- 1Crop Research Centre Oak Park, Teagasc, Carlow, Ireland
- 2Agri-food Business and Spatial Analysis Department, Teagasc, Dublin, Ireland
- 3Crop Protection Department, Weed Biology, ADAS Boxworth, Boxworth, United Kingdom
- 4Agriculture, School of Natural and Environmental Science, Newcastle University, Newcastle Upon Tyne, United Kingdom
The challenges of grass-weed control in a mild Atlantic climate, added to by the use of non-inversion tillage and limited herbicide options necessitated on-farm knowledge acquisition on grass weeds in Ireland. We surveyed 103 farms from 2020 to 2021 of which, 62 were plough-based and 41 non-inversion tillage. The survey comprised a questionnaire to determine grower demographics, grass-weed challenges, and the adoption of integrated grass-weed control methods (IWM); a grid-square assessment of key grass weeds encountered in one or more cereal fields in both years using weed scores from 0 (absent) to 10 (total weed cover); with samples collected for herbicide-resistance testing. Across the 103 farms, Bromus spp., (62%) and Avena fatua (56%) were the most prevalent but with moderate resistance (8% acetolactate synthase (ALS)-resistant Bromus, 10% acetyl-CoA carboxylase (ACCase)-resistant A. fatua); Lolium multiflorum (13%) and Alopecurus myosuroides (16%) were found on fewer farms but with higher resistance levels (56% ACCase and/or ALS-resistant L. multiflorum, 43% ACCase/ALS-resistant A. myosuroides). Of those who adopted non-inversion tillage, a higher proportion of growers practiced crop rotation (88% cereal/non-cereal break or 83% alternate spring/winter-sown) and used cover crops (71%) than those ploughing (52-66% crop rotation or 19% cover crops), but herbicide use was similar in both systems. Despite higher levels of IWM practices, non-inversion tillage farms had higher weed scores (2.2 ± 0.2 or 3.8 ± 0.7) of B. sterilis and L. multiflorum than ploughing (1.2 ± 0.2 or 2.0 ± 0.7). Considering the complex resistance profile of high resistance-risk species, there is a need for urgent determination of and adoption of effective IWM across systems, specifically, non-inversion tillage. Most of the growers were aware of herbicide-resistant grass weeds (>80%) and most (>90%) used IWM practices (4 or more) to some extent. In our survey, non-inversion tillage farms tended to have younger growers with more formal education and larger farms. This is the first multi-component survey in Ireland establishing long-term monitoring of grass-weed challenges, grower characteristics and IWM practices.
Introduction
In Ireland, agriculture occupies 4.5 million ha of which, 92% is devoted to grassland and 8% to arable crop production (CSO, 2016). Crop production in Ireland has evolved over the last 50 years from a historical mixed grassland/spring cropping systems to todays specialized cereal monoculture or rotations consisting entirely of annual crops (Forristal and Grant, 2011). Spring barley, winter wheat and winter barley comprise the largest crop areas with 120,000 ha, 60,000 ha and 60,000 ha grown respectively, each year (CSO, 2021). In Ireland, crop production is predominantly concentrated in the southeast, where there is a drier climate (average annual rainfall of between 660 and 1000 mm) than other areas of the country (annual rainfall of between 1000 and 2800 mm) (Met Eireann, 2021), and having light to medium texture, free draining soils (Schulte et al., 2010). The dominant system for crop establishment in this region is plough-based tillage (Forristal and Murphy, 2009). While the adoption of non-inversion crop establishment systems has been low (Soane et al., 2012), interest has recently increased mainly to reduce escalating production costs (Forristal and Murphy, 2009), and these systems are primarily used to establish winter cereals (Brennan et al., 2015), but also spring cereals (Brennan et al., 2014) and non-cereal break crops, including oilseed rape and beans (Byrne et al., 2022). Other reasons for interest in non-inversion tillage systems in this region include: labour shortage (Bijttebier et al., 2018), high work rates (Fortune et al., 2005), strong promotion by farmer-led organisations like BASE Ireland (Biodiversity, Agriculture, Soil and Environment) (BASE Ireland, 2018), the EU’s mandatory crop diversification requirements of the Common Agricultural Policy (i.e. three-crop rule) (DAFM, 2022a), and Irish policy makers’ support of carbon-efficient cropping systems via incentives in agri-climate rural environment schemes (DAFM, 2022b).
Ireland has an Atlantic-influenced mild, temperate humid climate and warm summers (Köppen-Geiger classification: Cfb) (Peel et al., 2007). These climatic conditions coupled with a soil quality legacy from past mixed rotations and improved varieties result in Irish winter cereal yields between 7.2 to 10.7 t ha-1 which are among the highest globally (CSO, 2021). Nevertheless, this same mild wet climate is conducive to the rapid development and proliferation of fungal diseases, grass weeds, and to some extent, insect vector-borne diseases, requiring the intensive use of pesticides to achieve these high grain yields (Jess et al., 2014; Lynch et al., 2017). Fungicides are the most commonly used pesticides accounting for 46% by weight, (DAFM, 2016) and 55% of costs per ha, (Teagasc, 2022) of all pesticides used, compared to 31% and 36% for herbicides, respectively. While the challenges associated with reliance on pesticide use for fungal disease control, in terms of reduced fungicide efficacy in this climate are known (e.g. Jess et al., 2014), the effects of similar pesticide approaches, added to by adoption of non-inversion tillage, and increased use of a more restricted range of herbicides, is less well known.
A wide spectrum of grass and broad-leaved weeds infest arable fields in Ireland, posing a complex management challenge to cereal growers. Several grass-weed species present herbicide-based control problems including Italian ryegrass (Lolium multiflorum), black-grass (Alopecurus myosuroides), spring wild oats (Avena fatua), barren or sterile brome (Bromus sterilis), lesser canary grass (Phalaris minor) and annual or rough-stalked meadow-grasses (Poa spp.). Most of these species rank in the top ten herbicide-resistant weeds, globally, due to their high risk of developing resistance to post-emergent herbicides (Heap, 2022). In Ireland, the two most widely used post-emergence herbicide types for selective grass-weed control within crops have acetyl-CoA carboxylase (ACCase)- (HRAC group 1) or acetolactate synthase (ALS)- (HRAC group 2) inhibiting modes of action (DAFM, 2016). More than 100,000 ha of barley and 10,000 ha of wheat (spring and winter) area were treated with ACCase-pinoxaden, highly active on A. fatua and/or L. multiflorum, in 2016 (DAFM, 2016). The graminicides ACCase-propaquizafop and cycloxydim were used on >7500 ha of oilseed rape and bean area to control a range of grass weeds, while ALS-mesosulfuron + iodosulfuron and pyroxsulam were used on >17,000 ha of winter wheat. The remaining chemical options available for grass-weed control include: non-selective (e.g. glyphosate) and pre-emergent selective residual herbicides (e.g. pendimethalin or flufenacet).
Since 2019, resistance to ACCase/ALS inhibitors via target-site resistance (TSR) and/or non-target-site resistance (NTSR) has been documented in A. fatua, A. myosuroides and L. multiflorum in cereal fields in Ireland (Alwarnaidu Vijayarajan et al., 2020; Byrne et al., 2021; Alwarnaidu Vijayarajan et al., 2021). To date, knowledge of the resistance problem has been almost exclusively based on ad-hoc basis of testing suspect field populations, where herbicide control was inadequate. This approach is not sufficient to identify the scale of resistance problems in Ireland.
To date, research in Ireland on non-inversion crop establishment systems, often associated with grass-weed challenges, has mainly focused on technical aspects, including economics (e.g. Forristal and Murphy, 2009), crop yields (e.g. Brennan et al., 2015), and soil quality and greenhouse gas emissions (e.g. Van Groenigen et al., 2011; Kennedy et al., 2013; Abdalla et al., 2014). While grower surveys focusing on problem weeds, weed pressure, resistance awareness and weed control practices have been conducted in the UK, USA, Australia, or elsewhere (e.g. Johnson and Gibson, 2006; Lewellyn et al., 2007; Riemens et al., 2010; Ulber and Rissel, 2018; Hicks et al., 2018; Liu et al., 2020), no such research has been carried out in the Atlantic-influenced Irish production conditions.
Given that grass weeds pose the greatest weed control challenge in herbicide-dependent systems, the increased challenge of non-inversion tillage [where wider adoption of these systems in conjunction with earlier-sowing and simplified rotations have exacerbated grass-weed challenges in the UK (Moss, 2017), which has a climate (Cfa) broadly similar to Ireland], and the added extra pressures of a mild wet Atlantic climate, which is even more favorable for grass-weed growth, it was considered necessary to generate climate and production specific knowledge on the occurrence of grass weeds and their resistance status and factors influencing them by surveying Irish growers. The specific aims were to: (a) identify the most problematic weeds on crop farms and their herbicide resistance status, (b) quantify weed control and associated practices on crop farms, and (c) identify management, including crop establishment systems and other factors associated with weed prevalence on farms. This research should help determine integrated grass-weed control methods (IWM) that need to be developed and deployed to help prevent further increases in challenging weeds. It will also generate a baseline from which the impact of future management can be benchmarked.
Materials and methods
Farm selection
One hundred and three Irish farms were selected using a stratified sampling approach to give adequate representation of crop establishment systems. The farms comprised: 62 using plough-based crop establishment, which included soil inversion by mouldboard ploughing to 150-300 mm deep, followed by secondary cultivation in advance of, or in combination, with drilling, and 41 using non-inversion establishment of which: 15 and 11 used shallow and deep min-till, respectively, where the tine and disc implements shatter and mixes crop residue to less than, or more than, 100 mm deep, without inversion; 4 used strip-tilling where the soil was cultivated in strips approximately 300 mm apart with the seed sown on the strip; and 11 used direct drill, where the seed was placed with minimal disturbance in slots opened by disc or tine coulters.
The growers were selected from contact lists from both Teagasc (the state-funded research and farm advisory organization) and commercial crop advisors on the basis of crop establishment system used (on >50% of their cropped land) and cereals (wheat, barley or rarely, oats) being their main crop type. The survey participants were interviewed to complete a detailed questionnaire and their selected cereals fields were surveyed in both 2020 and 2021, ensuring accurate and comparable grass-weed field data from both years, and grass-weed populations were collected for herbicide resistance evaluations.
Questionnaire survey
The 103 growers were interviewed in early 2020 by experienced Teagasc crop advisors using a structured questionnaire divided into three sections. The first section characterised the respondents socio-demographic profile, awareness and concerns of grass-weed resistance, using ‘yes/no’ response or on a scale of 1 (low) to 3 (high), and IWM familiarity, using a scale of 1 (not familiar) to 3 (extremely familiar). The second section identified the most problematic or difficult-to-control grass weeds (using a ranking method), and their prevalence on farms. The responses to additional questions regarding management history, which included years of specific crop establishment systems practiced, years of problems with difficult-to-control grass weeds, and crop sequence, allowed us to determine problem grass-weed cereal fields within farms for the 2020 and 2021 population surveys. Soil types and herbicide used each year for the surveyed fields were also noted. The last section examined the on-farm adoption of ten IWM (herbicide/non-herbicide) practices using ‘yes/no’ responses, where some ‘yes’ responses prompted growers to provide additional information, and then farms were classified as low (<3), medium (4-6) or high (>7) IWM users, based on the number of practices applied. The study met all ethical and data protection requirements of Teagasc. In addition, informed consent was obtained from participants prior to the survey.
Field survey and sample collection
Difficult-to-control grass weeds were assessed on the 103 farms from June to August of both 2020 and 2021 on the same selected cereal fields, which the growers/crop advisors selected on the basis of having grass-weed problems and where a specific crop establishment system was used. Teagasc crop advisors were trained, to assign weed scores from 0 (absent) to 10 (total weed cover) using visual guides, and to collect spatial information using a purpose-developed smartphone App (ArcGIS Survey 123, ESRI, county Dublin, Ireland) for position data logging. The visual guides were printed schematics and pictures that illustrated the individual score descriptions.
To assess the extent of weed populations in the surveyed fields, if the field was 3 to 5 ha, the entire field was studied, but for fields >5 ha a representative area of approximately 4 ha was selected using a measuring map tool in the App. Two random in-field tramlines and at least one headland tramline, where machines had been turning, were then selected within the representative area. The area was then divided into grid squares, normally 24m x 24m, based on tramline width. At least four equally-spaced grid squares per in-field tramline and three per headland tramline were used for assessments. At each grid square, a central GPS coordinate was logged, and a weed score was recorded. Each grid square was visually assigned a score from 0 to 10 that corresponds to the variation in weed pressure: 0 (absent), 1 (a single area of isolated individual heads), 2 (isolated individual heads in a number of area), 3 (small individual heads in many area), 4 (a single area of significant individual heads), 5 (significant individual heads in a number of area), 6 (significant individual heads in many area), 7 (a single area of dense patches), 8 (dense patches in a number of area), 9 (dense patches in many area) and 10 (total weed cover). The scores were validated by a limited number of weed counts (data not shown). Not all farms had difficult-to-control species present. In some farms, more than one field was surveyed, due to the incidence of more than one difficult-to-control species or due to the observed population pressure. In farms where mixed difficult-to-control species were present in the same survey fields, each species was assessed separately to obtain individual field-level data.
For herbicide resistance testing, in 2020, seed samples were taken on a grid-square basis from all seed heads where weed scores were 1 or from 10 heads per grid where scores were 2 or more. The seed samples were labelled with an identifier code, location and species information, and stored in paper bags at 15°C until used. In 2021, some samples were collected from farms with low seed amounts in 2020 or where they had not been previously sampled.
Glasshouse herbicide screening
One field population sample per farm of a specific species was used for resistance evaluation with a total of 161 grass-weed populations screened for sensitivity to ACCase/ALS inhibitors applied at half and full recommended label field application rates (Table 1). Due to large number of populations to be tested, the order in which initial screening conducted was based on the grower’s priority needs. A few populations were not tested, due to poor germination.
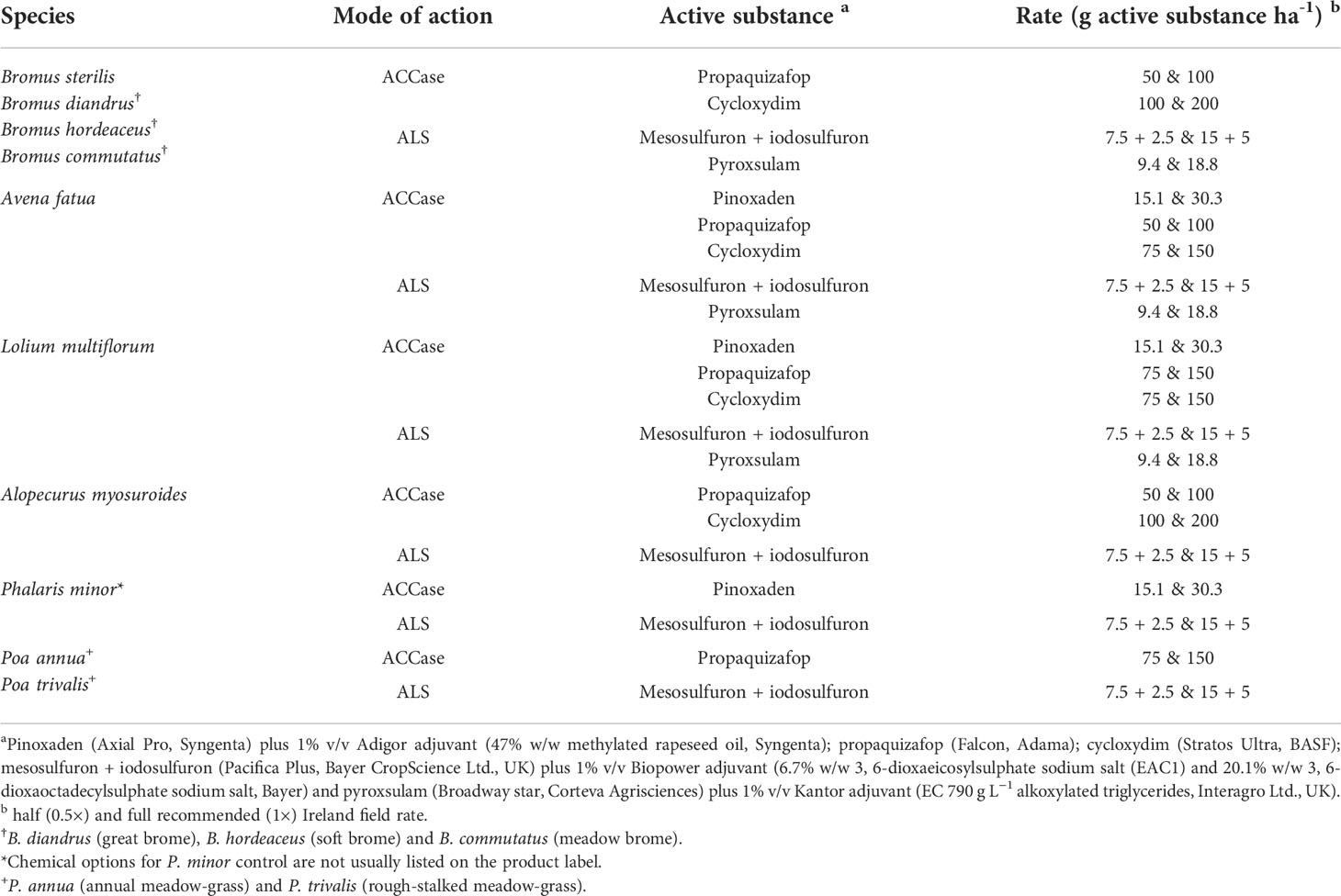
Table 1 Modes of action, active substance and application rates of herbicides used in the sensitivity screening of grass-weed populations.
Experiments were conducted at controlled glasshouse facilities located at the Teagasc Oak Park Crops Research Centre, Carlow, Ireland. Seed treatments to break seed dormancy, plant establishment methods, and growing conditions were as previously reported by Alwarnaidu Vijayarajan et al. (2020); Alwarnaidu Vijayarajan et al. (2021). Seeds were sown into Kettering loam and lime-free grit mix in a 4:1 ratio, with the addition of Osmocote Mini™ (1 kg t−1) in 20-cell 270 × 209 × 69 mm (external dimensions) plastic trays for Bromus and A. fatua populations or in 355 × 215 × 50 mm plastic trays (without cells) for the other grass-weed populations. Three (Bromus and A. fatua) or four (other grass weeds) replicates per population and herbicide rate were used. Each replicate had 10-20 seeds per tray for Bromus and A. fatua populations, while there were 10-30 seeds per replicate for others. Herbicides were applied to the plants at growth stage (GS) 12–13 (BBCH) except for A. fatua plants which were applied at GS 13–14, using a Generation III Research Track Sprayer (DeVries Manufacturing, Hollandale, MN, USA) with a Teejet 8002-EVS flat fan nozzle, at a pressure of 2.5 bar and a water volume equivalent of 200 L ha−1. In addition to untreated controls, known sensitive reference populations BROST-038 (from county Meath, 53°54′N 6°74′W; confirmed sensitive in 2019) or UK-SD-0385 for B. sterilis (Alwarnaidu Vijayarajan, unpublished); S1 or S2 for A. fatua (Alwarnaidu Vijayarajan et al., 2020); SORG-002 for L. multiflorum and S-006 or UK-SD-0827 for A. myosuroides (Alwarnaidu Vijayarajan et al., 2021) were included. For B. diandrus, B. hordeaceus, B. commutatus, P. minor or Poa spp. sensitive reference populations were not available at the time of the experiment. However, most of these grass weeds had 100% mortality to ACCase/ALS inhibitors applied at full field rate, except for one population of B. diandrus that has survived ALS-mesosulfuron + iodosulfuron at both half and full rate, requiring further investigation.
Visual assessment for plant survival was conducted at 28 or 35 days (Bromus and Poa spp. only) after spraying, and plants were rated as dead (susceptible) or alive (resistant). Above-ground shoots were harvested, dried at 70° C for 72 hours and weighed (data not shown). Plant survival was assessed for each population at each herbicide rate by expressing the percentage of surviving seedlings. To determine the proportion of farms with herbicide resistance, populations were classified as resistant if more than 10% of plants survive both half and full recommended field rates.
Statistical analysis
Data analysis was with R, version 3.6.3 (R Core Team, 2020). Descriptive statistics and frequencies for the variables in the dataset was estimated using the summarytools package (Comtosis, 2022). The chi-square (𝜒2) analysis function [chisq.test], was used to determine if there was any association between crop establishment system used, grower socio-demographics and weed control practices. A linear mixed-effects model using the lmerTest package (Kuznetsova et al., 2017) was developed to evaluate the influence of management factors on grass-weed population pressure based on weed scores. A binary logistic regression model (Scott, 1997) was used to measure the relationship between farms with different crop establishment systems and some independent variables like socio-demographic aspects, weed score, resistance status or weed control variables.
Results and discussion
Socio-demographics and herbicide resistance awareness
In our survey, nearly, all participating Irish farms were from the south and east of the country in a Louth-Wexford-Cork triangle (Figure 1), with a large number from Kildare, Meath and Wexford counties, where cereals crops are mainly grown due to favourable climate and soil types (Schulte et al., 2010).
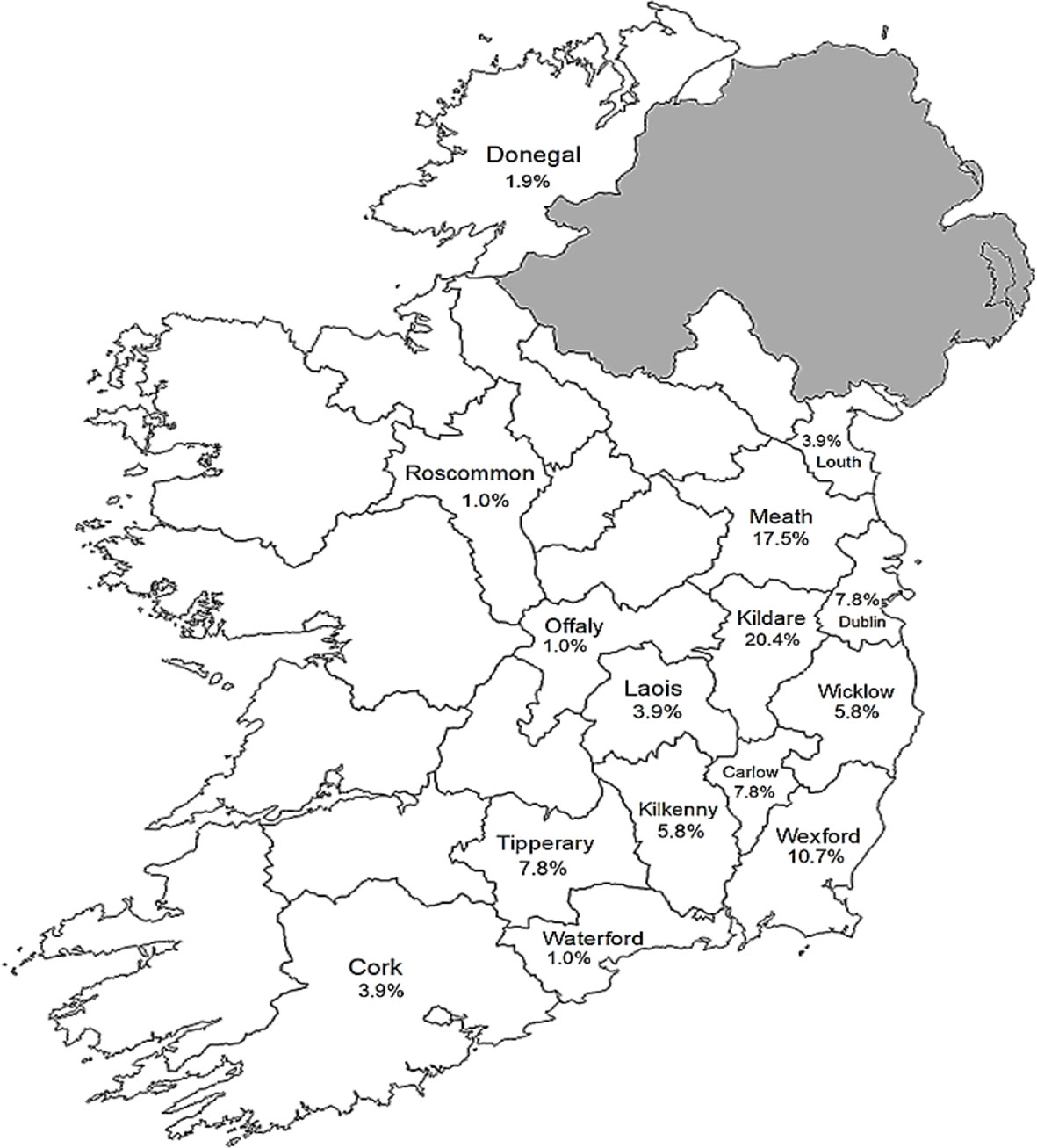
Figure 1 Distribution of the surveyed participants on a county basis (percentage of the surveyed growers in each county) in Ireland (n=103).
There was a significant association between crop establishment types used by growers and some demographic variables like age, education and farming size. Non-inversion tillage users were younger, compared to those using plough-based establishment (75% < 50 years vs. 47%), with ages well below the average Irish arable farmer’s age of 57 (CSO, 2020); 95% of them had a year or more of formal third level agricultural education, compared to 79% for those ploughing; and 71% were farming more than 100 ha, compared to 45% of those ploughing. As the average size of Irish arable or mixed crop and livestock farms is about 59 ha, so those in our survey, were classified as medium to larger farms (Table 2). Similarly, previous innovation adoption studies in Europe found young educated contemporary growers with large farms are more likely to embrace alternative farming practices, due to their ability to access information and distinguish between promising and unpromising innovations (e.g. Knowler and Bradshaw, 2007; Lobley et al., 2009; Heanue and O’Donoghue, 2014; Bartkowski et al., 2022). While it is possible that older growers are comfortable with their current practices (e.g. Bartkowski et al., 2022) or in some cases, their short-term experience with non-inversion tillage led to negative conclusions about its suitability for their situations (e.g. Forristal and Murphy, 2009; Lahmar, 2010).
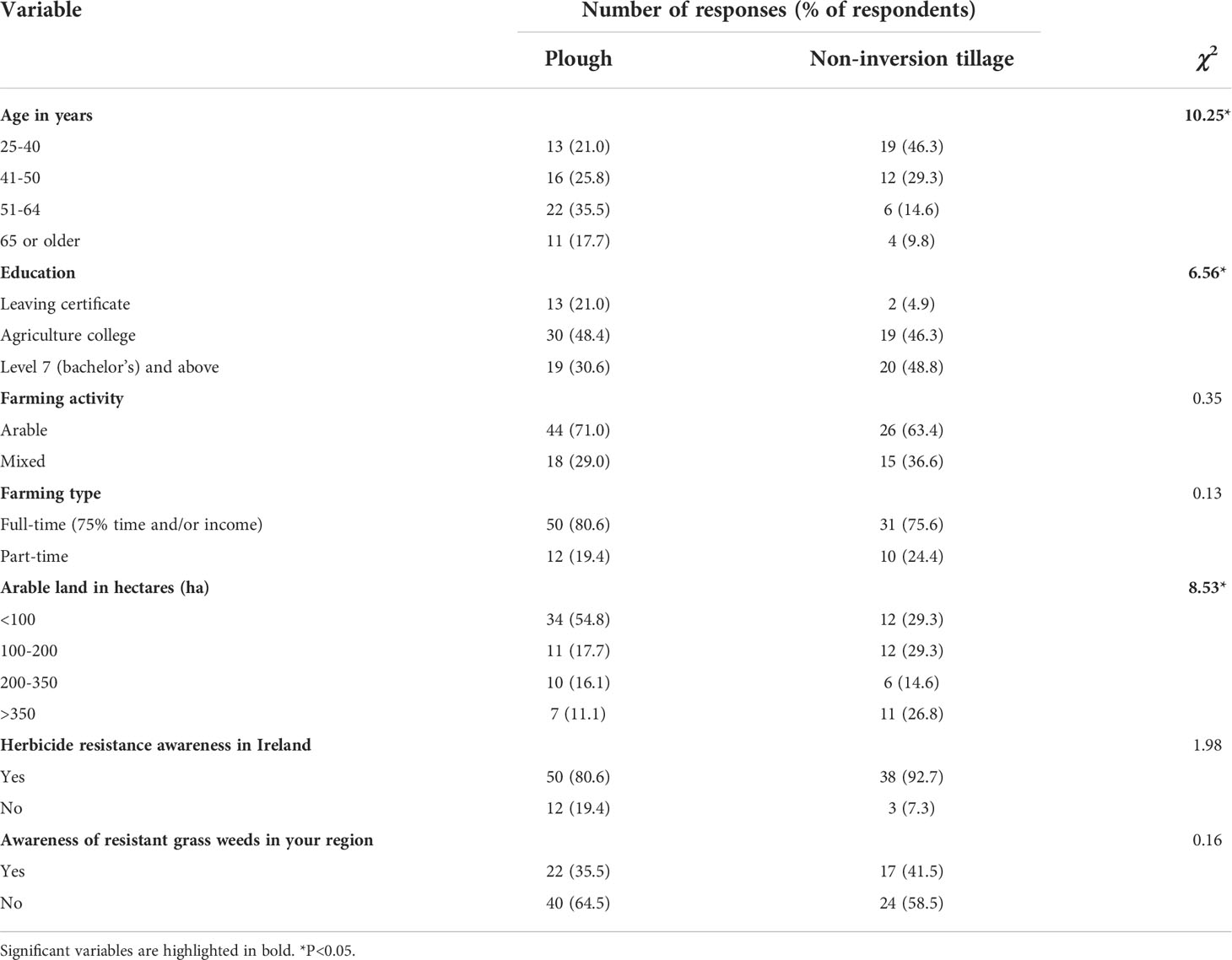
Table 2 Farmer profile across plough (n=62) and non-inversion (n=41) crop establishment farms in Ireland and their associations.
Of the 103 farms, 53% of arable growers (n=34 plough and n=21 non-inversion tillage) and 25% of mixed crop and livestock growers (n=16 and n=10) identified farming as their sole income source. The majority of the participants (>80%) were aware of the occurrence of herbicide-resistant grass weeds in the country but much less so of resistance in their own region, which is not surprising given the ad-hoc basis of testing to date (Table 2). More than 85% of growers using both systems (n=53 plough and n=38 non-inversion tillage) expressed high levels of concern about herbicide resistance on a three point scale, and felt it would pose a significant economic challenge. Regarding IWM familiarity, 69% were somewhat familiar (n=44 plough and n=27 non-inversion tillage), with 31% extremely familiar (n=18 and n=14).
Grass-weed challenges, frequency, distribution and population pressure
Grower responses when asked to list their 5 most difficult to control weeds, and subsequent frequency counts on selected cereal fields across the 103 farms, identified Bromus spp., A. fatua, L. multiflorum, A. myosuroides and P. minor, in order of importance or frequency, as the most problematic or difficult-to-control grass weeds on their farms (Table 3), confirming grower responses corresponded to actual weed incidence. In Ireland, very few weed surveys have been conducted; however, many of the problem grass weeds found in cereals here (Table 3) are similar to those found in the UK, which has a similar climate (Froud-Williams and Chancellor, 1982; Chancellor and Froud-Williams, 1984). These species have now spread rapidly in north-western Europe, as a result of changes in farming practices and herbicide resistance evolution (Moss, 2004; Moss et al., 2007). Of the most economically impacting grass weeds in the UK crop farms ranked in order of importance, A. myosuroides, L. multiflorum, Avena spp., and Bromus spp., (e.g. Hull et al., 2014; Davies and Hull, 2018), only two (Bromus spp., and A. fatua) are common in Ireland, while P. minor was not listed in the UK surveys.
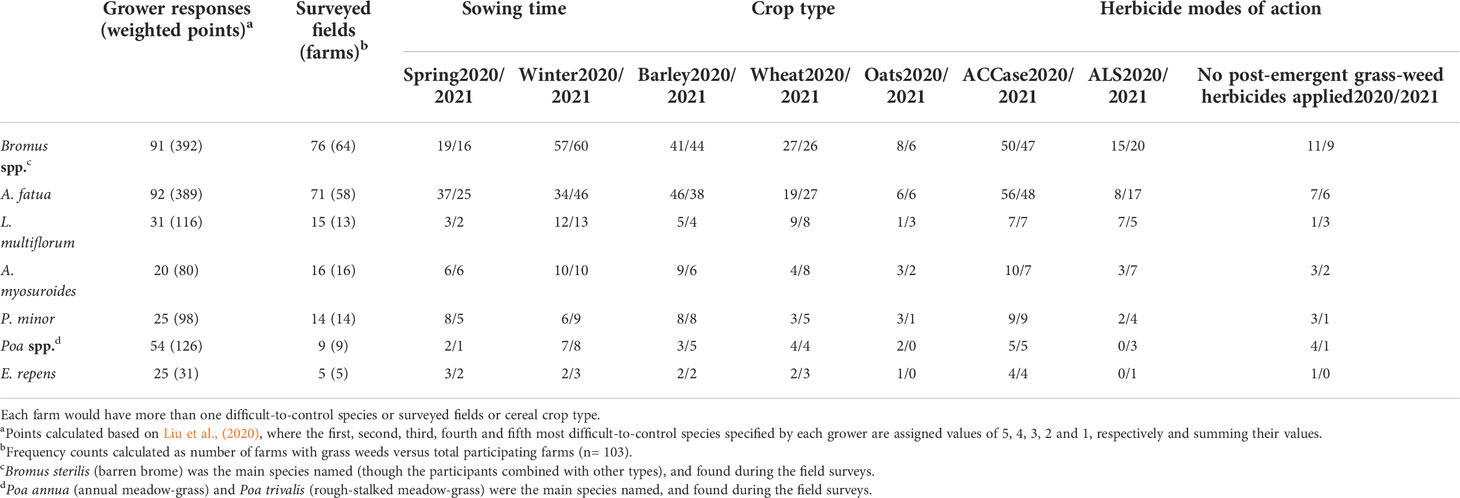
Table 3 Grower responses (n=103) and field survey information (based on counts) of the difficult-to-control grass weeds across 103 farms in both 2020 and 2021 assessment years.
The surveyed fields within plough (n=62) and non-inversion tillage (n=41) farms ranged in size from 2 to 110 ha, with around 61% (n=39 or n=24) being <20 ha. Nearly, 92% of surveyed fields within plough farms (n=57) were using that establishment system for 10 years or more, compared to only 17% of non-inversion tillage (n=7). The majority of the surveyed fields (61%) within non-inversion tillage farms (n=25) were in transition or had <5 years of their designated tillage practice. These characteristics suggest that Ireland is still at an early stage of non-inversion tillage adoption (Soane et al., 2012), while about 50% of UK farms are now using some form of non-inversion tillage (Townsend et al., 2016; Alskaf et al., 2020), despite interest from both countries since the 2000s (Forristal and Grant, 2011). Almost 65% (n=40) and 59% (n=24) of surveyed fields, respectively, within plough and non-inversion establishment farms had problems with the difficult-to-control grass weeds only recently (<5 years). Single and multiple grass-weed challenges were observed in 63% (n=39) and 37% (n=23), respectively, of surveyed fields within plough farms, compared to 56% (n=23) and 44% (n=18), respectively, within non-inversion tillage farms. Based on early adoption characteristics, the longer non-inversion tillage is practiced, the greater the grass-weed challenges becomes, especially for mixed grass weed populations (e.g. Hakansson, 2003; Cioni and Maines, 2010).
The weed score results based on grid-sampling are presented on a map, on an establishment system basis, for the key grass-weed species encountered (Figure 2A). A separate historical distribution based on botanical observations across all landscapes (BSBI, 2022) is also included (Figure 2B). Among 103 farms surveyed, the most widespread species in the main south and east arable region were Bromus spp., and A. fatua, which were found on 62% (n=64) and 56% (n=58) of farms, respectively (Table 3; Figure 2A), consistent with the historical distribution over all land types (Figure 2B). Previous Europe-wide surveys found that these two species have a high spread potential across Irish arable farms (e.g. Weber and Gut, 2005). In particular, B. sterilis was the most common type found on 51 of the 64 farms, the remaining farms consisted of eight with B. diandrus, four with B. hordeaceus and one with B. commutatus. Bromus sterilis is a competitive and fecund weed, found mainly at the bases of ditches, headlands and field margins, but also common within-crop fields, as it encroaches in from the field margins (Escorial et al., 2011; Davies et al., 2018). B. sterilis and B. diandrus have similar biology with peak autumn germination, the other types have more protracted emergence patterns. In the UK, a recent survey found that Bromus spp., were becoming more prevalent, increasing from 15% of the fields in 1982 (Chancellor and Froud-Williams, 1984) to approximately 60% in 2017 (Davies and Hull, 2018; Cook et al., 2021) with herbicide-resistant cases reported (Davies et al., 2018; Davies et al., 2020), coinciding with increased adoption of non-inversion tillage (Townsend et al., 2016). While in Ireland, the increase in winter barley area since 2014 coupled with earlier sowing and lack of effective herbicide options might be contributing factors for the increase in Bromus populations across all systems but especially, non-inversion crop establishment (Forristal and Murphy, 2009; CSO, 2021). Unlike the UK, where both winter-germinating (A. ludoviciana) and spring-germinating (A. fatua) wild oats occur, in Ireland, spring cereal cropping makes A. fatua by far the most common in cereal fields. High seedbank numbers coupled with long dormancy and seed survival rates on burying, make A. fatua highly competitive (Van Acker, 2009; Beckie et al., 2012). Herbicide-resistant A. fatua in Ireland (Alwarnaidu Vijayarajan et al., 2020) and herbicide-resistant Avena spp., in the UK (Hull et al., 2014) have been reported.
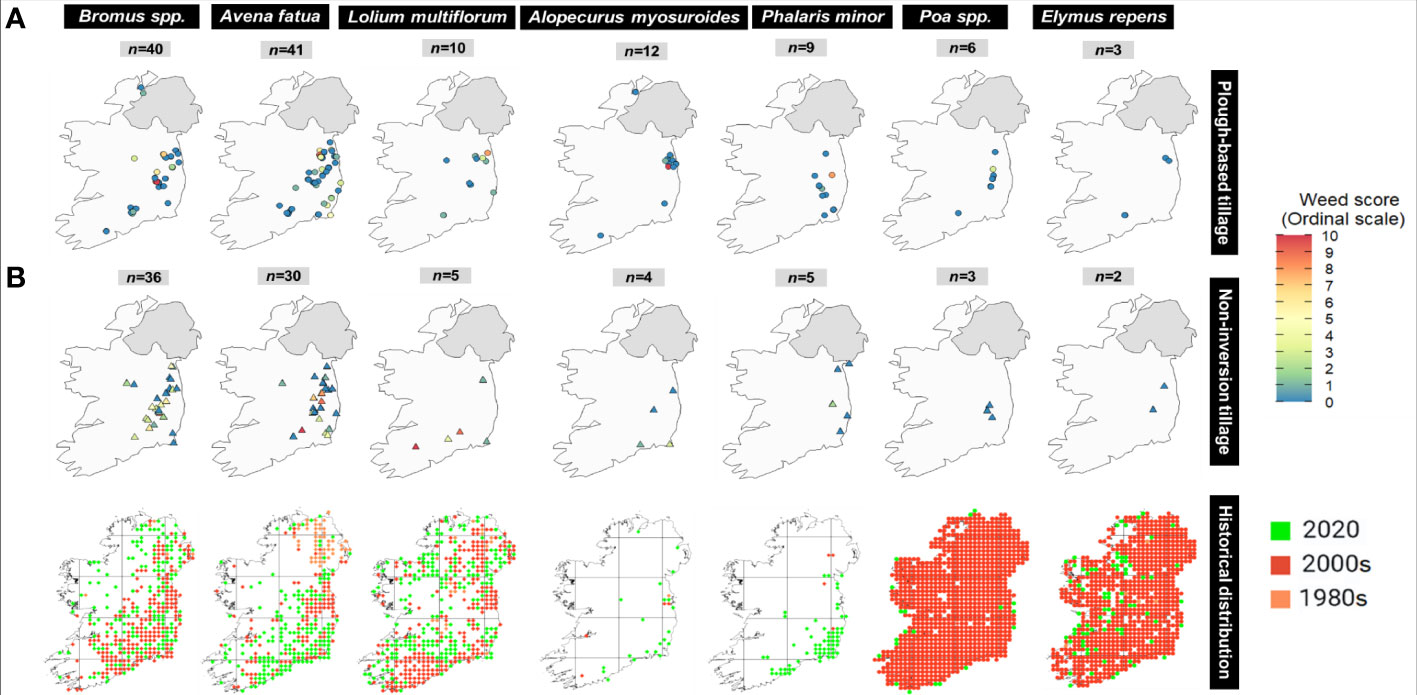
Figure 2 Field-level population pressure of the difficult-to-control grass weeds across plough (n=62) and non-inversion tillage (n=41) farms surveyed in 2020 and in 2021 (A) and the historical distribution derived from Botanical Society of Britain and Ireland (BSBI) (BSBI, 2022) atlases (B). The marker colors (A) relate to mean weed score measured on an ordinal scale from 0 (absent) to 10 (total weed cover). Frequency and distribution are indicated by the number and position of the markers. Data was recorded within 40 fields of 33 plough farms and 36 fields of 31 non-inversion tillage farms for Bromus spp. (n=64 farms); 41 fields of 35 plough and 30 fields of 23 non-inversion tillage for A fatua (n=58 farms); 10 fields of 8 plough and 5 fields of 5 non-inversion tillage for L. multiflorum (n=13 farms); and one field from each of plough and non-inversion tillage farms for P. minor (n=14 farms), A myosuroides (n=16 farms), Poa spp. (n=9 farms), and E repens (n=5 farms). Note: Each farm would have more than one difficult-to-control species or surveyed fields or cereal crop type.
While the frequency of L. multiflorum (n=13 farms), A. myosuroides (n=16 farms) or P. minor (n=14 farms) ranged between 13% and 16% of the 103 farms surveyed (Table 3; Figure 2A). These invasive species are relatively less prevalent in arable land (Figure 2B) but have increased only in recent years. Both L. multiflorum and A. myosuroides are becoming problematic in the east, especially, the Meath-Kildare-Dublin regions, where heavy soils are more suitable for winter cropping, and parts of Wexford-Waterford-Cork (Figure 2). Lolium multiflorum can occur as a weed of cereal crops in mixed rotations, which include grass, but it has not been reported as a serious weed in cereal rotations on arable farms in Ireland, until recently (Alwarnaidu Vijayarajan et al., 2021). The first incidence of A. myosuroides was reported in Ireland in 1980s (Figure 2B), it was only recorded as a problem in isolated cases, in contrast to the UK or Europe (Alwarnaidu Vijayarajan et al., 2021). In our survey, the majority of the growers with A. myosuroides believed that seeds entered their farm through use of second generation (C-2) seed imports from the UK, machinery moving from farm-to-farm, organic manure imports from other farms, or screenings from local mills. However, the exact source of A. myosuroides infestation is usually unknown, and it is possible that native and unintentionally imported seed may both be contributing to the recent increase in incidence, posing a significant challenge to the industry. In general, although both L. multiflorum and A. myosuroides were recorded only in few farms in Ireland, in contrast to the UK (e.g. Hull et al., 2014), the recent practices and associated resistance in isolated populations, and new weed introductions may contribute to the current importance assigned to L. multiflorum and A. myosuroides in Ireland (Alwarnaidu Vijayarajan et al., 2021). Phalaris minor is a relatively new weed, recorded since the 2000s, (Figure 2B), and there is little information on seed germination ecology necessary to devise IWM to stop the spread of P. minor in our climate. This species is problematic in the south and east especially, Wexford, east Cork and parts of Kildare regions (Figure 2), where continuous spring barley is the primary crop, and the continuous use of selective herbicides for controlling A. fatua and/or broad-leaved weeds, which, by reducing competition, may have facilitated its proliferation.
Although Poa spp. ranked third by weighted points (Table 3), each respondent specified it as only the third, fourth or fifth problem species. Similar to the UK (e.g. Chancellor and Froud-Williams, 1984), Poa spp. is widespread and abundant on all soils, including arable land (crop fields, headlands or field margins) in Ireland (Figure 2B), due to its high reproductive rate and germination. However, a low incidence as a problem weed on just 9% (n=9) of the 103 farms (Figure 2A) suggests effective control is possible with a range of herbicides (i.e. pre-emergence residual herbicides), accounting for growers considering it easier to control. Similarly, scutch grass (Elymus repens), a perennial propagating largely through rhizome, but also seed, in arable land, was specified by some respondents, but typically as the fourth or fifth problem species. This species had a huge impact on Irish and UK arable farms (e.g. Froud-Williams and Chancellor, 1982) before glyphosate was widely used, but only five of the surveyed 103 farms had E repens (Figures 2A, B), indicating that the use of glyphosate (pre-sowing and/or pre-harvest) has kept this weed under control with populations declining on arable land since the 1980s.
The overall weed scores (mean ± standard deviation) when averaged across all surveyed farms (Figure 2A), for B. sterilis, A. fatua, L. multiflorum, A. myosuroides, P. minor for both assessment years were 1.7 ± 2.5, 2.0 ± 2.7, 2.4 ± 3.2, 1.8 ± 2.6 and 1.6 ± 2.6, respectively, while this may indicate similar population pressure overall, the mean values can hide the variation between farms, where individual farms may have high scores for specific weeds. With a position-based weed scoring APP developed, these scores will serve as a baseline for monitoring changes in population pressure over time, where the same locations can be re-visited, similar to the monitoring of grass weeds on UK farms (e.g. Hull et al., 2014; Hicks et al., 2018). While farms with Poa spp., or E. repens had very low scores of 0.6 ± 1.3 and 0.5 ± 1.3, respectively, re-confirming that they both are currently not considered problematic or difficult-to-control.
Effects of management factors on grass-weeds
Management factors (except for soil type) had an impact on weed pressure of Bromus spp., A. fatua, L. multiflorum and A. myosuroides (Table 4 and Figure 3). In particular, the crop establishment systems had a statistically significant effect (P<0.05) on Bromus spp. and L. multiflorum, with non-inversion tillage farms having higher weed scores (2.2 ± 0.2 or 3.8 ± 0.7) than plough farms (1.2 ± 0.2 or 2.0 ± 0.7). We found 19% of the Bromus spp. (n=6 of 31) and 60% of L. multiflorum (n=3 of 5) with higher weed scores (i.e. mean scores from 4 to 10) on non-inversion tillage farms, compared to 6% (n=2 of 33) and 13% (n=1 of 8) respectively, for plough farms. For both species, the scores were higher on headlands than the in-field areas (Bromus spp., scores were 2.0 ± 2.5 on the headland and 0.8 ± 1.9 in-fields where ploughed vs. 3.0 ± 2.8 on the headland and 1.8 ± 2.6 in-fields with non-inversion tillage; L. multiflorum scores were 3.1 ± 3.6 on the headland and 1.4 ± 2.2 in-fields where ploughed vs. 4.4 ± 3.8 in the headland and 3.3 ± 3.8 within-fields with non-inversion tillage). Recent UK survey by Cook et al. (2021) also reported higher Bromus infestations in headlands and field margins (42% of fields), with density scores ranging from very low to high, as compared to in-crop (7% of fields), with very low to low scores.
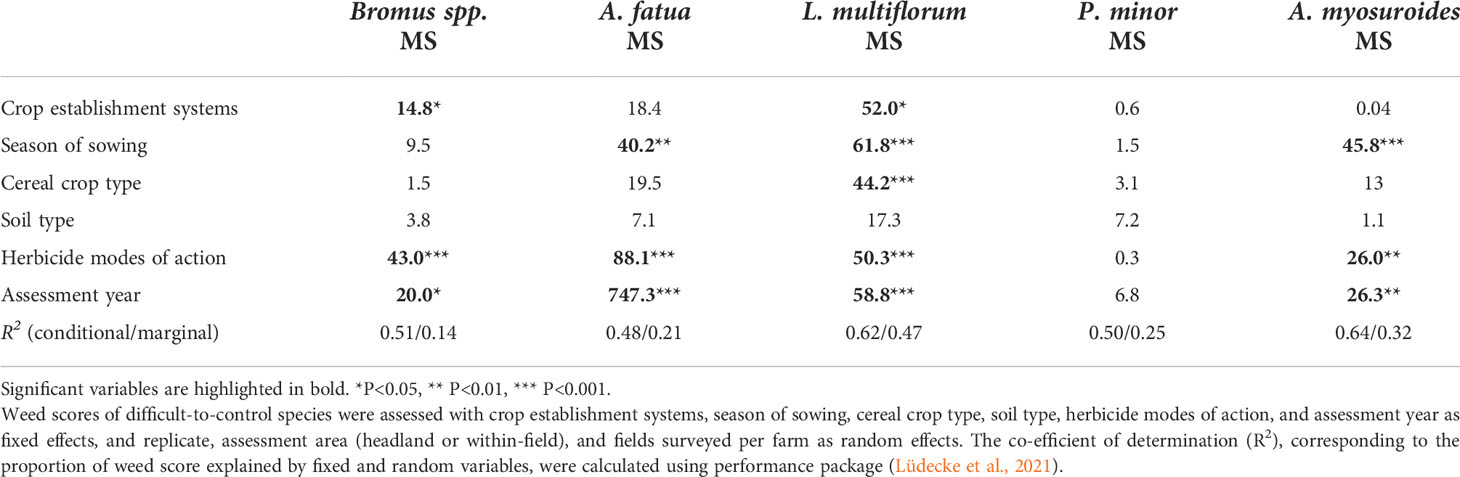
Table 4 Management factors which have an impact on weed pressure (weed score) as indicated by mean square (MS) values.
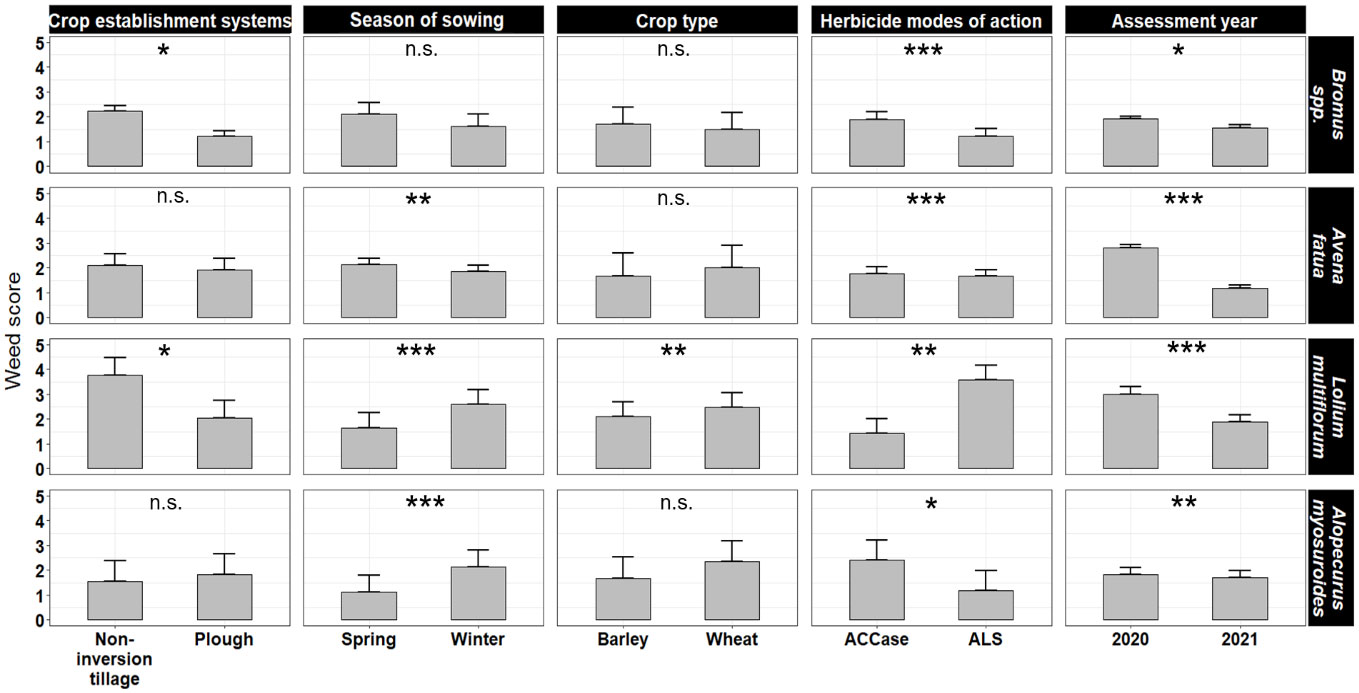
Figure 3 The impact of management factors on weed scores in four most problematic or difficult-to-control grass-weed species. *P<0.05, ** P<0.01, *** P<0.001, n.s. non-significant. Note: the emmeans package (Russell et al., 2022) was used for linear contrasts and standard errors for specified factors or factor combinations in a linear mixed model. Linear contrasts and standard errors for weed scores with oats (crop type) and no post-emergent herbicides applied (modes of action) could not be estimated, due to lack of factor combinations, hence, data was not included.
While overall, 18 farms had scores of 4 or more; 27% (n=11 of 41) of non-inversion tillage farms (including 6 with B. sterilis, 2 with L. multiflorum, 1 with A. fatua and 2 with mixed A. fatua and L. multiflorum, or A. fatua and P. minor combinations), and 11% (n=7 of 62) of plough farms (2 with A. fatua, 1 each with P. minor and A. myosuroides and 3 with mixed Bromus spp., or A. fatua and P. minor or A. fatua, B. diandrus and L. multiflorum combinations were present).
The season of sowing (i.e. winter vs. spring sowing) also had a significant effect on population pressure of A. fatua (P<0.01), and L. multiflorum and A. myosuroides (P<0.001) (Table 4). Independent of crop establishment systems, weed scores of A. fatua were higher with spring-sown cereals (2.1 ± 0.2) than with winter-sown (1.8 ± 0.2), while the opposite was the case for the two autumn-germinating grass weeds (1.6 ± 0.6 vs. 2.6 ± 0.6 for L. multiflorum or 1.1 ± 0.7 vs. 2.1 ± 0.2 for A. myosuroides) (Figure 3). These results highlight that non-inversion crop establishment systems in combination with autumn-sowing increase the abundance and proliferation of autumn-germinating species with short dormancy (<5 years), such as B. sterilis, L. multiflorum or A. myosuroides (Moss, 2005; Moss et al., 2007; Lutman et al., 2013; Davies et al., 2018).
With L. multiflorum, crop and herbicide types also affected the weed score (Table 4). This indicates that farms where ALS type herbicides (i.e. mesosulfuron + iodosulfuron or pyroxsulam) were used in wheat, there were higher weed scores (3.6 ± 0.6) for L. multiflorum where it was present (P<0.01) than those using ACCase-pinoxaden (1.4 ± 0.6) in wheat or barley (spring or winter) (Figure 3). For all other grass weeds (P<0.05), ALS use was associated with lower weed scores (Bromus, A. fatua and A. myosuroides scores, respectively, were 1.2 ± 0.3, 1.6 ± 0.3 or 1.2 ± 0.8) than ACCase type (i.e. pinoxaden or fenoxaprop) (1.9 ± 0.3, 1.8 ± 0.3 or 2.4 ± 0.8) (Figure 3), but this result should be interpreted with caution, as a high proportion of surveyed fields had barley (winter or spring) crops, which did not allow ALS inhibitor products to be used (Table 3). About 65% of farms (n=41 plough and n=26 non-inversion tillage), used the same herbicide for grass-weed control in the same surveyed fields in both assessment years. This is not surprising, as there is no effective herbicide options available for Bromus spp. or A. myosuroides control in barley, and only ALS inhibitors in wheat, and virtually, no post-emergent options available for grass-weed control in oats, in Ireland. Our results indicate that weed pressure is likely impacted by management (e.g. establishment system, season of sowing, herbicide use, etc.), but there are also other farm-specific factors relating to the presence of specific species and their resistance status, which impacts on their control, also noted by Herrmann et al (2016).
Weed scores for difficult-to-control grass weeds were significantly (P<0.05) higher in 2020 than in 2021 (Bromus, A. fatua, L. multiflorum and A. myosuroides scores, respectively, were 1.9 ± 0.1 vs. 1.6 ± 0.1, 2.8 ± 0.1 vs. 1.2 ± 0.1, 3.0 ± 0.3 vs. 1.9 ± 0.3, or 1.8 ± 0.2 vs. 1.6 ± 0.2) (Table 4; Figure 3), but it is known that population pressure varies across seasons, attributed to weather variations, or timing of weed control or herbicide use, or possibly, resistance (Yu and Powles, 2014). Also the lower 2021 weed scores could be the growers decision to use more effective herbicides or IWM, following communication of individual sensitivity results from the 2020 season.
Grass-weed Sensitivity Status
Among 161 grass-weed populations, including Bromus spp. (n=64), A. fatua (n=58), L. multiflorum (n=9), A. myosuroides (n=14), P. minor (n=14) and Poa spp. (n=2) screened for resistance, 22 populations found on 18 of 103 farms were found to be resistant, with three of those farms having two or more resistant species (Figure 4).
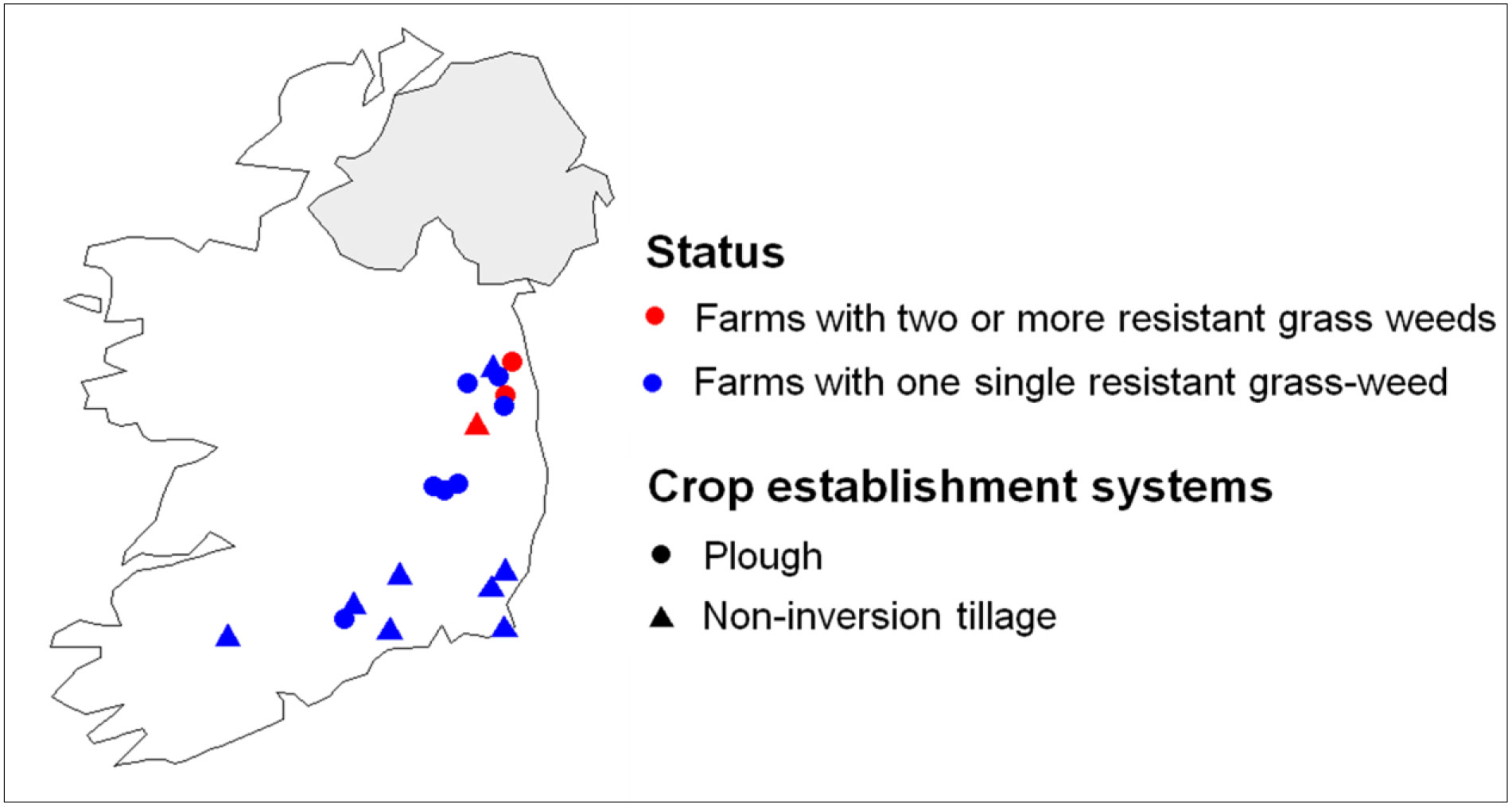
Figure 4 Twenty-two resistant grass weeds found on 18 of 103 farms, three of which contained two or more resistant species.
The individual herbicide sensitivity status of these populations and the corresponding weed scores for farms with a single resistant grass-weed species is presented in Table 5, for farms with herbicide resistance in multiple grass weeds in Table 6. Overall 18% of farms had resistance weeds; 15% of plough (n=9 of 62) farms and 22% of non-inversion tillage (n=9 of 41) farms (including one shallow min-till, five deep min-till, two direct-drill and one strip-till) had resistance recorded and this resistance was found in the main southeast regions (Figure 4; Tables 5, 6). Coupled with the wider occurrence of some weed species on non-inversion tillage farms (Table 4), and the existing reliance on glyphosate for pre-sowing weed control, these results may threaten the effectiveness of non-inversion tillage, and may limit opportunities for future adoption in this region (e.g. Soane et al., 2012). In 18 farms (10 had score from 0 to 3 and 8 had score from 4 to 10) that had developed resistance, no trend was observed in terms of weed scores (Tables 5, 6), indicating that reducing populations to manageable levels may not necessarily minimize resistance risk, if same herbicide (including rates and application timing) is used consecutively in the same field (Escorial et al., 2011; Hicks et al., 2018). While farms with resistant A. myosuroides, it is unclear if these resistant strains were introduced in which case there would be no relationship with population pressure.
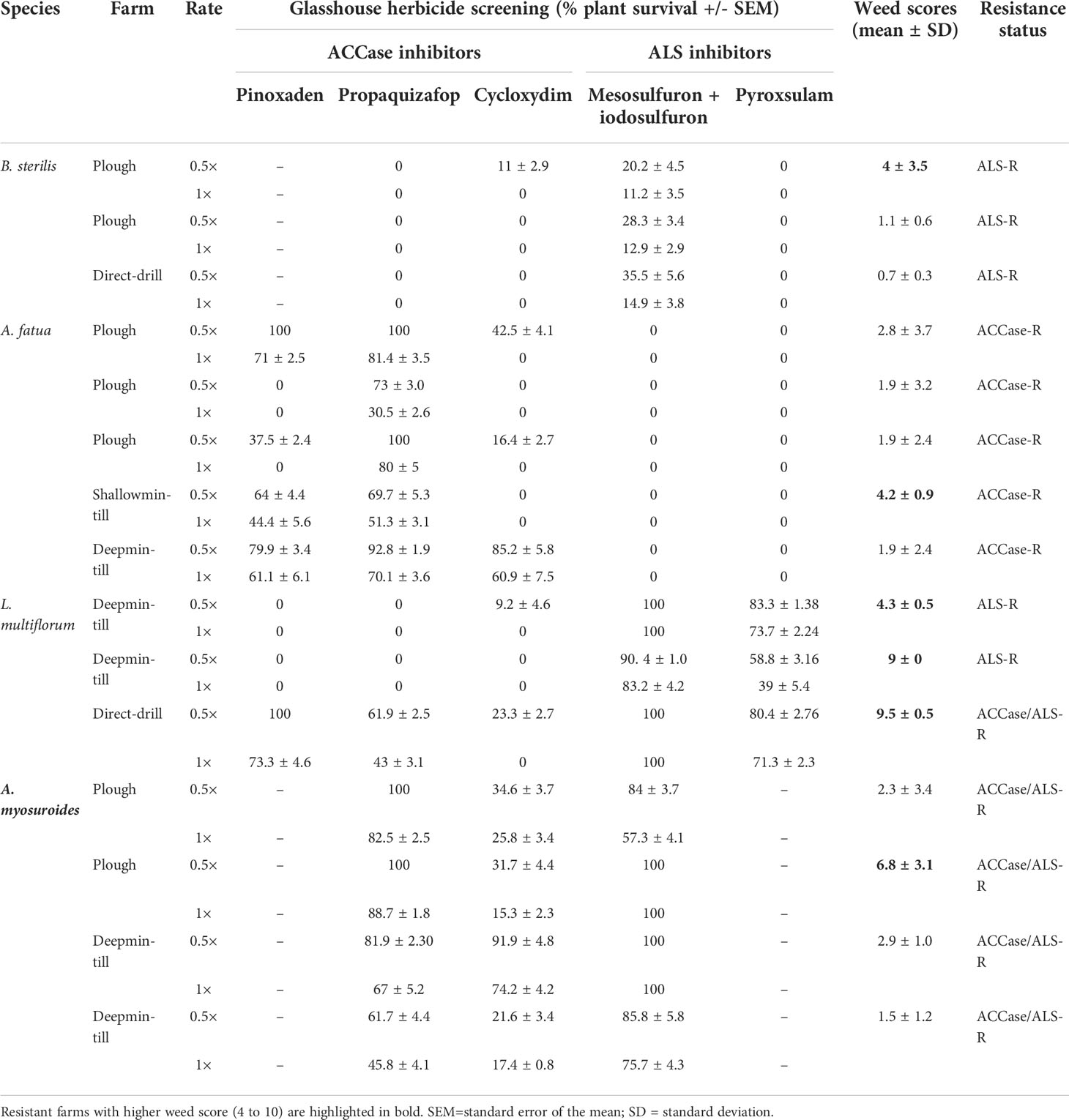
Table 5 Herbicide sensitivity status and weed scores for 15 farms with resistant grass-weed (single species) populations.
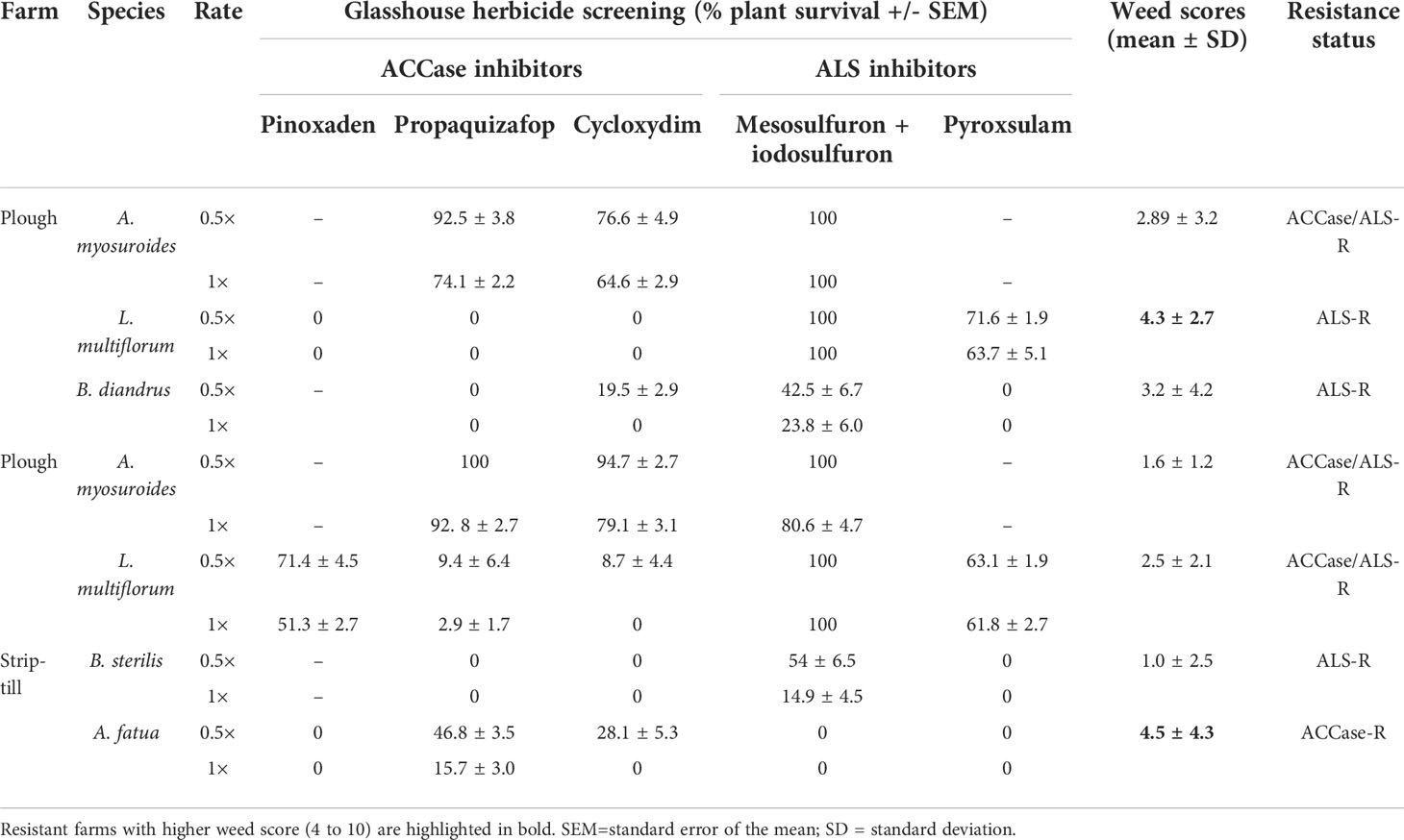
Table 6 Herbicide sensitivity status and weed scores for three farms with multiple resistant grass-weed populations.
Among the grass weeds screened for resistance, five of the 64 Bromus populations (four B. sterilis and one B. diandrus) survived both half and full rate of ALS-mesosulfuron + iodosulfuron (Tables 5, 6) with ten more populations showing variation in sensitivity to half rate of ACCase-cycloxydim and 20 more to half-rate of ALS-mesosulfuron + iodosulfuron, with percent plant survival varying from 10 ± 5.77 to 54 ± 8.45. For A. fatua, six of the 58 populations were ACCase-resistant, including one population resistant to all three ACCase types: pinoxaden, propaquizafop and cycloxydim; two populations resistant to pinoxaden and propaquizafop, and three populations were propaquizafop-resistant only (Tables 5, 6). For L. multiflorum, five of the nine populations were ALS-resistant with two of these ACCase-resistant (Tables 5, 6). The reduced efficacy of ALS inhibitors on L. multiflorum, may have contributed to significantly higher weed scores (3.6 ± 0.6 for ALS vs. 1.4 ± 0.6 for ACCase) specifically in the assessment year 2021 (3.0 ± 0.3 for 2020 vs. 1.9 ± 0.3 for 2021), on farms that used this herbicide type in winter wheat (Figure 3). For A. myosuroides, six of the 14 populations were resistant to both ACCase-(propaquizafop and cycloxydim) and ALS-(mesosulfuron + iodosulfuron) herbicides. Dose-response and genetic studies in some L. multiflorum and A. myosuroides populations from this survey revealed ACCase NTSR and/or ALS Pro-197 mutations, or stacked ACCase Ile-1781 and ALS Pro-197 mutations conferring resistance (Alwarnaidu Vijayarajan et al., 2021). No resistance to ACCase/ALS inhibitors were found in P. minor and Poa spp. populations.
Overall, we found herbicide resistance in 8% of Bromus and 10% of A. fatua populations, implying that resistance evolves slowly among self-pollinating Bromus or self-pollinating hexaploid A. fatua (e.g. Mansooji et al., 1992; Busi et al., 2016; Davies et al., 2020); these species also having alternative herbicide chemistries available to eliminate or control resistant populations. In contrast, only a few surveyed farms had L. multiflorum and/or A. myosuroides, but 56% and 43% of these populations, respectively, were resistant; confirming that genetically diverse obligate outcrossing species are more likely to develop complex resistance patterns including cross- and multiple resistance (Délye et al., 2010; Holt et al., 2013), leaving very little herbicide control options. Resistance can develop in as little as three years of consecutive use of high-risk ACCase/ALS inhibitors, in outcrossing species (Hicks et al., 2018). For L. multiflorum, ACCase-cycloxydim or clethodim, and for A. myosuroides, ACCase-clethodim, at full rate, in sequence with HRAC group 3 propyzamide may offer initial control of resistant populations in the year where non-cereal break crops are grown, but crop rotation sequence restrictions may limit the effectiveness of these alternative options (Alwarnaidu Vijayarajan et al., 2021).
On-farm grass-weed control practices
When asked about IWM practices adopted, the top 3 were herbicide based: targeting optimum timing of herbicide application (87%); using glyphosate prior to sowing (82%); and using pre-emergent selective residual herbicides (79%) (Table 7). Herbicide strategy did not differ between crop establishment types (Table 7).
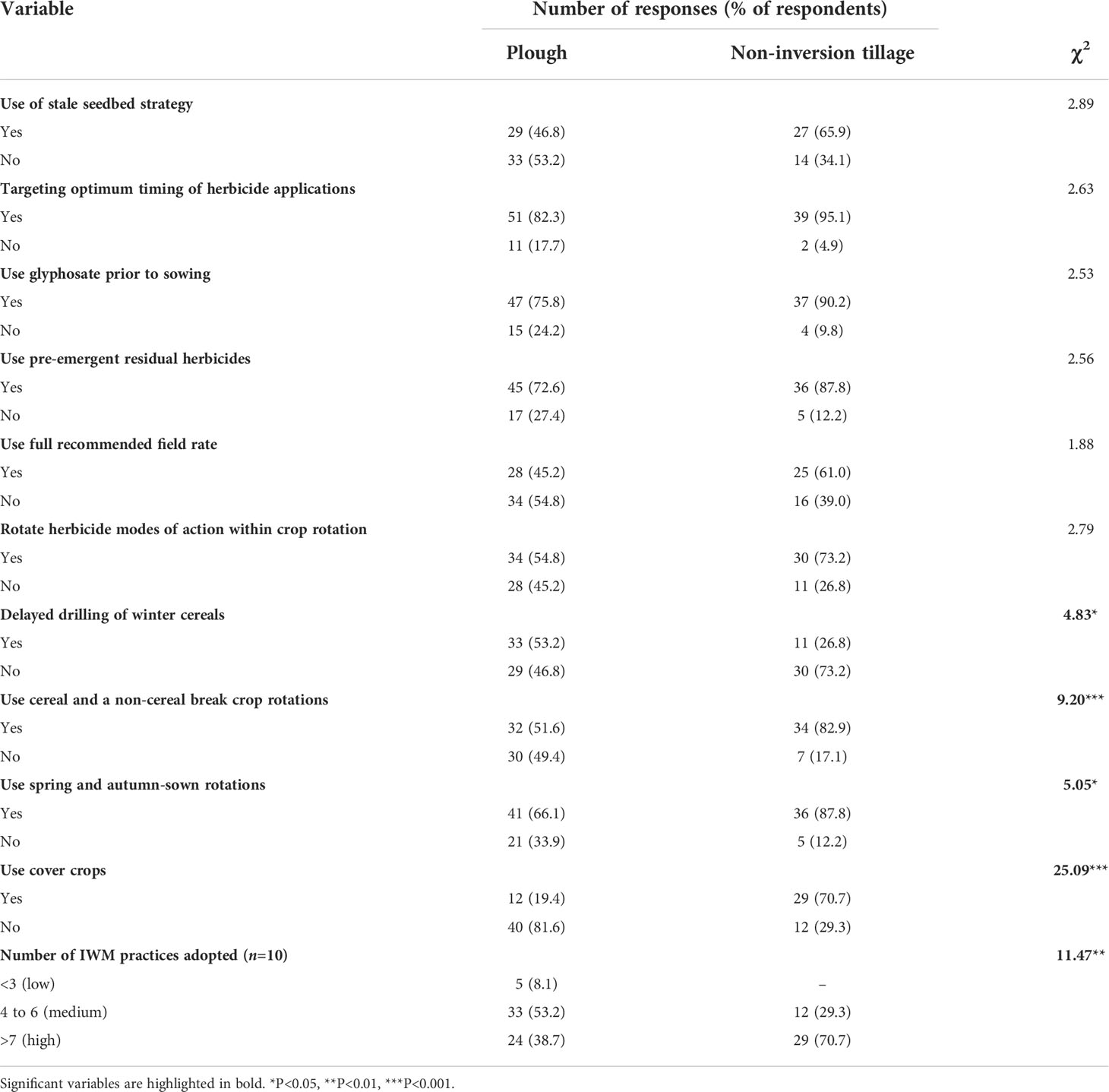
Table 7 On-farm grass-weed control practices of plough (n=62) and non-inversion tillage (n=41) farms and their associations.
Targeting optimum timing of herbicide application is key for ensuring maximum product efficacy. Glyphosate is applied before cultivation mainly for E. repens control, or prior to winter cereal sowing as part of a stale seedbed strategy (54%, n=56) (Table 7) to deplete the top soil layer of weed seedbank of B. sterilis, in particular. Residual herbicides are applied pre-emergence (24-48 hours after drilling) or early post-emergence (autumn treatment) of the crop and weed, mainly for Poa spp., control and frequently in combination with other herbicides to give broad-leaved weed control, or to get very limited control of B. sterilis. These strategies offer early season grass-weed control and may improve the efficacy of post-emergent spring ACCase/ALS herbicides. These strategies may delay the resistance evolution to high-risk ACCase/ALS inhibitors (Moss et al., 2019); rotating herbicide types within crop rotation (62%, n=64) was common on the surveyed farms (Table 7). Resistance to pre-emergence treatment, tends to be only partial and builds up slowly, compared to ACCase and ALS herbicides (Moss et al., 2019), therefore, its use is strongly promoted to manage populations resistant to single site post-emergent spring herbicides (Bailly et al., 2012; Marshall et al., 2013), and to protect the few post-emergent options (Ulber and Rissel, 2018).
Another parameter that affects product efficacy is the herbicide rate; half of the 103 farms, used less than the recommended field rate of ACCase/ALS inhibitors (Table 7). Previous research (e.g. Espeby et al., 2011; Escorial et al., 2011), suggests that shifts in susceptibility or creeping resistance in Bromus populations, such as recorded in this study (n=30 of 64 farms), may be related to the widespread use of lower rates. We also found a range of glyphosate rates used from 360 to 1440 g ha-1. The recommended field rate of glyphosate (i.e. 540 g ha-1 for annual grass-weed control on stubbles) effectively controlled ACCase- and/or ALS-resistant L. multiflorum populations but a higher rate of 810 g ha-1 was necessary to control both sensitive and resistant A. myosuroides (Alwarnaidu Vijayarajan et al., unpublished). In Europe, glyphosate-resistant Lolium spp. in Italy (Collavo and Sattin, 2014), and reduced glyphosate sensitivity in UK in B. sterilis and A. myosuroides (Davies and Neve, 2017; Davies et al., 2018) has been already documented.
Delayed autumn crop drilling can reduce the challenge of B. sterilis, L. multiflorum or A. myosuroides (Lintell Smith et al., 1999; Reverte, 2010; Moss, 2017). A larger proportion of plough farms (53%) adopted delayed drilling (from mid-October) than non-inversion tillage farms (27%) (Table 7). Ploughing is versatile and reliable across weather types, while wet autumns pose a greater challenge to non-inversion tillage, most practitioners adopt earlier autumn-sowing (from mid-September) to reduce the risk of poor crop establishment (Soane et al., 2012; Brennan et al., 2015). This approach, however, can exacerbate grass-weed challenge in our climate (Forristal and Murphy, 2009), and has impacted on non-inversion tillage adoption in these regions (Soane et al., 2012).
A higher proportion of non-inversion tillage farms (>80%) practice crop rotations, and more use cover crops (71%), compared to those ploughing (Table 7). Rotations offers the opportunity to use non-ACCase and non-ALS modes of action for controlling the seedbank of high resistance-risk species (e.g. propyzamide in winter oilseed rape) (e.g. Moss et al., 2019) or using spring cropping to control autumn-germinating grass weeds by avoiding crop establishment in the peak germination period in autumn (e.g. Zeller et al., 2018). Cover crops were probably adopted as a nutrient capture technique, but can have a weed control effect in some situations (e.g. Cussans and Storkey, 2018).
Non-inversion tillage growers used a greater number of IWM practices than ploughing (71% vs. 39% >7 practices). More than 90% of growers in general, use 4 or more IWM practices agreeing with Creissen et al. (2019) that Irish growers were open to adopting IPM type strategies. The combination of chemical and cultural practices may have contributed to the lower grass-weed population pressure, with 83% of the farms (n=85 of 103) having lower weed scores (0 to 3), and may have reduced resistance, with 86% of populations (n=139 of 161) sensitive to ACCase/ALS inhibitors at full field rate. The use of these practices may have helped slow the development of L. multiflorum or A. myosuroides. Ulber and Rissel (2018) also report similar pro-active behavior among German farmers. However, the situation relating to L. multiflorum and A. myosuroides resistance is of concern. IWM practices such as stale seedbed in autumn, delayed autumn-drilling or autumn-applied pre-emergent herbicides have little effect on spring A. fatua control. In addition, plants may escape ACCase/ALS herbicide applications, due to sporadic germination, resulting in the presence of herbicide-susceptible A. fatua at crop harvest. Specific actions like hand rogueing for surviving plants, and the use of other IWM measures (e.g. planting of more competitive crops, use of higher than normal seeding rate, etc.) is necessary to reduce the weed seedbank of A. fatua.
Characteristics of growers and their practices of different establishment systems
The binary logistic regression model allowed us characterise non-inversion practitioners (Table 8) as: young (<41 years); having formal education (level 7 or above education); managing larger-sized (>350 ha) mixed farms and practicing diversified rotations using cereal/non-cereal break crop combinations with a inclusion of cover crops. Several previous studies, similar to this one, show contemporary farms (characterised by young generations or new entrants into farming, high education level and large farm size) differed from older farmers, and are more willing to embrace alternative farming practices (e.g. non-inversion tillage, organic farming or environmental protection measures) in response to external drivers, including policy changes, for a sustainable, profitable and production competitiveness future for arable crop production (e.g. Knowler and Bradshaw, 2007; Lobley et al., 2009; Bartkowski et al., 2022).
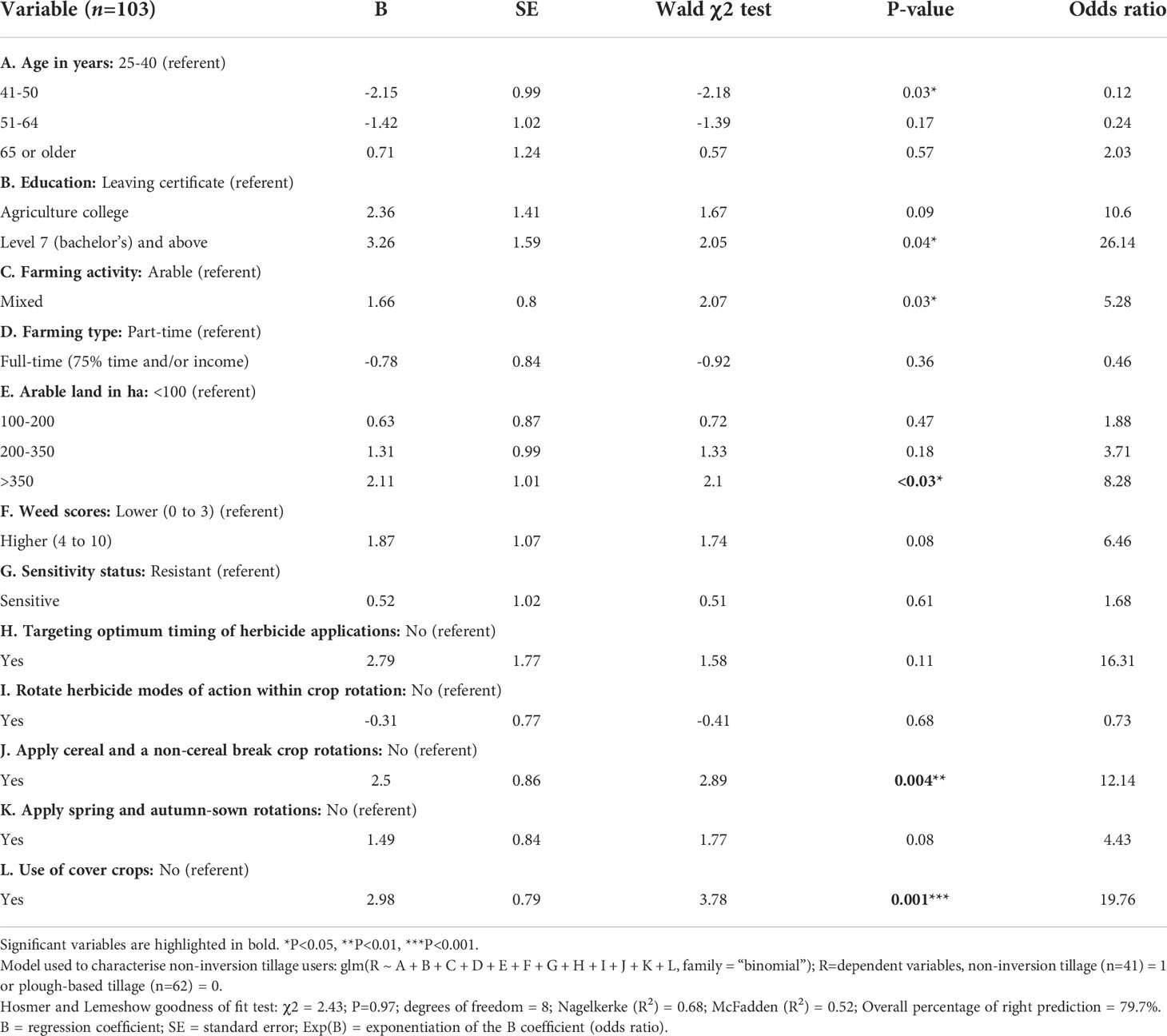
Table 8 Relationship of crop establishment types (in this case, non-inversion tillage farms) and selected independent variables using binary logistic regression model.
Conclusion
This is the first multi-component survey in Ireland exploring grass-weed frequency counts, population pressure based on weed scores, mapping herbicide-resistant grass-weed distribution, and IWM used, across farms with different crop establishment systems. The results indicate grass-weed challenges are increasing and specifically;
(a) Non-inversion tillage farms tend to have younger growers with formal education and larger farms, indicating the scope for adoption of newer weed control measures.
(b) The high level of awareness of grass-weed herbicide resistance (>80%) and IWM familiarity (>65%) coupled with their stated adoption of a number of IWM practices (>90% using 4 or more), including the use of herbicide rotations (62% n=64) and crop rotation types (64%, n=66 or 75%, n=77) indicates there is scope for uptake of more sustainable IWM actions that will be necessary to deal with the problem grass weeds against a background of evolving resistance and new weed introductions.
(c) While the adoption of cultural/non-chemical IWM was higher among growers practicing non-inversion tillage, herbicide use was no less; population pressure and/or herbicide resistance was indicating that early-sown winter cereals established after non-inversion tillage present a greater challenge for grass-weed control in our Atlantic climate requiring urgent development and adoption of effective IWM that will reduce reliance on herbicides.
(d) The apparent speed at which resistance is evolving in L. multiflorum (56% were ACCase and/or ALS-resistant) and A. myosuroides (43% were ACCase/ALS-resistant), considering these weeds were not that prevalent, is worrying because of their documented resistance profile and may challenge sustainable crop production.
(e) As Ireland, is at a relatively earlier point of resistance evolution, extreme IWM measures, such as whole cropping (removing crop before seed shed), use of grass leys or fallows, etc., should be adopted for these high resistance-risk species. On-farm biosecurity measures, such as machinery hygiene, avoiding straw and manure imports, using C-1 certified seeds, should be implemented, particularly for high resistance risk species. For low resistance-risk grass weeds, alternative herbicide options should be used in combination with site-specific IWM, for effective and immediate control of Bromus spp., and A. fatua.
(f) The weed scores, of widespread B. sterilis or A. fatua, as well as relatively new species like L. multiflorum, A. myosuroides, P. minor and other Bromus types, ranged from 0 to 3, emphasizing the need for resistance monitoring in all of these species. In addition, climate-specific research is critical to understand these developments and associated factors and to develop appropriate IWM strategies is necessary to limit their spread and prevent resistance development.
(g) It’s likely the resistance pattern evolution in Ireland relative to the UK, is related to cropping patterns and farming systems. Prior to the mid-1970s, most annual crops were produced on mixed farms with short- and medium-term grass leys restricting the opportunities for grass weeds to proliferate and avoiding herbicide selection pressure, unlike in the UK, where earlier crop specialization resulted allowed these problems to develop earlier (e.g. Moss, 2017; Hicks et al., 2018). But these challenges are developing in Ireland now.
(h) Overall, this survey research has generated baseline data from which future changes in weed types, population pressure and the impact of IWM efforts will be benchmarked.
Data availability statement
The raw data supporting the conclusions of this article will be made available by the authors, without undue reservation.
Author contributions
Conceptualization: VV, PF, SB and MH. Methodology and investigation: VV, RF, NO, and SC. Data curation, formal analysis and writing the original draft: VV. Supervision: SB, PF, and MH. Reviewing and editing: VV, PF, NO and SC. Funding acquisition: MH. All authors contributed to the article and approved the submitted version.
Funding
This research was funded through the project ECT (Enable Conservation Tillage, Grant No: LLOC1079) which is a European Innovation Partnership (EIP) funded by the Department of Agriculture, Food, and the Marine (DAFM) under the Rural Development Programme 2014–2020.
Acknowledgments
We thank John Mahon, Jimmy Staples, David Schilder and Teagasc crop advisors for their assistance with the questionnaire and field surveys. We acknowledge input of all participating growers and project stakeholders. A special thanks to Brian Moran for survey support, Dr. Seán Lacy (Munster Technological University, County Cork, Ireland) for his statistical advice, and to Drs. Stephen Byrne and Agnieszka Konkolewska for their assistance in mapping spatial data. We also thank Eoghan Stynes and Capucine Monier for their technical support with glasshouse experiments.
Conflict of interest
The authors declare that the research was conducted in the absence of any commercial or financial relationships that could be construed as a potential conflict of interest.
The funders had no role in the design of the study; in the collection, analyses, or interpretation of data; in the writing of the manuscript, or in the decision to publish the results.
Publisher’s note
All claims expressed in this article are solely those of the authors and do not necessarily represent those of their affiliated organizations, or those of the publisher, the editors and the reviewers. Any product that may be evaluated in this article, or claim that may be made by its manufacturer, is not guaranteed or endorsed by the publisher.
References
Abdalla M., Hastings A., Helmy M., Prescher A., Osbore B., Lanigan G., et al. (2014). Assessing the combined use of reduced tillage and cover crops for mitigating greenhouse gas emissions from arable ecosystem. Geoderma 223, 9–20. doi: 10.1016/j.geoderma.2014.01.030
Alskaf K., Sparkes D. L., Mooney J. S., Sjögersten S. (2020). The uptake of different tillage practices in England. Soil Use Manage. 36, 27–44. doi: 10.1111/sum.12542
Alwarnaidu Vijayarajan V. B., Forristal D. P., Cook S. K., Schilder D., Staples J., Hennessy M., et al. (2021). First identification and characterization of cross- and multiple resistance to acetyl-CoA carboxylase (ACCase)- and acetolactate synthase (ALS)-inhibiting herbicides in black-grass (Alopecurus myosuroides) and Italian ryegrass (Lolium multiflorum) populations from Ireland. Agriculture 11, 1272. doi: 10.3390/agriculture11121272
?>Alwarnaidu Vijayarajan V. B., Forristal D. P., Cook S. K., Staples J., Schilder D., Hennessy M., et al. (2020). First report on assessing the severity of herbicide resistance to ACCase inhibitors pinoxaden, propaquizafop and cycloxydim in six Avena fatua populations in Ireland. Agronomy 10, 1362. doi: 10.3390/agronomy10091362
Bailly G. C., Dale R. P., Archer S. A., Wright D. J., Kaundun S. S. (2012). Role of residual herbicides for the management of multiple herbicide resistance to ACCase and ALS inhibitors in a black-grass population. Crop Protect. 34, 96–103. doi: 10.1016/j.cropro.2011.11.017
Bartkowski B., Schüßler C., Müller B. (2022). Typologies of European farmers: approaches, methods and research gaps. Reg. Environ. Change. 22, 43. doi: 10.1007/s10113-022-01899-y
Base Ireland (2018). Conservation agriculture: submission to the joint commission on agriculture, food and the marine. In: Biodiversity agriculture soil & environment Ireland. Available at: https://data.oireachtas.ie/ie/oireachtas/committee/dail/32/joint_committee_on_agriculture_food_and_the_marine/submissions/2018/2018-05-15_submission-conor-o-flaherty-biodiversity-agriculture-soil-environment-base-ireland_en.pdf (Accessed 09 February 2022).
Beckie H. J., Francis A., Hall L. M. (2012). The biology of Canadian weeds. 27. Avena fatua l. (updated). Can. J. Plant Sci. 92, 1329–1357. doi: 10.4141/cjps2012-005
Bijttebier J., Ruysschaert G., Hijbeek R., Werner M., Pronk A. A., Zavattaro L., et al. (2018). Adoption of non-inversion tillage across Europe: Use of a behavioural approach in understanding decision making of farmers. Land Use Policy. 78, 460–471. doi: 10.1016/j.landusepol.2018.05.044
Brennan J., Hackett R., McCabe T., Forristal P. D. (2014). The effect of tillage system and residue management on grain yield and nitrogen use efficiency in winter wheat in a cool Atlantic climate. Eur. J. Agron. 54, 61–69. doi: 10.1016/j.eja.2013.11.009
Brennan J., McCabe T., Hackett R., Forristal P. D. (2015). The effect of soil tillage system on the nitrogen uptake, grain yield and nitrogen use efficiency of spring barley in a cool Atlantic climate. J. Agric. Sci. 153 (5), 862–875. doi: 10.1017/S0021859614000471
Busi R., Marcelo G., Powles S. B. (2016). Response to low-dose herbicide selection in self-pollinated Avena fatua. Pest Manage. Sci. 72, 603–608. doi: 10.1002/ps.4032
Byrne R. K., McCabe T., Forristal P. D. (2022). The impact of crop establishment systems in combination with applied nitrogen management on the establishment, growth and yield of winter oilseed rape in a mild Atlantic climate. Eur. J. Agron. 139, 126566. doi: 10.1016/j.eja.2022.126566
Byrne R., Vijaya Bhaskar A. V., Spink J., Freckleton R., Neve P., Barth S. (2021). The occurrence of herbicide-resistant Avena fatua (wild oats) populations to ACCase-inhibiting herbicides in Ireland. Irish J. Agric. Food Res. 60(1), 59–64 doi: 10.15212/ijafr-2020-0127
Chancellor R. J., Froud-Williams R. J. (1984). A second survey of cereal weeds in central southern England. Weed Res. 24, 29–36. doi: 10.1111/j.1365-3180.1984.tb00568.x
Cioni F., Maines G. (2010). Weed control in sugar beet. Sugar Tech. 12, 243–55 doi: 10.1007/s12355-010-0036-2
Collavo A., Sattin M. (2014). First glyphosate-resistant Lolium spp. biotypes found in a European annual arable cropping systems also affected by ACCase and ALS resistance. Weed Res. 54, 325–334. doi: 10.1111/wre.12082
Comtosis D. (2022) R core package ‘summary tools’: tools to quickly and neatly summarise data. Available at: https://cran.r-project.org/web/packages/summarytools/summarytools.pdf (Accessed 02 February 2022).
Cook S. K., Tatnell L., Godfrey K., Hull R., Moss S., Davies L. (2021). Investigating the distribution, presence and potential for herbicide resistance of UK brome species in arable farming. AHDB project Rep. No 636, 1–131.
Creissen H. E., Jones P. J., Tranter R. B., Girling R. D., Jess S., Burnett F. J., et al. (2019). Measuring the unmeasurable? a method to quantify adoption of integrated pest management practices in temperate arable farming systems. Pest Manage. Sci. 75, 3144–3152. doi: 10.1002/ps.5428
CSO (2016) Structure of farming in Ireland 2016, central statistics office (CSO). Available at: https://www.cso.ie/en/releasesandpublications/ep/p-fss/farmstructuresurvey2016/ (Accessed 01 March 2021).
CSO (2020) Demographic profile of farm holders 2020, central statistics office (CSO). Available at: https://www.cso.ie/en/releasesandpublications/ep/p-coa/censusofagriculture2020-preliminaryresults/demographicprofileoffarmholders/ (Accessed 01 March 2021).
CSO (2021) Area, yield and production of crops 2021, central statistics office (CSO). Available at: https://www.cso.ie/en/releasesandpublications/ep/p-aypc/areayieldandproductionofcrops2021/ (Accessed 01 March 2021).
Cussans J., Storkey J. (2018). 'Added value fallows' the use of customised cover cropping approaches within integrated grass weed management. AHDB Cereals Oilseeds Project Rep. No 597, 1–41.
DAFM (2016). Pesticide usage in Ireland, arable crops survey report 2016, pesticide registration and control divisions (PRCD) of the department of agriculture; food and the marine (DAFM), Co (Ireland: Kildare). Available at: https://www.pcs.agriculture.gov.ie/media/pesticides/content/sud/pesticidestatistics/ArableReport2016Final100620.pdf (Accessed 08 April 2022).
DAFM (2022a). Greening payment as a part of basic payment scheme 2022. department of agriculture, food and the marine (DAFM). Available at: https://www.gov.ie/en/service/be0e94-greening-payment/ (Accessed 12 May 2022).
DAFM (2022b) Overview of agri-climate rural environment scheme (ACRES): Ireland’s CAP strategic plan 2023-2027. In: Department of agriculture, food and the marine (DAFM). Available at: https://www.gov.ie/en/service/f5a48-agri-climate-rural-environment-scheme-acres/ (Accessed 12 May 2022).
Davies L. R., Hull R. (2018). Presence and distribution of brome weeds in UK arable farming. Aspects Appl. Biol. 141, 37–46.
Davies L. R., Hull R., Moss S., Neve P. (2018). The first cases of evolving glyphosate resistance in UK poverty brome (Bromus sterilis) populations. Weed Sci. 67, 41–47 doi: 10.1017/wsc.2018.61
Davies L. R., Neve P. (2017). Interpopulation variability and adaptive potential for reduced glyphosate sensitivity in Alopecurus myosuroides. Weed Res. 57, 323–332. doi: 10.1111/wre.12264
Davies L., Onkokesung N., Brazier-Hicks M., Edwards R., Moss S. (2020). Detection and characterization of resistance to acetolactate synthase inhibiting herbicides in Anisantha and Bromus species in the united kingdom. Pest Manage. Sci. 76, 2473–2482. doi: 10.1002/ps.5788
Délye C., Michel S., Bérard A., Chauvel B., Brunel D., Guillemin J. P., et al. (2010). Geographical variation in resistance to acetyl-coenzyme a carboxylase-inhibiting herbicides across the range of the arable weed Alopecurus myosuroides (black-grass). New Phytol. 186, 1005–1017. doi: 10.1111/j.1469-8137.2010.03233.x
Escorial C., Loureiro I., Rodriguez-Garcia E., Chueca C. (2011). Population variability in the response of ripgut brome (Bromus diandrus) to sulfosulfuron and glyphosate herbicides. Weed Sci. 59, 107–112. doi: 10.1614/WS-D-10-00033.1
Espeby L. A., Fogelfors H., Milberg P. (2011). Susceptibility variation to new and established herbicides: Examples of inter-population sensitivity of grass weeds. Crop Protect. 30, 429–435. doi: 10.1016/j.cropro.2010.12.022
Forristal D., Grant J. (2011). The impact of break-crop and cereal rotations on crop performance and profit margin: Making crop rotations fit for the future. Asp. Appl. Biol. 11(113), 29–36.
Forristal P. D., Murphy K. (2009). “Can we reduce costs and increase profits with Min till?,” in Proceedings of the national tillage conference 2009 (Carlow, Ireland: Teagasc), 48–67.
Fortune T., Kennedy T., Mitchell B., Dunne B., Murphy K., Connery J., et al. (2005). “Reduced cultivations – update from oak park experiments,” in National tillage conference proceedings, vol. 2005. (Oak Park, Carlow, Ireland: Crops Research Centre), 18–34.
Froud-Williams R. J., Chancellor R. J. (1982). A survey of cereal weeds in central southern England. Weed Res. 22, 163–171. doi: 10.1111/j.1365-3180.1982.tb00160.x
Hakansson S. (2003). Weeds and weed management on arable land: An ecological approach (Wallingford: CABI Publishing).
Heanue K., O’Donoghue C. (2014) The economic returns to formal agricultural education. teagasc rural economy and development programme, teagasc. Available at: https://www.teagasc.ie/media/website/publications/2014/Teagasc_Impact_of_Education_Report.pdf (Accessed 29 October 2022).
Heap I. M. (2022) International survey of herbicide resistant weeds. Available at: http://www.weedscience.org (Accessed 10 April 2022).
Herrmann J., Hess M., Strek H., Richter O., Beffa R. (2016). Linkage of the current ALS-resistance status with field history information of multiple fields infested with blackgrass. Jul.-Kuhn-Arch 443, 273–279.
Hicks H. L., Comont D., Coutts S. R., Crook L., Hull R., Norris K., et al. (2018). The factors driving evolved herbicide resistance at a national scale. Nat. Ecol. Evol. 2, 520–36. doi: 10.1038/s41559-018-0470-1
Holt J. S., Welles S. R., Silvera K., Heap I. M., Heredia S. M., Martinez-Berdeja A., et al. (2013). Taxonomic and life history bias in herbicide resistant weeds: Implications for deployment of resistant crops. PloS One 8, e71916. doi: 10.1371/journal.pone.0071916
Hull R., Tatnell L. V., Cook S. K., Beffa R., Moss S. R. (2014). Current status of herbicide-resistance weeds in the UK. Aspects Appl. Biol. 127, 261–271.
Jess S., Kildea S., Moody A., Rennick G., Murchie A., Cooke L. R. (2014). European Union policy on pesticides: implications for agriculture in Ireland. Pest. Manage. Sci. 70, 1646–1654. doi: 10.1002/ps.3801
Johnson W. G., Gibson K. D. (2006). Glyphosate-resistant weeds and resistance management strategies: an Indiana grower perspective. Weed Technol. 20, 768–772. doi: 10.1614/WT-05-122R1.1
Kennedy T., Fortune R. A., Forristal P. D., Connery J. (2013). A comparison of the effects of minimum-till and conventional-till methods, with and without straw incorporation, on slugs, slug damage, earthworms and carabid beetles in autumn-sown cereals. J. Agric. Sci. 151 (5), 605–629. doi: 10.1017/S0021859612000706
Knowler D., Bradshaw B. (2007). Farmers’ adoption of conservation agriculture: a review and synthesis of recent research. Food Policy. 32 (1), 25–48. doi: 10.1016/j.foodpol.2006.01.003
Kuznetsova A., Brockhoff P. B., Christensen R. H. B. (2017). lmerTest package: Tests in linear mixed effects models. J. Stat. Softw 82 (13), 1–26. doi: 10.18637/jss.v082.i13
Lahmar R. (2010). Adoption of conservation tillage in Europe, lessons of the KASSA project. Land Use Policy. 27, 4–10. doi: 10.1016/j.landusepol.2008.02.001
Lewellyn R. S., Lindner R. K., Pannell D. J., Powles S. (2007). Herbicide resistance and the adoption of integrated weed management by Western Australian grain growers. J. Agric. Econ. 36, 123–130. doi: 10.1111/j.1574-0862.2007.00182.x
Lintell Smith G., Freckleton R. P., Firbank L. G., Watkinson A. R. (1999). The population dynamics of Anisantha sterilis in winter wheat: Comparative demography and the role of management. J. Appl. Ecol. 36, 455–471. doi: 10.1046/j.1365-2664.1999.00420.x
Liu R., Singh V., Zhou X. G., Bagavathiannan M. (2020). Stakeholder and field surveys on weed issues and research needs in rice production in Texas. Weed Technol. 35, 242–250. doi: 10.1017/wet.2020.108
Lobley M., Butler A., Reed M. (2009). The contribution of organic farming to rural development: An exploration of the socio-economic linkages or organic and non-organic farms in England. Land Use Policy. 26, 723–735. doi: 10.1016/j.landusepol.2008.09.007
Lüdecke D., Ben-Shachar M. S., Patil I., Waggoner P., Makowski D. (2021). Performance: An r package for assessment, comparison and testing of statistical models. J. Open Source Software 6 (60), 3139. doi: 10.21105/joss.03139
Lutman P. J. W., Moss S. R., Cook S. K., Welham S. J. (2013). A review of the effects of crop agronomy on the management of Alopecurus myosuroides. Weed Res. 53, 299–313. doi: 10.1111/wre.12024
Lynch J. P., Glynn L., Kildea S., Spink J. (2017). Yield and optimum fungicide dose rates for winter wheat (Triticum aestivum l.) varieties with contrasting ratings for resistance to Septoria tritici blotch. Field Crops Res. 204, 89–100. doi: 10.1016/j.fcr.2017.01.012
Mansooji A. M., Holtum J. A., Boutsalis P., Matthews J. M., Powles S. B. (1992). Resistance to aryloxyphenoxypropionate herbicides in two wild oat species (Avena fatua and Avena sterilis ssp. ludoviciana). Weed Sci. 40, 599–605. doi: 10.1017/S0043174500058185
Marshall R., Hanley S. J., Hull R., Moss S. R. (2013). The presence of two different target-site resistance mechanisms in individual plants of Alopecurus myosuroides huds., identified using a quick molecular test for the characterization of six and seven ACCase SNPs. Pest Manage. Sci. 69, 727–737. doi: 10.1002/ps.3429
Met Eireann (2021) The Irish meteorological service. Available at: https://www.met.ie/climate/available-data (Accessed 02 March 2022).
Moss S. R. (2004). Herbicide-resistant weeds in Europe: The wider implications. Commun. Agric. Appl. Biol. 69, 3–11.
Moss S. R. (2005). Managing herbicide-resistant rye-grass: 42nd annual review of weed control Vol. 2005 (Peterborough, UK: British Crop Protection Council (BCPC), 40–47.
Moss S. R. (2017). Black-grass (Alopecurus myosuroides): Why has this weed become such a problem in Western Europe and what are the solutions? Outlooks Pest Manage. 10, 207–212. doi: 10.1564/v28_oct_04
Moss S. R., Perryman S. A. M., Tatnell L. V. (2007). Managing herbicide resistant black-grass (Alopecurus myosuroides): Theory and practice. Weed Techn. 21, 300–309. doi: 10.1614/WT-06-087.1
Moss S. R., Ulber L., Hoed I. D. (2019). A herbicide resistance-risk matrix. Crop Protect. 115, 13–19. doi: 10.1016/j.cropro.2018.09.005
Peel M., Finlayson B. L., McMahon T. A. (2007). Updated world map of the köppen-Geiger climate classification. Hydrol. Earth Syst. Sci. 11, 1633–1644. doi: 10.5194/hess-11-1633-2007
R Core Team (2020). R: A language and environment for statistical computing (Vienna, Austria: R Foundation for Statistical Computing).
Reverte R. (2010). Understanding and combating the threat posed by lolium multiflorum as a weed of arable crops (UK: School of Plant Sciences, University of Reading, Reading).
Riemens M. M., Groeneveld R. M. W., Kropff M. J. J., Lotz L. A. P., Renes R. J., Sukkel W., et al. (2010). Linking farmer weed management behaviour with weed pressure: more than just technology. Weed Sci. 58, 490–496. doi: 10.1614/WS-09-048.1
Russell V., Buerkner P., Herve M., Jung M., Love J., Miguez F., et al. (2022) R core package ‘emmeans’: estimated marginal means, aka least squares means. Available at: https://cran.r-project.org/web/packages/emmeans/emmeans.pdf (Accessed 04 March 2022).
Schulte R. P. O., Melland A., Richards K., Fenton O., Jordan. P. (2010). Modelling soil phosphorus decline; expectations of water framework directive policies in Ireland. Environ. Sci. Policy. 13, 472–484. doi: 10.1016/j.envsci.2010.06.002
Scott L. J. (1997). Regression models for categorical and limited dependent variables (Thousand Oaks, CA: Sage Publications).
Soane B. D., Ball B. C., Arvidsson J., Basch G., Moreno F., Roger-Estrade J. (2012). No-till in northern, western and south-western Europe: a review of problems and opportunities for crop production and the environment. Soil. Till. Res. 118, 66–87. doi: 10.1016/j.still.2011.10.015
Teagasc (2022). Crops costs and returns 2022. In: Teagasc (the state-funded research and farm advisory organization) (Carlow, Ireland). Available at: https://www.teagasc.ie/media/website/crops/crops/Teagasc-Crop-Costs-and-Returns-2022.pdf (Accessed 08 April 2022).
Townsend T. J., Ramsden S. J., Wilson P. (2016). How do we cultivate in England? tillage practices in crop production systems. Soil Use Manage. 32, 106–117. doi: 10.1111/sum.12241
Ulber L., Rissel D. (2018). Farmer’s perspective on herbicide-resistant weeds and application of resistance management strategies: results from a German survey. Pest Manage. Sci. 74, 2335–2345. doi: 10.1002/ps.4793
Van Acker R. C. (2009). Weed biology serves practical weed management. Weed Res. 49, 1–5. doi: 10.1111/j.1365-3180.2008.00656.x
Van Groenigen K. J., Hastings A., Forristal P. D., Roth B., Jones M., Smith P. (2011). Soil c storage as affected by tillage and straw management: An assessment using field measurements and model predictions. Agric. Ecosyst. Environ. 140, 218–225. doi: 10.1016/j.agee.2010.12.008
Weber E., Gut D. (2005). A survey of weeds that are increasingly spreading in Europe. Agron. Sustain. Dev. 25 (1), 109–121. doi: 10.1051/agro:2004061
Yu Q., Powles S. (2014). Metabolism-based herbicide resistance and cross-resistance in crop weeds: a threat to herbicide sustainability and global crop production. Plant Physiol. 166, 1106–1118. doi: 10.1104/pp.114.242750
Keywords: grass-weed challenges, Ireland, survey, awareness, herbicide resistance, crop establishment systems, integrated grass-weed control methods (IWM)
Citation: Vijayarajan VBA, Fealy RM, Cook SK, Onkokesung N, Barth S, Hennessy M and Forristal PD (2022) Grass-weed challenges, herbicide resistance status and weed control practices across crop establishment systems in Ireland’s mild Atlantic climate. Front. Agron. 4:1063773. doi: 10.3389/fagro.2022.1063773
Received: 07 October 2022; Accepted: 04 November 2022;
Published: 21 November 2022.
Edited by:
Mithila Jugulam, Kansas State University, United StatesReviewed by:
Ricardo Alcántara-de la Cruz, Federal University of São Carlos, BrazilSimerjee Kaur, Punjab Agricultural University, India
Copyright © 2022 Vijayarajan, Fealy, Cook, Onkokesung, Barth, Hennessy and Forristal. This is an open-access article distributed under the terms of the Creative Commons Attribution License (CC BY). The use, distribution or reproduction in other forums is permitted, provided the original author(s) and the copyright owner(s) are credited and that the original publication in this journal is cited, in accordance with accepted academic practice. No use, distribution or reproduction is permitted which does not comply with these terms.
*Correspondence: Vijaya Bhaskar Alwarnaidu Vijayarajan, VmlqYXlhLkJoYXNrYXJAdGVhZ2FzYy5pZQ==