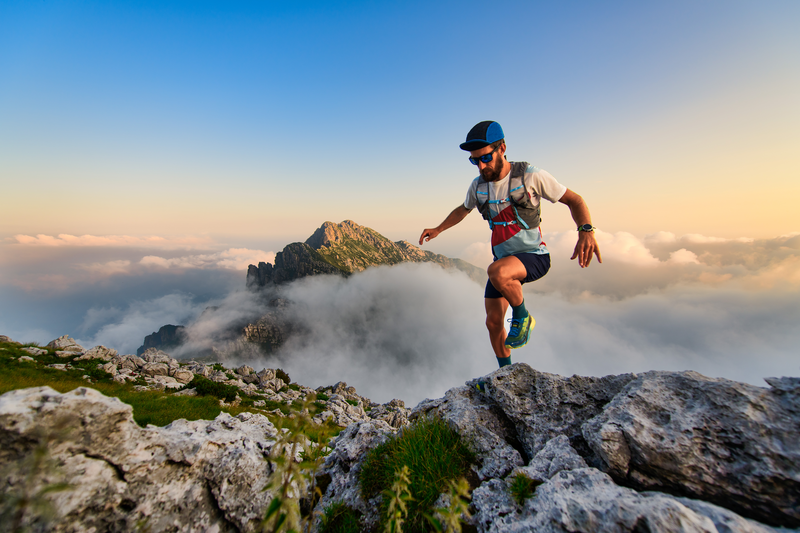
94% of researchers rate our articles as excellent or good
Learn more about the work of our research integrity team to safeguard the quality of each article we publish.
Find out more
ORIGINAL RESEARCH article
Front. Agron. , 20 January 2022
Sec. Plant-Soil Interactions
Volume 3 - 2021 | https://doi.org/10.3389/fagro.2021.758572
This article is part of the Research Topic Enhanced Nutrient Management in Agroecosystems View all 6 articles
The comprehensive use of organic, inorganic, and biological components of nutrient management in rice ecologies can potentially address the twin challenges of declining factor productivity and deteriorating soil health. A field study was thus conducted at Varanasi, India during the year 2013–14 and 2014–15 to assess the effect of the recommended dose of fertilizers (RDF) along with cow product (blends of 5 cow by-products i.e., dung, ghee, curd, urine, and milk that is known as panchagavya) on soil health, energy budget, and rice productivity. The results revealed that the inclusion of panchagavya as seedling root dip + 6% spray at 30 days after transplanting (DAT) + an application with irrigation water (15 l ha−1) at 60 DAT (D4) along with 100% RDF (F3) noted significantly higher rice grain yield (6.34 t ha−1) and higher dehydrogenase activity. However, the soil bacterial and actinomycetes population, soil microbial biomass carbon (SMBC), urease, and alkaline phosphatase activities were significantly higher with D4 along with 120% RDF (F4). Carbon output (5,608 kg CO2 eq ha−1), energy use parameters viz. energy output (187,867 MJ ha−1), net energy returns (164,319 MJ ha−1), and energy intensity valuation (5.08 MJ ) were significantly higher under F4. However, the energy ratio (8.68), energy productivity (0.292 kg MJ−1), and energy profitability (7.68) remained highest with 80% RDF (F2), while the highest carbohydrate equivalent yield (4,641 kg mha−1) was produced under F3. The combination of F3 with D4 resulted in the highest productivity, optimum energy balance, and maintaining soil quality. Therefore, a judicious combination of cow product (panchagavya) with RDF was found to improve the rice productivity, energy profitability, and soil quality under south Asian eastern Indo-Gangetic Plains (IGPs).
Grave concern has been raised on the sustainability of rice production due to the excessive use of market-driven inputs after the advent of the green revolution. An over-reliance on off-farm inputs under intensive agricultural practices has caused a decrease in factor productivity, accelerated negative environmental footprints, and energy inefficiencies at every production level (Ladha et al., 2009). The projections demand the doubling of cereal production by 2050 to feed a population of 1.6 billion (Swaminathan and Bhavani, 2013), which appears unrealistic without the realization of sustainable farming practices for yield enhancement with minimum environmental degradation.
Rice is the principal food crop globally and more than 87 % of rice is grown in South Asia (FAO, 2018). But the rice production systems in South East Asia are characterized by heavy non-renewable energy use (Jat et al., 2013), complete reliance on synthetic nutrient inputs (Singh, 2018), and overlooking organic manures (Saha et al., 2008) leading to environmental concerns (Ladha et al., 2009). These synthetic nutrients also release many greenhouse gases impairing the quality of soil and the agricultural environment (Gallaher et al., 2009; Perera, 2018). But, the complementary use of organic nutrients along with chemical fertilizers is of great importance for the maintenance of soil health and productivity. Further, the consideration and amalgamation of proper organic sources with fertilizers is of prime importance. The organic source like panchagavya that prepared from a blend of cow products (Natarajan, 2008) has numerous beneficial effects when applied as a spray, seedling root dip, and to the soil. When applied as a spray or through root dip increases quality parameters viz., crude fiber, protein, ascorbic acid, carotene content, and shelf life of rice (Beaulah, 2001), due to the presence of substantial quantities of Indole (FAO, 2018), Acetic Acid (IAA), and gibberellic acid (GA3) which act as stimuli for the production of growth regulators in plant systems (Somasundaram et al., 2003), thereby enhancing the growth and yield of rice (Balasubramanian et al., 2001). In addition to the various benefits of panchagavya, very few extensive studies were reported in the literature.
Soil health deterioration due to poor organic supplementation and higher inorganic inputs has resulted in the loss of soil microbial diversity as well as factor productivity over the past few decades in India (Bhatta et al., 2016). Panchagavya has been reported to be useful for crop production besides improving soil quality through enhanced soil microbial activity (Upadhyay et al., 2019). Apart from being a very good source of nutrients, its effect on soil qualities was not extensively studied. Therefore, it is essential to evaluate and standardize the integration of panchagavya as a nutrient source for enhancing crop productivity and soil quality, and the following study was planned.
Soil biological quality (Geisseler et al., 2017) is an important indicator to identify the environment-friendly production system and the energy analysis (Mansoori et al., 2012; Kulczycka and Smol, 2016). In the quest for sustainable low-input agricultural systems, the use of panchagavya (Upadhyay et al., 2018) and other on-farm inputs (Gundogmus, 2006) have shown promising results in several countries to improve the overall sustainability. It offers the twin benefits of improving resource vis-à-vis energy use efficiency in crop production by reducing the use of non-renewable nutrient resources (Upadhyay et al., 2019). Several studies comparing the energy use and its efficiency between organic and conventional farming systems have revealed higher crop yields and nutrient supplies, better energy efficiency with a lower cost of production (Deike et al., 2008; Mohammadi et al., 2014) but energy budgeting in rice for panchagavya and recommended dose of fertilizers (RDF) together was never computed. Therefore, to investigate the combined effect of RDF and panchagavya, the experiment was conducted for two consecutive years with the objectives (1) to know their effect on grain and biological yield of rice, (2) to assess the nutrient status in the soil, and plant uptake, (3) to monitor the changes in the soil biological activities, and (4) to work out the energetics.
The field trials were executed during 2013–14 and 2014–15 at the Agricultural Research Farm of Banaras Hindu University, Varanasi, India situated at 25°15′19.7″ N latitude, 82°59′34.2″ E longitude, 75 m above mean sea level. The experimental location was characterized by a semi-arid to sub-humid climate with a mean annual rainfall of 1,150 mm and potential evapotranspiration 1525 mm. The weekly weather condition was collected and is presented in Figure 1. During the cropping season, a total of 952.7 mm in 2013–14 and 834.7 mm in 2014–15 rainfall was received with the distribution being normal during the experimental period. The mean weekly maximum temperature was 28.03°C during 2013–14, and 28.65°C during 2014–15. The mean weekly sunshine length varied from 0.6 to 9.1 h in 2013–14 and 1.0 to 9.5 h in 2014–15.
The factors for experimentation were fitted in the split-plot design and replicated thrice. The main plots were allocated to fertilizer doses [60% of RDF (F1), 80% RDF (F2), 100% RDF (F3), and 120% RDF (F4)] while the panchagavya application {control (D0); 3% foliar sprays at 15, 30, and 45 days after transplanting (DAT) (D1); seedling root dip + 3% foliar spray at 30 DAT + application with irrigation water at 60 DAT (D2); 6% foliar sprays at 15, 30 and 45 DAT (D3) and seedling root dip + 6% foliar spray at 30 DAT + application with irrigation water at 60 DAT (D4)}to sub-plots. Rice hybrid PRH 10 - a basmati type was the test crop. The RDF for rice crops was 150, 75, 75, and 5 kg/ha of N, P2O5, K2O, and Zn respectively. One-fourth dose of nitrogen (N) and a full dose of phosphorus (P), potassium (K), and zinc (Zn) were applied 7 days after transplanting and the remaining three-fourths in two splits i.e., two-fourths at tillering stage (30 DAT), and one-fourths at panicle initiation stages (55 DAT).
Panchagavya – an age-old traditional organic source of plant nutrients used in India, was prepared in a wide-mouthed plastic container. Ghee (2.5 kg, made from cow milk) and fresh cow dung (12.5 kg) were put into a container and mixed meticulously by stirring twice a day for 3 days. On the 4th day, the other components (curd 5 kg, milk-7.5 l, cow urine-7.5 l, ripe banana-30 in numbers, and jaggery-1.25 kg) were added and the final volume was made up to 50 l by adding water and the contents were stirred twice daily for 15 days. The stock solution of panchagavya was ready for use after the 20th day. The stock solution of panchagavya was kept in the shade and to prevent houseflies from entering and laying eggs covered with a plastic mosquito net. Proper water was applied as and when required to preserve the slurry in a liquid state. Through irrigation, it was applied @ 15 l of the stock solution/ha. The chemical and biological parameters of the prepared panachagavya are presented in Tables 1, 2.
Seedlings for the system of rice intensification (SRI) were raised in 100 m2 area for transplanting in one hectare. Good quality seeds (5 kg ha−1) of the hybrid “PRH 10” were sown by spreading on the nursery bed. For raising nursery, beds of 1.0 m width, 10 m length along with 40 cm furrow were made for seed sowing. Raised nursery beds were made with a combination of FYM and soil at a 1:2 ratio. Carbendazim (@1 g dissolved in one liter water) and streptocycline (@ 6 g in 20 L of water) were used for seed treatment before sowing. To ensure quick and uniform germination, the paddy seeds were drenched in water for 2 days (48 h) and then allowed to shade dry before broadcasting on a raised nursery bed. Well sprouted seeds (20 g m−1) were sown unvaryingly on nursery bed in such a way that every seed had to be isolated from other very easily. Seeds were covered on seedbed with straw of wheat for at least 48 h following sowing to protect them from birds. Watering was done twice a day (early morning and evening). For transplanting, seedlings of 14 days of age (1 seedling hill−1) with a spacing of 25 × 25 cm were used. The net plots (2 rows from all side was removed as a border and 1 row as sampling area) were harvested with sickles and dried on a cemented floor on a sunny day for 5 days and then observation of biomass yield was noted. Grain yield (14% moisture using moisture meter) was noted while completing the process of threshing, cleaning, and drying. The biological yield was calculated using the following formula:
The comprehensive data of all the inputs (panchagavya, seeds, fertilizers, fuel, agro-chemicals, human as well as implements and machine) and outputs (by-product and main) were arranged for the study of energy input and output. Inputs were translated from the physical units to energy units (Yadava et al., 2017) by multiplying with conversion co-efficiency and energy input (Binning et al., 1983) and energy productivity (Tuti et al., 2012) were computed. The energy equivalents (Devasenapathy et al., 2009) of all inputs were added for the estimation of energy inputs (Devasenapathy et al., 2009) to provide an estimate for total energy input (Datta et al., 2014). Similarly, by multiplying the amount of production by its corresponding energy equivalent (Devasenapathy et al., 2009) the energy output (Chaudhary et al., 2006) was calculated. The energy output of the by-product (Mandal et al., 2015) was calculated by multiplying it with its corresponding equivalent (Chaudhary et al., 2009). The other energy parameters were calculated as described below.
NE = EO-EI
ERo = EO/EI
PE = ER/EI
EP = Ye/EI
EIE = GEO/CoC
Where
NE-Net energy (MJ ha−1); EO-Energy output (MJ ha−1); EI-Energy input (MJ ha−1); ERo-Energy ratio; PE-Energy profitability; ER-Energy returns (MJ ha−1); EP-Energy productivity (kg MJ−1), Ye-Crop economic yield (kg ha−1), EIE-Energy intensity in economic terms (MJ *); GEO-Gross energy output (MJ ha−1), CoC-Cost of cultivation (
ha−1) *Indian rupees.
The economic crop yield was converted into the equivalent value of carbohydrate (kg ha−1) (Gopalan et al., 2004). The carbon output was calculated based on the biomass of the plant which contains on an average 44% carbon (Lal, 2004).
Plant samples (grain and straw) were dried at 700C for 48 h, grounded with the help of a Macro-wiley mill, and passed through a 40 mesh sieve for determination of N, P, and K content. The total N and P content in the plant was determined by using the Kjeldahl and vanado molybdate method, respectively. The K content of the plant sample was estimated by using a flame photometer. Standard methodologies for the determination of N, P, and K content in rice were used as suggested by (Jackson, 1958).
The following formula as suggested by (Black, 1967) was used for the calculation of nutrient removal by grain and straw of rice:
Soil samples (0–15 cm soil profile) were collected at 30 and 60 DAT from each experimental plot. A soil auger having a diameter of 5 cm was used for the collection of composite soils from four different places. Just after, the dry shade soil samples were grounded with a pestle and mortar. After the removal of inert matter with the help of a 2 mm sieve, the soil samples were stored in a sterile polypropylene bag. Before the analysis of soil microbial biomass carbon (SMBC), enzymatic activities viz. dehydrogenase (DHA), urease (UA), alkaline phosphatase activity (APA), and microbial population (actinomycetes and bacteria) the soil samples were stored at 4°C. The soil microbial population, UA, DHA, APA, and SMBC were estimated as per the method as described by (Chhonkar et al., 2007).
The initial and post-harvest soil samples were collected (0–15 cm soil profile) from four different locations with the help of a core sampler having 5 cm diameter from each experimental plot for chemical analysis. The soil samples were ground with the help of pestle-mortar and sieved in a 2-mm sieve and analyzed for pH (Jackson, 1958) and electrical conductivity (Richards, 1954) following standard analytical procedures (Table 3). The processed samples were also analyzed for their available N content (Subbiah and Asija, 1973), organic carbon (Walkley and Black, 1934), Olsen P (Olsen et al., 1954), available K (Jackson, 1958), and DTPA-extractable Zn (Lindsay and Norvell, 1978) at depths of 0–15 cm.
The data recorded were analyzed using a standard statistical procedure to draw a valid conclusion. For computing, standard ANOVA and comparing treatment means the SPSS 17.0 (SPSS, 2008, New York, USA) statistical package was used. For comparing treatment means, critical difference (CD)/least significant difference (LSD) at p < 0.05 significance was used.
Rice grain yield (mean of 2 years) was significantly (p < 0.05) influenced by including panchagavya with RDF (Table 4). The application of RDF (100%) along with the D4 level of panchagavya produced an additional (2.12 t ha−1) grain yield over 60% RDF and no panchagavya. Interactions of RDF × panchagavya (p = 0.0001) were significant for grain and biological yield.
The application of 100% RDF (150, 75, 75, and 25 kg N, P2O5, K2O, and ZnSO4.7H2O ha−1) recorded significantly higher grain yield, which was 23.8, 6.1, and 3.31% higher over 60, 80, and 120% RDF respectively (Table 5), likewise, panchagavya level D4 (seedling root dip + 6% spray at 30 DAT + application with irrigation water at 60 DAT) resulted in the highest grain yield (5.93 t ha−1). The interaction effect between RDF and panchagavya was significant and it was found that the F3 along with D4 treatments recorded maximum grain yield (6.34 t ha−1) over RDF along with control (4.22 t ha−1).
Table 5. Interaction effect of fertilizer dose and time and rate of panchagavya application on grain and biological yield of hybrid basmati rice.
The higher biological yield (14.02 t ha−1) was recorded with the application of 120% RDF which produced 30.2, 13.8, and 6.1% higher biological yield than 60, 80, and 100% RDF, respectively. Among the panchgavya treatments, the maximum biological yield (13.36 t ha−1) was produced under D4, which was significantly higher than control, D1, and D2, while remaining at par with D3. The interaction effect of NPK levels and panchagavya on the biological yield of rice indicated that the highest biological yield (15.08 t ha−1) was noticed with the application of 120% RDF with D4.
Total N, P, and K uptake was significantly higher (p < 0.05) under 120% RDF along with the D4 level of panchagvya application over 60% RDF without panchagavya (Table 4). An increase of 78.6, 19.0, and 94.39 kg ha−1, respectively have been recorded in the total N, P, and K uptake with the D4 level of panchagvya application over 60% RDF with no panchagavya after 2 years of experimentation.
120% RDF application observed significantly more N uptake (132.27 kg ha−1) over 60, 80, and 100% RDF. Amongst the panchagavya levels, maximum N uptake (123.25 kg ha−1) by grain + straw was recorded with D4, followed by D3. The interaction effect of RDF (120%) and panchagavya (D4) was also found significant in N uptake (150.1 kg ha−1) by grain + straw.
The significantly higher total P uptake by grain + straw (26.73 kg ha−1) was recorded with 120% RDF application. The total P uptake by grain and straw under different levels of RDF was in the order as 120% RDF > 100% RDF > 80% RDF > 60% RDF. The application of panchagavya caused significant variation in the total P removal by the crop. Significantly higher P removal by rice grain + straw (23.92 kg ha−1) was noted with D4 than the remaining treatments. The conjoint application RDF (120%) with panchagavya (D4) exhibited the higher total P removal (31.07 kg ha−1) by the crop (Table 6).
Table 6. Interaction effect of recommended dose of fertilizer (RDF) and panchagavya on total nutrient (grain + straw) uptake (kg ha−1) by hybrid basmati rice.
Significantly higher K removal by rice (163.31 kg ha−1) was recorded with RDF (120%) as compared with 60, 80, and 100% RDF, and among the panchagavya treatments, D4 resulted in the higher total potassium removal by grain + straw (142.58 kg ha−1) while remaining at par with D3. The highest K removal (185.89) was recorded due to the interaction between the 120% RDF and with D4, however, the lowest K removal (91.5 kg ha−1) was noticed with 60% RDF without panchagavya (Table 6).
Higher available N (245 kg ha−1), P (26 kg ha−1), and K (205 kg ha−1) contents were observed with 120% RDF in combination with the D4 level of panchagavya (Figures 2A–C), which was 16.66, 44.44, and 9.1% higher, respectively, over the application of 60% RDF without panchagavya.
Figure 2. Interaction of fertilizer dose and time and rate of panchagavya application on available nitrogen (A), phosphorus (B), and potassium (C) in the soil after harvest of hybrid rice under SRI. F1, F2, F3 and F4 are the 60, 80, 100 and 120% RDF respectively, D0-Control; D1-3% foliar sprays at 15, 30 and 45 DAT; D2- seedling root dip + 3% foliar spray at 30 DAT + application with irrigation water at 60 DAT; D3-6% foliar sprays at 15, 30 and 45 DAT; D4- seedling root dip + 6% foliar spray at 30 DAT + application with irrigation water at 60 DAT.
The different levels of RDF had a significant result on the soil bacterial population. The maximum bacterial population (51.3 cfu × 105 g−1 soil at 30 DAT and 72.1 cfu × 105 g−1 soil at 60 DAT) was recorded with 120% RDF, which remained on par with 100% RDF, but significantly superior over 60 and 80% RDF. Amongst the panchagavya levels, D4 noticed a significantly higher population of bacteria (54.8 cfu × 105 g−1 soil at 30 DAT and 79.8 cfu × 105 g−1 soil at 60 DAT) than the rest of the treatments at 30 and 60 DAT. The interaction effect between the application of 120% RDF along with D4 significantly increased the bacterial population (55.7 at 30 DAT and 86.3 at 60 DAT) over other treatment combinations but remained at par with 100% RDF + D4 at 30 and 60 DAT (Table 7).
Table 7. Interaction effect of RDF and panchagavya on bacterial and actinomycetes population (cfu) at 30 and 60 DAT.
At 30 and 60 DAT, the actinomycetes population was significantly higher with 120% RDF (47.8 cfu × 104 g−1 soil at 30 DAT and 63.0 cfu × 104 g−1 soil at 60 DAT) over 60 and 80% RDF, however, remained on par with 100% RDF. The actinomycetes population at different growth stages (30 and 60 DAT) under D4 remained 55.1 cfu × 104 g−1 soil and 67.3 cfu × 104 g−1 soil at 30 and 60 DAT, respectively over the rest of the treatments. The interaction effect of the application of 120% RDF with D4 treatment significantly increased the actinomycetes population as 58.4 cfu × 104 g−1 soil and 74.9 cfu × 104 g−1 soil at 30 and 60 DAT, respectively, but remained at par with 100% RDF at D4 (Table 7).
The interaction effect of 120% RDF with D4 significantly increased the SMBC (135.8 and 199.2 μg C g−1 soil at 30 DAT and 60 DAT, respectively) over other treatment combinations but remained on par with 100% RDF under D4 (Table 8).
On the other side, 100% RDF and D4 exhibited significantly higher dehydrogenase activity (DHA) (139.6 and 158.2 μg TPF /g soil/24 h at 30 and 60 DAT, respectively), followed by 100% RDF with D3. Among the other interactions, the application of 120% RDF with D4 significantly increased the alkaline phosphatase activity (83.8 and 97.7 μg p-NP g−1 soil h−1 at 30 DAT and 60 DAT, respectively) (Table 9). Further, 120% RDF and D4 registered significantly higher urease activity (292 and 343.7 μg UH g−1 soil h−1 at 30 and 60 DAT, respectively) (Table 9).
Table 9. Interaction effect of RDF and panchagavya on soil enzymatic activity (urease and alkaline phosphatase) at 30 and 60 DAT.
Based on the energy equivalent (Table 10) parameters, like energy output (187,867 MJ ha−1), net energy returns (164,319 MJ ha−1), and energy intensity in economic terms (5.08 MJ ) were calculated and found significantly higher with 120% RDF than control (Table 11), while energy ratio (8.68), energy productivity (0.922 kg MJ−1), and energy profitability (7.68) were highest with 80% RDF followed by 60 and 100%, respectively.
Table 11. Carbohydrate equivalent, carbon output and energy use efficiency of rice as influenced by fertility levels and panchagavya (pooled data of 2 years).
Significantly higher energy output (180,004 MJ ha−1) and net energy returns (159,746 MJ ha−1) were recorded under D4, D3 remaining at par with it. But, the energy ratio (8.91), energy productivity (0.293 kg MJ−1), energy intensity in economic terms (4.97 MJ ), and energy profitability were significantly highest with D4. Among the fertilizer doses and panchagavya applications, the highest energy input was recorded with F4 (23,548 MJ ha−1) and D3 (20,259 MJ ha−1), respectively.
The carbohydrate equivalent yield (4,641 kg ha−1) was recorded highest with 100% RDF, however, carbon output was recorded higher (5,608 kg CO2 eq ha−1) with 120% RDF (Table 11). Among panchagavya applications, D4 resulted in the highest carbohydrate equivalent yield (4,611 kg ha−1) and carbon output (5,345 kg CO2 eq ha−1) over control.
The application of panchagavya increased rice yield during both years of experimentation. Panchagavya contains an appreciable amount of IAA and GA3 (Somasundaram et al., 2003), when sprayed twice along with root dip stimulates plants for increased production of growth regulators in the cell system (Yadav and Lourduraj, 2006). Besides, the beneficial effects of panchagavya could also be attributed to its micronutrient content, higher biological activity, and plant growth-promoting substances (Yadav and Lourduraj, 2006). Improvements in the yield attributes of sunflower, maize, green gram, French bean, and okra have already been reported for a foliar spray of panchagavya (Boomiraj, 2003; Somasundaram et al., 2003; Selvaraj et al., 2007). Panchagavya in different formulations as well as in combinations with various fertility levels brought about significant improvement in both grain and straw yields of rice. D4 treatment (seedling root dip + 6% spray at 30 DAT + application with water at 60 DAT) along with 100% RDF produced higher grain yield and straw yield 60% RDF plus D4 (Table 4). All the vegetative and reproductive characters followed a similar trend.
The performance of any crop in terms of yield depends entirely on its genetic ability (Elizabeth et al., 2007) and the capacity to assimilate the applied nutrients (Chen and Liao, 2017). Integration of an organic nutrient source with chemical fertilizers improves soil ambiance for optimum plant growth (Upadhyay et al., 2018). Thus, the application of panchagavya improves soil health and quality, thereby facilitating plants to extract more nutrients from the soil for transformation in the sink. As a result, a 23.7% yield increase was noticed over control due to the panchagavya application. The stimulatory effect in plant growth due to the release of phytohormones like IAA, GA3, cytokinin with the use of panchagavya in rice (Xu, 2001; Somasundaram and Amanullah, 2007) and several other crops (have been reported in several studies in the past also (Hossain et al., 2007).
The combination of panchagavya and RDF followed quadratic response function for rice grain yield with the successive increment in RDF up to 100% (150, 75, 75, and 5.25 of N, P2O5, K2O, and Zn kg ha−1, respectively) in the present study (Upadhyay et al., 2019), a positive response in seed yield. The optimum economic doses of fertilizer estimated for highest production were 107.3 kg N, 53.7 kg P2O5, and K2O, and 3.8 kg Zn per ha along with panchagavya. The enhanced microbial count, higher microbial biomass carbon, and improved microbial activities were recorded under D4 and higher soil availability of applied nutrients for better uptake and more efficient assimilation along with F3.
A linear relationship was recorded with panchagavya application between RDF and straw yield (Upadhyay et al., 2019). The higher vegetative growth and tillers with 120% RDF and panchagavya application (D4) led to higher straw yield, however, the harvest index remained highest under 100% RDF due to more effective tillers.
The application of graded levels of panchagavya enhanced the N, P, and K concentrations in both grain and straw. The application of panchagavya viz. D4 (seedling root dip + 6% spray at 30 DAT + application with irrigation water at 60 DAT) was found significantly superior to control and D1 for total NPK removal. In addition to major (N, P, and K and calcium) and minor (zinc, iron, copper, and magnesium) nutrients, a high amount of total reducing sugars was also recorded in panchagavya (Yadav and Lourduraj, 2006). Yadav and Kumar (2009) have also observed that various ammonifiers and nitrifiers in the form of chemo-lithotrops and autotrophic nitrifiers in panchagavya inhabit the leaves and increase the ammonia uptake and enhanced total N supply. N supply through panchagavya and recommended fertilizer nutrients ensure a steady and continuous supply of N in the plant. These results are in agreement with the views of Singh et al. (2019). The higher nutrient removal by plants with panchagavya is corroborated with prolonged nutrient availability in soil (Vasanthi and Kumuraswamy, 1999).
Soil available residual N significantly increased by different levels of panchagavya with D4 exhibiting the highest soil available N. In the present study, D4 along with 120% RDF maintained higher N availability in the soil (Figure 2). A steady decomposition of inherent organic nutrients from panchagavya containing chemolithotrops and autotrophic nitrifiers might have enhanced the total N supply in the soil (Papen et al., 2002; Yadav and Kumar, 2009).
Similarly, a higher available soil P was observed with the combined use of panchagavya (D4) and RDF. Various organic acids released by the micro-organisms from panchagavya favor the solubilization of insoluble soil phosphate. The dominant ionic organic compounds in panchagavya compete with the phosphate ion adsorbed by colloidal sites (Pavinato et al., 2008) and clay mineral lattice (Fink et al., 2016) reduce phosphate-fixation in soil. Organic nutrient sources when applied in combination with inorganic fertilizer enhance labile P in the soil by forming a complex with cations like Ca2+ and Mg2+ (Urkurkar et al., 2010). The maximum potassium status in soil was also observed in D4 along with 120% RDF. The application of panchagavya through seedling root dip reduced K-fixation and enhanced K concentration due to positive organic matter and clay interface (Prasad et al., 1997).
The highest microbial activity in terms of bacterial and actinomycetes population was recorded with D4 (Table 6). Panchagavya has abundant N-fixers and P-solubilizers (Sreenivasa et al., 2009) which produce various useful metabolites like, organic acids, and antibiotics (Sangeetha and Thevanathan, 2010). The optimum microbial activity lowers the electrical conductivity of panchagavya and promotes the uptake of salts and ions by soil microbes. All panchagavya preparations increased soil microbial populations and plant growth due to the presence of beneficial rhizospheric microbes in several crops (Radha and Rao, 2014; Shubha et al., 2014). The fluorescent pseudomonas in panchagavya (Bhat et al., 2005) facilitate the synthesis of phytohormones and several other growth-promoting amalgams (Vennila and Jayanthi, 2008). Xu (2001) has also concluded that the soil quality by the application of panchagavya could increase the growth and productivity of crops due to the presence of several beneficial microbes in the rhizosphere.
A significant effect on SMBC was observed with the application of different levels and methods of panchagavya. The highest MBC was recorded with D4 (seedling root dip + 6% spray at 30 DAT + application with irrigation water at 60 DAT), but it remained at par with D3 (three sprays at 15, 30, and 45 DAT @ 6%) and D2 (seedling root dip + 3% spray at 30 DAT + application with irrigation water at 60 DAT). Panchagavya acts as a fine substrate for microbial growth and thus increased MBC (Fraser et al., 1994; Cerny et al., 2008).
Likewise, urease, a vital extracellular enzyme for catalyzing the hydrolysis of urea to ammonia (NH3) and subsequently transforming it to ammonium ( and nitrate (NO) ions (Byrnes and Amberger, 1989). Its activity was recorded higher in D4 in which also improved the nitrogen fertilizer use efficiency.
The dehydrogenase activity was evaluated and was found to be consistently influenced by panchagavay. D4 (seedling root dip + 6% spray at 30 DAT + application with irrigation water at 60 DAT) treatment resulted in the highest dehydrogenase activity. The lowest activity was registered under control among all other treatments indicated the beneficial effect of panchagavya on enzymatic activity in the soil.
Dehydrogenase is a vital enzyme in all viable microbes and its activity is a measure of their vigorous metabolic state (Watts et al., 2010). Dehydrogenase activity (DHA) is thus an important bio-indicators for soil fertility (Wolinska and Stepniewska, 2012). Its activity depends on the microorganisms' abundance and dynamism (Järvan et al., 2014).
Panchagavya as applied in D4 caused a significant improvement in alkaline phosphatase activity which plays a critical role in P cycles (Speir and Ross, 1978) in soil ecosystems. The application of panchagvya also helps in providing P to the crop as it contains a good amount of P. The combined applications of 100% RDF and D4 registered maximum DHA which was higher than the only application of either 100% RDF or D4. The significant interaction of RDF and panchagavya were found in enhancing the activity of urease and alkaline phosphatase.
The energy inflow was primarily influenced by both fertilizer and panchgavya, but more so with panchagavya. Among energy sources, fertilization accounts for the major share of the energy input that directly influences the system's net energy output and energy efficiency (Devasenapathy et al., 2009). The application of panchgavya helps in improving the system's net energy returns and system energy ratio. The decrease in energy input is primarily achieved by avoiding mineral fertilization which is the main component of energy consumption in a conventional production system (Pratibha et al., 2015). The system sustainability and resilience can be further improved with agronomic interventions on crop/cultivars in combination with nutrient management practices.
Based on the experimentation, it can be concluded that the combination of 100% RDF with seedling root dipping in panchagavya followed by one spray @ 6% at 30 DAS and application with irrigation water (15 l ha−1) at 60 DAT resulted in the highest productivity, optimum energy balance and maintaining soil quality. In fact, the results of the present study emphasize that recommended fertilizers application alone remains insufficient in maintaining the productivity, soil quality, and energetics under rice ecology. A rich organic source like panchagavya alone also could not fulfill the demand of the crop. Therefore, the suitable integration of panchagavya and RDF would remain desirable to maintain the agronomic productivity of rice as well as optimum soil health under the semi-arid ecology of middle Indo Gangetic Plains. Further, it is recommended that the technology can be disseminated through a very popular program of the government of India i.e. Parampragat Krishi Yozna for large-scale adoption among the farmers of the South Asian eastern Indo-Gangetic Plains zone.
The original contributions presented in the study are included in the article/supplementary material, further inquiries can be directed to the corresponding author.
PU, ASe, YS, RS, and SP were actively involved in conducting/designing the research and writing the manuscript. RK and KS analyzed the data. VS, BK, AD, RA, SB, RS, SR, KR, and SD interpreted the data and edited manuscript. ASa participated in data collection and laboratory experimentation. All the authors reviewed and revised the manuscript.
The authors declare that the research was conducted in the absence of any commercial or financial relationships that could be construed as a potential conflict of interest.
All claims expressed in this article are solely those of the authors and do not necessarily represent those of their affiliated organizations, or those of the publisher, the editors and the reviewers. Any product that may be evaluated in this article, or claim that may be made by its manufacturer, is not guaranteed or endorsed by the publisher.
We are grateful to the Department of Science and Technology, Government of India for giving monetary assistance for this study. The support of Dr. R. K. Singh (GPB-Professor at Banaras Hindu University, Varanasi) in the analysis of microbial parameters is highly acknowledged.
Balasubramanian, A. V., Vijayalakshmi, K., Sridhar, S., and Arumugasamy, S. (2001). Vrkshayurveda experiments linking ancient texts and farmer practices. COMPAS Magazine. 36, 39.
Beaulah, A.. (2001). Growth and development of moringa (Moringa oleifera Lam.) under organic and inorganic systems of culture (Ph.D. Thesis). Tamil Nadu Agricultural University, Coimbatore.
Bhat, S. S., Vinu, A. K., and Naidu, R. (2005). Association of diverse groups of bacteria with ‘panchagavya' and their effect on growth promotion of coffee seedlings, in: ASIC (Eds), 20th International Conference on Coffee Science, Bangalore, India. p. 1192–98.
Bhatta, R., Kukalb, S. S., Busarid, M. A., Arorac, S., and Yadav, M. (2016). Sustainability issues on rice–wheat cropping system. Int. Soil Water Conserv. 4, 64–74. doi: 10.1016/j.iswcr.2015.12.001
Binning, A. S., Pathak, B. S., and Panesar. (1983). The Energy Audit of Crop Production System Research Report. Panjab, India: School of energy studies for agriculture, PAU, Ludhiana, Panjab, India.
Black, C. A.. (1967). Soil-Plant Relationship. 2nd edition. New York, USA: John Wiley & Sons Publication. p. 515–516.
Boomiraj, K.. (2003). Evaluation of organic sources of nutrients, panchagavya and botanicals spray on Bhendi (Abelmoschus esculentus Moench) (M.Sc. thesis). Tamil Nadu Agricultural University, Coimbatore.
Byrnes, B. H., and Amberger, A. (1989). Fate of broadcast urea in a flooded soil when treated with N-(nbutyl) thiophospherictriamide, a urease inhibitor. Fertilizer Res. 18, 221–231. doi: 10.1007/BF01049572
Cerny, J., Balik, J., Kulhanek, M., and Neded, V. (2008). The changes in microbial biomass C and N in long-term field experiments. Plant Soil Environ. 54, 212–218. doi: 10.17221/393-PSE
Chaudhary, V. P., Gangwar, B., and Pandey, D. K. (2006). Auditing of energy use and output of different cropping systems in India. Agric. Eng. Int. CIGR Ej. 8, 1–13.
Chaudhary, V. P., Gangwar, B., Pandey, D. K., and Gangwar, K. S. (2009). Energy auditing of diversified rice-wheat cropping systems in Indo-gangetic plains. Energy. 34, 1091–1096. doi: 10.1016/j.energy.2009.04.017
Chen, L., and Liao, H. (2017). Engineering crop nutrient efficiency for sustainable agriculture. J. Integr. Plant Biol. 59, 710–735. doi: 10.1111/jipb.12559
Chhonkar, P. K., Bhadraray, S., Patra, A. K., and Purakayastha, T. J. (2007). Experiments in Soil Biology and Biochemistry. New Delhi: Westville.
Datta, M., Yadav, G. S., and Chakraborty, S. (2014). Integrated nutrient management in groundnut (Arachis hypogaea) in a subtropical humid climate of north-east India. Indian J. Agron. 59, 322–326.
Deike, S., Pallutt, B., and Christen, O. (2008). Investigations on the energy efficiency of organic and integrated farming with specific emphasis on pesticide use intensity. Eur. J. Agron. 28, 461–470. doi: 10.1016/j.eja.2007.11.009
Devasenapathy, P., Senthilkumar, G., and Shanmugam, P. M. (2009). Energy management in crop production. Indian J. Agron. 54, 80–90.
Elizabeth, S. D., Ellis, J., Green, A., Llewellyn, D., Morell, M., Tabe, L., et al. (2007). Genetic contributions to agricultural sustainability. Philos. T. R. Soc. B. 363, 591–509. doi: 10.1098/rstb.2007.2172
FAO (2018). Available online at: http://www.fao.org/faostat/en/#data/QC
Fink, J. R., Inda, A. V., Tiecher, T., and Barrón, V. (2016). Iron oxides and organic matter on soil phosphorus availability. Cienc. Agrotec. 40, 369–379. doi: 10.1590/1413-70542016404023016
Fraser, P. M., Haynes, R. J., and Williams, P. H. (1994). Effects of pasture improvement and intensive cultivation on microbial biomass, enzyme activities, and composition and size of earthworm population. Biol. Fert. Soils. 17, 185–190. doi: 10.1007/BF00336320
Gallaher, M., Delhotal, K., and Petrusa, J. (2009). Estimating the potential CO2 mitigation from agricultural energy efficiency in the United States. Energ. Effic. 2, 207–220. doi: 10.1007/s12053-008-9039-1
Geisseler, D., Linquist, B. A., and Lazicki, P. A. (2017). Effect of fertilization on soil microorganisms in paddy rice systems – A meta-analysis. Soil Biol. Biochem. 115, 452–460. doi: 10.1016/j.soilbio.2017.09.018
Gopalan, C., Sastri, R., and Balasubramaniam. (2004). Nutritive value of Indian Foods (Rev. Ed.). Hyderabad, India: National Institute of Nutrition, ICMR. p. 47–57.
Gundogmus, E.. (2006). Energy use on organic farming: A comparative analysis on organic versus conventional apricot production on small holdings in Turkey. Energ. Convers. Manage. 47, 3351–3359. doi: 10.1016/j.enconman.2006.01.001
Hossain, M. S., Mosaddeque, H. Q. M., Alam, M. A., Moniruzzaman, S. M., and Ahmed, I. (2007). Effect of different organic manures and nitrogen levels on yield and yield attributes of Aman rice. Int. J. Sustain. Agri. Tech. 3, 21–26.
Järvan, M., Edesi, L., Adamson, A., and Vosa, T. (2014). Soil microbial communities and dehydrogenase activity depending on farming systems. Plant Soil Environ. 60, 459–463. doi: 10.17221/410/2014-PSE
Jat, M. L., Gathala, M. K., Saharawat, Y. S., Tetarwal, J. P., and Gupta, R. (2013). Double no-till and permanent raised beds in maize–wheat rotation of North–Western Indo-Gangetic Plains of India: effects on crop yields, water productivity, profitability and soil physical properties. Field Crops Res. 149, 291–299. doi: 10.1016/j.fcr.2013.04.024
Kulczycka, J., and Smol, M. (2016). Environmentally friendly pathways for the evaluation of investment projects using life cycle assessment (LCA) and life cycle cost analysis (LCCA). Clean Technol. Envir. 18, 829–842. doi: 10.1007/s10098-015-1059-x
Ladha, J. K., Kumar, V., Alam, M. M., Sharma, S., Gathala, M., Chandna, P., et al. (2009). “Integrating crop and resource management technologies for enhanced productivity, profitability, and sustainability,” in Integrated Crop and Resource Management in the Rice–Wheat System of South Asia, International Rice Research Institute, eds. Ladha, J. K., Singh, Y., Erenstein, O., Hardy, B (Los Baños, Philippines). p. 69–108.
Lal, R.. (2004). Carbon emission from farm operations. Environ. Int. 30, 981–990. doi: 10.1016/j.envint.2004.03.005
Lindsay, W. L., and Norvell, W. A. (1978). Development of a DTPA Soil Test for Zinc, Iron, Manganese, and Copper. Soil Sci. Soc. Am. J. 42, 421–428. doi: 10.2136/sssaj1978.03615995004200030009x
Mandal, S., Roy, S., Das, A., Ramkrushna, G. I., Lal, R., Verma, B. C., et al. (2015). Energy efficiency and economics of rice cultivation systems under subtropical Eastern Himalaya. Energy Sustain. Dev. 28, 115–121. doi: 10.1016/j.esd.2015.08.002
Mansoori, H., Moghaddam, P. R., and Moradi, R. (2012). Energy budget and economic analysis in conventional and organic rice production systems and organic scenarios in the transition period in Iran. Front. Energy. 6, 341–350. doi: 10.1007/s11708-012-0206-x
Mohammadi, A., Rafiee, S., Jafari, A., Keyhani, A., Mousavi-Avval, S. H., and Nonhebel, S. (2014). Energy use efficiency and greenhouse gas emissions of farming systems in north Iran. Renew. Sust. Energ. Rev. 30, 724–733. doi: 10.1016/j.rser.2013.11.012
Natarajan, K.. (2008). Panchagavya A Manual. Kodumudi-Tamil Nadu: Rural Community Action Center (RCAC).
Olsen, S. R. C. V., Watanabe, F. S., and Dean, L. A. (1954). “Estimation of available phosphorus in soil by extraction with sodium bicarbonate USDA Circular 939,” in Fine Method of Soil Analysis, Agronomy, ed Black, C.A. (Madison, Wisconsin: American Society of Agronomy, Inc.).
Papen, H., Gabler, A., Zumbusch, E., and Rennenberg, H. C. (2002). Autotrophic nitrifies in the phyllosphere of a spruce ecosystem receiving high nitrogen input. Curr. Microbiol. 44, 56–60. doi: 10.1007/s00284-001-0074-9
Pavinato, P. S., Merlin, A., and Rosolem, C. A. (2008). Organic compounds from plant extracts and their effect on soil phosphorus availability. Pesqui. Agropecu. Bras. 43, 1379–1388. doi: 10.1590/S0100-204X2008001000017
Perera, F.. (2018). Pollution from Fossil-Fuel Combustion is the Leading Environmental Threat to Global Pediatric Health and Equity: Solutions Exist. Int. J. Environ. Res. Public Health 15, 1–17. doi: 10.3390/ijerph15010016
Prasad, J., Mathur, B. S., and Srivastava, N. C. (1997). Changes in different forms of phosphorus in an acid Alfisols due to long term manuring and fertilization. J. Indian Soc. Soil Sci. 45, 190–192.
Pratibha, G., Srinivas, I., Rao, K. V., Raju, B. M. K., Thyagaraj, C. R., Korwar, G. R., et al. (2015). Impact of conservation agriculture practices on energy use efficiency and global warming potential in rainfed pigeonpea-castor systems. Eur. J. Agron. 66, 30–40. doi: 10.1016/j.eja.2015.02.001
Radha, T. K., and Rao, D. L. N. (2014). Plant growth promoting bacteria from cow dung based biodynamic preparations. Indian J. Microb. 54, 413–418. doi: 10.1007/s12088-014-0468-6
Richards, L. A.. (1954). Diagnosis and Improvement of Saline and Alkali Soils. USDA Handjournal 60, United States Dept. of Agriculture. doi: 10.1097/00010694-195408000-00012
Saha, S., Mina, B. L., Gopinath, K. A., Kundu, S., and Gupta, H. S. (2008). Organic amendments affect biochemical properties of a sub temperate soil of the Indian Himalayas. Nutr. Cycl. Agroecosys. 80, 233–242. doi: 10.1007/s10705-007-9139-x
Sangeetha, V., and Thevanathan, R. (2010). Biofertilizer potential of traditional and panchagavya amended with seaweed extract. Am. J. Sci. 6, 61–67.
Selvaraj, N., Anitha, B., Anusha, B., and Guru, S. M. (2007). Organic horticulture. Udhagamandalam: Horticultural Research Station. Tamil Nadu Agricultural University, Udhagamandalam.
Shubha, S., Devakumar, N., Rao, G. G. E., and Gowda, S. B. (2014). “Effect of seed treatment, panchagavya application and organic farming systems on soil microbial population, growth and yield of maize,” in Proceedings of the 4th ISOFAR Scientific Conference. ‘Building Organic Bridges', at the Organic World Congress 2014, 13-15 Oct. (Istanbul, Turkey).
Singh, B.. (2018). Are nitrogen fertilizers deleterious to soil health? Agronomy. 8, 1–19. doi: 10.3390/agronomy8040048
Singh, V. K., Dwivedi, B. S., Mishra, R. P., Shukla, A. K., Timsina, J., Upadhyay, P. K., et al. (2019). Yields, soil health and farm profits under a rice-wheat system: long-term effect of fertilizers and organic manures applied alone and in combination. Agronomy. 9, 1–22. doi: 10.3390/agronomy9010001
Somasundaram, E., and Amanullah, M. M. (2007). Panchagavya on growth and productivity of crops: A review. Green Farming. 1, 22–26.
Somasundaram, E., Sankaran, N., Meena, S., Thiyagarajan, T. M., Chandaragiri, K., and Pannerselvam, S. (2003). Response of greengram to varied levels of panchagavya (organic nutrition) foliar spray. Madras Agric. J. 90, 169–172.
Speir, T. W., and Ross, D. J. (1978). Soil phosphatase and sulphatase, in Soil enzymes, ed. Burns, R.G. (Academic, London, UK) p 197–250.
Sreenivasa, M. N., Naik, N., and Bhat, S. N. (2009). Beejamrutha; A source for beneficial bacteria. Karnataka J. Agric. Sci. 22, 1038–1040.
Subbiah, B. W., and Asija, G. L. (1973). A rapid procedure for estimation of available nitrogen in soil. Curr. Sci. 25, 259–260.
Swaminathan, M. S., and Bhavani, R. V. (2013). Food production & availability – Essential prerequisites for sustainable food security. Indian J. Med. Res. 138, 383–391.
Tuti, M. D., Prakash, V., Pandey, B. M., Bhattacharya, R., Mahanta, D., Bisht, J. K., et al. (2012). Energy budgeting of colocasia-based cropping systems in the Indian sub-Himalayas. Energy. 45, 986–993. doi: 10.1016/j.energy.2012.06.056
Upadhyay, P. K., Sen, A., Prasad, S. K., Singh, Y., Srivastava, J. P., Singh, S. P., et al. (2018). Effect of panchagavya and recommended dose of fertilizers on growth, nutrient content and productivity of transplanted rice (Oryza sativa) under middle Gangetic plain of India. Indian J. Agric. Sci. 88, 931–936.
Upadhyay, P. K., Sen, A., Rathore, S. S., Kumar, B., Singh, R. K., Prasad, S. K., et al. (2019). Scientific validation of indigenous organic formulation-panchagavya for sustaining rice productivity and residual effect in rice-lentil system under hot semi-arid eco-region of middle Indo-Gangetic plains. Indian J. Tradit. Know. 18, 104–113. Available online at: http://nopr.niscair.res.in/handle/123456789/45677
Urkurkar, J. S., Tiwari, A., Chitale, S., and Bajpai, R. K. (2010). Influence of long-term use of inorganic and organic manures on soil fertility and sustainable productivity of rice (Oryza sativa) and wheat (Triticum aestivum) in Inceptisols. Indian J. Agric. Sci. 80, 208–212.
Vasanthi, D., and Kumuraswamy, K. (1999). Efficacy of vermicompost to improve soil fertility and rice yield. J. Indian Soc. Soil Sci. 47, 268–272.
Vennila, C., and Jayanthi, C. (2008). Effect of integrated nutrient management on yield and quality of okra. Res. Crops. 9, 75–75.
Walkley, A., and Black, C. A. (1934). An examination of the Degitjareff method for determining soil organic matter, and a proposed modification of the chromic acid titration method. Soil Sci. 37, 29–38. doi: 10.1097/00010694-193401000-00003
Watts, D. B., Torbert, H. A., Feng, Y., and Prior, S. A. (2010). Soil microbial community dynamics as influenced by composted dairy manure, soil properties, and landscape position. Soil Sci. 175, 474–486. doi: 10.1097/SS.0b013e3181f7964f
Wolinska, A., and Stepniewska, Z. (2012). Dehydrogenase activity in the soil environment, in Dehydrogenases, eds. Canuto, R.A. (Intech, Rijeka).
Xu, H. L.. (2001). Effects of a microbial inoculant and organic fertilizers on the growth, photosynthesis and yield of sweet corn. J. Crop Prod. 3, 183–114. doi: 10.1300/J144v03n01_16
Yadav, B. K., and Lourduraj, C. A. (2006). Effect of organic manures and panchagavya spray on rice (Oryza sativa L.) quality. Crop Res. 32, 6–10.
Yadav, D. S., and Kumar, A. (2009). Long term effect of nutrient management on soil health and productivity of rice-wheat system. Indian J. Agron. 54, 15–23.
Keywords: carbohydrate equivalent, dehydrogenase, energy, microbial population, panchagavya, SMBC, urease
Citation: Upadhyay PK, Sen A, Singh Y, Singh RK, Prasad SK, Sankar A, Singh VK, Dutta SK, Kumar R, Rathore SS, Shekhawat K, Babu S, Singh RK, Kumar B, Dey A, Rajanna GA and Kulshekaran R (2022) Soil Health, Energy Budget, and Rice Productivity as Influenced by Cow Products Application With Fertilizers Under South Asian Eastern Indo-Gangetic Plains Zone. Front. Agron. 3:758572. doi: 10.3389/fagro.2021.758572
Received: 14 August 2021; Accepted: 03 December 2021;
Published: 20 January 2022.
Edited by:
Saddam Hussain, University of Agriculture, PakistanReviewed by:
Jeetendra Prakash Aryal, International Maize and Wheat Improvement Center, MexicoCopyright © 2022 Upadhyay, Sen, Singh, Singh, Prasad, Sankar, Singh, Dutta, Kumar, Rathore, Shekhawat, Babu, Singh, Kumar, Dey, Rajanna and Kulshekaran. This is an open-access article distributed under the terms of the Creative Commons Attribution License (CC BY). The use, distribution or reproduction in other forums is permitted, provided the original author(s) and the copyright owner(s) are credited and that the original publication in this journal is cited, in accordance with accepted academic practice. No use, distribution or reproduction is permitted which does not comply with these terms.
*Correspondence: Vinod Kumar Singh, dmt1bWFyc2luZ2hfMDFAeWFob28uY29t
Disclaimer: All claims expressed in this article are solely those of the authors and do not necessarily represent those of their affiliated organizations, or those of the publisher, the editors and the reviewers. Any product that may be evaluated in this article or claim that may be made by its manufacturer is not guaranteed or endorsed by the publisher.
Research integrity at Frontiers
Learn more about the work of our research integrity team to safeguard the quality of each article we publish.