- 1Research Group Ecological Plant Protection in Arable Crops, Agroscope, Zurich, Switzerland
- 2Research Group Seed Quality, Agroscope, Zurich, Switzerland
Due to increasing demands to reduce chemical plant protection products, including prophylactic chemical seed treatments, there is a renewed interest in thermal seed treatments for cereal crops. We carried out contemporary evaluations of various alternative seed treatments for economically relevant cereal diseases in Switzerland. Thermal seed treatments were evaluated for effectiveness against two seed-borne diseases, snow mold (Microdochium spp.) and loose smut of barley (Ustilago nuda), commonly found in Swiss cereal production. Field trials testing seed treatments against Microdochium spp., including M. majus and M. nivale, on wheat were conducted across four growing seasons from 2016/17 to 2019/20 and against U. nuda on barley across three growing seasons from 2016/17 to 2018/19. The foci of these trials were primarily on thermal seed treatments, including steam, hot air, and warm water. Additionally, a Cerall® treatment, based on the microorganism Pseudomonas chlororaphis strain MA 342, was included in two of the trials focusing on Microdochium spp. Steam, warm water, and hot air showed high efficacy against Microdochium spp., while Cerall® showed no disease reduction. In the Microdochium spp. 2018/19 trial, a combination of poor field conditions, low quality seed, and high disease pressure reduced seed germination. The 2019/20 Microdochium spp. field trial, which occurred during less challenging field conditions than those in 2018/19 and included the same seed lot from 2018/19 and a less diseased second lot, showed an improved efficacy of the steam treatments. The warm water treatments were found to be the only effective thermal treatment against U. nuda. Our results demonstrate that the steam treatments more readily affected germination rate in a highly diseased seed lot, while warm water treatments showed limited damage to the seed. Warm water was found to be the most consistently effective thermal treatment against both diseases, and constraints in implementing such a treatment are discussed. If the steam treatment parameters are correctly set to minimize damage to the plant, it offers effective protection against some seed-borne diseases. Overall, the results from this study give more information about effectiveness of alternative seed treatments under various field conditions.
Introduction
In conventional agriculture, preventive chemical-synthetic seed dressing is common practice for field crops. In Australia, the E.U. and the U.S., cereals account for some of the largest volumes of fungicide active ingredients applied as seed treatments (Lamichhane et al., 2020). Increasing interest in planting non-treated seed outside of organic grain cultivation may lead to a reduction of prophylactic seed treatments in cereals in integrated production (Lamichhane, 2020). Concerns about human and ecosystem health as well as the rise of fungal resistance to fungicides have led to increased societal pressure to reduce chemical pesticides (Nicolopoulou-Stamati et al., 2016; Berger et al., 2017). Several European countries have introduced national action plans since 2011 that mandate the reduction of pesticides (Directive 2009/128/EC), encouraging a reduction of preventative seed dressings. Similar to national action plans from several European countries (Barzman and Dachbrodt-Saaydeh, 2011; Möhring et al., 2020), a Swiss national action plan launched in 2017 aims to reduce the risk of plant protection products by 50% (Bundesrat, 2017).
Due to shifting public sentiment and the passage of national mandates, finding alternative seed treatments has gained more urgency. Synthetic chemical seed dressings are effective means to control seed-borne cereal diseases, which has made it challenging to adopt alternative methods. A few alternative seed treatments to combat seed-borne diseases are already available on the market for organic farming, including Cerall® and Cedomon® (Widén and Annas, 2004), which are both composed of the microorganism Pseudomonas chlororaphis strain MA 342 for wheat and barley, respectively. Additionally, Tillecur®, which is composed of mustard flour, has shown effectiveness against Tilletia caries, causing common bunt in wheat (Winter et al., 2001). However, these seed treatments can exhibit varying efficacies (Tinivella et al., 2009; Krebs et al., 2011), and Tillecur® currently is no longer registered in Switzerland. In addition to alternative treatments composed of microorganisms or plant products, thermal treatments have reemerged as an alternative possibility to existing synthetic chemical treatments (Winter et al., 1997; Forsberg et al., 2005; Koch et al., 2010).
Thermal treatment methods, such as the use of steam or warm water, are effective because pathogens are often more sensitive to elevated temperatures than the plant host (Baker, 1962). The temperature range and duration (i.e., treatment window) for controlling pathogens without damaging the seed embryo depends mainly on the seed's heat tolerance which is dependent on its physiological condition (Forsberg, 2004). Factors that affect the seed quality include age, handling history (i.e., possible exposure to mechanical damage from harvest or storage), and conditions during development or storage. A number of different thermal treatment methods have been described, including water baths and aerated steam with different derivations for various plant parts such as seeds, trees, cuttings, cut flowers, and sprouts (Grondeau et al., 1994). The use of heat to treat pathogenic organisms in wheat and barley was first reported over 100 years ago (Jensen, 1888). Since then, the effectiveness of warm water on cereal diseases has been documented to work against numerous diseases (Winter et al., 1997, 1998a). Despite their earlier documented efficacy, thermal methods have not been widely utilized on cereal seed due to the availability of inexpensive and efficient synthetic chemical treatments and low value of field crops (Lamichhane et al., 2020). As more pressure to reduce chemical synthetic seed treatments has grown, the reevaluation of thermal and alternative seed treatment methods is warranted.
In Switzerland, the main problematic seed-borne diseases in cereals include loose smut of barley (Ustilago nuda) (Hebeisen, unpublished data), snow mold (Microdochium spp.), and common [T. caries (DC.)] and dwarf bunt (Tilletia controversa Kühn) of wheat (Bänziger et al., 2012). The pathogens M. nivale (formerly M. nivale var. nivale) and M. majus (formerly M. nivale var. majus) are the causative agents of seedling blight, particularly under cool temperatures and sufficient snowfall, and can co-exist with each other (Nielsen et al., 2013). Using field and laboratory trials, various seed treatment methods, including a warm water and steam treatments as well as a biological control product were tested against a disease that is mostly present in non-embryo seed tissue (Microdochium spp.) and a disease located within the seed embryo (U. nuda). These experimental results help to inform which alternative seed treatments are appropriate to use against diseases that have different characteristics and locations within cereal seeds, and challenges associated with the effective treatments' implementation are discussed.
Materials and Methods
Seed
Winter wheat (Triticum aestivum L. subsp. aestivum) seed naturally infected with Microdochium spp. and winter barley (Hordeum vulgare L. subsp. vulgare) seed naturally infected with U. nuda were used for the laboratory investigations and field trials (Table 1). For the 2016/17 Microdochium spp. trials, the Wiwa variety was used, whereas the Nara variety was used during all subsequent Microdochium spp. trials (2017/18, 2018/19, and 2019/20). The Runal variety was also included in the 2019/20 trial. All varieties are on the Swiss list of recommended varieties (Courvoisier et al., 2016) and were chosen due to the availability of naturally infected seed. These varieties were self-propagated at Agroscope and infection had been observed in the field during propagation. The same seed lots were used in the laboratory tests and field trials. For U. nuda trials, the variety, Cassiopee, was used for the 2016/17 trial and the variety, Caravan, was used for the 2018/19 trial. At the time of the study, Caravan was on the Swiss list of recommended cereal varieties, and Cassioppee has been listed in the European Commission's common varieties (European Commission, 2012).
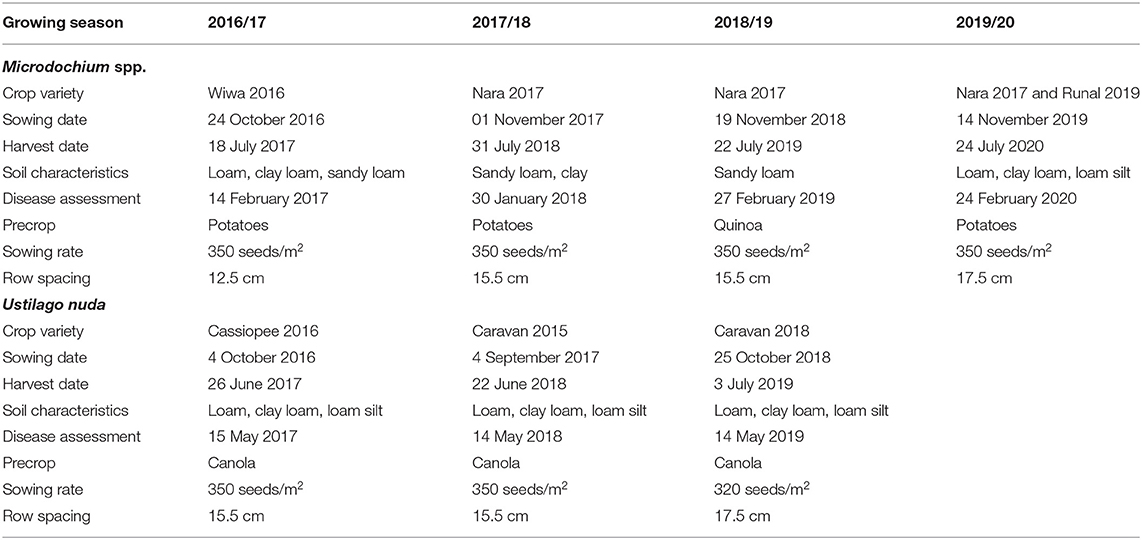
Table 1. Field conditions for Microdochium spp. on winter wheat and Ustilago nuda on barley field trials in growing seasons from 2016/17 to 2019/20.
Molecular Identification of Microdochium spp. in Seed
Because colonies of M. majus and M. nivale are difficult to visually distinguish from each other, we used species-specific PCR primers to determine if the seed used in this study was infected with M. majus, M. nivale, or a mixture of the two species. Samples of 100 g of each seed lot used in the field and laboratory trials were milled into flour. DNA was isolated from a 100 mg subsample of the milled flour using the NucleoSpin Plant II kit for DNA from plants (Macherey-Nagel, Düren, Germany) following the manufacturer's instructions. The primers used for the species-specific PCRs included distinct forward primers for each species: M. nivale (EFNiv/F: 5′-GTT CCC CTG TCT GAC TGT TGT-3′) and M. majus (EFMaj/F: 5′-CCC CTT CTC CCT ATC GC-3′) and a shared reverse primer (EFMic: 5′-GTC TCG ATG GAG TCG ATG G-3′), which have been previously published (Glynn et al., 2005). PCRs were performed in 20 uL volumes and included 50 ng of template DNA, 300 nM of each primer, 10 μL GoTaq® G2 Green Master Mix (Promega). PCR cycling conditions consisted of an initial denaturation of 95°C for 2 min 30 s and then 35 cycles of 95°C for 20 s, 54°C for 30 s, and 72°C for 45 s, followed by a final extension of 7 min at 72°C. Electrophoresis of the amplified product was performed on a gel containing agarose (1.0% w v-1). Bands on the gel were compared to positive controls of M. majus and M. nivale that had previously been identified.
Treatments
The trials on Microdochium spp., conducted in the growing seasons 2016/17 and 2017/18, included an untreated control and the same 11 seed experimental treatments (see Table 2 for list of included treatments). The treatments were composed of warm water (WW) at 45°C for 2 h (with and without subsequent drying), WW for 3 h (with and without subsequent drying), four steam treatment regimes (65°C for 90 s, 65°C for 120 s, 70°C for 90 s, 70°C for 120 s), hot air (70°C for 2 days), as well as a biological (Cerall®, Stähler) and a chemical (Celest® Trio, Syngenta) seed treatment. The procedures for the WW, steam, and hot air treatments are described in more detail below. Cerall®, composed of the soil bacterium P. chlororaphis, is registered for partial protection against snow mold. The water, steam, and hot air treatment parameters were chosen based on preliminary trials and published studies (Winter et al., 1996; Krebs et al., 2011). In the growing seasons of 2018/19 and 2019/20, only the 2 h WW treatment with subsequent drying was evaluated. The inclusion of only one WW treatment in 2018/19 and 2019/20 was due to consistently good results from the WW treatment in previous years and an interest to focus on steam treatments. A steam treatment of 68°C for 180 s as well as one performed at ThermoSeed Global AB (Uppsala, Sweden) with a ThermoSeed® machine were included for both seasons of 2018/19 and 2019/20, and an additional steam treatment of 70°C and for 180 s was included for the 2019/20 trial. The parameters for the ThermoSeed® machine treatments were determined by ThermoSeed Global AB following preliminary tests and are considered proprietary knowledge. The preliminary testing is carried out to optimize the machine treatment parameters based on the characteristics of the seed lot. All treatments using the same seed were assessed in field trials and laboratory tests with the exclusion of the seed treated for the 2016/17 growing season, which were only tested in the field trial.
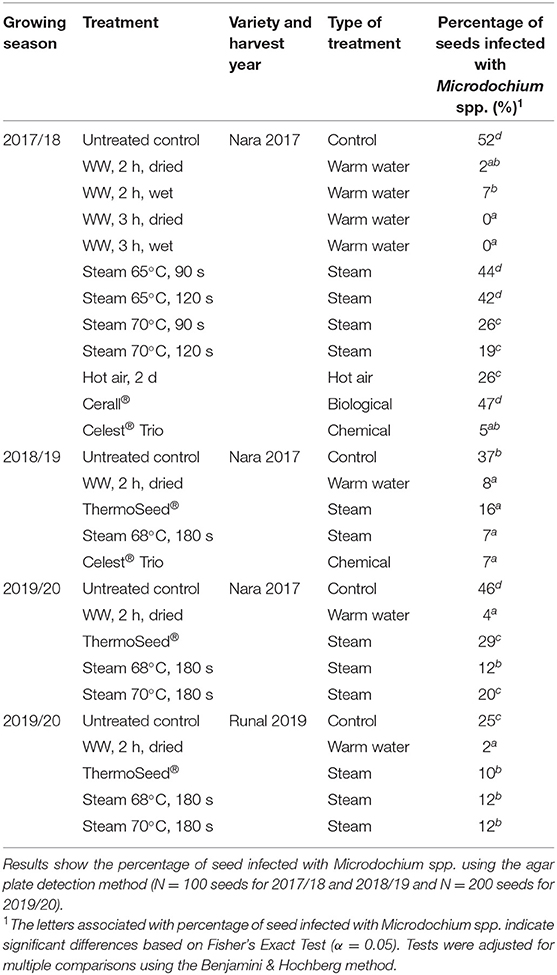
Table 2. The detection of Microdochium spp. in winter wheat seeds following treatments included in the field trials between 2017/18 and 2019/20.
The treatments to control loose smut of barley in the growing seasons 2016/17 and 2017/18 included an untreated control and nine experimental seed treatments (see Table 3 for list of included treatments). The treatments were composed of four WW treatments at 45°C (for 2 or 3 h with or without subsequent drying), three steam treatments (65°C for 90 s, 70°C for 90 s, 70°C for 120 s), hot air, and the synthetic chemical fungicide, Celest® Trio. For the season of 2018/19, only the 2 h WW treatment with subsequent drying was included as well as two longer steam treatments (68°C for 210 s, 70°C for 210 s). The longer steam treatment times were included to explore the treatment's limit with regard to its phytotoxic effects on barley.
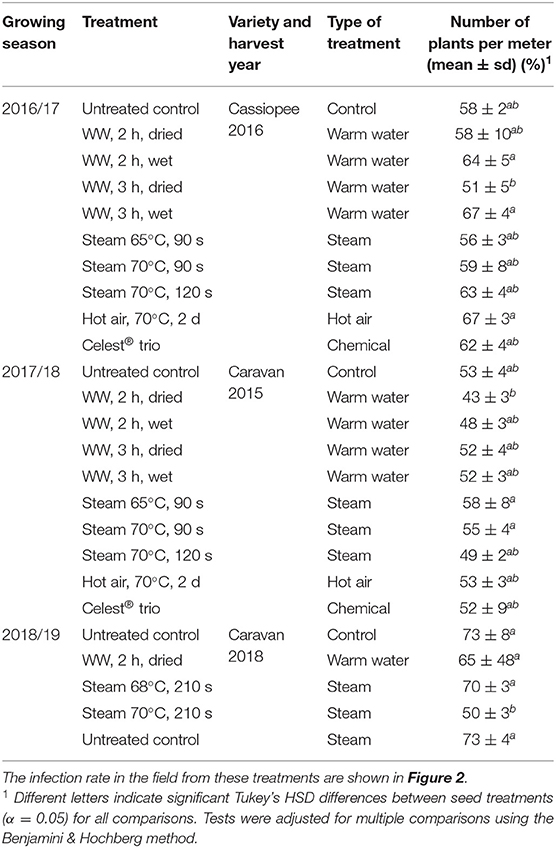
Table 3. Field trial results focusing on loose smut of barley (Ustilago nuda) control, showing the number of barley plants per meter from 2016/17 to 2018/19.
The WW treatments were performed using a flow through water bath, and seeds with a subsequent drying step were laid on a metal tray in a drying oven at 40°C for ~12 h with circulating air (WTB Binder, Germany). The seed was dried to the original 14% moisture content. The WW treatments without subsequent drying were treated the day prior to sowing and were laid out on an iron mesh overnight at room temperature to allow the seed to partially air-dry. The seed was then sown while still wet (~25–30% moisture content). The hot air treatment consisted of treating seeds in a drying oven without circulating air (Heraeus, Germany). All non ThermoSeed® steam treatments conducted in seasons 2016/17, 2017/18, and 2018/19 were performed at Sativa Rheinau, using a machine consisting of a conveyor belt and steam humidifier (Condair, Switzerland). The steam treatment for the 2019/20 growing season was performed at Agroscope, Reckenholz, Switzerland using an electric combi-steamer (Model XVC705E-0D00, UNOX). The relative humidity measured in the steam chamber was between 68 and 72%.
Laboratory Tests
In order to directly assess the efficacy of treatments against Microdochium spp., seeds included in the field trials in growing seasons 2017/2018, 2018/19, and 2019/20 were examined on agar following a modified ISTA protocol (International Seed Testing Association, 2014). The Microdochium infection levels of seed used in the field trials were determined based on 100 seeds on agar plates for the growing season 2017/18 and 200 seeds per treatment for growing seasons 2018/19 and 2019/20. Seeds were surface sterilized for 10 min in a 1% Chloramine T trihydrate solution (Sigma-Aldrich, China), and subsequently, the 100 or 200 seeds were divided into batches of 10 seeds, which were laid out equidistance from each other on a 9 cm diameter Petri plate filled with potato dextrose agar (PDA) (OXOID, United Kingdom) with 0.01% streptomycin (Sigma-Aldrich, China). The seed was then incubated for 7 days at 18°C (±1°C) at 12 h of near ultraviolet light and 12 h of darkness. Outgrowing colonies of Microdochium spp. were visually identified based on colony and aerial mycelium appearance using ISTA protocols and as done in other studies focused on detecting Microdochium spp. (Kammoun et al., 2009; Matušinsky et al., 2017). Each seed was assessed as either infected or uninfected based on Microdochium spp. mycelium growth or its absence around seed. From these data, a percentage of infected seed for each treatment was derived.
Field Trials
Field trials focusing on Microdochium spp. in winter wheat were conducted in growing seasons 2016/17, 2017/18, 2018/19, and 2019/20. Field trials focusing on U. nuda in winter barley were carried out in growing seasons 2016/17, 2017/18, and 2018/19. All trials were run on experimental fields at Agroscope, Reckenholz (Zurich, Switzerland) and were sown in a randomized complete block design with four replicates (see Table 1 for additional details). Approximately 1 month prior to sowing, the seedbed was ploughed, and all fields were harrowed with a rotary cultivator within 1 day prior to sowing. Seeds were sown with a Hege 76 disc coulter in 1.5 × 6 m plots with seven rows driven at a speed of ~1.7 km/h. The plot seeder was equipped with a mechanical rotational distributor that prevented clogging while sowing wet seed, and a set seeding rate was used across all treatments ensuring that the same number of seeds were distributed in the plot. Because the plots had been seeded consecutively, 1.5 m of the plot was destroyed by mulching or glyphosates (0.75 m from each end of the plots' lengths) at the booting stage to avoid any seed treatment mixtures that may have occurred at the ends of the plot. The remaining plot size was 4.5 × 1.5 m. Weather was recorded on a MeteoSwiss weather station located at Zurich-Reckenholz and within 1 km of experimental sites.
Disease Evaluation and Statistical Analysis
The disease assessment for Microdochium spp. in field trials was conducted every year in January or February (Table 1) by evaluating plant emergence at the growth stage of DC 11 to DC 12 (Zadoks et al., 1974). Microdochium spp. primarily affects seedling germination, so the evaluation of field emergence serves as an indicator of treatment efficacy (Vogelgsang et al., 2013). For the field assessment, four counts of plants per meter were averaged within each plot, using areas at least 1 m from the ends of the plot and from inside rows. To test the differences among treatments in each year and trial, ANOVAs were run on the average number of plant per meter within each plot. To see if the data satisfied the assumptions of an ANOVA, quantile comparisons and residuals vs. fitted values were plotted and visually inspected. Additionally, the Levene and Shapiro Wilk tests were run to check assumptions of ANOVAs. The data from the Microdochium spp. field trials in years 2016/17, 2018/19, and the trial with the variety Runal in 2019/20 satisfied the assumptions of ANOVA tests. For these years, a Tukey's honest significant difference (HSD) test was used for pairwise comparisons following significant ANOVA results. The data from the Microdochium spp. trials in 2017/18 and 2019/20 on the variety Nara exhibited a non-parametric distribution, and so a Kruskal Wallis test was used followed by the Pairwise Wilcoxon Rank Sum test with the Benjamini & Hochberg procedure to adjust for multiple comparisons.
Analyses of seeds set on agar plates in the laboratory were also used to determine Microdochium spp. infection in three of the growing seasons 2017/18, 2018/19, and 2019/20. To examine percentage of diseased seeds on agar plates, the difference in numbers of seeds with and without Microdochium spp. colonies were evaluated using the Fisher's Exact Test. Additionally, post-hoc pairwise tests of independence for nominal data was conducted to look for pairwise differences between treatments.
For loose smut of barley, the infection rate was assessed by counting the number of infected heads within a set area of the plot that differed between years to account for variable infection rates. Because of a very low infection rate in 2017, all infected heads per plot were counted. Due to a much higher infection rate in years 2018 and 2019 (Figure 2), infected heads were counted along a random 1 m transect. In all years, the U. nuda infection was evaluated before flowering (DC 59). In all growing seasons, the number of plants along the 1 m transect was also counted to help determine if any treatments had a phytotoxic effect and inhibited plant growth. The loose smut data from the growing season 2018/19 satisfied the assumptions of ANOVAs and were evaluated using Tukey's HSD following a significant ANOVA result. For the two other years, the assumptions of homogeneous variances and normal distribution were violated, so the non-parametric Kruskal-Wallis test was performed followed by a Pairwise Wilcoxon Rank Sum test with the Benjamini & Hochberg procedure to adjust for multiple comparisons. All statistical analyses and graphs were carried out in R (version 4.0.3) using the following R packages: car (Fox and Weisberg, 2019), multcompView (Graves et al., 2015), ggplot2 (Wickham, 2016), and rcompanion (Mangiafico, 2016).
Weather Conditions
Weather conditions in the 2016/17 season were extremely dry and devoid of snow. Precipitation totals from December 2016 to February 2017 were half of the normal values based on average totals from 1981 to 2010 (MeteoSchweiz, 2017, 2018, Supplementary Figure 1). The spring was also exceptionally warm. In the 2017/18 season, record warm temperatures were measured in January, but February was very cold, followed by a cool March and warm spring (MeteoSchweiz, 2019). Precipitation in January was very high compared to the normal levels followed by drier than normal spring. In December of the 2018/2019 growing season, it was particularly wet with over 150 mm of rain—nearly double the norm. February to April showed slightly warmer and drier than average temperatures and precipitation (MeteoSchweiz, 2020). The 2019/2020 growing season had a more mild winter with slightly more precipitation than average over the winter months and slightly warmer temperatures (MeteoSchweiz, 2021).
Results and Discussion
Microdochium spp.
The seed used in the field and laboratory trials was infected with a mixture of M. nivale and M. majus based on the presence of bands after the amplification of DNA using a species-specific primer set for each pathogen. These two species often co-exist (Nielsen et al., 2013), but previous work suggests that M. majus has a selective advantage over M. nivale on wheat, where it is typically more prevalent than M. nivale (Simpson et al., 2000). These findings correspond to the stronger bands we found in all of our seed samples while using the primer set for M. majus. Nonetheless, it is thought that the differentiation of M. majus and M. nivale may not be essential from a disease control and economic standpoint due to their widespread co-occurrence (Nielsen et al., 2013).
Because a seed-borne infection with Microdochium spp. primarily affects the germination ability of the seed, seedling emergence, and plant establishment (Nielsen et al., 2013; Vogelgsang et al., 2013), emergence rate was used to measure infection levels in field trials. Across all four years, the seed treatment had a significant effect on plant emergence in the field trial [2016/17: F(11, 36) = 6.51, p < 0.01; 2017/18: H(11) = 43.23, p < 0.01; 2018/2019: F(11, 36) = 46.16, p < 0.01; 2019/20-Nara: H(4) = 17.72, p = 0.01; 2019/2020-Runal: F(4, 15) = 12.76, p-value < 0.01], showing that the treatments had different effects on the control of Microdochium spp. In all trials, WW treatments showed the greatest plant emergence (Figure 1), and all WW treatments resulted in significantly more plant emergence than in the control treatments. Previous experiments have shown the WW results to be on par with those of synthetic chemically treated seed (Winter et al., 1994) as observed in this study (Table 2). In 2016/17 and 2017/18, the four variations of the WW treatment (2 h with and without drying, 3 h with and without drying) yielded similar levels of plant emergence with the exception of a decrease in the 2017/18 2 h WW without drying treatment (Figure 1). However, the 2 h WW without drying treatment in 2016/17 gave a slightly higher plant emergence rate, although not significantly different from the other WW treatments. Across both years, the majority of the WW treatments with and without subsequent drying showed no difference in treatment outcome, suggesting that the sowing of wet seed soon after the treatment did not negatively affect plant germination and growth. Wetting seed prior to planting can give them a priming effect (Harris, 2006), especially in salinity-stressed or dry climates (Bouaziz and Bruckler, 1989; Carrillo-Reche et al., 2018). Because the experiments were conducted in a humid continental climate and because measurements were taken at DC 11 to DC 12, giving plant sufficient time to germinate, it was assumed that the main observed differences in plants per meter among treatments were due to disease reduction. Nonetheless, there are reports of synergies between priming effects and disease reduction (Musa et al., 2001; Harris, 2006), so a positive effect of priming due to seed imbibition may also contribute to the observed treatment differences.
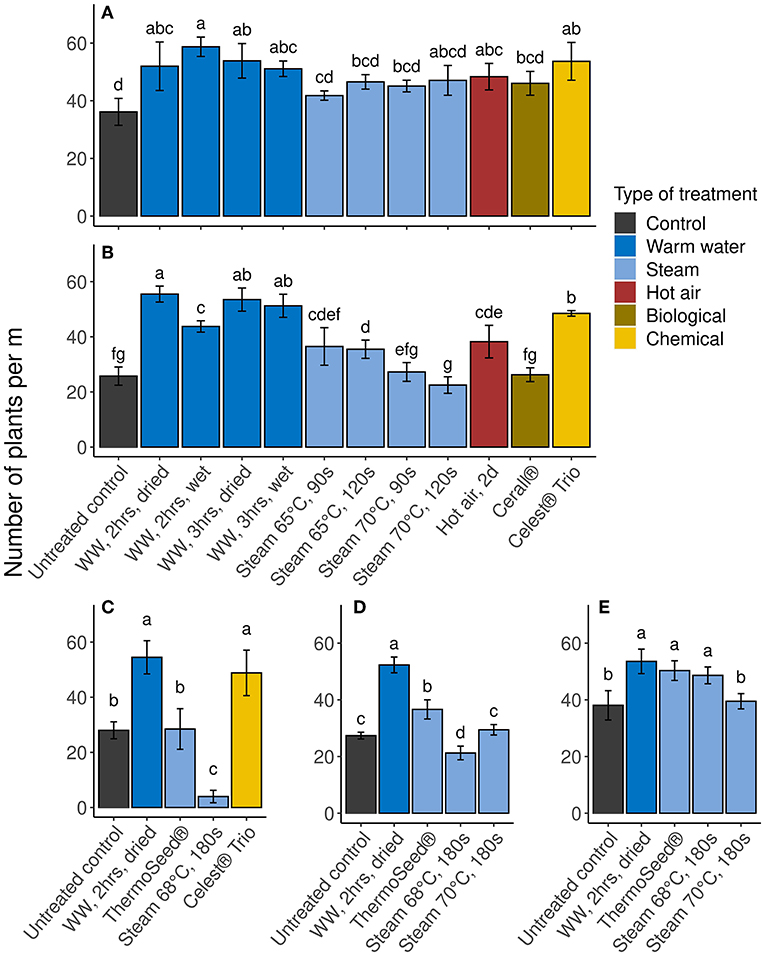
Figure 1. Efficacy of thermal and alternative treatments to control Microdochium spp. in wheat under field conditions based on field germination rates (number of plants per meter) in (A) 2016/17, (B) 2017/18, (C) 2018/19, (D) 2019/20 with the variety Nara, and (E) 2019/20 with the variety Runal. Treatments included warm water (WW), steam, hot air, and biological (Cerall®) and chemical (Celest® Trio) plant protection products. Times in thermal treatments indicate different treatment durations. Error bars indicate the standard deviation of the means. Means sharing a letter are not significantly different (α = 0.05).
The corresponding decrease of Microdochium spp. infections in treated seed based on the laboratory results reflects the differences in wheat emergence rate in the field for most treatments. Some phytotoxic effects of the higher temperature steam treatments are evident based on a lower germination rate in the field despite a decreased Microdochium spp. infection rate observed in the laboratory (i.e., the steam treatment at 68°C for 180 s in 2018/19 trial—discussed in more detail below). WW treatments have previously shown good effectiveness against Microdochium spp., and various time and temperature parameters have been explored (Winter et al., 1997, 1998a). Based on these previous results, 45°C was found to be most effective while minimizing phytotoxic effects, which were observed more often while using hot water (52°C) with shorter treatment times (Winter et al., 1998b). The phytotoxic effects become more noticeable at the 52°C WW treatment because seeds are more sensitive to higher temperatures at elevated moisture contents (Bewley et al., 2013; Tangney et al., 2019). This relationship between temperature tolerance and seed moisture content corresponds to the use of higher temperatures in steam and hot air treatments. The moisture content of seeds treated with steam or hot air is less than that of seeds treated in a water bath, and therefore, they can withstand higher temperatures.
In 2016/2017 and 2017/18, the hot air treatment showed a significant improvement over the untreated control, but it was not as effective as the WW treatment (Figure 1). Based on the results from the agar plate detection tests (Table 2), both hot air and WW treatments showed a reduction in Microdochium spp. The hot air, consisting of dry heat, has also shown good results on other seed-borne diseases, including bacterial blight caused by Pseudomonas syringae pv. Pisi in pea seeds (Grondeau et al., 1992) as well as a number of viral diseases (Koch and Roberts, 2014). On the other hand, Cerall® showed no improvement in plant emergence over the untreated control treatment in our 2016/17 and 207/28 field trials. The agar plate detection results of the Cerall® treated seeds from the 2017/18 season showed no significant reduction of Microdochium spp., indicating that the product in this study was not effective against the disease. This finding is in line with results from Johnsson and colleagues, who found limited effect of the active bacterium in Cerall®, P. chlororaphis MA 342, against Microdochium spp. in spring and winter wheat.
Overall, steam has the potential to be very effective at reducing Microdochium spp. infection, but its performance in the field within this study was dependent on the year—most likely due to a combination of seed quality, as well as field and weather conditions. In 2016/17, there were no differences between any of the steam treatments and the untreated control (Figure 1). In 2017/18, the 65°C 120 s treatment showed improvement over the control while the other treatments did not. In 2018/19, both the ThermoSeed® treatment and other steam treatments had poor results; they either showed no difference from the untreated control or reduced plant emergence, respectively. This was most likely a combination of challenging field conditions and low seed quality. Prior to treatment, ThermoSeed Global AB had advised against using the Nara 2017 seed lot because their preliminary tests suggested a possibility of poor seed condition and, thus, poor performance. Nevertheless, the Nara lot was used to assess the differences of treatments in a more challenging seed lot and to test the limitations of the treatment. Indeed, the steam treatments on the Nara seed lot did not perform well in 2018/19. This was most likely due to the interaction of the high infection rate and poor physiological condition of the seed as well as the poor field conditions. Due to especially high amount of rainfall and very wet conditions after sowing (Supplementary Figure 1), the field became flooded in 2018/19, making it especially challenging for the more heavily infected Nara seed lot. Waterlogging can particularly affect plants at the germination and emergence phase (Setter and Waters, 2003), and these challenging conditions combined with highly infected seed appeared to cause increased mortality to the plants at these stages in our study. Previous work has also noted the interaction of treatment effect and weather conditions (Osman et al., 2004), suggesting that the poor weather conditions were too difficult for the highly infected seed to overcome. Due to the additional stress from the thermal treatment in combination with the field and seed condition, the 68°C, 180 s steam treatment showed reduced germination compared to the control.
To examine the performance of the same Nara seed lot under better field conditions, the ThermoSeed® and the 68°C, 180 s steam treatments were repeated in 2019/20 with an additional stream treatment at 70°C. Indeed, the ThermoSeed® treatment in 2019/20 showed higher plant emergence than the untreated control treatment, while the 68 and 70°C steam treatments performed the same or worse compared to the untreated control. For the Runal seed lot, both the ThermoSeed® and 68°C steam treatment in 2019/20 showed significantly higher plant emergence than the untreated control, while the 70°C steam treatment showed no improvement. The agar plate detection results demonstrated disease reduction in all steam treatments over 65°C and a marginal decrease in the 65°C treatments (Table 2). Therefore, it appears that the reduced plant emergence observed in some of the steam treatments was due to phytotoxic damage to the seed rather than from infection with Microdochium spp. Phytotoxic effects resulting in reduced germination from steam treatments and dry heat treatments have been documented (Koch and Roberts, 2014), illustrating the usefulness of establishing optimal steam treatment parameters for each lot. Due to the potential for phytotoxic effects, the implementation of steam treatments in practice perform better following preliminary tests (Forsberg et al., 2003; Forsberg, 2004). Like the other thermal treatments, water has the potential to reduce germination, as previously found when the higher temperature of 52°C is used (Winter et al., 1996). Based on this work, the 2 h, 45°C WW treatment was found to result in minimal phytotoxic effects.
Ustilago nuda
In the U. nuda trial on barley, significant differences were found in all years among treatments [2016/17: H(9) = 34.77, p < 0.01; 2017/18: H(9) = 34.19, p < 0.01; 2018/2019: F(4, 15) = 16.29. p < 0.01]. Only the WW and chemical control treatments showed a consistent and significant reduction of diseased heads in the field (Figure 2). WW treatments have previously shown effectiveness against U. nuda (Batts, 1956; Doling, 1965). Interestingly, the chemical control showed a decreased effectiveness compared to the WW in the growing seasons 2017/18 and 2018/19. Resistance of U. nuda to other chemical fungicides that include carboxamides has been documented (Leroux and Berthier, 1988; Dhitaphichit and Jones, 1991; Menzies et al., 2005). However, U. nuda fungicide resistance was not assessed in this experiment. The WW treatment did not show any significant phytotoxic damage to the seed compared to the untreated control based on the number of plants per meter (Table 3). The steam 65°C, 90 s treatment in 2016/17 showed a significant improvement over the untreated control without a significant reduction in number of plants, but in this year, the rate of disease was relatively low. In 2018/19, the strongest steam treatment (70°C, 210 s) was not effective enough to significantly reduce the infection rate but, nonetheless, was accompanied by a severe reduction in the number of plants, suggesting a phytotoxic effect. Based on the results presented in the current study and previous studies (Doling, 1965; Winter et al., 1996), WW was the only seed treatment to work effectively and consistently against U. nuda.
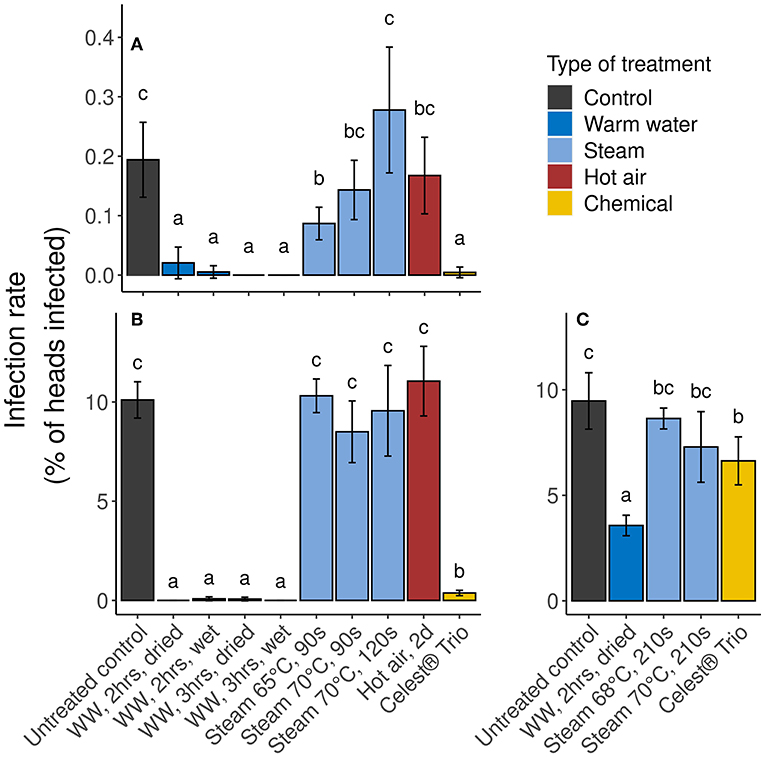
Figure 2. Efficacy of thermal treatments to control Ustilago nuda in barley based on percentage of infected heads from field trials in (A) 2016/17, (B) 2017/18, and (C) 2018/19. Different y-axis scales are used in (A) than those used in (B,C) to accomodate different infection rates. Times in thermal treatments indicate different treatment durations. Error bars show the standard deviation of the means. Means sharing a letter are not significantly different (α = 0.05).
Practical Considerations and Implications
The results from our field and laboratory trials to control Microdochium spp. and field trials to control U. nuda suggest that different alternative treatments are better suited for the diseases included in this study. The steam treatment can be very effective against Microdochium spp. as well as other seed-borne diseases, including T. caries (Forsberg et al., 2005). In the present study, steam was generally not effective against U. nuda with the exception of one steam treatment regime (steam 65°C, 90 s) in the 2016/17 season. The difficulty in using steam to combat U. nuda may be due to the pathogen's heat resistance or localization in the embryo, which makes it difficult to kill the pathogen without damaging the seed. Further work is needed to disentangle these two factors. Cerall® was found to be ineffective against Microdochium spp., which corroborates previous work (Johnsson et al., 1998). However, Cerall® was shown to be more effective against T. caries (Hökeberg et al., 1997), agreeing with its primary intended use according to its registration.
In this study, WW treatments were found to be effective against two diseases with diverse localities within seeds. However, previous work has shown only moderate effects of WW treatments against T. caries (Winter et al., 1998b), so it would not be optimal for all seed-borne diseases. Nonetheless, U. nuda is especially challenging to treat with alternative treatments due to its presence within the seed embryo. Previous research exploring various seed treatments with plant extracts and the bacterial biocontrol agent, P. chlororaphis, found insufficient success against U. nuda (Tombolini et al., 1999; Koch et al., 2010). The WW treatments used in the present study showed effective reduction of U. nuda and Microdochium spp. without adverse effects on the seed quality.
The introduction of WW as a prophylactic seed treatment for cereals would be extremely challenging due to the logistical and technical constraints associated with implementing it on a large-scale and the comparatively low value of cereal seeds. The energy requirement associated with re-drying the seed is one main obstacle (Borgen, 2004; Matanguihan et al., 2011), and many economic questions remain regarding its adoption (Osman et al., 2004). If an on-farm WW treatment option were available, the direct sowing of wet seed could offer a way to overcome the energy requirement for re-drying WW-treated seeds. Our results suggest that a WW treatment without subsequent drying could be a valid treatment option on a small-scale. This type of treatment may be appropriate for small organic producers or in special cases when there is a desire to propagate known U. nuda infected seed, but more work is needed to evaluate which equipment is suitable to sow wet seed. We have sown wet, but air-dried overnight, WW treated seed in an one hectare field trial unrelated to the present study with an Amazone D8-30 Special drill seeder (unpublished data). This type of seed driller is used in normal farm operations, whereas the plot seeder used in this study is usually only used for research purposes. For the one hectare trial, the sowing rate was reduced due to a decreased flow, however, clogging was not a problem. Therefore, the sowing of wet seed may require adaptation to farm equipment and could be tested with different types of seeders. For example, pneumatic seeders may be more capable to handle wet seed. The use of on-farm seed priming is appropriate for resource-poor, small-scale farmers (Harris, 2006), suggesting the feasibility, on a small-scale, of sowing wet, surface-dried seed. The incorporation of warm water in such treatments on a small-scale may be suitable in particular cases to combat U. nuda due to the lack of other effective alternative treatments against loose smut or for early generations of barley multiplication (pre-basic and basic seed). Further work and economic analysis are needed to determine when it would be advisable.
Steam, on the other hand, which is effective against many seed-borne diseases, but not U. nuda, is easier to implement on a larger scale. Steam has a reduced energy and water requirement compared to the WW treatment, lowering treatment costs (Sharma et al., 2015). The reduced water and energy consumption required for steam compared to hot water also makes steam advantageous for thermal weed control (Ascard et al., 2007). The use of steam for seed treatment is also more feasible because driers can be integrated into the treatment, enabling a flow-through process (Forsberg et al., 2005). The seed has a lower moisture content following steam treatment, easing the drying procedure compared to a warm water treatment. The use of preliminary tests for each seed lot is necessary to minimize possible detrimental effects to the seed. Seed lots with low thermal tolerances due to seed age, handling or storage conditions can be difficult to treat with steam, and such pre-tests can determine the steam treatment's suitability (Forsberg et al., 2003). Due to the reduction of germination that can result from the thermal treatments' damage to the seed, the goal is to find a balance between minimizing injury to the plant and maximizing disease reduction.
Conclusions
Our field trials suggest that, in general, thermal seed treatments show promise for Swiss agriculture. The implementation of these treatments is dependent on a number of factors, including ease of adoption, characteristics of the diseases to be controlled, and energy requirements. Additional work in diverse field and ecological conditions across Switzerland will further establish the effectiveness of alternative seed treatments and the ability to implement them into the Swiss market. Due to different efficacies of diverse alternative treatments across diseases, improved fungal pathogen detection methods will assist decision making regarding disease control (Vannacci et al., 2014). Such a testing strategy could match effective treatments with seed lots depending on disease presence and infection levels and, ultimately, reduce prophylactic seed dressings with synthetic chemical fungicides.
Data Availability Statement
The raw data supporting the conclusions of this article will be made available by the authors without undue reservation.
Author Contributions
IB and AK: conceptualization. KES and SK: data analysis. IB, AK, and SK: investigation. KES: writing—original draft preparation and visualization. SV, AB-M, and KES: writing—review and editing. KES and AB-M: project administration. SV, TH, KES, and AB-M: funding acquisition. All authors have read and agreed to the final version of the manuscript.
Funding
Funding from fenaco and the Hauser Stiftung supported this project.
Conflict of Interest
The authors declare that the research was conducted in the absence of any commercial or financial relationships that could be construed as a potential conflict of interest.
Publisher's Note
All claims expressed in this article are solely those of the authors and do not necessarily represent those of their affiliated organizations, or those of the publisher, the editors and the reviewers. Any product that may be evaluated in this article, or claim that may be made by its manufacturer, is not guaranteed or endorsed by the publisher.
Acknowledgments
We thank fenaco and the Hauser Stiftung for their support. We acknowledge and thank ThermoSeed Global AB and Sativa Rheinau for their collaboration on this project, Gustaf Forsberg for helpful feedback and discussion, Tomke Musa for assistance in retrieving the weather data, and Eveline Jenny and Cecilia Panzetti for their support in the laboratory. We would also like to acknowledge the constructive feedback from the reviewers.
Supplementary Material
The Supplementary Material for this article can be found online at: https://www.frontiersin.org/articles/10.3389/fagro.2021.775243/full#supplementary-material
References
Ascard, J., Hatcher, P. E., Melander, B., and Upadhyaya, M. K. (2007). “Thermal weed control,” in Non-Chemical Weed Management: Principles, Concepts and Technology, eds M. K. Upadhyaya and R. E. Blackshaw (Wallingford: CABI Publishing), 155–175.
Bänziger, I., Zanetti, S., Hebeisen, T., Graff, L., and Vogelgsang, S. (2012). Fifteen years of organic cereal seed health analyses at Agroscope ART [in German]. Agrarforsch. Schweiz 3, 12–19.
Barzman, M., and Dachbrodt-Saaydeh, S. (2011). Comparative analysis of pesticide action plans in five European countries. Pest Manag. Sci. 67, 1481–1485. doi: 10.1002/ps.2283
Batts, C. C. V. (1956). The control of loose smut in wheat and barley. Ann. Appl. Biol. 44, 437–452. doi: 10.1111/j.1744-7348.1956.tb02138.x
Berger, S., El Chazli, Y., Babu, A. F., and Coste, A. T. (2017). Azole resistance in Aspergillus fumigatus: a consequence of antifungal use in agriculture? Front. Microbiol. 8:1024. doi: 10.3389/fmicb.2017.01024
Bewley, J. D., Bradford, K. J., Hilhorst, H. W. M., and Nonogaki, H. (2013). Seeds. New York, NY: Springer. doi: 10.1007/978-1-4614-4693-4
Borgen, A. (2004). Organic seed treatment to control common bunt (Tilletia tritici) in wheat. Seed Testing Int. 128, 8–9.
Bouaziz, A., and Bruckler, L. (1989). Modeling of wheat imbibition and germination as influenced by soil physical properties. Soil Sci. Soc. Am. 53, 219–227. doi: 10.2136/sssaj1989.03615995005300010039x
Bundesrat der Schweizerischen Eidgenossenschaft (2017). Aktionsplan zur Risikoreduktion und Nachhaltigen Anwendung von Pflanzenschutzmitteln. Bern: Schweiz.
Carrillo-Reche, J., Vallejo-Marín, M., and Quilliam, R. S. (2018). Quantifying the potential of “on-farm” seed priming to increase crop performance in developing countries. A meta-analysis. Agron. Sustain. Dev. 38:64. doi: 10.1007/s13593-018-0536-0
Courvoisier, N., Levy Häner, L., Bertossa, M., Thévoz, E., Anders, M., Stoll, P., et al. (2016). List of recommended cereals for harvest 2017 [in German]. Agrarforsch. Schweiz 7.
Dhitaphichit, P., and Jones, P. (1991). Virulent and fungicide-tolerant races of loose smut (Ustilago nuda and U. tritici) in Ireland. Plant Pathol. 40, 508–514. doi: 10.1111/j.1365-3059.1991.tb02413.x
Doling, D. A. (1965). Single-bath hot-water treatment for the control of loose smut (Ustilago nuda) in cereals. Ann. Appl. Biol. 55, 295–301. doi: 10.1111/j.1744-7348.1965.tb07944.x
European Commission. (2012). Common Catalogue of Varieties of Agricultural Plant Species. Available Online at: https://ec.europa.eu/food/plant/plant_propagation_material/plant_variety_catalogues_databases_en
Forsberg, G. (2004). Control of cereal seed-borne diseases by hot humid air seed treatment. (Doctoral dissertation). Swedish University of Agricultural Sciences, Uppsala.
Forsberg, G., Johnsson, L., and Lagerholm, J. (2005). Effects of aerated steam seed treatment on cereal seed-borne diseases and crop yield. J. Plant Dis. Prot. 112, 247–256.
Forsberg, G., Kristensen, L., Eibel, P., Titone, P., and Hartl, W. (2003). Sensitivity of cereal seeds to short duration treatment with hot, humid air. J. Plant Dis. Prot. 110, 1–16.
Fox, J., and Weisberg, S. (2019). An R Companion to Applied Regression, 3rd Edn. Thousand Oaks, CA: Sage.
Glynn, N. C., Hare, M. C., Parry, D. W., and Edwards, S. G. (2005). Phylogenetic analysis of EF-1 alpha gene sequences from isolates of Microdochium nivale leads to elevation of varieties majus and nivale to species status. Mycol. Res. 109, 872–880. doi: 10.1017/S0953756205003370
Graves, S., Piepho, H., Selzer, L., and Dorai-Raj, S. (2015). Multcompview: Visualizations of Paired Comparisons, R Package Version 0.1-7. Available online at: https://cran.r-project.org/web/packages/multcompView/index.html
Grondeau, C., Ladonne, F., Fourmond, A., Poutier, F., and Samson, R. (1992). Attempt to eradicate Pseudomonas syringae pv. pisi from pea seeds with heat treatments. Seed Sci. Technol. 20, 515–525.
Grondeau, C., Samson, R., and Sands, D. C. (1994). A review of thermotherapy to free plant materials from pathogens, especially seeds from bacteria. Crit. Rev. Plant Sci. 13, 57–75. doi: 10.1080/07352689409701908
Harris, D. (2006). Development and testing of “on-farm” seed priming. Adv. Agron. 90, 129–178. doi: 10.1016/S0065-2113(06)90004-2
Hökeberg, M., Gerhardson, B., and Johnsson, L. (1997). Biological control of cereal seed-borne diseases by seed bacterization with greenhouse-selected bacteria. Eur. J. Plant Pathol. 103, 25–33. doi: 10.1023/A:1008681608400
International Seed Testing Association (2014). 7-022: Agar Method for the Detection of Microdochium nivale and M. majus on Triticum spp. International Rules For Seed Testing. Bassersdorf: International Seed Testing Association.
Jensen, J. (1888). The propagation and prevention of smut in oats and barley. J. R. Agric. Soc. 24, 397–415.
Johnsson, L., Hökeberg, M., and Gerhardson, B. (1998). Performance of the Pseudomonas chlororaphis biocontrol agent ma 342 against cereal seed-borne diseases in field experiments. Eur. J. Plant Pathol. 104, 701–711. doi: 10.1023/A:1008632102747
Kammoun, L. G., Gargouri, S., Hajlaoui, M. R., and Marrakchi, M. (2009). Occurrence and distribution of Microdochium and Fusarium species isolated from durum wheat in Northern Tunisia and detection of mycotoxins in naturally infested grain. J. Phytopathol. 157, 546–551. doi: 10.1111/j.1439-0434.2008.01522.x
Koch, E., and Roberts, S. J. (2014). “Non-chemical seed treatment in the control of seed-borne pathogens,” in Global Perspectives on the Health of Seeds and Plant Propagation Material, eds M. Gullino and G. Munkvold (Dordrecht: Springer Netherlands), 105–123. doi: 10.1007/978-94-017-9389-6_8
Koch, E., Schmitt, A., Stephan, D., Kromphardt, C., Jahn, M., Krauthausen, H.-J., et al. (2010). Evaluation of non-chemical seed treatment methods for the control of Alternaria dauci and A. radicina on carrot seeds. Eur. J. Plant Pathol. 127, 99–112. doi: 10.1007/s10658-009-9575-3
Krebs, H., Bänziger, I., Legro, R. J., and Vogelgsang, S. (2011). Alternative control of snow mold (Microdochium nivale) in organic wheat [in German]. Agrarforsch. Schweiz 2, 88–95.
Lamichhane, J. R. (2020). Parsimonious use of pesticide-treated seeds: an integrated pest management framework. Trends Plant Sci. 25, 1070–1073. doi: 10.1016/j.tplants.2020.08.002
Lamichhane, J. R., You, M. P., Laudinot, V., Barbetti, M. J., and Aubertot, J.-N. (2020). Revisiting sustainability of fungicide seed treatments for field crops. Plant Dis. 104, 610–623. doi: 10.1094/PDIS-06-19-1157-FE
Leroux, P., and Berthier, G. (1988). Resistance to carboxin and fenfuram in Ustilago nuda (jens.) rostr. The causal agent of barley loose smut. Crop Protect. 7, 16–19. doi: 10.1016/0261-2194(88)90031-2
Mangiafico, S. (2016). Summary and Analysis of Extension Program Evaluation in R, Version 1.18.8. New Brunswick, NJ: Rutgers Cooperative Extension.
Matanguihan, J. B., Murphy, K. M., and Jones, S. S. (2011). Control of common bunt in organic wheat. Plant Dis. 95, 92–103. doi: 10.1094/PDIS-09-10-0620
Matušinsky, P., Svačinová, I., Jonavičiene, A., and Tvaružek, L. (2017). Long-term dynamics of causative agents of stem base diseases in winter wheat and reaction of Czech Oculimacula spp. and Microdochium spp. populations to prochloraz. Eur. J. Plant Pathol. 148, 199–206. doi: 10.1007/s10658-016-1082-8
Menzies, J., McLeod, R., Tosi, L., and Cappelli, C. (2005). Occurrence of a carboxin-resistant strain of Ustilago nuda in Italy. Phytopathol. Mediterr. 44, 216–219. doi: 10.14601/Phytopathol_Mediterr-1796
MeteoSchweiz (2017). Klimareport 2016. Technical report, Bundesamt für Meteorologie und Klimatologie, Zürich, 80 S.
MeteoSchweiz (2018). Klimareport 2017. Technical report, Bundesamt für Meteorologie und Klimatologie, Zürich, 84 S.
MeteoSchweiz (2019). Klimareport 2018. Technical report, Bundesamt für Meteorologie und Klimatologie, Zürich, 94 S.
MeteoSchweiz (2020). Klimareport 2019. Technical report, Bundesamt für Meteorologie und Klimatologie, Zürich, 96 S.
MeteoSchweiz (2021). Klimareport 2020. Technical report, Bundesamt für Meteorologie und Klimatologie, Zürich, 96 S.
Möhring, N., Ingold, K., Kudsk, P., Martin-Laurent, F., Niggli, U., Siegrist, M., et al. (2020). Pathways for advancing pesticide policies. Nat. Food 1, 535–540. doi: 10.1038/s43016-020-00141-4
Musa, A. M., Harris, D., Johansen, C., and Kumar, J. (2001). Short duration chickpea to replace fallow after aman rice: the role of on-farm seed priming in the high barind tract of Bangladesh. Exp. Agric. 37, 509–521. doi: 10.1017/S0014479701000448
Nicolopoulou-Stamati, P., Maipas, S., Kotampasi, C., Stamatis, P., and Hens, L. (2016). Chemical pesticides and human health: the urgent need for a new concept in agriculture. Front. Publ. Health 4:148. doi: 10.3389/fpubh.2016.00148
Nielsen, L. K., Justesen, A. F., Jensen, J. D., and Jørgensen, L. N. (2013). Microdochium nivale and Microdochium majus in seed samples of Danish small grain cereals. Crop Protect. 43, 192–200. doi: 10.1016/j.cropro.2012.09.002
Osman, A., Groot, S., Köhl, J., Kamp, L., and Bremer, E. (2004). “Seed treatments against fusarium in organic spring wheat,” in Prodeedings of the First World Conference on Organic Seed, ed A. Martinez (Bonn: IFOAM), 133–137.
Setter, T. L., and Waters, I. (2003). Review of prospects for germplasm improvement for waterlogging tolerance in wheat, barley and oats. Plant Soil 253, 1–34. doi: 10.1023/A:1024573305997
Sharma, K. K., Singh, U. S., Sharma, P., Kumar, A., and Sharma, L. (2015). Seed treatments for sustainable agriculture–a review. J. Appl. Nat. Sci. 7, 521–539. doi: 10.31018/jans.v7i1.641
Simpson, D., Rezanoor, H., Parry, D., and Nicholson, P. (2000). Evidence for differential host preference in Microdochium nivale var. majus and Microdochium nivale var. nivale. Plant Pathol. 49, 261–268. doi: 10.1046/j.1365-3059.2000.00453.x
Tangney, R., Merritt, D. J., Fontaine, J. B., and Miller, B. P. (2019). Seed moisture content as a primary trait regulating the lethal temperature thresholds of seeds. J. Ecol. 107, 1093–1105. doi: 10.1111/1365-2745.13095
Tinivella, F., Hirata, L., Celan, M., Wright, S., Amein, T., Schmitt, A., et al. (2009). Control of seed-borne pathogens on legumes by microbial and other alternative seed treatments. Eur. J. Plant Pathol. 123, 139–151. doi: 10.1007/s10658-008-9349-3
Tombolini, R., van der Gaag, D. J., Gerhardson, B., and Jansson, J. K. (1999). Colonization pattern of the biocontrol strain Pseudomonas chlororaphis ma 342 on barley seeds visualized by using green fluorescent protein. Appl. Environ. Microbiol. 65, 3674–3680. doi: 10.1128/AEM.65.8.3674-3680.1999
Vannacci, G., Sarrocco, S., and Porta-Puglia, A. (2014). Improved Detection and Monitoring of Seed-Borne Fungal Plant Pathogens in Europe, Vol. 6. Dordrecht: Springer. doi: 10.1007/978-94-017-9389-6_6
Vogelgsang, S., Bänziger, I., Krebs, H., Legro, R. J., Sanchez-Sava, V., and Forrer, H.-R. (2013). Control of Microdochium majus in winter wheat with botanicals–from laboratory to the field. Plant Pathol. 62, 1020–1029. doi: 10.1111/ppa.12024
Wickham, H. (2016). ggplot2: Elegant Graphics for Data Analysis. New York, NY: Springer-Verlag. doi: 10.1007/978-3-319-24277-4
Widén, P., and Annas, P. (2004). “Cedomon® and cerall®” biological seed treatments for cereals,” in The First World Conference on Organic Seed: Challenges and Opportunities for Organic Agriculture and the Seed Industry, eds E. Lammerts van Bueren, R. Ranganathan, and N. Sorensen (Rome: FAO) 169–170.
Winter, W., Bänziger, I., Krebs, H., Rüegger, A., Frei, P., and Gindrat, D. (1996). Warm and hot water treatment of barley seed [in German]. Agrarforsch. Schweiz 3, 25–28.
Winter, W., Bänziger, I., Krebs, H., Rüegger, A., Frei, P., and Gindrat, D. (1997). Water treatments against damping-off diseases of cereals [in German]. Agrarforsch. Schweiz 4, 467–470.
Winter, W., Bänziger, I., Krebs, H., Rüegger, A., Frei, P., and Gindrat, D. (1998b). Alternatives to the chemical dressing against bunts and Helminthosporium diseases of cereal seeds [in German]. Agrarforsch. Schweiz 5, 29–32.
Winter, W., Bänziger, I., Rüegger, A, and Krebs, H. (1998a). Warm water seed-treatment realised in the practice for the control of the seed-borne snow mould in summer-wheat [in German]. Agrarforsch. Schweiz 5, 125–128.
Winter, W., Bänziger, I., Rüegger, A., Krebs, H., Frei, P., and Gindrat, D. (1994). Warm water treatment of wheat seeds [in German]. Agrarforsch. Schweiz 1, 492–495.
Winter, W., Bänziger, I., Rüegger, A., Schachermayr, G., and Krebs, H. (2001). Skim milk powder and yellow mustard flour against common bunt of wheat [in German]. Agrarforsch. Schweiz 8, 118–123.
Keywords: alternative seed treatments, seed-borne diseases, Ustilago nuda, loose smut, Microdochium spp., snow mold, organic, cereal
Citation: Bänziger I, Kägi A, Vogelgsang S, Klaus S, Hebeisen T, Büttner-Mainik A and Sullam KE (2022) Comparison of Thermal Seed Treatments to Control Snow Mold in Wheat and Loose Smut of Barley. Front. Agron. 3:775243. doi: 10.3389/fagro.2021.775243
Received: 13 September 2021; Accepted: 30 December 2021;
Published: 17 February 2022.
Edited by:
Nacer Bellaloui, United States Department of Agriculture, United StatesReviewed by:
Fernanda Maria Gamba, Universidad de la República, UruguayLinda Yuya Gorim, University of Alberta, Canada
Copyright © 2022 Bänziger, Kägi, Vogelgsang, Klaus, Hebeisen, Büttner-Mainik and Sullam. This is an open-access article distributed under the terms of the Creative Commons Attribution License (CC BY). The use, distribution or reproduction in other forums is permitted, provided the original author(s) and the copyright owner(s) are credited and that the original publication in this journal is cited, in accordance with accepted academic practice. No use, distribution or reproduction is permitted which does not comply with these terms.
*Correspondence: Karen E. Sullam, a2FyZW4uc3VsbGFtJiN4MDAwNDA7YWdyb3Njb3BlLmFkbWluLmNo
†Present address: Irene Bänziger, Andreas Kägi, and Susanne Vogelgsang, Research Group Extension Arable Crops, Agroscope, Zurich, Switzerland
Karen E. Sullam, Research Group Molecular Ecology, Agroscope, Zurich, Switzerland