- 1Department of Biology, The University of Western Ontario, London, ON, Canada
- 2Agri-Neo, Toronto, ON, Canada
The two-spotted spider mite, Tetranychus urticae, is a polyphagous agricultural pest that feeds on over a thousand plant species and more than 150 crops. Its status as an agricultural pest is exacerbated by its outstanding potential to evolve resistance to pesticides. The existence of multiresistant mite populations challenges mite management and creates a need for the development of pesticides with novel modes of action. In this study, we evaluated the acaricidal activity of Neo-Boost, a pesticide registered as a bactericide/fungicide/insecticide/miticide. Neo-Boost formulation contains three active ingredients: potassium silicate, sodium percarbonate and tetraacetylethylenediamine. In contact with water, the latter ingredients generate peroxyacetic acid, a potent contact bactericide and fungicide. Using the recommended field dose, we tested contact and residual effects of Neo-Boost on T. urticae egg, larval, and adult life stages. We found that Neo-Boost had no ovicidal effect. When tested on larvae, Neo-Boost caused 87% mortality over 48 hours post-treatment that was comparable to the positive control AGRI-mek SC (active ingredient abamectin), in addition to delaying larval developmental progression. On adult mites, Neo-Boost acted as a deterrent and caused 35% mortality (an intermediate effect compared to the positive control AGRI-mek SC). We also tested the proposed ability of Neo-Boost to induce a systemic response, however, it had no priming effect on treated tomato plants against mites. Of the individual Neo-Boost components tested, peroxyacetic acid and potassium silicate were not effective in any assay. However, sodium percarbonate, surfactant, and other non-listed components were sufficient for the full effectiveness of Neo-Boost formulations. Additionally, phytotoxicity of Neo-Boost was rare and minor when it occurred. Overall, despite the inefficiency of some compounds, the Neo-Boost formulation, either in its original or simplified formulation, may be useful for mite control on crops that are destined for fresh human consumption or medicinal use.
Introduction
Pesticides continue to be a major tool used to secure food and the production of plant-based materials. However, this approach to pest control is only effective in the absence of pesticide resistance in agricultural pests. The problems associated with pesticide resistance, such as pest resurgence and subsequent loss of crop production potential, are exemplified in the case of the two-spotted spider mite T. urticae Koch. Characterized as an extreme generalist, T. urticae feeds on over a thousand plant species, including over 150 field and greenhouse crops (Migeon and Dorkeld, 2021). Mite host range includes many staple fruit and vegetable crops in addition to corn, soybean, and cotton. The genetic and physiological characteristics of T. urticae prime it for the rapid evolution of resistance to pesticides. In addition to a short life cycle and high reproductive output, the T. urticae genome has expanded gene families encoding detoxification enzymes that are hypothesized to increase mite detoxification potential (Grbić et al., 2011; Dermauw et al., 2013). Observations so far suggest that T. urticae develops pesticide resistance within 2 to 4 years after the introduction of new acaricides (Cranham and Helle, 1985). As of April 2021, T. urticae is the most resistant arthropod pest with resistance recorded against 96 different active compounds and 554 reported cases of resistance worldwide (Mota-Sanchez and Wise, 2021). Mite's ability to develop resistance and cross-resistance to different pesticide active ingredients is a major challenge to crop protection. Due to a decreasing number of pesticides effective in controlling T. urticae, combined with climate change that favors the expansion of mite populations (Ximénez-Embún et al., 2017), there is an urgent need to develop new pesticides to control T. urticae.
Pesticide resistance can result from several mechanisms but ultimately involves changes that reduce pesticide binding to the target in the pest organism. This can include changes to the structure of the target binding site or any other chemical or physiological alteration in penetrance, detoxification, and transport/excretion of the effective chemical within the arthropod pest (Insecticide Resistance Action Committee, 2020). Changes to target-sites can lead to cross-resistance due to the change in the configuration of a target protein that may reduce the binding of other pesticides with the same mode of action. Additionally, cross-resistance stemming from changes in mite metabolism can result in the ability to detoxify unrelated pesticides. These phenomena have been well-documented in T. urticae (Cranham and Helle, 1985; Osakabe et al., 2009). The issues of pesticide resistance and cross-resistance necessitate development of novel control agents with different and varied modes of action. The need for additional pesticides with new modes of action parallels the demand for more environmentally friendly methods of pest control in agriculture. For example, new technologies such as RNA interference (RNAi) are currently being developed for T. urticae (Suzuki et al., 2017b; Bensoussan et al., 2020) and are under development for several plant pests, soon to be incorporated into Integrated Pest Management (IPM) strategies for some pests (Head et al., 2017; Zotti et al., 2018; Fletcher et al., 2020).
Neo-Boost (EPA Reg. Number: 88306-4, Agri-Neo Inc., Toronto, ON, Canada) is a novel product registered as a bactericide/fungicide/insecticide/miticide for the control of bacterial/fungal pathogens, chewing/sucking insects and mites. Its proposed mode of action is based on the oxidizing activity of peroxyacetic acid (PAA) which is a potent contact bactericide and fungicide. Upon addition of water to powdered Neo-Boost, the tetraacetylethylenediamine (TAED) and sodium percarbonate produce PAA in solution. Both PAA and sodium percarbonate are reactive oxidants that further break down to form hydrogen peroxide (Sode, 2019). As the hydrogen peroxide is synthesized by plants upon herbivory, including that of T. urticae (Santamaría et al., 2018), there is a possibility that it may be effective against mites. Moreover, Neo-Boost contains potassium silicate (KSi) that has been shown to enhance plant resistance to both abiotic and biotic stresses (Ma, 2004; Ma and Yamaji, 2006). KSi can act as a mechanical barrier and anti-digestive compound (Reynolds et al., 2009). It was also implicated in priming jasmonic acid-plant induced defense responses (Sakr, 2017). As jasmonic acid is a general inducer of plant defenses against herbivores (Pieterse et al., 2012) including spider mites (Li et al., 2002; Zhurov et al., 2014; Salehipourshirazi et al., 2021; Widemann et al., 2021), Si in the Neo-Boost formulation may contribute to plant resistance against a wide range of herbivores.
In this study, we assessed the contact, residual, and combined effects of Neo-Boost at the recommended field dose on different developmental stages of T. urticae. In addition, we examined the ability of Neo-Boost to enhance plant defenses against mite herbivory through prior treatments, using tomato as a representative greenhouse crop.
Materials and Methods
Plant and Mite Rearing and Bioassay Methods
Bean, Phaseolus vulgaris cv. California Red Kidney (Stokes Seeds Ltd., Thorold, ON, Canada), and tomato, Solanum licopersicum cv. Moneymaker (Halifax Seed Company Inc., Halifax, NS, Canada) plants were grown under 100 to 150 μmol m−2 sec−1 cool-white fluorescent light at 24°C with a 16:8 h (light:dark) photoperiod in controlled growth chambers. Detached bean leaves (Figure 1A), bean leaf disks (1.5 cm in diameter, Figure 1B), and 3rd and 4th emerged, terminally adjacent leaflets on 4-week-old tomato plants (Figure 1C) were used in experiments. The London reference strain (Grbić et al., 2011) of T. urticae was used in most experiments. An additional T. urticae strain, collected from a tomato-producing commercial greenhouse in Leamington ON, Canada (referred to as the “tomato mite” strain), was used for additional testing of the acaricidal effect of Neo-Boost on adult mites. Mite strains were reared on bean plants in growth chambers at 24°C, 60% relative humidity, and with a 16:8 h (light:dark) photoperiod.

Figure 1. Experimental set-ups. (A) The detached bean leaf experimental set-up. (B) The bean leaf disk experimental set-up. (C) The experimental set-up for priming experiment on tomato. (D) A PAA test-strip showing proper PAA activity.
Solution Preparation
We tested the commercially recommended concentration of 1% w/v (10 g/L) of Neo-Boost that results in the formation of 5% of peroxyacetic acid (PAA) in prepared solution. To verify PAA production, the Neo-Boost solution was tested using activity indicators, Peroxyacetic Acid Test Strips (LaMotte Company, Chestertown, MD, USA), that change color depending on the concentration of PAA in ppm (Figure 1D). We used water and the surfactant BIO-TERGE® AS-90 (Stepan Company, Northfield, IL, USA) as control treatments. The surfactant was tested at 0.04% w/v (0.4 g/L), as found in the complete Neo-Boost formulation. Neo-Boost, surfactant, and other components of the complete formulation were provided by Agri-Neo Inc. as powders and were dissolved in distilled water. Positive controls included TetraSan 5 WDG (active ingredient etoxazole; EPA Reg. Number: 59639-108, Valent Canada, Inc., Guelph, ON, Canada) and AGRI-mek SC (active ingredient abamectin; EPA Reg. Number: 100-1351, Syngenta Canada Inc., Guelph, ON, Canada). TetraSan 5 WDG was used on eggs at a concentration of 600 mg/L (etoxazole concentration of 30 mg/L). AGRI-mek SC was used on larval and adult stages at a concentration of 0.05 ml/L (abamectin concentration of 42 mg/L). Both concentrations of positive controls are within the lower range of the recommended field dose range for use against T. urticae as specified by the supplier.
Spray Treatments
Spray treatments used to test contact, residual, combined contact and residual, and priming effects of Neo-Boost are listed in Table 1. Spray treatments were carried out using a multi-purpose hand sprayer until runoff. Each sample was sprayed with an approximate total volume of 32 μl/cm2, resulting in uniform wetting of leaf material with some droplets forming on the leaf and some run-off.
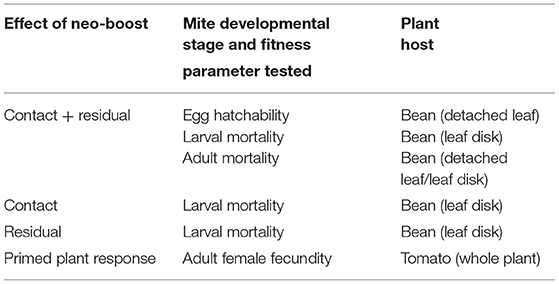
Table 1. Spray regimes for testing combined contact and residual, contact, residual, and priming effects of neo-boost.
Testing the Effectiveness of Topical Neo-Boost Application on Different T. urticae Developmental Stages
Egg Viability Assay
Thirty adult female London mites were placed on detached bean leaves and allowed to lay eggs for 2 hours before removal. The number of deposited eggs was counted (with an average of 45 eggs deposited per leaf, each leaf representing a sample). The bean leaves were then sprayed with water (mock control), surfactant, or Neo-Boost. 5 days following oviposition and treatment, the number of unhatched eggs was counted. The number of hatched eggs was used to calculate the proportion of successfully emerged mites out of the number of deposited eggs in each leaf sample. Ten detached leaves were used per treatment per trial (~450 eggs/treatment/trial). Three independent trials were performed. The same set-up was used in testing etoxazole on eggs.
Larval Mortality Assay
Five newly emerged larvae, placed on a bean leaf disk with a bristle from a fine brush, were sprayed with water, surfactant, or Neo-Boost. The number of live larvae/nymphs was scored at 6, 24, and 48 hours post-spraying. Twenty leaf disks were prepared per treatment per trial and the experiment was performed in three independent trials (100 mites/treatment/trial). The proportion of larvae/nymphs that died was calculated by subtracting the alive mites from the original number of mites placed on each sample. The same set-up was used in testing abamectin on larvae.
Adult Mortality Assay
The adult mortality assay was performed on detached bean leaves when assaying the London mite strain. Adult mites for all experiments were age-synchronized as described in Suzuki et al. (2017a). Thirty adult female mites, placed on a detached bean leaf with a fine brush, were sprayed with water, surfactant, or Neo-Boost. The number of live mites was determined after 6, 24, and 48 hours post-treatment. Ten detached leaves were used per treatment (total of 300 mites/treatment/trial) and the experiment was performed in three independent trials.
An additional assay of adult mortality was performed using a “tomato mite” strain, to test the effects of Neo-Boost treatment across different mite populations. The adult mortality assay of “tomato mites” was performed on bean leaf disks. Five, synchronized adult female mites were placed on each leaf disk and the mortality was determined 48 hours post-treatment. Twenty bean leaf disks were used per treatment with water, surfactant, or Neo-Boost per trial (100 mites/treatment/trial) in three independent trials. The leaf disk assay set-up was used in the positive control testing abamectin against adults.
Testing Neo-Boost Components for Acaricidal Activity
Tetraacetylethylenediamine and sodium percarbonate that generate the peroxyacetic acid (PAA) in water solution, and potassium silicate are listed as active ingredients in Neo-Boost formulation. To test their acaricidal properties, we prepared formulations lacking individual active ingredients. We tested the requirement of PAA by preparing a spray treatment that included all components except TAED (“no TAED”), Table 2. In this treatment, some hydrogen peroxide is expected to be generated from sodium percarbonate. In addition, spray treatments that contained all components except KSi (“no KSi”) or those containing only KSi (“only KSi”) were used to test the acaricidal activity of potassium silicate, Table 2. Individual components of the Neo-Boost formulation were topically applied to larvae and adults, and were tested for their effectiveness against mites. Twenty leaf disks, each infested with five newly emerged larvae, were sprayed with experimental solutions and larval mortality and the developmental progression of the survivors were determined 48 hours post-spraying. The time frame of the experiment allowed for the development of larvae to nymph stages. In addition, five adult mites were placed on each of 20 leaf disks, and were sprayed with experimental solutions. Adult mortality and mite dispersal were recorded 48 hours post-spraying. Adult mites on the edge of the leaf disk entering the wet filter paper were considered as escaping the leaf and were used as a measure of deterrence. Experiments were performed in three independent trials (100 mites/treatment/trial).
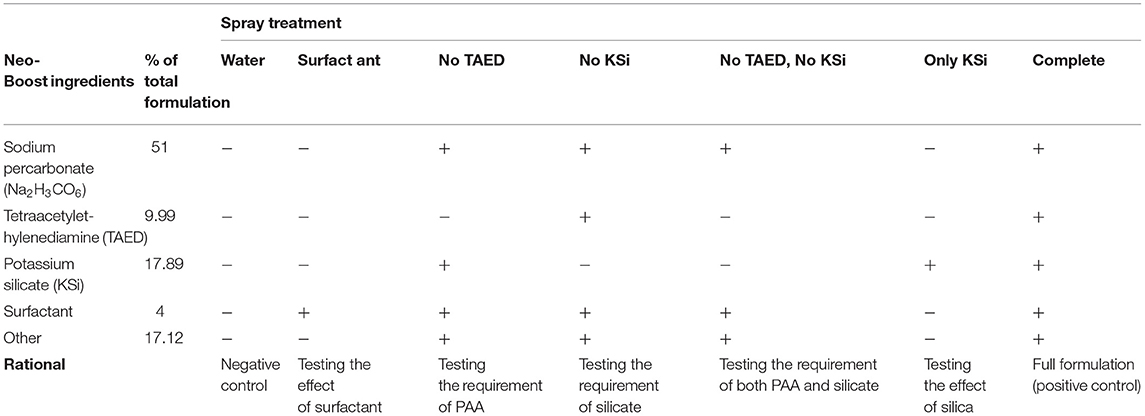
Table 2. Spray treatments containing different combinations of ingredients in the Neo-Boost formulation.
Testing the Residual and the Contact Effectiveness of Neo-Boost on Larvae
The bioassays described under 2.4 and 2.5 were performed by spraying treatments that simultaneously exposed mites and leaf tissues to experimental solutions. To uncouple residual and contact effects of Neo-Boost, we pre-treated bean leaf disks with Neo-Boost prior to mite application for determination of Neo-Boost's residual effects. In parallel, we sprayed mites directly–to determine Neo-Boost's contact effects. The residual effect of Neo-Boost against mites was assessed similarly to Golec et al. (2020). Twenty bean leaf disks were sprayed with water control and Neo-Boost formulations listed in Table 2 and allowed to dry under a fume hood (~1 hour) before they were inoculated with five newly emerged larvae. After 48 hours, the number and developmental stage of live mites were assessed. This experiment was performed in three independent trials (100 mites/treatment/trial). To determine the contact effect of Neo-Boost formulations on mites, 100–120 newly emerged larvae were placed on a Petri dish and were sprayed directly with Neo-Boost formulations listed in Table 2 as described above. The number of living and dead mites was assessed 1 hour post-spraying. The experiment was performed in one trial; therefore, each mite is considered a sample in this experiment, with a binomial outcome of alive or dead (100 ≤ n ≤ 120/treatment).
Testing the Ability of Neo-Boost to Induce Systemic Defense Responses Against Mites
Potassium silicate has been implicated in the establishment of induced systemic defense responses (Sakr, 2017). To test the ability of Neo-Boost to prime plant defenses against spider mites, 4-week-old tomato plants were sprayed with water, surfactant, or Neo-Boost before the application of mites. Whole plants were sprayed until runoff 10 and 3 days before the application of ten female mites whose fecundity was assessed 3 days post-plant inoculation. Lanolin-enclosed arenas on the third or fourth emerged leaf were inoculated with 10 adult female mites (Figure 1C). The number of live mites was counted daily and the number of eggs was counted 3 days post-inoculation. Fecundity was normalized to eggs/mite, using the average number of mites in each sample over 3 days as the denominator. Six tomato plants were used per treatment per trial (60 mites/treatment/trial). Three independent trials were performed.
Statistical Analysis
All proportion data (hatched eggs, mortality, deterrence) were analyzed using generalized linear models (GLMs) to perform logistic regression using binomial or quasibinomial distributions as required by the (over)distribution of residuals. The fecundity data (assay on tomato) was analyzed using a general linear model. All experiments with a single endpoint for data collection were analyzed as fixed factor models where the response variable (proportions or counts) was weighted by the total number of individuals in each sample. A repeated measures analysis was used to analyze the results of time-course experiments using a generalized linear mixed model (GLMM). The time points of data collection were included as a fixed factor in these models. In some cases involving the analysis of developmental stages, there was complete separation detected in the model, and therefore a penalized maximum likelihood method was used in fitting those models.
In all experiments, fixed factors included treatment as the main biological variable of interest and trial as a blocking factor with the secondary purpose of testing reproducibility between independent experiments as suggested by Brady et al. (2015) through the inclusion of an interaction term. Post-hoc tests were performed to determine differences between treatment predicted proportions/marginal means extracted from the models and were corrected for multiple comparisons using a Tukey method. A probability of 0.05 was used to test the significance of each factor in the analyses of deviance/variance and for comparisons between proportions/means in post-hoc analyses. The predicted proportions and marginal means generated by the logistic regression and linear models respectively were plotted with confidence intervals (95%) to illustrate plausible values for the parameter (proportion/mean) for the population from which samples were taken.
Specific details about analyses, including statistical model formulae, analysis of deviance/variance tables, interaction plots, and software packages used can be found in the Supplementary Material. All analyses were performed using R (R Core Team, 2019).
Results
The Effect of Combined Contact and Residual Application of Neo-Boost on T. urticae Eggs, Larvae, and Adult Mites
The application of Neo-Boost to T. urticae eggs deposited on a bean leaf disk had a biologically negligible but statistically significant effect on the proportion of hatched eggs (96, 95, and 94% in water, surfactant, and Neo-Boost treatments respectively, Figure 2A; F = 5.72; df = 2; P = 0.00479). In contrast, the application of 600 mg/L TetraSan 5 WDG (active ingredient etoxazole), used as a positive control, inhibited hatching such that only 6% of eggs hatched, compared to 91% in the water negative control (Figure 2E; F = 1774.92; df = 1, P < 0.0001). The effectiveness of Neo-Boost on larvae was significant and very high (χ2 = 234.92; df = 2; P < 0.0001), with mean mortalities of 93 and 96% at 24-and 48-hours post-treatment respectively, with no significant difference between the two time points (Figure 2B). Most of this effect was observed already at 6 hours post-treatment (79% mortality), indicating the fast action of Neo-Boost. A portion of this effect can be attributed to the surfactant, which gradually reached 41% mortality by 48 hours compared to 9% larval mortality in the water control. The positive control AGRI-mek SC at a concentration of 0.05 ml/L (active ingredient abamectin) caused a larval mortality of 99% compared to 5% in the water control (Figure 2F; χ2 = 83.84; df = 1; P < 0.0001). Adult mites were less susceptible to Neo-Boost treatment compared to larvae, but the effect was significant (χ2 = 129.26; df = 2; P < 0.0001). Their mortality gradually increased to 41% at 48 hours post-Neo-Boost treatment (Figure 2C) and was significantly higher relative to 6 and 13% mortality when adults were sprayed with water and surfactant, respectively. AGRI-mek SC cased a mortality of 97% for London adult mites compared to 4% in the water control (Figure 2G; χ2 = 184.78; df = 1; P < 0.0001). Neo-Boost was also tested against a multi-resistant T. urticae strain collected from a tomato-producing greenhouse, “tomato mites.” When this strain was sprayed with water, surfactant, or Neo-Boost, a statistically significant effect of treatment at 4, 19, and 47% mortality 48 hours post-treatment was observed, respectively (Figure 2D; χ2 = 92.58; df = 2; P < 0.0001). Thus, our data indicate that Neo-Boost has the greatest effect on larvae, an intermediate effect on adults, and no practical effect on eggs. Additionally, the effect on adults was consistent across two independent T. urticae populations.
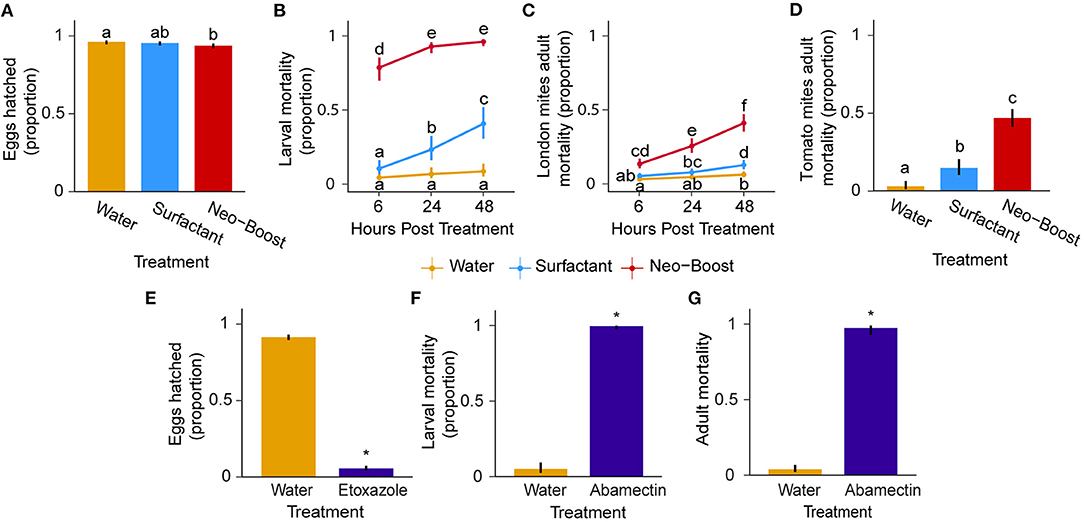
Figure 2. Combined contact and residual effects of Neo-Boost, surfactant and water on age-synchronized spider mites at 6, 24, and 48 hours post-spraying (5 days for hatchability). (A, E) Hatchability of London mite eggs (n = 30/treatment). (B, F) Mortality of larvae (n = 60/treatment). (C, D, G) Mortality of adult mites. London strain (C, G) and “tomato mite” strain (D), (n = 30/treatment each). Shown are proportions of hatched eggs/dead mites ± 95% confidence interval (CI). Means with different letters within graphs are significantly different by Tukey's HSD (P < 0.05). Asterisks represent differences between means (E, F, G).
Identification of the Effective Compound(s) in the Neo-Boost Formulation
As larvae were the most sensitive to Neo-Boost (Figure 2B), we first tested the effects of water, “no TAED,” “no KSi,” “only KSi,” or the complete formulation on larval mortality, Figures 3A,B. Statistical differences in larval mortality were found among treatments (F = 101.96; df = 4; P < 0.0001, Figure 3A; χ2 = 361.88; df = 3; P < 0.0001, Figure 3B). Low mortality was observed in water and “only KSi” treatments (9 and 11%, respectively) with no statistical differences between them. Surprisingly, the effectiveness of formulations that lacked PAA (“No TAED”), KSi (“No KSi”) or both active ingredients (“No TAED, No KSi”) on larval mortality were similar to the effects of the complete formulation (81, 78, and 86% larval mortality in “no TAED,” “no KSi,” and the complete formulation respectively, Figures 3A,B) indicating that neither PAA nor KSi are required for Neo-Boost's direct lethal effects on mites. A statistically significant increase in larval mortality was seen upon the application of surfactant (58%), indicating that surfactant is an important component that contributes to larval mortality in Neo-Boost formulation also seen in Figure 2B. Additional components included in the “other” category of components in Table 2 were sufficient to increase the effectiveness of the formulation to the level seen in the complete formulation, but were not tested individually in this study.
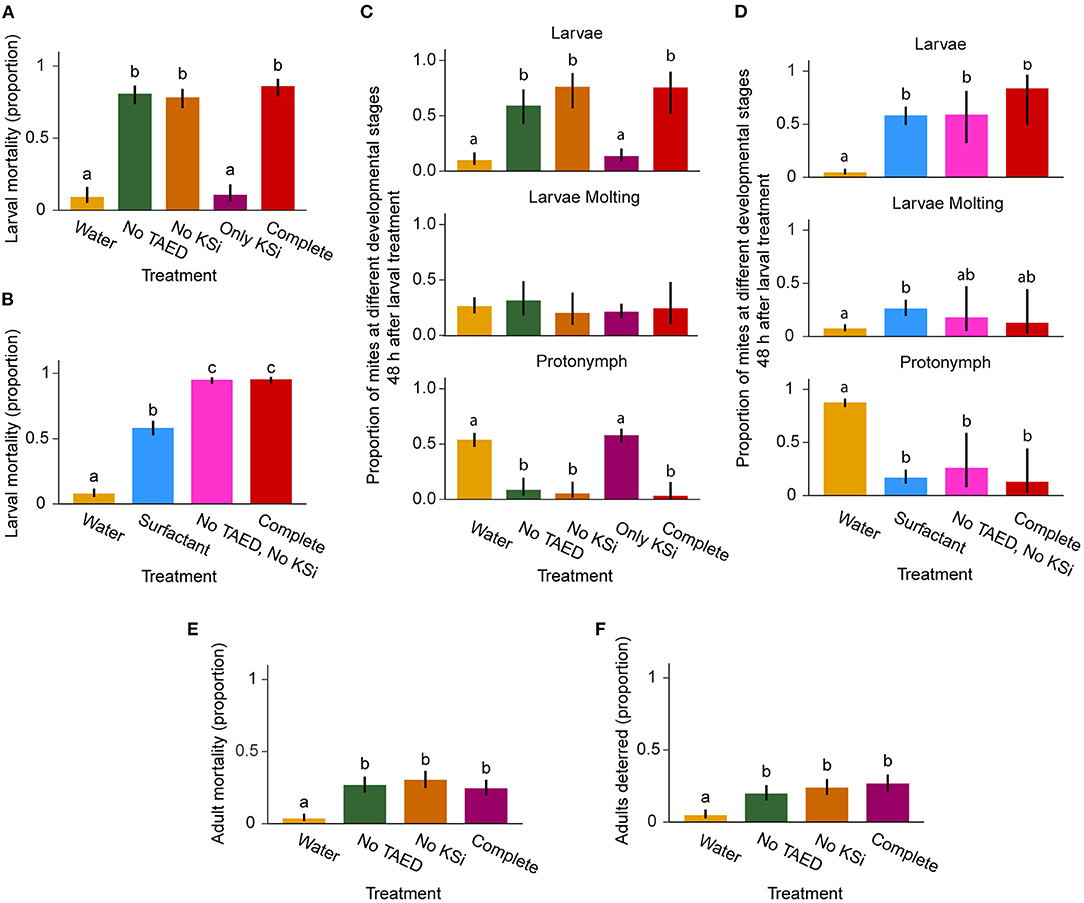
Figure 3. Effects of Neo-Boost components on larvae and adults at 48 hours post-treatment. (A, B) Larval mortality (n = 60/treatment). (C, D) Proportions of different mite developmental stages following larval treatment [n = 27–59/treatment, depending on number of samples with surviving mites from (A) and (B)]. (E) Adult mortality (n = 60/treatment). (F) Adult deterrence (n = 60/treatment). Shown are proportions of dead mites, developmental stage, and deterrence ± 95% confidence interval (CI). Letters within graphs represent differences between pairwise comparisons of means by Tukey's HSD (P < 0.05).
The effects of these formulations on larval development 48 hours post-treatment were also analyzed, Figures 3C,D. The proportion of mites that developed into protonymphs were significantly different between treatments (χ2 = 71.98; df = 4; P < 0.0001, Figure 3C; χ2 = 150.32; df = 3; P < 0.0001, Figure 3D) and followed the effectiveness trends observed for larval mortality. While 50–75% of larvae developed into protonymphs upon water treatment, larvae treated with complete formulation and formulations that lacked PAA (“No TAED”), KSi (“No KSi”) or both active ingredients (“No TAED, No KSi”), and to some extent with the surfactant, mostly remained at the larval stage. In these treatments, only 4–27% of larvae developed to the protonymph stage (Figures 3C,D).
Component testing was also performed on adults, where treatment had a significant effect (F = 26.98; df = 3; P < 0.0001). Mortality was lowest in the water treatment (3%) and increased in “no TAED,” “no KSi,” and complete treatments (27, 30, 24% respectively; Figure 3E). Characterization of a deterring effect was also recorded, where adults trapped at the edge of the leaf disk were considered attempting to escape. The analysis of deterrence revealed statistically significant effects of treatments (F = 16.95; df = 3; P < 0.0001). In the water treatment, 5% of mites were deterred, while deterrence in “no TAED,” “no KSi,” and complete treatments was 20, 24, and 27%, respectively (Figure 3F). In summary, neither PAA nor KSi contribute to Neo-Boost's effects on mites. Thus, Neo-Boost formulation can be simplified in terms of chemical complexity and still be able to preserve full effectiveness against larvae. Neo-Boost affected ~50% of adults that either die (Figure 3E) or try to escape treated leaves (Figure 3F).
Residual and Contact Effects of Neo-Boost on T. urticae
Residual effects of Neo-Boost formulations, tested by pre-treatment of leaves before mite application, revealed a significant effect of treatment (F = 184.45; df = 3; P < 0.0001). Residual effects of surfactant, “no TAED, no KSi,” and complete treatments led to larval mortality of 26, 70, and 87% respectively, and were all significantly different from the water treatment (6%; Figure 4A). The residual effects of these formulations on larval development 48 hours post-treatment showed that in water-treated mites 33 and 35% of mites progressed to molting or developed into protonymphs respectively, Figure 4B. In contrast, the application of surfactant, “no TAED, no KSi,” and complete formulations resulted in delayed mite development where 79–96% of mites remained in the larval developmental stage (Figure 4B).
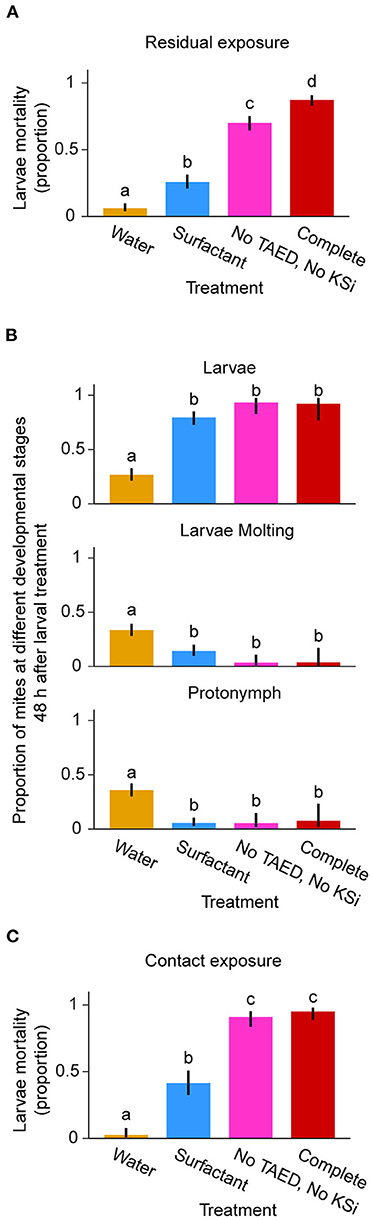
Figure 4. Contact and residual effects of Neo-Boost formulations on larvae. (A, B) Residual effect of neo-boost formulations on larval mortality 48 hours post-treatment (A, n = 60/treatment) and developmental progression [B, n = 29–60/treatment, depending on number of samples with surviving mites from (A)]. (C) Contact effect of neo-boost formulations on larval mortality 1-hour post-treatment (100 ≤ n ≤ 120/treatment). Shown are proportions of dead mites/developmental stages ± 95% confidence interval (CI). Letters within graphs represent differences between pairwise comparisons of means by Tukey's HSD (P < 0.05).
Evaluation of the contact effects of Neo-Boost formulations 1-hour post-direct spraying of larvae revealed a significant effect of treatment (χ2 = 123.32; df = 3; P < 0.0001). Larval mortality was 3% in water treatment, 41% in the surfactant treatment, and over 90% in the “no TAED, no KSi” and complete formulation treatments (91 and 95% respectively; Figure 4C). Therefore, Neo-Boost has both contact and residual acaricidal activities against T. urticae larvae.
The Systemic Effect of Neo-Boost Against Mites on Tomato
The experimental set-up for testing the ability of Neo-Boost to prime plant defenses against spider mites is shown in Figure 5A. Leaf damage associated with mite feeding on pre-treated tomato leaves, observed daily over 3 days, was similar across treatments as assessed through qualitative visual inspection, Figure 5B. Mite fecundity was 29, 24, and 27 eggs/mite upon treatment with water, surfactant, and Neo-Boost, respectively (Figure 5C), indicating that Neo-Boost did not trigger priming/systemic tomato defense responses, at least 3-days post-application, against mite herbivory.
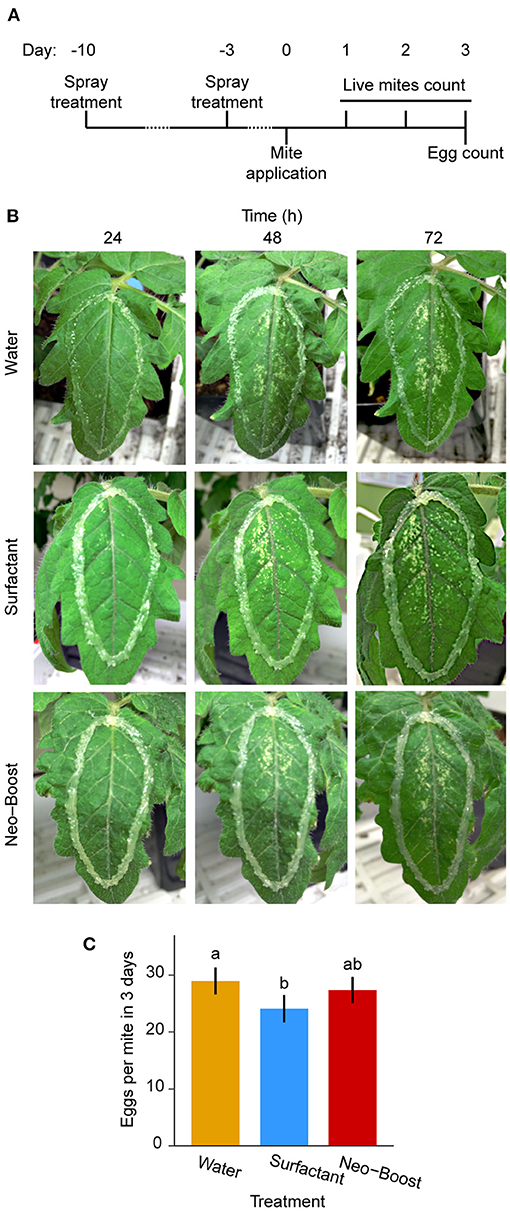
Figure 5. Systemic effect of Neo-Boost. (A) Schematic of the experiment. (B) Representative pictures of experimental tomato leaves over the 3-day period. (C) Fecundity of 10 London mites per treatment after 3 days of feeding. Shown are means of egg counts/mite ± 95% confidence interval (CI). Letters represent differences between pairwise comparisons of means by Tukey's HSD (P < 0.05).
Phytotoxicity of Neo-Boost
In general, Neo-Boost at the recommended concentration did not lead to phytotoxicity. In rare instances, sprayed droplets pooled within leaf creases, and when it persisted due to the humid environment created by the experimental setup, it led to phytotoxicity. These rare occurrences are documented visually in Figure 6.
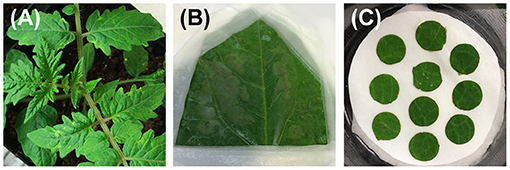
Figure 6. Phytotoxicity from Neo-Boost spraying. (A) A tomato plant from the priming experiment on the last day of spraying with neo-boost. Spots with yellowing represent the extent of phytotoxicity. (B) A detached bean leaf 48 hours post-infestation and spraying. Phytotoxicity was most evident in this experiment, where neo-boost did not dry completely after spraying in some spots. (C) Only a small amount of phytotoxicity was observed in the leaf-disk set-up, as neo-boost rarely pooled within disks.
Discussion
Neo-Boost was effective against larval and to some extent adult mite developmental stages tested under laboratory conditions. The effect on eggs was biologically negligible, though statistically significant. The greatest effect of Neo-Boost was seen on the larval stage, Figures 2–4. However, the proportion of larvae in any given mite population is relatively small compared to adults as the larval developmental stage lasts for 1–2 days (Herbert, 1981). Further tests of Neo-Boost efficacy on other immature mite stages (nymph stages) will clarify if Neo-Boost decreases in efficacy linearly as mite development progresses, or if all juvenile stages are affected to a similar extent as larvae. Additionally, the effect of Neo-Boost on the “tomato mite” strain was comparable to that of the London strain, suggesting that it may be effective across different mite populations.
Tetraacetylethylenediamine and sodium percarbonate that generate peroxyacetic acid (PAA) in water, and potassium silicate (KSi) were listed as active compounds in Neo-Boost formulation. However, formulations that lacked either PAA (“no TAED”) or KSi (“no KSi”) or both ingredients (“No TAED, No KSi”) had similar effectiveness against mites as a complete formulation, Figure 3. While the specific mode of Neo-Boost action against mites remains unknown, all maximally effective treatment formulations contained surfactant, sodium percarbonate, and “other components.” BIO-TERGE® AS-90, the surfactant used in the Neo-Boost formulation, affected larval mortality (Figures 2–4) but did not account for the full efficiency of the complete formulation. Surfactants can act directly as control agents causing drowning, destruction of biological membranes, enzyme inhibition, physical removal of individuals from leaf surfaces, and removal of cuticle wax or any combination thereof (Curkovic and Araya, 2004). The other disclosed component universally present in the effective treatments was sodium percarbonate. It is an adduct of sodium carbonate (also known as “soda ash” or “washing soda”) and hydrogen peroxide. When dissolved in water, sodium percarbonate produces hydrogen peroxide as well as sodium cations and carbonate, whose contributions should be further tested for efficacy against mites. Alternatively, the effect may be from “other” unspecified compound(s) within the formulation or any synergistic action therein. While specifics remain elusive, there is clear evidence that the Neo-Boost formulation can be simplified without a loss of its efficacy against mites. The effects of surfactant, sodium percarbonate, and “other” components likely affect mite physiology through several modes of action and suggests that target site mutation may be difficult to achieve. Therefore, should Neo-Boost prove to be effective in a production setting, as it is in the laboratory, it may be used as a tool in IPM strategies in mite pesticide resistance management. However, one can foresee resistance development through changes to the mite cuticle that would result in decreased penetrance of any effective compound, as has been documented or implicated in resistance to pyrethroids in several mosquito species that serve as vectors for malaria (Fang et al., 2015; Yahouédo et al., 2017; Simma et al., 2019). Resistance through decreased cuticle penetration has also been implicated in bed bugs, Cimex lectularius (Koganemaru et al., 2013).
Regarding the temporal aspect of Neo-Boost effects, the experiments performed on detached bean leaves/disks and tomato suggest that the effectiveness of formulations is rapid and last up to a maximum of 2 days as: (a) Neo-Boost did not affect mites 3 days after the final spray treatment (see Figure 5C); (b) high larval mortality was observed 1 hour after direct contact spraying (Figure 4C); and (c) effects of Neo-Boost are realized over 2 days as observed in Figures 2B,C (contact plus residual routs of exposure on larvae and adults respectively). Neo-Boost is generally safe in terms of human and environmental risk. Specifically, PAA and the components within the formulation that generate it degrade rapidly in the environment, and none of the products of the degradation process (acetic acid, hydrogen peroxide, water, and oxygen) are harmful at concentrations produced by Neo-Boost treatment at the recommended dose (Carrasco and Urrestarazu, 2010). The fast action and lack of toxic residue, usually associated with biopesticides, are important assets of Neo-Boost that are critical for production of fresh produce as well as other crops destined for human consumption (e.g., hops and cannabis). In addition, its independent mode of action makes it attractive for integrative pest and pesticide resistance management of mite populations. Several biopesticides have been tested against T. urticae (Ahn et al., 1998; Akyazi et al., 2018). Biopesticides often do not have the same degree of effectiveness as synthetic pesticides (Copping and Menn, 2000). For example, Chiasson et al. (2004) tested neem oil and insecticidal soap against mites and found that insecticidal soap caused very high egg and adult mite mortality while neem oil was highly effective against mite eggs and moderately against adults. In addition, Chandler et al. (2005) reported the effectiveness of entomopathogenic fungi Beauveria bassiana that reduces T. urticae populations by up to 97%. Furthermore, Chueca et al. (2010) tested several petroleum-derived oil formulations against mites on citrus leaves and found that they negatively affected egg hatchability as well as induced protonymph and adult mortality under laboratory conditions. At the mid-range concentrations, the oils from the Chueca et al. study had a moderate effect on eggs, a similar effect on protonymphs as seen with Neo-Boost, and were more effective than Neo-Boost on adults. Recently, Golec et al. (2020) tested a number of biopesticides including several bacterial products, a botanical product, and a mineral oil, using similar bioassays as described in this study. In comparison, Neo-Boost had greater efficacy against larvae and similar efficacy against adults as the biopesticides tested in the study by Golec et al. Therefore, even though the acaricidal effects of Neo-Boost are lagging behind synthetic pesticides, they are comparable to the efficiency of several biopesticides with regards to their effectiveness on larvae and adults. The effect of Neo-Boost on mite populations (structure and number) has yet to be determined.
Our data demonstrate that under laboratory settings Neo-Boost has high efficiency against larvae, moderate efficiency against adults, and no effect on eggs. These effects were observed when treatment was applied directly to mites or when mites are in contact with recently sprayed plant hosts. In addition, we did not detect the effect of Neo-Boost on the plant's ability to mount a systemic response against mites. Furthermore, we show that PAA and KSi are dispensable for Neo-Boost effects against mites, providing an opportunity to develop a novel acaricide with reduced chemical complexity without loss of efficacy against mites. Alternatively, it can be used in its current formulation or combined with other compatible active ingredients to derive a more potent acaricide that may be useful for mite control on crops that are destined for human consumption or medicinal use.
Data Availability Statement
The original contributions presented in the study are included in the article/Supplementary Material, further inquiries can be directed to the corresponding authors.
Author Contributions
KB, GS, VZ, FD, MG, and VG conceived, planned the study. KB and GS performed experimental procedures and collected data. KB performed statistical analyses. KB and VG wrote the manuscript. All authors contributed to the article and approved the submitted version.
Funding
This work was supported by the Government of Canada through the Ontario Research Fund (RE08-067) awarded to MG and VG, an NSERC Engage grant (EGP 484183 - 15 and EGP2 493182 - 16; co-funded by Agri-Neo Inc.) awarded to VG.
Conflict of Interest
The authors declare that this study was partially funded by Agri-Neo Inc. The funder was involved in the study design. The funder was not involved in the collection, analysis, interpretation of data, the writing of this article or the decision to submit it for publication.
Publisher's Note
All claims expressed in this article are solely those of the authors and do not necessarily represent those of their affiliated organizations, or those of the publisher, the editors and the reviewers. Any product that may be evaluated in this article, or claim that may be made by its manufacturer, is not guaranteed or endorsed by the publisher.
Acknowledgments
The authors thank Drs. James Walgenbach (NC State University) for critical comments on the manuscript.
Supplementary Material
The Supplementary Material for this article can be found online at: https://www.frontiersin.org/articles/10.3389/fagro.2021.701974/full#supplementary-material
Abbreviations
IPM, integrated pest management; KSi, potassium silicate; PAA, peroxyacetic acid; RNAi, RNA interference; TAED, tetraacetylethylenediamine.
References
Ahn, Y. J., Lee, S. B., Lee, H. S., and Kim, G. H. A. (1998). Insecticidal and acaricidal activity of carvacrol and β-thujaplicine derived from Thujopsis dolabrata var. hondai sawdust. J. Chem. Ecol. 24, 81–90. doi: 10.1023/A:1022388829078
Akyazi, R., Soysal, M., Altunç, E. Y., Lisle, A., Hassan, E., and Akyol, D. (2018). Acaricidal and sublethal effects of tobacco leaf and garlic bulb extract and soft soap on Tetranychus urticae Koch. (Acari: Trombidiformes: Tetranychidae). Syst. Appl. Acarol. 23, 2054–2069. doi: 10.11158/saa.23.10.13
Bensoussan, N., Dixit, S., Tabara, M., Letwin, D., Milojevic, M., Antonacci, M., et al. (2020). Environmental RNA interference in two-spotted spider mite, Tetranychus urticae, reveals dsRNA processing requirements for efficient RNAi response. Sci. Rep. 10:19126. doi: 10.1038/s41598-020-75682-6
Brady, S. M., Burow, M., Busch, W., Carlborg, Ö., Denby, K. J., Glazebrook, J., et al. (2015). Reassess the t test: interact with all your data via ANOVA. Plant Cell 27, 2088–2094. doi: 10.1105/tpc.15.00238
Carrasco, G., and Urrestarazu, M. (2010). Green chemistry in protected horticulture: the use of peroxyacetic acid as a sustainable strategy. Int. J. Mol. Sci. 11, 1999–2009. doi: 10.3390/ijms11051999
Chandler, D., Davidson, G., and Jacobson, R. J. (2005). Laboratory and glasshouse evaluation of entomopathogenic fungi against the two-spotted spider mite, Tetranychus urticae (Acari: Tetranychidae), on tomato, Lycopersicon esculentum. Biocontrol Sci. Technol. 15, 37–54. doi: 10.1080/09583150410001720617
Chiasson, H., Bostanian, N. J., and Vincent, C. (2004). Acaricidal properties of a chenopodium-based botanical. J. Econ. Entomol. 97, 1373–1377. doi: 10.1093/jee/97.4.1373
Chueca, P., Garcerá, C., Moltó, E., Jacas, J. A., Urbaneja, A., and Pina, T. (2010). Spray deposition and efficacy of four petroleum-derived oils used against tetranychus urticae (Acari: Tetranychidae). J. Econ. Entomol. 103, 386–393. doi: 10.1603/EC09242
Copping, L. G., and Menn, J. J. (2000). Biopesticides: a review of their action, applications and efficacy. Pest Manag. Sci. 56, 651–676. doi: 10.1002/1526-4998(200008)56:8andlt;651::AID-PS201andgt;3.0.CO;2-U
Cranham, J. E., and Helle, W. (1985). “Pesticide resistance in Tetranychidae,” in Spider Mites: Their Biology, Natural Enemies and Control, Vol. 1B, eds W. Helle, and M. W. Sabelis (Amsterdam: Elsevier), 405–421.
Curkovic, T., and Araya, J. E. (2004). Acaricidal action of two detergents against Panonychus ulmi (Koch) and Panonychus citri (Mcgregor) (Acarina: Tetranychidae) in the laboratory. Crop Prot. 23, 731–733. doi: 10.1016/j.cropro.2004.01.003
Dermauw, W., Osborne, E. J., Clark, R. M., Grbić, M., Tirry, L., and Van Leeuwen, T. (2013). A burst of ABC genes in the genome of the polyphagous spider mite Tetranychus urticae. BMC Genomics 14:317. doi: 10.1186/1471-2164-14-317
Fang, F., Wang, W., Zhang, D., Lv, Y., Zhou, D., Ma, L., et al. (2015). The cuticle proteins: a putative role for deltamethrin resistance in Culex pipiens pallens. Parasitol. Res. 114, 4421–4429. doi: 10.1007/s00436-015-4683-9
Fletcher, S. J., Reeves, P. T., Hoang, B. T., and Mitter, N. (2020). A perspective on RNAi-based biopesticides. Front. Plant Sci. 11:51. doi: 10.3389/fpls.2020.00051
Golec, J. R., Hoge, B., and Walgenbach, J. F. (2020). Effect of biopesticides on different Tetranychus urticae Koch (Acari: Tetranychidae) life stages. Crop Prot. 128:105015. doi: 10.1016/j.cropro.2019.105015
Grbić, M., Van Leeuwen, T., Clark, R. M., Rombauts, S., Rouzé, P., Grbić, V., et al. (2011). The genome of Tetranychus urticae reveals herbivorous pest adaptations. Nature 479, 487–492. doi: 10.1038/nature10640
Head, G. P., Carroll, M. W., Evans, S. P., Rule, D. M., Willse, A. R., Clark, T. L., et al. (2017). Evaluation of smartstax and smartstax PRO maize against western corn rootworm and northern corn rootworm: efficacy and resistance management. Pest Manag. Sci. 73, 1883–1899. doi: 10.1002/ps.4554
Herbert, H. J. (1981). Biology, life tables, and innate capacity for increase of the twospotted spider mite, Tetranychus urticae (Acarina: Tetranychidae). Can. Entomol. 113, 371–378. doi: 10.4039/Ent113371-5
Insecticide Resistance Action Committee (2020). Mode of Action classification Scheme. Available online at: https://irac-online.org/mode-of-action/ (accessed November 27, 2020).
Koganemaru, R., Miller, D. M., and Adelman, Z. N. (2013). Robust cuticular penetration resistance in the common bed bug (cimex lectularius L.) correlates with increased steady-state transcript levels of CPR-type cuticle protein genes. Pestic. Biochem. Physiol. 106, 190–197. doi: 10.1016/j.pestbp.2013.01.001
Li, C., Williams, M. M., Loh, Y.-T., Lee, G. I., and Howe, G. A. (2002). Resistance of cultivated tomato to cell content-feeding herbivores is regulated by the octadecanoid-signaling pathway. Plant Physiol. 130, 494–503. doi: 10.1104/pp.005314
Ma, J. F. (2004). Role of silicon in enhancing the resistance of plants to biotic and abiotic stresses. Soil Sci. Plant Nutr. 50, 11–18. doi: 10.1080/00380768.2004.10408447
Ma, J. F., and Yamaji, N. (2006). Silicon uptake and accumulation in higher plants. Trends Plant Sci. 11, 392–397. doi: 10.1016/j.tplants.2006.06.007
Migeon, A., and Dorkeld, F. (2021). Spider Mites Web: a Comprehensive Database for the Tetranychidae. Available online at: http://www1.montpellier.inra.fr/CBGP/spmweb (accessed August 17, 2020).
Mota-Sanchez, D., and Wise, J. C. (2021). The Arthropod Pesticide Resistance Database. Michigan State University. Available online at: https://www.pesticideresistance.org/display.php?page=speciesandarId=536 (accessed April 22 2021).
Osakabe, M., Uesugi, R., and Goka, K. (2009). Evolutionary aspects of acaricide-resistance development in spider mites. Psyche 2009:947439. doi: 10.1155/2009/947439
Pieterse, C. M. J., Van der Does, D., Zamioudis, C., Leon-Reyes, A., and Van Wees, S. C. M. (2012). Hormonal modulation of plant immunity. Annu. Rev. Cell Dev. Biol. 28, 489–521. doi: 10.1146/annurev-cellbio-092910-154055
R Core Team (2019). R: A Language and Environment for Statistical Computing. Vienna: R Foundation for Statistical Computing. Available online at: https://www.R-project.org/ (accessed June 30, 2020).
Reynolds, O. L., Keeping, M. G., and Meyer, J. H. (2009). Silicon-augmented resistance of plants to herbivorous insects: a review. Ann. Appl. Biol. 155, 171–186. doi: 10.1111/j.1744-7348.2009.00348.x
Sakr, N. (2017). The role of Silicon (Si) in increasing plant resistance against insect pests review article. Acta Phytopathol. Entomol. Hungarica 52, 185–203. doi: 10.1556/038.52.2017.020
Salehipourshirazi, G., Bruinsma, K., Ratlamwala, H., Dixit, S., Arbona, V., Widemann, E., et al. (2021). Rapid specialization of counter defenses enables two-spotted spider mite to adapt to novel plant hosts. Plant Physiol. (in press). doi: 10.1093/plphys/kiab412
Santamaría, M. E., Arnaiz, A., Velasco-Arroyo, B., Grbic, V., Diaz, I., and Martinez, M. (2018). Arabidopsis response to the spider mite Tetranychus urticae depends on the regulation of reactive oxygen species homeostasis. Sci. Rep. 8, 1–13. doi: 10.1038/s41598-018-27904-1
Simma, E. A., Dermauw, W., Balabanidou, V., Snoeck, S., Bryon, A., Clark, R. M., et al. (2019). Genome-wide gene expression profiling reveals that cuticle alterations and P450 detoxification are associated with deltamethrin and DDT resistance in Anopheles arabiensis populations from Ethiopia. Pest Manag. Sci. 75, 1808–1818. doi: 10.1002/ps.5374
Sode, F. (2019). Analytical methods for peroxo acids-a review. Anal. Methods 11, 3372–3380. doi: 10.1039/C9AY00860H
Suzuki, T., España, M. U., Nunes, M. A., Zhurov, V., Dermauw, W., Osakabe, M., et al. (2017a). Protocols for the delivery of small molecules to the two-spotted spider mite, Tetranychus urticae. PLoS ONE 12:e0180658. doi: 10.1371/journal.pone.0180658
Suzuki, T., Nunes, M. A., España, M. U., Namin, H., Jin, P., Bensoussan, N., et al. (2017b). RNAi-based reverse genetics in the chelicerate model Tetranychus urticae: a comparative analysis of five methods for gene silencing. PLoS ONE 12:e0180654. doi: 10.1371/journal.pone.0180654
Widemann, E., Bruinsma, K., Walshe-Roussel, B., Rioja, C., Arbona, V., Saha, R. K., et al. (2021). Multiple indole glucosinolates and myrosinases defend Arabidopsis against Tetranychus urticae herbivory. Plant Physiol. 187, 116–132. doi: 10.1101/2021.02.03.429630
Ximénez-Embún, M. G., Castañera, P., and Ortego, F. (2017). Drought stress in tomato increases the performance of adapted and non-adapted strains of Tetranychus urticae. J. Insect Physiol. 96, 73–81. doi: 10.1016/j.jinsphys.2016.10.015
Yahouédo, G. A., Chandre, F., Rossignol, M., Ginibre, C., Balabanidou, V., Mendez, N. G. A., et al. (2017). Contributions of cuticle permeability and enzyme detoxification to pyrethroid resistance in the major malaria vector Anopheles gambiae. Sci. Rep. 7, 1–10. doi: 10.1038/s41598-017-11357-z
Zhurov, V., Navarro, M., Bruinsma, K. A., Arbona, V., Santamaria, M. E., Cazaux, M., et al. (2014). Reciprocal responses in the interaction between arabidopsis and the cell-content-feeding chelicerate herbivore spider mite. Plant Physiol. 164, 384–399. doi: 10.1104/pp.113.231555
Keywords: agricultural pest, peroxyacetic/peracetic acid, silica, hydrogen peroxide, pesticide resistance management
Citation: Bruinsma K, Salehipourshirazi G, Zhurov V, Dagher F, Grbic M and Grbic V (2021) Effect of Neo-Boost Pesticide on Mortality and Development of Different Life Stages of Two-Spotted Spider Mite, Tetranychus urticae. Front. Agron. 3:701974. doi: 10.3389/fagro.2021.701974
Received: 28 April 2021; Accepted: 28 September 2021;
Published: 25 October 2021.
Edited by:
Salvatore Arpaia, Energy and Sustainable Economic Development (ENEA), ItalyReviewed by:
John Charles Wise, Michigan State University, United StatesCarlo Duso, University of Padua, Italy
Copyright © 2021 Bruinsma, Salehipourshirazi, Zhurov, Dagher, Grbic and Grbic. This is an open-access article distributed under the terms of the Creative Commons Attribution License (CC BY). The use, distribution or reproduction in other forums is permitted, provided the original author(s) and the copyright owner(s) are credited and that the original publication in this journal is cited, in accordance with accepted academic practice. No use, distribution or reproduction is permitted which does not comply with these terms.
*Correspondence: Kristie Bruinsma, a2JydWluc21AdXdvLmNh; Vojislava Grbic, dmdyYmljQHV3by5jYQ==
†These authors have contributed equally to this work