- 1Department of Ecology, Swedish University of Agricultural Sciences, Uppsala, Sweden
- 2Department of Plant Protection Biology, Swedish University of Agricultural Sciences, Alnarp, Sweden
- 3Department of Biosystems and Technology, Swedish University of Agricultural Sciences, Alnarp, Sweden
- 4Department of Forest Mycology and Plant Pathology, Swedish University of Agricultural Sciences, Uppsala, Sweden
- 5Swedish Rural Economy and Agricultural Society, Örebro, Sweden
- 6Department of Crop Production Ecology, Swedish University of Agricultural Sciences, Uppsala, Sweden
Oilseed rape (OSR; Brassica napus) is a globally important crop which is increasingly under pressure from pests, pathogens and weeds. We investigated the potential of achieving multifunctional crop protection benefits by intercropping oilseed rape with legumes. A field experiment was conducted in which winter oilseed rape was intercropped with the annual frost sensitive legumes berseem clover (Trifolium alexandrinum) or spring faba bean (Vicia faba), or with the winter grain legumes winter faba bean or winter peas (Pisum sativum). We tracked damage to winter oilseed rape by autumn and spring pests (slugs and insects), pathogens, weed biomass, as well as oilseed rape and intercrop yield in each treatment. Intercropping treatments resulted in pest damage that was equivalent or lower than in oilseed rape alone. Follow up field and lab assessments for the frost sensitive legume intercrops provided evidence for a reduction in autumn pest damage to OSR. Each legume intercrop had its own benefits and drawbacks in relation to pest, pathogen and weed suppression, suggesting that the plant species selected for intercropping with oilseed rape should be based on the pests, pathogens and weeds of greatest concern locally to achieve relevant multifunctional benefits. Our study provides a framework for further experiments in which the multifunctional effects of intercropping on pests, pathogens and weeds can be quantified.
Introduction
Oilseed rape (Brassica napus L., OSR) is a globally important crop, both for the production of high-value vegetable oil and protein-rich animal feed (as exhausted rapeseed meal, a by-product from oil extraction), and as a break crop in cereal-dominated cropping systems (Angus et al., 2015). A range of pests, pathogens and weeds constrain OSR production, resulting in intensive pesticide use in conventional farming systems and yield instability in organic systems (Valantin-Morison et al., 2007; Williams, 2010; Lutman, 2018; Zheng et al., 2020). Crop protection practices face challenges from stricter pesticide regulations and increased pesticide resistance (Duke, 2012; Brandes and Heimbach, 2018; Arthey, 2020; Zheng et al., 2020). New ways to reach profitable OSR yield levels by using more sustainable crop protection methods are urgently needed.
Diversification of OSR production by increasing the crop and non-crop plant diversity on the local (field) and landscape scale holds promise for the development of more sustainable crop protection strategies (Isbell et al., 2017). We focus on the potential of intercropping (growing a mixture of two or more species), as it may offer mechanisms for simultaneous control of pests, pathogens and weeds (Malézieux et al., 2009). Legumes are of special interest for intercropping with OSR since their capacity for symbiotic nitrogen fixation increases overall nitrogen-use efficiency and minimizes competition for soil and fertilizer-derived nitrogen (Verret et al., 2017; Dowling et al., 2021). Intercropping OSR with legumes is relatively understudied (but see Dowling et al., 2021), especially when it comes to the implications for crop protection. In addition, intercropping studies rarely evaluate crop protection strategies against multiple crop antagonists simultaneously.
Seminal intercropping studies, like Root (1973), have been conducted on vegetable Brassicaceae crops closely related to OSR (e.g., cabbage, collards, broccoli). Intercropping can reduce insect pest infestation and crop damage by disrupting visual or olfactory host plant location, reducing host plant quality or increasing natural enemy activity (Root, 1973; Trenbath, 1993; Hooks and Johnson, 2003; Finch and Collier, 2012). In the few intercropping studies exploring insect pest responses in OSR, legume intercrops reduced damage from cabbage stem weevil (Ceutorhynchus pallidactylus) (Paulsen et al., 2006) and rape winter stem weevil (C. picitarsis) (Cadoux et al., 2015), but not flea beetle (Phyllotreta cruciferae) (Weiss et al., 1994). Intercropping OSR with red clover (Trifolium pratense) did not affect the population of pollen beetles (Brassicogethes aeneus) the same season, but decreased the number of emerging pollen beetles in the next generation (Hokkanen, 2004).
There is to our knowledge no published research on the effects of intercropping on slugs in OSR. Research on the effects of crop residue on slug abundance and crop damage has been inconsistent and mostly done in tillage experiments (Voss et al., 1998; Glen and Symondson, 2003; Douglas and Tooker, 2012; Howlett, 2012; Rowen et al., 2020). A microclimatic effect from the intercrop similar to the effect of crop residue that is beneficial for slugs has been assumed (Gaba et al., 2015), yet studies have found a reduction in slug damage for the main crop in intercropped systems (Glen, 2000; Brooks et al., 2005; Choi et al., 2006). The mechanisms for damage reduction are not well-understood, but dilution effects, based on the generalist feeding requirements of slugs, antifeedant effects and benefits for slug predators have been documented (Cook et al., 1997; Dodds et al., 1999; Le Gall and Tooker, 2017).
Intercropping has been shown to reduce some plant diseases, especially foliar diseases (Boudreau, 2013). Several mechanisms may be involved, such as resource dilution, disruption of spatial or temporal disease cycles, changed microclimate, allelopathic effects, effects on the host morphology or physiology, or changes in the suppressiveness of plant or soil-associated microorganisms (Ratnadass et al., 2012; Boudreau, 2013). Despite having a denser canopy resulting in a microclimate favorable for foliar disease development, intercropping OSR and peas produced generally healthy crops (Soetedjo et al., 2003; VanKoughnet, 2016; Dowling et al., 2021). The effects of intercropping on disease susceptibility and mechanisms for resistance, however, are still relatively understudied in legume-OSR intercrops.
Growing crop species mixtures has been shown to result in lower weed density and weed biomass compared to sole crops (Liebman and Dyck, 1993). Weed suppression is particularly effective in grain legume and cereal intercrops, in which weed biomass is reduced compared to grain legume sole crops (Bedoussac et al., 2015). Weed suppression in grain legume-OSR intercrops has been less studied. Research on mixtures of winter OSR and legume service crops where the legume is sown simultaneously with oilseed rape and killed by frost during winter, has shown promising effects on weed suppression during autumn (Cadoux et al., 2015; Lorin et al., 2015). The suggested mechanism is that the frost-sensitive legumes increase the competition for light by occupying surfaces that are not covered by the small winter OSR plants during autumn (Cadoux et al., 2015).
In summary, intercropping OSR with legumes has the potential to make crop stands more competitive against weeds and reduce pathogen, insect pest and slug damage through physical, visual and olfactory disruption, habitat alteration and promotion of biological control. The empirical evidence base for such varied intercropping benefits for OSR is limited with few attempts to simultaneously measure crop protection against weeds, pests and pathogens using a multifunctional approach. Our aims were to assess the multifunctional crop protection implications of intercropping OSR with both frost-sensitive and overwintering legumes.
Materials and Methods
Experimental Design
To explore the crop protection implications of intercropping OSR we established a field experiment at the SITES (Swedish Infrastructure for Terrestrial Ecosystem Science) Lönnstorp field research station in southern Sweden in 2019 (55.668 N, 13.101 E). We used a randomized complete block design with four replicates of each treatment, and a 12 m by 6 m plot size with 0.5 m between plots and 12 m between blocks. The experiment had five winter OSR treatments, winter OSR sole crop (OSR) and winter OSR intercropped with each of the following legumes: berseem clover (Trifolium alexandrinum L., OSR + Clover), spring faba bean (Vicia faba L. var. minor, OSR + Sfaba), winter faba bean (Vicia faba L. var. minor, OSR + Wfaba) or winter peas (Pisum sativum L., OSR + Wpeas). Sole treatments of winter faba bean (Wfaba) and winter peas (Wpeas) were also included. We used a system approach, where sowing dates, mechanical weed control and N fertilization were adapted to the specific crop and intercrop species. Berseem clover and spring faba bean were selected to assess the potential for reducing autumnal pest damage and were not harvested since they are frost sensitive. Winter faba bean and winter peas were selected for their potential effects on spring pests and additional grain yield. Pests were only assessed in the five treatments with OSR since OSR pests were the focus. Pathogens of concern were assessed in treatments with OSR (Sclerotinia sclerotiorum causing stem rot) and opportunistically also in faba bean following detection of Uromyces fabae, which causes faba bean rust. Weeds and yield were assessed across all treatments.
The field was plowed (20–25 cm) after the harvest of spring barley in August 2019 and harrowed (5 cm) before sowing the experiment. Winter OSR seeds were treated with the fungicides thiram and dimethomorph; all legume seeds were untreated. Winter OSR was sown with a 50 cm row distance on August 29, 2019 in all OSR treatments (cv. Mentor, 2.8 kg/ha, corresponding to approximately 50 seeds/m2). Berseem clover (cv. Tigri, 20 kg/ha, 600 seeds/m2) was sown with a broadcast seeder (broadcast due to the small seeds and high target plant density to achieve satisfactory ground cover) just before sowing the winter OSR, on the same day August 29 (OSR + Clover). Spring faba bean (cv. Boxer, 110 kg/ha, 20 seeds/m2) was sown with a 50 cm row distance in between OSR rows on September 9 (OSR + Sfaba) directly after inter row hoeing against weeds. This weed control was performed in all OSR plots except in OSR + Clover. Winter faba bean (cv. Hiverna, 130 kg/ha, 25 seeds/m2) and winter peas (cv. EFB33, 120 kg/ha, 100 seeds/m2) were sown with a 50 cm row distance, in between OSR rows on October 7 (OSR + Wfaba, OSR + Wpeas), after a second hoeing treatment between the OSR rows in these plots and in the OSR sole crop plots. Sole crops of winter faba bean (Wfaba) and winter peas (Wpeas) were sown after harrowing the plots on October 7 at 200 kg seeds/ha (about 40 seeds/m2 for Wfaba and 165 seeds/m2 for Wpeas) with a row distance of 12 cm. Inter row hoeing was also performed on March 20 in the OSR sole crop and OSR + Clover and OSR + Sfaba treatments. Berseem clover and spring faba bean plants are frost sensitive and were grown as autumn service-crops, but due to a mild winter hoeing was performed in lieu of frost killing.
The fertilizer rates were chosen based on regional recommendations for conventional OSR cultivation. The N fertilizer rates in intercrops were reduced to test if the input of biologically fixed N in clover and spring faba bean, made available in spring when these legumes had been killed, could provide a N fertilizer saving effect, and to reduce OSR competitiveness against the intercropped overwintering legumes (Wfaba and Wpeas). The OSR sole crop received in total 170 kg N/ha, divided in one application of 40 kg N/ha (37 kg as , 3 kg as ) on September 19 and 130 kg N/ha (65 kg each as and ) on March 17. All plots where OSR was intercropped with legumes received in total 128 kg N/ha, with 30 kg N/ha (28 kg as , 2 kg as ) on September 19 and 98 kg N/ha (49 kg each as and ) on March 17. The entire experiment was fertilized with 20 kg P/ha, 50 kg K/ha, 12 kg Mg/ha and 36 kg S/ha on March 19. No pesticides were used in the experiment.
Assessments
Autumn OSR Pests: Damage and Preference of Cabbage Stem Flea Beetle (CSFB) and Slugs
In autumn the main pests of OSR seedlings in Northern Europe are cabbage stem flea beetle (Psylliodes chrysocephala, CSFB) and slugs (mostly the gray field slug Deroceras reticulatum). In each treatment 60 OSR plants were assessed for slug and CSFB damage (15 plants per plot, assessed plants were at least 1 m from any plot edge). On each plant, both slug and CSFB damage were separately scored (Williams, 2010; Douglas and Tooker, 2012) from 0–10 corresponding to 0–100% damage, 100% damage indicates no remaining leaves. On September 26, 2019 at crop stage BBCH 12–13 (Biologische Bundesanstalt, Bundessortenamt und Chemical Industry, Lancashire et al., 1991), slug and CSFB damage were quantified in OSR, OSR + Clover and OSR + Sfaba as these treatments are most likely to impact early season pests. Slug and CSFB damage was not assessed in OSR + Wpeas and OSR + Wfaba in September as these intercrops are sown later in autumn after most slug and CSFB damage has occurred. On November 4, 2019, at crop stage BBCH 15 slug and CSFB damage assessments were conducted in all five OSR treatments. Follow-up assessments in OSR, OSR + Clover and OSR + Sfaba were conducted both in the field and the lab to assess intercropping effects on OSR preference for CSFB and slugs, respectively. On March 2, 2020 (BBCH 30–32) CSFB larva were counted in OSR, OSR + Clover and OSR + Sfaba. Forty randomly selected plants per treatment were collected (10 plants per plot, assessed plants were at least 1 m from any plot edge). The plants were dissected in the laboratory and the number of CSFB larvae per OSR plant were counted (Walters et al., 2001). To assess the effect of intercropping on slug preference in a controlled environment, 15 cages per treatment with five OSR plants per cage were used (BBCH 12–13). The intercrop treatments included the addition of five spring faba (OSR + Sfaba) or berseem clover (OSR + Clover) seedlings. Adult slug specimens of D. reticulatum were collected around Uppsala, Sweden in the autumn of 2019. Slugs were starved for 24 h prior to the start of the experiment. One slug was placed in each of the 45 cages. On each OSR plant slug damage was separately scored after seven days from 0–10 corresponding to 0–100% damage.
Spring OSR Pests: Damage From Pollen Beetle (PB) and Brassica pod Midge (BPM)
Damage from pollen beetles (PB, Brassicogethes spp., primarily Brassicogethes aeneus) and brassica pod midge (BPM, Dasineura brassicae) were considered the spring pests of greatest concern. Damage to OSR seed pods was assessed on June 23, 2020 at approximate crop stage BBCH 80, when most seed pods were still somewhat green, which facilitated identification of galls from BPM. In each plot, 10 random OSR plants were selected across the length of the plot. On each plant, the number of pods and empty, podless stalks were counted on the top shoot and the two uppermost side shoots. Number of flower buds destroyed by PB was considered equal to the number of podless stalks (Seimandi-Corda et al., 2021), and damage was estimated as percentage of podless stalks. Number of pods on each shoot damaged by BPM was identified through characteristic yellowish, swollen galls (Zaller et al., 2008; Williams, 2010). In uncertain cases, pods were opened to check for the presence of larvae. Some cases of BPM damage, with already evacuated pods, were identified through characteristic cracking and splitting of the pod (Zaller et al., 2008; Williams, 2010). Damage by BPM was estimated as the percentage of damaged pods, not counting empty stalks previously damaged by PB.
Pathogens
Disease incidence of Sclerotinia stem rot (S. sclerotiorum) in each plot of the field experiment was determined at BBCH 80 (July 2, 2020) on 25 randomly chosen OSR plants, which were classified as healthy or infected based on a visual assessment of the presence of characteristic bleached, grayish lesions (Khangura and Beard, 2020). An assessment of faba bean rust (Uromyces faba) were conducted in treatments with winter faba bean: sole winter faba bean (Wfaba) and OSR + Wfaba. The top 10 leaves were collected from faba bean plants on July 10, 2020 and frozen until assessment. Leaves were assessed and classed based on the percentage of infected leaf area as follows: 0 = no visible symptoms, 1 = < 1%; 2 = 1–5%; 3 = 6–10%; 4 = 11–25%; 5 = 26–50%; 6 = > 50%; 7 = wilted. A disease severity index (DSI) was then calculated using the equation:
in which the DSI is the sum of the median value of all leaves with each infection rating over the total number of leaves assessed (n). A DSI of 0 means all assessed leaves are healthy and a DSI of 100 means all leaves are wilted (Stoltz et al., 2018).
Weeds
In November 2019 a visual assessment of the relative ground cover of weed monocots and dicots was performed in four 40 x 50 cm quadrats in each experimental plot. Weed aboveground biomass was sampled May 19–22, 2020, when the winter OSR crop was in between full flowering and end of flowering stages (BBCH 65–69) and before most weeds had set seed. In each plot, weed and crop biomass was collected by cutting all plants at the soil surface in four 0.25 m2 quadrats per plot. The quadrats were placed in each corner of the plot including one OSR row and the entire adjacent inter-row space. The quadrat was at least 1 m from the edges while avoiding the central 2 x 12 m part of the plot. The collected plants were sorted into: OSR; the sown legume species (if any); and weeds. All plants per sorted fraction were pooled from the four quadrats, dried at 80°C for at least 24 h (until stable weight) and weighed. Total weed biomass, OSR and legume biomass (dry weight per area unit in all cases) were recorded.
Intercrop and OSR Yield
The experiment was harvested on August 4, 2020 when OSR, winter faba bean and winter peas were mature. Total grain yield was measured by an experimental combine harvester, which collected all harvestable grains from a previously undisturbed central 2 m-wide strip in each plot (24 m2). A sub-sample of the harvested grains from each plot (~1 kg) was dried at 80 °C for at least 24 h (until stable weight) before manual sorting of OSR, winter faba bean and winter pea seeds. Grain and legume yields (kg/ha, dry weight) were calculated based on proportional subsample weight. Land equivalent ratio (LER) was calculated for OSR + Wfaba and OSR + Wpeas, since a monocrop reference yield was available for all crops, using the following equation:
in which the LER is equal to the sum of the ratio of the intercropped yield (IY) over the sole crop yield (SY) for both species being harvested.
Statistical Analyses
Differences in percent damage from slugs, CSFB, PB and BPM, and abundance of CSFB larva in spring were analyzed with treatment as a fixed effect and plot within block as a nested random effect using linear mixed models. For slug damage to OSR seedlings in the cage trials treatment was a fixed effect and cage was a random effect. All pest percent damage data was arcsine square root transformed to increase normality. A Poisson distribution was used for the CSFB larval count data. Sclerotinia stem rot was not detected in any treatment so no statistical analyses were performed. Differences in the disease severity index of faba bean rust were calculated using treatment as a fixed effect and block as a random effect. One sample per treatment plot (four samples per treatment) was collected for weed, OSR and legume biomass and OSR grain yield and overwintering legume yield. These response variables were analyzed using linear mixed models with treatment as a fixed effect and block as a random effect. Tukeys HSD was used to delineate significant differences between treatments in all models. Statistical analyses were performed in R version 4.0.3 (R Development Core Team, 2020) with the lme4 and multcomp packages (Hothorn et al., 2008; Bates et al., 2015).
Results
Slug damage to OSR plants in autumn 2019 did not differ between treatments in the September or November assessments, though the cage trial showed lower slug damage to OSR in the OSR + Sfaba treatment (Table 1). CSFB damage was significantly lower in OSR + Clover compared to other treatments in both September and November; CSFB larval abundance was significantly lower in both OSR + Clover and OSR + Sfaba compared to OSR alone (Table 1). In June 2020, damage from PB did not differ between treatments. Damage levels from BPM were low overall, but they were higher in OSR + Clover compared to OSR + Wpeas (Table 1). No symptoms of Sclerotinia root rot were detected in any OSR treatment. The assessment of faba bean rust (U. fabae) showed a significantly lower disease severity index on the leaves of winter faba bean in the OSR + Wfaba intercropped plots (17.86 ± 3.57) compared to the Wfaba sole crop (46.43 ± 2.70, F = 40.7, df = (1, 6), p <0.001). The November 2019 weed assessment showed dicots were predominant while monocots, including cereals, did not exceed 5% of the total weed cover. Weed biomass from May 2020 was lowest in Wfaba and Wpeas and highest in OSR + Wfaba (Table 2). OSR biomass was similar across treatments while legume biomass was highest in the legume sole crops (Table 2). Grain OSR yield was lower in OSR + Wpeas compared to other treatments and winter peas gave a higher legume yield than winter faba beans when intercropped with OSR (Table 2). The land equivalent ratio was 1.13 for OSR + Wfaba. No LER was calculated for OSR + Wpeas since the winter peas sole crop was severely lodged and minimal harvest was possible only in one block. The multifunctionality of intercrops is shown relative to the OSR sole crop in Figure 1.
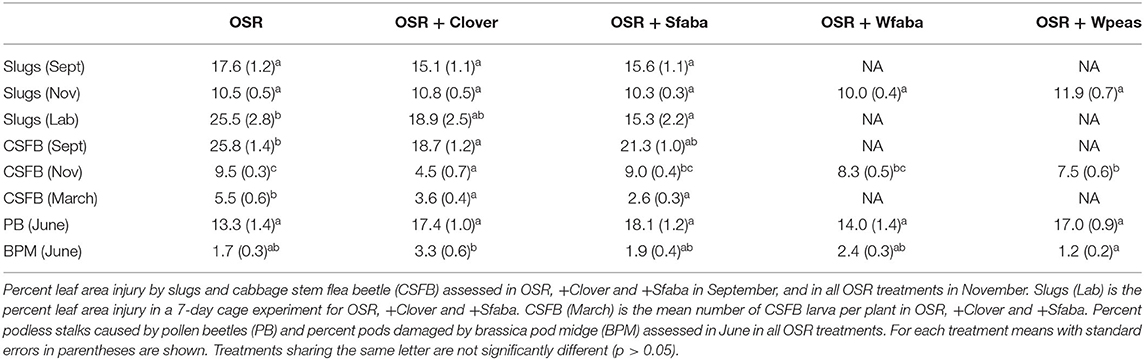
Table 1. Pest damage to oilseed rape (OSR) in winter OSR sole crop or winter OSR intercropped with berseem clover (+Clover), spring faba bean (+Sfaba), winter faba bean (+Wfaba) or winter peas (+Wpeas).

Table 2. Weed biomass, OSR biomass and legume biomass from May sampling (g/m2, dry weight) in each of the seven treatments: winter oilseed rape (OSR) sole crop or winter OSR intercropped with berseem clover (+Clover), spring faba bean (+Sfaba), winter faba bean (+Wfaba) or winter peas (+Wpeas) and winter faba sole crop (Wfaba) and winter peas sole crop (Wpeas), and August grain yield from winter OSR and the winter legume intercrops and sole crops (kg/ha, dry weight).
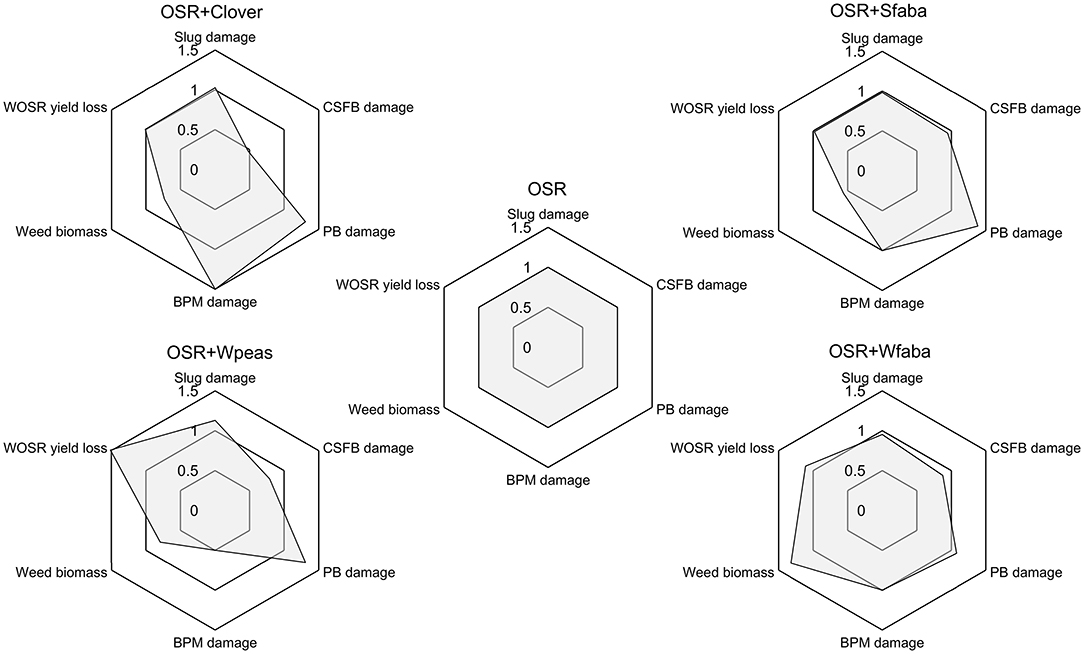
Figure 1. Radar plots displaying percent leaf area injury to winter OSR seedlings by slugs and cabbage stem flea beetle (CSFB), percent podless stalks caused by pollen beetle (PB), percent pods damaged by brassica pod midge (BPM), weed biomass and OSR yield in each of the five treatments that included OSR: winter oilseed rape (OSR) sole crop or winter OSR intercropped with berseem clover (+Clover), spring faba bean (+Sfaba), winter faba bean (+Wfaba) or winter peas (+Wpeas). Variables were standardized by dividing all numbers by the value from the OSR sole crop. The scale for OSR yield was reversed into yield loss, so that a lower value is more favorable for all response variables.
Discussion
Our field experiment and lab study supported the idea that intercropping OSR with legumes can improve crop protection in several cases and present a framework for multifunctional metrics to assess intercropping systems.
In the field experiment, slug damage in both September and November was low and similar in all treatments perhaps indicating the drawback of plot experiments in being able to evaluate the impacts of intercropping for mobile organisms. In contrast, the supplementary feeding experiment with cages showed reduced slug damage to OSR plants when intercropped with spring faba bean. Larger field studies are an important next step, but these findings indicate that intercropping does not increase slug damage to OSR plants and the cage study illustrates the potential for intercropping to reduce slug damage to OSR. Further experimentation is needed to explore any mechanisms involved in the potential of intercropping to reduce slug herbivory.
Cabbage stem flea beetle feeding damage to OSR seedlings was reduced in both September and November when intercropped with berseem clover and in OSR + Wpeas for November damage. CSFB larval abundances were reduced by both berseem clover and spring faba bean intercropping, as compared to the OSR sole crop indicating an effect on oviposition which was measurable despite the small plot size. These results are in line with Swiss field experiments where intercropping of OSR with mixtures that included berseem clover reduced adult flea beetle damage to OSR seedlings, though they found no effects on larval abundances (Breitenmoser et al., 2020). Our results suggest that annual legumes planted early in the season decrease oviposition by the early-season pest CSFB. The earlier sowing date, higher biomass and ground coverage of these legumes in autumn might increase their visual and olfactory interference with host plant location by CSFB. The late-planted winter grain legume intercrops (OSR + Wfaba and OSR + Wpeas) are, on the other hand, not present in the field early in the season when most damage from CSFB and slugs occurs.
Pollen beetle damage did not differ between treatments, while intercropping OSR with berseem clover increased the percentage of pods damaged by brassica pod midge, compared to when OSR was intercropped with winter peas. The level of BPM damage was low across treatments. Brassica pod midge damage occurs in late spring when most of the berseem clover intercrop is no longer present in the field though intercropping species-driven variation in OSR nutritional content, which affects insect herbivory in OSR, could also play a role (Veromann et al., 2013; Blake et al., 2014; Skellern and Cook, 2018).
Effects of intercropping on pathogen control for OSR could not be evaluated due to an absence of Sclerotinia stem rot in the experiment. The reduction of faba bean rust in intercropping is in line with a previous study on intercropping maize and faba bean, where the severity of chocolate spot disease (Botrytis fabae) was reduced compared to the faba bean sole crop (Stoltz et al., 2018).
Weeds were more successfully controlled in OSR when intercropped with spring faba bean or clover compared to OSR intercropped with winter faba bean (Table 2). This is in line with earlier findings and could be explained by the ability of spring faba bean to quickly produce biomass that competed with weeds (Lorin et al., 2015; Verret et al., 2017). Intercropping with frost sensitive legumes also facilitated mechanical weed control in spring, which was not possible for grain legumes sown in between OSR rows in late autumn and kept for harvest.
Nitrogen fertilizer use was reduced by 25% in the intercropping treatments without any OSR yield penalty, except when OSR was intercropped with winter peas. OSR yield was reduced by ~50% when intercropped with winter peas, which could be linked to the high biomass of the winter peas that were maintained until OSR harvest, but this treatment also resulted in a pea grain yield that was higher than the OSR yield and the most consistently stable pest control across the species measured in this study (Figure 1). The relatively low OSR yield in all treatments in this experiment, relative to the southern Swedish (Skåne) 2016–2020 average of 3,384 kg/ha in conventional production (Swedish Board of Agriculture., 2021), is attributed to the low N fertilization and lack of pesticide inputs.
Technical limitations when it comes to managing, harvesting and separating mixed crop species constitute an important barrier to the uptake of OSR intercropping (Dowling et al., 2021). This makes intercropping of OSR with service-crops that are not harvested, such as spring faba bean or berseem clover sown in autumn, of interest, as they can provide intercropping benefits without the need for harvesting and processing mixed crops. Intercropping with a grain legume intended for harvest also provides benefits with potential increased legume yield. This was the case with winter peas, where the OSR provided physical support until maturity in the OSR + Wpeas intercrop, facilitating pea grain harvest. Our experiment shows that intercrops not maintained for the full crop cycle of oilseed rape are less likely to provide crop protection benefits against pests that attack the crop at late growth stages, such as brassica pod midge, though they might be effective against early season pests like cabbage stem flea beetle thus improving overwintering capacity.
There are several potential benefits of intercropping OSR with legumes, some of which we could confirm in the field experiment. The concerns that intercropping would facilitate increased slug and pathogen problems due to changes in the microclimate were not supported in this study. Each legume intercrop, however, had its own benefits and drawbacks. Our results suggest that the choice of intercrop will depend on what aspects of pest, pathogen and weed control are rated as most important. This pilot study provides the foundation for intercropping studies on the field scale, which would overcome the limitation of using small plots to evaluate effects on mobile organisms like insects. Multi-year assessments would better capture the long-term consistency of multifunctional crop protection provided by different OSR and legume intercropping mixes. Due to the necessity of cropping systems to provide control against multiple crop antagonists, we propose a multifunctional perspective to be adopted more often to quantify how intercropping facilitates or creates resilience against pests, pathogens and weeds.
Data Availability Statement
The datasets generated for this study can be found in the Swedish National Data Service at https://doi.org/10.5878/ppwm-4826.
Author Contributions
SE, PA, GC, HF, ML, A-CW, and OL conceived the ideas and designed methodology. SE, GC, ML, PA, A-CW, and OL collected data. SE analyzed the data and led the writing of the manuscript. All authors contributed critically to the drafts and gave final approval for publication.
Funding
Funding was provided by Platform Plant Protection at the Swedish University of Agricultural Sciences and Swedish farmers' foundation for agricultural research, Grant O-18-20-165.
Conflict of Interest
The authors declare that the research was conducted in the absence of any commercial or financial relationships that could be construed as a potential conflict of interest.
Publisher's Note
All claims expressed in this article are solely those of the authors and do not necessarily represent those of their affiliated organizations, or those of the publisher, the editors and the reviewers. Any product that may be evaluated in this article, or claim that may be made by its manufacturer, is not guaranteed or endorsed by the publisher.
Acknowledgments
We thank Erik Rasmusson and his colleagues at the SITES Lönnstorp research station for managing the field experiment.
References
Angus, J. F., Kirkegaard, J. A., Hunt, J. R., Ryan, M. H., Ohlander, L., and Peoples, M. B. (2015). Break crops and rotations for wheat. Crop Pasture Sci. 66, 523–552. doi: 10.1071/CP14252
Arthey, T. (2020). Challenges and Perspectives in Global Rapeseed Production. Availabe online at: http://www.agribenchmark.org/fileadmin/Dateiablage/B-Cash-Crop/Reports/RapseedReport-2020-complete.pdf (accessed October 28, 2021).
Bates, D. M., Machler, M., Bolker, B., and Walker, S. (2015). Fitting linear mixed-effects models using lme4. J. Stat. Softw. 67, 1–51. doi: 10.18637/jss.v067.i01
Bedoussac, L., Journet, E. P., Hauggaard-Nielsen, H., Naudin, C., Corre-Hellou, G., Jensen, E. S., et al. (2015). Ecological principles underlying the increase of productivity achieved by cereal-grain legume intercrops in organic farming. A review. Agron. Sustain. Dev. 35, 911–935. doi: 10.1007/s13593-014-0277-7
Blake, A. J., Dosdall, L. M., and Tansey, J. A. (2014). Nutritional effects on the appearance of canola and its attractiveness to the cabbage seedpod weevil. J. Insect Behav. 27, 759–775. doi: 10.1007/s10905-014-9466-0
Boudreau, M. A. (2013). Diseases in intercropping systems. Annu. Rev. Phytopathol. 51, 499–519. doi: 10.1146/annurev-phyto-082712-102246
Brandes, M., and Heimbach, U. (2018). Pyrethroid resistance of insect pests of oilseed rape in Germany. IOBC WPRS Bull. 136, 69–72.
Breitenmoser, S., Steinger, T., Hiltpold, I., Grosjean, Y., Nussbaum, V., Bussereau, F., et al. (2020). Effet des plantes associées au colza d'hiver sur les dégâts d'altises. Recherche Agronomique Suisse 11, 16–25. doi: 10.34776/afs11-16
Brooks, A. S., Wilcox, A., Cook, R. T., and Crook, M. J. (2005). A laboratory-based comparison of a molluscicide and an alternative food source (red clover) as means of reducing slug damage to winter wheat. Pest Manag. Sci. 61, 715–720. doi: 10.1002/ps.1056
Cadoux, S., Sauzet, G., Valantin-Morison, M., Pontet, C., Champolivier, L., Robert, C., et al. (2015). Intercropping frost-sensitive legume crops with winter oilseed rape reduces weed competition, insect damage and improves nitrogen use efficiency. Oilseeds Fats Crops Lipids 22:D302. doi: 10.1051/ocl/2015014
Choi, Y. H., Bohan, D. A., Potting, R. P. J., Semenov, M. A., and Glen, D. M. (2006). Individual based model of slug population and spatial dynamics. Ecol. Model. 190, 336–350. doi: 10.1016/j.ecolmodel.2005.04.019
Cook, R. T., Bailey, S. E. R., and McCrohan, C. R. (1997). The potential for common weeds to reduce slug damange to winter wheat : laboratory and fields studies. J. Appl. Ecol. 34, 79–87. doi: 10.2307/2404849
Dodds, C. J., Henderson, I. F., Watson, P., and Leake, L. D. (1999). Action of extracts of Apiaceae on feeding behavior and neurophysiology of the field slug Deroceras reticulatum. J. Chem. Ecol. 25, 2127–2145. doi: 10.1023/A:1021045024896
Douglas, M. R., and Tooker, J. F. (2012). Slug (Mollusca: Agriolimacidae, Arionidae) ecology and management in no-till field crops, with an emphasis on the mid-Atlantic region. J. Integr. Pest Manag. 3, C1–C9. doi: 10.1603/IPM11023
Dowling, A., Sadras, V. O., Roberts, P., Doolette, A., Zhou, Y., and Denton, M. D. (2021). Legume-oilseed intercropping in mechanised broadacre agriculture – a review. Field Crops Res. 260:107980. doi: 10.1016/j.fcr.2020.107980
Duke, S. O. (2012). Why have no new herbicide modes of action appeared in recent years? Pest Manag. Sci. 68, 505–512. doi: 10.1002/ps.2333
Finch, S., and Collier, R. H. (2012). The influence of host and non-host companion plants on the behaviour of pest insects in field crops. Entomol. Exp. et Applicata 142, 87–96. doi: 10.1111/j.1570-7458.2011.01191.x
Gaba, S., Lescourret, F., Boudsocq, S., Enjalbert, J., Hinsinger, P., Journet, E. P., et al. (2015). Multiple cropping systems as drivers for providing multiple ecosystem services: from concepts to design. Agron. Sustain. Dev. 35, 607–623. doi: 10.1007/s13593-014-0272-z
Glen, D. M. (2000). The effects of cultural measures on cereal pests and their role in integrated pest management. Integr. Pest Manag. Rev. 5, 25–40. doi: 10.1023/A:1009609504464
Glen, D. M., and Symondson, W. O. C. (2003). “Influence of soil tillage on slugs and their natural enemies,” in Soil tillage in agroecosystems, ed Eli Titi, A, . (Boca Raton, FL: CRC Press LLC), 207–227. doi: 10.1201./9781420040609.ch8
Hokkanen, H. M. T. (2004). Impact of predators on pollen beetle Meligethes aeneus on rapeseed in Finland. Int. Organ. Biol. Control Bull. 27, 293–296.
Hooks, C. R. R., and Johnson, M. W. (2003). Impact of agricultural diversification on the insect community of cruciferous crops. Crop Prot. 22, 223–238. doi: 10.1016/S0261-2194(02)00172-2
Hothorn, T., Bretz, F., and Westfall, P. (2008). Simultaneous inference in general parametric models. Biom. J. 50, 346–363. doi: 10.1002/bimj.200810425
Howlett, S. A. (2012). Terrestrial slug problems: classical biological control and beyond. CAB Rev. 7, 1–10. doi: 10.1079/PAVSNNR20127051
Isbell, F., Adler, P. R., Eisenhauer, N., Fornara, D., Kimmel, K., Kremen, C., et al. (2017). Benefits of increasing plant diversity in sustainable agroecosystems. J. Ecol. 105, 871–879. doi: 10.1111/1365-2745.12789
Khangura, R., and Beard, C. (2020). Managing Sclerotinia Stem Rot in Canola. Available online at: https://www.agric.wa.gov.au/canola/managing-sclerotinia-stem-rot-canola (accessed October 28, 2021).
Lancashire, P. D., Bleiholder, H., Vandenboom, T., Langeluddeke, P., Stauss, R., Weber, E., et al. (1991). A uniform decimal code for growth-stages of crops and weeds. Ann. Appl. Biol. 119, 561–601. doi: 10.1111/j.1744-7348.1991.tb04895.x
Le Gall, M., and Tooker, J. F. (2017). Developing ecologically based pest management programs for terrestrial molluscs in field and forage crops. J. Pest Sci. 90, 825–838. doi: 10.1007/s10340-017-0858-8
Liebman, M., and Dyck, E. (1993). Crop rotation and intercropping strategies for weed management. Ecol. Appl. 3, 92–122. doi: 10.2307/1941795
Lorin, M., Jeuffroy, M. H., Butier, A., and Valantin-Morison, M. (2015). Undersowing winter oilseed rape with frost-sensitive legume living mulches to improve weed control. Eur. J. Agron. 71, 96–105. doi: 10.1016/j.eja.09.001
Lutman, P. J. (2018). “Sustainable weed control in oilseed rape,” in Weed Control: Sustainability, Hazards, and Risks in Cropping Systems Worldwide, eds Korres, N. E., Burgos, N. R., and Duke, S. O, (Boca Raton, FL: CRC Press), 325. doi: 10.1201./9781315155913-17
Malézieux, E., Crozat, Y., Dupraz, C., Laurans, M., Makowski, D., Ozier-Lafontaine, H., et al. (2009). Mixing plant species in cropping systems: concepts, tools and models. A review. Agron. Sustain Dev 29, 43–62. doi: 10.1051/agro:2007057
Paulsen, H. M., Schochow, M., Ulber, B., Kuhne, S., and Rahmann, G. (2006). Mixed cropping systems for biological control of weeds and pests in organic oilseed crops. Aspects Appl. Biol. 79, 215–219.
R Development Core Team (2020). R: A Language and Environment for Statistical Computing. Version 4, 0.3. Vienna: R Foundation for Statistical Computing.
Ratnadass, A., Fernandes, P., Avelino, J., and Habib, R. (2012). Plant species diversity for sustainable management of crop pests and diseases in agroecosystems: a review. Agron. Sustain. Dev. 32, 273–303. doi: 10.1007/s13593-011-0022-4
Root, R. B. (1973). Organization of a plant-arthropod association in simple and diverse habitats: the fauna of collards (Brassica oleracea). Ecol. Monogr. 43, 95–124. doi: 10.2307/1942161
Rowen, E. K., Regan, K. H., Barbercheck, M. E., and Tooker, J. F. (2020). Is tillage beneficial or detrimental for insect and slug management? A meta-analysis. Agric. Ecosyst. Environ. 294:106849. doi: 10.1016/j.agee.2020.106849
Seimandi-Corda, G., Jenkins, T., and Cook, S. M. (2021). Sampling pollen beetle (Brassicogethes aeneus) pressure in oilseed rape: which method is best? Pest Manag. Sci. 77, 2785–2794. doi: 10.1002/ps.6310
Skellern, M. P., and Cook, S. M. (2018). The potential of crop management practices to reduce pollen beetle damage in oilseed rape. Arthropod Plant Interact. 12, 867–879. doi: 10.1007/s11829-017-9571-z
Soetedjo, I., Martin, L., and Janes, A. (2003). “Intercropping with canola improves the productivity and sustainability of field pea,” in Proceedings of the 11th Australian Agronomy Conference (Geelong, VIC).
Stoltz, E., Wallenhammar, A. C., and Nadeau, E. (2018). Functional divergence effects of intercropped faba bean and maize in organic production for forage increase mineral contents and reduces leaf spots. Agric. Food Sci. 27, 110–123. doi: 10.23986/afsci.66541
Swedish Board of Agriculture. (2021). Harvest by County and Crop. Available online at: https://statistik.sjv.se/PXWeb/pxweb/sv/Jordbruksverketsstatistikdatabas/Jordbruksverketsstatistikdatabas__Skordar/JO0601J01.px/ (accessed October 28, 2021)
Trenbath, B. R. (1993). Intercropping for the management of pests and diseases. Field Crops Res. 34, 381–405. doi: 10.1016/0378-4290(93)90123-5
Valantin-Morison, M., Meynard, J. M., and Doré, T. (2007). Effects of crop management and surrounding field environment on insect incidence in organic winter oilseed rape (Brassica napus L.). Crop Prot. 26, 1108–1120. doi: 10.1016/j.cropro.2006.10.005
VanKoughnet, B. (2016). On-Farm Evaluation of Peaola Intercropping. Available online at: https://www.manitobapulse.ca/wp-content/uploads/2018/02/On-Farm-Evaluation-of-Peaola-Intercropping-2015.pdf (accessed October 28, 2021).
Veromann, E., Toome, M., Kännaste, A., Kaasik, R., Copolovici, L., Flink, J., et al. (2013). Effects of nitrogen fertilization on insect pests, their parasitoids, plant diseases and volatile organic compounds in Brassica napus. Crop Prot. 43, 79–88. doi: 10.1016/j.cropro.2012.09.001
Verret, V., Gardarin, A., Makowski, D., Lorin, M., Cadoux, S., Butier, A., et al. (2017). Assessment of the benefits of frost-sensitive companion plants in winter rapeseed. Eur. J. Agron. 91, 93–103. doi: 10.1016/j.eja.2017.09.006
Voss, M. C., Ulber, B., and Hoppe, H. H. (1998). Impact of reduced and zero tillage on activity and abundance of slugs in winter oilseed rape. J. Plant Dis. Prot. 105, 632–640.
Walters, K. F. A., Lane, A., Cooper, D. A., and Morgan, D. (2001). A commercially acceptable assessment technique for improved control of cabbage stem flea beetle feeding on winter oilseed rape. Crop Prot. 20, 907–912. doi: 10.1016/S0261-2194(01)00040-0
Weiss, M. J., Schatz, B. G., Garner, J. C., and Nead, B. A. (1994). Flea beetle (Coleoptera: Chrysomelidae) populations and crop yield in field pea and oilseed rape intercrops. Environ. Entomol. 23, 654–658. doi: 10.1093/ee/23.3.654
Williams, I. H. (2010). “The major insect pests of oilseed rape in Europe and their management: an overview,” in Biocontrol-Based Integrated Management of Oilseed Rape Pests, ed Williams, I. H., (New York, NY: Springer Science + Business Media LLC), 1-43. doi: 10.1007./978-90-481-3983-5_1
Zaller, J. G., Moser, D., Drapela, T., Schmöger, C., and Frank, T. (2008). Effect of within-field and landscape factors on insect damage in winter oilseed rape. Agric. Ecosyst. Environ. 123:233–238. doi: 10.1016/j.agee.2007.07.002
Keywords: weed, pathogen, insect pest, slug, intercrop, legume, Brassica napus
Citation: Emery SE, Anderson P, Carlsson G, Friberg H, Larsson MC, Wallenhammar A-C and Lundin O (2021) The Potential of Intercropping for Multifunctional Crop Protection in Oilseed Rape (Brassica napus L.). Front. Agron. 3:782686. doi: 10.3389/fagro.2021.782686
Received: 24 September 2021; Accepted: 02 November 2021;
Published: 03 December 2021.
Edited by:
Lionel Alletto, Institut National de recherche pour l'agriculture, l'alimentation et l'environnement (INRAE), FranceCopyright © 2021 Emery, Anderson, Carlsson, Friberg, Larsson, Wallenhammar and Lundin. This is an open-access article distributed under the terms of the Creative Commons Attribution License (CC BY). The use, distribution or reproduction in other forums is permitted, provided the original author(s) and the copyright owner(s) are credited and that the original publication in this journal is cited, in accordance with accepted academic practice. No use, distribution or reproduction is permitted which does not comply with these terms.
*Correspondence: Sara E. Emery, c2FyYS5lbWVyeUBzbHUuc2U=