- Department of Microbiology and Biotechnology, University School of Sciences, Gujarat University, Ahmedabad, India
A natural bacterial isolate that shows multiple plant growth-promoting activities was isolated from fermented panchagavya (a mixture of five indigenous cow products). It is a gram-positive, endospore-forming bacteria identified as Bacillus sp. PG-8 by 16S rRNA gene sequencing. The Bacillus sp. PG-8 have shown multiple plant growth-promoting activities as indole acetic acid (2.78 μg/ml), gibberellic acid (0.7 mg/ml), ammonia (6.51 μmol/ml), exopolysaccharide (2.6% w/v) production, and phosphate solubilization (198.27 μg/ml). The Bacillus sp. PG-8 has ability to survive under the abiotic stress conditions such as temperature (28–46◦C), pH (5.0–12.0), salt (0.5–20.0% w/v NaCl), and osmotic resistance (1–10% w/v PEG-6000). Due to its diverse characteristics, the effect of Bacillus sp. PG-8 was tested on Arachis hypogea (groundnut). The seeds treated with Bacillus sp. PG-8 demonstrated a 70% germination rate with seedling vigor indexes of 154. In pot study, Arachis hypogea growth showed 1.38, 1.38, 1.32, 1.39, and 1.52 times increase in root hair number, leaf numbers, leaf width, leaf length, and leaf area, respectively. The addition of Bacillus sp. PG-8 culture to the Arachis hypogea plant resulted in a significant improvement in plant growth. Bacillus sp. PG-8 is a spore producer with stress tolerance and multiple plant growth-promoting properties, which makes it a potential liquid biofertilizer candidate.
Introduction
Panchagavya refers to as “combination of five cow products of indigenous cow,” which includes dung, urine, milk, curd, and ghee. Traditionally, it has been used in many ancient Indian health and sustainable agriculture customs. Fermented panchagavya is an organic liquid preparation mentioned in ancient Indian literature such as Vrikshayurveda that depicts the good farming practices for different plants (Natarajan, 2002).
Bacillus sp. is the most attractive among the plant growth-promoting rhizobacteria due to their spore-forming ability, and therefore, they contribute significantly in commercial formulations used for field application. Fermented panchagavya also contains a diverse microbial population with a 54% cultivable bacterial population dominated by the Bacilli group in panchagavya preparation (Anandham et al., 2015). The bacteria isolated from panchagavya belonged to Bacillus safensis or Bacillus cereus, which showed plant growth-promoting attributes (Radha and Rao, 2014). Two plant growth-promoting bacteria (PGPB), Bacillus pumilus and Bacillus subtilis, were reported in the fermented panchagavya (Ram et al., 2019).
Biofertilizers are living microorganisms that increase the growth and also the development of plants by amassing the accessibility of mineral nutrients, solubilizing phosphorus, and production of phytohormones (Mushtaq et al., 2021). The production of plant growth regulators by microorganisms delivers several benefits to the host plant with the facilitation of root system expansion, which increases the absorption of water and nutrients and improves plant survival (Verma et al., 2017). The PGPB have some properties such as nutrient solubilization, stress tolerance, and phytohormone production (Numan et al., 2018). PGPB are colonized at or near the root of the plant, and their wave of efficacy hits the doorstep of major functional aspects of the entire plant (Mondal et al., 2020). The dominant PGPBs such as Bacillus subtilis and Bacillus subterraneus demonstrated considerable germination with Arachis hypogea in pot tests for plant growth and protection qualities (Syed et al., 2020).
The demand for pesticides and chemical fertilizers has increased enormously to get high-yield crops in contemporary agriculture practices. The current trend even upsets the delicate balance of the ecosystem. Conventional agriculture practices rely very much on the intensive use of synthetic inputs to produce the bulk of the world's food and energy crops. However, these farming systems also contribute to global climate change and environmental degradation (Crowder and Illan, 2021). Concerns over the negative impacts of conventional agriculture have spurred interest in more sustainable alternative farming systems that may replace the synthetic inputs with organic biofertilizers and pesticides (Chaudhary et al., 2017). Advantages of organic farming systems include greater profitability, increased biodiversity, enhanced soil quality, and reduced pesticides, and good quality nutritious food will lead to the overall economic development of a healthy society. The Arachis hypogea was used as model plant as it is a cash crop and widely sown across the state of Gujarat and many regions of India. Owing to the nutritional value and oil content of the groundnut seeds, it remains in high demand. In this context, this study provides the impact of Bacillus sp. PG-8 isolated from fermented panchagavya on Arachis hypogea growth.
Materials and Methods
Isolation of Bacteria From Fermented Panchagavya
For the preparation of panchagavya, five cow products were collected from the Bansi Gir Gaushala in Ahmedabad, Gujarat, India. Panchagavya was a prepared method described by Gohil et al. (2020).
Fermented panchagavya samples were diluted up to 10−7, and 0.1 ml of each dilution was spread onto nutrient agar plates for the isolation of bacteria. The nutrient agar plates were incubated at 30°C for 48 h. The colonies were enumerated and characteristics were recorded. Among the isolates, PG-8 bacterial isolate that shows multiple plant growth-promoting activities and survived in various abiotic stress conditions was selected for further study. The pure culture of PG-8 bacteria was maintained and preserved by periodic transfer onto nutrient agar slants at 4°C in the refrigerator.
Microbial Identification and Phylogenetic Analysis
Identification of bacterial strain PG-8 was attempted through sequence analysis of the 16S rRNA gene. Universal 16S rRNA gene primers 27F (5'-AGAGTTTGATCCTGGCTCAG-3') and 1492R (5'-GGTTACCTTGTTACGACTT-3') were used for amplification (Goldfarb et al., 2011). DNA sequencing reaction of PCR amplicon was carried out with BDT v3.1 Cycle sequencing kit on ABI 3730xl Genetic Analyzer. The gene sequence was used to carry out BLAST with the database of NCBI GenBank database and aligned using multiple alignment software programs (http://www.ncbi.nlm.nih.gov/).
The evolutionary history was arranged by the neighbor-joining method (Saitou and Nei, 1987). The bootstrap consensus tree inferred from 500 replicates is taken to represent the evolutionary history of the taxa analyzed (Felsenstein, 1985). The percentage of replicate trees in which the associated taxa clustered organized in the bootstrap test (500 replicates) are shown next to the branches (Felsenstein, 1985). This analysis involved 9 nucleotide sequences. All ambiguous sites were removed for each sequence pairwise deletion option. Phylogenetic and molecular evolutionary analyses were conducted using MEGA 11 (Kumar et al., 2018). The nucleotide sequence of Bacillus sp. PG-8 was submitted to the National Centre for Biotechnology Information (NCBI).
Inoculum Preparation
The Bacillus sp. PG-8 culture from slant was inoculated in sterile nutrient broth and incubated at 30°C for 24 h. Cells were separated by centrifugation at 5,000 rpm for 10 min and the supernatant was removed. The cell pellet was resuspended in sterile normal saline to get optical density values of 1.0 containing ~ 8 × 108 cells/ml. The prepared culture suspension was used as a 1.0% (v/v) inoculum to study the plant growth-promoting parameters. In the pot experiment, five ml of this culture suspension was used to treat the plants.
Plant Growth-Promoting Traits
The Bacillus sp. PG-8 qualitatively showed multiple plant growth-promoting activities such as the production of indole acetic acid (IAA), gibberellic acid (GA), ammonia (NH3), exopolysaccharides (EPSs), hydrogen cyanide (HCN), and also phosphate (P) and zinc (Zn) solubilization. It was further tested to quantify the plant growth-promoting activities.
IAA Production
For IAA production, 50.0 ml of Luria-Bertani broth (pH 7.5) containing 0.1% (w/v) L-tryptophan in 250-ml flask was inoculated with 1.0% (v/v) inoculum and incubated at 30°C in the dark, as IAA is light sensitive, 120 rpm on an orbital shaker. IAA production was carried out in 24-h intervals from 24–144 h until production was decreased.
IAA estimation
The spectrophotometric estimation of IAA was done according to Bric et al. (1991). Culture supernatant (1 ml) was mixed with 2.0 ml of Salkowski reagent and incubated at room temperature (RT) in the dark for 30 min. Development of pink color shows the production of IAA and absorbance was recorded at 530 nm. The standard calibration curve of IAA (5.0–50.0 μg/ml) was prepared to calculate the IAA production.
GA Production
Gibberellic acid production was carried out in 50.0 ml nutrient broth (pH 7.4) in 250-ml flask, 1.0% (v/v) inoculum, at 30°C, 120 rpm on an orbital shaker for 96 h. GA production was measured from 24–96 h.
GA Estimation
An equal volume of cell-free supernatant and ethyl acetate (EA) was taken in a test tube and shook vigorously. The EA extract was collected separately in a glass beaker, and the extraction was repeated three times. The separated EA extract was allowed to evaporate at room temperature. The residues were dissolved in 2.0 ml methanol. To this, 1.0 ml DNPH (2, 4 – dinitrophenylhydrazine) was added and incubated in a boiling water bath for 5 min. After incubation, it was cooled in an ice-water bath, and 5.0 ml of 10% (w/v) alcoholic potassium hydroxide was added, allowed to stand for 10 min at RT. To this, 15.0 ml of sterile distilled water was added, and the intensity of the color (red wine) produced was measured at 430 nm (Graham and Thomas, 1961). A standard calibration curve of GA (0.1–0.8 mg/ml) was prepared in methanol.
NH3 Production
Ammonia production was determined in a test tube containing 20.0 ml sterile peptone water, pH 7.2. It was inoculated with 1.0% (v/v) inoculum and incubated at 30°C, 120 rpm on an orbital shaker for 144 h. The amount of NH3 produced was measured every 24–144 h.
Estimation of NH3
Spectrophotometric estimation of ammonia was done as described by Cappucino and Sherman (1992). Culture supernatant (1 ml) was mixed with 0.1 ml Nessler's reagent, and the final volume was made to 5.0 ml by adding ammonia-free distilled water. NH3 production is indicated by a change in color from yellow to brown, and absorbance was measured at 425 nm. The NH3 produced was calculated using the ammonium hydroxide (0.2–2.0 μmol/ml) standard calibration curve.
EPS Production
The EPS production was checked qualitatively by spot inoculation on the brain–heart infusion (BHI) agar plate, at 30°C as mucoid colonies with blackening. For quantitative EPS estimation, 100.0 ml nutrient broth (pH 7.1) containing 4.0% (w/v) sucrose was inoculated with 1.0% (v/v) inoculum and incubated at 30°C, 120 rpm on an orbital shaker up to 72 h. The amount of EPS produced was measured over 24–72 h.
Estimation of EPS
The estimation of EPS was done by solvent extraction described by Azeredo and Oliveira (1996). For extraction, 10.0 ml cell-free culture supernatant plus 30.0 ml of chilled acetone were mixed for precipitation at 4°C for 12 h. Precipitates were collected on a preweighed aluminum foil and wet weight was recorded. The precipitates were allowed to dry at 50–60°C, and the dry weight of the precipitates was recorded until three consecutive constant readings.
P Solubilization
The qualitative phosphate solubilization test was carried out using the spot inoculation technique on an agar plate containing an insoluble tricalcium phosphate (TCP) and incubated at 30°C for 120 h (Pikovskaya, 1948). A yellow zone develops surrounding the colony as a result of phosphate solubilization. The phosphate solubilization index (PSI) was calculated using the formula below.
Quantitative P Solubilization
A total of 100.0 ml sterile Pikovskaya's broth (pH 7.0 ± 0.2) in 250-ml flasks were inoculated and incubated at 30°C, at 120 rpm on an orbital shaker for 15 days. The amount of phosphate soluble was measured every 5–15 days.
The amount of phosphate solubilized by Bacillus sp. PG-8 was estimated by the stannous chloride method (King, 1932) and pH reduction was noted.
A total of 2.0 ml sample was centrifuged at 10,000 rpm for 20 min to separate the cells. To 0.1 ml supernatant, 0.9 ml double distilled water and 1.0 ml chloromolybdic acid was added. Contents in test tubes were diluted by adding 4.0 ml double distilled water. To this, 25.0 μL chlorostannous acid reagent was added, and the test tubes were mixed well till blue color developed. Absorbance was measured at 600 nm. The total amount of phosphate solubilized was calculated against K2HPO4 (10.0–100.0 μg/ml) standard calibration curve.
HCN Production
The HCN produced was detected by picrate assay with some modifications (Bakker et al., 1987). Bacillus sp. PG-8 was streaked on King's B agar plate supplemented with 4.4 g/L glycine. The plate was sealed with parafilm and incubated at 30°C for 168 h. HCN production was indicated a color change of filter paper from yellow to orange-brown.
Zn Solubilization
Qualitative Zn solubilization was carried out on agar plates (pH 7.0) having 0.1% (w/v) insoluble zinc oxide by spot inoculation (Bunt and Rovira, 1955). The plates were incubated at 30°C for 12 days. Zn solubilizing bacteria produce a zone of clearance around the colony.
Abiotic Stress Response of Bacillus sp. PG-8
The ability of Bacillus sp. PG-8 to tolerate different stress conditions such as salinity, drought, temperature, and pH was checked. The bacterial culture was grown on various conditions, and bacterial growth was measured on an arbitrary scale. A triple plus symbol denoted higher bacterial growth, a double plus indicate moderate growth, and a single plus indicate lower growth.
Salinity Stress
The salt tolerance of isolates was checked by observing their growth on the nutrient agar medium supplemented with different NaCl concentrations (0.5, 5, 10, 15, and 20%; w/v). The Bacillus sp. PG-8 was streaked on these plates and incubated at 28°C for 72 h.
Drought Stress
The drought resistance of Bacillus sp. PG-8 was tested on nutrient agar medium supplemented with polyethylene glycol (6000) (1, 5, 10%; w/v). The pure culture was streaked on the plates and incubated at 28°C for 72 h.
Temperature Stress
The Bacillus sp. PG-8 was examined for its ability to grow at 28°C, room temperature (16–33), 37, 40, and 46°C on nutrient agar media. Pure culture suspension was streaked on the plates and incubated at different temperatures for 72 h.
pH Tolerance
On nutrient agar media, pH tolerance was checked by growing Bacillus sp. PG-8 at different pH values 5.0, 7.0, 9.0, 11.0, and 12.0. The pure culture was streaked on the nutrient agar plates with different pH values and incubated at 28°C for 72 h.
Seed Germination Test
Seeds of Arachis hypogea were collected from the local agriculture seed shop, Ahmedabad, Gujarat. The seeds were washed thoroughly with water to remove the dirt followed by washing with distilled water two times to remove any remaining tap water. Then, the seeds were treated with 0.1% (v/v) of HgCl2 solution for 2–3 min. Seeds were then cleaned three times with 70% (v/v) ethanol followed by rinsing with sterile distilled water five times. Disinfected seeds were used for the seed germination test and pot experiment.
Seeds (nos.100) were placed on sterile cotton in a sterile petri plate. Pure culture suspension of Bacillus sp. PG-8 was added to the petri plates containing seeds and incubated at room temperature. For comparative evaluation, untreated seeds were treated with distilled water used as controls. After 72 h, germinated seeds were counted, and the germination percent and seed vigor index were calculated by the following formula:
Pot Experiment
The pot study was conducted in plastic bags with 18.0 cm × 8.0 cm height and diameter in the month of February–March, 2020, with an average temperature (35 ± 4°C) in the departmental greenhouse, Gujarat University, Ahmedabad.
About 12.0 kg soil was collected from a depth of 4.0 inches in a sterile plastic bag from the central library garden of Gujarat University Campus for a pot experiment. For the preparation of the pot, garden soil was filled with approximately 500 g of UV sterile soil having a neutral pH. The disinfected seeds of Arachis hypogea were sowed and watered slightly after transplanting at a depth of 0.5 cm. The experiment was carried out in duplicate for both the plants. Plants were treated with 5.0 ml Bacillus sp. PG-8 suspension, and the treatments were administered every five days after seeding (DAS). Two control bags were treated with sterile distilled water instead of culture. The plant from each pot was uprooted on the 10th, 20th, and 30th day to study the effect of Bacillus sp. PG-8 on fresh plant weight, dry weight, shoot length, root length, stem diameter, root hair number, leaf number, leaf width, leaf length, and leaf area of individual plants.
Results and Discussion
Morphological and Cultural Characteristics
A total of sixty-four culturally different bacteria were isolated from the fermented panchagavya (Gohil et al., 2020). Bacterial cultures were screened for their plant growth-promoting activities and abiotic stress tolerance. Among the isolates, a gram-positive PG-8 bacteria showed multiple plant growth-promoting activities with abiotic stress responses, and it was selected for further study. High levels of bacterial diversity and richness were reported in the fermented panchagavya (Gunwantrao and Patil, 2015). The 15 bacterial cultures were isolated from panchagavya and shown to have a variety of plant growth-promoting traits by Nagaraj and Sreenivasa (2009). A number of thirty-seven diverse groups of bacteria were isolated from panchagavya (Bhat et al., 2005). Similarly, our results confirm that fermented panchagavya is having rich bacterial diversity and many bacteria show plant growth-promoting activities.
The PG-8 is having a 4–5-mm size colony with an irregular margin and “Medusa-head” (Figure 1). Colonies have sticky consistency when manipulated with a loop. PG-8 is a big rod-shaped, endospore-forming bacteria that showed a gram-positive reaction (Figure 1). Colonial characteristics of the PG-8 are recorded in Table 1. The gram-positive bacterial population was found higher in panchagavya (Ram et al., 2019). The microbial load of panchagavya showed a considerable number of gram-positive bacteria (Patnaik et al., 2012). The natural bacterial isolate PG-8 from the fermented panchagavya is a gram-positive, endospore-forming, rod-shaped bacteria closely related to the Bacillus genus.
Identification of PG-8 Strain Through 16S RRNA Gene Sequencing
A 16S rRNA gene sequencing was done to identify PG-8. Phylogenetic analysis showed 97.4% percent similarity with the gene sequence of MT225680.1 Bacillus haynesii strain 190311L225 MT516447.1 Bacillus sp. (in: Bacteria) strain L23, MT525268.1 Bacillus paralicheniformis strain SA25, MT527538.1 Bacillus paralicheniformis strain CB211, MT534566.1 Bacillus sonorensis strain NB35, MT534569.1:11-1370 Bacillus licheniformis strain NB45, MW819909.1 Bacillus spongiae strain DN-5, and MW876139.1 Bacillus haynesii strain XJB-19 that showed the highest degree of homology was used to construct a phylogenetic tree using the neighbor-joining method.
The evolutionary history was inferred using the maximum composite likelihood method (Tamura et al., 2004). The percentage of trees in which the associated taxa clustered together is shown next to the branches. The analysis involved 9 nucleotide sequences. There were a total of 1,520 positions in the final dataset. The nucleotide sequence of 1,500 base pairs Bacillus sp. PG-8 was deposited in the nucleotide GenBank database under the accession number OK175649.
The 16S rRNA gene sequence of the bacterial strains isolated from panchagavya belonged to Bacillus safensis and Bacillus cereus (Radha and Rao, 2014). Panchagavya contains Bacillus aquimaris, Bacillus subtilis, Bacillus cereus, Bacillus licheniformis, Bacillus endophyticus, and Bacillus barbaricus (Donaldson and Emmanuel, 2021). The bacterial strain PG-8 isolated from panchagavya has shown the phylogenetic relatedness with Bacillus strains (Figure 2). The results are in line with those reported earlier and show the potential application of genus Bacillus in plant growth promotion.
Primary Screening of Bacillus sp. PG-8 for Plant Growth-Promoting Parameters
The Bacillus sp. PG-8 was tested for various plant growth-promoting qualities qualitatively, which include phytostimulant production, nutrient solubilization, and plant protectants. The results revealed that the Bacillus sp. PG-8 possesses a variety of plant growth-promoting capabilities such as the production of IAA, GA, NH3, EPS, and phosphate solubilization (Table 2).
Bacillus sp. PG-8 formed a black mucoid colony on the brain–heart infusion agar plate after 48 h, which indicates that EPS producer. Phosphate solubilization by Bacillus sp. PG-8 was observed in the form of a yellow zone formed around the colony on the Pikovskaya agar plate after 72 h. Bacillus sp. PG-8 was incapable of producing hydrogen cyanide and zinc solubilization.
Various PGPB often exhibit one or more plant growth-stimulating traits, each with distinct activity under different environmental and soil circumstances (Olanrewaju et al., 2017). PGPB have numerous plant growth-promoting characteristics such as phosphate and zinc solubilization with IAA, HCN, and exopolysaccharide production (Kalam et al., 2020). Panchagavya isolates were shown to be effective in plant growth promotion through the solubilization of insoluble phosphate and the production of indole acetic acid (Panchal, 2016). Various Bacillus sp. have been reported for multiple PGP activities such as IAA, GA, EPS production, and P solubilization (Bonatelli et al., 2020; Miljaković et al., 2020; Magotra et al., 2021). As PG-8 shows multiple plant growth-promoting activities, it can be applied in the field to supplement nutrients and augment plant growth.
Secondary Screening of Bacillus sp. PG-8 for Plant Growth-Promoting Parameters
Indole Acetic Acid Production
Indole acetic acid production is very common among the PGPB because it helps in root expansion and uptake of nutrients. The Bacillus sp. PG-8 has produced 2.78 μg/ml IAA in the presence of 0.1% (w/v) tryptophan, at 30°C after 120 h (Figure 3A). Rhizospheric isolate Bacillus thuringiensis produced 0.20 μg/ml IAA with 0.1% (w/v) tryptophan after 21 h (Batista et al., 2021). Bacillus siamensis isolated from rhizospheric soil produced 5.14 μg/ml IAA with 0.1% (w/v) tryptophan after 72 h (Saleem et al., 2021). The Bacillus sp. PG-8 has reasonably produced a good amount of IAA compared to other PGPR.
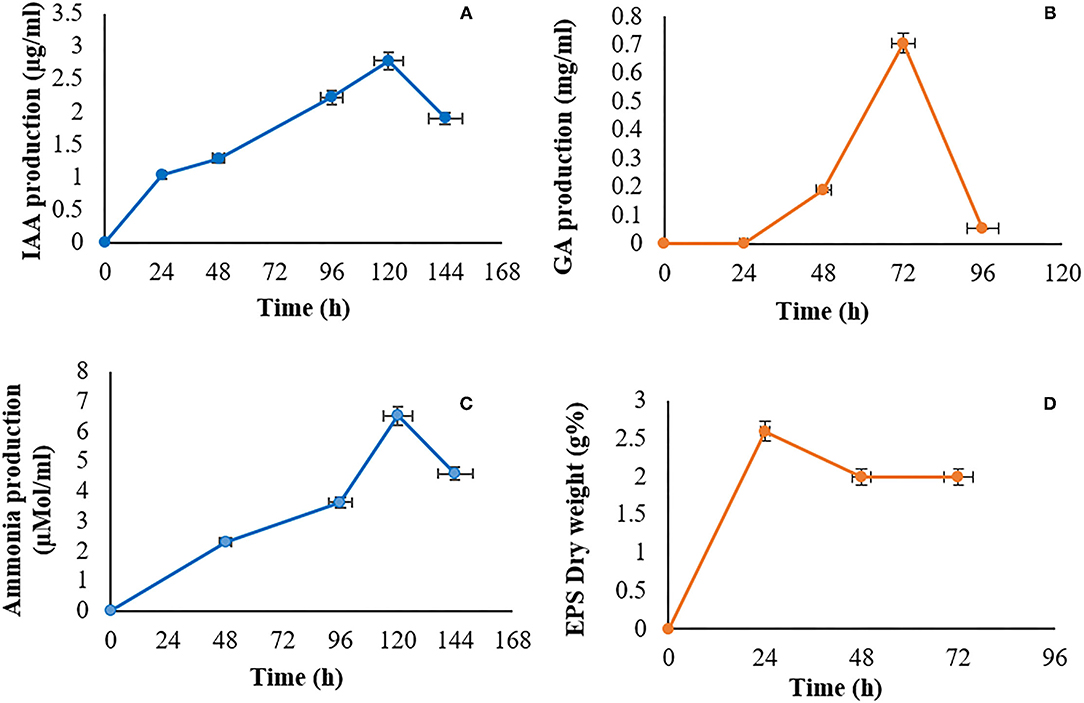
Figure 3. (A) Indole acetic acid production, (B) Gibberellic acid production, (C) Ammonia production, (D) Exopolysaccharide production.
Gibberellic Acid Production
Gibberellic acid is a phytohormone that helps in seed germination and plant growth. The Bacillus sp. PG-8 was able to produce the highest gibberellic acid 0.70 mg/ml at 30°C after 72 h in nutrient broth (Figure 3B). Rhizospheric isolate Bacillus siamensis produced 0.24 mg/ml GA after 96 h in broth (Ambawade and Pathade, 2015). Bacillus cereus isolated from the rhizosphere produced 0.39 mg/ml GA in a nutrient broth after 216 h (Pandya and Desai, 2013). PGPR, Bacillus pumilus, and Bacillus licheniformis produced physiologically active gibberellins in the host plant (Gutiérrez-Mañero et al., 2001). Our isolate produced double the amounts of gibberellic acid within 72 h than those reported earlier.
Ammonia Production
Ammonia production is the most common mechanism of PGPB. Considerable ammonia production was found in peptone broth by fermented panchagavya bacterial isolate. Bacillus sp. PG-8 produced the maximum 6.51 μmol/ml ammonia production after 120 h and was decreased after 144 h (Figure 3C). Agbodjato et al. (2015) reported that 80% of plant growth-promoting Bacillus species have shown ammonia production. Rhizobacteria, Bacillus cereus, and Bacillus megaterium produced 2.3 and 6.2 μmol/ml of ammonia, respectively, after 72 h (Dutta and Thakur, 2017). Bacillus megaterium isolated from soil produced 2.04 μmol/ml ammonia after 96 h (Krunal and Sanjay, 2020). Ammonia accumulation in soil may lead to alkaline conditions that suppress the growth of several plant pathogens. Production of ammonia is important for nitrogen accumulation because it promotes root, shoot, and biomass growth, which accelerates plant growth (Masclaux-Daubresse et al., 2010).
EPS Production
Bacterial exopolymer substances are released in response to the physiological stress encountered in the natural environment. Exopolysaccharide was recovered by acetone extraction, and significant production was found by panchagavya bacterial isolate. The Bacillus sp. PG-8 was able to produce 2.6% (w/v) EPS in nutrient broth containing 4.0% (w/v) sucrose after 24 h (Figure 3D). EPS production was gradually decreased then after 24 h in the broth. Bacillus licheniformis isolated from soil produced 0.6% (w/v) EPS after 96 h (Gupta et al., 2021). Bacillus licheniformis produced 4.8% (w/v) EPS after 96 h with 10.0% (w/v) sodium succinate (Malick et al., 2017). Paenibacillus polymyxa isolated from wasp honeycombs was able to produce 2.4% (w/v) EPS with 5.0% (w/v) sucrose after 48 h (Liyaskina et al., 2021). The source and concentration of sugar in the medium affect EPS production. Panchagavya isolates Bacillus sp. PG-8 produced considerable EPS with lower sugar concentrations within 24 h. The Bacillus sp. PG-8 is capable of secreting EPS that may protect themselves and the host plants against abiotic stresses such as drought, salinity, or heavy metal pollution.
Phosphate Solubilization
Phosphate solubilizing bacteria improve the phosphates availability by solubilizing them and making them available to the plant. Bacillus sp. PG-8 has shown the phosphate solubilization index of 1.8 after 72 h. The Bacillus sp. PG-8 has solubilized 198 μg/ml insoluble tricalcium phosphorus after 10 days in Pikovskaya's broth (Figure 4). Bacillus sp. PG-8 was able to solubilize tricalcium phosphate sources along with a pH drop from 7.0 to 4.5 after 10 days. The Bacillus cereus was able to solubilize 159 μg/ml tricalcium phosphate after 10 days in Pikovskaya's broth (Salwan et al., 2021). Rhizospheric isolate Bacillus cereus was able to solubilize 140 μg/ml phosphate after 3 days (Abdelmoteleb and Gonzalez-Mendoza, 2020). Bacillus xijun isolated from rhizospheric soil solubilized 130 μg/ml phosphate after 15 days showing pH drop from 7.0 to 4.7 (Zhong et al., 2021). The Bacillus sp. PG-8 showed better phosphate solubilization compared to the above PGPR.
Multiple plant growth-promoting activities, such as IAA, GA, EPS, ammonia production, and P solubilization by Bacillus sp. PG-8 are summarized in Table 3 with other Bacillus species. Panchagavya isolate Bacillus sp. PG-8 produced higher IAA than B. safensis and B. albus. GA production is about 30 times higher than other Bacillus sp. Our isolate also produced more ammonia production than B. cereus and showed similar phosphate solubilization.
Abiotic Stress Response
Abiotic stress is responsible for significant yield losses on crop scale worldwide. Bacillus sp. PG-8 responded well in abiotic stress such as, osmotic resistance, salt, temperatures, and pH and results are as noted in Table 4.
The growth of Bacillus sp. PG-8 was examined on a nutrient agar plate at temperatures ranging from 28 to 46°C, and the results revealed the growth of Bacillus sp. PG-8 was good over the range. Bacillus sp. PG-8 can survive at lower and also higher temperatures. Generally, bacterial growth is reduced at the field level in summer due to higher temperatures. Bacterial culture's ability to withstand higher and lower temperatures is important in the preparation of liquid biofertilizers. Plants and bacteria produce several proteins that increase their tolerance to extreme temperatures. Bacillus sp. is more tolerant to high temperatures due to the production of heat-shock proteins and the presence of extremely resistant and dormant endospores (Getahun et al., 2020). Bacillus cereus and Bacillus subtilis can tolerate temperatures up to 45°C (Ashraf et al., 2019). Bacillus sp. PG-8 can be employed at the field level because of its capacity to tolerate higher temperatures.
Bacillus sp. PG-8 showed good growth on the nutrient agar plate with 0.5–10.0% (w/v) salt. The decreased growth was observed at 15 to 20% (w/v) salt but it could survive the higher salt levels. Bacillus tequilensis and Bacillus aryabhattai can tolerate up to 12% (w/v) salt (Shultana et al., 2020). The formation of biofilm and exopolysaccharides kept the viability of bacterial cells under salt stress to protect them in the rhizosphere. Bacillus sp. PG-8 produces more EPS, and the blackening of the colony on the BHI plate indicates that it may form a biofilm.
The growth of Bacillus sp. PG-8 was examined on a nutrient agar plate having different pH values (5.0, 7.0, 9.0, 11.0, and 12.0), and better growth was observed with a pH range of 5.0–12.0. The Bacillus sp. PG-8 may thrive in a range of pH environments, which include acidic, neutral, and basic. Bacillus thuringiensis was able to survive in 4.0–10.0 pH (Getahun et al., 2020). Bacillus subtillus growth was observed in acidic and alkaline media with a pH range of 5.0 to 8.0 on nutrient agar plates (Zahid, 2015). Bacillus genus has chemoreceptor adaptation systems for sensing chemical gradients (Tohidifar et al., 2020). The presence of sodium chloride, sodium carbonate, sodium hydrogen carbonate, and sulfuric acid in the soil induces crop stress (Sairam et al., 2002). PGPB may thrive in both acidic and alkaline soils and aid in the growth of plants.
The PEG (6000) at 5, 10, and 20% (w/v) concentration is equivalent to osmotic potential levels of −0.27, −0.54, and −1.09 MPa, respectively. Bacillus sp. PG-8 can tolerate polyethylene glycol concentrations up to 10% (w/v) and stress up to −0.54 MPa to maintain a high water activity level. Bacillus amyloliquefaciens and Bacillus licheniformis could tolerate matric stress up to −0.73 MPa (Vardharajula et al., 2011). PGPB isolate Pseudomonas fluorescens was able to grow in stress at a minimum water potential (-0.30 MPa) with PEG-6000 (Niu et al., 2018). Bacillus amyloliquefaciens has the ability to sustain pepper growth in the face of drought, salt, and heavy metal stress (Kazerooni et al., 2021). Bacillus thuringiensis showed better temperature tolerance at 45°C, tolerance of acidic and alkaline pH, high salinity, and drought resistance (Getahun et al., 2020). Significant effects of plant tolerance to abiotic stress are that it will result in promoting the yield and production of crops to feed humans and livestock. Bacillus sp. PG-8 showed that multiple abiotic stress tolerance hence could be useful for field application.
Seed Germination
Seed germination is one of the most crucial phases of the growth cycle in plant development. The percentage germination ability of Bacillus sp. PG-8 is shown in Figure 5. The seeds of Arachis hypogea treated with Bacillus sp. PG-8 showed 75 percent seed germination with 154 seed vigor index whereas control showed 70 percent and 110, respectively. Bacillus licheniformis culture-treated Arachis hypogea seedlings showed 85% germination (Shifa et al., 2015). Seed germination is a parameter of prime significance and fundamental to total biomass and yield production. As Bacillus sp. PG-8 has a beneficial effect on Arachis hypogea seed germination, it can be used to promote plant growth in the field.
Pot Experiment
In primary tests, Bacillus sp. PG-8 was tested for its beneficial role in producing phytohormones and releasing phosphorus in the broth medium. The biological contribution of Bacillus sp. PG-8 on the growth of Arachis hypogea was investigated, and the results were recorded with control (Table 5). The results revealed that the pure culture of Bacillus sp. PG-8 has a great influence on the development of the plant.
It was observed that treated plants had longer roots and shoots, more number of leaves with better breadth and length than the untreated control plants (Figure 6). In addition, treated plants had a higher fresh weight and dry weight. Arachis hypogea inoculated with Bacillus sp. PG-8 showed remarkable improvements in the fresh weight, dry weight, shoot length, root length, root hair number, and stem diameter by 1.08, 1.15, 1.09, 1.08, 1.38, and 1.05 times, respectively, after the 30th day. Arachis hypogea inoculated with Bacillus sp. PG-8 showed remarkable improvements in the leaf numbers, leaf width, leaf length, and leaf area by 1.38, 1.32, 1.39, and 1.52 times, respectively, after the 30th day (Figure 6).
Bacillus species, such as Bacillus subtilis, Bacillus amyloliquoefaciens, Bacillus cereus, Bacillus pumilus, and Bacillus polymyxa, are well known for their plant growth and development abilities because they produce antibiotics and produce phytohormones and phosphate solubilization (Majumdar, 2017). The Bacillus sp. PG-8-treated plants showed growth parameters that indicated that they can survive easily in soil. The Bacillus sp. was able to solubilize the additional insoluble nutrients and simultaneously help the plant growth. Bacillus is the most common nonrhizobial endophytic genus in summer crops, and it can enhance plant root development (Bhutani et al., 2018). By regulating auxin-responsive genes, PGPR regulates endogenous IAA levels in rice roots, which causes root architectural alterations (Ambreetha et al., 2018). Bacillus sp. was effectively exploited to enhance the growth and yield of V. radiata. Bacillus megaterium, Bacillus pumilus, and Bacillus subtilis increased shoot length by 55.55, 46.46, and 46.20% over control, respectively (Kumari et al., 2018). Arachis hypogea treated with Bacillus licheniformis exhibited good plant development (Shifa et al., 2015). Inoculation of soil or crops with PGPB is a potential technique for boosting plant growth, thus reducing the usage of environmentally harmful chemical fertilizers (Alori et al., 2012). The increase in growth attributes of Arachis hypogea might be due to the involvement of Bacillus sp. PG-8 by increasing the availability of nutrients, biosynthesis of plant growth regulators such as auxins, gibberellic acid, and phosphate solubilization.
Conclusion
In this study, PGPB was isolated from fermented panchagavya. The Bacillus sp. PG-8 has multiple plant growth-promoting properties such as the production of gibberellic acid, indole acetic acid, ammonia, exopolysaccharide, and phosphate solubilization. Application of Bacillus sp. PG-8 improved Arachis hypogea growth as compared to control. It showed considerable improvements in the shoot length, root length, root hair number, leaf number, and leaf area in both plants. A spore-forming Bacillus sp. PG-8 shows multiple plant growth-promoting abilities and can survive in stress conditions, which makes it a potential candidate for application as bioinoculants and biofertilizers.
Data Availability Statement
The datasets presented in this study can be found in online repositories. The names of the repository/repositories and accession number(s) can be found in the article/supplementary material.
Author Contributions
RG: concept, experimental work, and manuscript writing. VR: data curation and review and editing. RP: language editing, data curation, and manuscript shaping. KR: conceptualization, data curation, supervision, writing original draft, review and editing. RG, VR, RP, and KR: contributed to manuscript revision, read, and approved the submitted version. All authors contributed to the article and approved the submitted version.
Conflict of Interest
The authors declare that the research was conducted in the absence of any commercial or financial relationships that could be construed as a potential conflict of interest.
Publisher's Note
All claims expressed in this article are solely those of the authors and do not necessarily represent those of their affiliated organizations, or those of the publisher, the editors and the reviewers. Any product that may be evaluated in this article, or claim that may be made by its manufacturer, is not guaranteed or endorsed by the publisher.
Acknowledgments
Infrastructure facility of the Department of Microbiology and Biotechnology, Gujarat University is duly acknowledged.
References
Abdelmoteleb, A., and Gonzalez-Mendoza, D. (2020). Isolation and identification of phosphate solubilizing Bacillus sp. from Tamarix ramosissima rhizosphere and their effect on the growth of Phaseolus vulgaris under salinity stress. Geomicrobiol. J. 37, 901–908. doi: 10.1080/01490451.2020.1795321
Agbodjato, N. A., Noumavo, P. A., Baba-Moussa, F., Salami, H. A., Sina, H., Sèzan, A., et al. (2015). Characterization of potential plant growth-promoting rhizobacteria isolated from Maize (Zea mays L.) in central and Northern Benin (West Africa). Appl. Environ. Soil Sci. 2015. doi: 10.1155/2015/901656
Alori, E. T., Fawole, O., and Afolayan, A. (2012). Characterization of arbuscular mycorrhizal spores isolated from Southern Guinea Savanna of Nigeria. J. Agric. Sci. 4(7), 13-19. doi: 10.5539/jas.v4n7p13
Ambawade, M. S., and Pathade, G. R. (2015). Production of gibberellic acid by Bacillus siamensis BE 76 isolated from banana plant (Musa sp.). Int. J. Sci. Res. 4, 394–398. Available online at: https://www.ijsr.net/archive/v4i7/SUB152006.pdf
Ambreetha, S., Chinnadurai, C., Marimuthu, P., and Balachandar, D. (2018). Plant-associated Bacillus modulates the expression of auxin-responsive genes of rice and modifies the root architecture. Rhizo. 5, 57–66. doi: 10.1016/j.rhisph.2017.12.001
Anandham, R., Premalatha, N., Jee, H. J., Weon, H. Y., Kwon, S. W., Krishnamoorthy, R., et al. (2015). Cultivable bacterial diversity and early plant growth promotion by the traditional organic formulations prepared using organic waste materials. Int. J. Recycl. Org. Waste Agric. 4, 279–289. doi: 10.1007/s40093-015-0107-1
Ashraf, A., Bano, A., and Ali, S. A. (2019). Characterisation of plant growth-promoting rhizobacteria from rhizosphere soil of heat-stressed and unstressed wheat and their use as bio-inoculant. J. Plant Biol. 21, 762–769. doi: 10.1111/plb.12972
Ashry, N. M., Alaidaroos, B. A., Mohamed, S. A., Badr, O. A., El-Saadony, M. T., and Esmael, A. (2021). Utilization of drought-tolerant bacterial strains isolated from harsh soils as a plant growth-promoting rhizobacteria (PGPR). Saudi J. Biol. Sci. doi: 10.1016/j.sjbs.2021.10.054
Azeredo, J., and Oliveira, R. (1996). A new method for precipitating bacterial exopolysaccharides. Biotech. Tech. 10, 341–344. doi: 10.1007/BF00173251
Bakker, A. W., Cosse, A. A., and Schippers, B. (1987). The role of HCN-producing Pseudomonas sp. in yield reductions in short potato rotations. Mededelingen van de Faculteit landbouwwetenschappen. Rijksuniversiteit Gent. 52, 1111–1117.
Batista, B. D., Dourado, M. N., Figueredo, E. F., Hortencio, R. O., Marques, J. P. R., Piotto, F. A., et al. (2021). The auxin-producing Bacillus thuringiensis RZ2MS9 promotes the growth and modifies the root architecture of tomato (Solanum lycopersicum cv. Micro-Tom). Arch. Microbiol. 1, 3869–3882. doi: 10.1007/s00203-021-02361-z
Bhat, S. S., Vinu, A. K., and Naidu, R. (2005). Association of diverse groups of bacteria with 'Panchagavya' and their effect on growth promotion of coffee seedlings. In: ASIC 2004. 20th International Conference on Coffee Science, Bangalore, India, 11-15 October 2004. Association Scientifique Internationale du Café (ASIC) p. 1192–1198. Available online at: https://www.tib.eu/en/search/id/BLCP:CN061085152
Bhutani, N., Maheshwari, R., Negi, M., and Suneja, P. (2018). Optimization of IAA production by endophytic Bacillus sp. from Vigna radiata for their potential use as plant growth promoters. Isr. J. Plant Sci. 65, 83–96. doi: 10.1163/22238980-00001025
Bonatelli, M. L., Li, J., Dumaresq, A., Quecine, M. C. A. N. D , and Settles, M. L. (2020). Complete genome sequence of Bacillus sp. Strain RZ2MS9, a multitrait plant growth promoter. Microbiol. Resour. Announc. 9, e00623–20. doi: 10.1128/MRA.00623-20
Bric, J. M., Bostock, R. M., and Silverstone, S. E. (1991). Rapid in situ assay for indoleacetic acid production by bacteria immobilized on a nitrocellulose membrane. Appl. Environ. Microbiol. 57, 535–538. 10.1128/aem.57.2.535-538.1991. doi: 10.1128/aem.57.2.535-538.1991
Bunt, J. S., and Rovira, A. D. (1955). Microbiological studies of some subantarctic soils. J. of Soil Sci. 6, 119–128. doi: 10.1111/j.1365-2389.1955.tb00836.x
Cappucino, J. C., and Sherman, N. (1992). Nitrogen Cycle. Microbiology: A Laboratory Manual, 3rd Edn. New York, NY: Benjamin/Cumming Publications.
Chaudhary, S., Kanwar, R. K., Sehgal, A., Cahill, D. M., Barrow, C. J., Sehgal, R., et al. (2017). Progress on Azadirachta indica based biopesticides in replacing synthetic toxic pesticides. Fron. Plant Sci. 8, 610. doi: 10.3389/fpls.2017.00610
Crowder, D. W., and Illan, J. G. (2021). Expansion of organic agriculture. Nature Food. 2, 324–325. doi: 10.1038/s43016-021-00288-8
Donaldson, E., and Emmanuel, C. J. (2021). Isolation and Identification of Bacillus Species Present in the Panchagavya Preparations. Undergraduate Research Symposium (URS 2021). Physics Society, University of Jaffna. Available online at: http://repo.lib.jfn.ac.lk/ujrr/handle/123456789/1457
Dutta, J., and Thakur, D. (2017). Evaluation of multifarious plant growth-promoting traits, antagonistic potential and phylogenetic affiliation of rhizobacteria associated with commercial tea plants grown in Darjeeling, India. PloS One. 12, e0182302. doi: 10.1371/journal.pone.0182302
Felsenstein, J (1985). Confidence limits on phylogenies: An approach using the bootstrap. Evol. 39:83–791. doi: 10.1111/j.1558-5646.1985.tb00420.x
Getahun, A., Muleta, D., Assefa, F., and Kiros, S. (2020). Plant Growth-Promoting Rhizobacteria Isolated from Degraded Habitat Enhance Drought Tolerance of Acacia (Acacia abyssinica Hochst. ex Benth.) Seedlings. Int J Microbiol. 10.1155/2020/8897998. doi: 10.1155/2020/8897998
Gohil, R., Patel, K., Shah, H., Vyas, S., Desai, M., and Rajput, K. (2020). Isolation and Screening of Plant Growth Promoting Bacteria from Fermented Panchagavya. Biosci. Biotechnol. Res. Commun. 13, 42–48. doi: 10.21786/bbrc/13/1
Goldfarb, K. C., Karaoz, U., Hanson, C. A., Santee, C. A., Bradford, M. A., Treseder, K. K., et al. (2011). Differential growth responses of soil bacterial taxa to carbon substrates of varying chemical recalcitrance. Front. Microbio. 2, 94. doi: 10.3389/fmicb.2011.00094
Graham, H. D., and Thomas, L. B. (1961). Rapid, Simple Colorimetric Method for the Determination of Micro Quantities of Gibberellic Acid. J. Pharmaceu. Sci. 50, 44–48. doi: 10.1002/jps.2600500110
Gunwantrao, B. B., and Patil, C. R. (2015). Bacterial succession during Panchagavya making as revealed by DGGE analysis. J Pure Appl Microbiol. 9, 3005–3014.
Gupta, J., Rathour, R., Dupont, C. L., Kaul, D., and Thakur, I. S. (2021). Genomic insights into waste valorized extracellular polymeric substances (EPS) produced by Bacillus sp. ISTL8. Environ. Res., 192, 110277. doi: 10.1016/j.envres.2020.110277
Gutiérrez-Mañero, F. J., Ramos-Solano, B., Probanza, A. N., Mehouachi, J.R, Tadeo, F., et al. (2001). The plant-growth-promoting rhizobacteria Bacillus pumilus and Bacillus licheniformis produce high amounts of physiologically active gibberellins. Physiol. Plant. 111, 206–211. doi: 10.1034/j.1399-3054.2001.1110211.x
Kalam, S., Basu, A., and Podile, A. R. (2020). Functional and molecular characterization of plant growth promoting Bacillus isolates from tomato rhizosphere. Heliyon. 6, e04734. doi: 10.1016/j.heliyon.2020.e04734
Kazerooni, E. A., Maharachchikumbura, S. S., Adhikari, A., Al-Sadi, A. M., Kang, S. M., Kim, L. R., et al. (2021). Rhizospheric Bacillus amyloliquefaciens protects Capsicum annuum cv. Geumsugangsan from multiple abiotic stresses via multifarious plant growth-promoting attributes. Front. Plant Sci. 12, 821. doi: 10.3389/fpls.2021.669693
King, E. J (1932). The colorimetric determination of phosphorus. Biochem. 26, 292–297. 10.1042/bj0260292. doi: 10.1042/bj0260292
Krunal, M., and Sanjay, J. (2020). Bacilli consortia positively regulates agronomic and growth traits in rice. Int. J. Chem. Stud. 8, 636–645. doi: 10.22271/chemi.2020.v8.i3h.9278
Kumar, S., Stecher, G., Li, M., Knyaz, C., and Tamura, K. (2018). MEGA X: Molecular Evolutionary Genetics Analysis across computing platforms. Mole. Biol. Evolu. 35:1547–1549. doi: 10.1093/molbev/msy096
Kumari, P., Meena, M., Gupta, P., Dubey, M. K., Nath, G., and Upadhyay, R. (2018). Plant growth promoting rhizobacteria and their biopriming for growth promotion in mung bean (Vigna radiata (L.) R. Wilczek). Biocatal. Agric. Biotechnol. 16, 163–171. doi: 10.1016/j.bcab.2018.07.030
Liyaskina, E. V., Rakova, N. A., Kitykina, A. A., Rusyaeva, V. V., Toukach, P. V., Fomenkov, A., et al. (2021). Production and characterization of the exopolysaccharide from strain PaeniBacillus polymyxa 2020. PloS ONE. 16, e0253482. doi: 10.1371/journal.pone.0253482
Magotra, S., Bhagat, N., Ambardar, S., Ali, T., Hurek, B. R., Hurek, T., et al. (2021). Field evaluation of PGP Bacillus sp. strain D5 native to Crocus sativus, in traditional and non traditional areas, and mining of PGP genes from its genome. Scientific Reports. 11, 1–16. doi: 10.1038/s41598-021-84585-z
Majumdar, S (2017). Evaluation of jute rhizospheric bacteria for plant growth promotion and disease suppression (Doctoral dissertation), University of North Bengal).
Malick, A., Khodaei, N., Benkerroum, N., and Karboune, S. (2017). Production of exopolysaccharides by selected Bacillus strains: Optimization of media composition to maximize the yield and structural characterization. Int. J. Biol. Macromol. 102, 539–549. 0.1016/j.ijbiomac.2017.03.151. doi: 10.1016/j.ijbiomac.2017.03.151
Masclaux-Daubresse, C., Daniel-Vedele, F., Dechorgnat, J., Chardon, F., Gaufichon, L., and Suzuki, A. (2010). Nitrogen uptake, assimilation and remobilization in plants: challenges for sustainable and productive agriculture. Annals Bot. 105, 1141–57. doi: 10.1093/aob/mcq028
Miljaković, D., Marinković, J., and Balešević-Tubić, S. (2020). The significance of Bacillus sp. in disease suppression and growth promotion of field and vegetable crops. Microorganisms. 8, 1037. doi: 10.3390/microorganisms8071037
Mondal, S., Halder, S. K., Yadav, A. N., and Mondal, K. C. (2020). Microbial consortium with multifunctional plant growth-promoting attributes: future perspective in agriculture. Advances in Plant Microbiome and Sustainable Agriculture. 219–258. doi: 10.1007/978-981-15-3204-7_10
Mukhtar, T., Ali, F., Rafique, M., Ali, J., Afridi, M. S., Smith, D., et al. (2022). Biochemical characterization and potential of Bacillus safensis strain SCAL1 to mitigate heat stress in Solanum lycopersicum L. J. Plant Growth Regul. 1–16. doi: 10.1007/s00344-021-10571-4
Mushtaq, Z., Faizan, S., and Hussain, A. (2021). “Role of Microorganisms as Biofertilizers”, in Microbio. and Bioferti. Cham: Springer. p. 83–98. doi: 10.1007/978-3-030-48771-3_6
Nagaraj, N., and Sreenivasa, M. N. (2009). Influence of bacteria isolated from panchagavya on seed germination and seed vigour in wheat. Karnataka J. of Agri. Sci. 22, 231–232.
Natarajan, K (2002). Book on Panchagavya – Panchagavya Manual. Mapusa, Goa, India: Other India Press.
Niu, X., Song, L., Xiao, Y., and Ge, W. (2018). Drought-tolerant plant growth-promoting rhizobacteria associated with foxtail millet in a semi-arid agroecosystem and their potential in alleviating drought stress. Front. Microbiol. 8, 2580. doi: 10.3389/fmicb.2017.02580
Numan, M., Bashir, S., Khan, Y., Mumtaz, R., Shinwari, Z. K., Khan, A. L., et al. (2018). Plant growth promoting bacteria as an alternative strategy for salt tolerance in plants: a review. Microbiol. Res. 209, 21–32. doi: 10.1016/j.micres.2018.02.003
Olanrewaju, O. S., Glick, B. R., and Babalola, O. O. (2017). Mechanisms of action of plant growth promoting bacteria. World J. Microbiol. Biotechnol. 33, 1–16. 10.1007/s11274-017-2364-9. doi: 10.1007/s11274-017-2364-9
Panchal, V. V (2016). Agriculturally beneficial bacterial isolates from panchagavya and establishing their PGP role in chilli (Capsicum annuum L.) (Doctoral dissertation). Anand: Anand Agricultural University).
Pandya, N., and Desai, P. (2013). Gibberellic Acid Production by Bacillus cereus Isolated from the Rhizosphere of Sugarcane. J. Pure Appl. Microbiol. 7, 3239–3242.
Patnaik, H. P., Dash, S. K., and Shailaja, B. (2012). Microbial composition of Panchagavya. J. Eco. Agri. 7, 101–103.
Pikovskaya, R. I (1948). Mobilization of phosphorus in soil in connection with the vital activity of some microbial species. Available online at: https://ci.nii.ac.jp/naid/10026513896/
Radha, T. K., and Rao, D. L. N. (2014). Plant growth promoting bacteria from cow dung based biodynamic preparations. Indian J. Microbiol. 54, 413–418. doi: 10.1007/s12088-014-0468-6
Ram, R. A., Ahmad, I., and Kumar, A. (2019). Isolation and characterization of PGPRs from organic preparations. J. Eco. Agricul. 14, 18–22. Available online at: http://krishi.icar.gov.in/jspui/handle/123456789/55317
Sairam, R. K., Rao, K. V., and Srivastava, G. C. (2002). Differential response of wheat genotypes to long term salinity stress in relation to oxidative stress, antioxidant activity and osmolyte concentration. Plant Sci. 163, 1037–1046. doi: 10.1016/S0168-9452(02)00278-9
Saitou, N., and Nei, M. (1987). The neighbor-joining method: a new method for reconstructing phylogenetic trees. Mol. Biol.Evol. 4, 406–425. 10.1093/oxfordjournals.molbev.a040454.
Saleem, S., Iqbal, A., Ahmed, F., and Ahmad, M. (2021). Phytobeneficial and salt stress mitigating efficacy of IAA producing Salt Tolerant strains in Gossypium hirsutum. S audi J. Biol. Sci. 10.1016/j.sjbs.2021.05.056. doi: 10.1016/j.sjbs.2021.05.056
Salwan, R., Sharma, V., Saini, R., and Pandey, M. (2021). Identification of plant beneficial Bacillus sp. for Resilient agricultural ecosystem. Cur. Res. in Micro. Sci. 2, 100046. doi: 10.1016/j.crmicr.2021.100046
Shifa, H., Gopalakrishnan, C., and Velazhahan, R. (2015). Efficacy of Bacillus subtilis G-1 in suppression of stem rot caused by Sclerotium rolfsii and growth promotion of groundnut. Int. J. Environ. 8, 111–118. doi: 10.5958/2230-732X.2015.00015.7
Shultana, R., Kee Zuan, A. T., Yusop, M. R., and Saud, H. M. (2020). Characterization of salt-tolerant plant growth-promoting rhizobacteria and the effect on growth and yield of saline-affected rice. Plos ONE. 15, e0238537. doi: 10.1371/journal.pone.0238537
Syed, S., Tollamadugu, N. P., and Lian, B. (2020). Aspergillus and Fusarium control in the early stages of Arachis hypogaea (groundnut crop) by plant growth-promoting rhizobacteria (PGPR) consortium. Microbiol. Res. 240, 126562. doi: 10.1016/j.micres.2020.126562
Tamura, K., Nei, M., and Kumar, S. (2004). Prospects for inferring very large phylogenies by using the neighbor-joining method. Proc. Natl. Acad. Sci. U.S.A (USA). 101, 11030–11035. doi: 10.1073/pnas.0404206101
Tohidifar, P., Plutz, M. J., Ordal, G. W., and Rao, C. V. (2020). The mechanism of bidirectional pH taxis in Bacillus subtilis. J. Bacteriol. 202, e00491–19. 10.1128/JBacillus00491-19. doi: 10.1128/JB.00491-19
Vardharajula, S., Zulfikar Ali, S., Grover, M., Reddy, G., and Bandi, V. (2011). Drought-tolerant plant growth promoting Bacillus sp.: effect on growth, osmolytes, and antioxidant status of maize under drought stress. J. Plant Interact. 6, 1–14. doi: 10.1080/17429145.2010.535178
Verma, P., Yadav, A. N., Kumar, V., Singh, D. P., and Saxena, A. K. (2017). Beneficial plant-microbes interactions: biodiversity of microbes from diverse extreme environments and its impact for crop improvement. Plant-Micro. Interct. Agro-eco. Perspe. Springer, Singapore. 543–580. doi: 10.1007/978-981-10-6593-4_22
Wang, X., Xie, H., Ku, Y., Yang, X., Chen, Y., Yang, N., et al. (2020). Chemotaxis of Bacillus cereus YL6 and its colonization of Chinese cabbage seedlings. Plant Soil. 447, 413–430. doi: 10.1007/s11104-019-04344-y
Zahid, M (2015). Isolation and identification of indigenous plant growth promoting rhizobacteria from Himalayan region of Kashmir and their effect on improving growth and nutrient contents of maize (Zea mays L.). Front. Microbiol. 6, 207. doi: 10.3389/fmicb.2015.00207
Keywords: Arachis hypogea (Ground nut), Bacillus sp. PG-8, fermented panchagavya, cow products, liquid biofertilizer
Citation: Gohil RB, Raval VH, Panchal RR and Rajput KN (2022) Plant Growth-Promoting Activity of Bacillus sp. PG-8 Isolated From Fermented Panchagavya and Its Effect on the Growth of Arachis hypogea. Front. Agron. 4:805454. doi: 10.3389/fagro.2022.805454
Received: 30 October 2021; Accepted: 31 January 2022;
Published: 04 March 2022.
Edited by:
Avishek Banik, Presidency University, IndiaReviewed by:
Subhrangshu Mandal, University of Minnesota Twin Cities, United StatesSudipta Maity, University of Rajasthan, India
Copyright © 2022 Gohil, Raval, Panchal and Rajput. This is an open-access article distributed under the terms of the Creative Commons Attribution License (CC BY). The use, distribution or reproduction in other forums is permitted, provided the original author(s) and the copyright owner(s) are credited and that the original publication in this journal is cited, in accordance with accepted academic practice. No use, distribution or reproduction is permitted which does not comply with these terms.
*Correspondence: Kiransinh N. Rajput, cmFqcHV0a24mI3gwMDA0MDtndWphcmF0dW5pdmVyc2l0eS5hYy5pbg==; cmFqcHV0a24mI3gwMDA0MDt5YWhvby5jb20=