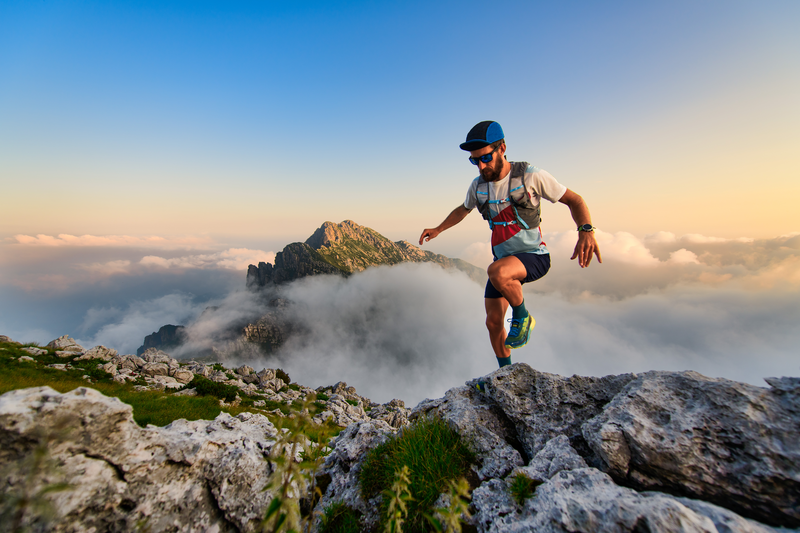
94% of researchers rate our articles as excellent or good
Learn more about the work of our research integrity team to safeguard the quality of each article we publish.
Find out more
SYSTEMATIC REVIEW article
Front. Agron. , 28 June 2022
Sec. Plant-Soil Interactions
Volume 4 - 2022 | https://doi.org/10.3389/fagro.2022.800365
Human population growth requires food production to increase at a matching pace. Crop productivity largely depends on GEM reciprocity and variation in any factor may potentially alter the overall response. Introduction of improved cultivars causes interactive responses within the farming system which may produce tangible benefits only in the presence of suitable environments and management practices. The yield gap which is defined as the difference between yield potential and average farm yield varies in extent among regions ranging from moderately high to alarmingly high. Variation in environments and management practices impacts both the quantity and quality of produce. The highest gains can be achieved if factors in the interaction model work complementarily. System efficiency indicators are useful for assessing the overall system performance. In the face of new challenges plant breeding is receptive to shifts in objectives and strategy. Extension experts are trained to transfer technology and help farmers optimise for better gains. The extension service is also crucial for feedback to researchers. Better crop management has helped realize the genetic potential of crop cultivars in specific settings. Once a productivity plateau has been reached following optimization of management practices for specified environments, then further improvement of the system can be attained through new genetic interventions. For higher productivity a stronger linkage among researchers, extension experts, and farmers is vital. To help the decision support systems GEM interactions need precise scientific analysis and interpretation. A general account rather than a specific view on GEM reciprocity is presented.
Genotypes, environments, and management practices are the factors that determine crop productivity (Beres et al., 2020). These factors need to be optimised precisely to attain best crop performance as variation in any factor could result in a swayed response. A detailed discussion of the various factors that influence this interaction follows:
Crop cultivars are generally compatible with specific environments and management practices (Kang, 1998). To select cultivars for a specific cropping system the growers need the best available genetic materials, ideally bred locally for these conditions. Improved crop cultivars grown in the right environment using optimized management practices will offer the highest value to the farmer (Monjardino et al., 2019). Nevertheless, examples of broad adaptation show that crop cultivars can be bred in one location and released and grown extensively in another (Trethowan et al., 2001; Trethowan et al., 2003). Such broad adaptation arose from the Green Revolution and the deployment and release of semi-dwarf wheat and rice cultivars globally (Trethowan et al., 2007; Oladosu et al., 2017). These gains were realised across a wide range of environments. However, recent gains in productivity of major crops have been much lower and future gains will likely be environment specific (Monjardino et al., 2019), requiring exploitation of the GEM interactions. Farmers may need to have a relatively large set of crop cultivars available to them to select one most suitable for the site.
Subsistence farmers grow produce locally that is used domestically, whereas corporate farmers tend to produce bulk commodity for national and even international markets. The quantity and quality of the produce, therefore, determines its market value which translates into cost-benefit ratios of the farming system.
Genetic diversity helps genetic gain among progenies [Yunbi et al., 2017], and lack of diversity or overlapping of genetic materials may lower the gain. When comparing BC1 and F2 foundation populations derived from 18 different crosses of adapted and exotic maize dent lines a study showed that repeated recombination of F2 had non-significant effect on mean performance and variance (Simic et al., 2003).
Rising atmospheric CO2 is driving temperatures and causing water stress which may impact future crop productivity. Simultaneously the CO2 rise has some beneficial aspects for the crops and C3 crops may directly increase photosynthesis by elevated CO2 however C4 crops may benefit in times and places of drought stress (Leakey, 2009).
Many studies recognize the primary role of temperature in determining the C3/C4 distributions and the success of the crossover temperature model in predicting these distributions. The studies acknowledge that most of the C4 plants were restricted to the herbaceous growth habit (Still et al., 2003).
An investigation involving maize, sorghum, winter wheat, and soybean crops conducted under full irrigation regime reported that the crop water productivity for C4 crops was 89% higher than their C3 counterparts (Kukal and Irmak, 2020).
With the discovery of C4 mechanism a couple of decades ago, an interest emerged in the research community to introduce this mechanism into C3 crops for higher productivity. The progress so far has been slow due to complexity of the processes involved (Cui, 2021; Covshoff and Hibberd, 2012). It is hard to estimate the exact time frame to change C3 anatomy and integrate the C4 photosynthetic cycle since C4 engineering hinges on more precise genome modification technologies, harmonious introduction of multigene set up into the plant, and ability to control gene expression in space and time (Schuler et al., 2016).
Crop yields are affected by soil type, tillage, climate, and the farmer decisions on crop management, crop choice and varietal selection. Various factors outside the control of farmers and landscape characteristics also significantly affect the yields (Bommarco et al., 2013; Perez-Alvarez et al., 2018). While reviewing the factors influencing crop yields Liliane and Charles (2020) suggested that yield influencing factors other than crop choice and varietal selection, could be grouped into three broader categories; a) technological (agricultural practices, managerial decision, etc.), b) biological (diseases, insects, pests, weeds), and c) environmental (climatic condition, soil fertility, topography, water quality, etc.).
Atmospheric temperature is an important determinant of plant development. Both extremely high and low temperatures adversely affect crop growth (Wahid et al., 2007). Under high temperatures the phenological development of plants is accelerated (Hatfield and Prueger, 2015). High temperature adversely affects pollen viability and fruit setting in tomato (Aref and John 1995) and other crops (Devasirvatham et al., 2013; Jha et al., 2014). High temperature stress lowers the sugar content of fruit, flower maintenance, and fruit quality and quantity is reduced (Alsamir et al., 2021).
The optimum temperature for growth varies among species (Hatfield and Prueger, 2015). Maximum and minimum temperatures in the 24hr cycle influence plant development depending on the severity of the temperature. The difference in night and day temperature significantly affects growth in ornamentals (Ohtaka et al., 2020) and other crops (Devasirvatham et al., 2016).
Solar radiation provides light and heat for photosynthesis. Poor solar radiation undermines photosynthesis whereas suitable conditions help achieve higher productivity (Aguilera et al., 1999). The relationship between intercepted solar radiation and biomass production is presented in Figure 1.
Figure 1 Relationship between biomass produced and amount of solar radiation intercepted by rapeseed crops between sowing and flowering (open symbols) and between flowering and harvest (closed symbols). Different symbols denote different cultivars and numbers next to each symbol indicate order of sowing. Lines drawn indicate RUE 1.0 and 1.5 gMI-1. (Adopted from: Mendham et al., 1990).
Although under low intensity solar radiation growth is slower compared to optimum levels, high intensity solar radiation may stress the plant, impacting growth and development. The solar radiation intensity utilised by plants for photosynthesis ranges from 379 to 710nm, and the PAR fraction is place and time specific (Amthor, 2010).
Solar radiation also determines crop phenology and ultimate production (Villegas et al., 2016). The prevalent solar radiation impacts the cropping system of a region (Xia et al., 2014). However, significant variation among genotypes for the induction of photosynthesis in varying levels of light has been observed, indicating that this trait can be optimised genetically in some species (William et al., 2019). Thus, G can be manipulated and to a lesser extent M in the GEM interaction to optimise the use of light.
Productive cropping requires suitable soils (Berg and Smalla, 2009). Soil provides anchorage and supplies aeration, nutrients and water to the roots (Figure 2). However, soils differ in their mineral elements and enzymes (Quan and Liang, 2017) and different plant species respond differently to various soil types (Markham et al., 2009). Favourable root environments can allow crop cultivars to express their maximum genetic potential (Abdul-Khalil et al., 2015).
Figure 2 Growing media provides anchorage. aeration, water and nutrients. (Adopted from: Dumroese et al., 2008).
However, greenhouse crop production required a suitable growing medium and unlike field production, the medium used is homogenous. Shallow depth and limited volume of the container necessitates amendment of the growing medium to provide appropriate physical and chemical properties for plant development. Field soils are generally unsuitable for plant production in containers, primarily because they do not provide the adequate aeration, drainage and water holding capacity. Several “soilless” growing medias have been developed to solve this problem (Aggie, 2021). For enhanced plant performance, the depths and attributes of various substrates need to be carefully considered when designing the root media (Kazemi and Mohorko, 2017). However, the E and M components of the GEM interaction can be controlled in these systems. Thus genetics is the primary way to increase productivity once these factors have been optimised.
Relative humidity is an important factor which impacts rice response to temperature (Stuerz and Asch, 2021) and is likely to impact other crops. High relative humidity favours plant growth, however, the response was stronger in light compared to dark conditions (Hirai et al., 2000). The number of flower and boll production in cotton was positively correlated with lower humidity (Sawan, 2018). Atmospheric CO2 also affects plant development; however, the response is complex. Increasing C02 will likely increase plant growth and yield (Taub, 2010; Reddy et al., 2010), but the associated impacts of higher temperature and more intense drought will likely negate any benefit (Bloomfield et al., 2019). In these instances, the E component of the GEM interaction will likely drive plant responses.
Water, nutrients, and plant protection are essential for successful crop production. Effective crop management needs precision agriculture principles to be effectively adopted. With precision agriculture new technologies are adopted that use inputs more efficiently and increase production (Finch et al., 2014). Three major precision agricultural technologies include GPS guidance machine control systems, GPS yield and soil maps, and variable-rate input application technologies (USDA, 2021). The precision agriculture technology adoption in USA is illustrated in Figure 3. However, there can be significant genotype x management practice interaction and these should be optimised. For example, sowing crops on raised beds can improve irrigation efficiency of rice and wheat crops (Kukal et al., 2010). Nevertheless, this change required cultivars that had spreading canopies rather than the upright habits better suited to sowing in dense stands on flat land (Fischer et al., 2019).
Figure 3 US dealerships using Global Navigation Satellite Systems (GNSS) guidance and sprayer section controllers. 2004 to 2017. (Adopted from: DeBoer and Erickson, 2019).
Agricultural practices to improve growth, development and crop yields are referred to as crop management. The management practices include seedbed preparation, sowing of seeds, addition of fertilisers and manures, irrigation, crop care and harvesting, storage of the produce and marketing. Best crop management practices help improve productivity and contribute to greater profitability.
The term “best management practice” is used in a variety of contexts and it flexibly transforms its objectives depending on the situation across different crops and geographic regions. Not every best practice is suitable universally and the adequacy may vary across geographic borders. Some widely used best management practices (Singh et al., 2021; Mahmud et al., 2021; Dhaliwal et al., 2021; Liava et al., 2022; and Cottney et al., 2022) for better crop productivity, profitability, and environmental protection may include:
- conservation tillage
- contour farming on slopy lands
- erosion and sedimentation control
- cover cropping
- use of better adapted cultivars
- integrated pest management
- irrigation and nutrient management
- crop rotation
- rotational grazing
- effective marketing
- waste management
- wildlife habitat management
Effects due to various factors in the GEM equation will need to be explained carefully when interactions are observed to be significant. It is likely that these interactions are region or even farm specific and will require expertise to interpret in consultation with the farmer. A simple illustration of a three-way interaction is presented in Figure 4. In most cases, the farmer can optimise the G and M components of the interaction using the most probable E (based on long-term records) as the baseline.
Figure 4 Linear responses of grain yield (GY) to P fertilizer for soil 'N'P intereactions with two soil types; black soil and chestnuts soil (Adopted from: Vargas et al., 2015).
The yield gap can be defined as the difference between yield potential and average farm yield expressed in kg ha–1 (Aggarwal et al., 2008). Innovative and efficient use of resources can increase farm profitability and enhance the system sustainability. Significant yield gaps in different crops have been reported in Australia (Figure 5), but the extent may differ in different countries. Clearly, optimal selection of genotypes and management practices for the most probable environment type in any region will decrease yield gaps in most years. Interestingly, on farm wheat yield has been relatively static in Australia for many years (https://www.csiro.au/en/news/news-releases/2017/australias-wheat-yields-stalled Retrieved 28.05.2021). However, this statistic did not tell the whole story. The production environment over the period of the study has also declined due to climate change. Farmers were able to maintain productivity under these conditions by adopting better management practices, such as reduced tillage, and better adapted cultivars (Chancellor et al., 2021).
Figure 5 Yield as reported by Yield Gaps Australia (https://yieldgapaustralia.com.au/maps/Retrieved 28.05.21).
A web based interactive application on yield gap with various crops has been presented by Yield Gap Australia.
At farm level, the following guidelines can help improve efficiency and productivity:
● Evaluation of local adaptation trials. Many countries have national trial networks for the identification of cultivars for release. Extension staff could help interpret these results or organise independent testing. This will help identify suitable G.
● Factors needing improvement could be identified periodically at the farm level through regular monitoring. These include rates of fertilizer and chemical applications, tillage practices, controlled traffic and crop rotations to manage diseases and fertility. This will help optimise M.
● Access information from extension professionals, field days and demonstrations, handouts, online data and contact with successful early technology adopters (Figure 4).
● Maintain good farm weather records long-term. These records, when combined with regional records provided by the Bureau of Meteorology, provide estimates of the likely environment type for the farm or region. This provides a baseline estimation of E.
Causes of yield gap vary among crops and regions. One example each from Australia and Pakistan representing widely different locations and socio-economic backgrounds is briefly discussed here.
Australian farming is characterised by large farm size and mechanised operations (Sheng and Chancellor, 2019; Australia Agronomy, 2019). A systematic study using the shire-level data found high yield gaps in Australia and on average a yield gap of 55% was reported (Hochman et al., 2016). The yield gap could be tackled by addressing the causes using improved technologies including new varieties, new agronomic or management techniques, and timely decisions by the farmer. The national crop variety trials are generally conducted using the best current management practices in the country and therefore demonstrate the best available technologies locally. Limited knowledge with farmers regarding causes of yield gap is a major reason of low progress but shortage of resources to implement the technological advancements is also evident in certain instances (Fischer et al., 2014). Figure 6 elaborates the progress with yield gap in Australia over time.
Figure 6 Progress with farm grain yield (FY) and water-limited potential grain yield (PY) of spring wheat in Western Australian over time. PY plot shows progress against year of variety release. The slope difference between FY and PY indicates the yield gap reduction over the years (Source: FY from ABARES (Australian Bureau of Agricultural and Resource Economics and Sciences (2012) and PY from NVT (National Variety Trials) (2009). Adopted from Fischer et al., 2014. **0.01. < P < 0.05, ***P < 0.01.
Small farm size and low mechanisation is a dominant feature with Pakistani farm sector (Rehman et al., 2016). Although yields have improved recently the yield gap has shown little improvement in the country (CIMMYT, 2021). Out of grain crops the highest yield gap was recorded in Maize, followed by wheat and rice (Khan et al., 2021) with only 42% of the current potential yield harvested in wheat (ZTBL, 2020).
The crop production constraints are diverse; some are regional while others are crop specific or may arise from the socio-economic background of the farmers. Some general constrains include soil fertility, poor weed management, soil degradation, drought stress, poor crop protection, financing problems, and poor marketing. In Asian rice-wheat system factors including late planting, heat during grain fill, weed competition, leaf and stem fungal diseases (other than rusts), use of unsuitable variety, unavailability of quality seed, inadequate farmer training, soil fertility depletion, inappropriate use and management of fertilizers accounted for 47% of the yield gap (Waddington et al., 2010). The study identified many interactions and cause and effect relationships which accounted for rest of the 53% yield gap.
Unavailability of inputs and high input costs, limited access to credit facilities, and adverse weather conditions are common in some developing countries (Quddus and Kropp, 2020) including Pakistan. Other constraints in developing countries may include, crop damage by wildlife, human disease pandemics, and lack of infrastructure to transport produce (Makuvaro et al., 2017). These constraints generally impact G and M in the GEM interaction.
The crop quality, value addition, and better marketing bring dividends to the farmers. However, to reap those dividends farmers need to be trained with best practices. Izaba (2021) analysed factors determining value addition, and market access and suggested that market access along with farm size, farmer’s networks, farmer’s perceptions, and employment growth influences were the significant drivers to value addition technology adoption. It was noticed that farmers adopting value addition technologies tended to experience economic growth.
Barriers with establishing a value-added business could be categorised as: regulations, resources, and marketing. Farmers perceive regulations as complicated, ambiguous, and restrictive (Chen et al, 2021).
The efficiency of a GEM system may be analysed by assessing its various components individually. Although the individual assessments may only partially portray the GEM efficiency, they still indicate the progress made in the technology adoption process. A diligent efficiency analysis will help monitor the system more effectively (Olesen et al., 2011).
GEM system includes genetic factors (e.g. crop cultivars), environments (e.g. growing conditions), and crop management (e.g. various inputs). Better quality produce may deliver higher dividends and marketing techniques including better presentation, value addition, and the right time of marketing were important (Cucagnaa and Goldsmith, 2018). As corporate farming aims at higher profits it seems prudent that the GEM system also factors in the marketability of the produce.
Adoption indicators depict the effectiveness of the adoption process. The process is considered effective when farmers benefit adequately. The various indicators are summarised in Table 1.
Most crop production systems generally use sub-optimal management practices, although these are often better implemented in protected environments. Protected cropping uses modified growing conditions providing protection from pests, diseases, and adverse weather. Protected cropping could increase profitability and productivity through high-value fresh produce (DPI, 2021). Maintaining best practices under protected environments helps maintain high productivity but how to bring further improvement when at plateau?
Further improvement may require a new genetic intervention which is superior to previously available options. These new cultivars will give rise to a new GEM reciprocity and help the system evolve positively. Through repeated cropping cycles the system will gradually improve and lead to higher productivity.
Successful breeding strategies assisted the development of crop cultivars that helped the Green Revolution materialise in the 20th century (Pingali, 2012). Plant breeding helps improve crops by refining the genetic makeup of the plants. Various authors have defined plant breeding objectives and suggested crop improvement strategies recently (Trethowan et al., 2010; FSANZ, 2013; Messmer, 2015; Allard, 2019).
Traditionally, improved cultivars have better agronomic type, tolerance against abiotic and biotic stresses, shorter maturity period, capacity to compete with weeds, lower water usage, and improved quality (Baenziger et al., 2006). Food crop cultivars are also required to have high yield, meet dietary standards and be compatible with the prevalent cropping systems (Pfeiffer and McClafferty, 2007). Contemporary plant breeding needs to address sustainability, lifestyle changes, and overall productivity of the systems (Voss-Fels et al., 2019; PFMPB, 2021; Hong and Jiayang, 2021). To respond to these challenges contemporary plant breeding needs to promote:
● Cultivars promising higher productivity both in the open field and under protected cropping. Reducing the genotype-phenotype gap is vital for improved productivity.
● Cultivars compatible with sustainable farming systems (organic farming, conservation agriculture).
● Cultivars responsive to higher atmospheric CO2 concentrations.
● Conservation of crop genetic diversity.
● Efficient use of plant breeding tools including transgenics, genomics, and genetic code editing.
● Improved systems of data capture, management and use including machine learning.
● Mechanical tools to deliver speedy and cost-effective cropping operations.
● Multidisciplinary research to deliver more effective outcomes.
Various conditions including temperature, solar radiation, and soil media define the crop environment. These conditions generally go uninterrupted under field settings. However, under protected cropping such conditions could be optimised to meet production targets (FFS, 2021). Ideally cop management needs to follow the production protocols specified when releasing the cultivars (Zabel et al., 2021). As conditions like temperature, light intensity, and soil substrate are manageable under protected cropping the interaction effects could be better designated as GxM rather than GxE interaction. To better explain the interactions Cooper et al. (2021) suggested a split of the GEM model into (GxM)xE components.
The GEM model depicting wider interactions in the system was proposed by Hatfield and Walthall, 2015. The model defines the boundaries of the interactions and may provide scientific explanations to researchers, extension experts and farmers who have been working to explain the complexity of the interactions differently (Hoffmann et al., 2007).
Multiple layers of each factor exist in the system and interactions within the GEM model need to be studied and explained holistically. To precisely explain the interactions, input from a whole spectrum of disciplines including plant breeding, agronomy, plant protection, biometry, engineering, food science, and extension is needed.
Precise scientific response to GEM analysis was possible once all possible interactions in the system were adequately explained and sources of low and high performance identified. For further elaboration a typical example from a small farm is presented (Table 2). The farmer at this typical farm adopted certain practices (bold faced) but it was uncertain if these practices were optimal at this specific site. A logical way to determine this could be to generate data through well planned GEM experiments at the farm. With three factors, each having multiple layers, the precise analysis would be a challenge. However, decoupling factors into GxE, GxM, and ExM components may help explain the interactions (Walthall et al., 2016). Interestingly, splitting the multi-layered GEM model into components may only partially explain the interactions.
To attain maximum productivity in a GEM system researchers and extension experts need to work closely with the farmers (Shelton and Tracy, 2016), to identify a precise production technology for every individual cultivar (Passioura, 2020).
Although extension is generally considered a one-way process in which the extension experts transfer technology and new ideas to farmers, feedback from farmers to extension and research experts can be vital. Effective linkage between extension experts and researchers also represents a two-way process that could enhance productivity. The two-way flow of ideas could happen at the time a problem is defined, when testing the recommendations, and when recommendations are adopted (FAO, 2021a).
Agricultural extension is regarded as a broader knowledge system that includes research and agricultural education. FAO and the World Bank refer to this larger system as AKIS/RD (Agricultural Knowledge and Information Systems for Rural Development) (FAO, 2021b).
Defining a comprehensive model to analyse the interactions holistically is a challenge (Cooper et al., 2021). Collaborative work among researchers, extension experts, and farmers may not adequately explain various interactions unless scientific analysis at every stage of the process was made available.
More scientific explanation on GEM reciprocity is required. However, this should not stop coordinated efforts to get stakeholders work together to develop site-specific transition strategies. To address the needs of the system, the slightly different approaches used in each region must be understood (Hoffmann et al., 2007) as their likelihood of success will vary.
Traditionally research and extension have been in the public sector in Australia. Recently, the balance shifted away from the public to private sector. Currently, research and extension exist in a shared domain between the private and public sectors (https://rirg.fvas.unimelb.edu.au/ag-extension/home Retrieved 11.09.2021). The private sector is an active part of crop research, and extension and is considered to be a reliable source of information on crop R&D (RIRG, 2021a).
In the absence of a ready to use scientific solution for explaining interactions at every level, a step by step strategy has emerged. The Australian system used a strategy which is gradually improving (DAWE, 2021a). The Australian farming system is evolving under increasingly hostile production conditions, yet crop productivity has improved significantly over time (DAWE, 2021b).
Operating a private-public sector partnership, the Australian system allows researchers and extension staff to work under the same umbrella or in the same team. Farmers are part of the consultative process during the formulation of research and extension strategies (RIRG, 2021b). New technology is rolled out through extension experts who conduct demonstration trials before adoption in farmer’s field. Demonstration sites form an important bridge between agricultural research and the adoption of the new technology through site specific testing and evaluation at commercial scale (AFA, 2021). The Australian and other similar examples (FM, 2021) may help pave the way for more responsive strategies to improve productivity of diverse GEM systems.
The original contributions presented in the study are included in the article/supplementary material. Further inquiries can be directed to the corresponding author.
TM developed the concept and prepared the manuscript. TA and RT contributed towards improvement of the original ideas. All authors contributed to the article and approved the submitted version.
The authors declare that the research was conducted in the absence of any commercial or financial relationships that could be construed as a potential conflict of interest.
All claims expressed in this article are solely those of the authors and do not necessarily represent those of their affiliated organizations, or those of the publisher, the editors and the reviewers. Any product that may be evaluated in this article, or claim that may be made by its manufacturer, is not guaranteed or endorsed by the publisher.
ABARES (Australian Bureau of Agricultural and Resource Economics and Sciences). (2012). Available at: www.abares.gov.au.
Abdul-Khalil H. P. S., Hossain M. S., Rosamah E., Azli N. A., Saddon N., Davoudpoura Y., et al. (2015). The Role of Soil Properties and its Interaction Towards Quality Plant Fiber: A Review. Renewable Sustain. Energy Rev. 43, 1006–1015. doi: 10.1016/j.rser.2014.11.099
AFA (2021). Available at: https://extensionaus.com.au/extension-practice/demonstration-sites-extension/.
Aggarwal P. K., Hebbar K. B., Venugopalan M. V., Rani S., Bala A. (2008). Quantification of yield gaps in rain-fed rice, wheat, cotton and mustard in India. Glob. Theme Agroecosyst. Rep. 43, Int. Crops Res. Inst. Semi-Arid Tropics (ICRISAT), Andhra Pradesh, India.
Aggie (2021) Aggie Horticulture. Available at: https://aggie-horticulture.tamu.edu/ornamental/greenhouse-management/growing-media/.
Aguilera J., Karsten U., Lippert H., Vogele B., Philipp E., Dieter H., et al. (1999). Effects of Solar Radiation on Growth, Photosynthesis and Respiration of Marine Macroalgae From the Arctic. Mar. Ecol. Prog. Ser. 191, 109–119. doi: 10.3354/meps191109
Allard R. W. (2019) Plant Breeding. Encyclopedia Britannica. Available at: https://www.britannica.com/science/plant-breeding (Accessed 30 August 2021).
Alsamir M., Mahmood T., Trethowan R., Ahmad N. (2021). An Overview of Heat Stress in Tomato (Solanum Lycopersicum L.). Saudi J. Biol. Sci. 28, 1654–1663. doi: 10.1016/j.sjbs.2020.11.088
Amthor J. S. (2010). From Sunlight to Phytomass: On the Potential Efficiency of Converting Solar Radiation to Phyto-Energy. New Phytol. 188, 939–959. doi: 10.1111/j.1469-8137.2010.03505.x
Australia, Agronomy (2019). Australian Agriculture in 2020: From Conservation to Automation. (Wagga Wagga NSW, Australia: Graham Centre for Agricultural Innovation, Charles Sturt University)
Baenziger P. S., Russell W. K., Graef G. L., Campbell B. T. (2006). Improving Lives: 50 Years of Crop Breeding, Genetics, and Cytology (C-1). Crop Sci. 46, 2230–2244. doi: 10.2135/cropsci2005.11.0404gas
Beres B. L., Hatfield J. L., Kirkegaard J. A., Eigenbrode S. D., Pan W. L., Lollato R. P., et al. (2020). Toward a Better Understanding of Genotype × Environment × Management Interactions—A Global Wheat Initiative Agronomic Research Strategy. Front. Plant Sci. 11. doi: 10.3389/fpls.2020.00828
Berg G., Smalla K. (2009). Plant Species and Soil Type Cooperatively Shape the Structure and Function of Microbial Communities in the Rhizosphere. FEMS Microbiol. Ecol. 68, 1–13. doi: 10.1111/j.1574-6941.2009.00654
Bloomfield J. P., Marchant B. P., McKenzie A. A. (2019). Changes in Groundwater Drought Associated With Anthropogenic Warming. Hydrology. Earth System. Sci. 23 (3), 1393–1408. doi: 10.5194/hess-23-1393-2019
Bommarco R., Kleijn D., Potts S. G. (2013). Ecological Intensification: Harnessing Ecosystem Services for Food Security. Trends Ecol. Evol. 28, 230–238. doi: 10.1016/j.tree.2012.10.012
Chancellor W., Hughes N., Zhao S., Soh W. Y., Valle H., Boult C. (2021). Controlling for the Effects of Climate on Total Factor Productivity: A Case Study of Australian Farms. Food Policy 102, 102091. doi: 10.1016/j.foodpol.2021.102091
Chen H., Ellett J. K., Phillips R., Feng Y. (2021). Small-Scale Produce Growers’ Barriers and Motivators to Value-Added Business: Food Safety and Beyond. Food Control. 130, 108192. doi: 10.1016/j.foodcont.2021.108192
CIMMYT (2021) Five Big Steps Toward Wheat Self-Sufficiency in Pakistan. Available at: https://www.cimmyt.org/news/five-big-steps-toward-wheat-self-sufficiency-in-pakistan/.
Cooper M., Voss-Fels K. P., Messina C. D., Tang T., Hammer G. L. (2021). Tackling G × E × M Interactions to Close on-Farm Yield-Gaps: Creating Novel Pathways for Crop Improvement by Predicting Contributions of Genetics and Management to Crop Productivity. Theor. Appl. Genet. 134, 1625–1644. doi: 10.1007/s00122-021-03812-3
Cottney P., Black L., Williams P., White E. (2022). How Cover Crop Sowing Date Impacts Upon Their Growth, Nutrient Assimilation and the Yield of the Subsequent Commercial Crop. Agronomy 12, 369. doi: 10.3390/agronomy12020369
Cucagnaa M. E., Goldsmith P. D. (2018). Value Adding in the Agri-Food Value Chain; Research Article. Int. Food Agribusiness. Manage. Rev. 21, 3. doi: 10.22434/IFAMR2017.0051
DAWE (2021a) Department of Agriculture, Water, and Environment. Available at: https://www.agriculture.gov.au/ag-farm-food/innovation.
DAWE (2021b). Available at: https://www.agriculture.gov.au/abares/research-topics/productivity/agricultural-productivity-estimates.
DeBoer J. L., Erickson B. (2019). Setting the Record Straight on Precision Agriculture. Agron. J. 111), 1552–1569. doi: 10.2134/agronj2018.12.0779
Devasirvatham V., Gaur P. M., Mallikarjuna N., Raju T. N., Trethowan R. M., Tan D. K. Y. (2013). Reproductive Biology of Chickpea Response to Heat Stress in the Field is Associated With the Performance in Controlled Environments. Field Crops Res. 142, 9–19. doi: 10.1016/j.fcr.2012.11.011
Devasirvatham V., Tan D. K. Y., Trethowan R. M. (2016). “Breeding Strategies for Enhanced Plant Tolerance to Heat Stress,” in Advances in Plant Breeding Strategies: Agronomic, Abiotic and Biotic Stress Traits. Ed. Al-Khayri J. M. (Springer International PublishingSwitzerland), pp 447–pp 469. doi: 10.1007/978-3-319-22518-0_12
Dhaliwal S. S., Sharma S., Sharma V., Shukla A. K., Walia S. S., Alhomrani M., et al. (2021). Long-Term Integrated Nutrient Management in the Maize–Wheat Cropping System in Alluvial Soils of North-Western India: Influence on Soil Organic Carbon, Microbial Activity and Nutrient Status. Agronomy 11, 2258. doi: 10.3390/agronomy11112258
DPI (2021) Department of Primary Industries. Available at: https://www.dpi.nsw.gov.au/agriculture/horticulture/greenhouse.
Dumroese R. K., Luna T., Landis T. D. (2008). Nursery Manual for Native Plants: Volume 1, a Guide for Tribal Nurseries. Agriculture Handbook 730 (Washington, DC: U.S. Department of Agriculture, Forest Service), 302 p.
FAO (2021a). Available at: http://www.fao.org/3/t0060e/T0060E03.htm.
FAO (2021b). Available at: http://www.fao.org/3/y2709e/y2709e05.htm.
FFS (2021) Future Food Systems. Available at: https://www.futurefoodsystems.com.au/wp-content/uploads/2021/04/P2-004-Protected-cropping.pdf.
Finch H. J. S., Samuel A. M., Lane G. P. F. (2014). “Precision Farming,” in Woodhead Publishing Series in Food Science, Technology and Nutrition, Lockhart & Wiseman’s Crop Husbandry Including Grassland, Ninth Edition (Cambridge, UK: Woodhead Publishing), p: 235–p: 244. Available at: https://www.sciencedirect.com/science/article/pii/B9781782423713500102, ISBN: ISBN 9781782423713. doi: 10.1533/9781782423928.2.235
Fischer R. A., Byerlee D., Edmeades G. (2014). Crop Yields and Global Food Security (Canberra, ACT: ACIAR), 8–11.
Fischer R. A., Ramos O. H. M., Monasterio I. O., Sayre K. D. (2019). Yield Response to Plant Density, Row Spacing and Raised Beds in Low Latitude Spring Wheat With Ample Soil Resources: An Update. Field Crops Res. 232, 95–105. doi: 10.1016/j.fcr.2018.12.011
FM. (2021). FARMENTOR. Available at: https://farmentor.com/research-extension-growers-collaboration-israels-key-for-success/.
FSANZ. (2013). New Plant Breeding Techniques: A Workshop Hosted by Food Standards Australia New Zealand (Kingston, Australia & Wellington, New Zealand: FSANZ Australia, Kingston ACT 2604). Available at: https://www.foodstandards.gov.au/publications/Pages/New-plant-breeding-techniques-workshop-report.aspx.
Hatfield J. L., Prueger J. H. (2015). Temperature Extremes: Effect on Plant Growth and Development. Weather. Climate Extremes. 10, 4–10. doi: 10.1016/j.wace.2015.08.001
Hatfield J. L., Walthall C. L. (2015). Soil Biological Fertility: Foundation for the Next Revolution in Agriculture? Commun. Soil Sci. Plant Analysis. 46 (6), 753–762. doi: 10.1080/00103624.2015.1005227
Hirai G., Okumura T., Takeuchi S. (2000). Effect of the Relative Humidity of the Atmosphere on the Growth and Physiology of Rice Plants. Plant Production. Sci. 3, 2 – p.129. doi: 10.1626/pps.3.129
Hochman Z., Gobbett D., Horan H., Garcia J. N. (2016). Data Rich Yield Gap Analysis of Wheat in Australia. Field Crops Res. 197, 97–106. doi: 10.1016/j.fcr.2016.08.017
Hoffmann V., Probst K., Christinck A. (2007). Farmers and Researchers: How can Collaborative Advantages be Created in Participatory Research and Technology Development? Agric. Hum. Values. 24, 355–368. doi: 10.1007/s10460-007-9072-2
Hong Y., Jiayang L. (2021). Short- and Long-Term Challenges in Crop Breeding. Natl. Sci. Rev. 8. doi: 10.1093/nsr/nwab002
Izaba O. F. R. (2021). Value-added strategies in the specialty crop industry: exploring farmers' drivers and strategies at the farm level (doctoral dissertation, purdue university graduate school.
Jha U. C., Bohra A., Singh N. P. (2014). Heat Stress in Crop Plants: Its Nature, Impacts and Integrated Breeding Strategies to Improve Heat Tolerance. Plant Breed. 133), 679–701. doi: 10.1111/pbr.12217
Kang M. S. (1998). Using Genotype-By-Environment Interaction for Crop Cultivar Development. Adv. Agron. 62, 199–252. doi: 10.1016/S0065-2113(08)60569-6
Kazemi F., Mohorko R. (2017). Review on the Roles and Effects of Growing Media on Plant Performance in Green Roofs in World Climates. Urban. Forestry. Urban. Greening. 23, 13–26. doi: 10.1016/j.ufug.2017.02.006
Khan I., Lei H., Khan A., Muhammad I., Javeed T., Khan A., et al. (2021). Yield Gap Analysis of Major Food Crops in Pakistan: Prospects for Food Security. Environ. Sci. pollut. Res. 28, 7994–8011. doi: 10.1007/s11356-020-11166-4
Kukal M. S., Irmak S. (2020). Characterization of Water Use and Productivity Dynamics Across Four C3 and C4 Row Crops Under Optimal Growth Conditions. Agric. Water Manage. 227, 105840. doi: 10.1016/j.agwat.2019.105840
Kukal S. S., Yadav S., Humphreys E., Kaur A., Singh Y., Thaman S., et al. (2010). Factors Affecting Irrigation Water Savings in Raised Beds in Rice and Wheat. Field Crops Res. 118, 43–50. doi: 10.1016/j.fcr.2010.04.003
Leakey A. D. (2009). Rising Atmospheric Carbon Dioxide Concentration and the Future of C4 Crops for Food and Fuel. Proc. Biol. Sci. 276, 2333–2343. doi: 10.1098/rspb.2008.1517
Liava V., Karkanis A., Danalatos N., Tsiropoulos N. (2022). Effects of Two Varieties and Fertilization Regimes on Growth, Fruit, and Silymarin Yield of Milk Thistle Crop. Agronomy 12, 105. doi: 10.3390/agronomy12010105
Liliane T. N., Charles M. S. (2020). “Factors Affecting Yield of Crops,” in Agronomy - Climate Change & Food Security. Ed. Amanullah A. (London: IntechOpen). Available at: https://www.intechopen.com/chapters/70658. doi: 10.5772/intechopen.90672
Mahmud A.-A., Alam M. J., Heck S., Grüneberg W. J., Chanda D., Rahaman E. H. M. S., et al. (2021). Assessing the Productivity, Quality and Profitability of Orange Fleshed Sweet Potatoes Grown in Riverbank of the Tista Floodplain Agro-Ecological Zone of Bangladesh. Agronomy 11, 2046. doi: 10.3390/agronomy11102046
Makuvaro V., Walker S., Munodawafa A., Chagonda I., Masere P., Murewi C., et al. (2017). Constraints to Crop Production and Adaptation Strategies of Smallholder Farmers in Semi-Arid Central and Western Zimbabwe. Afr. Crop Sci. J. 25, 221– 235. doi: 10.4314/acsj.v25i2.7
Markham J. H., Grime J. P., Buckland S. (2009). Reciprocal Interactions Between Plants and Soil in an Upland Grassland. Ecol. Res. 24, 93–98. doi: 10.1007/s11284-008-0485-1
Mendham N., Russell J., Jarosz N. (1990). Response to Sowing Time of Three Contrasting Australian Cultivars of Oilseed Rape (Brassica Napus). J. Agric. Sci. 114 (3), 275–283. doi: 10.1017/S002185960007266X
Messmer M. M. (2015). Plant Breeding Techniques: An Assessment for Organic Farming (Frick, Switzerland: Research Institute of Organic Agriculture), 37.
Monjardino M., Hochman Z., Horan H. (2019). Yield Potential Determines Australia Wheat Growers’ Capacity to Close Yield Gaps While Mitigating Economic Risk. Agron. Sustain. Dev. 39, 49. doi: 10.1007/s13593-019-0595-x
NVT (National Variety Trials) (2009) Grains Research and Development Corporation, Australia. Available at: www.nvtonline.com.au.
Ohtaka K., Yoshida A., Kakei Y., Fukui K., Kojima M., Takebayashi Y., et al. (2020). Difference Between Day and Night Temperatures Affects Stem Elongation in Tomato (Solanum Lycopersicum) Seedlings via Regulation of Gibberellin and Auxin Synthesis. Front. Plant Sci. 11. doi: 10.3389/fpls.2020.577235
Oladosu Y., Rafii M. Y., Abdullah N., Magaji U., Miah G., Hussin G., et al. (2017). Genotype × Environment Interaction and Stability Analyses of Yield and Yield Components of Established and Mutant Rice Genotypes Tested in Multiple Locations in Malaysia. Acta Agriculturae. Scandinavica. Section. B. — Soil Plant Sci 67 (7), 590–606. doi: 10.1080/09064710.2017.1321138
Olesen J. E., Trnka M., Kersebaum K. C., Skjelvåg A. O., Seguin B., Peltonen-Sainio P., et al. (2011). Impacts and Adaptation of European Crop Production Systems to Climate Change. Eur. J. Agron. 34, 96–112. doi: 10.1016/j.eja.2010.11.003
Passioura J. B. (2020). Translational Research in Agriculture. Can We do it Better? Crop Pasture Sci. 71, 517–528. doi: 10.1071/CP20066
Perez-Alvarez R., Nault B. A., Poveda K. (2018). Contrasting Effects of Landscape Composition on Crop Yield Mediated by Specialist Herbivores. Ecol. Appl. 28, 842–853. doi: 10.1002/eap.1695
Pfeiffer W. H., McClafferty B. (2007). Harvest Plus: Breeding Crops for Better Nutrition. Crop Sci. 47, S–88-S-105. doi: 10.2135/cropsci2007.09.0020IPBS
PFMPB (2021). Past and Future Milestones of Plant Breeding. Trends Plant Sci. 26 (6), 530–538. doi: 10.1016/j.tplants.2021.03.013
Pingali P. (2012). Green Revolution: Impacts, Limits, and the Path Ahead. Proc. Natl. Acad. Sci. 109, 12302 – 12308. doi: 10.1073/pnas.0912953109
Quan M., Liang J. (2017). The Influences of Four Types of Soil on the Growth, Physiological and Biochemical Characteristics of Lycoris Aurea (L' Her.) Herb. Sci. Rep. 7, 43284. doi: 10.1038/srep43284
Quddus A., Kropp J. D. (2020). Constraints to Agricultural Production and Marketing in the Lagging Regions of Bangladesh. Sustainability 12, 3956. doi: 10.3390/su12103956
Reddy A., Rasineni G., Raghavendra A. (2010). The Impact of Global Elevated CO₂ Concentration on Photosynthesis and Plant Productivity. Curr. Sci. 99 (1), 46–57.
Rehman T., Khan M. U., Tayyab M., Akram M. W., Faheem M. (2016). Current Status and Overview of Farm Mechanization in Pakistan–A Review. Agric. Eng. International.: CIGR J. 18 (2), 83–93.
RIRG (2021a) Rural Innovation Research Group, Faculty of Veterinary & Agricultural Sciences University of Melbourne. Available at: https://rirg.fvas.unimelb.edu.au/:data/assets/pdf_file/0004/2800255/Privatising-Ag-Ext-Rpt-B-Adviser-supply-V3.pdf.
RIRG (2021b). Available at: https://rirg.fvas.unimelb.edu.au/ag-extension/home.
Sawan Z. M. (2017). Climatic Variables: Evaporation, Sunshine, Relative Humidity, Soil and Air Temperature and its Adverse Effects on Cotton Production. Inf. Process. Agric. 5. doi: 10.1016/j.inpa.2017.09.006
Schuler M. L., Mantegazza O., Andreas P. M. W. (2016). Engineering C4 Photosynthesis Into C3 Chassis in the Synthetic Biology Age. Plant J. 87, 51–65. doi: 10.1111/tpj.13155
Shelton A. C., Tracy W. F. (2016). Participatory Plant Breeding and Organic Agriculture: A Synergistic Model for Organic Variety Development in the United States. Elementa: Sci. Anthropocene. 4, 000143.
Sheng Y., Chancellor W. (2019). Exploring the Relationship Between Farm Size and Productivity: Evidence From the Australian Grains Industry. Food Policy 84, 196–204. doi: 10.1016/j.foodpol.2018.03.012
Simic D., Presterl T., Seitz G., Geiger H. H. (2003). Comparing Methods for Integrating Exotic Germplasm Into European Forage Maize Breeding Programs. Crop Sci. 43, 1952–1959. doi: 10.2135/cropsci2003.1952
Singh V. K., Gautam P., Nanda G., Dhaliwal S. S., Pramanick B., Meena S. S., et al. (2021). Soil Test Based Fertilizer Application Improves Productivity, Profitability and Nutrient Use Efficiency of Rice (Oryza Sativa L.) Under Direct Seeded Condition. Agronomy 11, 1756. doi: 10.3390/agronomy11091756
Still C. J., Berry J. A., Collatz G. J., DeFries R. S. (2003). Global Distribution of C3 and C4 Vegetation: Carbon Cycle Implications. Global biogeochem. cycles 17 (1), 6–14.doi: 10.1029/2001GB001807
Stuerz S., Asch F. (2021). Responses of Rice Growth Today and Night Temperature and Relative Air Humidity—Leaf Elongation and Assimilation. Plants 10 (1), 134. doi: 10.3390/plants10010134
Taub D. (2010). Effects of Rising Atmospheric Concentrations of Carbon Dioxide on Plants. Nat. Educ. Knowledge. 3 (10), 21.
Trethowan R. M., Crossa J., van Ginkel M., Rajaram S. (2001). Relationships Among Bread Wheat International Yield Testing Locations in Dry Areas. Crop Sci. 41, 1461 – 1469. doi: 10.2135/cropsci2001.4151461x
Trethowan R. M., Reynolds M. P., Ortiz-Monasterio J. I., Ortiz R. (2007). The Genetic Basis of the on-Going Green Revolution in Wheat Production. Plant Breed. Rev. 28, 38–58. doi: 10.1002/9780470168028.ch2
Trethowan R. M., Turner M. A., Chattha T. M. (2010). “Breeding Strategies to Adapt Crops to a Changing Climate,” in Climate Change and Food Security. Advances in Global Change Research, vol. vol 37 . Eds. Lobell D., Burke M. (Dordrecht: Springer). doi: 10.1007/978-90-481-2953-9_9
Trethowan R. M., Van-Ginkel M., Ammar K., Crossa J., Payne T. S., Cukadar B., et al. (2003). Associations Among Twenty Years of Bread Wheat Yield Evaluation Environments. Crop Sci. 43, 1698–1711. doi: 10.2135/cropsci2003.1698
USDA (2021) United States Department of Agriculture. Available at: https://www.ers.usda.gov/amber-waves/2016/december/precision-agriculture-technologies-and-factors-affecting-their-adoption/.
Vargas M., Glaz B., Alvarado G., Pietragalla J., Morgounov A., Zelenskiy Y., et al. (2015). Analysis and Interpretation of Interactions in Agricultural Research. Agron. J. 107), 748–762. doi: 10.2134/agronj13.0405
Villegas D., Alfaro C., Ammar K., Catedra M., Crossa J., Moral L. G. D., et al. (2016). Daylength, Temperature and Solar Radiation Effects on the Phenology and Yield of Spring Durum Wheat. J. Agron. Crop Sci. 202, 203–216. doi: 10.1111/jac.12146
Voss-Fels K. P., Stahl A., Hickey L. T. (2019). Q&A: Modern Crop Breeding for Future Food Security. BMC Biol. 17, 18. doi: 10.1186/s12915-019-0638-4
Waddington S. R., Li X., Dixon J., Hymen G., De-Vicente M. C. (2010). Getting the Focus Right: Production Constraints for Six Major Food Crops in Asian and African Farming Systems. Food Sec. 2, 27–48. doi: 10.1007/s12571-010-0053-8
Wahid A., Gelani S., Ashraf M., Foolad M. R. (2007). Heat Tolerance in Plants: An Overview. Environ. Exp. Bot. 61, 199–223. doi: 10.1016/j.envexpbot.2007.05.011
Walthall C., Hatfield J., Schneider S., Vigil M. (2016). “Closing Yield Gaps With GxExM and Precision Agriculture,” in Proceedings of the 13th International Conference on Precision Agriculture (Monticello, IL: International Society of Precision Agriculture).
William T. S., Merchant A. M., Richards R. A., Trethowan R., Buckley T. N. (2019). Rate of Photosynthetic Induction in Fluctuating Light Varies Widely Among Genotypes of Wheat. J. Exp. Bot. 70, 2787–2796. doi: 10.1093/jxb/erz100
Xia L., Robock A., Cole J., Curry C. L., Ji D., Jones A., et al. (2014). Solar Radiation Management Impacts on Agriculture in China: A Case Study in the Geoengineering Model Intercomparison Project (GeoMIP). J. Geophys. Res. Atmos. 119, 8695–8711. doi: 10.1002/2013JD020630
Yunbi X., Ping L., Cheng Z., Yanli L., Chuanxiao X., Xuecai Z., et al. (2017). Enhancing Genetic Gain in the Era of Molecular Breeding. J. Exp. Bot. 68, 2641–2666. doi: 10.1093/jxb/erx135
Zabel F., Müller C., Elliott J., Minoli S., Jägermeyr J., Schneider J. M., et al. (2021). Large Potential for Crop Production Adaptation Depends on Available Future Varieties. Glob. Change Biol. 27, 3870–3882. doi: 10.1111/gcb.15649
ZTBL (2020) Crop Yield Analysis Pakistan. Available at: https://www.ztbl.com.pk/wp-content/uploads/Documents/Publications/Research-Studies/CropYieldGapAnalysis.pdf.
Keywords: crop productivity, GEM reciprocity, factor interactions, cultivars, environments, management practices, protected cropping, extension services
Citation: Mahmood T, Ahmed T and Trethowan R (2022) Genotype x Environment x Management (GEM) Reciprocity and Crop Productivity. Front. Agron. 4:800365. doi: 10.3389/fagro.2022.800365
Received: 23 October 2021; Accepted: 18 May 2022;
Published: 28 June 2022.
Edited by:
Munir H. Zia, Fauji Fertilizer Company Limited, PakistanReviewed by:
Abdul Khaliq, University of Agriculture, Faisalabad, PakistanCopyright © 2022 Mahmood, Ahmed and Trethowan. This is an open-access article distributed under the terms of the Creative Commons Attribution License (CC BY). The use, distribution or reproduction in other forums is permitted, provided the original author(s) and the copyright owner(s) are credited and that the original publication in this journal is cited, in accordance with accepted academic practice. No use, distribution or reproduction is permitted which does not comply with these terms.
*Correspondence: Tariq Mahmood, dGFyaXEuY2hhdHRoYUBzeWRuZXkuZWR1LmF1
Disclaimer: All claims expressed in this article are solely those of the authors and do not necessarily represent those of their affiliated organizations, or those of the publisher, the editors and the reviewers. Any product that may be evaluated in this article or claim that may be made by its manufacturer is not guaranteed or endorsed by the publisher.
Research integrity at Frontiers
Learn more about the work of our research integrity team to safeguard the quality of each article we publish.