- 1Department of Biological and Agricultural Engineering, University of Arkansas, Fayetteville, AR, United States
- 2Department of Plant and Soil Sciences, University of Delaware, Newark, DE, United States
- 3School of Civil and Environmental Engineering, Cornell University, Ithaca, NY, United States
- 4Department of Civil and Environmental Engineering, University of California, Davis, Davis, CA, United States
- 5USDA Agricultural Research Service, Delta Water Management Research Unit, Jonesboro, AR, United States
- 6USDA Agricultural Research Service, Dale Bumpers National Rice Research Center, Stuttgart, AR, United States
- 7Isbell Farms, England, AR, United States
Rice is a staple food and primary source of calories for much of the world. However, rice can be a dietary source of toxic metal(loid)s to humans, and its cultivation creates atmospheric greenhouse gas emissions and requires high water use. Because rice production consumes a significant amount of natural resources and is a large part of the global agricultural economy, increasing its sustainability could have substantial societal benefits. There are opportunities for more sustainable field production through a combination of silicon (Si) management and conservation irrigation practices. As a Si-rich soil amendment, rice husks can limit arsenic and cadmium uptake, while also providing plant vigor in drier soil conditions. Thus, husk addition and conservation irrigation may be more effective to attenuate the accumulation of toxic metal(loid)s, manage water usage and lower climate impacts when implemented together than when either is implemented separately. This modified field production system would take advantage of rice husks, which are an underutilized by-product of milled rice that is widely available near rice farm sites, and have ~10% Si content. Husk application could, alongside alternate wetting and drying or furrow irrigation management, help resolve multiple sustainability challenges in rice production: (1) limit arsenic and cadmium accumulation in rice; (2) minimize greenhouse gas emissions from rice production; (3) decrease irrigation water use; (4) improve nutrient use efficiency; (5) utilize a waste product of rice processing; and (6) maintain plant-accessible soil Si levels. This review presents the scientific basis for a shift in rice production practices and considers complementary rice breeding efforts. It then examines socio-technical considerations for how such a shift in production practices could be implemented by farmers and millers together and may bring rice production closer to a bio-circular economy. This paper's purpose is to advocate for a changed rice production method for consideration by community stakeholders, including producers, millers, breeders, extension specialists, supply chain organizations, and consumers, while highlighting remaining research and implementation questions.
Introduction
Rice (Oryza sativa L.) is a staple food for half of the global population (Seck et al., 2012), yet its production has several potential undesirable outcomes. Rice is typically grown in flooded fields to inhibit non-aquatic weed growth and their competition for nutrients (Arao et al., 2009). However, flooding also decreases the soil reduction-oxidation (redox) value, which intensifies emissions of the greenhouse gas (GHG) methane, CH4, and increases soil mobility and plant-availability of arsenic (As) to rice (Ponnamperuma, 1972; Kirk, 2004). Irrigation management decisions thus greatly affect not just grain yield, but also field GHG emissions and the content and distribution of toxic metal(loid)s in grain (Arao et al., 2009; Adviento-Borbe et al., 2015; Linquist et al., 2015; Matsumoto et al., 2015).
The plant-available As that enters rice grain is either naturally occurring in soils, exists as a residual from previous pesticide use (Bednar et al., 2002), or is delivered via irrigation with As-contaminated groundwater (Roberts et al., 2007; Khan et al., 2009). The mobilized form of As in reduced soil environments (i.e., with low redox values), mainly as inorganic arsenite (As(III) or H3AsO), is chemically similar to the important plant nutrient silicon (Si or H4SiO), (Epstein, 2009). This chemical similarity facilitates the transport of As into rice via the Si pathway (Ma et al., 2008). For these reasons, rice cultivated under flooded fields typically exceeds other cereals in As content (Williams et al., 2007b) and thus its consumption increases exposure to toxic inorganic As (iAs), (Meharg et al., 2009) and presents a public health risk (Hojsak et al., 2015). There is particular concern regarding the iAs content of rice-based baby foods (Meharg et al., 2008), and the U.S. FDA has identified an action level of 100 μg kg−1 iAs in infant rice cereals (US FDA, 2020). Regulations focus on iAs species because of their higher reported toxicity than pentavalent mono-or di-methylated arsenic species (IARC, 2012), both of which can also accumulate in rice grains (Zhao et al., 2010).
One way to limit toxicity is by increasing the redox state by shortening the duration of flooding. This change can be achieved by irrigation management that allows soil drying during some growth periods or in some portions of the field. One such technique is alternate wetting and drying (AWD) water management (Lampayan et al., 2015), a practice gaining in popularity in the U.S. and in Asia that replaces traditional cultivation under season-long inundation with deliberate drainage or drying to decrease the duration of flooding (Linquist et al., 2015; Chou et al., 2016; Das et al., 2016). Under AWD, fields are sowed, fertilized, and flooded normally, but fields are allowed to dry to below saturation prior to re-flooding at least once during the rice growth cycle (Figure 1). Through additional rainfall capture during drying periods, AWD decreases irrigation water use and associated pumping costs by 18–44% (Linquist et al., 2015; Atwill et al., 2020). As a water saving strategy, AWD is incentivized through conservation payments in the U.S. (Reba and Massey, 2020). The introduction of oxic conditions during the rice growth cycle in AWD management increases the average soil redox potential, thereby decreasing CH4 production and decreasing As availability in the soil and accumulation by the plant (Linquist et al., 2015; Chou et al., 2016; Das et al., 2016). The grower has many implementation options that control the intensity, frequency, and duration of dry periods based on specific weather and field conditions (Carrijo et al., 2017).
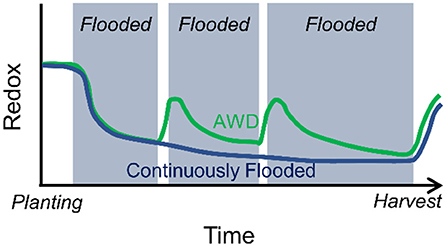
Figure 1. The effect of water management on soil and pore water reduction-oxidation conditions (soil “redox”). Under continuously flooded conditions, sufficient labile carbon and limited oxygen influx result in low redox values throughout the growing season. Under AWD, the field is occasionally dried between flooded periods (shaded), raising the redox value. The timing, duration, and intensity of dry downs in AWD can be optimized to field specific conditions.
Unfortunately, potential negative consequences of AWD include a risk of decreased yield (Carrijo et al., 2017), increased grain cadmium (Cd) content (Arao et al., 2009), and increased emissions of nitrous oxide (N2O), (Lagomarsino et al., 2016; Kritee et al., 2018), (Figure 2). These undesirable outcomes are not trivial. First, changes in yield can decrease food availability and impact farm profits (Nalley et al., 2016; Stuart et al., 2016). Second, while AWD is effective at decreasing grain As concentrations, it may increase grain Cd from some soils (Arao et al., 2009; Li et al., 2019) because Cd is more mobile and plant-available under increasingly oxic soil conditions. Whether the elevated Cd in grain leads to an increased risk to consumers depends on the intensity of AWD and the soil properties (i.e., soil pH, Cd concentration). Both Cd and As are toxic at low concentrations; the FAO/WHO Codex Maximum Level values for polished rice are 0.4 mg kg−1 (Cd) and 0.2 mg kg−1 (As), respectively (FAO/WHO, 2013). Third, on a mass basis over a 100-year time horizon, N2O is nine times more potent as a GHG than CH4 (Myhre et al., 2013). The loss of reactive N from nitrate or ammonium fertilizers as N2O loss via microbial nitrification and denitrification (Butterbach-Bahl et al., 2013) also represents a decrease in nutrient use efficiency, requiring careful coordination of nutrient and water applications (Liao et al., 2020). For sustainable rice management, strategies must consider all these factors to simultaneously conserve natural resources, achieve high yield, low levels of both As and Cd in the grain, and minimize GHG emissions.
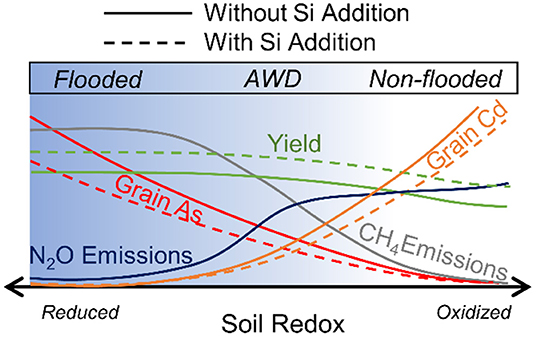
Figure 2. Conceptual plot of the effect of soil redox on biogeochemistry in rice paddies; y-axis is conceptual and normalized for each term's magnitude. Flooded rice creates reduced conditions that are conducive to high methane emissions and high grain As, while moving toward non-flooded rice increases grain Cd and N2O emissions. Si-rich soil amendments can decrease As and Cd in grain while also improving yield in some situations, as increased plant Si helps alleviate biotic and abiotic stresses in rice.
An emerging strategy (Figure 2) to decrease grain As without increasing (and potentially decreasing) Cd (Meharg and Meharg, 2015; Das et al., 2021) is Si fertilization. Increasing plant-available Si can benefit rice in Si-depleted soils by improving rice yield (Savant et al., 1997a,b; Teasley et al., 2017) and providing resistance to stress (Deren et al., 1994; Seebold et al., 2001), pests (Sidhu et al., 2013; Bakhat et al., 2018), straight head disorder (Teasley et al., 2017; Limmer et al., 2018b), and disease (Datnoff et al., 1997; Rodrigues et al., 2003), including rice blast (Osuna-Canizalez et al., 1991; Brunings et al., 2009; Griffith et al., 2021). Silicon can also lower As uptake by roots by direct competition and by downregulating the expression of root Si or As(III) transporters as long as the Si source provides high Si:As ratios (Ma et al., 2008; Seyfferth and Fendorf, 2012; Seyfferth et al., 2016). Silicon fertilization seems to have more benefits to rice yield and disease resistance when plants are stressed with As or grown in Si-depleted environments (i.e., low Si:As ratios), (Teasley et al., 2017; Limmer et al., 2018b; Dwivedi et al., 2020; Griffith et al., 2021). Previous work shows that as plant-available Si increases, grain iAs decreases (Bogdan and Schenk, 2008; Seyfferth and Fendorf, 2012; Seyfferth et al., 2016). Si fertilization can be implemented sustainably via recycling Si-rich rice husk residues (Limmer et al., 2018a; Leksungnoen et al., 2019; Seyfferth et al., 2019a), which act as a slow-release Si fertilizer and potential source of other nutrients like NPK (Teasley et al., 2017; Linam et al., 2021) but without the negative impacts of straw incorporation such as higher GHG emissions and less Si dissolution (Gutekunst et al., 2017).
While AWD and Si management can further the sustainability of rice production, there is currently little research on their synergistic benefits in either laboratory or field trial settings, let alone at the scale of the production farm (1–1000 ha). Likely due to its history of use in organic, Si-poor soils, Si fertilization is rarely emphasized in wider agricultural practice (Tubana et al., 2016) and is not yet encouraged in US university rice production handbooks (e.g., UADA, 2018). AWD has been suggested only recently in rice handbooks (Henry et al., 2017). In this review, we propose that combined AWD and Si management is beneficial, as rice husk amendments may decrease As uptake and alleviate some of the unwanted side effects of the AWD practice. We first present the research basis for this changed production system alongside a description of how rice breeders could develop varieties that are optimized for production in this management system. We emphasize the sustainability benefits of such a transition and highlight the need for an integrated socio-technical transition to ensure a successful shift in production practice. We examine how the co-implementation of these practices could function within the US Mid-South. This region is responsible for ~80% of US rice production (USDA-NASS, 2021) and is agronomically similar to mechanized, irrigated, predominantly drill-seeded rice production systems elsewhere, including Northeast China, Australia, and South America's MERCOSUR region (Rao et al., 2017). While no regional soil survey of As in rice soils in this region exists, many locations in this region are in the 90th percentile of soil As levels in the US (Smith et al., 2019) and some rice produced in this region has been shown to have more total As than that from California (Williams et al., 2007a; Heitkemper et al., 2009).
Scientific Basis For Recommended Changes and Outstanding Challenges
In this section, we present evidence that supports a rice production system focused on (1) water saving irrigation practices that generate oxic soil conditions, (2) soil Si management, (3) their synergies, (4) a move toward a circular bio-economy, and (5) complementary efforts in rice breeding efforts. We also emphasize current knowledge gaps and describe areas where further research is needed.
Water Management Effects on Biogeochemistry
Rice irrigation practices can decrease CH4 emissions by interrupting (in AWD) or preventing (in at least the top portion of furrow-irrigated rice fields, where water is channeled down furrows rather than held in paddies or basins) the reducing soil redox conditions conducive to methanogenesis. Research in this area is maturing beyond pot and plot trials. An experiment on commercial-scale rice fields in Arkansas demonstrated that AWD can decrease CH4 emissions by 64.5% without inducing a change in yield (Runkle et al., 2019). Others have found relatively high off-season CH4 emissions when fields are flooded, e.g., to attract water fowl (Twedt and Nelms, 1999), particularly if that flood immediately follows straw incorporation (Martínez-Eixarch et al., 2018; Reba et al., 2019). Studies have also shown that N2O emissions can increase as a result of earlier oxygenation of the soils during a period when N-based fertilizers are still present (Zhou et al., 2020). AWD-induced decreases in CH4 emissions tend to greatly outweigh increases in N2O on a global warming potential basis (Linquist et al., 2012, 2015; Sander et al., 2020).
Field-scale monitoring of pore water chemistry under AWD practices reveals that drainage or dry-down events can alter As biogeochemistry. For example, a single dry-down to a soil tension of −25 kPa (or 20% volumetric water content at 10 cm) inhibited the reductive dissolution of Fe (oxyhydr)oxides, and consequent mobilization of As into pore water, for at least 1 month after the soils are re-flooded (Maguffin et al., 2020). While AWD practices almost always decrease total As in rice grain, they do not always decrease the iAs concentration (Somenahally et al., 2011; Linquist et al., 2015; Carrijo et al., 2019; Li et al., 2019; Fernández-Baca et al., 2021a), an important limitation given the high toxicity of iAs and its use as a legal standard to set exposure limits. Because iAs is methylated to dimethylarsinic acid (DMA) under reducing soil conditions, more oxic conditions may lead to less DMA production and therefore more iAs uptake relative to DMA (Li et al., 2009; Jia et al., 2013; Chen et al., 2019a; Dykes et al., 2021). These findings underscore the need for further biogeochemical research on the effects of redox conditions on microbial As methylation-demethylation reactions in the soil solution, and whether oxic conditions may enhance As demethylation via oxygen-dependent cleavage of As–C bonds by ArsI enzymes (Yoshinaga and Rosen, 2014; Nadar et al., 2016; Pawitwar et al., 2017).
Together, these studies imply the need for researchers to apply continuous measurement and monitoring systems such as (1) micrometeorological techniques to capture short “hot moments” (hours to days) of high N2O emissions and controls on CH4 dynamics and (2) process-oriented models driven by hydro-biogeochemical interactions and finer-scale soil and soil water monitoring. These approaches could account for year-round inundation dynamics on GHG emissions and soil biogeochemistry, particularly in response to amendments, fertilizers, and plant litter management, and allow climate benefits additional to AWD's current practice. Research is also needed to assess the biogeochemistry associated with furrow-irrigated rice, an emerging cultivation technique in the US Mid-South (Chlapecka et al., 2021; Della Lunga et al., 2021; Karki et al., 2021) that creates spatial gradients of inundation dynamics through the production season.
Si-Rich Rice Husks as Soil Amendments and Their Impact on Biogeochemistry
Not all Si sources behave similarly (Seyfferth et al., 2018): the opaline Si that forms within Si-accumulating plant tissues is poorly crystalline, highly soluble, and plant-available (Savant et al., 1997a). Even though Si is the second most abundant element in the Earth's crust, most Si is held tightly in the crystal lattice of soil minerals and is not readily plant-available (Savant et al., 1997a). When Si-rich rice residues are incorporated into soils, pore water Si increases more than expected from equilibrium with soil minerals (Seyfferth et al., 2013). When added on an equivalent Si basis, husks, also known as hulls, provide 50% more Si to pore water than straw-based amendments (Penido et al., 2016). However, much of the biomass of a rice plant is found in straw with husk comprising ~20% of the harvested rough rice yield. While Si-rich fertilizers like calcium silicate can also provide Si, research has shown that calcium silicate rapidly dissolves and mainly provides Si during vegetative growth, whereas husk acts as a slow-release Si fertilizer providing Si at the critical stage of grain-filling when it can most impact accumulation of As in grains (Teasley et al., 2017).
The impact of soil incorporation of Si-rich rice husk is well-tested on As cycling, root plaque Fe mineral composition, and GHG production in flooded and non-flooded soils (Penido et al., 2016; Seyfferth et al., 2016, 2019a; Amaral et al., 2017; Gutekunst et al., 2017; Limmer et al., 2018a; Wu et al., 2020). In addition to having more Si than straw, rice husks contain approximately one order of magnitude less As and have less labile carbon than rice straw due to over twice the lignin content (Contreras et al., 2012; Penido et al., 2016), making their application advantageous from both metalloid and GHG perspectives. Husk incorporation results in an order of magnitude less CH4 production than straw incorporation to flooded soils (Penido et al., 2016). Husk-derived Si has been shown to beneficially compete against As in its assimilation into the plant and can combat the As-induced yield loss in flooded rice (Teasley et al., 2017; Limmer et al., 2018b). Husk addition increases the proportion of ferrihydrite in root plaques, which may contribute to As retention in the rhizosphere and less As uptake (Seyfferth et al., 2019b). Moreover, soil incorporation of husks can decrease toxic iAs in rice grain by 25–50% without affecting grain Cd in flooded and non-flooded soils (Seyfferth et al., 2016, 2019a; Teasley et al., 2017). Added husks may even decrease plant Cd through a number of mechanisms. First, husks allow growth dilution of Cd due to increased biomass and second, increased soil retention of Cd due to slightly higher pH with husk addition to acidic, oxic soils (Seyfferth et al., 2016, 2019a). Third, Si may also decrease Cd via other biochemical mechanisms such as a rice root Si-wall matrix complexation that inhibits Cd ion uptake (Liu et al., 2013a; Ma et al., 2015).
Husks are under-utilized and are often viewed as low-value waste in most parts of the world (Mansaray and Ghaly, 1998; Lim et al., 2014; Kumar et al., 2017), but we argue that they should be used as a nutrient-rich soil amendment in rice production. Occasional studies have promoted its use as an organic fertilizer in rice fields (Savant et al., 1997b; Ebaid and El-Refaee, 2007) or as soil amendment in the form of rice husk ash (Haefele et al., 2011), and some small-scale farmers reincorporate their husk residues, but it is not yet a widespread practice. In addition providing a slow-release of Si to rice plants, husks also contain K and P, which may help to boost plant defense against As stress (Teasley et al., 2017). More research is needed to understand (1) contribution of husk to soil N supplying capacity and its impacts on N cycling (2) influence of husk incorporation compared to straw incorporation on rice production, and (3) if there is a critical plant-available Si level at which husk amendment would be beneficial, as it may be most beneficial in Si-depleted soils. Extension efforts can help to address these knowledge gaps and provide farmers with decision-making tools to determine if husk amendment would be beneficial in their fields.
Potential Synergies of Combining Conservation Irrigation and Husk Amendments
The two management practices (water management and Si-rich husk amendments) are likely synergistic, though more research is needed to assess the added value of both practices in concert over either individually. In terms of metal(loid) uptake and content, grain As accumulation is known to be decreased more by the combined use of AWD (which allows adsorption of As to Fe-mineral surfaces) and Si (which outcompetes As into the root) than either alone (Seyfferth et al., 2018). Because under anoxic, sulfate-reducing conditions that are associated with traditional irrigation management, Cd would largely exist as sparingly soluble cadmium sulfide, thus plant-availability of Cd would be strongly limited. However, in more oxic conditions, Cd availability increases due to oxidation of sulfide to sulfate and Cd release (Reddy and Patrick, 1977; Arao et al., 2009). While AWD conditions may thus increase grain Cd to concentrations nearer to the Codex Maximum Level (Arao et al., 2009; Seyfferth et al., 2019a), Si-rich husk additions may limit grain Cd accumulation because Si can sequester Cd in the root cell wall (Liu et al., 2009, 2013a; Ma et al., 2015; Ji et al., 2017; Shao et al., 2017; Guo et al., 2018). In addition, Si deposits in the cuticle decrease transpiration, increase water use efficiency (Agarie et al., 1998; Nwugo and Huerta, 2008) and mitigate drought stress (Chen et al., 2011). Rice grown under more oxic conditions may also be more susceptible to rice blast, but increasing plant Si can alleviate blast infection (Deren et al., 1994; Griffith et al., 2021). Collectively, Si may then enhance water savings from AWD and increase the ability of rice to withstand more intense soil dry downs (either intentional or accidental).
For nutrient use efficiency, the two practices may also be synergistic. While soil drying can stimulate nitrification-denitrification and N losses through ammonia volatilization (Dong et al., 2012), field reports show AWD either increases (Liu et al., 2013b; Wang et al., 2016) or does not affect (Yao et al., 2012; Mofijul Islam et al., 2016) nitrogen use efficiency. Though the mechanisms remain unclear, Si has also been reported to both increase nitrogen use efficiency (Pati et al., 2016) and raise the optimum N application rate to enable greater yields (Savant et al., 1996). Some evidence points to enhanced flag leaf N content and chlorophyll concentration resulting from Si application (Mohanty et al., 2020); other evidence indicates lower chlorophyll but higher yields with increasing Si (Limmer et al., 2018b), particularly under As stress. Further field scale studies should determine the optimal N application rate under combined Si and AWD treatments to allow farmers to capture a tangible benefit of this potential synergy.
Complementary Rice Breeding Research Efforts
In looking to the future, an important question is: How can modern rice breeding programs change to optimize rice yield and grain nutritional value within the rice husk, Si-amended AWD production system we propose? Other agro food system changes have been limited from a lack of integrating practice change with participation from breeding researchers (Magrini et al., 2016). Currently, while pure line rice varieties can be grown successfully under AWD, U.S. hybrid varieties often have better blast resistance along with higher yield potential (Lyman and Nalley, 2013; Nalley et al., 2016, 2017; Zhao et al., 2019). Hybrid varieties also enhance competitiveness against weeds from early tiller and canopy production and have improved nutrient uptake and drought tolerance due to more extensive root systems (Kang et al., 1994; Zhang and Wang, 2005; Wang et al., 2019). Higher hybrid grain yield is also a benefit when considering net water usage and GHG changes on a per-yield basis (Pittelkow et al., 2013; Brodt et al., 2014; Zhao et al., 2019). Hybrid varieties have also decreased both yield-and area-scaled CH4 emissions relative to pure-line varieties, with suggested mechanisms including changed soil microbial activity and root exudation (Ma et al., 2010; Smartt et al., 2018). Genetic approaches are also used to generate low-CH4 rice through the altered allocation of photosynthates to aboveground biomass rather than roots (Su et al., 2015; Kim et al., 2018). The carryover effect of these shifts into the soil microbial populations is an ongoing area of research (Fernández-Baca et al., 2021b). Genetic approaches are also used to decrease root to shoot translocation of Cd (Ueno et al., 2009; Yan et al., 2019), and to identify genes associated with uptake of multiple essential elements in different growth conditions (Zhang et al., 2014; Liu et al., 2021), locations (Norton et al., 2014), and varieties (Pinson et al., 2015).
Developing rice varieties with increased tolerance to water deficits would decrease risk of yield losses and could allow extended drying times during AWD to further decrease irrigation costs, CH4 emissions, and grain As accumulation. Deeper root systems can increase drought tolerance via access to deeper water reserves, and can be achieved by breeders using genes such as the Deeper Rooting one gene (Uga et al., 2013). Constant access to deep anoxic pore water might, however, offset the decrease in CH4 from AWD, an effect observed in coastal marshes (Kim et al., 2020). Similarly, access to deeper anoxic soil, below the region amended by rice husks, may enable more As uptake and should be further explored. Other drought tolerance mechanisms include alterations in xylem morphology (Ouyang et al., 2020; Ramachandran et al., 2020), increased expression of water-channeling aquaporin genes (Lian et al., 2004, 2006), or increased scavenging of reactive oxygen species (ROS) to decrease drought-induced cell damage (Zhu et al., 2020; Panda et al., 2021). Increasing ROS scavenging would likely also increase tolerance to temperature stress (Zhu et al., 2020) that can affect grain yield, kernel size, milling quality, and chalkiness (Counce et al., 2005; Xu et al., 2020). Further investigation should determine which mechanisms best allow rice plants to avoid or tolerate stress from intermittent water deficits and can benefit from recently developed visual stress rating tools (McClung et al., 2020).
With Si known to protect plants from stress and diseases by increasing ROS scavenging (Kim et al., 2017) and increasing host plant responses to bacterial and fungal pathogens (Wang et al., 2017), it is reasonable to ask if varieties can be bred to increase Si uptake without increasing grain As accumulation. Both iAs and DMA enter rice roots using a transporter protein conferred by the Low silica 1 (Lsi1) gene (Ma et al., 2008). Removing this gene decreases grain As concentrations, but also substantially decreases Si uptake, plant growth, and grain yield (Ma et al., 2008). Rather than As uptake, other studies have tried to reduce grain As accumulation via As metabolism in the plant, such as As-chelation in root, stem or leaf tissues (Song et al., 2014; Heuschele et al., 2017). These studies suggest that breeding efforts might focus on restricting grain As concentrations by altering post-uptake transport, metabolism, and sequestration rather than by altering root uptake. This mix of challenging pathways for achieving significant reductions in grain As through breeding highlight the importance of simultaneously pursuing alternative irrigation methods such as AWD, along with Si amendment, to limit the accumulation of As in U.S. rice.
Circular Bio-Economy in Rice
The reuse of husk material in rice production would help close one material flow loop in the rice production system and supports the circular economy goal of a regenerative system with less material waste (Geissdoerfer et al., 2017; Kadoglidou et al., 2019). This activity aligns with Principle 2 of circularity in agricultural production (de Boer and van Ittersum, 2018), that food system by-products should be recycled back into the food system, including for soil quality and other benefits. Si-rich rice husks can be considered as a sustainable source of Si for farmers worldwide, as ~5 Tg yr−1 of husks are produced globally (FAO, 2015). Its disposal is generally considered a nuisance by farmers in developed countries (Savant et al., 1997b) and it is a major waste stream for millers (Lim et al., 2013; Kumar et al., 2016; Quispe et al., 2017). In Arkansas it is burned at mills as a low-value fuel, producing a potential health risk for those exposed to the ash silica particles (Liu et al., 1996; Okutani et al., 2018), while still requiring land disposal of the residuals.
Moving rice production toward a more circular system is challenging because current production practices such as husk burning or landfilling, chemical fertilizer sales, and the assumption of continuous flood water use are embedded into technology development, trade, and financial services (Chen et al., 2019b). Currently, promoters of material reuse and recovery often fail to recognize the large-scale, cross-sectoral shifts necessary for successful implementation (Kirchherr et al., 2017). For example, production adaptation may require regional coordination and integration between input and output nodes of the supply chain. In this case, farm re-use of husks will likely require ad-hoc or experimental coordination among mills and farmers to encourage husk return to the farms. This effort may then require supply-chain or public sector incentive programs to motivate a shift in operations (Magrini et al., 2018). Over time, these relationships may become more efficient so that, for example, empty or deadhead truck miles are minimized. That is, the same trucks that bring stored grain to the mill may return husk by-products to the farm for storage or application.
Application of rice husks or their by-products can help close agronomic element budgets beyond the Si cycle and may restore some carbon to the soil that is lost in the common practice of residue burning (McCarty et al., 2007). Rice husks contain high concentrations of Si, N, and K, with lesser concentrations of P, S, and Zn (Table 1). With an average production of 8,300 kg rough rice ha−1 from 2010 to 2020 in the U.S. (USDA-NASS, 2020) and assuming 20% husk in rough rice, 1,650 kg husk ha−1 are annually harvested in the U.S. Husk removal then corresponds to a noticeable removal of nutrients from the field, and our hypothesis is that returning husks represents a fertilization event of available nutrients. Because Si fertilization is known to increase uptake of K (Teasley et al., 2017) along with P, N, and Si (Pati et al., 2016; Cuong et al., 2017), researchers could test whether applying husks to a field could lessen the amount of fertilizer needed and what field conditions are needed to facilitate this reduction. In principle, if Si significantly boosts nutrient use efficiency, decreased application rates could then mitigate some of the N2O-emissions risk associated with AWD and decrease NPK fertilizer costs. Husk addition may also provide soil health benefits that can benefit rice production in the long-term and should be further investigated.
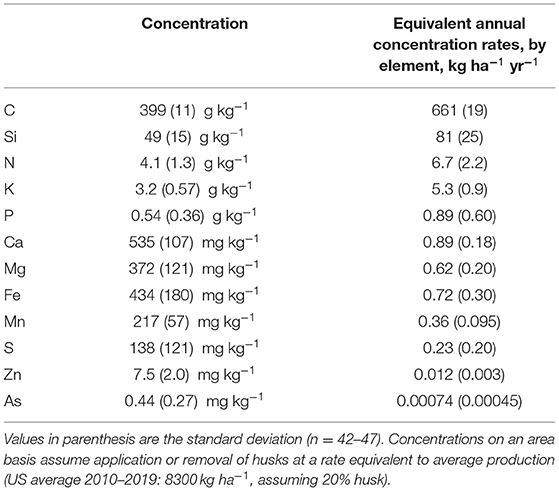
Table 1. Average elemental concentrations of rice husks harvested in Arkansas from several different farms in 2012, 2013, 2018, and 2019, determined by digestion and inductively coupled plasma analysis.
Discussion: Path Toward Adaptation
While the supporting evidence is generally clear, there are still research needs to better understand the details of a practice that combines husk incorporation with more intense and longer duration oxic soil conditions via water management. Potential side effects should be investigated prior to widespread adaptation of this pair of practices for millers, farmers, and other regional players (such as other water users). Moreover, adaptation of these practices must account for and be informed by local knowledge and needs, incentive structures, and behaviors (Clark et al., 2016).
Optimizing Science and Management for Socio-Technical Change
We suggest that further research across the rice production system, including farm and mill activities and processes, and user co-designed decision-support tools are both needed to optimize water management and amendment strategies that balance As and Cd uptake, CH4 and N2O emissions, harvest yield, and farm and supply chain management. Altering water management practices is, to date, unable to simultaneously eliminate rice uptake of As and Cd, although a site-specific optimum could be achieved based on soil physical and chemical characteristics, including the soil's As and Cd content and chemical speciation (Arao et al., 2009; Hu et al., 2013; Honma et al., 2016; Xu et al., 2019) and the rice genotype (Monaco et al., 2021). In practice, it may be difficult to target and maintain a specific soil moisture, tension, or redox status, especially at the production scale with heterogeneous soil conditions and drainage patterns across a field. Thus, additional research is needed to test, simplify, and develop methods to translate soil conditions into farm decision protocols (e.g., Owens et al., 2008; Rabenhorst, 2018), developed in collaboration with users and their perceptions of uncertainty in the delivered guidance (Ara et al., 2021). It is likely that to be successful, these technology changes and practice implementations must be driven by local, farm-level experimentation as well as changes in institutional structures such as incentives or research translation (Ruttan, 1999; Duru et al., 2015; Tittonell et al., 2016). These changes are termed socio-technical as they combine consumer and producer behaviors, market practices, and infrastructure shifts (Geels, 2019) and highlight the need for social science, natural science, and engineering perspectives to collaborate toward effective change (Lowe et al., 2008).
In research, there is increasing ability to model and simulate GHG responses to water management strategies. However, even stronger understanding of field GHG emissions is important as they represent the largest GHG source within the rice production system (Blengini and Busto, 2009; Moreno-García et al., 2021). Research has been enhanced with recent eddy covariance data on GHG fluxes (Knox et al., 2016; Oikawa et al., 2017); their high temporal resolution (i.e., near continuously) can detect the marked diurnal and weekly variations in flux magnitude to enable more precise understanding of the impact of shorter or longer drainage periods (Fertitta-Roberts et al., 2019), particularly under different husk amendment scenarios. Further continuous, field-scale experimental measurements of CO2, CH4, and N2O simultaneously will inform policy-makers regarding emission reduction (Hemes et al., 2021) and will enable a more holistic approach to rice cultivation.
On the farm side, additional research and cooperation is needed for these management changes. Efficient husk storage options at either the mill or farm need to be tested and account for lags between milling (e.g., in fall, or after a delay from bin storage) and cultural management practices (e.g., in spring, prior to or near the sowing date). Mills that parboil rice–cooking in the husk–may be able to contribute a more pure husk product—i.e., one with less contamination from other waste, such as weed seeds or unsterilized material that risk a disease spread (noted in Bartz et al., 2017). However, doing so may also require additional conveyer and storage bins to keep parboiled husk separate from other waste streams. Research is needed to examine the logistics and feasibility of husk distribution to the farm from the mill (Miao et al., 2012). Before adoption by farmers, spreading and incorporation techniques (e.g., with a manure spreader), timing, and application rate should be tested. For example, prioritizing husk allocations among fields would require an assessment of soils most likely to benefit from their incorporation. Likely, application in drill-seeded production systems should occur either in the fall, prior to sowing in spring, or before the first flush irrigation event at the latest (in Arkansas, at the 5-leaf stage). Additionally, research from a life cycle perspective (Renouf et al., 2018) could test the benefits of husk incorporation relative to current, lower value uses of husks (Sun and Gong, 2001). These other applications may include waste water treatment, using its sorbent properties (Daifullah et al., 2003; Ahmaruzzaman and Gupta, 2011), energy production (Pode, 2016), animal bedding (Corrêa et al., 2009) or silica provision for nanomaterials (Shen, 2017).
Producers may need decision support tools to guide implementation of new management practices. Several farm-level decision support tools have been developed to help the farmers in this process (Renouf et al., 2018; Arulnathan et al., 2020). One of these tools, Field Print Platform (https://fieldtomarket.org/our-programs/fieldprint-platform/) offers rice assessment for the two different growing areas in the USA (California and the mid-south), and other tools such as Cool Farm Tool offer a worldwide scope (Hillier et al., 2011). While these tools evaluate the impact of management practices such as intermittent flooding or residue incorporation on GHG emissions or energy use, they do not offer metal(loid) content and grain quality as metric outputs. They also do not yet have an option to evaluate or guide selection of husk application techniques. For example, soil tests could be developed to determine which fields would most benefit from husk amendment. A wide variety of soil tests have been developed and tested in different regions, ranging from dilute extractions such as 0.01 M CaCl2 to weak acids such as 0.5 M acetic acid (Barbosa-Filho et al., 2001; Narayanaswamy and Prakash, 2010; Klotzbücher et al., 2015). While some work has identified a critical soil Si level in Louisiana (Paye et al., 2018), more work will be needed to develop appropriate soil Si tests and critical levels for rice grown in the mid-south under varying water managements. Other tools can help guide water management via depictions of redox conditions or soil arsenic levels (Evans et al., 2021; Huhmann et al., 2021). Improving management tools can thus help farmers to evaluate practice adoption from a holistic perspective that includes all necessary aspects such as potential yield loss.
We suggest attention to the following implementation questions: (Q1) How can mills and farmers be co-incentivized to store and organize husk handling and co-implement husk application and conservation irrigation? (Q2) What other systemic changes to rice farming can provide guidance about how to implement large-scale shifts in practice? For Q1, we encourage a coordinated incentive program co-developed by public (e.g., by the Natural Resources Conservation Service), private (millers, food companies, consumers), and market-based entities (e.g., carbon markets, water markets, health markets). External support may be especially appropriate given the public and societal benefits associated with decreased CH4 emissions and grain As concentrations. This support can come in many forms: public research funding, crop insurance programs, payments for eco-system services, safety net programs, and expanded post-secondary education should all be both increased and directed to align with these outcomes (Miles et al., 2017; Yost et al., 2019). In the proposed cultivation practice changes, there will be farm budget savings from decreased irrigation pumping costs and nutrient applications (Nalley et al., 2015) or during drought periods when water availability is decreased, and a price premium for rice grain could reflect marketing benefits of sustainability and public health. Consumers could be incentivized to pay this premium through a trusted sustainability-related food label organized by the supply chain (Asioli et al., 2020); farmers could be incentivized by either the public or private sector to document practices if part of this premium were returned to them in higher sales prices (Burbi et al., 2016; Borsellino et al., 2020).
For Q2, example shifts in farming systems in the US include the increase in use and distribution of on-farm grain bins (Parker and Nalley, 2020) in Asia, the shift to the System of Rice Intensification (SRI) may offer guidance on implementation of a broad, systems-level change involving farmers, agronomists, extension specialists, public funders, and other actors (Satyanarayana et al., 2007). In both cases, we note that successfully raising farm profit and harvest yields helped incentivize producers to implement these shifts. In water conservation, public investment, dialogue and knowledge co-production with farmers, and incentive programs have also induced increased adaptation of the multiple inlet irrigation system, saving water and increasing control of irrigation flows (Shew et al., 2021). Further, agricultural extension specialists and farmer peer networks each have a role to supporting changes in cultivation practices (Baumgart-Getz et al., 2012; Leeuwis and Aarts, 2016), and neither may be sufficient without deliberate and increased investment (Magrini et al., 2018). Concerted, multi-actor planning is needed to design innovation across the many types of participants in this proposed change to the agro food system (Meynard et al., 2017).
Concluding Thoughts
The management change toward combined use of husk residue application and water management to enable oxic soil conditions over longer durations and extent may offer a more sustainable rice production system than either individually. The intersection of the proposed practices also creates new biogeochemical research opportunities to test how the amendments affect the redox potential in addition to the duration or intensity of a flood or drying irrigation cycle. Further investigation of nutrient co-management, effects of increased soil organic matter on soil redox biogeochemistry, and the economics of milling and farming should be integrated into a multi-sectoral systems perspective. There are known and, as-of-yet, unknown challenges to altering long-held agronomic practices. However, there is wide interest in increasing sustainability in the food and agriculture sectors from an economic systems perspective (Yu et al., 2012; Clark and Tilman, 2017). Rice farmers need to participate in the development of tools to help in water and residue management than can adjust to different soil types, soil and root microbial communities. An integrated systems approach with cooperation between farmers, the mills, and researchers may enable more effective practice change, thus increasing the sustainability of rice production.
Data Availability Statement
The raw data supporting the conclusions of this article will be made available by the authors, without undue reservation.
Author Contributions
BR, AS, and MCR devised the hypothesis and drafted the manuscript with input from ML, BM-G, CR, JP, MLR, MA-B, SP, and CI. AS and ML designed the figures, collected, and organized data for Table 1 from samples collected by BR, AS, BM-G, and CR. All authors contributed to manuscript revisions, read, and approved the final version.
Funding
This research was funded through grant USDA-NIFA-2018-67019-27796.
Conflict of Interest
The authors declare that the research was conducted in the absence of any commercial or financial relationships that could be construed as a potential conflict of interest.
Publisher's Note
All claims expressed in this article are solely those of the authors and do not necessarily represent those of their affiliated organizations, or those of the publisher, the editors and the reviewers. Any product that may be evaluated in this article, or claim that may be made by its manufacturer, is not guaranteed or endorsed by the publisher.
Acknowledgments
We thank the numerous participants in the rice economy in Arkansas with whom we discussed this work. We specifically thank Anna McClung of the USDA-ARS Dale Bumpers National Rice Research Center, Stuttgart, AR, for her insightful contributions.
References
Adviento-Borbe, M. A., Necita Padilla, G., Pittelkow, C. M., Simmonds, M., van Kessel, C., and Linquist, B. A. (2015). Methane and nitrous oxide emissions from flooded rice systems following the end-of-season drain. J. Environ. Qual. 44:1071. doi: 10.2134/jeq2014.11.0497
Agarie, S., Uchida, H., Agata, W., Kubota, F., and Kaufman, P. B. (1998). Effects of silicon on transpiration and leaf conductance in rice plants (Oryza sativa L.). Plant Prod. Sci. 1, 89–95. doi: 10.1626/pps.1.89
Ahmaruzzaman, M., and Gupta, V. K. (2011). Rice husk and its ash as low-cost adsorbents in water and wastewater treatment. Ind. Eng. Chem. Res. 50, 13589–13613. doi: 10.1021/ie201477c
Amaral, D. C., Lopes, G., Guilherme, L. R. G., and Seyfferth, A. L. (2017). A new approach to sampling intact Fe plaque reveals Si-induced changes in Fe mineral composition and shoot as in rice. Environ. Sci. Technol. 51, 38–45. doi: 10.1021/acs.est.6b03558
Ara, I., Turner, L., Harrison, M. T., Monjardino, M., de Voil, P., and Rodriguez, D. (2021). Application, adoption and opportunities for improving decision support systems in irrigated agriculture: a review. Agric. Water Manag. 257:107161. doi: 10.1016/j.agwat.2021.107161
Arao, T., Kawasaki, A., Baba, K., Mori, S., and Matsumoto, S. (2009). Effects of water management on cadmium and arsenic accumulation and dimethylarsinic acid concentrations in Japanese rice. Environ. Sci. Technol. 43, 9361–9367. doi: 10.1021/es9022738
Arulnathan, V., Heidari, M. D., Doyon, M., Li, E., and Pelletier, N. (2020). Farm-level decision support tools: a review of methodological choices and their consistency with principles of sustainability assessment. J. Clean. Prod. 256:120410. doi: 10.1016/j.jclepro.2020.120410
Asioli, D., Aschemann-Witzel, J., and Nayga, R. M. (2020). Sustainability-related food labels. Annu. Rev. Resour. Econ. 12, 171–185. doi: 10.1146/annurev-resource-100518-094103
Atwill, R. L., Krutz, L. J., Bond, J. A., Golden, B. R., Spencer, G. D., Bryant, C. J., et al. (2020). Alternate wetting and drying reduces aquifer withdrawal in Mississippi rice production systems. Agron. J. 112, 5115–5124. doi: 10.1002/agj2.20447
Bakhat, H. F., Bibi, N., Zia, Z., Abbas, S., Hammad, H. M., Fahad, S., et al. (2018). Silicon mitigates biotic stresses in crop plants: a review. Crop Prot. 104, 21–34. doi: 10.1016/j.cropro.2017.10.008
Barbosa-Filho, M. P., Snyder, G. H., Elliott, C. L., and Datnoff, L. E. (2001). Evaluation of soil test procedures for determining rice-available silicon. Commun. Soil Sci. Plant Anal. 32, 1779–1792. doi: 10.1081/CSS-120000249
Bartz, W. C., Pill, W. G., and Evans, T. A. (2017). Yield of greenhouse-grown tomato in substrates containing coir and parboiled rice or burnt rice hulls. J. Hortic. Sci. Biotechnol. 92, 231–239. doi: 10.1080/14620316.2016.1271289
Baumgart-Getz, A., Prokopy, L. S., and Floress, K. (2012). Why farmers adopt best management practice in the United States: a meta-analysis of the adoption literature. J. Environ. Manage. 96, 17–25. doi: 10.1016/j.jenvman.2011.10.006
Bednar, A. J., Garbarino, J. R., Ranville, J. F., and Wildeman, T. R. (2002). Presence of organoarsenicals used in cotton production in agricultural water and soil of the Southern United States. J. Agric. Food Chem. 50, 7340–7344. doi: 10.1021/jf025672i
Blengini, G. A., and Busto, M. (2009). The life cycle of rice: LCA of alternative agri-food chain management systems in Vercelli (Italy). J. Environ. Manage. 90, 1512–1522. doi: 10.1016/j.jenvman.2008.10.006
Bogdan, K., and Schenk, M. K. (2008). Arsenic in rice (Oryza sativa L.) related to dynamics of arsenic and silicic acid in paddy soils. Environ. Sci. Technol. 42, 7885–7890. doi: 10.1021/es801194q
Borsellino, V., Schimmenti, E., and El Bilali, H. (2020). Agri-food markets toward sustainable patterns. Sustainability 12:2193. doi: 10.3390/su12062193
Brodt, S., Kendall, A., Mohammadi, Y., Arslan, A., Yuan, J., Lee, I.-S., et al. (2014). Life cycle greenhouse gas emissions in California rice production. Field Crops Res. 169, 89–98. doi: 10.1016/j.fcr.2014.09.007
Brunings, A. M., Datnoff, L. E., Ma, J. F., Mitani, N., Nagamura, Y., Rathinasabapathi, B., et al. (2009). Differential gene expression of rice in response to silicon and rice blast fungus Magnaporthe oryzae. Ann. Appl. Biol. 155, 161–170. doi: 10.1111/j.1744-7348.2009.00347.x
Burbi, S., Baines, R. N., and Conway, J. S. (2016). Achieving successful farmer engagement on greenhouse gas emission mitigation. Int. J. Agric. Sustain. 14, 466–483. doi: 10.1080/14735903.2016.1152062
Butterbach-Bahl, K., Baggs, E. M., Dannenmann, M., Kiese, R., and Zechmeister-Boltenstern, S. (2013). Nitrous oxide emissions from soils: how well do we understand the processes and their controls? Philos. Trans. R Soc. Lond. B Biol. Sci. 368:20130122. doi: 10.1098/rstb.2013.0122
Carrijo, D. R., Li, C., Parikh, S. J., and Linquist, B. A. (2019). Irrigation management for arsenic mitigation in rice grain: timing and severity of a single soil drying. Sci. Total Environ. 649, 300–307. doi: 10.1016/j.scitotenv.2018.08.216
Carrijo, D. R., Lundy, M. E., and Linquist, B. A. (2017). Rice yields and water use under alternate wetting and drying irrigation: a meta-analysis. Field Crops Res. 203, 173–180. doi: 10.1016/j.fcr.2016.12.002
Chen, C., Li, L., Huang, K., Zhang, J., Xie, W.-Y., Lu, Y., et al. (2019a). Sulfate-reducing bacteria and methanogens are involved in arsenic methylation and demethylation in paddy soils. ISME J. 13, 2523–2535. doi: 10.1038/s41396-019-0451-7
Chen, W., Oldfield, T. L., Katsantonis, D., Kadoglidou, K., Wood, R., and Holden, N. M. (2019b). The socio-economic impacts of introducing circular economy into Mediterranean rice production. J. Clean. Prod. 218, 273–283. doi: 10.1016/j.jclepro.2019.01.334
Chen, W., Yao, X., Cai, K., and Chen, J. (2011). Silicon alleviates drought stress of rice plants by improving plant water status, photosynthesis and mineral nutrient absorption. Biol. Trace Elem. Res. 142, 67–76. doi: 10.1007/s12011-010-8742-x
Chlapecka, J. L., Hardke, J. T., Roberts, T. L., Mann, M. G., and Ablao, A. (2021). Scheduling rice irrigation using soil moisture thresholds for furrow irrigation and intermittent flooding. Agron. J. 113, 1258–1270. doi: 10.1002/agj2.20600
Chou, M. L., Jean, J. S., Sun, G. X., Yang, C. M., Hseu, Z. Y., Kuo, S. F., et al. (2016). Irrigation practices on rice crop production in arsenic-rich paddy soil. Crop Sci. 56, 422–431. doi: 10.2135/cropsci2015.04.0233
Clark, M., and Tilman, D. (2017). Comparative analysis of environmental impacts of agricultural production systems, agricultural input efficiency, and food choice. Environ. Res. Lett. 12:064016. doi: 10.1088/1748-9326/aa6cd5
Clark, W. C., Kerkhoff, L., van Lebel, L., and Gallopin, G. C. (2016). Crafting usable knowledge for sustainable development. PNAS 113, 4570–4578. doi: 10.1073/pnas.1601266113
Contreras, L. M., Schelle, H., Sebrango, C. R., and Pereda, I. (2012). Methane potential and biodegradability of rice straw, rice husk and rice residues from the drying process. Water Sci. Technol. 65, 1142–1149. doi: 10.2166/wst.2012.951
Corrêa, É. K., Bianchi, I., Perondi, A., de los Santos, J. R. G., Corrêa, M. N., Castilhos, D. D., et al. (2009). Chemical and microbiological characteristics of rice husk bedding having distinct depths and used for growing–finishing swine. Bioresour. Technol. 100, 5318–5322. doi: 10.1016/j.biortech.2009.02.071
Counce, P. A., Bryant, R. J., Bergman, C. J., Bautista, R. C., Wang, Y.-J., Siebenmorgen, T. J., et al. (2005). Rice milling quality, grain dimensions, and starch branching as affected by high night temperatures. Cereal Chem. 82, 645–648. doi: 10.1094/CC-82-0645
Cuong, T. X., Ullah, H., Datta, A., and Hanh, T. C. (2017). Effects of silicon-based fertilizer on growth, yield and nutrient uptake of rice in tropical zone of Vietnam. Rice Sci. 24, 283–290. doi: 10.1016/j.rsci.2017.06.002
Daifullah, A. A. M., Girgis, B. S., and Gad, H. M. H. (2003). Utilization of agro-residues (rice husk) in small waste water treatment plans. Mater. Lett. 57, 1723–1731. doi: 10.1016/S0167-577X(02)01058-3
Das, S., Chou, M. L., Jean, J. S., Liu, C. C., and Yang, H. J. (2016). Water management impacts on arsenic behavior and rhizosphere bacterial communities and activities in a rice agro-ecosystem. Sci. Total Environ. 542, 642–652. doi: 10.1016/j.scitotenv.2015.10.122
Das, S., Hwang, H. Y., Song, H. J., Cho, S. R., Van Nostrand, J. D., and Kim, P. J. (2021). Soil microbial response to silicate fertilization reduces bioavailable arsenic in contaminated paddies. Soil Biol. Biochem. 159:108307. doi: 10.1016/j.soilbio.2021.108307
Datnoff, L. E., Deren, C. W., and Snyder, G. H. (1997). Silicon fertilization for disease management of rice in Florida. Crop Prot. 16, 525–531. doi: 10.1016/S0261-2194(97)00033-1
de Boer, I. J., and van Ittersum, M. K. (2018). Circularity in Agricultural Production. Wageningen University and Research.
Della Lunga, D., Brye, K. R., Slayden, J. M., Henry, C. G., and Wood, L. S. (2021). Relationships among soil factors and greenhouse gas emissions from furrow-irrigated rice in the mid-southern, USA. Geoderma Reg. 24:e00365. doi: 10.1016/j.geodrs.2021.e00365
Deren, C. W., Datnoff, L. E., Snyder, G. H., and Martin, F. G. (1994). Silicon concentration, disease response, and yield components of rice genotypes grown on flooded organic histosols. Crop Sci. 34, 733–737. doi: 10.2135/cropsci1994.0011183X003400030024x
Dong, N. M., Brandt, K. K., Sørensen, J., Hung, N. N., Hach, C. V., Tan, P. S., et al. (2012). Effects of alternating wetting and drying vs. continuous flooding on fertilizer nitrogen fate in rice fields in the Mekong Delta, Vietnam. Soil Biol. Biochem. 47, 166–174. doi: 10.1016/j.soilbio.2011.12.028
Duru, M., Therond, O., Martin, G., Martin-Clouaire, R., Magne, M.-A., Justes, E., et al. (2015). How to implement biodiversity-based agriculture to enhance ecosystem services: a review. Agron. Sustain. Dev. 35, 1259–1281. doi: 10.1007/s13593-015-0306-1
Dwivedi, S., Kumar, A., Mishra, S., Sharma, P., Sinam, G., Bahadur, L., et al. (2020). Orthosilicic acid (OSA) reduced grain arsenic accumulation and enhanced yield by modulating the level of trace element, antioxidants, and thiols in rice. Environ. Sci. Pollut. Res. 27, 24025-24038. doi: 10.1007/s11356-020-08663-x
Dykes, G. E., Limmer, M. A., and Seyfferth, A. L. (2021). Silicon-rich soil amendments impact microbial community composition and the composition of arsM bearing microbes. Plant Soil. doi: 10.1007/s11104-021-05103-8
Ebaid, R. A., and El-Refaee, I. S. (2007). “Utilization of rice husk as an organic fertilizer to improve productivity and water use efficiency in rice fields,” in Proceedings of the 8th African Crop Science Society Conference (El-Minia), 27-31.
Epstein, E. (2009). Silicon: its manifold roles in plants. Ann. Appl. Biol. 155, 155–160. doi: 10.1111/j.1744-7348.2009.00343.x
Evans, A. E., Limmer, M. A., and Seyfferth, A. L. (2021). Indicator of redox in soil (IRIS) films as a water management tool for rice farmers. J. Environ. Manage. 294:112920. doi: 10.1016/j.jenvman.2021.112920
FAO (2015). FAOSTAT. Available online at: http://www.fao.org/faostat/en (accessed March 20, 2019) [WWW Document].
FAO/WHO (2013). Codex General Standard for Contaminants and Toxins in Food and Feed. CODEX STAN 193, 1995. Rome: Food and Agriculture Organization.
Fernández-Baca, C. P., McClung, A. M., Edwards, J. D., Codling, E. E., Reddy, V. R., and Barnaby, J. Y. (2021a). Grain inorganic arsenic content in rice managed through targeted introgressions and irrigation management. Front. Plant Sci. 11:612054. doi: 10.3389/fpls.2020.612054
Fernández-Baca, C. P., Rivers, A. R., Kim, W., Iwata, R., McClung, A. M., Roberts, D. P., et al. (2021b). Changes in rhizosphere soil microbial communities across plant developmental stages of high and low methane emitting rice genotypes. Soil Biol. Biochem. 156:108233. doi: 10.1016/j.soilbio.2021.108233
Fertitta-Roberts, C., Oikawa, P. Y., and Darrel Jenerette, G. (2019). Evaluating the GHG mitigation-potential of alternate wetting and drying in rice through life cycle assessment. Sci. Total Environ. 653, 1343–1353. doi: 10.1016/j.scitotenv.2018.10.327
Geels, F. W. (2019). Socio-technical transitions to sustainability: a review of criticisms and elaborations of the multi-level perspective. Curr. Opin. Environ. Sustain. 39, 187–201. doi: 10.1016/j.cosust.2019.06.009
Geissdoerfer, M., Savaget, P., Bocken, N. M. P., and Hultink, E. J. (2017). The circular economy–a new sustainability paradigm? J. Clean. Prod. 143, 757–768. doi: 10.1016/j.jclepro.2016.12.048
Griffith, A., Wise, P., Gill, R., Paukett, M., Donofrio, N., and Seyfferth, A. L. (2021). Combined effects of arsenic and Magnaporthe oryzae on rice and alleviation by silicon. Sci. Total Environ. 750:142209. doi: 10.1016/j.scitotenv.2020.142209
Guo, L., Chen, A., He, N., Yang, D., and Liu, M. (2018). Exogenous silicon alleviates cadmium toxicity in rice seedlings in relation to Cd distribution and ultrastructure changes. J. Soils Sediments 18, 1691–1700. doi: 10.1007/s11368-017-1902-2
Gutekunst, M. Y., Vargas, R., and Seyfferth, A. L. (2017). Impacts of soil incorporation of pre-incubated silica-rich rice residue on soil biogeochemistry and greenhouse gas fluxes under flooding and drying. Sci. Total Environ. 593–594, 134–143. doi: 10.1016/j.scitotenv.2017.03.097
Haefele, S. M., Konboon, Y., Wongboon, W., Amarante, S., Maarifat, A. A., Pfeiffer, E. M., et al. (2011). Effects and fate of biochar from rice residues in rice-based systems. Field Crops Res. 121, 430–440. doi: 10.1016/j.fcr.2011.01.014
Heitkemper, D. T., Kubachka, K. M., Halpin, P. R., Allen, M. N., and Shockey, N. V. (2009). Survey of total arsenic and arsenic speciation in US-produced rice as a reference point for evaluating change and future trends. Food Addit. Contam. Part B. 2, 112–120. doi: 10.1080/02652030903148298
Hemes, K. S., Runkle, B. R. K., Novick, K. A., Baldocchi, D. D., and Field, C. B. (2021). An ecosystem-scale flux measurement strategy to assess natural climate solutions. Environ. Sci. Technol. 55, 3494-3504. doi: 10.1021/acs.est.0c06421
Henry, C. G., Massey, J. H., Hardke, J., Krutz, J., Reba, M. L., and Adviento-Borbe, M. A. A. (2017). Using alternate wetting and drying (AWD) rice flooding management. Arkansas Exp. Stn. Res. FSA62, 1–5. Available online at: https://www.uaex.uada.edu/publications/pdf/fsa62.pdf
Heuschele, D. J., Pinson, S. R. M., and Smith, A. P. (2017). Metabolic responses to arsenite in rice seedlings that differed in grain arsenic concentration. Crop Sci. 57, 2671–2687. doi: 10.2135/cropsci2016.06.0493
Hillier, J., Walter, C., Malin, D., Garcia-Suarez, T., Mila-i-Canals, L., and Smith, P. (2011). A farm-focused calculator for emissions from crop and livestock production. Environ. Model. Softw. 26, 1070–1078. doi: 10.1016/j.envsoft.2011.03.014
Hojsak, I., Braegger, C., Bronsky, J., Campoy, C., Colomb, V., Decsi, T., et al. (2015). Arsenic in rice: a cause for concern. J. Pediatr. Gastroenterol. Nutr. 60, 142–145. doi: 10.1097/MPG.0000000000000502
Honma, T., Ohba, H., Kaneko-Kadokura, A., Makino, T., Nakamura, K., and Katou, H. (2016). Optimal soil Eh, pH, and water management for simultaneously minimizing arsenic and cadmium concentrations in rice grains. Environ. Sci. Technol. 50, 4178–4185. doi: 10.1021/acs.est.5b05424
Hu, P., Huang, J., Ouyang, Y., Wu, L., Song, J., Wang, S., et al. (2013). Water management affects arsenic and cadmium accumulation in different rice cultivars. Environ. Geochem. Health 35, 767–778. doi: 10.1007/s10653-013-9533-z
Huhmann, L. B., Harvey, C. F., Gross, J., Uddin, A., Choudhury, I., Ahmed, K. M., et al. (2021). Evaluation of a field kit for testing arsenic in paddy soil contaminated by irrigation water. Geoderma 382:114755. doi: 10.1016/j.geoderma.2020.114755
IARC (2012). Arsenic, metals, fibres, and dusts. IARC Monogr. Eval. Carcinog. Risks Hum. 100, 11–465.
Ji, X., Liu, S., Juan, H., Bocharnikova, E. A., and Matichenkov, V. V. (2017). Effect of silicon fertilizers on cadmium in rice (Oryza sativa) tissue at tillering stage. Environ. Sci. Pollut. Res. 24, 10740–10748. doi: 10.1007/s11356-017-8730-1
Jia, Y., Huang, H., Zhong, M., Wang, F.-H., Zhang, L.-M., and Zhu, Y.-G. (2013). Microbial arsenic methylation in soil and rice rhizosphere. Environ. Sci. Technol. 47, 3141–3148. doi: 10.1021/es303649v
Kadoglidou, K., Kalaitzidis, A., Stavrakoudis, D., Mygdalia, A., and Katsantonis, D. (2019). A novel compost for rice cultivation developed by rice industrial by-products to serve circular economy. Agronomy 9:553. doi: 10.3390/agronomy9090553
Kang, S.-Y., Morita, S., and Yamazaki, K. (1994). Root growth and distribution in some japonica-indica hybrid and japonica type rice cultivars under field conditions. Jpn. J. Crop Sci. 63, 118–124. doi: 10.1626/jcs.63.118
Karki, S., Adviento-Borbe, M. A. A., Massey, J. H., and Reba, M. L. (2021). Assessing seasonal methane and nitrous oxide emissions from furrow-irrigated rice with cover crops. Agriculture 11:261. doi: 10.3390/agriculture11030261
Khan, M. A., Islam, M. R., Panaullah, G. M., Duxbury, J. M., Jahiruddin, M., and Loeppert, R. H. (2009). Fate of irrigation-water arsenic in rice soils of Bangladesh. Plant Soil 322, 263–277. doi: 10.1007/s11104-009-9914-3
Kim, J., Chaudhary, D. R., Lee, J., Byun, C., Ding, W., Kwon, B.-O., et al. (2020). Microbial mechanism for enhanced methane emission in deep soil layer of phragmites-introduced tidal marsh. Environ. Int. 134:105251. doi: 10.1016/j.envint.2019.105251
Kim, W.-J., Bui, L. T., Chun, J.-B., McClung, A. M., and Barnaby, J. Y. (2018). Correlation between methane (CH4) emissions and root aerenchyma of rice varieties. Plant Breed. Biotechnol. 6, 381–390. doi: 10.9787/PBB.2018.6.4.381
Kim, Y.-H., Khan, A. L., Waqas, M., and Lee, I.-J. (2017). Silicon regulates antioxidant activities of crop plants under abiotic-induced oxidative stress: a review. Front. Plant Sci. 8:510. doi: 10.3389/fpls.2017.00510
Kirchherr, J., Reike, D., and Hekkert, M. (2017). Conceptualizing the circular economy: an analysis of 114 definitions. Resour. Conserv. Recycl. 127, 221–232. doi: 10.1016/j.resconrec.2017.09.005
Kirk, G. (2004). The Biogeochemistry of Submerged Soils. Hoboken, NJ: John Wiley and Sons. doi: 10.1002/047086303X
Klotzbücher, T., Marxen, A., Vetterlein, D., Schneiker, J., Türke, M., van Sinh, N., et al. (2015). Plant-available silicon in paddy soils as a key factor for sustainable rice production in Southeast Asia. Basic Appl. Ecol. 16, 665–673. doi: 10.1016/j.baae.2014.08.002
Knox, S. H., Matthes, J. H., Sturtevant, C., Oikawa, P. Y., Verfaillie, J., and Baldocchi, D. (2016). Biophysical controls on interannual variability in ecosystem-scale CO2 and CH4 exchange in a California rice paddy. J. Geophys. Res. Biogeosci. 121:2015JG003247. doi: 10.1002/2015JG003247
Kritee, K., Nair, D., Zavala-Araiza, D., Proville, J., Rudek, J., Adhya, T. K., et al. (2018). High nitrous oxide fluxes from rice indicate the need to manage water for both long-and short-term climate impacts. PNAS 115, 9720–9725. doi: 10.1073/pnas.1809276115
Kumar, A., Priyadarshinee, R., Roy, A., Dasgupta, D., and Mandal, T. (2016). Current techniques in rice mill effluent treatment: emerging opportunities for waste reuse and waste-to-energy conversion. Chemosphere 164, 404–412. doi: 10.1016/j.chemosphere.2016.08.118
Kumar, A., Roy, A., Priyadarshinee, R., Sengupta, B., Malaviya, A., Dasguptamandal, D., et al. (2017). Economic and sustainable management of wastes from rice industry: combating the potential threats. Environ. Sci. Pollut. Res. 24, 26279–26296. doi: 10.1007/s11356-017-0293-7
Lagomarsino, A., Agnelli, A. E., Linquist, B., Adviento-Borbe, M. A., Agnelli, A., Gavina, G., et al. (2016). Alternate wetting and drying of rice reduced CH4 emissions but triggered N2O peaks in a clayey soil of central Italy. Pedosphere 26, 533–548. doi: 10.1016/S1002-0160(15)60063-7
Lampayan, R. M., Rejesus, R. M., Singleton, G. R., and Bouman, B. A. M. (2015). Adoption and economics of alternate wetting and drying water management for irrigated lowland rice. Field Crops Res. 170, 95–108. doi: 10.1016/j.fcr.2014.10.013
Leeuwis, C., and Aarts, N. (2016). Communication as intermediation for socio-technical innovation. JCOM 15:C02. doi: 10.22323/2.15060302
Leksungnoen, P., Wisawapipat, W., Ketrot, D., Aramrak, S., Nookabkaew, S., Rangkadilok, N., et al. (2019). Biochar and ash derived from silicon-rich rice husk decrease inorganic arsenic species in rice grain. Sci. Total Environ. 684, 360–370. doi: 10.1016/j.scitotenv.2019.05.247
Li, C., Carrijo, D. R., Nakayama, Y., Linquist, B. A., Green, P. G., and Parikh, S. J. (2019). Impact of alternate wetting and drying irrigation on arsenic uptake and speciation in flooded rice systems. Agric. Ecosyst. Environ. 272, 188–198. doi: 10.1016/j.agee.2018.11.009
Li, R. Y., Stroud, J. L., Ma, J. F., McGrath, S. P., and Zhao, F. J. (2009). Mitigation of arsenic accumulation in rice with water management and silicon fertilization. Environ. Sci. Technol. 43, 3778–3783. doi: 10.1021/es803643v
Lian, H.-L., Yu, X., Lane, D., Sun, W.-N., Tang, Z.-C., and Su, W.-A. (2006). Upland rice and lowland rice exhibited different PIP expression under water deficit and ABA treatment. Cell Res. 16, 651–660. doi: 10.1038/sj.cr.7310068
Lian, H.-L., Yu, X., Ye, Q., Ding, X.-S., Kitagawa, Y., Kwak, S.-S., et al. (2004). The role of aquaporin RWC3 in drought avoidance in rice. Plant Cell Physiol. 45, 481–489. doi: 10.1093/pcp/pch058
Liao, B., Wu, X., Yu, Y., Luo, S., Hu, R., and Lu, G. (2020). Effects of mild alternate wetting and drying irrigation and mid-season drainage on CH4 and N2O emissions in rice cultivation. Sci. Total Environ. 698:134212. doi: 10.1016/j.scitotenv.2019.134212
Lim, J. S., Abdul Manan, Z., Hashim, H., and Wan Alwi, S. R. (2013). Toward an integrated, resource-efficient rice mill complex. Resour. Conserv. Recycl. 75, 41–51. doi: 10.1016/j.resconrec.2013.04.001
Lim, J. S., Manan, Z. A., Hashim, H., and Wan Alwi, S. R. (2014). Synthesis of a sustainable integrated rice mill complex. J. Clean. Prod. 71, 118–127. doi: 10.1016/j.jclepro.2013.12.036
Limmer, M. A., Mann, J., Amaral, D. C., Vargas, R., and Seyfferth, A. L. (2018a). Silicon-rich amendments in rice paddies: effects on arsenic uptake and biogeochemistry. Sci. Total Environ. 624, 1360–1368. doi: 10.1016/j.scitotenv.2017.12.207
Limmer, M. A., Wise, P., Dykes, G. E., and Seyfferth, A. L. (2018b). Silicon decreases dimethylarsinic acid concentration in rice grain and mitigates straighthead disorder. Environ. Sci. Technol. 52, 4809–4816. doi: 10.1021/acs.est.8b00300
Linam, F., McCoach, K., Limmer, M. A., and Seyfferth, A. L. (2021). Contrasting effects of rice husk pyrolysis temperature on silicon dissolution and retention of cadmium (Cd) and dimethylarsinic acid (DMA). Sci. Total Environ. 765:144428. doi: 10.1016/j.scitotenv.2020.144428
Linquist, B. A., Adviento-Borbe, M. A., Pittelkow, C. M., van Kessel, C., and van Groenigen, K. J. (2012). Fertilizer management practices and greenhouse gas emissions from rice systems: a quantitative review and analysis. Field Crops Res. 135, 10–21. doi: 10.1016/j.fcr.2012.06.007
Linquist, B. A., Anders, M., Adviento-Borbe, M. A., Chaney, R., Nalley, L., et al. (2015). Reducing greenhouse gas emissions, water use and grain arsenic levels in rice systems. Glob. Chang. Biol. 21, 407–417. doi: 10.1111/gcb.12701
Liu, C., Li, F., Luo, C., Liu, X., Wang, S., Liu, T., et al. (2009). Foliar application of two silica sols reduced cadmium accumulation in rice grains. J. Hazard. Mater. 161, 1466–1472. doi: 10.1016/j.jhazmat.2008.04.116
Liu, H., Long, S.-X., Pinson, S. R. M., Tang, Z., Guerinot, M. L., Salt, D. E., et al. (2021). Univariate and multivariate qtl analyses reveal covariance among mineral elements in the rice ionome. Front. Genet. 12:638555. doi: 10.3389/fgene.2021.638555
Liu, J., Ma, J., He, C., Li, X., Zhang, W., Xu, F., et al. (2013a). Inhibition of cadmium ion uptake in rice (Oryza sativa) cells by a wall-bound form of silicon. New Phytol. 200, 691–699. doi: 10.1111/nph.12494
Liu, L., Chen, T., Wang, Z., Zhang, H., Yang, J., and Zhang, J. (2013b). Combination of site-specific nitrogen management and alternate wetting and drying irrigation increases grain yield and nitrogen and water use efficiency in super rice. Field Crops Res. 154, 226–235. doi: 10.1016/j.fcr.2013.08.016
Liu, S., Liu, N., and Li, J. (1996). Silicosis caused by rice husk ashes. J. Occup. Health 38, 57–62. doi: 10.1539/joh.38.57
Lowe, P., Phillipson, J., and Lee, R. P. (2008). Socio-technical innovation for sustainable food chains: roles for social science. Trends Food Sci. Technol. 19, 226–233. doi: 10.1016/j.tifs.2007.11.005
Lyman, N., and Nalley, L. L. (2013). Economic analysis of hybrid rice performance in arkansas. Agron. J. 105:977. doi: 10.2134/agronj2012.0461
Ma, J., Cai, H., He, C., Zhang, W., and Wang, L. (2015). A hemicellulose-bound form of silicon inhibits cadmium ion uptake in rice (Oryza sativa) cells. New Phytol. 206, 1063–1074. doi: 10.1111/nph.13276
Ma, J. F., Yamaji, N., Mitani, N., Xu, X.-Y., Su, Y.-H., McGrath, S. P., et al. (2008). Transporters of arsenite in rice and their role in arsenic accumulation in rice grain. Proc. Nat. Acad. Sci. U.S.A. 105, 9931–9935. doi: 10.1073/pnas.0802361105
Ma, K., Qiu, Q., and Lu, Y. (2010). Microbial mechanism for rice variety control on methane emission from rice field soil. Glob. Chang. Biol. 16, 3085–3095. doi: 10.1111/j.1365-2486.2009.02145.x
Magrini, M.-B., Anton, M., Chardigny, J.-M., Duc, G., Duru, M., Jeuffroy, M.-H., et al. (2018). Pulses for sustainability: breaking agriculture and food sectors out of lock-in. Front. Sustain. Food Syst. 2:64. doi: 10.3389/fsufs.2018.00064
Magrini, M.-B., Anton, M., Cholez, C., Corre-Hellou, G., Duc, G., Jeuffroy, M.-H., et al. (2016). Why are grain-legumes rarely present in cropping systems despite their environmental and nutritional benefits? Analyzing lock-in in the French agrifood system. Ecol. Econ. 126, 152–162. doi: 10.1016/j.ecolecon.2016.03.024
Maguffin, S. C., Abu-Ali, L., Tappero, R. V., Pena, J., Rohila, J. S., McClung, A. M., et al. (2020). Influence of manganese abundances on iron and arsenic solubility in rice paddy soils. Geochim. Cosmochim. Acta 276, 50–69. doi: 10.1016/j.gca.2020.02.012
Mansaray, K. G., and Ghaly, A. E. (1998). Thermal degradation of rice husks in nitrogen atmosphere. Bioresour. Technol. 65, 13–20. doi: 10.1016/S0960-8524(98)00031-5
Martínez-Eixarch, M., Alcaraz, C., Viñas, M., Noguerol, J., Aranda, X., Prenafeta-Boldú, F. X., et al. (2018). Neglecting the fallow season can significantly underestimate annual methane emissions in Mediterranean rice fields. PLoS ONE 13:e0198081. doi: 10.1371/journal.pone.0198081
Matsumoto, S., Kasuga, J., Taiki, N., Makino, T., and Arao, T. (2015). Inhibition of arsenic accumulation in Japanese rice by the application of iron and silicate materials. Catena 135, 328–335. doi: 10.1016/j.catena.2015.07.004
McCarty, J. L., Justice, C. O., and Korontzi, S. (2007). Agricultural burning in the Southeastern United States detected by MODIS. Remote Sens. Environ. 108, 151–162. doi: 10.1016/j.rse.2006.03.020
McClung, A. M., Rohila, J. S., Henry, C. G., and Lorence, A. (2020). Response of U.S. Rice cultivars grown under non-flooded irrigation management. Agronomy 10:55. doi: 10.3390/agronomy10010055
Meharg, A. A., Sun, G., Williams, P. N., Adomako, E., Deacon, C., Zhu, Y.-G., et al. (2008). Inorganic arsenic levels in baby rice are of concern. Environ. Pollut. 152, 746–749. doi: 10.1016/j.envpol.2008.01.043
Meharg, A. A., Williams, P. N., Adomako, E., Lawgali, Y. Y., Deacon, C., Villada, A., et al. (2009). geographical variation in total and inorganic arsenic content of polished (white) rice. Environ. Sci. Technol. 43, 1612–1617. doi: 10.1021/es802612a
Meharg, C., and Meharg, A. A. (2015). Silicon, the silver bullet for mitigating biotic and abiotic stress, and improving grain quality, in rice? Environ. Exp. Bot. 120, 8–17. doi: 10.1016/j.envexpbot.2015.07.001
Meynard, J.-M., Jeuffroy, M.-H., Le Bail, M., Lefèvre, A., Magrini, M.-B., and Michon, C. (2017). Designing coupled innovations for the sustainability transition of agrifood systems. Agric. Syst. 157, 330–339. doi: 10.1016/j.agsy.2016.08.002
Miao, Z., Shastri, Y., Grift, T. E., Hansen, A. C., and Ting, K. C. (2012). Lignocellulosic biomass feedstock transportation alternatives, logistics, equipment configurations, and modeling. Biofuel Bioprod. Biorefin. 6, 351–362. doi: 10.1002/bbb.1322
Miles, A., DeLonge, M. S., and Carlisle, L. (2017). Triggering a positive research and policy feedback cycle to support a transition to agroecology and sustainable food systems. Agroecol. Sustain. Food Syst. 41, 855–879. doi: 10.1080/21683565.2017.1331179
Mofijul Islam, S. M., Gaihre, Y. K., Shah, A. L., Singh, U., Sarkar, M. I. U., Abdus Satter, M., et al. (2016). Rice yields and nitrogen use efficiency with different fertilizers and water management under intensive lowland rice cropping systems in Bangladesh. Nutr. Cycl. Agroecosyst. 106, 143–156. doi: 10.1007/s10705-016-9795-9
Mohanty, S., Nayak, A. K., Swain, C. K., Dhal, B., Kumar, A., Tripathi, R., et al. (2020). Silicon enhances yield and nitrogen use efficiency of tropical low land rice. Agron. J. 112, 758–771. doi: 10.1002/agj2.20087
Monaco, S., Volante, A., Orasen, G., Cochrane, N., Oliver, V., Price, A. H., et al. (2021). Effects of the application of a moderate alternate wetting and drying technique on the performance of different European varieties in Northern Italy rice system. Field Crops Res. 270:108220. doi: 10.1016/j.fcr.2021.108220
Moreno-García, B., Coronel, E., Reavis, C. W., Suvočarev, K., and Runkle, B. R. K. (2021). Environmental sustainability assessment of rice management practices using decision support tools. J. Clean. Prod. 315:128135. doi: 10.1016/j.jclepro.2021.128135
Myhre, G., Shindell, D., Bréon, F.-M., Collins, W., Fuglestvedt, J., Huang, J., et al. (2013). “Anthropogenic and natural radiative forcing, chapter 8, climate change 2013: the physical science basis,” in Contribution of Working Group I to the Fifth Assessment Report of the Intergovernmental Panel on Climate Change, eds T. S. Stocker, D. Qin, G.-K. Plattner, M. Tignor, S. K. Allen, J. Boschung, A. Nauels, Y. Xia, V. Bex, and P. M. Midgley (Cambridge: Cambridge University Press), 659–740.
Nadar, V. S., Yoshinaga, M., Pawitwar, S. S., Kandavelu, P., Sankaran, B., and Rosen, B. P. (2016). Structure of the ArsI C–as lyase: insights into the mechanism of degradation of organoarsenical herbicides and growth promoters. J. Mol. Biol. 428, 2462–2473. doi: 10.1016/j.jmb.2016.04.022
Nalley, L., Linquist, B. A., Kovacs, K., and Anders, M. (2015). The economic viability of alternative wetting and drying irrigation in arkansas rice production. Agron. J. 107:579. doi: 10.2134/agronj14.0468
Nalley, L., Tack, J., Barkley, A., Jagadish, K., and Brye, K. (2016). Quantifying the agronomic and economic performance of hybrid and conventional rice varieties. Agron. J. 108:1514. doi: 10.2134/agronj2015.0526
Nalley, L., Tack, J., Durand, A., Thoma, G., Tsiboe, F., Shew, A., et al. (2017). The production, consumption, and environmental impacts of rice hybridization in the United States. Agron. J. 109, 193–203. doi: 10.2134/agronj2016.05.0281
Narayanaswamy, C., and Prakash, N. B. (2010). Evaluation of selected extractants for plant-available silicon in rice soils of southern India. Commun. Soil Sci. Plant Anal. 41, 977–989. doi: 10.1080/00103621003646063
Norton, G. J., Douglas, A., Lahner, B., Yakubova, E., Guerinot, M. L., Pinson, S. R. M., et al. (2014). Genome wide association mapping of grain arsenic, copper, molybdenum and zinc in rice (Oryza sativa L.) Grown at four international field sites. PLoS ONE 9:e89685. doi: 10.1371/journal.pone.0089685
Nwugo, C. C., and Huerta, A. J. (2008). Effects of silicon nutrition on cadmium uptake, growth and photosynthesis of rice plants exposed to low-level cadmium. Plant Soil 311, 73–86. doi: 10.1007/s11104-008-9659-4
Oikawa, P. Y., Jenerette, G. D., Knox, S. H., Sturtevant, C., Verfaillie, J., Dronova, I., et al. (2017). Evaluation of a hierarchy of models reveals importance of substrate limitation for predicting carbon dioxide and methane exchange in restored wetlands. J. Geophys. Res. Biogeosci. 122:2016JG003438. doi: 10.1002/2016JG003438
Okutani, T., Ichikawa, E., Nagai, H., Hashimoto, T., and Makihata, T. (2018). Crystalline silica in compressed rice hull coke ash. J. Met. Mater. Miner. 28, 30–38. doi: 10.14456/jmmm.2018.6
Osuna-Canizalez, F. J., De Datta, S. K., and Bonman, J. M. (1991). Nitrogen form and silicon nutrition effects on resistance to blast disease of rice. Plant Soil 135, 223–231. doi: 10.1007/BF00010910
Ouyang, W., Yin, X., Yang, J., and Struik, P. C. (2020). Comparisons with wheat reveal root anatomical and histochemical constraints of rice under water-deficit stress. Plant Soil 452, 547–568. doi: 10.1007/s11104-020-04581-6
Owens, P. R., Wilding, L. P., Miller, W. M., and Griffin, R. W. (2008). Using iron metal rods to infer oxygen status in seasonally saturated soils. CATENA 73, 197–203. doi: 10.1016/j.catena.2007.07.009
Panda, D., Mishra, S. S., and Behera, P. K. (2021). Drought tolerance in rice: focus on recent mechanisms and approaches. Rice Sci. 28, 119–132. doi: 10.1016/j.rsci.2021.01.002
Parker, C., and Nalley, L. (2020). The economics of on-farm rice drying in Arkansas. Stud. J. Dale Bumpers Coll. Agric. Food Life Sci. 21, 69–74. Available online at: https://scholarworks.uark.edu/discoverymag/vol21/iss1/14/
Pati, S., Pal, B., Badole, S., Hazra, G. C., and Mandal, B. (2016). Effect of silicon fertilization on growth, yield, and nutrient uptake of rice. Commun. Soil Sci. Plant Anal. 47, 284–290. doi: 10.1080/00103624.2015.1122797
Pawitwar, S. S., Nadar, V. S., Kandegedara, A., Stemmler, T. L., Rosen, B. P., and Yoshinaga, M. (2017). Biochemical characterization of ArsI: a novel C–as lyase for degradation of environmental organoarsenicals. Environ. Sci. Technol. 51, 11115–11125. doi: 10.1021/acs.est.7b03180
Paye, W., Tubana, B., Harrell, D., Babu, T., Kanke, Y., and Datnoff, L. (2018). Determination of critical soil silicon levels for rice production in louisiana using different extraction procedures. Commun. Soil Sci. Plant Anal. 49, 2091–2102. doi: 10.1080/00103624.2018.1495731
Penido, E. S., Bennett, A. J., Hanson, T. E., and Seyfferth, A. L. (2016). Biogeochemical impacts of silicon-rich rice residue incorporation into flooded soils: implications for rice nutrition and cycling of arsenic. Plant Soil 399, 75–87. doi: 10.1007/s11104-015-2682-3
Pinson, S. R. M., Tarpley, L., Yan, W., Yeater, K., Lahner, B., Yakubova, E., et al. (2015). Worldwide genetic diversity for mineral element concentrations in rice grain. Crop Sci. 55, 294–311. doi: 10.2135/cropsci2013.10.0656
Pittelkow, C. M., Adviento-Borbe, M. A., Hill, J. E., Six, J., van Kessel, C., and Linquist, B. A. (2013). Yield-scaled global warming potential of annual nitrous oxide and methane emissions from continuously flooded rice in response to nitrogen input. Agric. Ecosyst. Environ. 177, 10–20. doi: 10.1016/j.agee.2013.05.011
Pode, R. (2016). Potential applications of rice husk ash waste from rice husk biomass power plant. Renew. Sustain. Energy Rev. 53, 1468–1485. doi: 10.1016/j.rser.2015.09.051
Ponnamperuma, F. N. (1972). The chemistry of submerged soils. Adv. Agro. 4, 29–96. doi: 10.1016/S0065-2113(08)60633-1
Quispe, I., Navia, R., and Kahhat, R. (2017). Energy potential from rice husk through direct combustion and fast pyrolysis: a review. Waste Manag. 59, 200–210. doi: 10.1016/j.wasman.2016.10.001
Rabenhorst, M. C. (2018). A system for making and deploying oxide-coated plastic films for environmental assessment of soils. Soil Sci. Soc. Am. J. 82, 1301–1307. doi: 10.2136/sssaj2018.05.0178
Ramachandran, P., Augstein, F., Nguyen, V., and Carlsbecker, A. (2020). Coping with water limitation: hormones that modify plant root xylem development. Front. Plant Sci. 11:570. doi: 10.3389/fpls.2020.00570
Rao, A. N., Wani, S. P., Ramesha, M. S., and Ladha, J. K. (2017). “Rice production systems,” in Rice Production Worldwide, eds B. S. Chauhan, K. Jabran, and G. Mahajan (Berlin: Springer), 185–205. doi: 10.1007/978-3-319-47516-5_8
Reba, M. L., Fong, B. N., and Rijal, I. (2019). Fallow season CO2 and CH4 fluxes from US mid-south rice-waterfowl habitats. Agric. For. Meteorol. 279:107709. doi: 10.1016/j.agrformet.2019.107709
Reba, M. L., and Massey, J. H. (2020). Surface irrigation in the lower mississippi river basin: trends and innovations. Trans. ASABE 63, 1305–1314. doi: 10.13031/trans.13970
Reddy, C. N., and Patrick, W. H. (1977). Effect of redox potential and pH on the uptake of cadmium and lead by rice plants. J. Environ. Qual. 6, 259–262. doi: 10.2134/jeq1977.00472425000600030005x
Renouf, M. A., Renaud-Gentié, C., Perrin, A., van der Werf, H. M. G., Kanyarushoki, C., and Jourjon, F. (2018). Effectiveness criteria for customised agricultural life cycle assessment tools. J. Clean. Prod. 179, 246–254. doi: 10.1016/j.jclepro.2017.12.170
Roberts, L. C., Hug, S. J., Dittmar, J., Voegelin, A., Saha, G. C., Ali, M. A., et al. (2007). Spatial distribution and temporal variability of arsenic in irrigated rice fields in Bangladesh. 1. Irrigation water. Environ. Sci. Technol. 41, 5960–5966. doi: 10.1021/es070298u
Rodrigues, F. Á., Benhamou, N., Datnoff, L. E., Jones, J. B., and Bélanger, R. R. (2003). Ultrastructural and cytochemical aspects of silicon-mediated rice blast resistance. Phytopathology® 93, 535–546. doi: 10.1094/PHYTO.2003.93.5.535
Runkle, B. R. K., Suvočarev, K., Reba, M. L., Reavis, C. W., Smith, S. F., Chiu, Y.-L., et al. (2019). Methane emission reductions from the alternate wetting and drying of rice fields detected using the eddy covariance method. Environ. Sci. Technol. 53, 671–681. doi: 10.1021/acs.est.8b05535
Ruttan, V. W. (1999). The transition to agricultural sustainability. PNAS 96, 5960–5967. doi: 10.1073/pnas.96.11.5960
Sander, B. O., Schneider, P., Romasanta, R., Samoy-Pascual, K., Sibayan, E. B., Asis, C. A., et al. (2020). Potential of alternate wetting and drying irrigation practices for the mitigation of ghg emissions from rice fields: two cases in central Luzon (Philippines). Agriculture 10:350. doi: 10.3390/agriculture10080350
Satyanarayana, A., Thiyagarajan, T. M., and Uphoff, N. (2007). Opportunities for water saving with higher yield from the system of rice intensification. Irrig. Sci. 25, 99–115. doi: 10.1007/s00271-006-0038-8
Savant, N. K., Datnoff, L. E., and Snyder, G. H. (1997a). Depletion of plant-available silicon in soils: a possible cause of declining rice yields. Commun. Soil Sci. Plant Anal. 28, 1245–1252. doi: 10.1080/00103629709369870
Savant, N. K., Snyder, G. H., and Datnoff, L. E. (1996). “Silicon management and sustainable rice production,” in Advances in Agronomy, ed L. S. Donald (Cambridge, MA: Academic Press), 151–199. doi: 10.1016/S0065-2113(08)60255-2
Savant, N. K., Snyder, G. H., and Datnoff, L. E. (1997b). Silicon management and sustainable rice production. Adv. Agron. 58, 151–199.
Seck, P. A., Diagne, A., Mohanty, S., and Wopereis, M. C. S. (2012). Crops that feed the world 7: rice. Food Sec. 4, 7–24. doi: 10.1007/s12571-012-0168-1
Seebold, K. W., Kucharek, T. A., Datnoff, L. E., Correa-Victoria, F. J., and Marchetti, M. A. (2001). The influence of silicon on components of resistance to blast in susceptible, partially resistant, and resistant cultivars of rice. Phytopathology 91, 63–69. doi: 10.1094/PHYTO.2001.91.1.63
Seyfferth, A. L., Amaral, D., Limmer, M. A., and Guilherme, L. R. G. (2019a). Combined impacts of Si-rich rice residues and flooding extent on grain As and Cd in rice. Environ. Int. 128, 301–309. doi: 10.1016/j.envint.2019.04.060
Seyfferth, A. L., and Fendorf, S. (2012). Silicate mineral impacts on the uptake and storage of arsenic and plant nutrients in rice (Oryza sativa L.). Environ. Sci. Technol. 46, 13176–13183. doi: 10.1021/es3025337
Seyfferth, A. L., Kocar, B. D., Lee, J. A., and Fendorf, S. (2013). Seasonal dynamics of dissolved silicon in a rice cropping system after straw incorporation. Geochim. Cosmochim. Acta 123, 120–133. doi: 10.1016/j.gca.2013.09.015
Seyfferth, A. L., Limmer, M. A., and Dykes, G. E. (2018). On the use of silicon as an agronomic mitigation strategy to decrease arsenic uptake by rice. Agronomy 149, 49–91. doi: 10.1016/bs.agron.2018.01.002
Seyfferth, A. L., Limmer, M. A., and Wu, W. (2019b). Si and water management drives changes in fe and mn pools that affect as cycling and uptake in rice. Soil Syst 3:58. doi: 10.3390/soilsystems3030058
Seyfferth, A. L., Morris, A. H., Gill, R., Kearns, K. A., Mann, J. N., Paukett, M., et al. (2016). Soil incorporation of silica-rich rice husk decreases inorganic arsenic in rice grain. J. Agric. Food Chem. 64, 3760–3766. doi: 10.1021/acs.jafc.6b01201
Shao, J., Che, J., Yamaji, N., Fang Shen, R., and Feng Ma, J. (2017). Silicon reduces cadmium accumulation by suppressing expression of transporter genes involved in cadmium uptake and translocation in rice. J. Exp. Bot. 68, 5641–5651. doi: 10.1093/jxb/erx364
Shen, Y. (2017). Rice husk silica derived nanomaterials for sustainable applications. Renew. Sustain. Energy Rev. 80, 453–466. doi: 10.1016/j.rser.2017.05.115
Shew, A. M., Nalley, L. L., Durand-Morat, A., Meredith, K., Parajuli, R., Thoma, G., et al. (2021). Holistically valuing public investments in agricultural water conservation. Agric. Water Manag. 252:106900. doi: 10.1016/j.agwat.2021.106900
Sidhu, J. K., Stout, M. J., Blouin, D. C., and Datnoff, L. E. (2013). Effect of silicon soil amendment on performance of sugarcane borer, Diatraea saccharalis (Lepidoptera:Crambidae) on rice. Bull. Entomol. Res. 103, 656–664. doi: 10.1017/S0007485313000369
Smartt, A. D., Brye, K. R., and Norman, R. J. (2018). Methane emissions among hybrid rice cultivars in the midsouthern United States. Ann. Adv. Agric. Sci. 2, 1–13. doi: 10.22606/as.2018.21001
Smith, D. B., Solano, F., Woodruff, L. G., Cannon, W. F., and Ellefsen, K. J. (2019). Geochemical and Mineralogical Maps, with Interpretation, for Soils of the Conterminous United States: U.S. Geological Survey Scientific Investigations Report 2017-5118. USGS. doi: 10.3133/sir20175118
Somenahally, A. C., Hollister, E. B., Yan, W., Gentry, T. J., and Loeppert, R. H. (2011). Water management impacts on arsenic speciation and iron-reducing bacteria in contrasting rice-rhizosphere compartments. Environ. Sci. Technol. 45, 8328–8335. doi: 10.1021/es2012403
Song, W.-Y., Yamaki, T., Yamaji, N., Ko, D., Jung, K.-H., Fujii-Kashino, M., et al. (2014). A rice ABC transporter, OsABCC1, reduces arsenic accumulation in the grain. PNAS 111, 15699–15704. doi: 10.1073/pnas.1414968111
Stuart, A. M., Pame, A. R. P., Silva, J. V., Dikitanan, R. C., Rutsaert, P., Malabayabas, A. J. B., et al. (2016). Yield gaps in rice-based farming systems: insights from local studies and prospects for future analysis. Field Crops Res. 194, 43–56. doi: 10.1016/j.fcr.2016.04.039
Su, J., Hu, C., Yan, X., Jin, Y., Chen, Z., Guan, Q., et al. (2015). Expression of barley SUSIBA2 transcription factor yields high-starch low-methane rice. Nature 523, 602–606. doi: 10.1038/nature14673
Sun, L., and Gong, K. (2001). Silicon-based materials from rice husks and their applications. Ind. Eng. Chem. Res. 40, 5861–5877. doi: 10.1021/ie010284b
Teasley, W. A., Limmer, M. A., and Seyfferth, A. L. (2017). How rice (Oryza sativa L.) responds to elevated as under different Si-rich soil amendments. Environ. Sci. Technol. 51, 10335-10343. doi: 10.1021/acs.est.7b01740
Tittonell, P., Klerkx, L., Baudron, F., Félix, G. F., Ruggia, A., van Apeldoorn, D., et al. (2016). “Ecological intensification: local innovation to address global challenges,” in Sustainable Agriculture Reviews, Vol. 19, ed E. Lichtfouse (Cham: Springer International Publishing), 1–34. doi: 10.1007/978-3-319-26777-7_1
Tubana, B. S., Babu, T., and Datnoff, L. E. (2016). A review of silicon in soils and plants and its role in us agriculture: history and future perspectives. Soil Sci. 181, 393–411. doi: 10.1097/SS.0000000000000179
Twedt, D. J., and Nelms, C. O. (1999). Waterfowl density on agricultural fields managed to retain water in winter. Wildl. Soc. Bull. 27, 924–930.
UADA (2018). University of Arkansas Division of Agriculture Rice Production Handbook, MP192. Available online at: https://www.uaex.edu/farm-ranch/crops-commercial-horticulture/rice/ (accessed July 11, 2020).
Ueno, D., Kono, I., Yokosho, K., Ando, T., Yano, M., and Ma, J. F. (2009). A major quantitative trait locus controlling cadmium translocation in rice (Oryza sativa). New Phytol. 182, 644–653. doi: 10.1111/j.1469-8137.2009.02784.x
Uga, Y., Sugimoto, K., Ogawa, S., Rane, J., Ishitani, M., Hara, N., et al. (2013). Control of root system architecture by DEEPER ROOTING 1 increases rice yield under drought conditions. Nat. Genet. 45, 1097–1102. doi: 10.1038/ng.2725
US FDA (2020). Supporting Document for Action Level for Inorganic Arsenic in Rice Cereals for Infants, Available online at: https://www.fda.gov/media/97121/download (accessed May 8, 2020).
USDA-NASS (2020). Quickstats-Rice Yield. Available online at: https://quickstats.nass.usda.gov/results/F3523840-3B81-3C4A-8808-12DEB6811FB7?pivot=short_desc (accessed November 3, 2020).
USDA-NASS (2021). Census of Agriculture. Quick Stats. 2020 Survey. National Agriculture Statistics Service. Available online at: https://www.nass.usda.gov/AgCensus/index.php (accessed January 13, 2021).
Wang, M., Gao, L., Dong, S., Sun, Y., Shen, Q., and Guo, S. (2017). Role of silicon on plant–pathogen interactions. Front. Plant Sci. 8:701. doi: 10.3389/fpls.2017.00701
Wang, X., Samo, N., Li, L., Wang, M., Qadir, M., Jiang, K., et al. (2019). Root distribution and its impacts on the drought tolerance capacity of hybrid rice in the sichuan basin area of China. Agronomy 9:79. doi: 10.3390/agronomy9020079
Wang, Z., Zhang, W., Beebout, S. S., Zhang, H., Liu, L., Yang, J., et al. (2016). Grain yield, water and nitrogen use efficiencies of rice as influenced by irrigation regimes and their interaction with nitrogen rates. Field Crops Res. 193, 54–69. doi: 10.1016/j.fcr.2016.03.006
Williams, P. N., Raab, A., Feldmann, J., and Meharg, A. A. (2007a). Market basket survey shows elevated levels of as in south central U.S. Processed rice compared to California: consequences for human dietary exposure. Environ. Sci. Technol. 41, 2178–2183. doi: 10.1021/es061489k
Williams, P. N., Villada, A., Deacon, C., Raab, A., Figuerola, J., Green, A. J., et al. (2007b). Greatly enhanced arsenic shoot assimilation in rice leads to elevated grain levels compared to wheat and barley. Environ. Sci. Technol. 41, 6854–6859. doi: 10.1021/es070627i
Wu, W., Limmer, M. A., and Seyfferth, A. L. (2020). Quantitative assessment of plant-available silicon extraction methods in rice paddy soils under different management. Soil Sci. Soc. Am. J. 84, 618–626. doi: 10.1002/saj2.20013
Xu, J., Henry, A., and Sreenivasulu, N. (2020). Rice yield formation under high day and night temperatures—a prerequisite to ensure future food security. Plant Cell Environ. 43, 1595–1608. doi: 10.1111/pce.13748
Xu, Y., Gu, D., Li, K., Zhang, W., Zhang, H., Wang, Z., et al. (2019). Response of grain quality to alternate wetting and moderate soil drying irrigation in rice. Crop Sci. 59, 1261–1272. doi: 10.2135/cropsci2018.11.0700
Yan, H., Xu, W., Xie, J., Gao, Y., Wu, L., Sun, L., et al. (2019). Variation of a major facilitator superfamily gene contributes to differential cadmium accumulation between rice subspecies. Nat. Commun. 10:2562. doi: 10.1038/s41467-019-10977-5
Yao, F., Huang, J., Cui, K., Nie, L., Xiang, J., Liu, X., et al. (2012). Agronomic performance of high-yielding rice variety grown under alternate wetting and drying irrigation. Field Crops Res. 126, 16–22. doi: 10.1016/j.fcr.2011.09.018
Yoshinaga, M., and Rosen, B. P. (2014). A C·As lyase for degradation of environmental organoarsenical herbicides and animal husbandry growth promoters. PNAS 111, 7701–7706. doi: 10.1073/pnas.1403057111
Yost, M. A., Sudduth, K. A., Walthall, C. L., and Kitchen, N. R. (2019). Public–private collaboration toward research, education and innovation opportunities in precision agriculture. Precis. Agric. 20, 4–18. doi: 10.1007/s11119-018-9583-4
Yu, Q., Wu, W., Yang, P., Li, Z., Xiong, W., and Tang, H. (2012). Proposing an interdisciplinary and cross-scale framework for global change and food security researches. Agric. Ecosyst. Environ. 156, 57–71. doi: 10.1016/j.agee.2012.04.026
Zhang, M., Pinson, S. R., Tarpley, L., Huang, X.-Y., Lahner, B., Yakubova, E., et al. (2014). Mapping and validation of quantitative trait loci associated with concentrations of 16 elements in unmilled rice grain. Theor. Appl. Genet. 127, 137–165. doi: 10.1007/s00122-013-2207-5
Zhang, Q., and Wang, G. (2005). Studies on nutrient uptake of rice and characteristics of soil microorganisms in a long-term fertilization experiments for irrigated rice. J. Zhejiang Univ. Sci. B 6, 147–154. doi: 10.1631/jzus.2005.B0147
Zhao, F.-J., McGrath, S. P., and Meharg, A. A. (2010). Arsenic as a food chain contaminant: mechanisms of plant uptake and metabolism and mitigation strategies. Annu. Rev. Plant Biol. 61, 535–559. doi: 10.1146/annurev-arplant-042809-112152
Zhao, X., Pu, C., Ma, S.-T., Liu, S.-L., Xue, J.-F., Wang, X., et al. (2019). Management-induced greenhouse gases emission mitigation in global rice production. Sci. Total Environ. 649, 1299–1306. doi: 10.1016/j.scitotenv.2018.08.392
Zhou, S., Sun, H., Bi, J., Zhang, J., Riya, S., and Hosomi, M. (2020). Effect of water-saving irrigation on the N2O dynamics and the contribution of exogenous and endogenous nitrogen to N2O production in paddy soil using 15N tracing. Soil Tillage Res. 200:104610. doi: 10.1016/j.still.2020.104610
Keywords: rice, sustainability, circular food system, conservation agriculture, grain quality, silicon, arsenic, methane
Citation: Runkle BRK, Seyfferth AL, Reid MC, Limmer MA, Moreno-García B, Reavis CW, Peña J, Reba ML, Adviento-Borbe MAA, Pinson SRM and Isbell C (2021) Socio-Technical Changes for Sustainable Rice Production: Rice Husk Amendment, Conservation Irrigation, and System Changes. Front. Agron. 3:741557. doi: 10.3389/fagro.2021.741557
Received: 14 July 2021; Accepted: 27 September 2021;
Published: 22 October 2021.
Edited by:
Walter A. H. Rossing, Wageningen University and Research, NetherlandsReviewed by:
Eve Veromann, Estonian University of Life Sciences, EstoniaMargot Leclère, Institut National de Recherche pour l'agriculture, l'alimentation et l'environnement, France
Copyright © 2021 Runkle, Seyfferth, Reid, Limmer, Moreno-García, Reavis, Peña, Reba, Adviento-Borbe, Pinson and Isbell. This is an open-access article distributed under the terms of the Creative Commons Attribution License (CC BY). The use, distribution or reproduction in other forums is permitted, provided the original author(s) and the copyright owner(s) are credited and that the original publication in this journal is cited, in accordance with accepted academic practice. No use, distribution or reproduction is permitted which does not comply with these terms.
*Correspondence: Benjamin R. K. Runkle, YnJydW5rbGUmI3gwMDA0MDt1YXJrLmVkdQ==