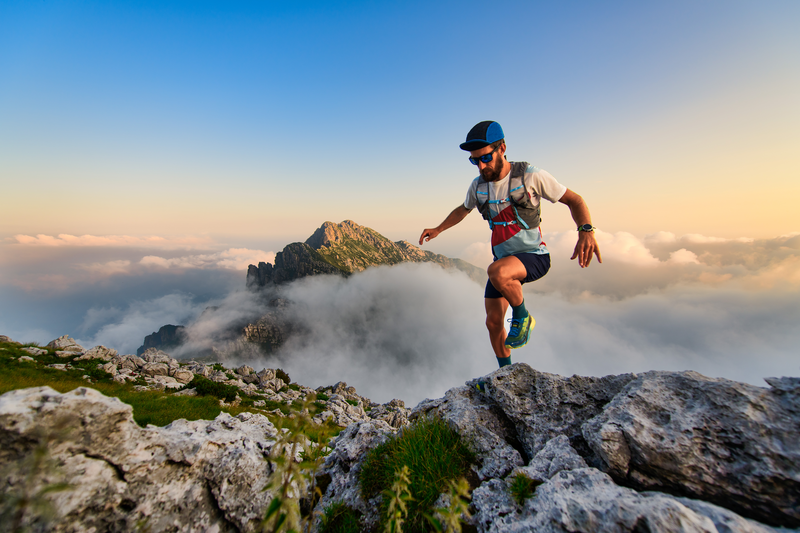
94% of researchers rate our articles as excellent or good
Learn more about the work of our research integrity team to safeguard the quality of each article we publish.
Find out more
ORIGINAL RESEARCH article
Front. Agron. , 15 October 2021
Sec. Agroecological Cropping Systems
Volume 3 - 2021 | https://doi.org/10.3389/fagro.2021.704979
This article is part of the Research Topic Crop Diversification: A Key Pillar for the Agroecological Transition View all 7 articles
In agroecosystems, crop diversification plays a fundamental role in maintaining and regenerating biodiversity and ecosystem services, such as natural pest control. Temporal diversification of cropping systems can affect the presence and activity of natural enemies by providing alternative hosts and prey, food, and refuges for overwintering. However, we still lack studies on the effects of temporal diversification on generalist predators and their biocontrol potential conducted at field scale in commercial agricultural settings. Here, we measured proxies of ecosystem functions related with biological pest control in 29 commercial agricultural fields characterized by cereal-based cropping system in Lower-Saxony, northern Germany. The fields differed in the number of crops and cover crops cultivated during the previous 12 years. Using the Rapid Ecosystem Function Assessment approach, we measured invertebrate predation, seed predation and activity density of generalist predators. We aimed at testing whether the differences in the crop rotations from the previous years would affect activity of predators and their predation rates in the current growing season. We found that the length of the crop rotation had neutral effects on the proxies measured. Furthermore, predation rates were generally lower if the rotation comprised a higher number of cover crops compared to rotation with less cover crops. The activity density of respective taxa of predatory arthropods responded differently to the number of cover crops in the crop rotation. Our results suggest that temporal crop diversity may not benefit the activity and efficiency of generalist predators when diversification strategies involve crops of very similar functional traits. Adding different resources and traits to the agroecosystems through a wider range of cultivated crops and the integration of semi-natural habitats are aspects that need to be considered when developing more diverse cropping systems aiming to provide a more efficient natural pest control.
Biological control of pests by natural enemies represents an important ecosystem service in agriculture. Natural enemies can significantly affect population dynamics of many phytophagous invertebrates, contributing to their regulation in arable agroecosystems (Kremen and Chaplin-Kramer, 2007; Bengtsson, 2015). Generalist ground-dwelling predators such as carabids (Coleoptera: Carabidae), spiders (Arachnida: Araneae), and staphylinids (Coleoptera: Staphylinidae), can occur in arable fields in large number and, given their polyphagous feeding habits, they can play a relevant role in suppressing pest populations (Ekschmitt et al., 1997). The occurrence of these generalist predators and hence the provision of the natural biocontrol service is threatened in many cropping systems through agricultural intensification resulting in loss of landscape elements, enlarged farm and field sizes, higher inputs of fertilizer and altogether a homogenization of land use and land management (Holland and Luff, 2000; Geiger et al., 2010; Rusch et al., 2013a). It has been shown, that more diverse cropping systems may enhance abundance and diversity of beneficial arthropods (Letourneau et al., 2011; Kremen and Miles, 2012; Lichtenberg et al., 2017). Diversified agroecosystems may provide alternative prey and hosts, food and suitable habitats for predators and parasitoids, allowing them to overwinter and persist (Altieri and Nicholls, 2004). The structure and heterogeneity of landscapes and in particular a large proportion of semi-natural land cover are suggested as key factors in determining biodiversity and associated ecosystem services in agricultural landscapes (Fahrig, 2013; Martin et al., 2019). Pests and natural pest control, however, show inconsistent responses to landscape composition, landscape configuration and semi-natural habitats surrounding the crop fields (Chaplin-Kramer et al., 2011; Birkhofer et al., 2018; Karp et al., 2018). Also, in many intensively managed agricultural landscapes, cover of semi-natural habitats is low as some agri-environmental and greening measures are rarely implemented by farmers, often because they do not receive the appropriate economic support and advisory service for their participation in nature protection measures (Stupak et al., 2019). Increasing landscape heterogeneity by increasing the number and diversity of crops in such intensively managed landscapes may therefore be an option more readily adopted by farmers (Zinngrebe et al., 2017). There is evidence that increasing crop heterogeneity can be an effective way to increase biodiversity in agricultural landscapes without taking land out of agricultural production (Sunderland and Samu, 2000; Sirami et al., 2019). Bosem Baillod et al. (2017) reported that cereal aphid numbers can be reduced by optimizing the composition, configuration, and temporal heterogeneity of the crop mosaic. Increasing the diversity of crop types in a landscape can contribute to the conservation of service-providing arthropod communities such as carabids, particularly if the diversification happens in landscapes with a high proportion of semi-natural habitats (Aguilera et al., 2020). However, an increase in enemy abundance does not always translate to a concomitant increase in pest control (Chaplin-Kramer et al., 2011), since both positive and negative interactions may occur among natural enemy species (Letourneau et al., 2009). Thus, it is necessary not only to measure abundance and diversity of generalist predators, but also to assess their potential contribution to pest control.
Temporal (e.g., crop rotations, catch, or cover crops) and spatial (e.g., intercropping, strip cropping) diversification strategies of cropping-systems are available for modifying the structure and the arrangement of habitats and resources in time and space, in order to maintain and regenerate biodiversity (Altieri, 1999). Several studies have investigated the effects of cropping systems' temporal management on abundance and diversity of generalist aboveground arthropods, reporting different responses. Brust and King (1994), for instance, found that these organisms might be positively affected by crop rotation in comparison to monocultural systems. A more recent study by Meyer et al. (2019), however, did not show any consistent effects of temporal crop diversity on generalist predators in terms of activity density. Contradictory results have been reported also by studies assessing the effects of winter cover crops on activity density of generalist predators (Lundgren and Fergen, 2010; Fox et al., 2016). All those studies on temporal diversification reported data from experimental trials. What is largely lacking are studies on the topic conducted at field scale in commercial agricultural settings.
We conducted the present study in a water protection area under commercial agricultural management in Northern Germany, which is under the counsel of the Landwirtschaftskammer Niedersachsen (LWK). We selected fields of a cereal-based cropping system within the water protection area that differed in the length of the crop rotation and in the number of cover crops cultivated in the previous 12 years. The aim of this study was to measure biocontrol potential of generalist predators at the moment during the season when they achieve high densities. We hypothesized that (I) a higher number of crops in the rotation and (II) a higher number of winter cover crops during the previous years would result in increased activity density and predation rates of generalist predators in the fields in the current growing season. We also predicted that (III) current crop and phenological growth stage at the time of sampling would affect activity density of generalist predators. To test these hypotheses, we applied a Rapid Ecosystem Function Assessment (REFA, Meyer et al., 2015) approach measuring a set of ecosystem function proxies relevant for biological pest and weed control. In addition, we measured the activity density of the ground-dwelling predatory arthropods at the time of the REFA-sampling to link the observed predation patterns to the presence and activity of different predator taxa.
To explain whether the emerging differences in activity density and predation rates are determined more by the recent or by the past crop rotation management, we first analyzed the effects of crop rotation and cover crop management for the period 2007–2018, and then for the period 2015–2018 [i.e., the current period of the Common Agricultural Policy (CAP) of the European Union].
The study was conducted in a water protection area near the town of Liebenau, Lower Saxony, Germany (Figure 1). In this area, the mean annual temperature is 9.7°C, yearly precipitation is 762 mm and total summer rainfall (from April through September) is 374 mm in the long-term average (1981–2010). In 2018, this region experienced particularly dry and hot conditions, which resulted in a total summer rainfall (Apr-Sep) of 161 mm and a mean annual temperature of 11.05°C measured by the meteorological station in Nienburg an der Weser (Deutscher Wetterdienst, 2020).
Figure 1. Location of the 29 investigated fields (in light gray) within the water protection area of Liebenau (Copernicus Sentinel-2 data, 2020).
The study region is characterized by farms specialized in dairy production and arable crops. The typical crop rotation is based on the cultivation of silage maize (Zea mays L.) as main cash crop in rotation with other cereals, in particular rye (Secale cereale L.), barley (Hordeum vulgare L.), and wheat (Triticum aestivum L.). Winter cover crops are mainly cultivated mixtures of crucifers (e.g., radish [Raphanus L.] and mustard [Sinapis L.]), sometimes in association with Phacelia (Phacelia tanacetifolia Benth.) and cereal grasses. While ryegrass (e.g., genus Lolium L.) is often cultivated as sole cover crop. Under the German rules of EU's ecological focus areas (Zinngrebe et al., 2017), cover crops must be made up of a sown mix of at least two different crop types. Farmers can use any percentage of a sown mix, as long as there is a visible mix of at least two different crops. They are typically sown in the fall after the main crops or are established through undersowing, and are retained until late winter (mid-January at least, mid-February under greening rules). The way of termination of the cover crops depends on the species cultivated, and in particular, whether they are sensible to frost or not. All the cover crops have in common that their residues were incorporated in the soil through tillage after termination and before the cash crop is planted.
A high level of farming intensification in the region, combined with large use of external inputs, e.g., nitrogen fertilizers, has led to increased nitrate concentration in the ground drinking water. The LWK works together with the conventional farmers in the area toward more sustainable farming systems aiming to reduce the negative impacts of intensive farming production on the quality of drinking water in the basin. Reduced use of nitrogen fertilizers, reduced tillage, diversification of the crop rotations as well as addition of cover crops and undersown crops have been identified as effective measures to challenge the nitrate leaching issue in the area. Some of the farmers have subscribed to those measures on a voluntary basis. For each field included in the voluntary programme, the LWK has documented the number of crops in the rotation, the number of winter cover crops and hence the number of crop changes from 1 year to the next during a period of 12 years (2007–2018). The reform of the European Common Agricultural Policy (European Commission, 2013) affected the rotation regimes of the selected fields. During this time (2015–2018), several farmers in the region implemented the cultivation of cover crops and green cover, transposing the new “greening” measures.
The fields were selected among those under the guidance of the local LWK office, excluding those for which the information on crop rotation management was incomplete. Further care was taken that the fields represented the typical crop rotation and land management of the region and that they were cultivated continuously during the investigated period. Therefore, fields cultivated with specialized crops, managed as grassland or lying fallow for some period were excluded. This selection resulted in a final number of 30 available fields, from which one was excluded due to technical reasons. Detailed information on crop rotations of the 29 investigated fields can be found in the Supplementary Tables S1, S2.
The fields were allocated in different categories based on the length of the rotation, the number of annual changes of crops, and the number of winter cover crops during a period of 12 years (2007–2018). In particular, the “short rotation” fields contained ≤ 2 main crops while the “long rotation” contained at least three main crops. In addition, in the fields of the category “long rotation,” the main crop cultivated (among those present in the rotation) was different from 1 year to the next at least six times during the 12 years' period. Concerning the number of cover crops, the fields were divided into two categories: fields with more than or equal to five times cover crop cultivation during those 12 years (“WCC”: With Cover Crops), and fields with less than or equal to two times of cover crop cultivation during the same period (“NCC”: No Cover Crops).
Following the entry into force of the CAP reform, more cover crops have been cultivated in some of the investigated fields, modifying their crop temporal management. Therefore, the same fields were reallocated in different categories based on the length of the rotation and the number of cover crops of the last 4 years of crop rotation management (2015–2018, i.e., the current CAP period). In terms of length of rotation, we identified and separated in categories fields with three crops (“long”) and fields with only one or two crops in the rotation (“short”). In terms of number of cover crops, we had fields with two or three cover crop cultivation (“wcc”: with cover crops) and fields with one or zero cover crop cultivation during those 4 years (“ncc”: no cover crops). The allocation of the investigated fields in the different categories for both periods 2007–2018 and 2015–2018 is summarized in Table 1. In addition, the cultivation of a cover crop in winter 2017/2018, preceding the main crop at the time of sampling, was considered in our analyses.
Table 1. Overview of the different categories based on the crop rotation management for the periods 2007–2018 and 2015–2018 and number of fields investigated for each category.
At the time of our sampling, surveyed fields were sown mainly with maize, with a smaller number of surveyed fields sown to barley, rye, field grass and rapeseed (Brassica napus L.). Furthermore, fields differed in terms of phenological growth stage of the plants. In particular, maize plants were in early reproductive stage (BBCH ≥ 51) in nine fields, and in vegetative stage (BBCH ≤ 39) in ten fields, while the remaining cereals were in late reproductive growth stage (BBCH ≥ 73). Thus, we grouped the fields in three categories: maize early growth stage, maize late growth stage and cereals (Supplementary Table S3).
Information on field management practices were obtained directly from the farmers through questionnaires. In particular, data on soil management operations and pesticide applications in the season 2018 were collected. We received feedback from the farmers for 16 of the investigated fields. Therefore, we performed an additional analysis on a smaller dataset in order to test the effects caused by field operations on predators' activity density regardless of crop rotation management variables and current crop cultivated. The number of pesticide (considering both insecticides and herbicides) applications during the growing season up to the time of our observations varied between one and three, while only one field was not treated. Fields were divided based on the time interval between the last pesticide application and our observations [<45 days (n = 6) and >45 days (n = 9)]. Regarding soil management, we had 7 fields managed under conventional tillage and 9 under no-tillage regimes (Supplementary Table S10).
Landscape composition of the study area was analyzed in order to calculate the proportion of semi-natural habitats. We defined semi-natural land cover as grassland, moorland and small patches of woodland. Considering the spatial proximity of the investigated fields, we did not calculate the landscape composition for each field separately. We selected an area of the landscape which embedded the buffers of 1-km radius (Aguilera et al., 2020) surrounding all the 29 investigated fields (Supplementary Figure S2). Proportion of semi-natural habitats was calculated using a land-use digitalized map layer (Digitales Landbedeckungsmodell für Deutschland DLM-DE2009, Referenzjahr 2009) in ArcMap software (version 10.5.1, ESRI). Land cover classes were identified based on the CORINE Land Cover Nomenclature. The total proportion of semi-natural habitats in the landscape was 12.7%, with the most common semi-natural habitats represented by grassland patches.
Data were collected the first week of July 2018. We measured the ecosystem function proxies following the Rapid Ecosystem Function Assessment (REFA, Meyer et al., 2015) approach. From all the proxies proposed by the REFA, we selected those of particular interest for biological pest control. These proxies were measured mid-season when predators achieve highest densities and may provide an important contribution to pest control. In particular, we assessed insect predation calculating the predation rates of standardized baits and the attack rates on artificial caterpillars, and seed predation calculating the removal rates of weed seeds. Furthermore, we measured the activity density of epigeal predatory arthropods during the exposure of the baits in the fields. We expected to observe differences in terms of activity of predators and predation rates driven by the previous crop rotation and cover crop management. Proxies were measured on the ground during 48 h in three sampling stations per field, which were installed at a distance of 20 meter from the field edge and 15 meter from each other. Each sampling station consisted of three 90 mm-diameter Petri dishes buried flush to the ground with the bait items (i.e., two artificial caterpillars, ten mealworms, and ten seeds of three different weed species, respectively) fixed on them and one pitfall trap to assess activity density. Sampling items were placed at each corner of a square of 50 cm side length. Vertebrates were excluded from mealworms and weed seeds using exclosure cages made of wire net (12.5 × 12.5 cm) with 1.2 cm2 mesh size secured to the ground with four steel staples.
Artificial caterpillars made from light green modeling clay (Staedtler, Nürnberg, Germany) were used to estimate the predation rates by different predator groups, both invertebrate and vertebrate (Howe et al., 2009). The dummies were 25 mm long and 5 mm wide (Low et al., 2014), glued on small pads of wood and fixed on the dishes previously sprayed with an aerosol glue and covered with fine sand. Dummies were collected and checked for attack marks using a stereo microscope. Attack marks were attributed to either arthropod, mammal, or bird predators based on the collection of images from Low et al. (2014) (Supplementary Figure S1). Each dummy was scored for presence or absence of arthropod and vertebrate predation (i.e., binary response 0/1). This binary dependent variable was tested in the statistical models.
Insect predation rates were assessed also using dried mealworms (larval stage of the flour beetle Tenebrio molitor L.) as a bait (Hertzog et al., 2017). Mealworms were placed on dishes previously sprayed with an aerosol glue and covered with fine sand. The number of removed or attacked mealworms was recorded, counting as predated also those partially consumed. The proportion of mealworms predated per number of mealworms exposed was used as response variable in the statistical models.
Seed predation was assessed by measuring removal rates of artificially placed seeds of the three weed species Sinapis arvensis L., Capsella bursa-pastoris (L.) Medik., and Anthriscus sylvestris (L.) Hoffm. Seeds were fixed on the dish previously covered with a layer of sand paper (grain size 80) sprayed with repositionable glue (3M Spray mount) (Westerman et al., 2003). The weed species were selected on the basis of their different size and shape, allowing the predation by a wide range of granivory arthropods. The dishes were collected and closed with a parafilm strip to avoid losses due to handling and transport. Seed predation rates were assessed by counting removed seeds of each species. The proportion of predated seeds per number of exposed seeds was used as response variable in the statistical models.
The activity density of epigeal predatory arthropods at the time of REFA-sampling was investigated using pitfall traps. The traps consisted of 120 ml jars (70 mm high with a diameter at the mouth of 50 mm) flush buried with the soil surface. The containers were filled (40 ml) with a saturated salt (NaCl) water solution, which allowed for a long and good preservation of the arthropods (Teichmann, 1994). Some drops of detergent were added to reduce surface tension. Traps were collected and stored in a cold room before sorting. Based on morphological features, the specimen were classified into different taxa of ground dwelling predators: carabids, spiders, staphylinids, harvestmen (Arachnida: Opiliones); and others arthropods (e.g., ants [Hymenoptera: Formicidae]). The number of specimens in each group were counted and used as response variable in the models. After sorting, pitfall-trap catches were stored in 80% ethanol solution, prior to weighing. The weight was obtained in an alcohol-wet state following the method described by Schwan et al. (1993). The fluid content was subtracted pouring the arthropods onto a sieve of 0.8 mm mesh width. The time between two drops was measured and when it reached 10 s for the first time, the weight was assessed with a laboratory scale.
Statistical analyses were conducted with R (R Core Team, 2018). The effects of crop rotation management 2007–2018, crop rotation management 2015–2018, crop identity and phenological growth stage were tested for each proxy separately using generalized linear mixed models. In a first set of models, the categories based on the length of the rotation (“long” and “short”) and on the number of cover crops (“WCC” and “NCC”) during the period 2007–2018 were used as explanatory variables. In a second analysis, the categories obtained considering the last 4 years (2015–2018) based on the length of the rotation (“long” and “short”) and on the number of cover crops (“wcc” and “ncc”) were used as explanatory variables in the new models. In this last set of models, the cultivation of a cover crop in winter 2017/2018 was also included as fixed factor and considered in the final models only if significant. Finally, a separate set of models was used to test the effects of current crop and phenological growth stage on the proxies investigated.
All the models were fit using the package glmmTMB (Brooks et al., 2017). Predation rates data (binary and proportional response) were modeled using a binomial error structure with logit link function, while a Poisson distribution structure with log link function was used for activity density models. If necessary, a negative binomial error structure with log link function was used in order to account for overdispersion. For the proxies activity density and weed seeds predation, the terms predator taxa and seed species were included as explanatory variables in the models, respectively. Random effect for field was included in the models. For the period 2007–2018, two-way interactions between each of the categories based on the crop rotation management and the terms taxa and species were included. Two-way interactions were included also between taxa and categories of crop rotation management 2015–2018 and current crop 2018.
Regardless of crop rotation management and crop identity, an additional analysis was carried out to test whether field management practices (tillage and time of last pesticide application) had any effects on the activity density of predators' taxa. Models included the same error structure as those in the main analysis.
The Wald Chi-Squared Test through the Anova function in the car package (Fox and Weisberg, 2011) was used to test if the explanatory variables in the models were significant. Type III Chi-Square Test was used in the models with significant interaction terms. After fitting the models, pairwise differences among variables were tested using Tukey's post hoc test through the emmeans function from the emmeans package (Lenth, 2018).
After exposure, 99.4% of dummy caterpillars were recovered and could be assessed. On average, 24.8% of artificial caterpillars showed predation marks. In terms of identity of predator groups, attack marks caused by arthropods were found in 50% of the dummies attacked, while marks caused by vertebrates were found in 54,7% of the dummies attacked, with almost the totality of the latter were caused by rodents and only one case was attributed to birds.
Predation rates on mealworms were on average 39%; whereas predation rates on weed seeds were overall extremely low. For all the three weed species exposed, predation rates were below 1%.
The weight of the biomass of the arthropods collected was on average 600 mg per pitfall trap, with predators (carabids and spiders) and ants representing the most collected taxa. In respect to the different taxa of predators sampled, spiders showed the highest share (198 individuals collected), followed by carabids (124 individuals), while staphylinids were collected in a very low number (20 individuals).
Total attack rates on dummy caterpillars caused by both arthropod and vertebrate predators were not affected by the long-term crop rotation management 2007–2018 (Supplementary Table S8). Arthropod attack rates on dummies were also not influenced by the length of rotation (6% ± 4; P = 0.983; Table 2). In contrast, arthropod attack rates were strongly influenced by the number of cover crops cultivated (Table 2). In particular, attack rates were higher in the NCC system (14% ± 6) compared to the WCC system (3% ± 2) (P = 0.040).
Table 2. Results of the generalized linear mixed models on the effects of all explanatory variables on ecosystem function proxies measured for predatory arthropods.
The length of rotation did not affect predation rates on mealworms significantly (P = 0.347), with 26% ± 9 of mealworms predated in fields of the category long rotation and 42% ± 15 of predation in those of the category short rotation (Table 2, Figure 2A). This proxy was also strongly affected by the number of cover crops cultivated (Table 2, Figure 2B). NCC fields showed significantly higher predation rates on mealworms than WCC fields (68% ± 12 vs. 11% ± 5; P < 0.001).
Figure 2. Effects of crop rotation management 2007–2018. Arthropod predation rates on mealworms in relation to length of rotation (A), number of cover crops (B), activity density of the main taxa of epigeal predatory arthropods collected through pitfall traps in relation to length of the rotation (C), and number of cover crops (D). Boxplots show the raw data while red points and bars show the estimated marginal mean with standard error (SE).
Weed seed predation rate was overall below 1% and was not affected neither by the length of the rotation nor by the number of cover crops cultivated (Table 2). Regarding the proportion of predation of the different seed species, for the smaller seeds of C. bursa-pastoris we registered higher predation in comparison to the other species in NCC fields (P = 0.020 vs. A. sylvestris, P = 0.035 vs. S. arvensis). No differences were observed for the length of the rotation (Table 2).
The biomass of arthropods collected with pitfall traps did not differ in relation neither to the length of the rotation nor to the number of cover crops cultivated (Supplementary Table S9). Activity density of epigeal predatory arthropods was not affected by the length of the rotation for the period 2007–2018 (P = 0.490) (Table 2, Figure 2C). When considering the cultivation of cover crops, activity density of spiders was significantly higher in NCC than WCC (P = 0.018), while the number of cover crops cultivated did not influence activity density of carabids (P = 0.558) and staphylinids (P = 0.339) (Table 2, Figure 2D).
In relation to the crop rotation management of the last 4 years (2015–2018), total attack rates on artificial caterpillars were not affected by any of the factors (Supplementary Table S8). In the same way, attack rates by arthropods did not differ in relation to the number of crops in the rotation (7% ± 5 long rotation, 6% ± 3 short rotation, P = 0.799). Arthropods attack rates were on average lower in wcc fields (4% ± 3) compared with ncc fields (10% ± 5), but no significant differences were registered (P = 0.221) (Table 2).
Marginal significant effects of the length of the rotation on the predation rates of mealworms were observed (P = 0.085), with a predation rate of 13% ± 8 in the long and 41% ± 12 in the short rotation (Table 2, Figure 3A). The number of cover crops in the rotation had consistent effects on the observed predation rates on mealworms (P = 0.004). Specifically, predation rates were 9% ± 5 in wcc system and 51% ± 13 in the ncc system (Table 2, Figure 3B).
Figure 3. Effects of crop rotation management of the last 4 years (2015–2018). Arthropod predation rates on mealworms in relation to length of rotation (A), number of cover crops (B), activity density of the main taxa of epigeal predatory arthropods collected through pitfall traps in relation to length of rotation (C), and number of cover crops (D). Boxplots show the raw data while red points and bars show the estimated marginal mean with standard error (SE).
Seed predation was not affected by any of the parameters considered, showing no differences between different length of rotation and cover crop management (Table 2). For the smaller seed species C. bursa-pastoris we observed higher predation rates in comparison to A. sylvestris (P = 0.009), but not compared to S. arvensis (P = 0.376).
Total biomass of the arthropods collected by pitfall traps was not affected by the crop rotation management 2015–2018 (Supplementary Table S9). The number of crops in the rotation did not affect significantly the activity density of any of the predators' taxa investigated (P = 0.546) (Table 2, Figure 3C). With regards to the cover crops, activity density of carabids (P < 0,001) and spiders (P = 0.041) were significantly higher in ncc fields (carabids: 5.3 ± 1.6, spiders: 6.8 ± 1.3) in comparison to wcc (carabids: 1.6 ± 0.4, spiders: 3.9 ± 0.8), while activity density of staphylinids did not differ between the two systems (P = 0.243) (Figure 3D).
Finally, the cultivation of a cover crop in winter 2017–2018 before the main crop did not affect any of the ecosystem function proxies investigated (Table 2).
The crop grown in 2018 did not have significant effects on the predation rates by epigeal predators on dummy caterpillars (P = 0.193), mealworms (P = 0.628), and weed seeds (P = 0.632). Also, no significant effects were observed between different phenological growth stages of the plants (BBCH Scale) in the maize fields when considering only arthropod attack rates on artificial caterpillars (P = 0.177). Activity density of single taxa was not affected by crop identity and phenological growth stage (P = 0.297). In maize fields at advanced stage (BBCH ≥ 51), the number of spiders was significantly higher than the number of carabids collected (P < 0.001). This difference between taxa was not observed in the maize fields at earlier growth stage (BBCH ≤ 39) (P = 0.218) and in cereal fields (P = 0.972).
The impact of vertebrate predation (mostly caused by rodents) on the dummy caterpillars was considerable in relation to the crops present in the fields and their growth stage. Total attack rates by arthropods and vertebrates on dummy caterpillars were lower in maize fields (17% on average) in comparison to fields cultivated with other cereals (36% ± 7) (Figure 4). Differences were observed also between maize fields at advanced stage of development (BBCH ≥ 51) and those at earlier stage of development (BBCH ≤ 39), showing significantly lower percentage of attacked dummies in the latter (29% vs. 6%) (P = 0.013) (Figure 4).
Figure 4. Total attack rates by vertebrates and arthropods on dummy caterpillars in relation to current crop (2018) and phenological growth stage. Bars show the estimated marginal mean with standard error (SE).
Pesticides were applied in almost all of the fields considered for the analysis, with the time of the last pesticide application before our measurements not affecting the activity density of any of the investigated taxa. On the contrary, soil management affected the activity density of carabids, which was lower in fields that were plowed during the cropping season compared to fields under no tillage regime (Table 3). Finally, activity density of all predators and spiders was not influenced by soil management practices (Supplementary Table S11).
Table 3. Effects of soil management and time of last pesticide application on the activity density of generalist predators.
The main objective of this study was to determine whether a wider crop rotation and the addition of winter cover crops in the cropping system during the previous years influenced predation rates and activity density of predatory epigeal arthropods. We expected to observe these effects driven by the previous crop rotation management on the ecosystem functions measured in the field during the current growing season. To test these hypotheses, we measured a set of standardized proxies at a specific moment following the Rapid Ecosystem Function Assessment (REFA) approach. The use of insect and artificial prey is a common method used to measure predation rates (Lövei and Ferrante, 2017), and in our study dummy caterpillars and mealworms were effective in measuring predation pressure and detecting differences between systems tested. Differences in the predation rates between types of bait could be explained by the higher attraction of predators to the mealworms compared to the artificial caterpillars. Weed seed predation rates were overall low and were not affected by any of the crop rotation management variables. Finally, the pitfall traps used in this study allowed us to assess the presence and activity of the main arthropod predator taxa during the REFA-sampling.
In contrast to our first hypothesis (I), we found that the predation rates and the activity density of generalist predators were not affected by crop rotation. Neither long (2007–2018) nor short (2015–2018) term effects of crop rotation were detected. Winter cover crops in the crop rotations did show effects on predators' activity density and on predation rates but not in the way we had expected (second hypothesis, II). In fact, we found that the winter cover crop cultivation did not increase either predation by epigeal generalist predators nor their activity density in the field. Finally, the current crop cultivated on the fields had no effects on the parameters investigated (third hypothesis, III). Only the total attack rates on artificial caterpillars were affected by the current crop and the growth stage of the plants when we considered also the vertebrate attack rates, which was mainly caused by small mammals.
We observed that a wider crop rotation did not enhance any of the ecosystem function proxies investigated, showing neutral effects on the levels of predation and activity density of predators. None of the parameters investigated was affected by the number of crops present in rotation, when considering both long (2007–2018) and short (2015–2018) term management periods. Other studies have reported similar results. Meyer et al. (2019), for instance, found inconsistent effects of temporal crop diversity on activity density of arthropods, reporting that species groups had different responses to the number of crops in the rotation. Similarly, Dunbar et al. (2016) showed that activity density of individual predator taxa was not affected by rotation scheme, with no positive effects for the ground-dwelling predator community. Cropping systems in our study are dominated by maize, with few other crops (mainly annual cereals) added in the rotations. This leads to a temporal variation among cereal crops having similar vegetation structure, microhabitat and resources. It is therefore possible that the addition of these crops with similar functional traits in the rotation did not benefit natural enemies. In this context, it is relevant to specify that the study region is located within a water protection area, which is subjected to strict rules concerning the crops allowed for the cultivation, narrowing the range of species available to the farmers.
Landscape-scale habitat composition and configuration can also influence pest pressure and biological pest control. Although previous studies often failed to find a clear landscape-biocontrol relationship, showing inconsistent effects of these variables on the performance of natural enemies (Tscharntke et al., 2016; Karp et al., 2018), landscape complexity remains one of the key factors driving the conservation of service-providing arthropod communities in agricultural environments (Aguilera et al., 2020). Rusch et al. (2013b) demonstrated that combined management of semi-natural habitat and crop rotation can stabilize and enhance natural pest control in agricultural landscapes, independent of crop rotation intensity alone. Aguilera et al. (2020) showed that the diversity of arthropod communities benefits from a higher crop diversity in landscapes that are also rich in semi-natural habitats, compared to landscapes with low proportions (10%) of semi-natural habitats, and Tscharntke et al. (2016) suggested a threshold level of 20% natural habitat at the landscape scale as the minimum amount of natural habitat necessary to support biocontrol services. Hence, it is plausible that the amount of semi-natural habitats in our study area (i.e., 12.7%) may have been too low to support the benefits on ecosystem functions expected from the temporal crop diversification.
In terms of predation rates of weed seeds, we observed overall very low predation rates. No effects of the length of rotation and cultivation of cover crops were found for this ecosystem function proxy. The generally low activity density of ground-dwelling predators observed in our fields may be the explanation for such low predation of weed seeds, with particular regards to carabids, which are one of the most important seed predators in crop fields (Honek et al., 2003). Higher predation of the species C. bursa-pastoris can be explained with the fact that smaller seeds are preferred by arthropods (Saska et al., 2014), while small mammals and birds prefer larger seeds, with the vertebrates playing a very important role as weed seeds predators in agroecosystems (Westerman et al., 2003; Navntoft et al., 2009). Vertebrates were excluded from our assessment using an exclosure cage, therefore their contribution to weed seeds predation was not investigated here.
We found that predation rates were generally lower in fields that comprised a higher number of cover crops compared to fields with less cover crops in the rotation. In particular, for predation on mealworms this difference was remarkable in both management periods investigated, while attack rates on artificial caterpillars were significantly different only in the long-term period. Predation rates were in line with the activity density, which was generally higher in fields with lower number of cover crops, but with different results between taxonomic groups. We found more spiders in fields with low number of cover crops when considering both long and short-term management periods, and more carabids when considering the short-term period only. Staphylinids, on the contrary, were not influenced by cover crop management at all. Cover crops cultivation is expected to increase many supporting and regulating ecosystem services in agroecosystems, including beneficial insect activity, as result of legacy effects of the cover crops on the following cash crop (Schipanski et al., 2014). However, contrasting results on cover crop effects on predators are often reported in literature, highlighting the difficulty in understanding the real contribution of winter cover crops to natural enemies and their pest control potential (Lundgren and Fergen, 2010; Fox et al., 2016). We were not able to detect any legacy effect in our study, with the cash crops not showing advantages from the previous cultivation of a winter cover crop in terms of activity density of predators and biocontrol potential at mid-season. The activity of natural enemies in the following crop can be influenced by how cover crops are terminated. It has been demonstrated that the termination strategy of cover crops may impact the activity of predators (Magagnoli et al., 2018), and less disruptive practices (e.g., by a roller-crimper) are preferable in order to retain arthropod predators to the field (Rivers et al., 2018). In our fields, cover crops were incorporated into the soil in late winter after termination. This practice is likely disturbing those species of predatory arthropods overwintering within the fields (Pfiffner and Luka, 2000). In addition, it is not creating the most favorable spring habitat, in respect of microclimate, shelter, and food, for an early colonization of the fields by beneficial arthropods from the surrounding environment (Wissinger, 1997; Wamser et al., 2011). Mechanical operations in arable crops can cause mortality and emigration of epigeal predators (Thorbek and Bilde, 2004), and in particular carabids can be both directly and indirectly negatively affected by tillage across all life stages (Blubaugh and Kaplan, 2015). Furthermore, the use of pesticides is known to have consistent negative effects on natural enemies reducing the potential of pest control (Geiger et al., 2010). Additional analyses of the field management practices in our study highlighted that tillage had an influence on carabids regardless of crop rotation schemes, decreasing their activity density in fields that were plowed during the growing season. Thus, a high level of anthropogenic disturbance through conventional soil management and widespread application of pesticides in the investigated fields may have had more prominent effects on generalist predators compared to crop rotation management, precluding the detection of any effect caused by the implemented diversification strategies. Investigating the effects of field management practices in interaction with crop rotation was not possible in this study, due to the limited feedback by farmers on their respective field management. Therefore, further research is needed in order to evaluate the combined effects caused by field and crop rotation management.
Surprisingly, we did not find any differences in the proxies of biocontrol investigated between maize and grain cereal fields. Also, arthropods predation and attack rates and activity density were not affected by the plants' growth stage either. On the contrary, we found that total attack rates by both arthropods and vertebrates on dummy caterpillars were influenced by current crop and growth stage. This difference was due to the contribution to predation by mammals, showing that these organisms may also provide pest control service in agricultural landscapes, as shown by Tschumi et al. (2018).
To conclude, we observed in our study less positive effects of temporal diversification than we expected. Our results suggest that crop temporal diversification alone may have not been enough to enhance the potential of natural pest control under the conditions studied here at a specific period during the growing season. In fact, it is important to consider that our study did not account for temporal dynamics and the results have been obtained once at mid-season, in a year characterized by particularly dry and hot climate conditions, which may have influenced the presence and activity of the organisms investigated. As climate change and extreme weather events such as drought affect trophic interactions in food webs (Diehl et al., 2013), this aspect should not be omitted when interpreting the results. Nevertheless, adding different resources and traits to the agroecosystems through a wider range of cultivated crops and the integration of semi-natural habitats and perennial crops are aspects that should be considered when developing more resilient and diverse cropping systems. Finally, we were not able to demonstrate benefits of winter cover crops for biocontrol, however, this does not necessarily imply that their introduction in the rotation does not provide several beneficial services to agroecosystems. Therefore, in order to better exploit the potential benefits of winter cover crop for predators and their biocontrol services it is necessary to consider and investigate the effects of all the specific management practices related with their cultivation.
The raw data supporting the conclusions of this article will be made available by the authors, without undue reservation.
JD, HA, and GP contributed to conception and design of the study. GP collected the field data and wrote the original draft of the manuscript. GP and JT analyzed the data. All authors contributed to the manuscript and approved the submitted version.
This study was carried out within the framework of the EU project DiverIMPACTS, funded by the European Union's Horizon 2020 research and innovation programme under grant agreement No. 727482.
The authors declare that the research was conducted in the absence of any commercial or financial relationships that could be construed as a potential conflict of interest.
All claims expressed in this article are solely those of the authors and do not necessarily represent those of their affiliated organizations, or those of the publisher, the editors and the reviewers. Any product that may be evaluated in this article, or claim that may be made by its manufacturer, is not guaranteed or endorsed by the publisher.
We are grateful to farmers for research permission. We thank Katja Steininger for assistance in preparing and processing the samples, and Sonja Wichmann and Niklas Fribel for helping during the fieldwork. We also thank Stefan Mecke for support with GIS analysis and Katrin Ronnenberg for valuable comments on the statistical models.
The Supplementary Material for this article can be found online at: https://www.frontiersin.org/articles/10.3389/fagro.2021.704979/full#supplementary-material
Aguilera, G., Roslin, T., Miller, K., Tamburini, G., Birkhofer, K., Caballero-Lopez, B., et al. (2020). Crop diversity benefits carabid and pollinator communities in landscapes with semi-natural habitats. J. Appl. Ecol. 57, 2170–2179. doi: 10.1111/1365-2664.13712
Altieri, M. A. (1999). The ecological role of biodiversity in agroecosystems. Agric. Ecosyst. Environ. 74, 19–31. doi: 10.1016/S0167-8809(99)00028-6
Altieri, M. A., and Nicholls, C. I. (2004). Biodiversity and Pest Management in Agroecosystems. Binghamton, NY: Food Products Press. doi: 10.1201/9781482277937
Bengtsson, J. (2015). Biological control as an ecosystem service: partitioning contributions of nature and human inputs to yield. Ecol. Entomol. 40, 45–55. doi: 10.1111/een.12247
Birkhofer, K., Andersson, G. K. S., Bengtsson, J., Bommarco, R., Dänhardt, J., Ekbom, B., et al. (2018). Relationships between multiple biodiversity components and ecosystem services along a landscape complexity gradient. Biol. Conserv. 218, 247–253. doi: 10.1016/j.biocon.2017.12.027
Blubaugh, C. K., and Kaplan, J. (2015). Tillage compromises weed seed predator activity across developmental stages. Biol. Control. 81, 76–82. doi: 10.1016/j.biocontrol.2014.11.007
Bosem Baillod, A., Tscharntke, T., Clough, Y., and Batáry, P. (2017). Landscape-scale interactions of spatial and temporal cropland heterogeneity drive biological control of cereal aphids. J. Appl. Ecol. 54, 1804–1813. doi: 10.1111/1365-2664.12910
Brooks, M. E., Kristensen, K., van Benthem, K. J., Magnusson, A., Berg, C. W., Nielsen, A., et al. (2017). glmmTMB balances speed and flexibility among packages for zero-inflated Generalized Linear Mixed modeling. R J. 9, 378–400. doi: 10.32614/RJ-2017-066
Brust, G. E., and King, L. R. (1994). Effects of crop rotation and reduced chemical inputs on pests and predators in maize agroecosystems. Agric. Ecosyst. Environ. 48, 77–89. doi: 10.1016/0167-8809(94)90077-9
Chaplin-Kramer, R., O'Rourke, M. E., Blitzer, E. J., and Kremen, C. (2011). A meta-analysis of crop pest and natural enemy response to landscape complexity. Ecol. Lett. 14, 922–932. doi: 10.1111/j.1461-0248.2011.01642.x
Deutscher Wetterdienst (2020). Climate Data Center CDC. Available online at: https://cdc.dwd.de/portal/ (accessed January 28, 2020).
Diehl, E., Sereda, S., Wolters, V., and Birkhofer, K. (2013). Effects of predator specialization, host plant and climate on biological control of aphids by natural enemies: a meta-analysis. J. Appl. Ecol. 50, 262–270. doi: 10.1111/1365-2664.12032
Dunbar, M. W., Gassmann, A. J., and O'Neal, M. E. (2016). Impacts of rotation schemes on ground-dwelling beneficial arthropods. Environ. Entomol. 45, 1154–1160. doi: 10.1093/ee/nvw104
Ekschmitt, K., Wolters, V., and Weber, M. (1997). “Spiders, carabids, and staphylinids: the ecological potential of predatory macroarthropods,” in Fauna in Soil Ecosystems, eds G. Benckiser (New York, NY: Marcel Dekker), 307–362.
European Commission (2013). Regulation (EU) No 1307/2013 of the European Parliament and of the Council of 17 December 2013 establishing rules for direct payments to farmers under support schemes within the framework of the common agricultural policy and repealing Council Regulation (EC) No 637/2008 and Council Regulation (EC) No 73/2009. Official J. Eur. Union L. 347, 608–670.
Fahrig, L. (2013). Rethinking patch size and isolation effects: the habitat amount hypothesis. J. Biogeogr. 40, 1649–1663. doi: 10.1111/jbi.12130
Fox, A. F., Kim, T. N., Bahlai, C. A., Woltz, J. M., Gratton, C., and Landis, D. A. (2016). Cover crops have neutral effects on predator communities and biological control services in annual cellulosic bioenergy cropping systems. Agric. Ecosyst. Environ. 232, 101–109. doi: 10.1016/j.agee.2016.07.003
Fox, J., and Weisberg, S. (2011). An {R} Companion to Applied Regression, 2nd Edn. Thousand Oaks CA: Sage. Available online at: http://socserv.socsci.mcmaster.ca/jfox/Books/Companion.
Geiger, F., Bengtsson, J., Berendse, F., Weisser, W. W., Emmerson, M., Morales, M. B., et al. (2010). Persistent negative effects of pesticides on biodiversity and biological control potential on European farmland. Basic Appl. Ecol. 11, 97–105. doi: 10.1016/j.baae.2009.12.001
Hertzog, L. R., Ebeling, A., Weisser, W. W., and Meyer, S. T. (2017). Plant diversity increases predation by ground-dwelling invertebrate predators. Ecosphere 8:1–14. doi: 10.1002/ecs2.1990
Holland, J. M., and Luff, M. L. (2000). The effects of agricultural practices on carabidae in temperate agroecosystems. Integrated Pest Manag. Rev. 5, 109–129. doi: 10.1023/A:1009619309424
Honek, A., Martinkova, Z., and Jerosik, V. (2003). Ground beetles (Carabidae) as seed predators. Eur. J. Entomol. 100, 531–544. doi: 10.14411/eje.2003.081
Howe, A., Lövei, G. L., and Nachman, G. (2009). Dummy caterpillars as a simple method to assess predation rates on invertebrates in a tropical agroecosystem. Entomol. Exp. Appl. 131, 325–329. doi: 10.1111/j.1570-7458.2009.00860.x
Karp, D. S., Chaplin-Kramer, R., Meehan, T. D., Martin, E. A., DeClerck, F., Grab, H., et al. (2018). Crop pests and predators exhibit inconsistent responses to surrounding landscape composition. Proc. Natl. Acad. Sci. U.S.A. 115, 7863–7870. doi: 10.1073/pnas.1800042115
Kremen, C., and Chaplin-Kramer, R. (2007). “Insects as providers of ecosystem services: crop pollination and pest control. Insect Conservation Biology,” in Proceeding of the Royal Entomological Society's 23nd Symposium, eds A. J. A. Stewart, T. R. New and O. T Lewis (Wallingford: CABI), 349–382. doi: 10.1079/9781845932541.0349
Kremen, C., and Miles, A. (2012). Ecosystem services in biologically diversified versus conventional farming systems: benefits, externalities, and trade-offs. Ecol. Soc. 17:40. doi: 10.5751/ES-05035-170440
Lenth, R. (2018). emmeans: Estimated Marginal Means, aka Least-Squares Means. R package version 1.3.0. Available online at: https://CRAN.R-project.org/package=emmeans.
Letourneau, D. K., Armbrecht, I., Rivera, B. S., Lerma, J. M., Carmona, E. J., Daza, M. C., et al. (2011). Does plant diversity benefit agroecosystems? A synthetic review. Ecol. Appl. 21, 9–21. doi: 10.1890/09-2026.1
Letourneau, D. K., Jedlicka, J. A., Bothwell, S. G., and Moreno, C. R. (2009). Effects of natural enemy biodiversity on the suppression of arthropod herbivores in terrestrial ecosystems. Annu. Rev. Ecol. Evol. Syst. 40, 573–592. doi: 10.1146/annurev.ecolsys.110308.120320
Lichtenberg, E. M., Kennedy, C. M., Kremen, C., Batáry, P., Berendse, F., Bommarco, R., et al. (2017). A global synthesis of the effects of diversified farming systems on arthropod diversity within fields and across agricultural landscapes. Glob. Change Biol. 23, 4946–4957. doi: 10.1111/gcb.13714
Lövei, G. L., and Ferrante, F. (2017). A review of the sentinel prey method as a way of quantifying invertebrate predation under field conditions. Insect Sci. 24, 528–542. doi: 10.1111/1744-7917.12405
Low, P. A., Sam, K., McArthur, C., Posa, M. R. C., and Hochuli, D. F. (2014). Determining predator identity from attack marks left in model caterpillars: guidelines for best practice. Entomol. Exp. Appl. 152, 120–126. doi: 10.1111/eea.12207
Lundgren, J. G., and Fergen, J. K. (2010). The effects of a winter cover crop on Diabrotica virgifera (Coleoptera: Chrysomelidae) populations and beneficial arthropod communities in no-till maize. Environ. Entomol. 39, 1816–1828. doi: 10.1603/EN10041
Magagnoli, S., Masetti, A., Depalo, L., Sommaggio, D., Campanelli, G., Leteo, et al. (2018). Cover crop termination techniques affect ground predation within an organic vegetable rotation system: a test with artificial caterpillars. Biol. Control. 117, 109–114. doi: 10.1016/j.biocontrol.2017.10.013
Martin, E. A., Dainese, M., Clough, Y., Báldi, A., Bommarco, R., Gagic, V., et al. (2019). The interplay of landscape composition and configuration: new pathways to manage functional biodiversity and agroecosystem services across Europe. Ecol. Lett. 22, 1083–1094. doi: 10.1111/ele.13265
Meyer, M., Ott, D., Götze, P., Koch, H., and Scherber, C. (2019). Crop identity and memory effects on aboveground arthropods in a long-term crop rotation experiment. Ecol. Evol. 9, 7307–7323. doi: 10.1002/ece3.5302
Meyer, S. T., Koch, C., and Weisser, W. W. (2015). Towards a standardized Rapid Ecosystem Function Assessment (REFA). Trends Ecol. Evol. 30, 390–397. doi: 10.1016/j.tree.2015.04.006
Navntoft, S., Wratten, S. D., Kristensen, K., and Esbjerg, P. (2009). Weed seed predation in organic and conventional fields. Biol. Control. 49, 11–16. doi: 10.1016/j.biocontrol.2008.12.003
Pfiffner, L., and Luka, H. (2000). Overwintering of arthropods in soils of arable fields and adjacent semi-natural habitats. Agric. Ecosyst. Environ. 78, 215–222. doi: 10.1016/S.0167-8809(99)00130-9
R Core Team (2018). R: A Language and Environment for Statistical Computing. R Foundation for Statistical Computing, Vienna, Austria. Available online at: https://www.R-project.org/.
Rivers, A. N., Mullen, C. A., and Barbercheck, M. E. (2018). Cover crop species and management influence predatory arthropods and predation in an organically managed, reduced-tillage cropping system. Environ. Entomol. 47, 340–355. doi: 10.1093/ee/nvx149
Rusch, A., Bommarco, R., Chiverton, P., Öberg, S., Wallin, H., Wiktelius, S., et al. (2013a). Response of ground beetle (Coleoptera, Carabidae) communities to changes in agricultural policies in Sweden over two decades. Agric. Ecosyst. Environ. 176, 63–69. doi: 10.1016/j.agee.2013.05.014
Rusch, A., Bommarco, R., Jonsson, M., and Smith, H. G. (2013b). Flow and stability of natural pest control services depend on complexity and crop rotation at the landscape scale. J. Appl. Ecol. 50, 345–354. doi: 10.1111/1365-2664.12055
Saska, P., Koprdov,á, S., Martinková, Z., and Honěk, A. (2014). Comparing methods of weed seed exposure to predators: comparing seed exposure methods. Ann. Appl. Biol. 164, 301–312. doi: 10.1111/aab.12102
Schipanski, M. E., Barbercheck, M., Douglas, M. R., Finney, D. M., Haider, K., Kaye, J. P., et al. (2014). A framework for evaluating ecosystem services provided by cover crops in agroecosystems. Agric. Syst. 125, 12–22. doi: 10.1016/j.agsy.2013.11.004
Schwan, H., Sorg, M., and Stenmans, W. (1993). Naturkundliche Untersuchungen zum Naturschutzgebiet “Die Spey” (Stadt Krefeld, Kreis Neuss) - I. Untersuchungsstandorte und Methoden - Natur am Niederrhein (N.F.) 8:1–13, 4 Abb., 2 Tab., 2 Taf.: Krefeld.
Sirami, C., Gross, N., Baillod, A. B., Bertrand, C., Carri,é, R., Hass, A., et al. (2019). Increasing crop heterogeneity enhances multitrophic diversity across agricultural regions. Proc. Natl. Acad. Sci. U.S.A. 116, 16442–16447. doi: 10.1073/pnas.1906419116
Stupak, N. Sanders, J., and Heinrich, B. (2019). The role of farmers' understanding of nature in shaping their uptake of nature protection measures. Ecol. Econ. 116, 16442–16447. doi: 10.1016/j.ecolecon.2018.11.022
Sunderland, K., and Samu, F. (2000). Effects of agricultural diversification on the abundance, distribution, and pest control potential of spiders: a review. Entomol. Exp. Appl. 95, 1–13. doi: 10.1046/j.1570-7458.2000.00635.x
Teichmann, B. (1994). Eine wenig bekannte Konservierungsflüssigkeit für Bodenfallen. Ento-mologische Nachrichten Berichte 38, 1994/1991.
Thorbek, P., and Bilde, T. (2004). Reduced numbers of generalist arthropod predators after crop management. J. Appl. Ecol. 41, 526–538. doi: 10.1111/j.0021-8901.2004.00913.x
Tscharntke, T., Karp, D. S., Chaplin-Kramer, R., Batáry, P., DeClerck, F., Gratton, C., et al. (2016). When natural habitat fails to enhance biological pest control – Five hypotheses. Biol. Conserv. 204, 449–458. doi: 10.1016/j.biocon.2016.10.001
Tschumi, M., Ekroos, J., Hjort, C., Smith, H. G., and Birkhofer, K. (2018). Rodents, not birds, dominate predation-related ecosystem services and disservices in vertebrate communities of agricultural landscapes. Oecologia 188, 863–873. doi: 10.1007/s00442-018-4242-z
Wamser, S., Dauber, J., Birkhofer, K., and Wolters, V. (2011). Delayed colonisation of arable fields by spring breeding ground beetles (Coleoptera: Carabidae) in landscapes with a high availability of hibernation sites. Agr. Ecosyst. Environ. 144, 235–240. doi: 10.1016/j.agee.2011.08.019
Westerman, P. R., Hofman, A., and Vet, L. E. M. (2003). Relative importance of vertebrates and invertebrates in epigeaic weed seed predation in organic cereal fields. Agric. Ecosyst. Environ. 95, 417–425. doi: 10.1016/S0167-8809(02)00224-4
Wissinger, S. A. (1997). Cyclic colonization in predictably ephemeral habitats: a template for biological control in annual crop systems. Biol. Control. 10, 4–15. doi: 10.1006/bcon.1997.0543
Keywords: biocontrol potential, crop diversification, dummy caterpillars, generalist predators, Rapid Ecosystem Function Assessment (REFA)
Citation: Puliga GA, Thiele J, Ahnemann H and Dauber J (2021) Effects of Temporal Crop Diversification of a Cereal-Based Cropping System on Generalist Predators and Their Biocontrol Potential. Front. Agron. 3:704979. doi: 10.3389/fagro.2021.704979
Received: 04 May 2021; Accepted: 21 September 2021;
Published: 15 October 2021.
Edited by:
Lionel Alletto, Institut National de recherche pour l'agriculture, l'alimentation et l'environnement (INRAE), FranceReviewed by:
Megan Woltz, Lindenwood University, United StatesCopyright © 2021 Puliga, Thiele, Ahnemann and Dauber. This is an open-access article distributed under the terms of the Creative Commons Attribution License (CC BY). The use, distribution or reproduction in other forums is permitted, provided the original author(s) and the copyright owner(s) are credited and that the original publication in this journal is cited, in accordance with accepted academic practice. No use, distribution or reproduction is permitted which does not comply with these terms.
*Correspondence: Giovanni Antonio Puliga, Z2lvdmFubmkuYS5wdWxpZ2FAZ21haWwuY29t
Disclaimer: All claims expressed in this article are solely those of the authors and do not necessarily represent those of their affiliated organizations, or those of the publisher, the editors and the reviewers. Any product that may be evaluated in this article or claim that may be made by its manufacturer is not guaranteed or endorsed by the publisher.
Research integrity at Frontiers
Learn more about the work of our research integrity team to safeguard the quality of each article we publish.