- 1Département de Production végétale, Faculté d'Agronomie, Université de Parakou, Parakou, Benin
- 2Laboratory of Hydraulics and Environmental Modeling (HydroModE Lab), Faculté d'Agronomie (FA), Université de Parakou (UP), Parakou, Benin
- 3International Maize and Wheat Improvement Center (CIMMYT), International Center for Research in Agroforestry (ICRAF) House, Nairobi, Kenya
In sub-Saharan Africa, smallholder farming systems continue to record very low yields despite the availability of appropriate crop management technologies. A 2-year field experiment was conducted in 2018 and 2019 at the Agricultural Research Station of Northern Benin to evaluate the growth response, grain yield attributes, and nutrient- and water- use efficiencies of different drought-tolerant (DT) maize varieties (TZEE-W-POP-STR QPM, TZE-Y-POP-STR, 2008Syn-EE-W DT-STR, and 2000Syn-EE-W of 80–90 days cycle), compared to DMR-ESR-W, a drought-susceptible (DS) variety of 90 days cycle, under different fertilizer options [no fertilizer control, microdosing option 1 (MD1, 25 kg N ha−1 + 4 kg P ha−1), option 2 (MD2, 25 kg N ha−1 + 8 kg P ha−1), option 3 (MD3, 35 kg N ha−1 + 8 kg P ha−1), and broadcast fertilizer at recommended rate (RR, 76 kg N ha−1 + 13 kg P ha−1). Generally, combining microdosing with DT varieties showed a very good performance by increasing the leaf area and biomass by 71 and 85%, respectively, at anthesis compared to the conventional practice. Microdosing increases grain and stover yields by 171 and 98%, respectively, compared to an unfertilized control with generally no difference between MD3 and RR treatments. Also, the DT varieties obtained the best yields (+19%), with the highest value observed with the TZE-Y variety. Significant interaction was found between varieties and fertilization for grain and stover yields. During the two cropping seasons, microdose fertilization increases the DT grain yield and gross return by 658 kg ha−1 and 203$USD, respectively, than their application on drought sensitive variety. The results of the current study demonstrated that there is a considerable potential for smallholder farmers of northern Benin to improve the maize productivity by means of DT varieties and of fertilizer microdosing. Using, especially, the MD3 option on the DT variety, TZE-Y, may be the best combination in optimizing the maize production in the sub-humid region of Benin, instead of the climate sensitive variety of the DMR-ESR under the microdosing or a common fertilization practice. However, further testing of the technologies through on-farm experiments are required before a precise advice can be given. Precisely, there is a need to evaluate these management options in farmer's fields, taking into account the climatic, soil, and management conditions to better assess and understand the variation in response and in economic benefits and risk.
Introduction
Maize plays an important role in food security and in the rural economy of Sub-Saharan Africa (SSA). However, its production in SSA is drastically affected by several constraints such as low inherent and decreasing soil fertility, poor crop and farm management practices (choice of crop varieties, planting, fertilization, etc.), recurrent droughts exacerbated by climate change, and socioeconomic conditions (Traore et al., 2013; Vanlauwe et al., 2014; Barrett and Bevis, 2015). Improvement of maize production can be achieved through appropriate management techniques to reduce the yield gap due to environmental constraints and socioeconomic conditions of the farms of the smallholders. The development of new agricultural techniques in SSA that are specific to socio-economic conditions of the farmers requires not only drought resistant crops, but also more resilient methods that can reduce the vulnerability of crops to drought, improve their efficiency of the nutrient use, and their economic profitability to ensure food security.
More than 160 drought-tolerant maize varieties, hereafter DT, have been implemented in 13 African countries over the last decades through the DT Maize for Africa (DTMA) program in collaboration with International Maize and Wheat Improvement Center (CIMMYT), International Institute of Tropical Agriculture (IITA), and African Agricultural Research Centers (Beyene et al., 2013; Fisher et al., 2015; Setimela et al., 2017; Makumbi et al., 2018). These high numbers of DT maize varieties led to various growing cycle crops including extra early, early, intermediaries, and late ones (Yallou et al., 2010; Badu-Apraku et al., 2011a,b; Baco, 2019). The use of such improved maize varieties can help farmers to produce maize during the drought periods, especially when the drought occurs at the crucial crop development stages such as the flowering and grain filling stages. Their climatic endurance, highest yield, and grain quality were the strong points of their adoption (Yeboah et al., 2019). Varieties of DT maize are expected to improve the resilience of maize-based farming systems in Benin by enhancing food security, nutrition and farmer's income, and reducing poverty (Makate et al., 2017; Wossen et al., 2017; Abdoulaye et al., 2018; Lunduka et al., 2019). Varieties of DT can produce more than 30% of their potential yield after severe drought, before and during flowering, and grain-filling stages (La Rovere et al., 2010). Moreover, these varieties have some interesting additional features including resistance to major diseases, high efficiency of resource used, high protein content, etc., that can encourage their use by smallholder farmers (Fisher et al., 2015). The DT maize varieties have similar labor requirements and seed costs with those of traditional and non-DT varieties, but they perform better than the latter under drought and rain-fed conditions (Cooper et al., 2013; Holden and Fisher, 2015). Therefore, the DT varieties have been adopted by 85% of the farmers in the different agro-ecological zones of Benin (Arouna and Arodokoun, 2013), mainly because of their potential to improve the economic performance of smallholder farmers.
Drought-tolerant maize varieties are usually produced in smallholder maize farming systems to increase crops yields by using a combination of fertilizers. For instance, previous research across SSA countries have found a better yield of DT maize varieties compared to local varieties for different levels of fertilizers, and various soil fertility and rainfall conditions (La Rovere et al., 2010; Kamara et al., 2014; Sanou et al., 2016; Simtowe et al., 2019). However, the use of fertilizers by smallholder farmers of the SSA is limited by their socioeconomic conditions including inaccessibility to farm inputs and credit (high cost, lack of credit, and poor transport and marketing infrastructure), and poor agricultural management such as inappropriate applications: right source, rate, time, and placement (Waithaka et al., 2007; Boniphace et al., 2015; Mucheru-Muna et al., 2021). Thus, fertilizer microdosing has been widely promoted in the SSA region (Buerkert et al., 2001). This technique consists of localized application of small quantities of mineral fertilizer together with the seed at sowing or shortly after planting, instead of evenly broadcasting the fertilizers across the field with or without the subsequent incorporation as commonly as most farmers do. Fertilizer microdosing is an effective technique that helps to improve the efficiency of nutrient and water use (Ibrahim et al., 2015; Tovihoudji et al., 2017), to crop yield (Buerkert et al., 2001; Aune et al., 2007; Camara et al., 2013; Sime and Aune, 2014; Okebalama et al., 2016; Tovihoudji et al., 2018), and to reduce the investment costs and financial risk for smallholder farmers (Twomlow et al., 2010; Tovihoudji et al., 2019). Therefore, fertilizer microdosing would enhance the yield of DT maize varieties compared to local varieties, inducing better food security and higher net returns for the benefit of smallholder farmers. Several studies show a high potential of maize grain production under microdose fertilization in Africa (Twomlow et al., 2010; Sime and Aune, 2014), including northern Benin Tovihoudji et al., 2017, 2019. However, we still lack knowledge of the performance and nutrient- and water- use efficiencies of the combined DT maize varieties and of the fertilizer microdosing in the Sub-humid region of West-Africa. This study sets to fill the gap and to expand our knowledge to understand the impact of fertilizer microdosing on the productivity of various DT maize varieties, as well as to help farmers optimize the use of fertilizers.
This study primarily aims to evaluate the growth, biomass accumulation, and yield attributes under the combined DT varieties and fertilizer microdosing. Moreover, we investigated the effect of various DT maize varieties and fertilizer microdosing combination on nutrient and rainwater use efficiency and economic profitability. The findings from this research will improve our understanding on the additive or synergistic effects of combined DT maize varieties and fertilizer microdosing. Moreover, this study will provide information for a better DT maize crop management, for the use of fertilizers through microdosing in smallholders' farms, and for a better maize yield in the drought environments of the SSA countries.
Materials and Methods
Experimental Site
The present study was conducted in north-eastern Benin (in the department of Borgou) in agro-ecological zone 3 (Figure 1).
The experiments were conducted at the Agricultural Research Station of Northern Benin (CRA- Nord) located at Ina village (Ina district, municipality of Bembèrèkè) (9° 57′N and 2° 43′E, and altitude 382 m). The annual rainfall ranges between 900 and 1,200 mm with an average of 1,148 ± 184 mm. The average daily temperature is 27.5°C. The climate is characterized by a single rainy season from May to October. The soil is ferruginous tropical in the French soil classification system with low inherent fertility, which corresponds to Lixisols according to the World Reference Base (Youssouf and Lawani, 2002). The soil is acidic at all depths (pH <7) with a loam-sandy texture in the topsoil (0–40 cm) (Table 1), and poor in nitrogen (<750 mg kg−1) and phosphorus (<18 mg kg−1). The level of exchangeable K is medium (>0.10 cmol kg−1) at all depths. The rate of organic carbon in the soil is low (<0.45%).
Experimental Design, Trial Installation, and Management
The experimental design was a randomized complete block with a split-plot arrangement in three replicates. The main plots were subjected to five fertilizer rates, and five maize varieties were tested in the sub-plots. The main plots were 29 m wide × 5 m long, and the subplots were 4 m wide × 5 m long. Five mineral fertilizer options were tested: (i) a control (no fertilizer); fertilizer microdosing at a rate of (ii) 25 kg N + 4 kg P ha−1 (MD1), (iii) 25 kg N + 8 kg P ha−1 (MD2), (iv) 35 kg N + 8 kg P ha−1 (MD3), and (v) the recommended rate at 76 kg N ha−1 + 13 kg P ha−1 (RR). The K was applied at the recommended rate of 25 kg ha−1 to all plots. Full dose of P and K was provided as triple superphosphate (46% P2O5) and muriate of potash (60% K2O) at plant emergence (10 days after sowing). Half of urea-N was applied as basal at plant emergence and the remaining quantity of N was applied before the flowering stage (45 days after sowing). Fertilizer microdosing and the RR treatments were applied according to Tovihoudji et al. (2017). Four DT maize cultivars (TZEE-W POP-STR-QPM, TZE-Y-POP-STR, 2008Syn EE-W-DT-STR, and 2000Syn-EE-W with 80–90 days cycle) were compared to a drought-susceptible (DS) control cultivar (DMR-ESR-W of 90 days cycle).
Land preparation was uniformly done across all plots by tractor disk-plowing to a depth of 0.2 m for the 2 years. At planting, the experimental plots were leveled manually using rakes and were sown after the first rainfall event that is greater than 20 mm on June 6, 2018 and June 9, 2019. The planting hills were spaced by 0.8 × 0.4 m with a total of six rows each comprising of 13 pockets. Two weeks after sowing, the maize seedlings were thinned to two plants per hill to attain a plant population of 62,500 plant ha−1 (currently recommended density). The plots were weeded twice (15 and 30 days after sowing, DAS) and ridged at 45 DAS immediately after the urea application with a hand hoe. Harvesting took place on September 22, 2018 and October 2, 2019.
Agronomic and Physiological Measurements and Calculations
Agronomic and Physiological Measurements
Daily rainfall data were recorded each year with a rain gauge located at the experimental field. Plant growth characteristics including plant height, leaf area index (LAI), and above-ground biomass were measured at two-week intervals from 20 DAS until 90 DAS. In each, plot five plants from three inner rows were randomly selected and were tagged for measurements of plant height and leaf area. Individual leaf area was estimated non-destructively from the leaf length (l, cm) and from maximum leaf width (w, cm) measured with a ruler on green leaves on three plants in the net plot. Dry biomass of three whole plants was measured by the destructive method and samples were initially oven-dried at 105°C for 30 min after further drying at 70°C until constant weight and expressed in g plant–1.
Harvest was done on three inner rows of each plot for yield determination. In all plots, two neighboring plants in each row were left as a buffer zone to reduce the edge and/or the neighbor effects. The cobs and stover (stems and leaves + husks + central axis) were weighed in the field. The grain moisture content was then determined for each replication after oven-drying at 70°C to a constant weight (after an initial oven-drying at 105°C for 30 min). Maize stover subsamples were taken to the laboratory for further drying (oven-drying at 70°C to constant weight) and for moisture correction. The number of plants and the number of maize cobs per plant were counted. The thousand grains weight (TGW) were estimated by counting and weighing five replicates of 100 randomly sampled grains per plot. The number of grains per cob was calculated by dividing the weight of grains per cob by the average weight of one grain. The harvest index (HI) was calculated by dividing the dry weight of grains by the total dry biomass at harvest. Maize grain and stover yields were then calculated and expressed in kg ha−1 on a dry weight basis.
Calculations
Leaf area (LA) was calculated from the formula used by Abbasi et al. (2013) as follows: LA = leaf length × maximum width × k, where k is a shape factor with a value of 0.75. LAI was obtained by the ratio of total leaf area to per unit ground area.
Post-anthesis DM accumulation (DMa) was estimated as the total DM at harvest minus that of the anthesis. Translocation of DM, accumulated before anthesis (TrDM), was estimated from DM at flowering minus the accumulated non-grain DM at harvest. The DM translocation efficiency (DME, %) was calculated as the translocation of DM accumulated before anthesis (TrDM) divided by total DM at harvest.
Yield increases were calculated using the following equation:
where Yt = yield treatment t (MD1; MD2, MD3, and RR) (kg ha−1) and Yc = yield mean of the control (kg ha−1).
The agronomic efficiency (AE-X) of each nutrient X (X = N or P) was calculated as a proxy of the nutrient use efficiency as follows: AE-X = (Yt – Yc) / Ft, where Yt and Yc are the yields per hectare of the treatment considered and of the control, respectively; The Ft is the quantity of nutrients provided per hectare for this treatment.
Also, the rainwater use efficiency (RUE) was determined by the ratio between the grain yield and the total amount of rainfall during the experiment.
Economic Analysis
Economic profitability of the different treatments was analyzed based on gross margin, gross return, benefit/cost ratio (BCR), and value-cost ratio (VCR). Fixed costs included the cost of seeds and all major labor charges (field preparation, seeding, weeding, ridging, harvesting, and threshing), whereas variable costs included the cost of fertilizers and the application of the different fertilizer. Input and output prices were taken as the average market price of the two cropping seasons (2018–2019; Table 2). The price of seeds and fertilizers fixed by the Beninese government through the Agricultural Research Station of Northern Benin (CRA- Nord) were used. Labor costs for land preparation, planting, manure/fertilizer application, weeding, and harvesting were collected during the experiments. The costs required for digging the hole and the labor costs involved in its application were summed and were used as labor costs of hill application of mineral fertilization (Table 2). For the maize grain price, we used the average official price for the past five years (Bulletin économique sur le marché des céréales en Afrique, https://roac-wagn.blogspot.fr). The maximum price was 175 FCFA kg−1 and the minimum price was 125 FCFA kg−1, with an average price of 150 FCFA kg−1 (1 US$ = 570 FCFA). Total revenue was calculated by multiplying grain yield with the average grain unit price. The gross margin (GM) was calculated by subtracting variable costs from total revenue. The gross return was also calculated by subtracting the sum of the fixed and the variable costs from the revenue. The benefit/cost ratio (BCR) was obtained by dividing the gross return by the total cost of cultivation (fixed and variable costs). The value-cost ratio (VCR) was computed as the difference in grain yield between the fertilized plots and the control plot multiplied by the unit market price of grain, divided by the cost of applied fertilizer.
Statistical Analysis
The collected data were first checked for normality and homogeneity of error variances. Then, we computed an ANOVA using a linear mixed-model. Fixed effects entered into the model included variety and fertilizer and the year of the variety/fertilizer interaction. Cropping years and replicates were included as random factors. Secondly, when there was a significant year × variety × fertilizer interaction effect for a data, we separately fitted a model for each year (2018 and 2019), where variety, fertilizer, and variety × fertilizer were considered as fixed factors and replicates within variety as a random factor. Mean separations were performed using the honestly significant difference (HSD)/Tukey's test at an error probability < 0.05. The relationship between maize grain yield and the other measured variables was evaluated by a two-sided Pearson correlation analysis over years and replicates. The significance of correlations was done for each variety for easier interpretation and comparison. All analyses were done with GenStat Release 12.1 statistical software (Lawes Agricultural Trust, 2009).
Results
Rainfall Distribution During the Cropping Periods
Forty-two (42) and thirty-six (36) rainfall events fall on the experimental site in 2018 and 2019, respectively. The rain amounts were 750 mm in 2018 and 650 mm in 2019 (Figure 2). The rainfall was more evenly distributed in 2018 than in 2019. The total rainfall at vegetative stages, flowering time, and filling to maturity stages were 29, 68, and 19, respectively, in 2018; while 37, 36, and 27%, respectively, in 2019. The dry spells were frequent (four times) and long (10 days) in 2019, and occurred during the critical phase of maize growth (between the 30 and 65 DAS).
Plant Height (Ph), Leaf Area Index (LAI), and Total Dry Matter (TDM)
The plant height, LAI, and TDM differ between the cropping years (Figures 3A,B; Supplementary Table 1) except LAI at 48 DAS and TDM at 62 DAS. For all paramaters, the highest values are recorded in 2018. Likewise, each of these three variables was significantly different between maize varieties (p < 0.001) and between fertilizer treatments (p < 0.001). The Ph values were 42.6, 44.8, 43.1, and 26.6% higher for TZEE-W, TZE-Y, 2008Syn-EE, and 2000Syn-EE varieties, respectively, compared to the DMR variety; and 55.2, 83.1, 151, and 121.5% higher for the MD1, MD2, MD3, and RR microdosing treatments, respectively, compared to the control treatment.
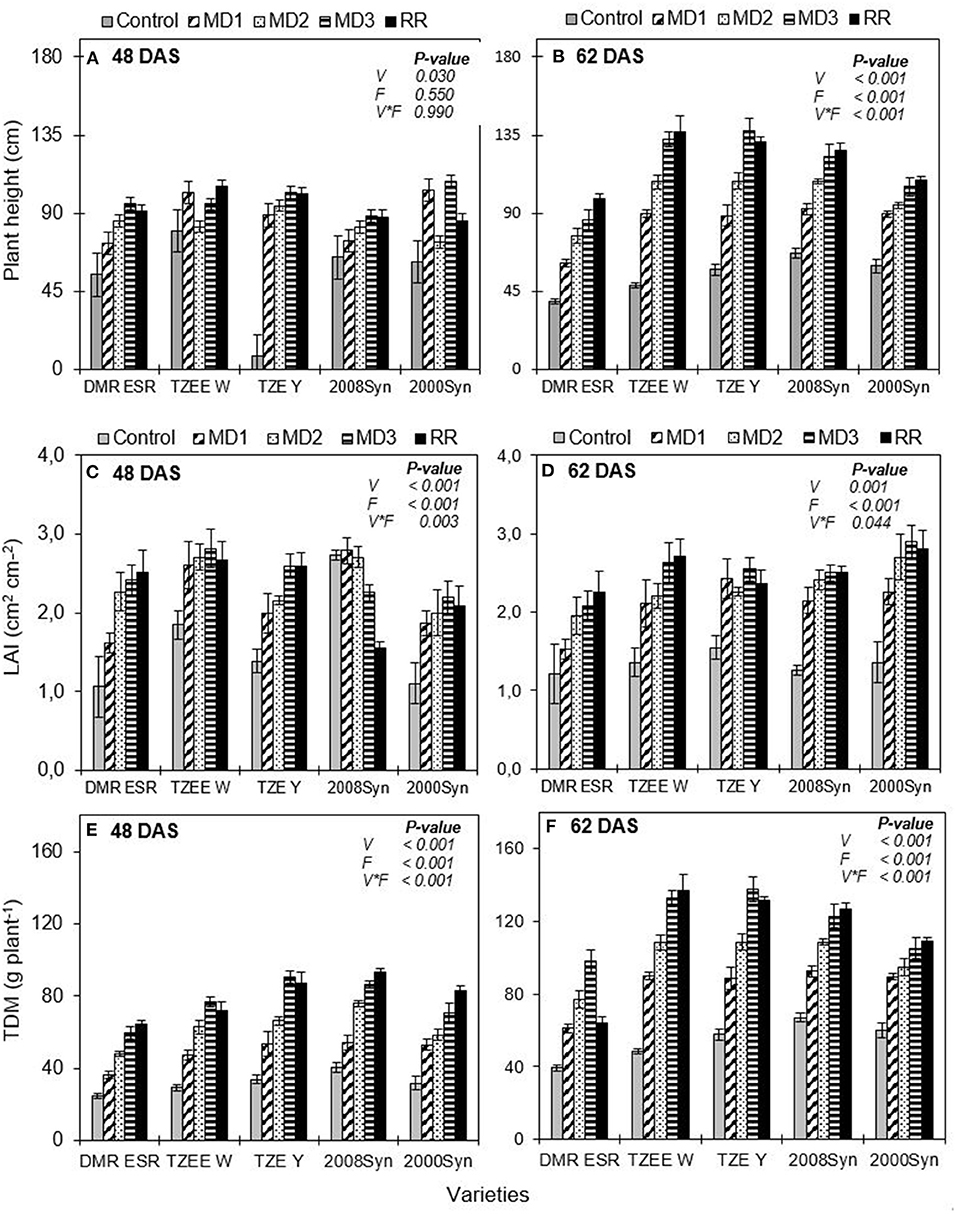
Figure 3. Plant height (A,B), leaf area index [LAI, (C,D)], and total dry matter [TDM, (E,F)] at 48 (A,C,E) and 62 DAS (B,D,F) as affected by the interactions between varieties and mineral fertilizer. Control refers to the absolute unfertilized treatment; MD1 (N25P4), MD2 (N25P8), MD3 (N35P8), and RR (N76P13) refer to fertilizer microdosing options 1, 2, and 3 and the recommended fertilizer rate, respectively. ns, non-significant; Error bars represent standard deviations. V, Variety; F, Fertilizer.
Generally, the LAI peaked at the flowering stage in the two experimental years, increased from the juvenile stage to the flowering stage and then gradual decrease until the maturity stage (Supplementary Figure 1). The LAI of maize plants reached their maximum values (LAImax) at 48 DAS irrespective of the years, varieties, and treatments; except for 2000Syn variety, where the LAImax was found at 62 DAS in 2019 (Supplementary Figure 1). The LAImax was significantly higher for DT varieties (TZEE-W, TZE-Y, 2008Syn-EE, and 2000Syn-EE) compared to the DS variety (DMR-ESR-W) for both measurement dates (Table 3). Growing DT varieties with fertilizer microdosing significantly raised the LAImax at 48 DAS by 33, 45, and 51% for MD1, MD2 and MD3, respectively, compared to the conventional practice (DMR variety + unfertilized control); while the LAImax at 62 DAS increased by 55, 71, and 87% compared to the conventional practice (Figures 3C,D; Supplementary Table 1).
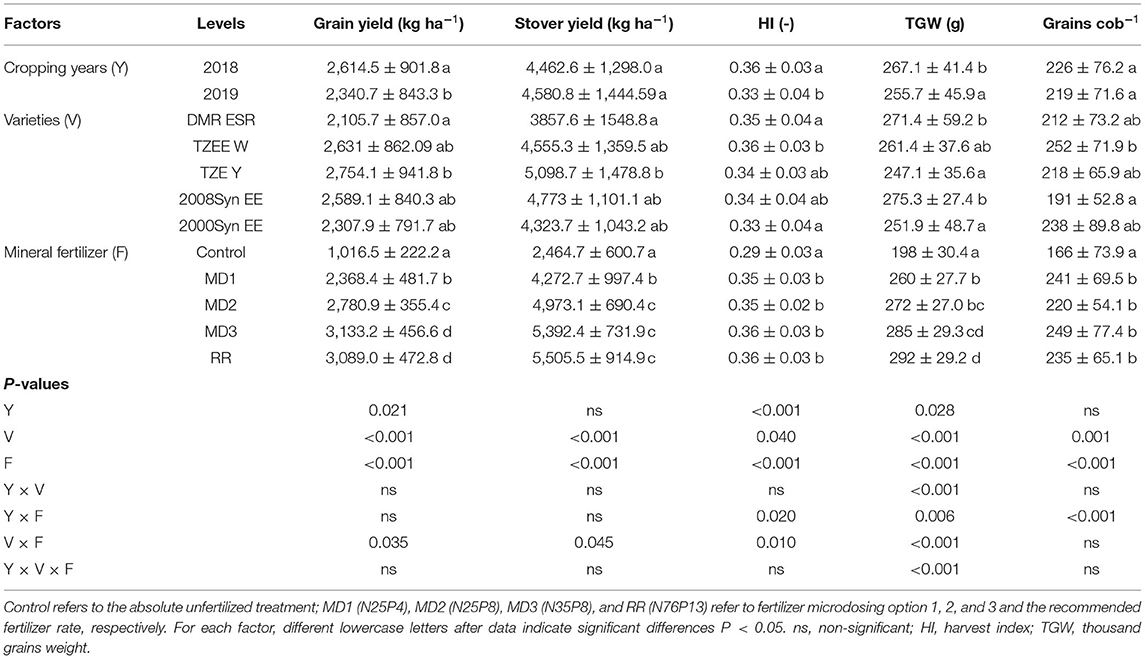
Table 3. Grain yield and components (mean ± standard error) as affected by the cropping years, varieties and mineral fertilizer.
Variety and fertilization significantly affected TDM during the growing period (P < 0.001; Figures 3E,F; Supplementary Figure 2). However, we mainly focused on data from the flowering stage (48 and 62 DAS) across the two years to evaluate the potential impacts of maize varieties and fertilizers treatments on TDM (Supplementary Table 1). The TDM was higher for the TZE Y variety (104.7 g plant−1) compared to DMR-ESRW (72.3 g plant−1). Generally, the TDM of DT varieties increased by 47.6–80.5% at 62 DAS compared to their corresponding TDM at 48 DAS; but raised by 96.3–124.6% compared to DMR-ESRW variety at 48 DAS. Considering the fertilizer treatments, the TDM at 48 DAS increased by 53, 95, 140, and 145% for MD1, MD2, MD3, and RR, respectively; and those at 62 DAS by 55, 83, 115, and 122% for MD1, MD2, MD3, and RR, respectively, compared to the unfertilized control. A significant interaction occurred between varieties and fertilization at both 48 and 62 DAS (Figures 3E,F; p = 0.001). As a result, the combined use of DT varieties and of fertilizer microdosing significantly increased the TDM by 52, 94, 140, and 149% at 48 DAS, and 55, 82, 119, and 108% at 62 DAS for MD1, MD2, and MD3, respectively, compared to the conventional practice (24.8 and 39.3 g plant−1 at 48 and 62 DAS, respectively) (Figures 3E,F). The TDM of RR treatment was not different from that of MD3, but was higher than those of MD1 and MD2 (Figures 3E,F).
Three physiological parameters concerning TrDM, DMtE, and DMa were estimated for the maize varieties, years, and fertilizer treatments (Supplementary Table 2; Figure 4). All of them (TrDM, DMtE, and DMa) were significantly different between maize varieties, and were affected by mineral fertilization (Figure 4). The average TrDM of the four DT varieties was 74% higher than the DMR variety. However, the high value of TrDM and DMtE were observed in a recommended rate (RR) and those of DMa were found for MD2 treatment. Overall, TrDM and DMa of the microdosing treatments significantly increased by 52 and 160%, respectively, compared to the unfertilized control treatment; and TrDM and DMa of the RR treatment raised by 127 and 233%, respectively, compared to the unfertilized control. The DMtE oppositely decreased by 32% for microdosing fertilization, and by10% for RR treatment compared to the unfertilized control (Supplementary Table 2; Figure 4). The interaction variety-fertilizer was significant for each of the three estimated parameters (P < 0.05, Supplementary Table 2). The TrDM was higher under the DT varieties (694–2,810 kg ha−1) than the DS variety DMR (721–1,011 kg ha−1) for the microdosing treatments (Figure 4A). The results showed that fertilizer microdosing was more efficient in terms of dry matter translocation on DT varieties (Figure 4B). Generally, the TrDM and DMtE have an overall increase with the increasing rate of fertilizer microdosing on DT varieties (i.e., from MD1 to MD3) (Figures 4B,C). Nonetheless, the DMa was lower for DT varieties (492–2,143 kg ha−1) than for DMR variety (823–2,099 kg ha−1) under the microdosing treatments. The DMa decreased with the fertilizer rate under the DT varieties but the opposite was observed under the DS variety DMR (Figure 4C).
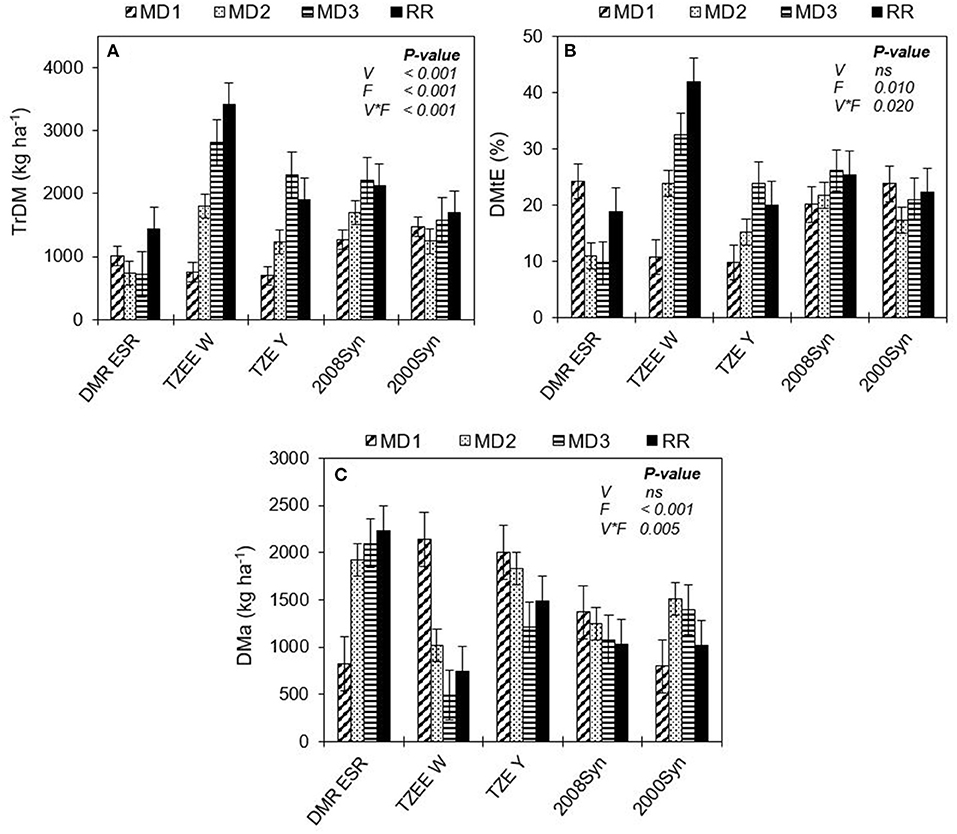
Figure 4. (A) Tanslocation of DM accumulated before anthesis (TrDM), (B) the DM translocation efficiency (DMtE), and (C) the post-anthesis DM accumulation (DMA) as affected by the interactions between varieties and mineral fertilizer. Control refers to the absolute unfertilized treatment; MD1 (N25P4), MD2 (N25P8), MD3 (N35P8), and RR (N76P13) refer to fertilizer microdosing options 1, 2, and 3 and the recommended fertilizer rate, respectively. Error bars represent standard deviations. V, Variety; F, Fertilizer.
Maize Grain and Stover Yields
There were significant effects of year, varieties, and fertilizers on grain yield. Interactions were also found between varieties and fertilizers (Table 3). More grain was produced in 2018 than in 2019. The DT varieties have significantly improved grain yields on average by 19% compared to the DT variety (DMR-ESR) across years (Table 3; P < 0.01). Average grain yields increased in the following order: DMR-ESR = 2000Syn-EE ~ TZEE-W < TZE-Y <2008Syn-EE. Fertilization has significantly increased the grain yields compared to the control across years (Table 3; P < 0.01). Grain yields tended to be higher with the RR treatment than with the microdosing treatments, with no significant difference between MD3 and RR. Microdose treatments have significantly increased the grain yield by 132, 173, and 208%, respectively, for MD1, MD2, and MD3, compared to the control (1,017 kg ha−1). There was a significant variety by fertilizer interaction (P = 0.035; Table 3). Microdose fertilization performs better under the sensitive variety (DMR-ESR; an increase of 183% compared to the control against an increase of 169%) under the DT varieties (Figure 5A).
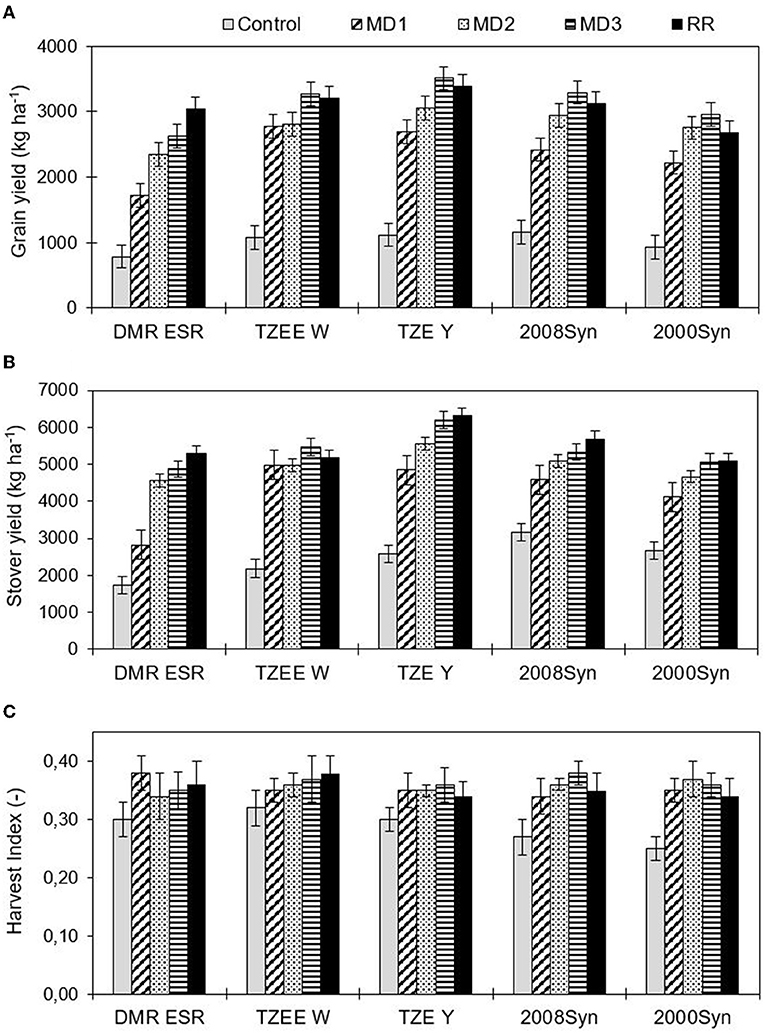
Figure 5. Grain (A), stover yield (B), and harvest index (C) as affected by the interactions between varieties and mineral fertilizer. Control refers to the absolute unfertilized treatment; MD1 (N25P4), MD2 (N25P8), MD3 (N35P8), and RR (N76P13) refer to fertilizer microdosing options 1, 2, and 3 and the recommended fertilizer rate, respectively. Error bars represent standard deviations.
As for the grain, there was a significant effect of variety and of fertilizer on stover yield with the same trend (p < 0.05; Table 3). The stover yields of the DMR variety was 3,857.6 kg ha−1, while those of the DT varieties ranged from 4,323.3 to 5,098.7 kg ha−1 (increase ranged from 120 to 322%, compared to the DMR-ESR variety) (Table 3). On average, stover yields was improved by 1,808 kg ha−1 (73%), 2,508 kg ha−1 (101%), and 2,927 kg ha−1 (119%), respectively, for MD1, MD2, and MD3. In general, yields in RR were similar to MD2 and MD3, and greater than MD1 for most varieties and for both years (Table 3). There was a significant variety by fertilizer interaction. Under the TZEE-W and 2008Syn-EE varieties, there was no difference in stover yields between all fertilized treatments, while stover yield in RR was always similar to MD2 and MD2, and >MD1 under the other three varieties (Figure 5B).
Yield Components
The harvest index (HI) was significantly different across growing seasons, with mean yields larger in 2018 compared to 2019 (P < 0.001; Table 3). Mineral fertilization had a significant effect on the HI, with the lowest values observed in the unfertilized control (Table 3). There was a slight significant variety by fertilizer interaction (P = 0.040; Table 3; Figure 5C).
Thousand grain weight (TGW) was affected by fertilizer, variety × fertilizer, and year × variety × fertilizer interactions (P < 0.001; Table 3; Figure 6). The MD2 (272 g) and MD3 (285 g) treatments were found to have a similar TGW as the RR treatment (292 g), but significantly higher than the unfertilized control (198 g). In 2018, TGW responded very well to MD1 for all DT varieties with average increase ranging from 24 to 51%, compared to the unfertilized control with DMR variety (195 g). For varieties DMR-ESR, 2008Syn-EE, and 2000Syn-EE, the TGW increase with fertilizer rates except for TZEE-W and TZE-Y varieties, where TGW respond very well under MD1 (Figure 6).
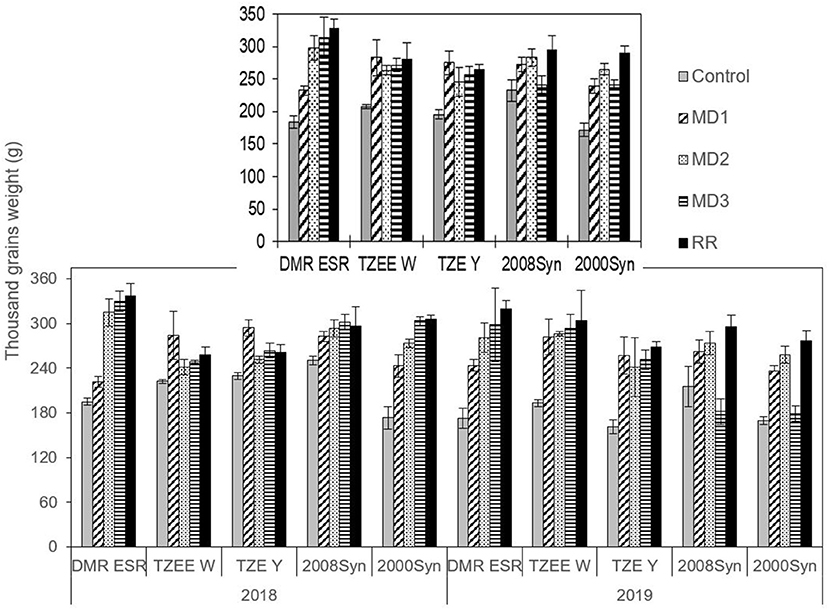
Figure 6. Thousand grains weight (TGW) as affected by the interactions between year, variety, and mineral fertilizer. Control refers to the absolute unfertilized treatment; MD1 (N25P4), MD2 (N25P8), MD3 (N35P8), and RR (N76P13) refer to fertilizer microdosing options 1, 2, and 3 and the recommended fertilizer rate, respectively. Error bars represent standard deviations. HI, harvest index; TGW, thousand grains weight.
The number of grains per cob was significantly affected by variety and fertilization (Table 3; P < 0.001) with the highest values observed with the varieties TZEE-W (252 grains cob−1) and 2000Syn-EE (238 grains cob−1). Mineral fertilization had a significant effect on the number of grains per cob, with the lowest values in the unfertilized control (Table 3). Compared to the unfertilized treatment, fertilizer application significantly increased the number of grains per cob by 32, 45, 50, and 41% for MD1, MD2, MD3, and RR, respectively.
Agronomic Efficiency and Rainfall Use Efficiency
The N and P use efficiencies (AE-N and AE-P) were influenced by varieties and by fertilizer application (Table 4). The DT varieties resulted in the highest AE-N and AE-P across years, ranging from 51.3 to 59.7 kg grain kg−1 N and 234.3 to 341.1 kg grain kg−1 P, respectively, whereas the DS variety had AE-N and AE-P of 45.5 kg grain kg−1 N, and 208.1 kg grain kg−1 P, respectively (Table 4). Microdosing treatments resulted in the highest AE-N and AE-P across years, ranging from 54.5 to 60.8 kg grain kg−1 N, and 222.1 to 341.1 kg grain kg−1 P, respectively, whereas the RR treatment had AE-N and AE-P of 27 kg grain kg−1 N, and 160.4 kg grain kg−1 P, respectively (Table 4). Any variety by fertilizer interaction was found (Table 4).
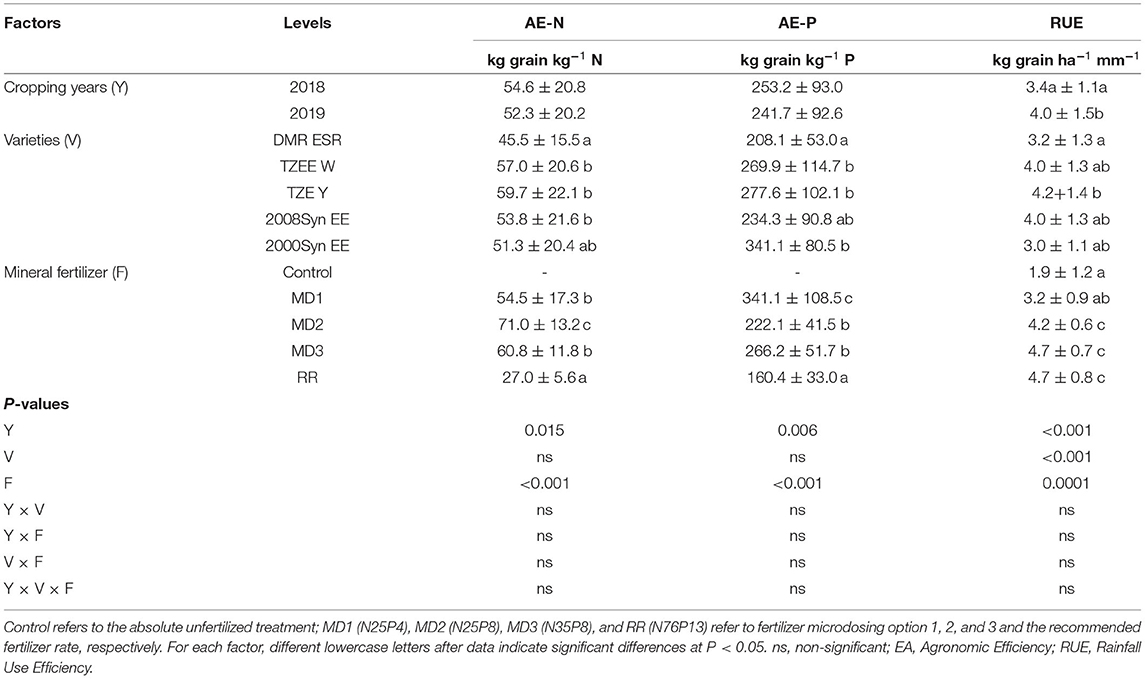
Table 4. Agronomic efficiency of N (AE-N) and P (AE-P) and rainfall use efficiency (RUE) (mean ± standard error) as affected by the cropping years, varieties, and mineral fertilizer.
Unlike AEs, the rainwater use efficiency (RUE) was significantly higher in 2019 than in 2018 as a result of the high differences in rainfall amount between the 2 years (Table 4; P < 0.001). Likewise, RUEs were significantly affected by variety and fertilization (Table 4; P < 0.05). Such DT varieties resulted in the highest RUEs, ranging from 3 of 4.2 kg grain ha−1 mm−1, whereas the DMR variety had RUEs ranging from 3 of 4.2 kg grain ha−1 mm−1 (Table 4). As a result of the similar grain yields produced with RR treatment, microdosing treatments resulted in the similar RUEs with RR treatment, ranging from 3.3 to 4.2 kg grain ha−1 mm−1. Like AEs, interaction was found (Table 4).
Economic Performance Indicators
The economic analysis based on average costs of inputs and outputs (Table 5) revealed a larger benefit for DT varieties compared to DS variety. Overall, the partial gross margin, the gross return, the VCR, and BCR were significantly higher on DT varieties than on DS variety. For both years, DT varieties have increased the GM and GR by 137 USD (67%) and 156 USD (85%), respectively, compared to DS variety (DMR ESR). The VCR and BCR were increased by 57 and 85% compared to the DS variety.
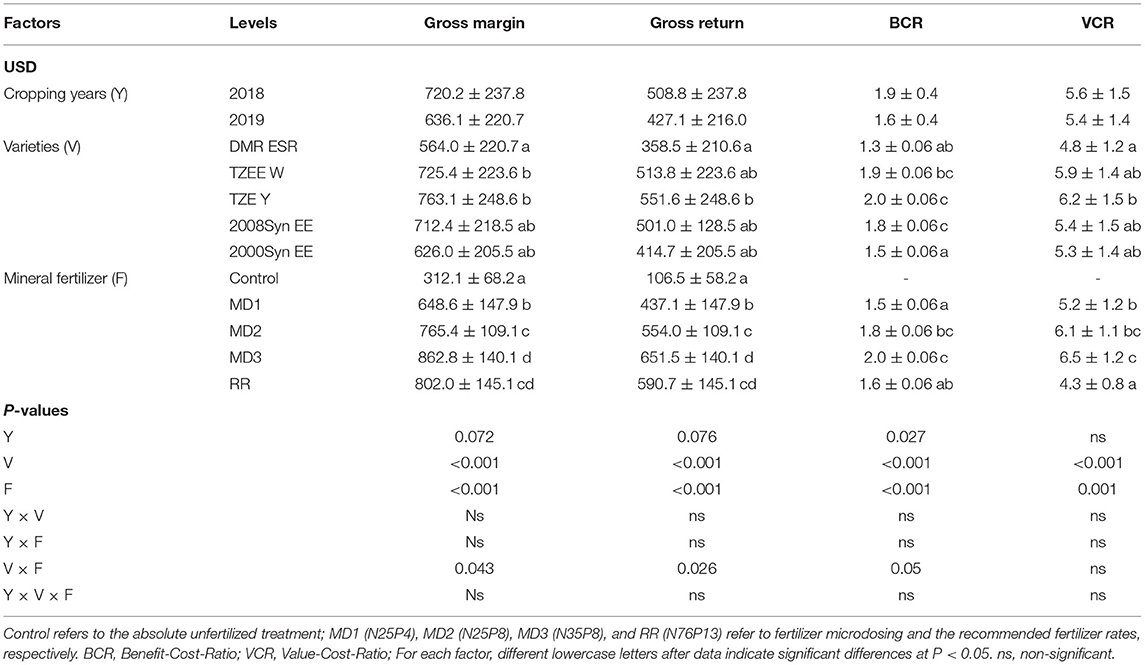
Table 5. Gross margin (GM, USD), gross return (GR, USD), benefit-cost-ratio (BCR), and value-cost-ratio (VCR) (mean ± standard error) as affected by the cropping years, varieties, and mineral fertilizer.
Like DT varieties, microdose fertilization obtained the highest performances. The MD1, MD2, and MD3 options have increased the GM by 336 USD (108%), 453 USD (145%), and 550 USD (176%), respectively, compared to the control without fertilizer, whereas RR has increased the GM by 489 USD (156%). Also, GR was increased by 310, 419, 511, and 454% for MD1, MD2, MD3, and RR, respectively, compared to the unfertilized control. However, MD3 and RR were statistically similar.
Overall, benefit-cost-ratio (BCR) and value-cost-ratio (VCR) tended to increase with the increasing microdosing application rates. The VCR on microdosing treatments vary between 5.6 and 6.5. The highest values of BCR and VCR were observed in MD3, while the lowest value in RR for BCR and MD1 for VCR (p < 0.001; Table 5). MD2 and MD3 significantly increase BCR and VCR by 19% and 47% on average, respectively, compared to RR (p < 0.001; Table 5). Also, there was a high significant effect of year on all economic indicators except for VCR (Table 5). As for yields, the highest GM, GR, and BCR was observed in 2018 and the lowest in 2019. A significant interaction was found between variety and fertilization for GM, GR, and BCR (p < 0.05; Table 5). Under the combined use of DT varieties and microdosing, GM and GR were increased by 156 and 450%, respectively, compared to control while the increases were 33 and 52%, respectively, under the combined use of the DS variety and microdosing (Supplementary Figure 3).
Relationship Among Yield, Growth Metrics, and Yield Components
For all varieties, positive and significant correlation was found between grain yield and LAI (r = 0.66–0.93; P < 0.001), and TDM (r = 0.70–0.94; P < 0.001) throughout the two growing seasons (Table 6). Unlike LAI and TDM, very few correlations were found between plant height and grain yield except for DMR-ESR-W and TZE-Y-Pop varieties where positive and significant correlation was found between the grain yield and plant height at 48 and 62 DAS (r = 0.42–0.68; P < 0.05; Table 6). Likewise, generally, no significant and very few correlation were found between grain yield and DMA (r = 0.28–0.76), TrDM (r = 0.21–0.56), and DMtE (r = 0.08–0.55). There was a strong positive correlation between grain yields and stover yields (r = 0.85–0.92; P < 0.001) and thousand grains weight (r = 0.65–0.94; P < 0.001), regardless of the variety. Generally, for the number of grains per cob, correlations are very few and non-significant (r = 0.11–0.82; P > 0.05; Table 6).
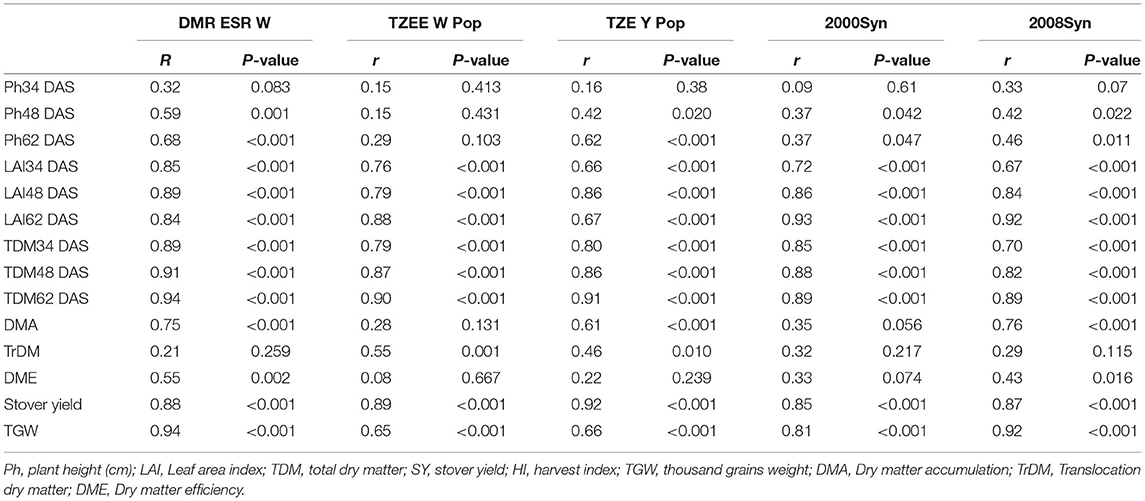
Table 6. Relationship between maize grain yield and other agronomic variables taken over the two cropping seasons (two-sided test of correlations different from zero, r, and probabilities, P).
Discussion
Agronomic Performances of Different DT Maize Varieties
Drought-tolerant (DT) varieties show an excellent capacity to accumulate dry matter than the DS variety, respectively, at 48 and 62 DAS (Figure 3). Likewise, these varieties recorded the greatest leaf area index, despite the climate-sensitive variety are reputed to produce more leaves during their cycle. Compared to climate-sensitive varieties, DT varieties loss less leaves after silking (not shown). These leaves actively participate in accumulating more biomass than the climate-sensitive variety. Faster senescence of the leaves in maize production can be the result of genetic and N-stress (Schulte auf'm Erley et al., 2007), water stress (Sah et al., 2020), and disease. But, in our study, this can be attributed to genetic reason because these parameters are not a variation source. Tollenaar and Lee (2006) and Echarte et al. (2008) have obtained similar conclusions when comparing an older hybrid and a modern hybrid of maize. Duvick et al. (2004) concluded that the delayed leaf senescence during grain filling has been one of the traits that was the most visually distinctive between older and newer hybrids.
The DT varieties have improved the grain and stover yields compared to the climate-sensitive variety. These results show that genetic improvement greatly contributes to increasing grain and stover yields. Abbassene et al. (1997) revealed that in semi-arid altitude conditions, the early genotypes are characterized by high filling speeds per m2, which are opposed to late genotypes by efficiently using the assimilates stored in the stem. This contributes to accumulation of more dry matter than the late genotype. Equally, climate-sensitive varieties invest little carbohydrates during the filling of cob because of the effects of heat and water stress, many of their tillers abort (Abbassene et al., 1997; Brancourt-Hulmel et al., 2003). Other studies have come to the same result that the DT varieties have shown a yield advantage over conventional hybrids under drought stress conditions (Varshney et al., 2011; Ziyomo et al., 2013; Sammons et al., 2014; Vanlauwe et al., 2014).
Indeed, under conditions of water stress, early varieties are genetically more predisposed to produce more carbohydrates than late genotypes. In controlled drought trials, DT maize has been found to have a yield advantage of as high as 137% over a commercial comparison variety (Fisher et al., 2015). Vernooy (2003) have concluded that the use of local genotypes is a limiting factor in the productivity of maize. Also, DT varieties obtained the highest value of TrDM contrary to climate-sensitive variety. The high part of dry matter was produce after the anthesis day. Ying et al. (2002) confirmed the ability of a newer hybrid to maintain a relatively high leaf carbon exchange during the grain-filling period (i.e., functional “stay green”), which positively influenced the dry matter accumulation. Inconsistently, DM accumulation values were high with the climate-sensitive variety. Indeed, a great part of dry matter was produced before the anthesis day for this variety. Climate-sensitive variety did more stock of assimilates in their stem for being used in the grain filling before anthesis in opposite to DT varieties, which was done after the anthesis day. In the same trend, Ciampitti and Vyn (2012) showed a high total plant biomass accumulation during the post-silking period in recently released cultivars (1991–2011) compared with older genotypes (1949–1990). Conversely, the high DM accumulation in modern cultivars during the post-silking stage may be largely explained by the delayed leaf senescence from silking to maturity (Tollenaar et al., 2004). Stay-green leaves at maturity are considered to be a major contributor to the genetic improvement in maize yield in the United States of America (Duvick, 2005).
These DT varieties also show a significant economic advantage under fertilizer microdosing over climate-sensitive varieties. Gross margin and the gross return were improved by DT varieties by 67 and 85%, respectively, compared to the climate-sensitive variety. In Zimbabwe, for example, DT varieties increased yields of climate-resilient maize, and translated to in an income of 240 USD per hectare (Lunduka et al., 2019). Equally, VCR and BCR were increased by 57 and 85%, respectively, compared to the climate-sensitive variety. These economic performances are due to the perfect adaptation of these varieties to the actual context of climate change. The adoption of climate-smart maize across 13 African countries has the potential to generate between 362 million USD to 590 million USD over a 7-year period, through both yield gains and an increase in yield stability (Kostandini et al., 2013). This is an opportunity for small farmers to make profitable maize production.
Maize Response to Fertilizer Microdosing Application
The results show that the ability of plants to accumulate the total dry matter at harvest varied greatly with fertilizer treatments. Mineral fertilization increased the average biomass by 73, 101, and 119% respectively for MD1, MD2, and MD3 compared to the unfertilized control. Among all the microdoses tested, MD3 produced the most dry matter as the RR treatment at 62 DAS. This is probably due to the availability of nutrients that are indispensable for the plant at the right time and right placement under the microdose fertilization technique. Likewise, fertilization significantly affected the leaf area index with the highest values obtained under MD3 and RR treatments showing the high potentiality of microdosing to increase photosynthesis rate.
The addition of nitrogen and phosphorus through microdosing helped to boost average grain productivity by 171% and straw by 98%. This could be explained by the low nitrogen (N) and phosphorus (P) content in the arable layers of cultivated African soils (Bationo et al., 1998). Conventional fertilization is, therefore, an interesting method of intensifying cereal production systems (Kamara et al., 2005; Wopereis et al., 2006; Vanlauwe et al., 2014; Naab et al., 2015). However, its rational and sustainable use is desirable. Microdoses of MD1, MD2, and MD3 have increased the average yields by 132, 173, and 208%, respectively, compared to the control without fertilizer. The International Crops Research Institute for the Semi-Arid Tropics, ICRISAT (2009) showed an increase of sorghum and millet grain yields from 44 to 120% compared to the control without fertilizer. Twomlow et al. (2010) also concluded an increase in grain yields of 30–40% with an application of 17 kg N ha−1 on the skeletal soils of Zimbabwe. In addition, Ncube et al. (2007) showed that farmers can increase their yield by 50% with only 9 kg/ha of nitrogen compared to control without fertilizers. Likewise, Buerkert et al. (2001) obtained an increase from 70 to 74% on cereals in stations under microdose fertilization with NPK (Nitrogen, Phosphorus and Potassium) compound fertilizers. Indeed, microdose fertilization increases the efficient use of the provided nutrients (Ibrahim et al., 2015). A recent meta-analysis realized by Ouedraogo et al. (2020) on microdosing performance in Sub-Sahara Africa revealed a 68% increase of cereal yields (maize, millet, and sorghum). Microdosing provides sufficient nutrients, especially on poor soils or degraded lands in amounts that are not too costly and are not damaging to the environment (Murendo and Wollni, 2015). This technology stimulates premature growth of the plant root system (Ibrahim et al., 2015). This increases the nutrient absorption capacity and, thus, accelerates the capacity of the plant to store more matter and to have an optimal growth and development. Also, localized application reduces the risk of losses by leaching and volatilization.
The harvest index (HI) is used to assess the ability of a variety to produce more grain than above-ground biomass. The control without fertilizer tends to produce more aerial biomass than grain. This can be explained by the deficit of nutrients in the soil at the filling grain stage. The average harvest index varied considerably between years with the largest indices obtained in 2018 compared to 2019. This could be the direct consequence of the rainfall fluctuations and dry spells observed in 2019. Indeed, under conditions of water stress, the maize plant tends to produce more biomass than grain. According to Tshiabukole (2018), water stress during flowering results in a sharp reduction of the number of seeds per cob. Likewise, the fertilizer treatments improved significantly the TGW by 43% compared to the control. This can be a result of a much high photosynthetic activity on these treated plots.
Likewise, microdosing technology hugely increased the GM and GR compared to the control without fertilizer. By using this technology, farmers can increase the GM and GR by 143 and 413%, respectively, against the increases of 156 and 454% under RR treatment. However, MD3 shows a similar performance to RR despite the large difference in the applied fertilizer rates between the two treatments. Indeed, despite the high variable cost of microdosing fertilizer, this technology incurs more return on investment than the conventional practice. Aune et al. (2012) showed that gross margin could be doubled through the use of microdosing in Mali. Likewise, GM increase of 170 USD was found by Tovihoudji et al. (2019) on maize under combined hill-placed manure and microdose fertilization.
The VCR under microdose fertilization varies between 5.6 and 6.5. In economic terms, a VCR value greater than 1 means that the cost of investment in fertilizer and in additional labor costs are recovered, while a VCR of 2 represents a 100% return on investment (Kihara et al., 2016). These values are well greater than values of 3–4 that may be required in risky environments (CIMMYT, 1988). Aune and Ousman (2011) reported under the on-farm experiments in Sudan, VCRs of 6.1 and 6.8 for sorghum and millet, respectively, under the application of 0.3 g fertilizer per pocket and priming. Likewise, the BCR was high on microdosing treatments. The microdosing option 3 has increased the BCR to 26% compared to RR, thus, showing its economic potential.
Efficiency of Fertilizer Microdosing Across Different DT Maize Varieties
Many studies separately reported the efficacy of microdosing and DT varieties as climate-smart technologies, but few have reported their effect together. Indeed, the efficiency of fertilizer microdosing varied across varieties. When comparing the microdosing treatments or the recommended rate, the greatest yield and yield attributes, profitability, and agronomic efficiency were observed on DT varieties. Microdosing fertilization application offers an average yield surplus of 658 kg (+30%) under DT varieties compared to its combination with the climate-sensitive variety. This performance was undoubtedly the result of an additive effect of these two climate-smart technologies. In fact, DT varieties are able to optimize yield under water stress, while fertilizer microdosing favors the efficient use of nutrients. Likewise, DT varieties revealed their highest growth and yield potential under low nutrient conditions than climate-sensitive varieties. Collectively, these effects strengthen the ability of the maize cropping systems to resist to environmental constraints and increase the economic profitability. Tshiabukole et al. (2019) recently reported in their study the high potential of DT and agroecological adaptation varieties under low fertilizers use. However, using low N fertilization (30 kg N ha−1) and 46 kg ha−1 of superphosphate. Yeboah et al. (2019) observed no significant effect of DT varieties and nitrogen fertilizer in farm conditions during the major and minor seasons. Kabate et al. (2015), by reviewing the factors driving the doubling of maize yields in recent years in Ethiopia, showed that this was due to the adoption of modern (tolerant and high yielding) varieties coupled with the application of a small amount of nitrogen (34 kg ha). When using microdosing on DS variety, GM and GR were increased by 33% (202 USD) and 263% (279 USD), respectively, while microdosing use under DT varieties increases these economic parameters by 156% (487 USD) and 450% (482 USD) compared to the control. With the combined use of microdosing and DT varieties, a gain of almost triple and double of the GM and GR was obtained. Here, this combination proves to be a net and gross income multiplier in the maize farming system. Indeed, this combination optimizes yields and nutrient use, minimizes inputs by reducing the amount of fertilizer used, and minimizes the effect of environmental constraints, notably water stress.
However, the agronomic and economic performance of microdose fertilization is variable across environmental conditions and agricultural practices, as mentioned by Ouedraogo et al. (2020). Likewise, several studies showed that microdose fertilization strategy resulted in negative partial nutrient balances in the order of −24 to −64 kg N ha−1 (Ibrahim et al., 2015, 2016; Tovihoudji et al., 2017). Even though this negative balance has not been demonstrated with DT varieties in this study, it may be necessary to combine these two technologies with organic amendments (farmyard manure, crop residues, etc.) to ensure a long term sustainability.
Conclusion
The results of the current study show that the adequate supply of fertilizers through microdosing technology have considerably improved the growth and yield performances of maize such as the recommended rate, which is more expensive. Microdosing has also been shown to be more efficient in the use of nutrients. Likewise, DT varieties were better for all the studied parameters than the climate-sensitive variety. Also, the economic evaluation shows that these are lucrative compared to the conventional practice. The combination of microdose and DT varieties (especially 35 kg ha−1 of nitrogen and 8 kg ha−1 of phosphorus), under TZE Y Pop STR QPM variety, seems to be a very interesting alternative in our current context of declining soil fertility and climatic variability. However, further testing of the technologies through on-farm experiments are required to confirm our findings. There is a need to evaluate these management options in fields of farmers, taking into account the different climatic, soil, and management conditions, to better assess and understand the variation in maize response and economic benefits and risk.
Data Availability Statement
The raw data supporting the conclusions of this article will be made available by the authors, without undue reservation.
Author Contributions
PT: conceptualization, methodology, formal analysis, investigation, visualization, writing—original draft, writing—review and editing, and funding acquisition. BB: conceived and designed the experiments, performed the experiment, analyzed the data and writing—original draft. MBH, ZT, and PA: methodology and writing—review and editing. All authors have read and approved the final manuscript.
Funding
This work was received funding from Africa Climate Leadership Program (AfriCLP, https://africlp.or.ke/).
Conflict of Interest
The authors declare that the research was conducted in the absence of any commercial or financial relationships that could be construed as a potential conflict of interest.
Publisher's Note
All claims expressed in this article are solely those of the authors and do not necessarily represent those of their affiliated organizations, or those of the publisher, the editors and the reviewers. Any product that may be evaluated in this article, or claim that may be made by its manufacturer, is not guaranteed or endorsed by the publisher.
Acknowledgments
The authors are grateful to the Africa Climate Leadership Program (AfriCLP, https://africlp.or.ke/) for their financial support and the staff of Agricultural Research Center of Northern Benin (CRA-Nord) for their technical help. The authors are also grateful to Zakari Sissou for reviewing the draft version of this manuscript.
Supplementary Material
The Supplementary Material for this article can be found online at: https://www.frontiersin.org/articles/10.3389/fagro.2021.763430/full#supplementary-material
References
Abbasi, M. K., Tahir, M. M., and Rahim, N. (2013). Effect of N fertilizer source and timing on yield and N use efficiency of rainfed maize (Zea mays L.) in Kashmir?Pakistan. Geoderma. 195, 87–93. doi: 10.1016/j.geoderma.2012.11.013
Abbassene, F., Bouzerzour, H., and Hachemi, L. (1997). Phenologie et production du blé dur (T. durum) en zone semi-aride d'altitude. Anal. Agron. 18, 106–113.
Abdoulaye, T., Wossen, T., and Awotide, B. (2018). Impacts of improved maize varieties in Nigeria: ex-post assessment of productivity and welfare outcomes. Food Secur. 10, 369–379. doi: 10.1007/s12571-018-0772-9
Arouna, A., and Arodokoun, U. (2013). Analysis of strategies and policies for adaptation to climate change in West and Central africa: benin case study. Agron. Africaine 6, 41–55.
Aune, J. B., Doumbia, M., and Berthe, A. (2007). Microfertilizing sorghum and pearl millet in Mali: agronomic, economic and social feasibility. Outl. Agri. 36, 199–203. doi: 10.5367/000000007781891504
Aune, J. B., and Ousman, A. (2011). Effect of seed priming and micro-dosing of fertilizer on sorghum and pearl millet in Western Sudan. Exp. Agri. 47, 419–430. doi: 10.1017/S0014479711000056
Aune, J. B., Traoré, C. O., and Mamadou, S. (2012). Low-cost technologies for improved productivity of dryland farming in Mali. Outl. Agri. 41, 103–108. doi: 10.5367/oa.2012.0084
Baco, M. N. (2019). Drought-tolerant varieties as an adaptation strategy in the context of climate change: insight from maize production systems in benin. Asian J. Agri. Extens. Econ. Sociol. 37, 1–7. doi: 10.9734/ajaees/2019/v37i430287
Badu-Apraku, B., Fontem, L. A., Akinwale, R. O., and Oyekunle, M. (2011a). Biplot analysis of diallel crosses of early maturing tropical yellow maize inbreds in stress and nonstress environments. Crop Sci. 51, 173–188. doi: 10.2135/cropsci2010.06.0366
Badu-Apraku, B., Oyekunle, M., Akinwale, R. O., and Fontem, L. A. (2011b). Combining ability of early-maturing white maize inbreds. Agron. J. 103, 544–557. doi: 10.2134/agronj2010.0345
Barrett, C. B., and Bevis, L. E. M. (2015). The self-reinforcing feedback between low soil fertility and chronic poverty. Nat. Geosci. 8, 907–912. doi: 10.1038/ngeo2591
Bationo, A., Lompo, F., and Koala, S. (1998). Research on nutrient ows and balances in west Africa : state of the art. Agri. Ecosyst. Environ. 71, 19–35. doi: 10.1016/S0167-8809(98)00129-7
Beyene, Y., Mugo, S., Semagn, K., Asea, G., Trevisan, W., Tarekegne, A., et al. (2013). Genetic distance among doubled haploid maize lines and their testcross performance under drought stress and non-stress conditions. Euphytica 192, 379–392. doi: 10.1007/s10681-013-0867-5
Boniphace, N. S., Fengying, N., and Chen, F. (2015). An analysis of smallholder farmers' socio-economic determinants for inputs use: a case of major rice producing regions in Tanzania. Russian J. Agri. Socio-Econ. Sci. 38, 41–55. doi: 10.18551/rjoas.2015-02.05
Brancourt-Hulmel, M., Doussinault, G., Lecomte, C., Bérard, P., Le Buanec, B., and Trottet, M. (2003). Genetic improvement of agronomic traits of winter wheat cultivars released in France from 1946 to 1992. Crop Sci. 43, 37–45. doi: 10.2135/cropsci2003.3700
Buerkert, A., Bationo, A., and Piepho, H. (2001). Efficient phosphorus application strategies for increased crop production in sub-Saharan West Africa. Field Crops Res. 72, 1–15. doi: 10.1016/S0378-4290(01)00166-6
Camara, B. S., Camara, F., Berthe, A., and Oswald, A. (2013). Microdosing of fertilizer – a technology for farmers' needs and resources. Int. J. AgriSci. 3, 387–399.
Ciampitti, I. A., and Vyn, T. J. (2012). Physiological perspectives of changes over time in maize yield dependency on nitrogen uptake and associated nitrogen efficiencies: a review. Field Crops Res. 133, 48–67. doi: 10.1016/j.fcr.2012.03.008
CIMMYT Economics Program International Maize, and Wheat Improvement Center. (1988). From Agronomic Data to Farmer Recommendations: An Economics Training Manual (No. 27). CIMMYT.
Cooper, P. J. M., Cappiello, S., Vermeulen, S. J., Campbell, B. M., Zougmoré, R., and Kinyangi, J. (2013). Large-scale implementation of adaptation and mitigation actions in agriculture. CCAFS Working Paper No. 50. Copenhagen: CGIAR Research Program on Climate Change, Agriculture and Food Security (CCAFS) 50, 1–99. Available online at: http://ccafs.cgiar.org/bigfacts (accessed December 12, 2021).
Duvick, D. N. (2005). The contribution of breeding to yield advances in maize (Zea mays L.). Adv. Agron. 86, 83–145. doi: 10.1016/S0065-2113(05)86002-X
Duvick, D. N., Smith, J. S. C., and Cooper, M. (2004). Long-term selection in a commercial hybrid maize breeding program. Plant Breed. Rev. 24, 109–152. doi: 10.1002/9780470650288.ch4
Echarte, L., Rothstein, S., and Tollenaar, M. (2008). The response of leaf photosynthesis and dry matter accumulation to nitrogen supply in an older and a newer maize hybrid. Crop Sci. 48, 656–665. doi: 10.2135/cropsci2007.06.0366
Fisher, M., Abate, T., Lunduka, R. w., Asnake, W., Alemayehu, Y., and Madulu, R. B. (2015). Drought tolerant maize for farmer adaptation to drought in sub-Saharan Africa : determinants of adoption in eastern and southern Africa. Climate Change 133, 283–299. doi: 10.1007/s10584-015-1459-2
Holden, S. T., and Fisher, M. (2015). Subsidies promote use of drought tolerant maize varieties despite variable yield performance under smallholder environments in Malawi. Food Secur. 7, 1225–1238. doi: 10.1007/s12571-015-0511-4
Ibrahim, A., Abaidoo, R. C., Fatondji, D., and Opoku, A. (2016). Fertilizer microdosing increases crop yield in the Sahelian low-input cropping system: a success with a shadow. Soil Sci. Plant Nutr. 62, 277–288. doi: 10.1080/00380768.2016.1194169
Ibrahim, A., Clement, R., Fatondji, D., and Opoku, A. (2015). Detrminants of fertilizer microdosing-induced yield increment of pearl millet on and acid sandy soil. Exp. Agri. 52, 562–578. doi: 10.1017/S0014479715000241
ICRISAT (2009). Fertilizer Microdosing, Technical Report, 6. Available online at: www.icrisat.org (accessed December 12, 2021).
Kabate, T., Shiferaw, B., Menkir, A., Wegary, D., Kebede, Y., Tesfaye, K., et al. (2015). Factors that transformed maize productivity in Ethiopia. Food Secur. 7, 965–981. doi: 10.1007/s12571-015-0488-z
Kamara, A. Y., Ewansiha, S. U., and Menkir, A. (2014). Assessment of nitrogen uptake and utilization in drought tolerant and Striga resistant tropical maize varieties. Archiv. Agron. Soil Sci. 60, 195–207. doi: 10.1080/03650340.2013.783204
Kamara, B. A. Y., Menkir, A., Ajala, S. O., and Kureh, I. (2005). Performance of diverse maize gentoype under nitrogen deffiency in the northen guinea savana of Nigeria. Expl. Agric. 41, 199–212. doi: 10.1017/S0014479704002479
Kihara, J., Huising, J., Nziguheba, G., Waswa, B. S., Njoroge, S., Kabambe, V., et al. (2016). Maize response to macronutrients and potential for profitability in sub-Saharan Africa. Nutr. Cycl. Agroecosyst. 105, 171–181. doi: 10.1007/s10705-015-9717-2
Kostandini, G., La Rovere, R., and Abdoulaye, T. (2013). Potential impacts of increasing average yields and reducing maize yield variability in Africa. Food Pol. 43, 213–226. doi: 10.1016/j.foodpol.2013.09.007
La Rovere, R., Kostandini, G., Abdoulaye, T., Dixon, J., Mwangi, W., and Banziger, M. (2010). Potential Impact of Investments in Drought Tolerant Maize in Africa. Addis Ababa: CIMMYT, 38.
Lawes Agricultural Trust (2009). Genstat Release 12.1. Rothamsted: Lawes Agricultural Trust(Rothamsted Experimental Station).
Lunduka, R. W., Mateva, K. I., Magorokosho, C., and Manjeru, P. (2019). Impact of adoption of drought-tolerant maize varieties on total maize production in south Eastern Zimbabwe. Climate Dev. 11, 35–46. doi: 10.1080/17565529.2017.1372269
Makate, C., Wang, R., Makate, M., and Mango, N. (2017). Impact of drought tolerant maize adoption on maize productivity, sales and consumption in rural Zimbabwe. Agrekon 56, 67–81. doi: 10.1080/03031853.2017.1283241
Makumbi, D., Assanga, S., Diallo, A., Magorokosho, C., Asea, G., Worku, M., et al. (2018). Genetic analysis of tropical midaltitude- adapted maize populations under stress and nonstress conditions. Crop Sci. 58, 1492–1507. doi: 10.2135/cropsci2017.09.0531
Mucheru-Muna, M. W., Ada, M. A., Mugwe, J. N., Mairura, F. S., Mugi-Ngenga, E., Zingore, S., et al. (2021). Socio-economic predictors, soil fertility knowledge domains and strategies for sustainable maize intensification in Embu County, Kenya. Heliyon 7:e06345. doi: 10.1016/j.heliyon.2021.e06345
Murendo, C., and Wollni, M. (2015). Ex-post Impact Assessment of Fertilizer Microdosing as a Climate-Smart Technology in Sub-Saharan Africa. Working paper, International Crops Research Institute for the Semi-Arid Tropics (ICRISAT), 27. Available online at: https://cgspace.cgiar.org/handle/10568/67122
Naab, J. B., Mahama, G. Y., Koo, J., Jones, J. W., and Boote, K. J. (2015). Nitrogen and phosphorus fertilization with crop residue retention enhances crop productivity, soil organic carbon, and total soil nitrogen concentrations in sandy-loam soils in Ghana. Nutr. Cycl. Agroecosyst. 102, 33–43. doi: 10.1007/s10705-015-9675-8
Ncube, B., Dimes, J. P., Twomlow, S. J., Mupangwa, W., and Giller, K. E. (2007). Raising the productivity of smallholder farms under semi-arid conditions by use of small doses of manure and nitrogen : a case of participatory research. Nutr. Cycl. Agroecosyst. 77, 53–67. doi: 10.1007/s10705-006-9045-7
Okebalama, C. B., Safo, E. Y., Abaido, R. C., and Logah, V. (2016). Fertilizer use and management pratices among maize and cowpea smallholder farmers in Ghana. Tropicultura 34, 113–126.
Ouedraogo, Y., Taonda, J. B. S., Sermé, I., Tychon, B., and Bielders, C. L. (2020). Factors driving cereal response to fertilizer microdosing in sub-Saharan Africa: a meta-analysis. Agron. J. 112, 2418–2431. doi: 10.1002/agj2.20229
Sah, R. P., Chakraborty, M., Prasad, K., Pandit, M., Tudu, V. K., Chakravarty, M. K., et al. (2020). Impact of water deficit stress in maize: phenology and yield components. Sci. Rep. 10, 1–15. doi: 10.1038/s41598-020-59689-7
Sammons, B., Whitsel, J., Stork, L. A. G., Reeves, W., and Horak, M. (2014). Characterization of drought-tolerant maize MON 87460 for use in environmental risk assessment. Crop Sci. 54, 719–729. doi: 10.2135/cropsci2013.07.0452
Sanou, J., Bationo, B. A., Barry, S., Nabie, L. D., Bayala, J., and Zougmore, R. (2016). Combining soil fertilization, cropping systems and improved varieties to minimize climate risks on farming productivity in northern region of Burkina faso. Agri. Food Secur. 5, 1–12. doi: 10.1186/s40066-016-0067-3
Schulte auf'm Erley, G., Begum, N., Worku, M., Bänziger, M., and Horst, W. J. (2007). Leaf senescence induced by nitrogen deficiency as indicator of genotypic differences in nitrogen efficiency in tropical maize. J. Plant Nutr. Soil Sci. 170, 106–114. doi: 10.1002/jpln.200625147
Setimela, P. S., Magorokosho, C., Lunduka, R., Gasura, E., Makumbi, D., Tarekegne, A., et al. (2017). On-farm yield gains with stress-tolerant maize in Eastern and Southern Africa. Agron. J. 109, 406–417. doi: 10.2134/agronj2015.0540
Sime, G., and Aune, J. B. (2014). Maize response to fertilizer dosing at three sites in the central rift valley of Ethiopia. Agronomy 4, 436–451. doi: 10.3390/agronomy4030436
Simtowe, F., Amondo, E., Marenya, P., Rahut, D., Sonder, K., Erenstein, O., et al. (2019). Land use policy impacts of drought-tolerant maize varieties on productivity, risk, and resource use: evidence from Uganda. Land Use Pol. 88:104091. doi: 10.1016/j.landusepol.2019.104091
Tollenaar, M., and Lee, E. A. (2006). Dissection of physiological processes underlying grain yield in maize by examining genetic improvement and heterosis. Maydica 51:399.
Tollenaar, M. A. A. L. E. A., Ahmadzadeh, A., and Lee, E. A. (2004). Physiological basis of heterosis for grain yield in maize. Crop Sci. 44, 2086–2094. doi: 10.2135/cropsci2004.2086
Tovihoudji, P. G., Akponikpè, I. P., Agbossou, E. K., and Bielders, C. L. (2019). Using the DSSAT model to support decision making regarding fertilizer microdosing for maize production in the sub-humid region of Benin. Front. Environ. Sci. 7, 1–13. doi: 10.3389/fenvs.2019.00013
Tovihoudji, P. G., Akponikpè, P. I., Adjogboto, A., Djenontin, J. A., Agbossou, E. K., and Bielders, C. L. (2018). Combining hill-placed manure and mineral fertilizer enhances maize productivity and profitability in northern. Nutr. Cycl. Agroecosyst. 110, 375–393. doi: 10.1007/s10705-017-9872-8
Tovihoudji, P. G., Akponikpè, P. I., Agbossou, E. K., Bertin, P., and Bielders, C. L. (2017). Fertilizer microdosing enhances maize yields but may exacerbate nutrient mining in maize cropping systems in northern Benin. Field Crops Res. 213, 130–142. doi: 10.1016/j.fcr.2017.08.003
Traore, B., Corbeels, M., Wijk, M. T., Van Rufino, M. C., and Giller, K. E. (2013). Effects of climate variability and climate change on crop production in southern Mali. Eur. J. Agron. 49, 115–125. doi: 10.1016/j.eja.2013.04.004
Tshiabukole, J. P. K. (2018). Evaluation de la sensibilité aux stress hydriques du maïs (zea mays L) cultivé dans la savane du sud-ouest de la RD Congo, cas de Mvuazi. Université Pedagogique Nationale de Kinshassa, Thèse. Available online at: https://hal.archives-ouvertes.fr/tel-02062632/ (accessed December 12, 2021).
Tshiabukole, J. P. K., Khonde, G. P., Kankolongo, A. M., Jadika, C. T., and Vumilia, R. K. (2019). Comparative study of maize agronomics performance under low nitrogen soil availability, in southern savanna of DR Congo. Afrika Focus 32, 57–64. doi: 10.1163/2031356X-03202006
Twomlow, S., Rohrbach, A. D., Dimes, A. J., Rusike, A. J., Mupangwa, W., Ncube, B., et al. (2010). Microdosing as a pathway to Africa's green revolution: evidence from broad-scale on-farm trials. Nat. Commun. 88, 3–15. doi: 10.1007/s10705-008-9200-4
Vanlauwe, B., Coyne, D., Gockowski, J., Hauser, S., Huising, J., Masso, C., et al. (2014). Sustainable intensification and the African smallholder farmer. Curr. Opin. Environ. Sustain. 8, 15–22. doi: 10.1016/j.cosust.2014.06.001
Varshney, R. K., Bansal, K. C., Aggarwal, P. K., Datta, S. K., and Craufurd, P. Q. (2011). Agricultural biotechnology for crop improvement in a variable climate: hope or hype? Trends Plant Sci. 16, 363–371. doi: 10.1016/j.tplants.2011.03.004
Vernooy, R. (2003). Seeds That Give. Participatory Plant Breeding. Ottawa: International Development Research Centre. Available online at: https://www.idrc.ca/en/book/infocus-seeds-give-participatory-plant-breeding (accessed November 16, 2020).
Waithaka, M. M., Thornton, P. K., Shepherd, K. D., and Ndiwa, N. N. (2007). Factors affecting the use of fertilizers and manure by smallholders: the case of Vihiga, western Kenya. Nutr. Cycl. Agroecosyst. 78, 211–224. doi: 10.1007/s10705-006-9087-x
Wopereis, M. C., Tamélokpo, A., Ezui, K., Gnakpénou, D., Fofana, B., and Breman, H. (2006). Mineral fertilizer management of maize on farmer fields differing in organic inputs in the West African savanna. Field Crops Res. 96, 355–362. doi: 10.1016/j.fcr.2005.08.004
Wossen, T., Abdoulaye, T., Alene, A., Feleke, S., Menkir, A., and Manyong, V. (2017). Measuring the impacts of adaptation strategies to drought stress: the case of drought tolerant maize varieties. J. Environ. Manag. 203, 106–113. doi: 10.1016/j.jenvman.2017.06.058
Yallou, C. G., Aïhou, K., Adjanohoun, A., Baco, M. N., Sanni, O. A., and Amadou, L. (2010). Document Technique d'Information et de vulgarisation : Répertoire des Variétés de Maïs Vulgarisées au Bénin. MAEP/INRAB/BENIN. Bénin: Bibliothèque Nationale (BN) du Bénin, 19.
Yeboah, S., Amengor, N. E., Oteng-Darko, P., and Ribeiro, P. F. (2019). Determinants of nutritious drought tolerant maize adoption and mineral fertilizer application under smallholder farm conditions in Ghana. J. Agri. Sci. 11:10. doi: 10.5539/jas.v11n10p121
Ying, J., Lee, E. A., and Tollenaar, M. (2002). Response of leaf photosynthesis during the grain-filling period of maize to duration of cold exposure, acclimation and incident PPD. Crop Sci. 42, 1164–1172 doi: 10.2135/cropsci2002.1164
Youssouf, I., and Lawani, M. (2002). Les sols béninois: classification dans la base de référence mondiale, in Quatorziéme réunion du sous-comité ouest et centre africain de corrélation des sols pour la mise en valeur des terres, 9?13 octobre 2000 Abomey, Bénin. Rapport sur les Ressources en Sols du Monde n° 98 (Rome: FAO). 29–50.
Keywords: maize cropping systems, drought-tolerant, fertilizer micro-dosing, resource use efficiency, profitability
Citation: Tovihoudji PG, Bagri BM, Batamoussi Hermann M, Tonnang ZEH and Akponikpè PBI (2022) Interactive Effects of Drought-Tolerant Varieties and Fertilizer Microdosing on Maize Yield, Nutrients Use Efficiency, and Profitability in the Sub-Humid Region of Benin. Front. Agron. 3:763430. doi: 10.3389/fagro.2021.763430
Received: 23 August 2021; Accepted: 28 December 2021;
Published: 17 March 2022.
Edited by:
Tida Ge, Ningbo University, ChinaReviewed by:
Ayman E. L. Sabagh, Siirt University, TurkeyKokou Adambounou Amouzou, African Plant Nutrition Institute (APNI), Morocco
Copyright © 2022 Tovihoudji, Bagri, Batamoussi Hermann, Tonnang and Akponikpè. This is an open-access article distributed under the terms of the Creative Commons Attribution License (CC BY). The use, distribution or reproduction in other forums is permitted, provided the original author(s) and the copyright owner(s) are credited and that the original publication in this journal is cited, in accordance with accepted academic practice. No use, distribution or reproduction is permitted which does not comply with these terms.
*Correspondence: Pierre G. Tovihoudji, cGllcnJldG92aWhvdWRqaUB5YWhvby5mcg==