- 1Department of Biology, University of British Columbia Okanagan, Kelowna, BC, Canada
- 2Department of Biology, University of Ottawa, Ottawa, ON, Canada
- 3Agriculture and Agri-Food Canada, Ottawa Research and Development Centre, Ottawa, ON, Canada
- 4Department of Plant Sciences, University of Saskatchewan, Saskatoon, SK, Canada
There is a global industry built upon the production of “bioinoculants,” which include both bacteria and fungi. The recent increase in bioinoculant uptake by land users coincides with a drive for more sustainable land use practices. But are bioinoculants sustainable? These microbes are believed to improve plant performance, but knowledge of their effect on resident microbial communities is scant. Without a clear understanding of how they affect soil microbial communities (SMC), their utility is unclear. To assess how different inoculation practices may affect bioinoculant effects on SMC, we surveyed the existing literature. Our results show that bioinoculants significantly affect soil microbial diversity and that these effects are mediated by inoculant type, diversity, and disturbance regime. Further, these changes to soil microbes affect plant outcomes. Knowledge that these products may influence crop performance indirectly through changes to soil microbial diversity attests to the importance of considering the soil microbiome when assessing both bioinoculant efficacy and threats to soil ecosystems.
Introduction
Bioinoculants are soil additives composed primarily of fungal and/or bacterial isolates, and occasionally contain other abiotic additives (i.e., nutrients, or inorganic/organic carriers) (Figure 1). They are deliberately applied to soil or plants to improve plant nutrient status (Kang et al., 2014; Singh et al., 2019) especially where local soil microbial activity has been reduced by anthropogenic activity (Ohsowski et al., 2012; Berruti et al., 2017), heavy metal (Ahemad, 2019) and pesticide pollution (Verma et al., 2014), and biocontrol of pathogens (Wu et al., 2017) (Figure 1).
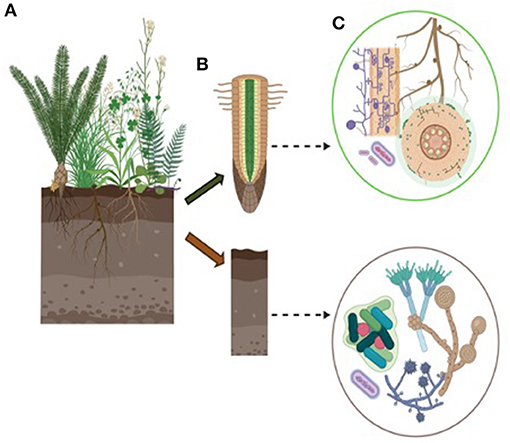
Figure 1. Plants interact with a plethora of rhizosphere and endophytic microbes. These microbes, mainly bacteria and fungi, can positively affect plant development and performance, especially under abiotic and biotic stressful conditions. (A) Phytostimulation, which is the direct stimulation of the plant growth via the expression of phytohormones as seen with phytohormones as seen with several species from the Bacillus (Singh et al., 2019) and Pseudomonas genus (Kumari et al., 2016). (B) Accession of essential nutrients by acting as biofertilizers, as seen with the nitrogen-fixing and phosphate-solubilizing bacteria or the mycorrhizal fungi, (C) Defense against herbivory and pathogens by acting as biological control agents, such as the fungi Trichoderma (Elad, 2000), Penicillium (Rani et al., 2007), or the bacterial genus Bacillus (Pérez-García et al., 2011). In an attempt to harness these benefits, propagules are applied intentionally in the soil, roots, or seeds.
While questions remain about the use of these products (Kokkoris et al., 2019a; Thomsen et al., 2021), their effects in natural ecosystems including their invasiveness and interactions with the indigenous soil microbes have received little attention (Trabelsi and Mhamdi, 2013; Ambrosini et al., 2016; Hart et al., 2017). Many studies include information about soil microbial communities (SMC) post-inoculation, but there is little consensus on the effect of inoculants on SMC diversity. For example, inoculation with fungal inoculants can decrease (Koch et al., 2011; Symanczik et al., 2015; Islam et al., 2021), increase (Albertsen et al., 2006), or have no effect on resident fungal diversity (Antunes et al., 2009; Jin et al., 2013; Werner et al., 2014). This inconsistency also exists for bacterial inoculants; for example, studies found no difference in resident bacterial communities post bacterial inoculation (Lottmann et al., 2000; Piromyou et al., 2011; Chowdhury et al., 2013) while other studies showed bacterial inoculants increased (Gupta et al., 2014; Pang et al., 2017; Dong et al., 2019) and decreased bacterial diversity (Zhang et al., 2010).
A framework allowing producers to make informed decisions surrounding bioinoculant use is needed. In order to understand how bioinoculants affect SMC and plant hosts under different conditions, we identified six questions to understand the potential for inoculants to influence SMC.
Do Bioinoculants Affect Soil Microbial Communities?
Inoculation may affect SMC multiple ways. If the bioinoculant establishes, SMC may increase, at least by one taxon. While studies exist showing that bioinoculants can establish (Pellegrino et al., 2012; Sýkorová et al., 2012), given the complexity and diversity of most soil ecosystems, it may not be possible to determine small changes in species richness as result of our ability to measure small changes in SMC. Beyond additive effects, inoculation may increase biodiversity by outcompeting dominant taxa, thereby facilitating competitive release (Hardin, 1960; Mawarda et al., 2020). Alternatively, an inoculant may promote the colonization of taxa already present in the species pool if its establishment increases resource availability (Kang et al., 2014; Singh et al., 2019) or if it ameliorates stress (Irshad et al., 2020; Vahter et al., 2020). Conversely, inoculation may reduce microbial diversity if the inoculant becomes dominant and suppresses native taxa (Gomes et al., 2005; 2017) but the conditions under which this may occur are not yet known.
Do Fungal and Bacterial Inoculants Have Different Effects on SMC?
It may be that resident fungal communities are inherently more susceptible to changes in community structure post-inoculation. First, fungal communities are typically less diverse than bacterial communities (Li et al., 2016; Wang et al., 2018; Liu et al., 2020), which may predispose them to perturbation by an invader (Knops et al., 1999; Stachowicz et al., 1999; Naeem et al., 2003). Additionally, the superior dispersal ability of bacteria compared to fungi (Schmidt et al., 2014; Ma et al., 2017; Vannette et al., 2020) is more likely to create cosmopolitan bacterial communities that may already contain similar or closely related taxa to the inoculants, reducing the possibility for intense competition (Sommaruga and Casamayor, 2009; O'Brien et al., 2016).
Does Inoculum Diversity Affect SMC Response?
The diversity of the bioinoculant consortia may determine impacts on resident SMC. Inoculants including more than one taxon may increase the likelihood that one of the isolates is able to establish in the new environment. Studies show a consortium has a greater effect on resident microbial diversity than single inoculum (Anandaraj and Leema Rose Delapierre, 2010; Bharti et al., 2016; Ju et al., 2019). Still, others show that a single inoculant is more effective (Lladó et al., 2012).
Does Disturbance Regime Affect Bioinoculant Effects on SMC?
Inoculants in disturbed systems (i.e., agricultural settings) may have a greater effect on resident microbes. The system may be depauperated of its soil microbes by disturbance, increasing the likelihood of bioinoculant establishment (Gomes et al., 2005; Antunes et al., 2009). Soil disturbance may put bioinoculants on even footing with residents as all may be required to re-establish from propagules (Ketola et al., 2017; Albright et al., 2020). Commercial bioinoculant establishment may be further enhanced in disturbed sites by virtue of the manner in which they are produced, such as highly artificial, in vitro production systems. Industrial conditions such as these may impose selective pressures on the bioinoculants for a more ruderal life history (Kokkoris et al., 2019b). Such conditions may promote microbes that are more tolerant to physical disturbance (Jack et al., 2021). If so, such disturbance tolerant bioinoculants may have more pronounced effects on residents.
Does Experiment Setting Affect SMC Response to Bioinoculants?
Inoculation effects in greenhouse studies may be more pronounced than under field inoculations simply because bioinoculant establishment may be enhanced in greenhouse. Greenhouse studies report greater bioinoculant establishment (Lekberg and Koide, 2005; Martínez-Viveros et al., 2010) likely because there are fewer factors inhibiting establishment. Additionally, greenhouse studies often have one plant per pot (Pallez et al., 2002; Kawaletz et al., 2014), which might encourage a symbiosis that may occur under natural, field conditions. Further, greenhouse soil is typically sterilized, allowing for easy bioinoculant establishment (Martínez-Viveros et al., 2010). Thus, changes to SMC may be more pronounced simply due to increased likelihood of bioinoculant establishment.
Does Inoculation Affect Plant Performance?
Regardless of unintended consequences on SMC, the goal of inoculation is to improve plant performance. However, soil microbial diversity is also an important determinant of plant performance and productivity (van der Heijden et al., 1998; Mangan et al., 2010; Bardgett and Van Der Putten, 2014). Thus, if bioinoculant use results in a change to resident soil diversity, plant performance may be affected indirectly by the bioinoculant. This could reduce plant performance/productivity if SMC diversity decreases (Chen et al., 2020) or, it may enhance plant performance if SMC diversity is enhanced (van der Heijden et al., 1998). Alternatively, changes to plant performance could be due to direct effects of the bioinoculant. For example, the same isolate of fungi can be beneficial on some plant genotypes (Oliveira et al., 2017; Garg and Singh, 2018; Le Pioufle et al., 2019), negative on others (Janoušková et al., 2013; Symanczik et al., 2015; Loján et al., 2017), or have no effect on yet others (Rosa et al., 2020). This is particularly true for microbes with strict host requirements, such as rhizobia (Rebah et al., 2002; Sanz-sáez et al., 2015; Adissie et al., 2020). Whatever the outcome, the connection between plant performance and SMC diversity is paramount to ecosystem stability and resilience.
In this study, we asked whether there is evidence for SMC changes following bioinoculant use. We surveyed the literature for all studies reporting SMC response to an inoculation event. We predicted that SMC response would depend on the type of community being assayed (fungal or bacterial), the identity of the bioinoculant, the level of disturbance and the response of the target plant to inoculation.
Methods
Our approach was based on standard protocols described in Moher et al. (2015) and Gurevitch et al. (2018).
Literature Search
To answer the question of whether bioinoculants influence SMC, scientific articles were collected from Web of Science (Web of Science [v.5.35], 2021) in June of 2019 using the search terms (Microb* AND Inocul* NOT pesticid* NOT insecticid* AND soil AND bacteri* AND fung* AND communit* NOT review*) which could be within the title or the abstract of the paper. This returned 445 papers.
For analysis purposes, we included only studies that measured changes in the diversity of bacterial or fungal communities. We included multiple cases from individual studies if those cases represented either a different response variables (i.e., bacterial and fungal diversity), different bioinoculants, or different conditions (e.g., disturbed vs. undisturbed). In all cases, where responses were measured as a time series, we only used the final measurement. We also excluded other cases based on specific modifiers. For bioinoculant type, we included only cases where either fungi or bacteria were inoculated. We included inoculants for all purposes, including growth promotion and biocontrol. Inoculants containing both bacteria and fungi, or other microbes were too rare to allow reliable inference. For disturbance, we classified all disturbances as either chemical or physical. These included both agricultural and industrial disturbances. Disturbances that could not be classified as such were excluded. For experimental system, we excluded all cases that could not be classified as field, greenhouse/growth chamber, or laboratory microcosm. This resulted in a total of 243 cases. Not all studies included plants and plant responses were measured variously among studies. For analyses including plant response data, we include only studies where plant responses could be plausibly associated with potential fitness (e.g., survival, growth, yield, defense, etc.). This limited the number of cases to 143.
Statistical Analysis
To determine how bioinoculants affect SMC, we analyzed the data from the systematic review in two stages. First, we tested whether the bioinoculants caused a significant change in the diversity of bacterial or fungal communities. Second, we tested whether the changes were more likely to be positive or negative for those studies where a significant effect was detected. In both cases, the response variables were binary. The statistical models used differed depending on whether moderators were included. In absence of moderators, we used chi-square tests to determine whether significant responses were more likely than expected by chance. When moderators were included, we used binomially distributed generalized linear models that included the moderator as a factor. We use these models, rather than more typical meta-analysis models, because we did not include estimates of effect size or of variation in the analysis due variation in methods used to estimate changes to SMC, which included different diversity metrics. Separate models were run for bacterial and fungal communities because fungal studies were much less prevalent, which restricted our ability to include certain modifiers. We also ran separate models to test the effect of each modifier as data from certain modifiers was unavailable for some studies. These results, however, did not differ from models where all modifiers were included (Supplementary Tables 1–3). For bacteria, we included bioinoculant type (bacterial or fungal), inoculum diversity (single species or mixed), disturbance (chemical, physical, or undisturbed), and experimental system (field, greenhouse, or microcosm). For fungi, we were only able to include bioinoculant type and experimental system as modifiers. Most analyses used base R (R Core Team, 2021); however, we used the package “emmeans” (Lenth, 2021) to test for pairwise differences among categories of disturbance and experimental setting when the main effects was significant.
In addition to models testing bioinoculant effects on microbial diversity, we tested whether changes in either fungal or bacterial diversity were associated with changes in plant performance, with fungi and bacteria analyzed separately as before. In these cases, the response variables were ordinal: either a reduction in plant performance, no effect, or an increase in plant performance. Thus, we used ordinal logistic regression in the R package “ordinal” (Christensen, 2019) to account for the data type. In these models, we used whether bacterial or fungal diversity changed or whether that change was positive or negative as the factors in the model.
Results
Do Microbial Inoculants Affect Microbial Communities?
Fungi Response
Fungal diversity was not more likely to change following inoculation (Figure 2A). That is, we observed equal number of instances showing change and no change (χ2 = 0, P = 1). Among cases where fungal diversity did change, it was more likely to increase than decrease following inoculation, with fungal diversity increasing in 23 studies vs. eight where diversity decreased (χ2 = 7.2581, P = 0.007058).
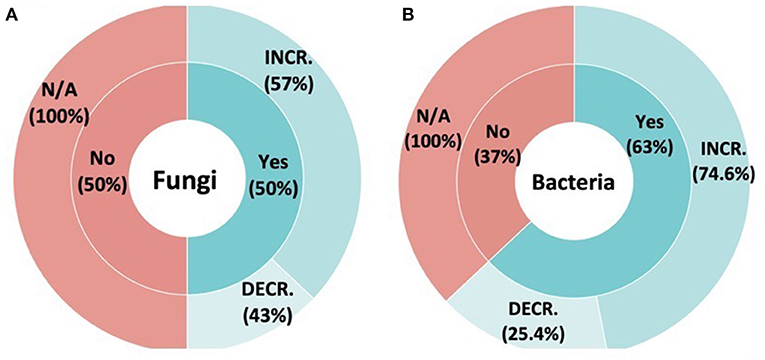
Figure 2. The effect of inoculation on the native (A) fungal and (B) bacterial communities. The inner rings demonstrate whether inoculation affects the native microbial communities' diversity. The outer rings demonstrate the directional change on SMC diversity (increase vs. decrease) in those samples that were affected by inoculation.
Bacteria Response
Bacteria diversity was more likely to change following inoculation (Figure 2B) That is, we observed more instances showing diversity increase (114) compared to no change (67) and no change (χ2 = 12.20, P < 0.001). Among studies where change was observed, bacteria diversity was more likely to increase, with 85 studies reporting increases vs. 29 where bacterial diversity decreased (χ2 = 27.51, P = P < 0.001).
Do Fungal and Bacterial Inoculants Have Different Effects on SMC?
Fungi Response
We did not detect an effect of inoculum type on fungal diversity changes (Figure 3A). That is, no more studies showed fungal diversity changes when bacterial inoculants were used (44%) compared to fungal inoculants (43%) (F = 2.478, P = 0.120). Among cases where diversity changed following inoculation (Figure 3B), inoculum type did not affect the direction of the change, with 79% of studies showing increased diversity for bacterial inoculants, vs. 70% for fungal inoculants, but this difference was not significant (F = 0.24, P = 0.620).
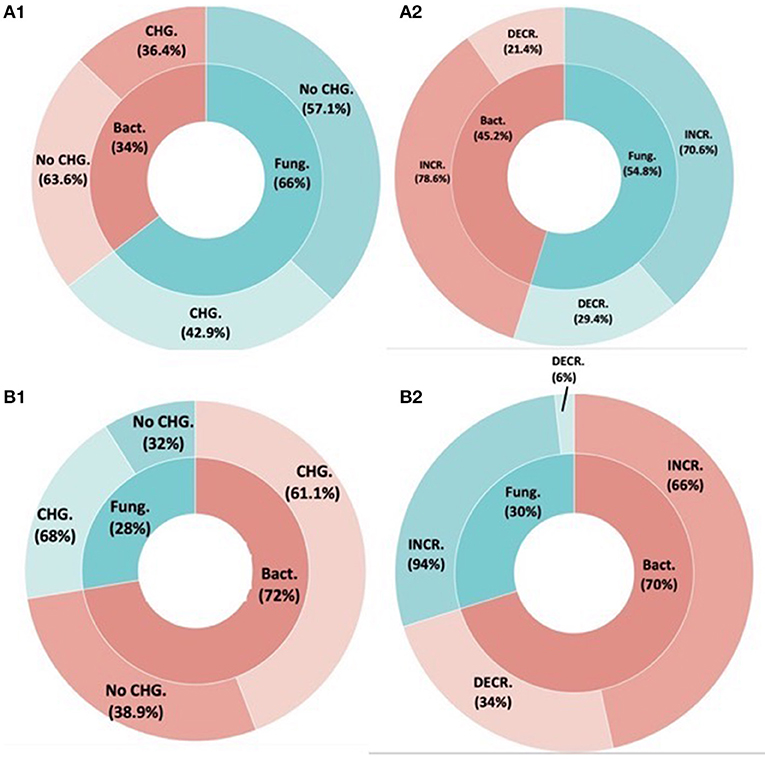
Figure 3. Effect of bacterial and fungal inoculants on the native (A) fungal and (B) bacterial communities. (A1,B1) The inner rings demonstrate the inoculant identity (bacterial or fungal). The outer rings demonstrate the proportion of studies that found a change or did not find a change on the resident fungal and bacterial communities. (A2,B2) Boxed pie charts demonstrate the directional community changes per inoculant type (blue = fungal inoculants and red = bacterial inoculant) for the studies that showed SMC changes following inoculation.
Bacteria Response
We did not detect an effect of inoculum type on bacterial diversity changes (Figure 4A). That is, no more studies showed bacterial diversity changes when bacterial inoculants were used (61%) compared to fungal inoculants (68%) (F = 0.747, P = 0.3885). Among cases where bacterial diversity changed (Figure 4B), bacterial inoculants increased bacterial diversity in only 66% of studies, whereas fungal inoculants increased bacterial diversity in 94% of studies (F = 11.58, P = 0.0009248).
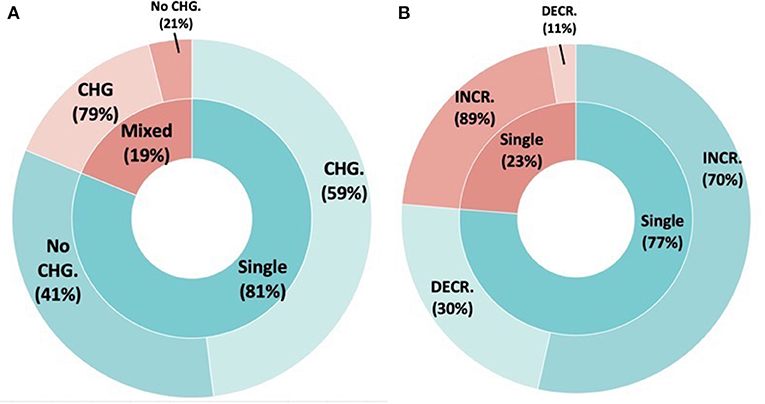
Figure 4. The effect of inoculum diversity on the resident bacterial communities. (A) The inner rings demonstrates bioinoculant diversity category (single species vs. mixed species). The outer ring demonstrates the proportion of studies that found on the resident bacterial community diversity. (B) Boxed pie chart demonstrates the directional community changes (increased vs. decline) per inoculant type (blue = single species inoculants and red = mixed species inoculants) for the studies that showed SMC changes following inoculation.
Does Inoculum Diversity Affect SMC Response?
Bacteria Response
Bacterial diversity was more likely to change when using a mixed inoculum (79% of cases) vs. a single species inoculum (59% of cases) (F = 5.144, P = 0.0245). Among cases where bacterial diversity changed, bacterial diversity was more likely to increase regardless of inoculum type, but more so for single species inoculum (89% studies showed increases for single species inocula compared to 70% of cases using mixed inocula) (F = 4.27, P = 0.04119).
There were too few fungi studies with mixed inoculum to test the effect of inoculum diversity.
Does Disturbance Regime Affect Bioinoculant Effects on SMC?
Bacteria Response
While the incidence of diversity changes associated with disturbance was higher for chemical (64%) and physical disturbance (81%) compared to no disturbance, these differences were not statistically significant (F = 1.0475, P = 0.353) (Figure 5). When we looked at only the cases where diversity changes were detected (Figure 4B), inoculation increased bacterial diversity in 80 and 77% of the cases for no disturbance and chemical disturbance, but decreased bacterial diversity in 89% samples experiencing physical disturbance (F = 8.7935, P = 0.0002851). Whereas, there was no difference in the response between no disturbance and chemical disturbance (z ratio = 0.359, P = 0.9313), physical disturbance caused significant reductions in bacterial diversity compared to no disturbance (z ratio = 3.202, P = 0.0039), and chemical diversity (z ratio = 2.808, P = 0.0138).
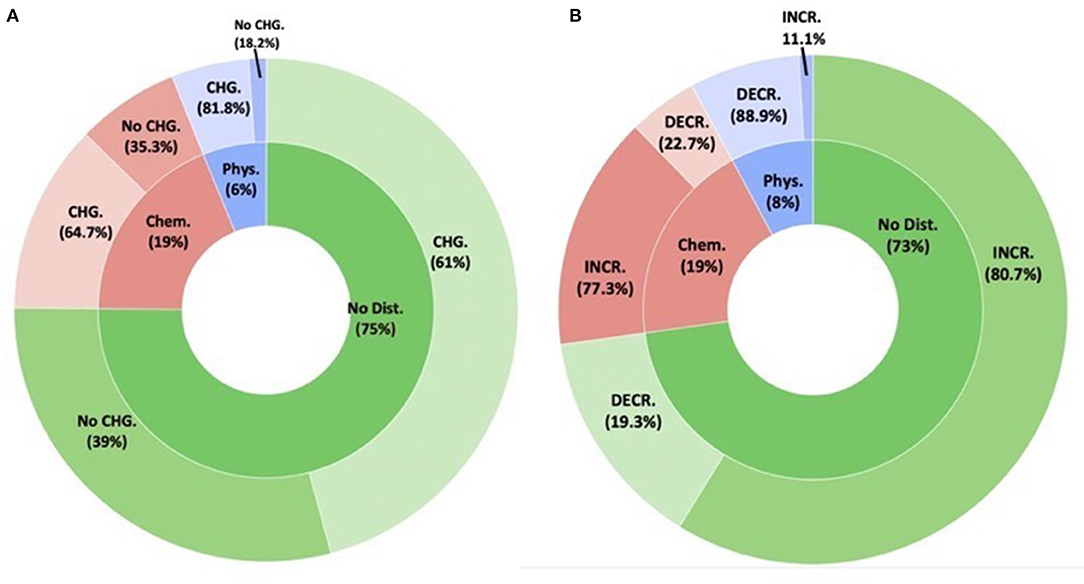
Figure 5. The effect of inoculation on the resident bacterial communities in the presence of disturbance. (A) The inner ring demonstrates the disturbance type (physical disturbance, chemical disturbance, and no disturbance). The outer rings demonstrates the proportion of studies that showed bacterial community changes following inoculation. (B) Boxed pie chart demonstrates the directional community changes (increased vs. decline) per disturbance type for the studies that showed SMC changes following inoculation.
There were too few fungi studies with mixed inoculum to test the effect of inoculum diversity.
Does Experiment Setting Affect SMC Response to Bioinoculants?
Fungi Response
Inoculation changed fungal diversity more often in greenhouse (81%) compared to field (54%) and microcosms (22%) (F = 8.8211, P = 0.000445) (Figure 6). While there was no statistical difference in the effect among Field and Greenhouse studies (z ratio = −1.721, P = 0.1972) or Field and Microcosm studies (z ratio = 1.944, P = 0.1265), greenhouse and microcosm studies were statistically different (z ratio = 3.24, P = 0.0004). When we looked at only those cases where diversity changed, diversity generally increased. There were no significant differences among settings (F = 0.6109, P = 0.441) despite equal numbers of positive and negative cases in microcosms, potentially due to the small number of microcosm studies (N = 6).
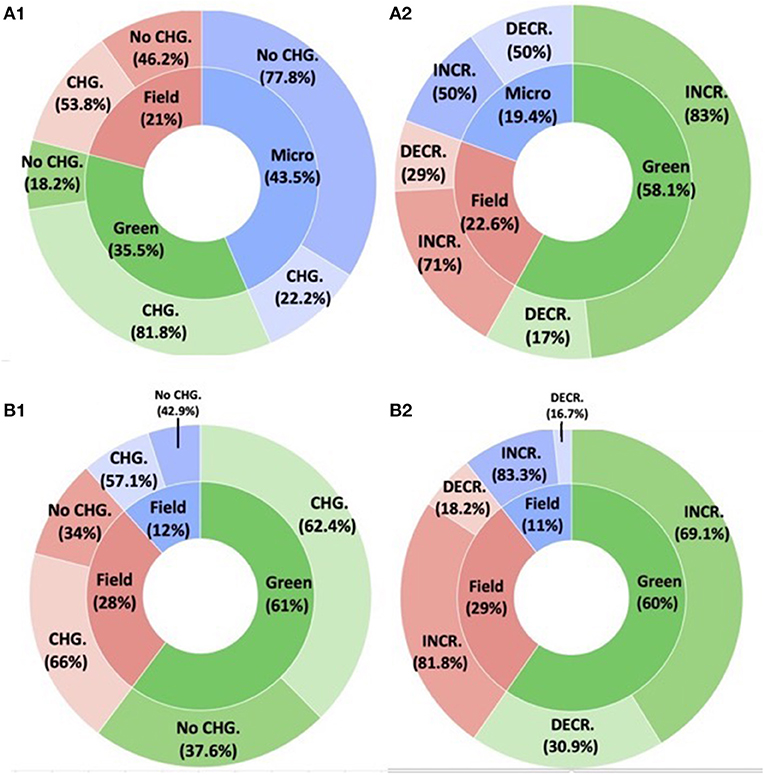
Figure 6. The role of the environment to the inoculation effect on the native (A) fungal and (B) bacterial communities. (A1,B1) The inner pies demonstrate the environment category (blue = microcosm red = field and green = greenhouse). The outer rings demonstrate the number of studies that found a change or did not find a change on the (A1) native fungal and on the (B1) native bacterial community diversity. (A2,B2) Boxed pie charts demonstrate the directional community changes (increased vs. decline = outer ring) per environment category (blue = microcosm red = field and green = greenhouse) for the studies that showed SMC changes following inoculation.
Bacteria Response
Experimental setting (Greenhouse/microcosm/field) did not change the effect of inoculation on bacterial diversity (F = 0.310, P = 0.740), with all scenarios showing diversity changes in approximately 62% of studies.
Does Inoculation Affect Plant Performance?
Fungi
Plant performance changed in response to fungal inoculation in 75% of cases, but this was not dependent on whether fungal diversity changed (F = 0.6498, P = 0.4292) (Figure 7A). Similarly, the direction of fungal diversity change was not strongly associated with plant responses (F = 3.1714, P = 0.0966). The lack of significant associations may be attributable to the lack of negative plant responses and small number of neutral plant responses to fungal inoculation (N = 7; N = 4 when considering cases where fungal diversity changed). When we looked only at cases where fungal diversity changed, a similar pattern emerged for both fungal diversity decreases and increases. The most common response was increased fungal diversity and positive plant response (F = 3.1714, P = 0.0966).
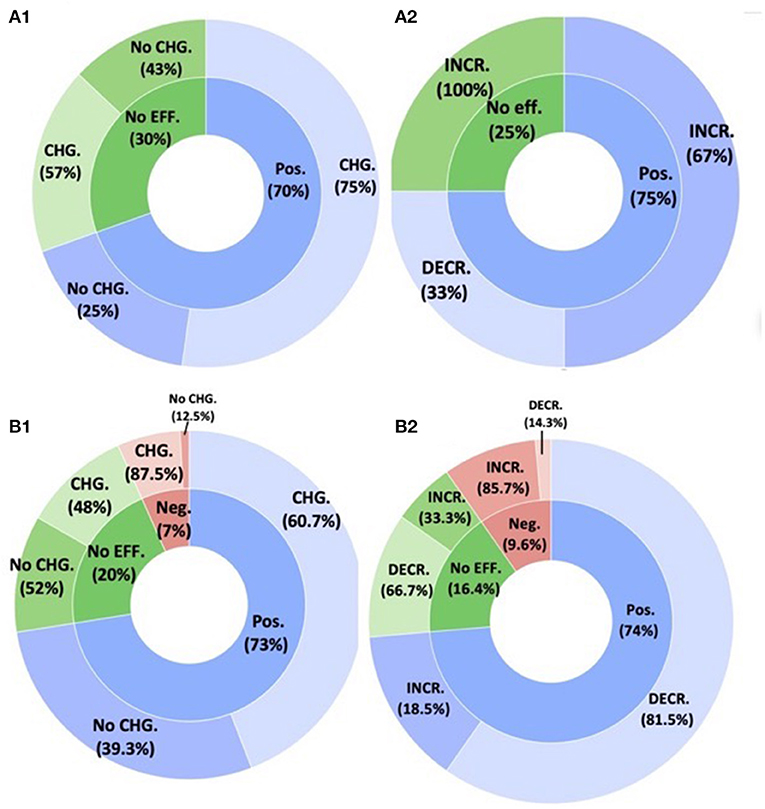
Figure 7. The effect of the native (A) fungal and (B) bacterial community changes in plant performance post inoculation. (A1,B1) The inner rings demonstrate plant response (positive, negative, or no response). The outer rings demonstrate the proportion of studies that showed SMC changes following inoculation (A1) resident fungal and (B1) resident bacterial community. (A2,B2) Boxed pie charts demonstrate the directional community changes (increased vs. decline) per environment category (microcosm, field, or greenhouse) for the studies that showed SMC changes following inoculation.
Bacteria
For bacteria, 88% of studies showed negative plant responses were associated with bacterial diversity changes, compared with only 61% of plants showing positive changes, and 48% of plants showing no effects of inoculation (F = 3.892, P = 0.02198) (Figure 7B). When we looked only at cases where bacterial diversity changed, the most common response was increased bacterial diversity and positive plant response (F = 5.5004, P = 0.02189). However, in this case plants showing decreased response were more commonly associated with decreases in bacterial diversity.
Discussion
Do Microbial Inoculants Affect Microbial Communities?
Inoculation affected SMC but fungal and bacterial communities responded differently to inoculation. Fungal communities were equally likely to change or not following inoculation. This is supported by the literature as there is evidence for fungal communities being resistant (Aung et al., 2013; Werner et al., 2014), resilient (Antunes et al., 2009; Karpouzas et al., 2011), and susceptible to invasion (Schmidt et al., 2012; Symanczik et al., 2015; Janoušková et al., 2017).
Bacterial communities, in contrast, were more likely show increased diversity following inoculation. Basic differences between bacterial and fungal ecology may play a role in this difference. There may simply be more, unestablished taxa in bacterial communities, which, in contrast to fungi, are typically not dispersal limited (Schmidt et al., 2014; Ma et al., 2018; Vannette et al., 2020). This means that perturbations via inoculation may allow for the recruitment of heretofore unestablished bacterial taxa. Similarly, differences in reproduction (i.e., sexuality is present in fungi but not bacteria) may mean that fungi are not able to capitalize upon altered conditions as quickly as bacteria (Rousk and Bååth, 2011; Kirchman, 2012). Additionally, faster growth rates and larger populations sizes of bacteria compared to fungi mean there may be more opportunity for novel mutations able to quickly adapt to changes to the environment (Hibbing et al., 2010). As well, soil fungal communities tend to be more closely aligned with plant communities both for mutualists (Cassman et al., 2016; Zhang et al., 2021) and saprobes (Francioli et al., 2020), thus we may expect less change in their communities without concurrent changes to vegetation (van der Heijden et al., 1998; Beck et al., 2020).
Do Fungal and Bacterial Inoculants Have Different Effects on SMC?
Fungal communities were equally affected by fungal and bacterial inoculants (Figure 3A). It may be that inoculation itself, either due to the physical disruption of the soil, or the co-amendment with carriers and nutrients is drives changes to fungal communities. There is ample evidence that soil disturbance (Rodriguez-Ramos et al., 2021) and soil amendments (Ezawa et al., 2002; Lucas et al., 2014) can increase fungal diversity. Whatever the mechanism, these results suggest that inoculant identity (bacterial vs. fungal) is not an important factor in determining inoculation effects on SMC.
Similarly, bacterial residents were likely to be affected by bacterial and fungal inoculants (Figure 3B). In this case, however, there were directional changes associated with the different bioinoculants. Fungal inoculation led to increased bacterial diversity, which supports the idea that fungal inoculants act as a novel resource. There are many studies showing the hyphosphere as hosting a diverse community of bacteria, both in and on the mycelia network (Marschner and Baumann, 2003; Rillig et al., 2006). For example, Scheublin et al. (2010) showed that there are distinct bacterial communities that adhere to hyphal surfaces which differ from those found in the surrounding soil. These hyphal communicates were enriched with members of the Oxalobacerteaceae. Bacterial inoculants, conversely, seem to have less of an effect on bacterial communities despite the addition of new bacterial species. This suggests that bacterial inoculants may not alter the carrying capacity of an environment sufficiently to allow for the establishment of new taxa.
Does Inoculum Diversity Affect SMC Diversity?
Single taxon inoculants had the biggest effect on bacterial communities (Figure 3). This is contrary to our prediction that more diverse inoculants should have a greater chance of containing a taxon likely to establish (Huston, 1997; Tilman, 1997; Hooper et al., 2005). It may be that competition among the taxa in the mixed formulations may inhibit colonization of novel resident taxa, given that most bioinoculant taxa are chosen or are grown/incubated before inoculation in conditions that experience little disturbance, competition, or stress which might inadvertently select for ruderal growth habits (Fortin et al., 2005; Litchman, 2010; Van Elsas et al., 2012). Such differences in microbial life history strategies can manifest as differences in SMC. For example, Leff et al. (2015) showed that soil bacterial and fungal communities differed consistently across grassland sites in response to nutrient addition.
It is possible that interactions among consortia members may inhibit bioinoculant establishment. Consortia are typically formulated based on individual taxon effects (Sarma et al., 2015; Niu et al., 2020). This approach may result in bioinoculants with decreased effects (Sarma et al., 2015), and interactions among consortia members may inhibit each other (Haas and Défago, 2005). Clearly more work is needed to understand how interactions among bioinoculant consortia affect establishment, and interactions with resident SMC.
Does Disturbance Regime Affect Bioinoculant Effects?
Because soil disturbance is known to be associated with changes in bacterial (Wang et al., 2010; Silva et al., 2013; Sengupta and Dick, 2015; Zhang et al., 2019) and fungal (Schnoor et al., 2011; Li et al., 2012; Chen et al., 2014) communities, we predicted inoculation would have a greater impact on microbial communities that had been subjected to soil disturbance. While there were too few studies looking at fungal community responses, we found that inoculation with chemical disturbance led to increased bacterial diversity, but inoculation with physical disturbance decreased bacterial diversity.
In our study, soil disturbance increased the likelihood that inoculation would suppress resident bacterial communities. This may be in part due to physical disturbance destabilizing the resident diversity via changes in abiotic conditions (Schimel et al., 2007; Wang et al., 2016, 2017; Naylor and Coleman-Derr, 2018). This decrease in diversity might enhance the chances of establishment of a bioinoculant (Litchman, 2010; Van Elsas et al., 2012; Yang et al., 2017) which on account of their superior competitive abilities (Litchman, 2010; Van Elsas et al., 2012; Kaminsky et al., 2019) might allow for further effects on the already weakened resident community.
Does Experimental Setting Affect SMC Response to Bioinoculants?
Because greenhouse studies do not accurately represent soil ecosystems, some variation in SMC response to inoculation could be due to differences between greenhouse and field studies. We predicted diversity changes would be enhanced in greenhouse studies vs. field because there is evidence that simpler systems are more invasible (Stachowicz et al., 1999; Bonanomi et al., 2014). In our study, inoculation increased fungal diversity more often in greenhouse studies compared to field and microcosm studies, but only field and microcosm studies were statistically different. This was surprising as we expected microcosms to represent conditions intermediate to field and greenhouse studies, and many of them lacked plants. It may be that microcosms studies are more closely aligned with greenhouse studies in terms of SMC as they typically must disturb soil (e.g., autoclaved or sieved).
For bacterial communities, study location (greenhouse vs. field) had no effect on changes to their communities. This makes sense in that bacterial communities are organized at a smaller spatial scale than fungal communities (Coleman and Crossloey, 1996) so it may be easier to approximate field conditions in greenhouse than for fungal communities, which vary greatly their spatial resolution (Peay et al., 2008).
Does Inoculation Affect Plant Performance?
Positive plant outcomes were more likely to be associated with changes in fungal diversity—but there were no studies showing negative plant outcomes to fungal inoculation (Figure 7A). This is surprising because the literature has many examples of fungal inoculants suppressing plant performance (Verbruggen et al., 2012; Janoušková et al., 2013). While the number of studies is too low to make definitive conclusions, it may be that fungal inoculants can suppress plants directly, rather than through concomitant changes in soil fungal communities. Studies have shown this in artificial conditions (Kokkoris et al., 2019a), but this remains to be robustly tested in natural systems.
Positive plant responses were more often associated with increase fungal diversity. This makes sense in that there is a considerable literature showing a positive relationship between fungal species richness and plant biodiversity (van der Heijden et al., 1998; Klironomos et al., 2000; Hiiesalu et al., 2014) and productivity (van der Heijden et al., 1998, 2008; Klironomos et al., 2000; Jonsson et al., 2001; Maherali and Klironomos, 2007).
This association was not true of bacterial inoculation studies. Negative plant performance was most often negative when inoculation was associated with bacterial diversity changes (Figure 7B). Among all cases of bacterial community change in response to inoculation, plants associated with increased bacterial diversity tended to respond positively to inoculation (Zuppinger-Dingley et al., 2014; Kolton et al., 2017; Laforest-Lapointe et al., 2017), while plants associated with decreased bacterial diversity tended to respond negatively. Which does align with what multiple studies have found in that reductions in soil microbial diversity lead to reduced plant diversity (van der Heijden et al., 1998; Wagg et al., 2014) and productivity (van der Heijden et al., 1998; Chen et al., 2020).
Both of these results support our prediction that inoculations affect plant performance indirectly via concomitant changes to resident communities. This may explain why plant response to bioinoculants is not always positive, despite inoculants often undergoing rigorous testing during development. Most bioinoculants are developed and marketed to affect target hosts directly, either by establishing a functional symbiosis with the host itself, or by reducing stress (Khan et al., 2011; Petriccione et al., 2013; Kuan et al., 2016; Singh et al., 2019). Our results suggest that inoculum development should include interactions with resident communities in order to both create more robust bioinoculants and guard against potential risks to resident SMC.
Conclusion
In this study, we asked whether there is evidence for SMC changes following bioinoculant use. While it clear from our analysis that inoculation changes SMC, there exists variation in the degree and the direction of those changes. Based on our results, we can say that bacterial communities may be more sensitive to changes following inoculation, and that they tend to increase in diversity following inoculation compared to fungal communities. However, there are too few studies examining changes to fungal communities that we cannot say with any confidence how fungal communities respond to inoculation.
We can also say that inoculation accompanied by disturbance tends to exacerbate inoculation effects, positive or negative. This is particularly important for producers who might want to consider the mode of inoculum delivery to either enhance or reduce concurrent changes to SMC.
In a similar way, we might expect differences in soil chemistry to also exacerbate inoculation effects. Unfortunately, studies returned by our literature search did not consistently report soil chemistry, so we could not evaluate this in our model. Soil additives, including fertilizers, may exacerbate inoculant effects on resident microbes. In general, fertilizers, whether organic or inorganic, tend to decrease soil microbial diversity by selecting for few, competitive species at the expense of those more adapted to nutrient stress (Leff et al., 2015; Francioli et al., 2016; Zhang et al., 2016). Nitrogen fertilizers, for example, can reduce ectomycorrhizal activity (Treseder, 2004) and may restrict decomposer communities (Allison et al., 2007; Kamaa et al., 2011) by altering plant carbon input. Similarly, phosphorus can reduce root colonization (Wang et al., 2017), abundance (Abbott and Robson, 1984; Treseder and Allen, 2002), and diversity (Gosling et al., 2013) of arbuscular mycorrhizal fungi.
Commercially produced bioinoculants may have a competitive advantage over residents in conditions where nutrients are not limiting, such as most agroecosystems (Goulding et al., 2008). Inoculants are typically propagated in a high nutrient environment (Bécard and Fortin, 1988; Berruti et al., 2017) and display, rapid growth rates and high reproduction rates (Kaminsky et al., 2019) and occupy broad realized niches (Antunes et al., 2008). Thus, we might expect inoculants to have more substantial effects on resident communities especially considering both Niwa et al. (2018) and Bender et al. (2019), suggested that an inoculant's success is more significant or more effective in conditions where the resident fungal population was smaller, performing poorer, or had overall lower diversity. Clearly, soil chemistry will be an important aspect determining inoculation outcomes, and should be the focus of future research.
Finally, our study suggests that plant response is dependent on SMC response to inoculation. It may be impossible to predict outcomes in plant performance based solely on the action of the bioinoculant. Rather, changes to SMC will mediate that response.
Our study did not differentiate among guilds of fungi and bacterial in response to inoculation. It is likely that endophytes respond differently to inoculation compared to free living microbes. This may be important depending on the nature of the bioinoculant (endophyte or free living). Further, due to sample size, we were unable to discriminate among the various ways SMC could change. We categorized changes as diversity increases or decreases. However, changes due to species loss vs. changes to evenness among taxa represent very different scenarios. It will be important for future studies to qualify diversity changes as well. Similarly, we did not distinguish among the various methods used to calculate SMC diversity. It is possible that differences in diversity estimates could affect the resolution of our analysis; for example, studies that used high throughput sequencing vs. morphotyping.
While our study marks an important starting place to understand the relationship between microbial inoculants and SMC, many questions remain unanswered. What is important for future studies is to understand how the context of inoculation events affect SMC changes, and what these changes mean for soil ecosystems.
Data Availability Statement
The original contributions presented in the study are included in the article/Supplementary Material, further inquiries can be directed to the corresponding author/s.
Author Contributions
MH conceived of the study. CC, VK, AR, CH, DR, MH, and JB performed the research. JB performed the analyses. CC, MM, and JB wrote the manuscript. All authors contributed to the article and approved the submitted version.
Funding
This work was supported by Natural Sciences and Engineering Research Council (MH).
Conflict of Interest
The authors declare that the research was conducted in the absence of any commercial or financial relationships that could be construed as a potential conflict of interest.
Publisher's Note
All claims expressed in this article are solely those of the authors and do not necessarily represent those of their affiliated organizations, or those of the publisher, the editors and the reviewers. Any product that may be evaluated in this article, or claim that may be made by its manufacturer, is not guaranteed or endorsed by the publisher.
Supplementary Material
The Supplementary Material for this article can be found online at: https://www.frontiersin.org/articles/10.3389/fagro.2021.753474/full#supplementary-material
References
Abbott, L. K., and Robson, A. D. (1984). The effect of root density, inoculum placement and infectivity of inoculum on the development of vesicular-arbuscular mycorrhizas. Source New Phytol. 97, 285–299. doi: 10.1111/j.1469-8137.1984.tb04133.x
Adissie, S., Adgo, E., and Feyisa, T. (2020). Effect of rhizobial inoculants and micronutrients on yield and yield components of faba bean (Vicia faba L.) on vertisol of Wereillu district, South Wollo, Ethiopia. Cogent Food Agric. 6, 1747854. doi: 10.1080/23311932.2020.1747854
Ahemad, M. (2019). Remediation of metalliferous soils through the heavy metal resistant plant growth promoting bacteria: paradigms and prospects. Arab. J. Chem. 12, 1365–1377. doi: 10.1016/J.ARABJC.2014.11.020
Albertsen, A., Ravnskov, S., Green, H., Jensen, D. F., and Larsen, J. (2006). Interactions between the external mycelium of the mycorrhizal fungus Glomus intraradices and other soil microorganisms as affected by organic matter. Soil Biol. Biochem. 38, 1008–1014. doi: 10.1016/j.soilbio.2005.08.015
Albright, M. B. N., Sevanto, S., Gallegos-Graves, L. V., and Dunbar, J. (2020). Biotic interactions are more important than propagule pressure in microbial community invasions. MBio 11, 1–16. doi: 10.1128/mBio.02089-20
Allison, S. D., Hanson, C. A., and Treseder, K. K. (2007). Nitrogen fertilization reduces diversity and alters community structure of active fungi in boreal ecosystems. Soil Biol. Biochem. 39, 1878–1887. doi: 10.1016/J.SOILBIO.2007.02.001
Ambrosini, A., de Souza, R., and Passaglia, L. M. P. (2016). Ecological role of bacterial inoculants and their potential impact on soil microbial diversity. Plant Soil 400, 193–207. doi: 10.1007/s11104-015-2727-7
Anandaraj, B., and Leema Rose Delapierre, A. (2010). Studies on influence of bioinoculants (Pseudomonas fluorescens, Rhizobium sp., Bacillus megaterium) in green gram. J. Biosci. Technol. 1, 95–99.
Antunes, P. M., Koch, A. M., Dunfield, K. E., Hart, M. M., Downing, A., Rillig, M. C., et al. (2008). Influence of commercial inoculation with Glomus intraradices on the structure and functioning of an AM fungal community from an agricultural site. Plant Soil 317, 257–266. doi: 10.1007/S11104-008-9806-Y
Antunes, P. M., Koch, A. M., Dunfield, K. E., Hart, M. M., Downing, A., Rillig, M. C., et al. (2009). Influence of commercial inoculation with Glomus intraradices on the structure and functioning of an AM fungal community from an agricultural site. Plant Soil 317, 257–266. doi: 10.1007/s11104-008-9806-y
Aung, T. T., Buranabanyat, B., Piromyou, P., Longtonglang, A., Tittabutr, P., Boonkerd, N., et al. (2013). Enhanced soybean biomass by co-inoculation of Bradyrhizobium japonicum and plant growth promoting rhizobacteria and its effects on microbial community structures. African J. Microbiol. Res. 7, 3858–3873. doi: 10.5897/AJMR2013.5917
Bardgett, R. D., and Van Der Putten, W. H. (2014). Belowground biodiversity and ecosystem functioning. Nature 515, 505–511. doi: 10.1038/nature13855
Bécard, G., and Fortin, J. A. (1988). Early events of vesicular-arbuscular mycorrhiza formation on Ri T-DNA transformed roots. New Phytol. 108, 211–218. doi: 10.1111/j.1469-8137.1988.tb03698.x
Beck, J. L., Cale, J. A., Rodriguez-Ramos, J. C., Kanekar, S. S., Karst, J., Cahill, J. F., et al. (2020). Changes in soil fungal communities following anthropogenic disturbance are linked to decreased lodgepole pine seedling performance. J. Appl. Ecol. 57, 1292–1302. doi: 10.1111/1365-2664.13628
Bender, S. F., Schlaeppi, K., Held, A., and Van der Heijden, M. G. A. (2019). Establishment success and crop growth effects of an arbuscular mycorrhizal fungus inoculated into Swiss corn fields. Agric. Ecosyst. Environ. 273, 13–24. doi: 10.1016/j.agee.2018.12.003
Berruti, A., Lumini, E., and Bianciotto, V. (2017). AMF components from a microbial inoculum fail to colonize roots and lack soil persistence in an arable maize field. Symbiosis 72, 73–80. doi: 10.1007/s13199-016-0442-7
Bharti, N., Barnawal, D., Wasnik, K., Tewari, S. K., and Kalra, A. (2016). Co-inoculation of Dietzia natronolimnaea and Glomus intraradices with vermicompost positively influences Ocimum basilicum growth and resident microbial community structure in salt affected low fertility soils. Agric. Ecosyst. Environ. Appl. Soil Ecol. 100, 211–225. doi: 10.1016/j.apsoil.2016.01.003
Bonanomi, G., Capodilupo, M., Incerti, G., Gaglione, S. A., and Scala, F. (2014). Fungal diversity increases soil fungistasis and resistance to microbial invasion by a non resident species. Biol. Control 72, 38–45. doi: 10.1016/j.biocontrol.2014.02.005
Cassman, N. A., Leite, M. F. A., Pan, Y., De Hollander, M., Van Veen, J. A., and Kuramae, E. E. (2016). Plant and soil fungal but not soil bacterial communities are linked in long-term fertilized grassland. Sci. Rep. 6, 1–11. doi: 10.1038/srep23680
Chen, J., He, F., Zhang, X., Sun, X., Zheng, J., and Zheng, J. (2014). Heavy metal pollution decreases microbial abundance, diversity and activity within particle-size fractions of a paddy soil. FEMS Microbiol. Ecol. 87, 164–181. doi: 10.1111/1574-6941.12212
Chen, J., Wang, P., Wang, C., Wang, X., Miao, L., Liu, S., et al. (2020). Fungal community demonstrates stronger dispersal limitation and less network connectivity than bacterial community in sediments along a large river. Environ. Microbiol. 22, 832–849. doi: 10.1111/1462-2920.14795
Chowdhury, S. P., Dietel, K., Rändler, M., Schmid, M., Junge, H., Borriss, R., et al. (2013). Effects of Bacillus amyloliquefaciens FZB42 on lettuce growth and health under pathogen pressure and its impact on the rhizosphere bacterial community. PLoS ONE 8, e68818. doi: 10.1371/journal.pone.0068818
Christensen, R. H. B. (2019). ordinal - Regression Models for Ordinal Data. R package version. Available online at: https://cran.r-project.org/package=ordinal (accessed May 4, 2021).
Coleman, D. C., and Crossloey, D. A. (1996). Fundamentals of Soil Ecology. Cambridge: Cambridge University Press. doi: 10.1017/s0014479797264100
Dong, L., Li, Y., Xu, J., Yang, J., Wei, G., Shen, L., et al. (2019). Biofertilizers regulate the soil microbial community and enhance Panax ginseng yields. Chin. Med. 14, 20. doi: 10.1186/s13020-019-0241-1
Elad, Y. (2000). Biological control of foliar pathogens by means of Trichoderma harzianum and potential modes of action. Crop Prot. 19, 709–714. doi: 10.1016/S0261-2194(00)00094-6
Ezawa, T., Yamamoto, K., and Yoshida, S. (2002). Enhancement of the effectiveness of indigenous arbuscular mycorrhizal fungi by inorganic soil amendments. Soil Sci. Plant Nutr. 48, 897–900. doi: 10.1080/00380768.2002.10408718
Fortin, J. A., Declerck, S., and Strullu, D.-G. (2005). “In vitro culture of mycorrhizas,” in In Vitro Culture of Mycorrhizas (Berlin/Heidelberg: Springer-Verlag), 3–14. doi: 10.1007/3-540-27331-X_1
Francioli, D., Schulz, E., Lentendu, G., Wubet, T., Buscot, F., and Reitz, T. (2016). Mineral vs. organic amendments: microbial community structure, activity and abundance of agriculturally relevant microbes are driven by long-term fertilization strategies. Front. Microbiol. 7:1446. doi: 10.3389/fmicb.2016.01446
Francioli, D., van Rijssel, S. Q., van Ruijven, J., Termorshuizen, A. J., Cotton, T. E. A., Dumbrell, A. J., et al. (2020). Plant functional group drives the community structure of saprophytic fungi in a grassland biodiversity experiment. Plant Soil 461, 91–105. doi: 10.1007/s11104-020-04454-y
Garg, N., and Singh, S. (2018). Arbuscular mycorrhiza rhizophagus irregularis and silicon modulate growth, proline biosynthesis and yield in Cajanus cajan L. Millsp. (pigeonpea) genotypes under cadmium and zinc stress. J. Plant Growth Regul. 37, 46–63. doi: 10.1007/s00344-017-9708-4
Gomes, N. C. M., Kosheleva, I. A., Abraham, W.-R., and Smalla, K. (2005). Effects of the inoculant strain Pseudomonas putida KT2442 (pNF142) and of naphthalene contamination on the soil bacterial community. FEMS Microbiol. Ecol. 54, 21–33. doi: 10.1016/j.femsec.2005.02.005
Gosling, W. D., Miller, C. S., and Livingstone, D. A. (2013). Atlas of the tropical West African pollen flora. Rev. Palaeobot. Palynol. 199, 1–135.
Goulding, K., Jarvis, S., and Whitmore, A. (2008). Optimizing nutrient management for farm systems. Philos. Trans. R. Soc. B Biol. Sci. 363:667. doi: 10.1098/RSTB.2007.2177
Gupta, R., Mathimaran, N., Wiemken, A., Boller, T., Bisaria, V. S., and Sharma, S. (2014). Non-target effects of bioinoculants on rhizospheric microbial communities of Cajanus cajan. Agric. Ecosyst. Environ. Appl. Soil Ecol. 76, 26–33. doi: 10.1016/j.apsoil.2013.12.001
Gurevitch, J., Koricheva, J., Nakagawa, S., and Stewart, G. (2018). Meta-analysis and the science of research synthesis. Nature 555, 175–182. doi: 10.1038/nature25753
Haas, D., and Défago, G. (2005). Biological control of soil-borne pathogens by fluorescent pseudomonads. Nat. Rev. Microbiol. 3, 307–319. doi: 10.1038/nrmicro1129
Hardin, G. (1960). The competitive exclusion principle. Science 131, 1292–1297. doi: 10.1126/science.131.3409.1292
Hart, M. M., Antunes, P. M., and Abbott, L. K. (2017). Unknown risks to soil biodiversity from commercial fungal inoculants. Nat. Ecol. Evol. 1, 1–1. doi: 10.1038/s41559-017-0115
Hibbing, M. E., Fuqua, C., Parsek, M. R., and Peterson, S. B. (2010). Bacterial competition: surviving and thriving in the microbial jungle. Nat. Rev. Microbiol. 8, 15–25. doi: 10.1038/nrmicro2259
Hiiesalu, I., Pärtel, M., Davison, J., Gerhold, P., Metsis, M., Moora, M., et al. (2014). Species richness of arbuscular mycorrhizal fungi: associations with grassland plant richness and biomass. New Phytol. 203, 233–244. doi: 10.1111/nph.12765
Hooper, D. U., Chapin, F. S., Ewel, J. J., Hector, A., Inchausti, P., Lavorel, S., et al. (2005). Effects of biodiversity on ecosystem functioning: a consensus of current knowledge. Ecol. Monogr. 75, 3–35. doi: 10.1890/04-0922
Huston, M. A. (1997). Hidden treatments in ecological experiments: re-evaluating the ecosystem function of biodiversity. Oecologia 110, 449–460. doi: 10.1007/s004420050180
Irshad, S., Xie, Z., Wang, J., Nawaz, A., Luo, Y., Wang, Y., et al. (2020). Indigenous strain Bacillus XZM assisted phytoremediation and detoxification of arsenic in Vallisneria denseserrulata. J. Hazard. Mater. 381, 120903. doi: 10.1016/j.jhazmat.2019.120903
Islam, M. N., Germida, J. J., and Walley, F. L. (2021). Survival of a commercial AM fungal inoculant and its impact on indigenous AM fungal communities in field soils. Agric. Ecosyst. Environ. Appl. Soil Ecol. 166, 103979. doi: 10.1016/j.apsoil.2021.103979
Jack, C. N., Petipas, R. H., Cheeke, T. E., Rowland, J. L., and Friesen, M. L. (2021). Microbial inoculants: silver bullet or microbial jurassic park? Trends Microbiol. 29, 299–308. doi: 10.1016/j.tim.2020.11.006
Janoušková, M., Krak, K., Vosátka, M., Püschel, D., and Štorchová, H. (2017). Inoculation effects on root-colonizing arbuscular mycorrhizal fungal communities spread beyond directly inoculated plants. PLoS ONE 12:e0181525. doi: 10.1371/journal.pone.0181525
Janoušková, M., Krak, K., Wagg, C., Štorchová, H., Caklová, P., and Vosátka, M. (2013). Effects of inoculum additions in the presence of a preestablished arbuscular mycorrhizal fungal community. Appl. Environ. Microbiol. 79, 6507–6515. doi: 10.1128/AEM.02135-13
Jin, H., Germida, J. J., and Walley, F. L. (2013). Impact of arbuscular mycorrhizal fungal inoculants on subsequent arbuscular mycorrhizal fungi colonization in pot-cultured field pea (Pisum sativum L.). Mycorrhiza 23, 45–59. doi: 10.1007/s00572-012-0448-9
Jonsson, L. M., Nilsson, M. C., Wardle, D. A., and Zackrisson, O. (2001). Context dependent effects of ectomycorrhizal species richness on tree seedling productivity. Oikos 93, 353–364. doi: 10.1034/j.1600-0706.2001.930301.x
Ju, W., Liu, L., Fang, L., Cui, Y., Duan, C., and Wu, H. (2019). Impact of co-inoculation with plant-growth-promoting rhizobacteria and rhizobium on the biochemical responses of alfalfa-soil system in copper contaminated soil. Ecotoxicol. Environ. Saf. 167, 218–226. doi: 10.1016/j.ecoenv.2018.10.016
Kamaa, M., Mburu, H., Blanchart, E., Chibole, L., Chotte, J.-L., Kibunja, C., et al. (2011). Effects of organic and inorganic fertilization on soil bacterial and fungal microbial diversity in the Kabete long-term trial, Kenya. Biol. Fertil. Soils 47, 315–321. doi: 10.1007/S00374-011-0539-3
Kaminsky, L. M., Trexler, R. V., Malik, R. J., Hockett, K. L., and Bell, T. H. (2019). The inherent conflicts in developing soil microbial inoculants. Trends Biotechnol. 37, 140–151. doi: 10.1016/j.tibtech.2018.11.011
Kang, S.-M., Radhakrishnan, R., You, Y.-H., Joo, G.-J., Lee, I.-J., Lee, K.-E., et al. (2014). Phosphate solubilizing Bacillus megaterium mj1212 regulates endogenous plant carbohydrates and amino acids contents to promote mustard plant growth. Indian J. Microbiol. 54, 427–433. doi: 10.1007/s12088-014-0476-6
Karpouzas, D. G., Karatasas, A., Spiridaki, E., Rousidou, C., Bekris, F., Omirou, M., et al. (2011). Impact of a beneficial and of a pathogenic Fusarium strain on the fingerprinting-based structure of microbial communities in tomato (Lycopersicon esculentum Milll.) rhizosphere. Eur. J. Soil Biol. 47, 400–408. doi: 10.1016/j.ejsobi.2011.07.011
Kawaletz, H., Mölder, I., Annighöfer, P., Terwei, A., Zerbe, S., and Ammer, C. (2014). Pot experiments with woody species – a review. For. An Int. J. For. Res. 87, 482–491. doi: 10.1093/forestry/cpu017
Ketola, T., Saarinen, K., and Lindström, L. (2017). Propagule pressure increase and phylogenetic diversity decrease community's susceptibility to invasion. BMC Ecol. 17, 15. doi: 10.1186/s12898-017-0126-z
Khan, A. L., Hamayun, M., Kim, Y.-H., Kang, S.-M., and Lee, I.-J. (2011). Ameliorative symbiosis of endophyte (Penicillium funiculosum LHL06) under salt stress elevated plant growth of Glycine max L. Plant Physiol. Biochem. 49, 852–861. doi: 10.1016/J.PLAPHY.2011.03.005
Kirchman, D. L. (2012). Processes in Microbial Ecology. 1st ed. New York, NY: Oxford University Press. doi: 10.1093/acprof:oso/9780199586936.001.0001
Klironomos, J. N., McCune, J., Hart, M., and Neville, J. (2000). The influence of arbuscular mycorrhizae on the relationship between plant diversity and productivity. Ecol. Lett. 3, 137–141. doi: 10.1046/j.1461-0248.2000.00131.x
Knops, J. M. H., Tilman, D., Haddad, N. M., Naeem, S., Mitchell, C. E., Haarstad, J., et al. (1999). Effects of plant species richness on invasion dynamics, disease outbreaks, insect abundances and diversity. Ecol. Lett. 2, 286–293. doi: 10.1046/j.1461-0248.1999.00083.x
Koch, A. M., Antunes, P. M., Barto, E. K., Cipollini, D., Mummey, D. L., and Klironomos, J. N. (2011). The effects of arbuscular mycorrhizal (AM) fungal and garlic mustard introductions on native AM fungal diversity. Biol. Invasions 13, 1627–1639. doi: 10.1007/s10530-010-9920-7
Kokkoris, V., Hamel, C., and Hart, M. M. (2019a). Mycorrhizal response in crop versus wild plants. PLoS ONE 14, e0221037. doi: 10.1371/journal.pone.0221037
Kokkoris, V., Li, Y., Hamel, C., Hanson, K., and Hart, M. (2019b). Site specificity in establishment of a commercial arbuscular mycorrhizal fungal inoculant. Sci. Total Environ. 660, 1135–1143. doi: 10.1016/j.scitotenv.2019.01.100
Kolton, M., Graber, E. R., Tsehansky, L., Elad, Y., and Cytryn, E. (2017). Biochar-stimulated plant performance is strongly linked to microbial diversity and metabolic potential in the rhizosphere. New Phytol. 213, 1393–1404. doi: 10.1111/nph.14253
Kuan, K. B., Othman, R., Abdul Rahim, K., and Shamsuddin, Z. H. (2016). Plant growth-promoting rhizobacteria inoculation to enhance vegetative growth, nitrogen fixation and nitrogen remobilisation of maize under greenhouse conditions. PLoS ONE 11, e0152478. doi: 10.1371/journal.pone.0152478
Kumari, S., Vaishnav, A., Jain, S., Choudhary, D. K., and Sharma, K. P. (2016). In vitro screening for salinity and drought stress tolerance in plant growth promoting bacterial strains. Int. J. Agric. Life Sci. 2, 60–66. doi: 10.9379/sf.ijals-122067-010-0081-x
Laforest-Lapointe, I., Paquette, A., Messier, C., and Kembel, S. W. (2017). Leaf bacterial diversity mediates plant diversity and ecosystem function relationships. Nature 546, 145–147. doi: 10.1038/nature22399
Le Pioufle, O., Ganoudi, M., Calonne-Salmon, M., Ben Dhaou, F., and Declerck, S. (2019). Rhizophagus irregularis MUCL 41833 improves phosphorus uptake and water use efficiency in maize plants during recovery from drought stress. Front. Plant Sci. 10, 897. doi: 10.3389/fpls.2019.00897
Leff, J. W., Jones, S. E., Prober, S. M., Barberán, A., Borer, E. T., Firn, J. L., et al. (2015). Consistent responses of soil microbial communities to elevated nutrient inputs in grasslands across the globe. Proc. Natl. Acad. Sci. U.S.A. 112, 10967–10972. doi: 10.1073/PNAS.1508382112
Lekberg, Y., and Koide, R. T. (2005). Is plant performance limited by abundance of arbuscular mycorrhizal fungi? A meta-analysis of studies published between 1988 and 2003. New Phytol. 168, 189–204. doi: 10.1111/j.1469-8137.2005.01490.x
Lenth, R. V. (2021). Emmeans: Estimated Marginal Means, Aka Least-Squares Means. R Packag. Version 1.6.1. doi: 10.1080/00031305.1980.10483031
Li, H. Y., Li, D. W., He, C. M., Zhou, Z. P., Mei, T., and Xu, H. M. (2012). Diversity and heavy metal tolerance of endophytic fungi from six dominant plant species in a Pb-Zn mine wasteland in China. Fungal Ecol. 5, 309–315. doi: 10.1016/j.funeco.2011.06.002
Li, Q., Zhang, B., He, Z., and Yang, X. (2016). Distribution and diversity of bacteria and fungi colonization in stone monuments analyzed by high-throughput sequencing. PLoS ONE 11, e0163287. doi: 10.1371/journal.pone.0163287
Litchman, E. (2010). Invisible invaders: non-pathogenic invasive microbes in aquatic and terrestrial ecosystems. Ecol. Lett. 13, 1560–1572. doi: 10.1111/j.1461-0248.2010.01544.x
Liu, X., Li, Y., Ren, X., Chen, B., Zhang, Y., Shen, C., et al. (2020). Long-term greenhouse cucumber production alters soil bacterial community structure. J. Soil Sci. Plant Nutr. 20, 306–321. doi: 10.1007/s42729-019-00109-9
Lladó, S., Solanas, A. M., de Lapuente, J., Borràs, M., and Viñas, M. (2012). A diversified approach to evaluate biostimulation and bioaugmentation strategies for heavy-oil-contaminated soil. Sci. Total Environ. 435–436, 262–269. doi: 10.1016/j.scitotenv.2012.07.032
Loján, P., Senés-Guerrero, C., Suárez, J. P., Kromann, P., Schüßler, A., and Declerck, S. (2017). Potato field-inoculation in Ecuador with Rhizophagus irregularis: no impact on growth performance and associated arbuscular mycorrhizal fungal communities. Symbiosis 73, 45–56. doi: 10.1007/s13199-016-0471-2
Lottmann, J., Heuer, H., De Vries, J., Mahn, A., Düring, K., Wackernagel, W., et al. (2000). Establishment of introduced antagonistic bacteria in the rhizosphere of transgenic potatoes and their effect on the bacterial community. FEMS Microbiol. Ecol. 33, 41–49. doi: 10.1111/j.1574-6941.2000.tb00725.x
Lucas, S. T., D'Angelo, E. M., and Williams, M. A. (2014). Improving soil structure by promoting fungal abundance with organic soil amendments. Agric. Ecosyst. Environ. Appl. Soil Ecol. 75, 13–23. doi: 10.1016/j.apsoil.2013.10.002
Ma, B., Dai, Z., Wang, H., Dsouza, M., Liu, X., He, Y., et al. (2017). Distinct biogeographic patterns for archaea, bacteria, and fungi along the vegetation gradient at the continental scale in Eastern China. mSystems 2, 1–14. doi: 10.1128/MSYSTEMS.00174-16
Ma, M., Jiang, X., Wang, Q., Guan, D., Li, L., Ongena, M., et al. (2018). Isolation and identification of PGPR strain and its effect on soybean growth and soil bacterial community composition. Int. J. Agric. Biol. 20, 1289–1297. doi: 10.17957/IJAB/15.0627
Maherali, H., and Klironomos, J. N. (2007). Influence of phylogeny on fungal community assembly and ecosystem functioning. Science 316, 1746–1748. doi: 10.1126/science.1143082
Mangan, S. A., Schnitzer, S. A., Herre, E. A., Mack, K. M. L., Valencia, M. C., Sanchez, E. I., et al. (2010). Negative plant–soil feedback predicts tree-species relative abundance in a tropical forest. Nature 466, 752–755. doi: 10.1038/nature09273
Marschner, P., and Baumann, K. (2003). Changes in bacterial community structure induced by mycorrhizal colonisation in split-root maize. Plant Soil 251, 279–289. doi: 10.1023/A:1023034825871
Martínez-Viveros, O., Jorquera, M. A., Crowley, D. E., Gajardo, G., and Mora, M. L. (2010). Mechanisms and practical considerations involved in plant growth promotion by Rhizobacteria. J. Soil Sci. Plant Nutr. 10, 293–319. doi: 10.4067/S0718-95162010000100006
Mawarda, P. C., Le Roux, X., Dirk van Elsas, J., and Salles, J. F. (2020). Deliberate introduction of invisible invaders: a critical appraisal of the impact of microbial inoculants on soil microbial communities. Soil Biol. Biochem. 148, 107874. doi: 10.1016/j.soilbio.2020.107874
Moher, D., Shamseer, L., Clarke, M., Ghersi, D., Liberati, A., Petticrew, M., et al. (2015). Preferred reporting items for systematic review and meta-analysis protocols (PRISMA-P) 2015 statement. Syst. Rev.4, 1. doi: 10.1186/2046-4053-4-1
Naeem, S., Knops, J. M. H., Tilman, D., Howe, K. M., Kennedy, T., and Gale, S. (2003). Plant diversity increases resistance to invasion in the absence of covarying extrinsic factors. Oikos 91, 97–108. doi: 10.1034/j.1600-0706.2000.910108.x
Naylor, D., and Coleman-Derr, D. (2018). Drought stress and root-associated bacterial communities. Front. Plant Sci. 8:2223. doi: 10.3389/fpls.2017.02223
Niu, B., Wang, W., Yuan, Z., Sederoff, R. R., Sederoff, H., Chiang, V. L., et al. (2020). Microbial interactions within multiple-strain biological control agents impact soil-borne plant disease. Front. Microbiol. 11:2452. doi: 10.3389/fmicb.2020.585404
Niwa, R., Koyama, T., Sato, T., Adachi, K., Tawaraya, K., Sato, S., et al. (2018). Dissection of niche competition between introduced and indigenous arbuscular mycorrhizal fungi with respect to soybean yield responses. Sci. Rep. 8:7419. doi: 10.1038/s41598-018-25701-4
O'Brien, S. L., Gibbons, S. M., Owens, S. M., Hampton-Marcell, J., Johnston, E. R., Jastrow, J. D., et al. (2016). Spatial scale drives patterns in soil bacterial diversity. Environ. Microbiol. 18, 2039–2051. doi: 10.1111/1462-2920.13231
Ohsowski, B. M., Klironomos, J. N., Dunfield, K. E., and Hart, M. M. (2012). The potential of soil amendments for restoring severely disturbed grasslands. Agric. Ecosyst. Environ. Appl. Soil Ecol. 60, 77–83. doi: 10.1016/j.apsoil.2012.02.006
Oliveira, R. S., Carvalho, P., Marques, G., Ferreira, L., Nunes, M., Rocha, I., et al. (2017). Increased protein content of chickpea (Cicer arietinum L.) inoculated with arbuscular mycorrhizal fungi and nitrogen-fixing bacteria under water deficit conditions. J. Sci. Food Agric. 97, 4379–4385. doi: 10.1002/jsfa.8201
Pallez, L. C., Dole, J. M., and Whipker, B. (2002). Production and postproduction studies with potted sunflowers. Horttechnology 12, 206–210. doi: 10.21273/HORTTECH.12.2.206
Pang, G., Cai, F., Li, R., Zhao, Z., Li, R., Gu, X., et al. (2017). Trichoderma-enriched organic fertilizer can mitigate microbiome degeneration of monocropped soil to maintain better plant growth. Plant Soil 416, 181–192. doi: 10.1007/s11104-017-3178-0
Peay, K. G., Kennedy, P. G., and Bruns, T. D. (2008). Fungal community ecology: a hybrid beast with a molecular master. Bioscience 58, 799–810. doi: 10.1641/B580907
Pellegrino, E., Turrini, A., Gamper, H. A., Cafà, G., Bonari, E., Young, J. P. W., et al. (2012). Establishment, persistence and effectiveness of arbuscular mycorrhizal fungal inoculants in the field revealed using molecular genetic tracing and measurement of yield components. New Phytol. 194, 810–822. doi: 10.1111/j.1469-8137.2012.04090.x
Pérez-García, A., Romero, D., and de Vicente, A. (2011). Plant protection and growth stimulation by microorganisms: biotechnological applications of Bacilli in agriculture. Curr. Opin. Biotechnol. 22, 187–193. doi: 10.1016/j.copbio.2010.12.003
Petriccione, M., Di Patre, D., Ferrante, P., Papa, S., Bartoli, G., Fioretto, A., et al. (2013). Effects of Pseudomonas fluorescens seed bioinoculation on heavy metal accumulation for Mirabilis jalapa phytoextraction in smelter-contaminated soil. Water Air Soil Pollut. 224:1645. doi: 10.1007/s11270-013-1645-7
Piromyou, P., Buranabanyat, B., Tantasawat, P., Tittabutr, P., Boonkerd, N., and Teaumroong, N. (2011). Effect of plant growth promoting rhizobacteria (PGPR) inoculation on microbial community structure in rhizosphere of forage corn cultivated in Thailand. Eur. J. Soil Biol. 47, 44–54. doi: 10.1016/j.ejsobi.0.2010.11.004
R Core Team (2021). R: A Language and Environment for Statistical Computing. Available online at: https://www.r-project.org/ (accessed May 13, 2021).
Rani, A., Bhat, M. N., and Singh, B. P. (2007). Effect of potato phylloplane fungi on potato late blight pathogen Phytophthora infestans. J. Mycol. Plant Pathol. 37, 413–417. doi: 10.3389/fmicb.2018.02213
Rebah, F., Ben Prévost, D., and Tyagi, R. D. (2002). Growth of alfalfa in sludge-amended soils and inoculated with rhizobia produced in sludge. J. Environ. Qual. 31, 1339–1348. doi: 10.2134/jeq2002.1339
Rillig, M. C., Mummey, D. L., Ramsey, P. W., Klironomos, J. N., and Gannon, J. E. (2006). Phylogeny of arbuscular mycorrhizal fungi predicts community composition of symbiosis-associated bacteria. FEMS Microbiol. Ecol. 57, 389–395. doi: 10.1111/j.1574-6941.2006.00129.x
Rodriguez-Ramos, J. C., Cale, J. A., Cahill, J. F., Simard, S. W., Karst, J., and Erbilgin, N. (2021). Changes in soil fungal community composition depend on functional group and forest disturbance type. New Phytol. 229, 1105–1117. doi: 10.1111/nph.16749
Rosa, D., Pogiatzis, A., Bowen, P., Kokkoris, V., Richards, A., Holland, T., et al. (2020). Performance and establishment of a commercial mycorrhizal inoculant in viticulture. Agricuture 10:539. doi: 10.3390/agriculture10110539
Rousk, J., and Bååth, E. (2011). Growth of saprotrophic fungi and bacteria in soil. FEMS Microbiol. Ecol. 78, 17–30. doi: 10.1111/j.1574-6941.2011.01106.x
Sanz-sáez, Á., Heath, K. D., Burke, P. V., and Ainsworth, E. A. (2015). Inoculation with an enhanced N2-fixing Bradyrhizobium japonicum strain (USDA110) does not alter soybean (Glycine max Merr.) response to elevated [CO2]. Plant Cell Environ. 38, 2589–2602. doi: 10.1111/pce.12577
Sarma, B. K., Yadav, S. K., Singh, S., and Singh, H. B. (2015). Microbial consortium-mediated plant defense against phytopathogens: readdressing for enhancing efficacy. Soil Biol. Biochem. 87, 25–33. doi: 10.1016/j.soilbio.2015.04.001
Scheublin, T. R., Sanders, I. R., Keel, C., and Van Der Meer, J. R. (2010). Characterisation of microbial communities colonising the hyphal surfaces of arbuscular mycorrhizal fungi. ISME J. 4, 752–763. doi: 10.1038/ismej.2010.5
Schimel, J., Balser, T. C., and Wallenstein, M. (2007). Microbial stress-response physiology and its implications for ecosystem function. Ecology 88, 1386–1394. doi: 10.1890/06-0219
Schmidt, C. S., Alavi, M., Cardinale, M., Müller, H., and Berg, G. (2012). Stenotrophomonas rhizophila DSM14405T promotes plant growth probably by altering fungal communities in the rhizosphere. Biol. Fertil. Soils 48, 947–960. doi: 10.1007/s00374-012-0688-z
Schmidt, R., Köberl, M., Mostafa, A., Ramadan, E. M., Monschein, M., Jensen, K. B., et al. (2014). Effects of bacterial inoculants on the indigenous microbiome and secondary metabolites of chamomile plants. Front. Microbiol. 5:64. doi: 10.3389/fmicb.2014.00064
Schnoor, T. K., Lekberg, Y., Rosendahl, S., and Olsson, P. A. (2011). Mechanical soil disturbance as a determinant of arbuscular mycorrhizal fungal communities in semi-natural grassland. Mycorrhiza 21, 211–220. doi: 10.1007/s00572-010-0325-3
Sengupta, A., and Dick, W. A. (2015). Bacterial community diversity in soil under two tillage practices as determined by pyrosequencing. Microb. Ecol. 70, 853–859. doi: 10.1007/s00248-015-0609-4
Silva, A. P., Babujia, L. C., Matsumoto, L. S., and Fatima, M. (2013). Bacterial diversity under different tillage and crop rotation systems in an oxisol of Southern Brazil. Open Agric. J. 7, 40–47. doi: 10.2174/1874331501307010040
Singh, R., Singh, M., and Pandey, K. D. (2019). Isolation and characterization of plant growth promoting rhizobacteria from Momordica charantia L. PGPR Amelior. Sustain. Agric. 1, 217–238. doi: 10.1016/B978-0-12-815879-1.00011-2
Sommaruga, R., and Casamayor, E. O. (2009). Bacterial “cosmopolitanism” and importance of local environmental factors for community composition in remote high-altitude lakes. Freshw. Biol. 55, 994–1005. doi: 10.1111/j.1365-2427.2008.02146.x
Stachowicz, J. J., Whitlatch, R. B., and Osman, R. W. (1999). Species diversity and invasion resistance in a marine ecosystem. Science 286, 1577–1579. doi: 10.1126/science.286.5444.1577
Sýkorová, Z., Börstler, B., Zvolenská, S., Fehrer, J., Gryndler, M., Vosátka, M., et al. (2012). Long-term tracing of Rhizophagus irregularis isolate BEG140 inoculated on Phalaris arundinacea in a coal mine spoil bank, using mitochondrial large subunit rDNA markers. Mycorrhiza 22, 69–80. doi: 10.1007/s00572-011-0375-1
Symanczik, S., Courty, P. E., Boller, T., Wiemken, A., and Al-Yahya'ei, M. N. (2015). Impact of water regimes on an experimental community of four desert arbuscular mycorrhizal fungal (AMF) species, as affected by the introduction of a non-native AMF species. Mycorrhiza 25, 639–647. doi: 10.1007/s00572-015-0638-3
Thomsen, C., Loverock, L., Kokkoris, V., Holland, T., Bowen, P. A., and Hart, M. (2021). Commercial arbuscular mycorrhizal fungal inoculant failed to establish in a vineyard despite priority advantage. PeerJ 9:e11119. doi: 10.7717/peerj.11119
Tilman, D. (1997). Distinguishing between the effects of species diversity and species composition. Oikos 80:185. doi: 10.2307/3546532
Trabelsi, D., and Mhamdi, R. (2013). Microbial inoculants and their impact on soil microbial communities: a review. Biomed. Res. Int. 2013:863240. doi: 10.1155/2013/863240
Treseder, K. K. (2004). A meta-analysis of mycorrhizal responses to nitrogen, phosphorus, and atmospheric CO2 in field studies. New Phytol. 164, 347–355. doi: 10.1111/j.1469-8137.2004.01159.X
Treseder, K. K., and Allen, M. F. (2002). Direct nitrogen and phosphorus limitation of arbuscular mycorrhizal fungi: a model and field test. New Phytol. 155, 507–515. doi: 10.1046/J.1469-8137.2002.00470.X
Vahter, T., Bueno, C. G., Davison, J., Herodes, K., Hiiesalu, I., Kasari-Toussaint, L., et al. (2020). Co-introduction of native mycorrhizal fungi and plant seeds accelerates restoration of post-mining landscapes. J. Appl. Ecol. 57, 1741–1751. doi: 10.1111/1365-2664.13663
van der Heijden, M. G. A., Bardgett, R. D., and van Straalen, N. M. (2008). The unseen majority: soil microbes as drivers of plant diversity and productivity in terrestrial ecosystems. Ecol. Lett. 11, 296–310. doi: 10.1111/j.1461-0248.2007.01139.x
van der Heijden, M. G. A. A., Klironomos, J. N., Ursic, M., Moutoglis, P., Streitwolf-Engel, R., Boller, T., et al. (1998). Mycorrhizal fungal diversity determines plant biodiversity, ecosystem variability and productivity. Nature 396, 69–72. doi: 10.1038/23932
Van Elsas, J. D., Chiurazzi, M., Mallon, C. A., Elhottova, D., Krištufek, V., and Salles, J. F. (2012). Microbial diversity determines the invasion of soil by a bacterial pathogen. Proc. Natl. Acad. Sci. U.S.A. 109, 1159–1164. doi: 10.1073/pnas.1109326109
Vannette, R., Hall, G., and Munkres, I. (2020). Fungi are more dispersal-limited than bacteria among flowers. bioRxiv 2020.05.19.104968. doi: 10.1101/2020.05.19.104968
Verbruggen, E., Kiers, E. T., Bakelaar, P. N. C., Röling, W. F. M., and van der Heijden, M. G. A. (2012). Provision of contrasting ecosystem services by soil communities from different agricultural fields. Plant Soil 350, 43–55. doi: 10.1007/s11104-011-0828-5
Verma, J. P., Jaiswal, D. K., and Sagar, R. (2014). Pesticide relevance and their microbial degradation: a-state-of-art. Rev. Environ. Sci. Biotechnol. 13, 429–466. doi: 10.1007/s11157-014-9341-7
Wagg, C., Bender, S. F., Widmer, F., and van der Heijden, M. G. A. (2014). Soil biodiversity and soil community composition determine ecosystem multifunctionality. Proc. Natl. Acad. Sci. U.S.A. 111, 5266–5270. doi: 10.1073/pnas.1320054111
Wang, F., Yao, J., Si, Y., Chen, H., Russel, M., Chen, K., et al. (2010). Short-time effect of heavy metals upon microbial community activity. J. Hazard. Mater. 173, 510–516. doi: 10.1016/j.jhazmat.2009.08.114
Wang, S., Chen, H. Y. H., Tan, Y., Fan, H., and Ruan, H. (2016). Fertilizer regime impacts on abundance and diversity of soil fauna across a poplar plantation chronosequence in coastal Eastern China. Sci. Rep. 6:20816. doi: 10.1038/SREP20816
Wang, X., Wang, C. C., Sui, J., Liu, Z., Li, Q., Ji, C., et al. (2018). Isolation and characterization of phosphofungi, and screening of their plant growth-promoting activities. AMB Express 8:63. doi: 10.1186/s13568-018-0593-4
Wang, Z., Li, T., Wen, X., Liu, Y., Han, J., Liao, Y., et al. (2017). Fungal communities in rhizosphere soil under conservation tillage shift in response to plant growth. Front. Microbiol. 8:1301. doi: 10.3389/fmicb.2017.01301
Web of Science [v.5.35] (2021). Web of Science Core Collection Basic Search. Available online at: https://apps.webofknowledge.com/ (accessed May 13, 2021).
Werner, G. D. A., Strassmann, J. E., Ivens, A. B. F., Engelmoer, D. J. P., Verbruggen, E., Queller, D. C., et al. (2014). Evolution of microbial markets. Proc. Natl. Acad. Sci. U.S.A. 111, 1237–1244. doi: 10.1073/pnas.1315980111
Wu, Q., Sun, R., Ni, M., Yu, J., Li, Y., Yu, C., et al. (2017). Identification of a novel fungus, Trichoderma asperellum GDFS1009, and comprehensive evaluation of its biocontrol efficacy. PLoS ONE 12:e0179957. doi: 10.1371/journal.pone.0179957
Yang, T., Wei, Z., Friman, V., Xu, Y., Shen, Q., Kowalchuk, G. A., et al. (2017). Resource availability modulates biodiversity-invasion relationships by altering competitive interactions. Environ. Microbiol. 19, 2984–2991. doi: 10.1111/1462-2920.13708
Zhang, H. H., Tang, M., Chen, H., and Zheng, C. L. (2010). Effects of inoculation with ectomycorrhizal fungi on microbial biomass and bacterial functional diversity in the rhizosphere of Pinus tabulaeformis seedlings. Eur. J. Soil Biol. 46, 55–61. doi: 10.1016/j.ejsobi.2009.10.005
Zhang, J., Quan, C., Ma, L., Chu, G., Liu, Z., and Tang, X. (2021). Plant community and soil properties drive arbuscular mycorrhizal fungal diversity: a case study in tropical forests. Soil Ecol. Lett. 3, 52–62. doi: 10.1007/s42832-020-0049-z
Zhang, L.-N., Wang, D.-C., Hu, Q., Dai, X.-Q., Xie, Y.-S., Li, Q., et al. (2019). Consortium of plant growth-promoting rhizobacteria strains suppresses sweet pepper disease by altering the Rhizosphere microbiota. Front. Microbiol. 10:1668. doi: 10.3389/fmicb.2019.01668
Zhang, T., Yang, X., Guo, R., and Guo, J. (2016). Response of AM fungi spore population to elevated temperature and nitrogen addition and their influence on the plant community composition and productivity. Sci. Rep. 6:24749. doi: 10.1038/srep24749
Keywords: microbial diversity, bacteria, fungi, plant performance, co-inoculation, disturbance, biofertilizer
Citation: Cornell C, Kokkoris V, Richards A, Horst C, Rosa D, Bennett JA and Hart MM (2021) Do Bioinoculants Affect Resident Microbial Communities? A Meta-Analysis. Front. Agron. 3:753474. doi: 10.3389/fagro.2021.753474
Received: 04 August 2021; Accepted: 20 October 2021;
Published: 23 November 2021.
Edited by:
Mahaveer P. Sharma, ICAR Indian Institute of Soybean Research, IndiaReviewed by:
Dilfuza Egamberdieva, Leibniz Center for Agricultural Landscape Research (ZALF), GermanyKamlesh Kumar Meena, National Institute of Abiotic Stress Management (ICAR), India
Copyright © 2021 Cornell, Kokkoris, Richards, Horst, Rosa, Bennett and Hart. This is an open-access article distributed under the terms of the Creative Commons Attribution License (CC BY). The use, distribution or reproduction in other forums is permitted, provided the original author(s) and the copyright owner(s) are credited and that the original publication in this journal is cited, in accordance with accepted academic practice. No use, distribution or reproduction is permitted which does not comply with these terms.
*Correspondence: Miranda M. Hart, miranda.hart@ubc.ca
†Present address: Vasilis Kokkoris, Department of Ecological Science, Vrije Universiteit Amsterdam, Amsterdam, Netherlands