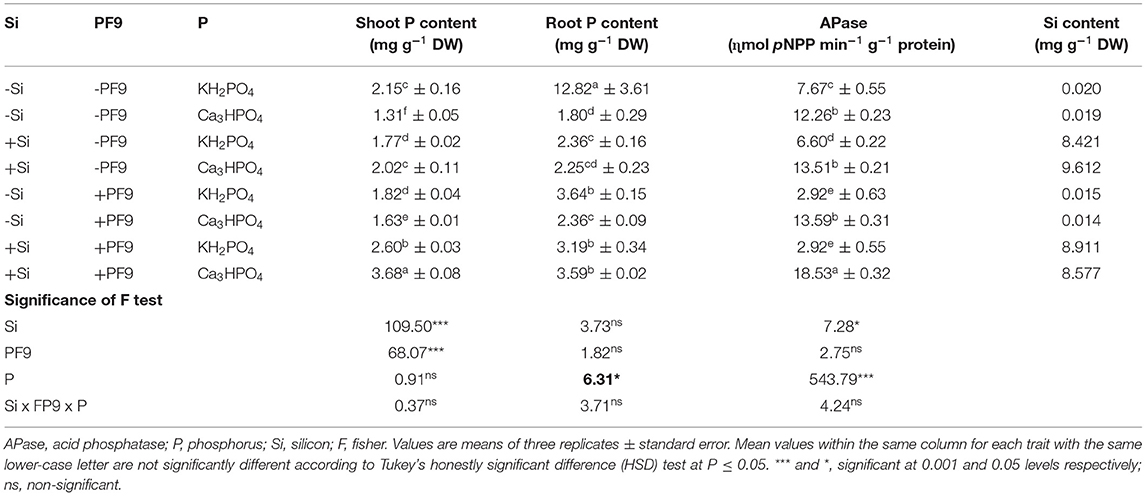
Table 2. Effect of 3 mM Si treatment, PF9 strain inoculation, and their combination on shoot and root P content, APase activity, and Si content of Medicago sativa (OL population) plants grown under 250 μmol of soluble (KH2PO4) or insoluble (Ca3HPO4) phosphorus conditions.
Acid phosphatase activity in the root of alfalfa plants grown under either soluble or insoluble P form and treated with Si and/or inoculated with the PF9 strain is shown in Table 2. The obtained results indicated that APase activity in the root of alfalfa plants was increased significantly (p <0.001; F = 543.8) upon P deficiency and, interestingly, this activity was much more elevated when P-deficient alfalfa plants were simultaneously treated with Si and inoculated with the PF9 strain. Under normal conditions, the separate or combined application of Si and PF9 strain reduced significantly (p <0.001; F = 72.7) APase activity as compared to the untreated control.
Effect of Si Treatment and PF9 Strain Inoculation on Si Content
Results related to Si content revealed that in the absence of Si treatment, the Si contents were low and similar in plants grown under either the sufficient or deficient-P conditions (Table 2). However, Si treatment alone or in combination with PF9 strain increased the Si content in alfalfa plants whatever the applied P form reflecting the Si uptake by alfalfa roots. The inoculation of the Si-untreated alfalfa plants with PF9 strain has no significant effect on Si content of both the P-stressed and P-unstressed plants.
Effect of Si Treatment and PF9 Strain Inoculation on Oxidative Stress Markers
The effect of the applied treatments on MDA and EL under low P availability is given in Figure 2. Results indicated that MDA and EL were found higher in the untreated and stressed alfalfa plants. Indeed, the substitution of the soluble P form by an insoluble form elevated MDA and EL by 140 and 108%, respectively. However, this effect seemed to be alleviated by Si and/or PF9 strain inoculation. Indeed, P-deficient alfalfa plants treated with Si and inoculated with PF9 strain showed less MDA content (18.35 μmol MDA g−1 FW) and EL (14.91%) as compared to the untreated P-deficient plants (34.78 μmol g−1 FW and 19.62% for MDA and EL, respectively). Under P-sufficient conditions, the applied treatments have no significant effect on EL (p > 0.05).
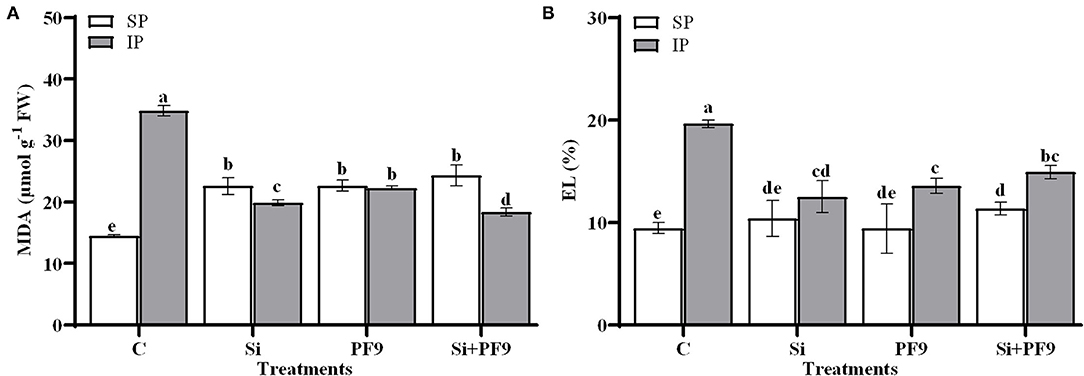
Figure 2. Effect of 3 mM Si treatment and PF9 strain inoculation on malondialdehyde (MDA) (A) and electrolyte leakage (EL) (B) of Medicago sativa (OL population) plants grown under 250 μmol of either soluble (KH2PO4) (SP) or insoluble (Ca3HPO4) (IP) phosphorus conditions. Values are means of three replicates and error bars are SD. Different letters indicate significant difference at p <0.05.
The effect of the applied Treatments on the visual distribution of H2O2 and under soluble or insoluble P forms is shown in Figure 3. The obtained results indicated that H2O2 and accumulated in alfalfa leaves upon P stress. However, although the staining intensity was not quantified, H2O2 and appeared lower in leaves from plants treated with either Si, PF9 strain, or their combination. Under P-sufficient conditions, the applied treatments had no effect on H2O2 and accumulation.
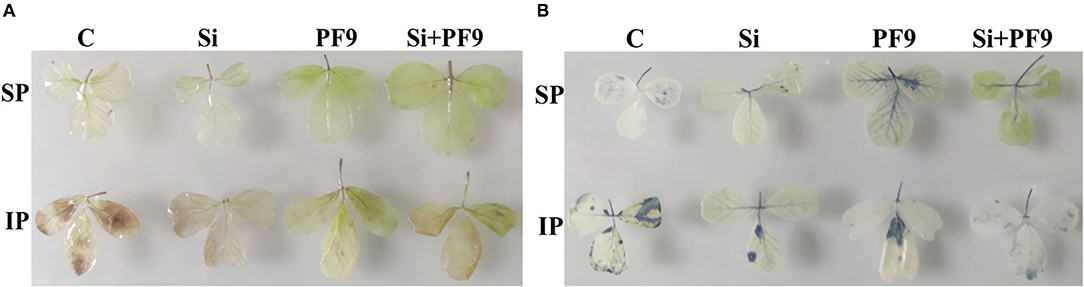
Figure 3. Effect of 3 mM Si treatment and PF9 strain inoculation on hydrogen peroxide (H2O2) (A) and peroxide () (B) accumulation in the leaves of Medicago sativa (OL population) plants grown under 250 μmol of either soluble (KH2PO4) (SP) or insoluble (Ca3HPO4) (IP) phosphorus conditions. H2O2 and accumulation in the leaves were detected using 3,3′-diaminobenzidine and nitroblue tetrazolium methods, respectively.
Effect of Si Treatment and PF9 Strain Inoculation on the Antioxidant System
The effect of P, Si, and PF9 strain on the non-enzymatic antioxidant contents is shown in Table 3. The results revealed that the application of P in the form of Ca3HPO4 significantly increased (p <0.001; F = 481.9) total polyphenols (135%) and flavonoids (48%) contents with no effect on proline content as compared to the control (KH2PO4). Furthermore, Si treatment or PF9 strain inoculation induced more increase of total polyphenols, flavonoids, and proline contents and, interestingly, this increase was much furthered when Si and PF9 strain were applied together (p <0.01; F = 12.6). Indeed, as compared to the untreated P-deficient plants, the combined application of Si and PF9 strain increased total polyphenols, flavonoids, and proline contents by 49, 19, and 136%, respectively. Under P-sufficient conditions, the combined application of Si and PF9 strain increased significantly (p <0.001) proline content.
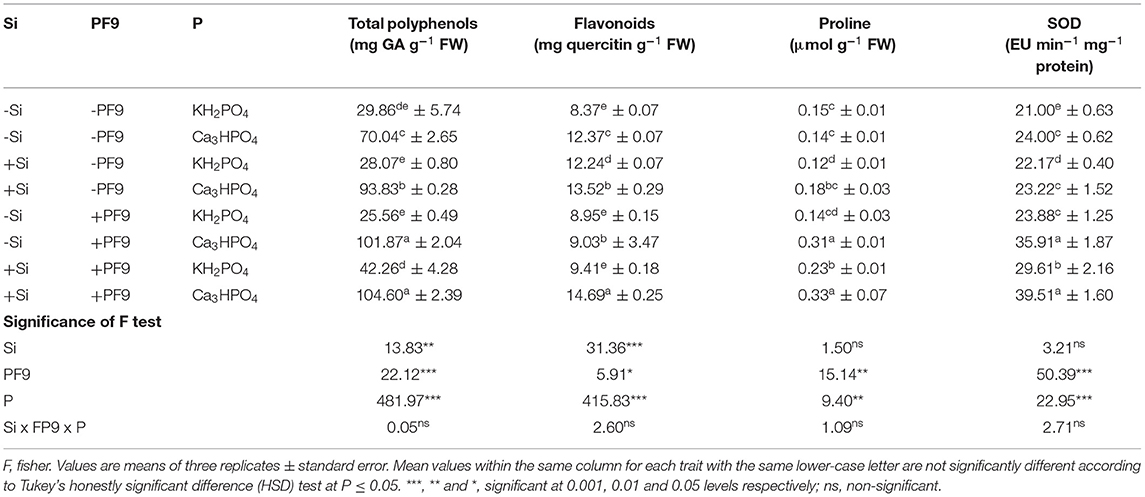
Table 3. Effect of 3 mM Si treatment, PF9 strain inoculation, and their combination on the enzymatic and non-enzymatic antioxidant activities of Medicago sativa (OL population) plants grown under 250 μmol of the soluble (KH2PO4) or insoluble (Ca3HPO4) phosphorus conditions.
The activity of the enzymatic antioxidant SOD was found higher in the untreated P-stressed plants (24 EU min−1 mg−1 proteins) as compared to the control (21 EU min−1 mg−1 proteins) (Table 3). Furthermore, the inoculation with PF9 strain caused an additional increase in the SOD activity (35.91 EU min−1 mg−1 proteins) and, interestingly, this activity was much furthered (39.51 EU min−1 mg−1 proteins) when the inoculated P-deficient alfalfa plants were treated with 3 mM Si. Under P-sufficient conditions, the co-application of Si and PF9 strain caused an additional increase in the activity of SOD.
Discussion
Phosphorus has been reported as one of the most important nutrient, which directly or indirectly involved in several physiological and biochemical processes during plant growth. Although P is abundant in soils, its availability is vastly restricted and only 0.1% of the total P is available to be directly used by plants because of poor solubility and its fixation in soil (Sharma et al., 2013). Under P-limiting conditions, plants are generally characterized by low biomass production, short plants height, less LN, and small LA (Malhotra et al., 2018). In this study, exposure to P deficiency impaired alfalfa plants growth. Indeed, the substitution of the soluble P form with an insoluble form decreased SDW, RDW, TDW, LN, PH, and LA by 18, 60, 51, 9, 5, and 46%, respectively, compared to the control. However, Si treatment or PF9 strain inoculation significantly increased shoot, root, and TDW of alfalfa plants under P deficiency and the effect was more obvious when both the treatments were applied together. Additionally, the simultaneous application of Si and PF9 strain increased LN, PH, and LA by 37, 35, and 235%, respectively, as compared to the untreated P-deficient alfalfa plants. Our results corroborate with the findings of Rezakhani et al. (2020), where the application of Si and the inoculation with Pseudomonas spp. FA1 significantly improved S. bicolor SDW and RDW under P deficiency. Similarly, under salt stress, Si treatment and PGPR inoculation improved weight, length, and diameter of both the shoot and root of Z. mays (Kubi et al., 2021). Fatemi et al. (2020) also reported that the combined application of Si and PGPR alleviated the negative impact of Pb on plant biomass and height, root length, and LA of C. sativum.
Under P deficiency, low biomass accumulation could be the result of photosynthesis reduction (Wissuwa et al., 2005). In this study, P deficiency drastically reduced total Chl (up to 22%) as compared to control. However, either Si supplementation or PF9 inoculation significantly improved Chl content of P-deficient alfalfa plants and, interestingly, the effect was more pronounced when Si and PF9 strain were used together. Indeed, total Chl in P-deficient alfalfa plants treated with Si and inoculated with PF9 strain was 21% higher when compared to those grown under P deficiency without Si treatment or PF9 strain inoculation. The reduction in Chl content under P deficiency could be explained by the low P content in the shoots (Xu et al., 2007). However, this was alleviated by Si supplementation (Zhang et al., 2019) or PSB inoculation (Farssi et al., 2021). Moreover, under salt, drought, or heavy metals stress, investigations indicated that the effects of Si supplementation and bacterial inoculation on Chl content were more obvious when both the treatments were applied together as compared to their single application (Fatemi et al., 2020; Akhtar et al., 2021; Kubi et al., 2021). Additionally, the increased total Chl content under P deficiency in response to Si treatment and PF9 strain inoculation might be the reason of increased plant growth, since the positive correlation observed between total Chl and plant growth parameters.
This study indicated that the treatment of alfalfa plants with an insoluble P form drastically reduced P content in both the shoots (39%) and roots (86%) of alfalfa plants relative to those received soluble P form. However, as suggested by Fuentes et al. (2014) and Billah et al. (2019), Si incorporation to the growth medium of P-deficient alfalfa plants or their inoculation with PF9 strain significantly improved APase activity, which consequently improved P acquisition and the improvements for both the shoots (plus 180%) and roots (plus 99%) were more pronounced when the alfalfa plants were simultaneously treated with Si and inoculated with PF9. Similar results have been found in T. aestivum under P starvation (Rezakhani et al., 2019). Here, the authors demonstrated that the simultaneous application of Si and PGPR had a synergistic effect on plant P acquisition in comparison to their individual application. The same was observed by Rezakhani et al. (2020) in P-deficient S. bicolor. Moreover, when grown under water stress, Z. mays plants treated with Si and inoculated with PGPR showed higher dehydrogenase and alkaline phosphatase and consequently higher P content as compared to the untreated stressed plants (Hafez et al., 2021). Previous investigations found that legumes growth was positively correlated with the P concentration in the soil solution and the reduction of P uptake under low P availability could cause a depressive effect on plant growth and photosynthesis (Sulieman and Hago, 2009). In this study, the reduction in P uptake under P deficiency drastically reduced plant growth. However, Si treatment and PF9 strain inoculation increased P acquisition that led to massive physiological changes as manifested by high Chl content and, consequently, alfalfa plants growth in terms of dry biomass, PH, LN, and area.
Phosphorus is an essential constituent of the fatty acid and phospholipid composing the cell membranes of plants (Qari and Oves, 2020). Thus, any P deficiency could cause an over synthesis of ROS resulting in an oxidative stress devastating for cell membrane integrity (Bargaz et al., 2013; Dokwal et al., 2021). In this study, alfalfa plants received insoluble P exhibited dramatic accumulation of H2O2 and as revealed by in-situ visualization. Moreover, the accumulation of both the H2O2 and under P deficiency caused membrane damage, which was evident from the increased MDA content and EL percentages. However, Si treatment or PF9 strain inoculation resulted in a significant decrease in MDA and EL and the decrease was much higher (47 and 24% for MDA and EL, respectively) when P-deficient alfalfa plants were treated with Si and inoculated with the PF9 strain. The reduced oxidative stress in response to Si treatment or PGPR inoculation was appeared to be related to the induction of both the enzymatic and non-enzymatic antioxidants (Israr et al., 2016; Zhang et al., 2019; Silva and Prado, 2021). In this study, Si treatment or PF9 strain inoculation modulated the activity of SOD together with the induction of a significant increase in the content of the non-enzymatic antioxidant including total polyphenols, flavonoids, and proline as compared to the untreated P-deficient alfalfa plants. Additionally, the comparison between the applied treatments revealed that the effect was more pronounced under the simultaneous application of Si and PF9 strain as compared to their individual supply. Similar results have been found in T. aestivum under P deficiency (Rezakhani et al., 2019). The authors demonstrated that under both the soluble and insoluble P fertilization conditions, Si treatment or PGPR inoculation increased the antioxidant activities and the effects were more obvious in plants simultaneously treated with Si and inoculated with PGPR. The same has been reported in Z. mays under salt stress (Kubi et al., 2021), in T. aestivum under drought (Akhtar et al., 2021), and in C. sativum under heavy metals (Fatemi et al., 2020).
Conclusion
The outcome of this study revealed that both the Si treatment and PF9 strain inoculation were able to attenuate P deficiency in alfalfa plants. The PF9 strain was more effective for most of the parameters focused, whereas its combination with Si was more effective than their single applications in alleviating low P availability in alfalfa. Indeed, used together, Si and PF9 strain alleviated the negative effects of P deficiency by improving plant biomass, PH, LN, and area. They were also effective in improving Chl and P contents in P-deficient alfalfa plants. Moreover, P-deficient alfalfa plants treated with Si and inoculated with PF9 strain displayed higher membrane stability as reflected by lower MDA content and less electrolyte leakage. This was seemed to be related to the ability of Si and PF9 strain to reduce H2O2 and contents by stimulating the activity of both the enzymatic and non-enzymatic antioxidant molecules. Our findings strongly suggest and recommend the combined use of Si and PSB tested in agricultural as sustainable strategy to alleviate low P availability effects on alfalfa growth.
Data Availability Statement
The original contributions presented in the study are included in the article/supplementary material, further inquiries can be directed to the corresponding author/s.
Author Contributions
MF, ML, QL, and ZT: methodology. MF and QL: supervision. AE, IE, NL, OF, and AO: investigation. AE, NL, and OF: writing-original draft. AO: statistical analysis. OF and MF: reviewing draft article. MF and ML: funding acquisition. All authors contributed to the article and approved the submitted version.
Funding
This study was supported by the CROSYMED Project, funded through the ARIMNet2 2017 Joint Call by the funding agency MESRSFC, Morocco. The ARIMNet2 (ERA-NET) has received funding from the European Union's Seventh Framework Program for Research, Technological Development and Demonstration under grant agreement no. 618127.
Conflict of Interest
The authors declare that the research was conducted in the absence of any commercial or financial relationships that could be construed as a potential conflict of interest.
Publisher's Note
All claims expressed in this article are solely those of the authors and do not necessarily represent those of their affiliated organizations, or those of the publisher, the editors and the reviewers. Any product that may be evaluated in this article, or claim that may be made by its manufacturer, is not guaranteed or endorsed by the publisher.
Acknowledgments
The authors are grateful to all those who participated in the elaboration of this study. We thank all the partners involved in CROSYMED-ARIMNet2 Project, administrative and technical staff of the Polydisciplinary Faculty of Beni-Mellal, Sultan Moulay Slimane University and the staff of MESRSFC agency, and ARIMNet2 program for their support.
References
Akhtar, N., Ilyas, N., Mashwani, Z. R., Hayat, R., Yasmin, H., Noureldeen, A., et al. (2021). Synergistic effects of plant growth promoting rhizobacteria and silicon dioxide nano-particles for amelioration of drought stress in wheat. Plant Physiol. Biochem. 166, 160–176. doi: 10.1016/j.plaphy.2021.05.039
Araújo, A. P., Plassard, C., and Drevon, J. J. (2008). Phosphatase and phytase activities in nodules of common bean genotypes at different levels of phosphorus supply. Plant Soil. 312, 129–138. doi: 10.1007/s11104-008-9595-3
Arnon, D. I.. (1949). Copper enzymes in isolated chloroplasts. polyphenoloxidase in beta vulgaris. Plant Physiol. 24, 1. doi: 10.1104/pp.24.1.1
Bargaz, A., Elhaissoufi, W., Khourchi, S., Benmrid, B., Borden, K. A., and Rchiad, Z. (2021). Benefits of phosphate solubilizing bacteria on belowground crop performance for improved crop acquisition of phosphorus. Microbiol. Res. 252, 126842. doi: 10.1016/j.micres.2021.126842
Bargaz, A., Faghire, M., Farissi, M., Drevon, J. J., and Ghoulam, C. (2013). Oxidative stress in the root nodules of Phaseolus vulgaris is induced under conditions of phosphorus deficiency. Acta Physiol. Plant. 35, 1633–1644. doi: 10.1007/s11738-012-1206-5
Bates, L. S., Waldren, R. P., and Teare, I. D. (1973). Rapid determination of free proline for water-stress studies. Plant Soil. 39, 205–207. doi: 10.1007/BF00018060
Beyer, W. F., and Fridovich, I. (1987). Assaying for superoxide dismutase activity: Some large consequences of minor changes in conditions. Anal. Biochem. 161, 559–566. doi: 10.1016/0003-2697(87)90489-1
Billah, M., Khan, M., Bano, A., Hassan, T. U., Munir, A., and Gurmani, A. R. (2019). Phosphorus and phosphate solubilizing bacteria: keys for sustainable agriculture. Geomicrobiol. J. 36, 904–916. doi: 10.1080/01490451.2019.1654043
Bouizgaren, A., Farissi, M., Ghoulam, C., Kallida, R., Faghire, M., Barakate, M., et al. (2013). Assessment of summer drought tolerance variability in Mediterranean alfalfa (Medicago sativa L.) cultivars under Moroccan fields conditions. Arch. Agron. Soil Sci. 59, 147–160. doi: 10.1080/03650340.2011.606216
Carlsson, G., and Huss-Danell, K. (2003). Nitrogen fixation in perennial forage legumes in the field. Plant Soil. 253, 353–372. doi: 10.1023/A:1024847017371
Chang, C.-C., Yang, M.-H., Wen, H.-M., and Chern, J.-C. (2002). Estimation of total flavonoid content in propolis by two complementary colometric methods. J. Food Drug Anal. 10, 3 doi: 10.38212/2224-6614.2748
Dokwal, D., Romsdahl, T. B., Kunz, D. A., Alonso, A. P., and Dickstein, R. (2021). Phosphorus deprivation affects composition and spatial distribution of membrane lipids in legume nodules. Plant Physiol. 185, 1847–1859. doi: 10.1093/plphys/kiaa115
Ekardt, F.. (2016). Justice and sustainability: normative criteria for the use of phosphorus. In: Phosphorus in Agriculture: 100 % Zero. Springer, Dordrecht. p. 317–330. doi: 10.1007/978-94-017-7612-7_15
El Moukhtari, A., Carol, P., Mouradi, M., Savoure, A., and Farissi, M. (2021a). Silicon improves physiological, biochemical, and morphological adaptations of alfalfa (Medicago sativa L.) during salinity stress. Symbiosis. 85, 305–324. doi: 10.1007/s13199-021-00814-z
El Moukhtari, A., Lamsaadi, N., Oubenali, A., Mouradi, M., Savoure, A., and Farissi, M. (2021b). Exogenous silicon application promotes tolerance of legumes and their N2 fixing symbiosis to salt stress. Silicon. 1–18. doi: 10.1007/s12633-021-01466-w
Elhaissoufi, W., Khourchi, S., Ibnyasser, A., Ghoulam, C., Rchiad, Z., Zeroual, Y., et al. (2020). Phosphate solubilizing rhizobacteria could have a stronger influence on wheat root traits and aboveground physiology than rhizosphere p solubilization. Front. Plant Sci. 11, 979. doi: 10.3389/fpls.2020.00979
El-Ramady, H., Abdalla, N., Kovacs, S., Domokos-Szabolcsy, E., Bákonyi, N., Fari, M., et al. (2020). Sustainable Biorefinery and Production of Alfalfa (Medicago sativa L.). Egypt. J. Bot. 60, 621–639. doi: 10.21608/ejbo.2020.37749.1532
Etesami, H., Jeong, B. R., and Glick, B. R. (2021). Contribution of arbuscular mycorrhizal fungi, phosphate–solubilizing bacteria, and silicon to P uptake by plant. Front. Plant Sci. 12, 1355. doi: 10.3389/fpls.2021.699618
Farissi, M., Bouizgaren, A., Faghire, M., Bargaz, A., and Ghoulam, C. (2011). Agro-physiological responses of Moroccan alfalfa (Medicago sativa L.) populations to salt stress during germination and early seedling stages. Seed Sci. Technol. 39, 389–401. doi: 10.15258/sst.2011.39.2.11
Farissi, M., Bouizgaren, A., Faghire, M., Bargaz, A., and Ghoulam, C. (2013). Agrophysiological and biochemical properties associated with adaptation of Medicago sativa populations to water deficit. Turk. J. Bot. 37, 1166–1175. doi: 10.3906/bot-1211-16
Farssi, O., Saih, R., El Moukhtari, A., Oubenali, A., Mouradi, M., Lazali, M., et al. (2021). Synergistic effect of Pseudomonas alkylphenolica PF9 and Sinorhizobium meliloti Rm41 on Moroccan alfalfa population grown under limited phosphorus availability. Saudi J. Biol. Sci. 28, 3870–3879. doi: 10.1016/j.sjbs.2021.03.069
Fatemi, H., Esmaiel Pour, B., and Rizwan, M. (2020). Isolation and characterization of lead (Pb) resistant microbes and their combined use with silicon nanoparticles improved the growth, photosynthesis and antioxidant capacity of coriander (Coriandrum sativum L.) under Pb stress. Environ. Pollut. 266, 114982. doi: 10.1016/j.envpol.2020.114982
Fatima, F., Ahmad, M. M., Verma, S. R., and Pathak, N. (2021). Relevance of phosphate solubilizing microbes in sustainable crop production: a review. Int. J. Environ. Sci. Technol. 1–14. doi: 10.1007/s13762-021-03425-9
Fuentes, S., Wikfors, G. H., and Meseck, S. (2014). Silicon deficiency induces alkaline phosphatase enzyme activity in cultures of four marine diatoms. Estuaries Coasts. 37, 312–324. doi: 10.1007/s12237-013-9695-z
Ghoulam, C., Foursy, A., and Fares, K. (2002). Effects of salt stress on growth, inorganic ions and proline accumulation in relation to osmotic adjustment in five sugar beet cultivars. Environ. Exp. Bot. 47, 39–50. doi: 10.1016/S0098-8472(01)00109-5
Greger, M., Landberg, T., and Vaculík, M. (2018). Silicon influences soil availability and accumulation of mineral nutrients in various plant species. Plants. 7, 41. doi: 10.3390/plants7020041
Hafez, E. M., Osman, H. S., Gowayed, S. M., Okasha, S. A., Omara, A. E. D., Sami, R., et al. (2021). Minimizing the adversely impacts of water deficit and soil salinity on maize growth and productivity in response to the application of plant growth-promoting rhizobacteria and silica nanoparticles. Agron. 11, 676. doi: 10.3390/agronomy11040676
Heath, R. L., and Packer, L. (1968). Photoperoxidation in isolated chloroplasts. I. Kinetics and stoichiometry of fatty acid peroxidation. Arch. Biochem. Biophys. 125, 189–198. doi: 10.1016/0003-9861(68)90654-1
Hoagland, D. R., and Arnon, D. I. (1950). The water-culture method for growing plants without soil. Circular. California Agric Exper Stat. 347.
Israr, D., Mustafa, G., Khan, K. S., Shahzad, M., Ahmad, N., and Masood, S. (2016). Interactive effects of phosphorus and Pseudomonas putida on chickpea (Cicer arietinum L.) growth, nutrient uptake, antioxidant enzymes and organic acids exudation. Plant Physiol. Biochem. 108, 304–312. doi: 10.1016/j.plaphy.2016.07.023
Kostic, L., Nikolic, N., Bosnic, D., Samardzic, J., and Nikolic, M. (2017). Silicon increases phosphorus (P) uptake by wheat under low P acid soil conditions. Plant Soil. 419, 447–455. doi: 10.1007/s11104-017-3364-0
Kubi, H. A. A., Khan, M. A., Adhikari, A., Imran, M., Kang, S. M., Hamayun, M., et al. (2021). Silicon and plant growth-promoting rhizobacteria Pseudomonas psychrotolerans CS51 mitigates salt stress in Zea mays L. Agric. 11, 272. doi: 10.3390/agriculture11030272
Kumar, D., Yusuf, M., Singh, P., Sardar, M., and Sarin, N. (2014). Histochemical detection of superoxide and H2O2 accumulation in Brassica juncea seedlings. Bio-protocol. 4, e1108. doi: 10.21769/BioProtoc.1108
Mahmood, S., Daur, I., Al-Solaimani, S. G., Ahmad, S., Madkour, M. H., Yasir, M., et al. (2016). Plant growth promoting rhizobacteria and silicon synergistically enhance salinity tolerance of mung bean. Front. Plant Sci. 7, 876. doi: 10.3389/fpls.2016.00876
Malhotra, H., Vandana, S.harma, S., and Pandey, R. (2018). Phosphorus nutrition: plant growth in response to deficiency and excess. In: Plant Nutrients and Abiotic Stress Tolerance. p. 171–190. doi: 10.1007/978-981-10-9044-8_7
Neu, S., Schaller, J., and Dudel, E. G. (2017). Silicon availability modifies nutrient use efficiency and content, C:N:P stoichiometry, and productivity of winter wheat (Triticum aestivum L.). Sci. Rep. 7, 1–8. doi: 10.1038/srep40829
Oukaltouma, K., El Moukhtari, A., Lahrizi, Y., Mouradi, M., Farissi, M., Willems, A., et al. (2021). Phosphorus deficiency enhances water deficit impact on some morphological and physiological traits in four faba bean (Vicia faba L.) varieties. Ital. J. Agron. 16, 1662. doi: 10.4081/ija.2020.1662
Owino-Gerroh, C., and Gascho, G. J. (2005). Effect of silicon on low pH soil phosphorus sorption and on uptake and growth of maize. Commun. Soil Sci. Plant Anal. 35, 2369–2378. doi: 10.1081/LCSS-200030686
Pavlovic, J., Kostic, L., Bosnic, P., Kirkby, E. A., and Nikolic, M. (2021). Interactions of silicon with essential and beneficial elements in plants. Front. Plant Sci. 12, 1224. doi: 10.3389/fpls.2021.697592
Penn, C. J., and Camberato, J. J. (2019). A critical review on soil chemical processes that control how soil ph affects phosphorus availability to plants. Agric. 9, 120. doi: 10.3390/agriculture9060120
Qari, H. A., and Oves, M. (2020). Fatty acid synthesis by Chlamydomonas reinhardtii in phosphorus limitation. J. Bioenerg. Biomembr. 52, 27–38. doi: 10.1007/s10863-019-09813-8
Radovic, J., Sokolovic, D., and Markovic, J. (2009). Alfalfa-most important perennial forage legume in animal husbandry. Biotechnol. Anim. Husb. 25, 465–475. doi: 10.2298/BAH0906465R
Rezakhani, L., Motesharezadeh, B., Tehrani, M. M., Etesami, H., and Mirseyed Hosseini, H. (2019). Phosphate–solubilizing bacteria and silicon synergistically augment phosphorus (P) uptake by wheat (Triticum aestivum L.) plant fertilized with soluble or insoluble P source. Ecotoxicol. Environ. Saf. 173, 504–513. doi: 10.1016/j.ecoenv.2019.02.060
Rezakhani, L., Motesharezadeh, B., Tehrani, M. M., Etesami, H., and Mirseyed Hosseini, H. (2020). Effect of silicon and phosphate-solubilizing bacteria on improved phosphorus (p) uptake is not specific to insoluble p-fertilized sorghum (Sorghum bicolor L.) plants. J. Plant Growth Regul. 39, 239–253. doi: 10.1007/s00344-019-09978-x
Sharma, S. B., Sayyed, R. Z., Trivedi, M. H., and Gobi, T. A. (2013). Phosphate solubilizing microbes: Sustainable approach for managing phosphorus deficiency in agricultural soils. Springerplus. 2, 1–14. doi: 10.1186/2193-1801-2-587
Shi, D., Li, D., Zhang, Y., Li, X., Tao, Y., Yan, Z., et al. (2019). Effects of Pseudomonas alkylphenolica KL28 on immobilization of Hg in soil and accumulation of Hg in cultivated plant. Biotechnol. Lett. 41, 1343–1354. doi: 10.1007/s10529-019-02736-9
Silva, J. L. F. D., and Prado, R. D. M. (2021). Elucidating the action mechanisms of silicon in the mitigation of phosphorus deficiency and enhancement of its response in sorghum plants. J. Plant Nutr. 44, 1–11. doi: 10.1080/01904167.2021.1918155
Skotti, E., Anastasaki, E., Kanellou, G., Polissiou, M., and Tarantilis, P. A. (2014). Total phenolic content, antioxidant activity and toxicity of aqueous extracts from selected Greek medicinal and aromatic plants. Ind. Crops Prod. 53, 46–54. doi: 10.1016/j.indcrop.2013.12.013
Suleman, M., Yasmin, S., Rasul, M., Yahya, M., Atta, B. M., and Mirza, M. S. (2018). Phosphate solubilizing bacteria with glucose dehydrogenase gene for phosphorus uptake and beneficial effects on wheat. PLoS ONE. 13, e0204408. doi: 10.1371/journal.pone.0204408
Sulieman, S. A., and Hago, T. E. M. (2009). The effects of phosphorus and farmyard manure on nodulation and growth attributes of common bean (Phaseolus vulgaris L.) in shambat soil under irrigation. Res. J. Agric. Biol. Sci. 5, 458–464.
Vance, C. P., Uhde-Stone, C., and Allan, D. L. (2003). Phosphorus acquisition and use: Critical adaptations by plants for securing a nonrenewable resource. New Phytol. 157, 423–447. doi: 10.1046/j.1469-8137.2003.00695.x
Wissuwa, M., Gamat, G., and Ismail, A. M. (2005). Is root growth under phosphorus deficiency affected by source or sink limitations? J. Exp. Bot. 56, 1943–1950. doi: 10.1093/jxb/eri189
Xu, H. X., Weng, X. Y., and Yang, Y. (2007). Effect of phosphorus deficiency on the photosynthetic characteristics of rice plants. Russ. J. Plant Physiol. 54, 741–748. doi: 10.1134/S1021443707060040
Keywords: Medicago sativa, Pseudomonas alkylphenolica, silicon, phosphorus deficiency, acid phosphatase, oxidative stress
Citation: El Moukhtari A, Lamsaadi N, Farssi O, Oubenali A, El Bzar I, Lahlimi Alami Q, Triqui ZEA, Lazali M and Farissi M (2022) Silicon- and Phosphate-Solubilizing Pseudomonas alkylphenolica PF9 Alleviate Low Phosphorus Availability Stress in Alfalfa (Medicago sativa L.). Front. Agron. 4:823396. doi: 10.3389/fagro.2022.823396
Received: 27 November 2021; Accepted: 24 January 2022;
Published: 02 March 2022.
Edited by:
Magdi T. Abdelhamid, National Research Centre, EgyptReviewed by:
Mahmoud Sofy, Al-Azhar University, EgyptShikha Singh, Allahabad University, India
Abdelaziz Bouizgaren, INRA Morocco, Morocco
Copyright © 2022 El Moukhtari, Lamsaadi, Farssi, Oubenali, El Bzar, Lahlimi Alami, Triqui, Lazali and Farissi. This is an open-access article distributed under the terms of the Creative Commons Attribution License (CC BY). The use, distribution or reproduction in other forums is permitted, provided the original author(s) and the copyright owner(s) are credited and that the original publication in this journal is cited, in accordance with accepted academic practice. No use, distribution or reproduction is permitted which does not comply with these terms.
*Correspondence: Mohamed Farissi, farissimohamed@gmail.com; mohamed.farissi@usms.ac.ma
†ORCID: Mohamed Farissi orcid.org/0000-0003-2853-9115; Ahmed El Moukhtari orcid.org/0000-0003-3581-2589