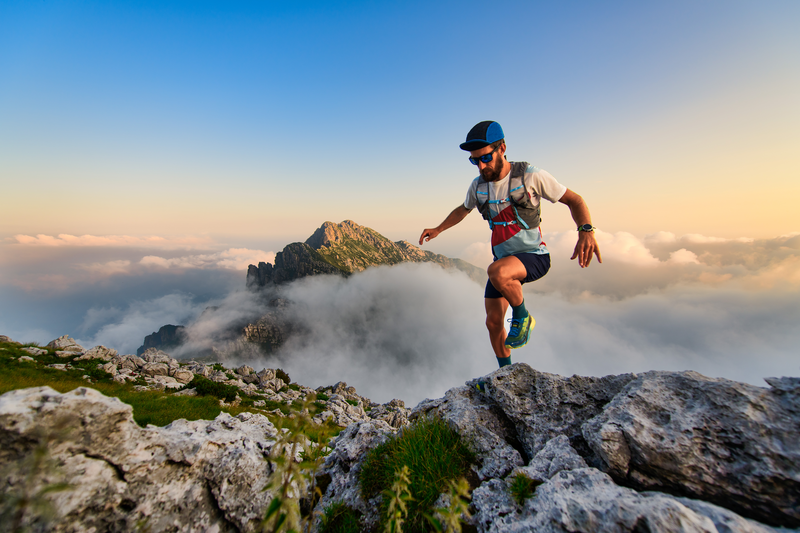
94% of researchers rate our articles as excellent or good
Learn more about the work of our research integrity team to safeguard the quality of each article we publish.
Find out more
ORIGINAL RESEARCH article
Front. Agron. , 11 June 2021
Sec. Disease Management
Volume 3 - 2021 | https://doi.org/10.3389/fagro.2021.684023
European grapevine, Vitis vinifera, carries no major resistances against Plasmopara viticola, the causal agent of grapevine downy mildew. The introgression of quantitative trait loci conferring resistance to P. viticola (Rpv) from American and Asian donor species has resulted in a range of resistant cultivars. In light of the perennial nature of grapevine and the high evolutionary potential of P. viticola, the durability of this quantitative resistance is an important challenge. Durability of host resistance and variability in pathogen virulence may be evaluated by describing interactions between pathogen isolates and grapevine cultivars in terms of Rpv loci. A set of 16 cultivars carrying different combinations of Rpv loci, was challenged with five P. viticola isolates, obtained from susceptible or Rpv3.1+ V. vinifera cultivars. Based on the severity of sporulation, different host and pathogen phenotypes might be distinguished, which could be related to the presence of different Rpv loci. The hormonal responses before and during some interactions were compared to assess the resistance mechanisms underlying Rpv3.1, Rpv10, and Rpv12 and the infection mechanisms of the different isolates. This paper reports on the strength of some of the commonly used Rpv loci, single or stacked. The isolates derived from Rpv3.1+ hosts, GREPv1 and GPHPv1, were able to sporulate intensely on cultivars carrying Rpv3.1, without triggering necrosis. Moreover, Rpv10 was not able to efficiently halt the development of the Rpv3.1-breaking isolate GPHPv1. Cultivars carrying Rpv12, however, were resistant to all five P. viticola isolates. Phytohormones might be implicated in the basal resistance against this pathogen, but during the early defense response, no significant hormonal responses to the isolates were observed. The isolate-specificity of the Rpv3- and Rpv10-mediated resistance suggests that these loci do not result in the most sustainable resistance. Furthermore, the isolate-specific behavior of the pathogen emphasizes the need for a characterization system for P. viticola. A standardized phenotyping assay may be used to determine P. viticola pathogen phenotypes or measure the durability, strength, and isolate-specificity of the host quantitative resistances. The characterization of both components of the pathosystem may lead to an increased understanding of the resistance mechanisms, beneficial for a durable deployment of resistance genes.
The obligatory biotrophic pathogen Plasmopara viticola, the causal agent of grapevine downy mildew, is considered one of the most economically important oomycetes (Kamoun et al., 2015). Vitis vinifera, the only grapevine species endemic in Europe, carries no resistance to this pathogen, except for a minor locus identified in Chardonnay (Bellin et al., 2009). Therefore, viticulture is heavily dependent on chemical protection. The recurrent use of high amounts of pesticides has led to the emergence of P. viticola strains tolerant to the most commonly applied groups of site-specific fungicides (Chen et al., 2007; Gisi et al., 2007; Blum et al., 2010; Genet and Jaworska, 2013). Breeding of new cultivars resistant to the pathogen is being explored as a promising strategy to minimize chemical control. Controlled grapevine breeding was first launched in Europe following the introduction of phylloxera and powdery and downy mildews during the mid to late 19th century (Töpfer et al., 2011). Mildew resistances are still among the first criteria in a breeding program to select prime candidates from the seedling mass, before the evaluation of their viticultural performance and wine quality.
Breeders utilize the resistance of American and Asian donor species and the closely related Muscadinia rotundifolia (Töpfer et al., 2011). Their genetic resistance to downy mildew is assumed to arise from gene-for-gene recognition and subsequent activation of the vine's defense (Peressotti et al., 2010). Recognition occurs when a resistance (R) gene and the corresponding avirulence (Avr) gene are present in the interacting plant and pathogen, respectively. Knowledge about Avr and R genes in the P. viticola/grapevine pathosystem is still limited. To our knowledge, nothing is known about the nature of the Avr genes in P. viticola, although it is likely that they encode RxLR effectors as was shown in other oomycetes (Anderson et al., 2015). R genes encode proteins containing a nucleotide-binding site (NBS) and leucine-rich repeats (LRR) (Zini et al., 2019). The development of molecular markers has allowed the description of 31 quantitative trait loci (QTLs) (Maul et al., 2020) where the R-genes are located, referred to as Rpv (resistance to Plasmopara viticola). Some of these QTLs only have a minor effect on the downy mildew resistance level. These weak QTLs, e.g., Rpv4 in Regent (Welter et al., 2007) and Rpv11 in Chardonnay (Bellin et al., 2009), Regent (Fischer et al., 2004), and Solaris (Schwander et al., 2012), are not particularly suited for use in breeding programs. Major QTLs, on the other hand, significantly affect the susceptibility to P. viticola. Rpv3 is historically the most common major resistance locus in European resistant cultivars (Foria et al., 2020). The Rpv3 locus on chromosome 18 (Welter et al., 2007) originates from North-American Vitis sp. (Bellin et al., 2009). Peculiarly, seven conserved Rpv3 haplotypes have been identified, named according to the UDV305-UDV737 allele sizes: Rpv3299−297 (Rpv3.1), Rpv3null−297 (Rpv3.2), Rpv3null−271 (Rpv3.3), Rpv3321−312, Rpv3null−287, Rpv3361−299, and Rpv3299−314 (Di Gaspero et al., 2012). The most frequently occurring haplotypes Rpv3.1 (Welter et al., 2007; Bellin et al., 2009; van Heerden et al., 2014) and Rpv3.2 (Zyprian et al., 2016), but also Rpv3.3 (Vezzulli et al., 2019), have been validated in segregating populations. The major QTLs Rpv1, Rpv10, and Rpv12 are also frequently deployed in grapevine breeding. Rpv1, originating from the American M. rotundifolia, was mapped to chromosome 12 (Merdinoglu et al., 2003). It is closely associated with Run1, the locus conferring resistance to grapevine powdery mildew (Feechan et al., 2013). Rpv10 and Rpv12, mapped on chromosome 9 (Schwander et al., 2012) and 14 (Venuti et al., 2013), respectively, are both donated by V. amurensis, native to Asia.
One of the most important challenges of breeding for resistance is durability. Disease resistances should preserve their effectiveness over time, even when they are employed widely in a favorable environment for the pathogen (Johnson, 1984). However, like fungicides, host genetic resistance might impose a strong directional selection for local adaptation. In grapevine, this deserves special consideration, since its perennial nature requires a vine to grow for decennia in the vineyard and the cultivar itself to be used for centuries (Töpfer et al., 2011). Moreover, P. viticola is suggested to have a high evolutionary potential (Gobbin et al., 2006) and the shape of its population is strongly determined by selection (Stark-Urnau et al., 2000). The breakdown of host resistance by some P. viticola isolates has already been reported in the resistant cultivars Bianca (Peressotti et al., 2010) and Regent (Kast et al., 2000; Delmotte et al., 2014), both carrying Rpv3 (Welter et al., 2007; Di Gaspero et al., 2012). These isolates might be escaping specific recognition by the Rpv3 gene product through a mutation in the corresponding Avr gene (Peressotti et al., 2010).
To improve durability, modern breeding focuses on pyramiding of resistance genes. For a durable deployment of resistance genes, the characterization of both components of the pathosystem is essential. On the plant's side, many studies have been mapping the Rpv loci but not much is known about how they convey resistance and how the resistance benefits from stacking. On the pathogen's side, more insight into the virulence variability of the pathogen is crucial. The genetic diversity of P. viticola populations in the field is high (Stark-Urnau et al., 2000; Gobbin et al., 2006; Scherer and Gisi, 2006) and increases through recombination in oospores during the sexual reproduction phase. The diversity in virulence among isolates might be explained by the level of expression of RxLR effector genes (Gómez-Zeledón and Spring, 2018). Although the challenge with single-action fungicide or single-gene host resistance drives the appearance of physiological races or pathotypes (Gessler et al., 2011), the classification of the interaction between P. viticola and grapevine rarely focuses on the pathogen. The virulence of P. viticola isolates has been characterized previously and their behavior on a limited set of wild or cultivated hosts from Europe, Asia, and North-America has been described (Gómez-Zeledón et al., 2013, 2017). Performing this kind of characterization in terms of Rpv loci could provide a more robust idea about the durability of the Rpv loci.
The current study aimed to assess the durability of some of the commonly used loci conferring resistance to P. viticola and the influence of stacking and heritage, with regards to different isolates of P. viticola. At the same time, this study provides an indication of the diversity in virulence of P. viticola isolates in a vineyard with a high occurrence of these Rpv loci. We also assessed the role of phytohormones in this interaction. Phytohormones are implicated in the resistance of the donor species against P. viticola (Liu et al., 2016; Wang et al., 2018), but not much is known about their role in resistant cultivars. P. viticola isolates were collected from host cultivars from a collection in the German Pfalz region, where the presence of many cultivars carrying various resistances to P. viticola causes an increased selection pressure, and from the traditional French Champagne region. A set of 16 cultivars, carrying different resistance loci, was challenged with these isolates to determine host and pathogen phenotypes. The hormonal responses before and during some of these interactions were compared to assess the resistance mechanisms underlying Rpv3.1, Rpv10, and Rpv12.
The 16 grapevine cultivars used in this work are represented in Table 1. A simplified pedigree showing the relationship between various cultivars is shown in Supplementary Figure 1. Cuttings of these 16 cultivars were rooted in Jiffy-7 peat pellets for 2 months, before transplantation in four-liter pots containing universal potting soil (type 1; Snebbout, Kaprijke, Belgium). Plants were grown in a greenhouse at 24/19°C, 16/8 h light/dark regime. To guarantee a high and continuous availability of young, healthy and uniform plant material, the susceptible cultivar Chardonnay (clone 7535) and the resistant cultivars Solaris, Regent, and Lela were also grown in tissue culture. To establish a tissue culture of the resistant cultivars, shoots from adult greenhouse plants were cut into uninodal segments and surface-sterilized for 5 min in 1% NaOCl. After three rinses, the dried nodal explants were transferred to semisolid MM medium (Martin et al., 1987). Plantlets from the four cultivars were micro-propagated as described by Ait Barka et al. (2006).
A simplified pedigree of the cultivars (Supplementary Figure 1) was reconstructed according to the data confirmed by markers reported in the Vitis International Variety Catalogue (Maul et al., 2020) (VIVC). Most information about the resistance traits was also found in the VIVC. Supplementary information about the Rpv3 locus was added in accordance with Di Gaspero et al. (2012). Delmotte et al. (2014) and Zini et al. (2019) confirmed the presence of the Rpv1 locus in Montpellier 3082-1-42 and of the Rpv12 locus in Lela. Solaris was described to carry Rpv10 (Schwander et al., 2012), as well as Rpv3.3 (Possamai et al., 2020).
Infected leaves were sampled from a traditional grapevine collection in the University of Reims Champagne-Ardenne (URCA) in Reims, France, and in the breeding collection of the Julius Kühn Institute (JKI) for grapevine breeding in Siebeldingen, Germany. The URCA is located in the French Champagne region, primarily cultivated with the susceptible V. vinifera cv. Pinot Noir (38%), Pinot Meunier (31%), and Chardonnay (30%) (Le Comité Champagne, 2020). The German Pfalz region, where the JKI is situated, has a higher varietal diversity, still dominated by susceptible V. vinifera cultivars, with Riesling (25%) and Dornfelder (11.8%) on top (Statistisches Landesamt Rheinland-Pfalz, 2020). Since 2009, the fungus-tolerant variety Regent has also been covering an important part of the Pfalz viticultural area (2.2%). The grapevine collection of the JKI in Siebeldingen is particularly diverse, counting more than 3,000 wild relatives, breeding lines, and cultivars, of which more than half carry resistance genes against powdery or downy mildew.
A sterile needle was used to pick up a single sporangiophore from a lesion of the sampled field material. This single sporangiophore was transferred to a drop of sterile, distilled water on the abaxial side of a Chardonnay leaf, previously detached from an in vitro plantlet. Inoculated leaves were incubated on water agar (0.65%) at 16/8 h photoperiod, 22/18°C. This isolation step was repeated at least three times. In the end, five single sporangiophore isolates were obtained (Table 2). The cultivars from which these isolates were isolated, are highlighted in the simplified pedigree in Supplementary Figure 1. The isolates were preserved on infected leaves at −20°C. They were weekly propagated on leaves of Chardonnay plantlets.
The 3rd and 4th fully expanded leaves, counted from the apex of the shoot, were collected from adult greenhouse plants. In this assay, a set of 16 V. vinifera cultivars, carrying different resistance loci (Table 1), was challenged with 5 P. viticola isolates (Table 2). Leaves were punched into disks (11 mm diameter), pooled, and placed abaxial side up on water agar. Subsequently, the leaf disks were inoculated with a 20 μL drop of a P. viticola suspension (5 × 104 sporangia mL−1). The disease progression was evaluated at 6 days post inoculation (dpi). Each disk was assigned to one of five classes based on the number of sporangiophores (Figure 1A). A disease severity index (DSI), ranging from 0% (no disease) to 100% (maximally diseased), was calculated as the mean disease grade divided by the maximal disease grade. The experiment was repeated twice with on average 20 disks per pathogen-cultivar combination. The average level of necrosis at the evaluation stage was estimated according to the scale in Figure 2.
Figure 1. Scoring system used to classify the severity of the P. viticola infection following (A) drop inoculation. The 5-point grading scale, adapted from Schwander et al. (2012), consists of: 0, 0 sporangiophores; 1, 1–6 sporangiophores; 2, 7–20 sporangiophores; 3, more than 20 sporangiophores; 4, numerous sporangiophores. (B) spray inoculation. The five classes are: 0, no symptoms; 1, single sporangiophores; 2, dispersed sporulation; 3, intermediate sporulation; 4, heavy sporulation.
Figure 2. Scoring system used to classify the necrosis of the P. viticola infection, adapted from Gómez-Zeledón et al. (2016). The necrosis scale consists of the following four classes: 0, absent (no necrosis); 1, weak (scattered, small necrotic spots); 2, moderate (many small necrotic spots); 3, strong (large or abundant small necrotic spots).
Based on the phenotyping assay, different host and pathogen phenotypes could be distinguished. The isolate-specific resistance conferred by Rpv3.1 and Rpv10 and the powerful resistance conferred by Rpv12 were of particular interest. To investigate the involvement of phytohormone response before and during the first stages of the interaction, additional cross-inoculation assays were conducted using entire detached leaves or plantlets, rather than leaf disks, to reduce the influence of wounding. Regent, Solaris, and Lela were chosen to represent Rpv3.1+, Rpv10+, and Rpv12+ cultivars, respectively. Chardonnay or Diana were included as a susceptible control. They were challenged with one representative of each pathogen phenotype (GPN1, GRE1, and GPH1).
To closer inspect these differential interactions, a spray inoculation was performed on detached leaves of greenhouse plants (Regent, Solaris, and Lela). Eight leaves were detached per plant and immediately placed abaxial side up on water agar (0.65%). Per plant, the leaves were sprayed pairwise with distilled water or with a sporangia suspension (5 × 104 sporangia ml−1) of P. viticola (GPN1, GRE1, and GPH1) within 8 h after detachment. At 6, 12, and 24 h post inoculation (hpi), three replicates were collected for hormone analysis. The sporulation severity was evaluated at 8 dpi on 8 leaves per treatment. Each leaf was assigned to a 5-point grading scale for spray-inoculation (Figure 1B).
To limit the biological variability, an additional infection assay was performed on intact plantlets of Chardonnay, Regent, Solaris, and Lela. Two-months old tissue culture plantlets were transplanted in sterilized, universal potting soil in a propagator (at 24°C, 16/8 h light/dark). After a 4-week acclimatization and 6-week growing period, all leaves were spray-inoculated with distilled water or with a sporangial suspension (5 × 104 sporangia ml−1) of P. viticola (GPN1, GRE1, and GPH1). Per treatment, twenty young plantlets were used. They were incubated at 100% relative humidity during the first 24 h following inoculation. Five plants were sampled at 12 hpi for phytohormone analysis. The sporulation severity of each plant was evaluated at 10 dpi according to the 5-point grading scale for spray-inoculation (Figure 1B). At the same time, the necrosis was scored as follows: 0, no necrosis; 1, scattered small necrotic lesions; 2, many small necrotic lesions; 3, large or abundant small necrotic spots. Similar to the disease severity index, a necrosis index was calculated, ranging from 0% (no disease) to 100% (maximally diseased), as the mean necrosis grade divided by the maximal necrosis grade.
The levels of abscisic acid (ABA), indole-3-acetic acid (IAA), jasmonic acid (JA), and salicylic acid (SA) were determined at the beginning of the infection and during the initial stages of infection. The basal phytohormone content was determined on leaves of adult greenhouse plants (Diana, Regent, Solaris, and Lela), with 5 replicates. The evolution of the phytohormone content during the infection was analyzed in leaves sampled from the detached leaf assay and the plant assay, with 3 and 5 replicates, respectively. For each replicate, two leaves of the same plant were pooled, immediately frozen in liquid N2, and kept at −80°C until analysis. The procedure for the quantification of phytohormones is described in detail by Haeck et al. (2018) and Heyman et al. (2021).
Statistical analysis was conducted using R, version 3.6.1 (R Core Team, 2019). The data were analyzed using the Kruskal-Wallis test, followed by the Dunn test (p = 0.05). The heat map was constructed using the ComplexHeatmap package (Gu et al., 2016).
The level of host adaptation of the five P. viticola isolates to the 16 grapevine cultivars was assessed in a leaf disk assay. Leaf disks representative for each interaction are presented in Supplementary Figure 2. For each interaction, the severity of sporulation was evaluated (Supplementary Figure 3) and an index calculated. This DSI revealed substantial differences between cultivars inoculated with the same isolate (Figure 3). Five cultivar groups (CGs) were distinguished by cluster analysis. Moreover, the contrasting interaction of some cultivars with the different isolates uncovered heterogeneous pathogen phenotypes. The DSI clustered the isolates into three isolate groups (IGs). The Rpv loci present in the tested cultivars supported the grouping of isolates and cultivars based on the disease severity (Figure 3) to a great extent.
Figure 3. Heat map of the disease severity index (DSI), based on the severity of sporulation on 40 leaf disks on average. Single-linkage hierarchical clustering of the DSI divided the isolates into 3 isolate groups (IG 1–3) and the cultivars into 5 cultivar groups (CG 1–5). The average level of necrosis for each interaction is indicated, as well as the major resistance loci for each cultivar.
The highest susceptibility was observed in CG 2 (Diana, Calardis Musqué, Bacchus, Chardonnay, Merzling, and Seyval), which contained the cultivars without major resistance alleles, as well as cultivars solely carrying Rpv3.2 and/or Rpv3.3. As expected, no resistance was observed in the cultivars without major resistance alleles. In cultivars carrying only Rpv3.2 (Calardis Musqué), Rpv3.3 (Merzling), or their combination (Seyval) a comparable, high susceptibility was observed, though combined with some necrosis. In this phenotyping assay, conducted under in vitro conditions, these alleles seemed to trigger a resistance mechanism that did not efficiently influence the disease development. CG 4 (2011-64-0002) was only carrying Rpv1. In this breeding line, Rpv1 merely imparted partial resistance with high necrosis, reducing the disease severity for all P. viticola isolates equally. In CG 1 (Lela and 2012-114-0133), showing no susceptibility, the common resistance allele was Rpv12. No prominent necrosis or sporulation was associated with these interactions. In Lela, this locus alone resulted in powerful resistance. In the breeding line 2012-114-0133, Rpv12 was stacked with Rpv1 and Rpv3.1, causing complete inhibition of sporulation. This conclusive resistance seemed primarily mediated by Rpv12 since the Rpv1 and Rpv3.1-mediated resistance response included noteworthy necrosis.
For the other CGs, the susceptibility showed a sharp contrast between the IGs. The cultivars in CG 5 (Calardis Blanc, Villard Blanc, 2004-051-0001, Chambourcin, and Regent) were all associated with Rpv3.1. Contrary to the other Rpv3 haplotypes (Rpv3.2 and Rpv3.3), Rpv3.1 seemed to provide an efficacious resistance response against IG 3 (GPN1, FCH1, and GPN2). Necrosis was triggered upon challenge with these isolates. IG 3 isolates had been obtained from cultivars that do not carry major Rpv loci. In contrast, the resistance conferred by Rpv3.1 seemed no longer efficacious against IG 2 (GRE1) and IG 1 (GPH1). The latter isolates had been isolated from Regent and Phoenix, respectively, both carrying Rpv3.1. All cultivars, and Villard Blanc foremost, were heavily infected by GRE1 and GPH1. No necrosis was observed after the successful infection of single Rpv3.1+ cultivars with GRE1 and GPH1. The additional presence of the weak Rpv3.2 in Calardis Blanc or Chambourcin did not prevent the breakdown of the Rpv3.1-mediated resistance by these isolates. The breeding line 2004-051-0001, carrying Rpv10 on top of Rpv3.1, was less susceptible to GRE1 compared to the other cultivars in CG 5. This breeding line still showed major susceptibility to GPH1.
Leaf disks of CG 3 (Seyve Villard 39-639 and Solaris) were susceptible to GPH1 but resistant to the other isolates. Despite its strong resistance, Seyve Villard 39-639 is currently known to carry only Rpv3.1. Seyve Villard 39-639 behaved remarkably similar to Solaris in this phenotypic evaluation. Solaris is known to carry Rpv3.3 and Rpv10. Since Rpv3.3 on its own did not reduce the disease severity in CG 2, Rpv10 is expected to be its primary source of resistance against the IG 2 (GRE1) and IG 3 (GPN1, FCH1, and GPN2). GPH1 (IG 1) was able to sporulate on the Rpv10+ cultivar Solaris, though not as densely as on Rpv3.1+ cultivars (CG 5).
The phenotyping assay showed that Rpv12 conferred the strongest resistance, while Rpv3.1- and Rpv10-mediated resistances were isolate-specific. To further examine these interesting interactions, the phenotyping assay was extended with a detached leaf assay (Figure 4A) and plant assay (Figures 4B,C). Representatives of CG 1 (Lela), CG 3 (Solaris), and CG 5 (Regent), were challenged with representatives of IG 1 (GPH1), IG 2 (GRE1), and IG 3 (GPN1). Pathogen inoculum was used to spray-inoculate the cultivars, either via in vitro inoculation of detached leaves of adult greenhouse plants, or via in planta inoculation of acclimatized plantlets obtained through tissue culture. In the latter, Chardonnay (CG 2) was included as a susceptible control. The results of both assays complemented the leaf disk assay, used for phenotyping (Figure 3). In all three assays, a high disease severity was observed in the interaction of Regent (Rpv3.1) with the isolates GRE1 and GPH1, comparable to the interaction with cultivars carrying no major resistance alleles, like Chardonnay. Interestingly, the isolate-specificity of the interaction between Solaris (Rpv3.3 and Rpv10) and GPH1 was revealed in the three assays, although the degree of sporulation varied between the assays. GPH1 sporulated less on intact Solaris plantlets compared to intact Regent or Chardonnay plantlets (Figure 4B). Both Regent and Solaris showed high resistance to GPN1 (Figures 4A,B), associated with necrosis (Figure 4C). Infections caused by GRE1 and GPH1 were associated with a reduced degree of necrosis compared to GPN1 infections, except in Solaris plants infected with GPH1, where an increased degree of necrosis was apparent (Figure 4C). None of the isolates was able to sporulate or induce necrosis in Lela (Rpv12). These results confirm the powerful resistance of Lela to P. viticola observed in the leaf disk assay.
Figure 4. Evaluation of the disease severity and necrosis in different cultivar-isolate combinations. (A) Disease severity following in vitro inoculation of detached leaves of adult plants (n = 8). (B) Disease severity and (C) necrosis following in planta inoculation of intact, young plantlets (n = 20). The disease severity index was calculated based on the severity of sporulation. Different letters indicate significant differences in the corresponding scoring of disease or necrosis (Kruskal-Wallis test followed by Dunn test; p = 0.05).
To assess the involvement of phytohormones in the defense underlying Rpv3.1, Rpv10, and Rpv12, the hormonal responses before and during some specific interactions were compared. The isolate-specific behavior of Rpv3.1 and Rpv10, in particular, allowed to examine the mechanisms underlying these resistances in more detail. The phytohormone content before and during the first stages of the interaction with the different pathogen phenotypes IG 1 (GPH1), IG 2 (GRE1), and IG 3 (GPN1) was determined. The basal differences in hormone content were determined in non-infected leaves of susceptible cultivars (Diana and Chardonnay, representing CG 2) and resistant cultivars (Lela, Solaris, and Regent as representatives of CG 1, CG 3, and CG 5, respectively) (Table 3). These leaves had been collected in three conditions in which P. viticola can easily infect. Uninfected leaves were collected from young plantlets (in the plant assay) or from adult greenhouse plants. Leaves from adult plants were sampled either immediately following detachment from the plant, or after 8 h of detachment on water agar (in the detached leaf assay). To assess the phytohormone content during the early stages of the interaction between CG 1, CG 3, and CG 5 (Lela, Solaris, and Regent respectively) with IG 1, IG 2, and IG 3 (isolates GPH1, GRE1, and GPN1 respectively), the levels of ABA, IAA, SA, and JA were determined in leaves from the detached leaf assay (at 6, 12, and 24 hpi; Supplementary Figure 4) and plant assay (at 12 hpi; Supplementary Figure 5). In the latter, Chardonnay (CG 2) was included as a susceptible control.
Table 3. Basal phytohormone levels in leaves of different cultivars, according to the sampling condition.
In non-infected leaves, the hormone levels differed depending on the conditions and cultivar (Table 3). Although the levels of the phytohormones IAA, ABA, and SA were much lower in the young plantlets than in the adult greenhouse plants, prior or after detachment, the trends were generally similar. Of the resistant cultivars, Regent and Lela tended toward the highest and lowest basal levels of ABA, respectively. Solaris showed high basal levels of SA and JA. The JA levels might have peaked with detachment since the JA content was particularly high in detached leaves of Solaris. However, the detachment of the leaf caused the levels of JA to decrease strongly over time in Solaris (Supplementary Figure 4). ABA, IAA, and SA levels remained fairly constant during this detachment period.
To investigate the hormone response in the Rpv3.1, Rpv10, and Rpv12 resistance mechanism, the phytohormone content of the resistant cultivars Regent, Solaris, and Lela was analyzed during their interaction with the isolates GPN1, GRE1, and GPH1. The analysis was first performed on leaves from the detached leaf assay, at 6, 12, and 24 hpi (Supplementary Figure 4). IAA seemed to accumulate in Regent and Solaris following inoculation with GPN1 and GRE1. However, if hormonal differences between interactions with different isolates existed, they were masked by the large biological variability. Some fluctuations were observed, not only between cultivars interacting with the same isolate but also between isolates interacting with the same cultivar. To reduce the biological variability, the phytohormone content was also measured in leaves from the plant assay at 12 hpi (Supplementary Figure 5). In the end, no obvious trends could be found when comparing the interactions of a cultivar with different isolates across both experiments.
A set of 16 cultivars carrying different Rpv loci was challenged with five P. viticola isolates to assess the durability of some of the commonly used Rpv loci and the influence of stacking and heritage. We were able to distinguish different host phenotypes based on the severity of sporulation. The phenotyping assay was able to efficiently group the cultivars according to their susceptibility to the isolates. The level of necrosis proved to add valuable information to the assay. The specific symptomatologies could be related to the presence of different Rpv loci. However, carriers of the same resistance allele also exhibited slightly varying degrees of resistance, as shown for Rpv3.1. Differences in historic use and genetic background may be the cause of these variations in phenotypes (Sánchez-Mora et al., 2017).
Regarding the isolates obtained from susceptible V. vinifera cultivars (GPN1, GPN2, and FCH1), the resistances derived from the Asian V. amurensis, Rpv12 and Rpv10, were extremely efficient in restricting the sporulation. A steady resistance was observed for Rpv3.1, derived from North-American V. rupestris. However, cultivars carrying Rpv12 and Rpv10 were much more effective in restricting pathogen growth than those carrying Rpv3.1, in accordance with Schwander et al. (2012), Venuti et al. (2013) and Zini et al. (2019). Rpv1, originating from M. rotundifolia, only conferred an intermediate resistance, lower than the degree of resistance provided by Rpv3.1, as reported by Eibach et al. (2007) and Sánchez-Mora et al. (2017). Finally, we observed that Rpv3.2 and Rpv3.3, also derived from North-American Vitis species, were unable to reduce sporulation despite evoking necrosis. Although Rpv3.2 was confirmed to provide a less prominent resistance than Rpv3.1, some studies have found that Rpv3.2 and Rpv3.3 can cause mid-level resistance (Zyprian et al., 2016; Foria et al., 2018; Vezzulli et al., 2019). The observed necrosis indicates that effector-triggered immunity (ETI) was activated in all Rpv3 haplotypes but, under the highly conducive, in vitro conditions, its intensity may have been insufficient to restrict pathogen growth (Foria et al., 2018).
When isolates derived from Rpv3.1 carriers (GPH1 and GRE1) were also taken into account, it became apparent that Rpv12 and Rpv1 provided durable levels of resistance. The Rpv3.1 and Rpv10-mediated resistances, however, appeared to be isolate-specific. Rpv3.1 cultivars showed high susceptibility to GPH1 and GRE1. GPH1 was also able to infect cultivars carrying Rpv10. The sporulation on cultivars not carrying Rpv loci was comparable between all isolates, indicating that all isolates have a similar aggressiveness. This suggests that the isolates GRE1 and GPH1 were not simply selected for a higher aggressiveness, but for a higher virulence particularly on carriers of Rpv3.1 and Rpv10, without apparent fitness costs. While Rpv10 could efficiently restrict the development of GRE1, this QTL did not halt the spread of isolate GPH1. This suggests that the loci Rpv3.1 and Rpv10, single or stacked, might not result in a sustainable resistance. A more durable stacking partner for Rpv3.1 or Rpv10 may be found in Rpv12 or Rpv1 since both QTLs were shown to efficiently inhibit a P. viticola population that breaks through Rpv3-mediated resistance (Calonnec et al., 2013; Venuti et al., 2013).
The heavy sporulation and absence of a hypersensitive response (HR) indicated that isolates GRE1 and GPH1, derived from Rpv3.1+ cultivars, were able to overcome Rpv3.1-mediated resistance. The breakdown of the resistance conferred by Rpv3.1 was first described by Peressotti et al. (2010). The inter-isolate variation in the Rpv3.1 resistance was attributed to a putative mutation in the avirulence factor, through which these isolates escape recognition by the Rpv3.1 locus and fail to trigger HR and resistance on Rpv3.1 carriers (Peressotti et al., 2010). This mutation is suggested to be facilitated by the narrow recognition capabilities of the Rpv3 gene product (Casagrande et al., 2011), allowing P. viticola to adapt in a couple of years (Delmotte et al., 2014). The large spatial scale at which these avrRpv3.1− isolates are found (Delmotte et al., 2014) and the restricted gene flow in P. viticola populations in Europe (Gobbin et al., 2006; Fontaine et al., 2013) suggested that this fast adaptation occurred at multiple sites independently. Moreover, genetic evidence showed these avrRpv3.1− isolates had indeed adapted from the European gene pool and did not segregate into a separate phylogenetic group (Peressotti et al., 2010; Delmotte et al., 2014).
Despite the fast and multiregional adaptation of European P. viticola to Rpv3.1, P. viticola isolates overcoming other Rpv genes in the grapevine-downy mildew interaction have not specifically been reported. However, Li et al. (2015) identified a Chinese P. viticola isolate able to break down the resistance of V. amurensis. We demonstrated that only isolate GPH1 can sporulate heavily on cultivars carrying Rpv10. The idea that specific isolates have gained virulence against Rpv10, is supported by the instability of the Rpv10 resistance of cultivars across studies, using different P. viticola isolates or field mixtures. Although many studies have found Rpv10+ vines to be remarkably resistant to P. viticola (Boso and Kassemeyer, 2008; Vezzulli et al., 2018), other studies do not entirely confirm this foliar resistance (Gómez-Zeledón et al., 2013; Possamai et al., 2020). Moreover, in Cabernet Cortis (Rpv3.3, Rpv10), offspring of Solaris, isolates of P. viticola have shown varying levels of sporulation (Gómez-Zeledón et al., 2013). The behavior of field isolate 1136 described by Gómez-Zeledón et al. (2013), sporulating profusely on Regent and Cabernet Cortis, is phenotypically similar to that of our isolate GPH1.
Interestingly, both GPH1 and 1136 were derived from Rpv3.1+ cultivars. The adaptation to Rpv10 might have been inherited or developed during an earlier encounter with an Rpv10+ cultivar, but it also possible that, in adapting to Rpv3.1, these isolates simultaneously gained virulence on Rpv10+ cultivars (so-called cross-virulence). This is supported by our observation that GPH1 sporulated more profusely on Rpv3.1+ cultivars than on Rpv10+ cultivars. The necrotic response to GPH1 in Solaris was retained, yet this could also be attributed to the Rpv3.3-mediated resistance. Cross-virulence would also imply that the adaptation of GPH1 to overcome Rpv3.1-mediated resistance, is substantially different from the adaptation of GRE1. Gómez-Zeledón et al. (2013) identified an isolate like GRE1, isolate 1137, capable to break through the resistance of Regent (Rpv3.1) but with reduced sporulation on the Rpv10+ cultivar. Field isolate 1136 may be more efficiently adapted to Rpv3.1 compared to isolate 1137, because it sporulated more profusely on V. rupestris (Gómez-Zeledón et al., 2013), the donor of Rpv3.1 (Di Gaspero et al., 2012). After all, the intensity of the Rpv-dependent defense is assumed to be highest in wild-type genetic backgrounds and decreases with an increasing number of backcross generations (Foria et al., 2018).
It is not unlikely that plant pathogens overcoming one resistance source, can gain virulence on another, even when the resistance loci are distinct and the isolates have never been exposed to that resistance source. It is possible that the presence of one QTL might have selected for genetic adaptations linked to genes responsible for the development of virulence on other QTLs. A similar observation of cross-virulence was made by Eisenmann et al. (2019), who suggested Rpv3.1 and Rpv3.2 may recognize the same effector. The most obvious explanation for cross-virulence would be the two QTLs sharing similarities in resistance mechanism. From what is known, there are many similarities between Rpv3.1 and Rpv10-mediated resistance. The early colonization of Rpv3.1+ cultivars (Eisenmann et al., 2019) and Solaris or the Rpv10 wild-type V. amurensis (Boso and Kassemeyer, 2008; Jürges et al., 2009) does not differ from cultivars carrying no major resistance genes. The Rpv3.1-mediated resistance has been traced to a TIR-NB-LRR (Toll/interleukin-1 receptor-nucleotide binding-leucine-rich repeats, also known as TNL) gene pair, which triggers pathogen-induced necrosis when transiently co-expressed (Foria et al., 2020). The necrotic response occurred from 32 hpi (Bellin et al., 2009; Casagrande et al., 2011; Eisenmann et al., 2019; Vezzulli et al., 2019) and hinders the pathogen development in the mesophyll air spaces from 48 hpi (Foria et al., 2018; Eisenmann et al., 2019). Accumulation of peroxidase (Kortekamp et al., 1998) and induction of the biosynthesis of fungitoxic stilbenes (Eisenmann et al., 2019) have been implicated in this resistance. Stilbenoids were also implicated in the Rpv3.3-mediated resistance (Malacarne et al., 2011; Vezzulli et al., 2019). Similarly, the resistance of Solaris has been linked to a necrotic response (Gindro et al., 2003; Boso and Kassemeyer, 2008) and the induction of fungi-toxic stilbenes (Pezet et al., 2004; Alonso-Villaverde et al., 2011), as well as the fast synthesis of callose in infected stomata at 7 hpi (Gindro et al., 2003). Isolate GPH1 may have developed a strategy to circumvent or suppress the Rpv3.1 defense, which might promote its virulence on Rpv10+ cultivars. To examine whether this is an issue of cross-virulence, or rather the independent development of virulence on Rpv3.1 and Rpv10, single Rpv10 carriers and Rpv3.1-breaking isolates without prior exposure to Rpv10+ would be necessary.
Many studies have compared defense responses between susceptible and wild or cultivated resistant grapevine species (Gindro et al., 2003; Kortekamp and Zyprian, 2003; Polesani et al., 2010; Liu et al., 2015; Figueiredo et al., 2017; Lazazzara et al., 2018; Fröbel et al., 2019). The interaction between P. viticola and a grapevine cultivar has not often been described from the perspective of virulent and avirulent strains. The availability of Rpv3.1-breaking isolates allows examining the Rpv3.1 resistance mechanism in more detail. Previous studies have reported that phytohormones play a role in the resistance of the donor species against P. viticola. In response to the successful infection of P. viticola, only small fluctuations in ABA, JA, and SA have been observed in V. vinifera cultivars (Liu et al., 2016; Wang et al., 2018). However, in incompatible interactions between P. viticola and V. amurensis (Liu et al., 2016) or M. rotundifolia (Liu et al., 2016; Wang et al., 2018), SA, JA, and ABA were found to accumulate during the first 12 h following inoculation. The discovery of the resistance-breaking isolate ZJ-1-1 allowed to also describe a compatible interaction in V. amurensis (Li et al., 2015). In the compatible interaction, the fast surges in JA and SA upon pathogen inoculation were no longer observed. However, not much is known about the role of phytohormones in resistant V. vinifera cultivars. It has been suggested that in Regent (Rpv3.1), an incompatible P. viticola interaction triggers an early increase in α-linolenic acid and subsequent biosynthesis of JA, which is conversed to jasmonic acid-isoleucine (JA-Ile) within 12 hpi (Guerreiro et al., 2016). The authors recorded peaks of endogenous JA and SA levels in response to unsuccessful P. viticola infections at 6 and 12 hpi.
To investigate the role of phytohormones in the Rpv3.1, Rpv10, and Rpv12-mediated resistance, we determined the content of ABA, IAA, JA, and SA in grapevine leaves before and during differential interactions. However, we were unable to detect isolate-specific hormonal responses during the interaction of detached leaves or intact plants with Regent, Solaris, or Lela. This is in accordance with Casagrande et al. (2011), who did not observe significant differences in the expression of genes involved in the SA, ET, and JA signaling pathway in Rpv3+/Rpv3− cultivars in response to avrRpv3+/avrRpv3− isolates. The hormonal responses might have been concealed by the large biological variability or by some aborted infections, which often occur in compatible interactions. Moreover, it is possible that sampling the whole leaf diluted the early response beyond detection. On the other hand, it may well be that no significant hormonal responses occurred within these early stages of infection. It is possible that the plant's early defenses were primarily activated by the pathogen-specific signaling transmission pathway, instead of the phytohormone-mediated pathway. There are some indications for the occurrence of a secondary defense mechanism. In V. riparia, a dramatic increase in JA was observed only at 48 hpi (Polesani et al., 2010) and in Rpv12 carriers, genes related to SA synthesis and signaling were only differentially expressed from 48 hpi (Chitarrini et al., 2020). Moreover, the first infection steps of Rpv3.1+ cultivars (Eisenmann et al., 2019) and Solaris (Busam et al., 1997; Boso and Kassemeyer, 2008) are essentially the same as in the susceptible V. vinifera, even though gene expression studies show that the defenses are induced at the first interaction between host cells and pathogen hyphae (Polesani et al., 2010; Eisenmann et al., 2019). The delay and restriction of invasive pathogen growth by the Rpv3.1-mediated defense initiates only from 24 hpi and is effective before 48 hpi (Eisenmann et al., 2019). Therefore, it may be worthwhile to track the hormonal changes over a longer period after inoculation.
While we were unable to establish what hormonal responses are triggered during the resistance mechanism of Regent, Solaris, and Lela, we were able to distinguish some basal hormonal differences between cultivars. The relative contents observed were similar in all three conditions in which P. viticola can easily infect (on adult plants, on detached leaves, and on young plantlets). Given the important influence of these hormones on disease development, the levels of hormones basally present in the resistant cultivars might be associated with their basal resistance. Of the resistant cultivars, Regent tended toward the highest basal levels of ABA. Wang et al. (2018) concluded ABA in particular is an important positive regulator of defense against P. viticola, because ABA was found to regulate stilbene accumulation. Solaris, on the other hand, showed high basal levels of SA and JA. SA and especially JA are hypothesized to be involved in the defense responses against P. viticola (Guerreiro et al., 2016). Moreover, exogenous JA has been shown to protect grapevine leaf disks against P. viticola through callose deposition (Hamiduzzaman et al., 2005). However, the role of the phytohormone pathway in the resistance to P. viticola remains to be elucidated.
The deployment of various combinations of R-loci decreases the chances of the pathogen overcoming the genetic barriers. Pyramiding R-loci is crucial in delaying the erosion of laboriously introgressed resistance loci, yet a higher overall disease resistance is a great bonus. However, estimating disease resistance based on the QTLs is not straightforward. Stacking might result in higher disease resistance, as observed for Rpv12, Rpv1, and Rpv3.1 in 2012-114-0133, resulting in absence of sporulation. For a higher disease resistance, the strength of the resistances is of great importance. For example, we did not observe a clear additive effect in stacking with the weaker QTLs Rpv3.2 or Rpv3.3. Still, the resistance resulting from pyramiding seems to be strongly reliant on the genetic background. Despite the strong resistance provided by both Rpv10 and Rpv3.1, stacking of Rpv10 and Rpv3.1 in 2004-051-0001 resulted in a reduced resistance compared to its parent Solaris (Rpv10 and Rpv3.3). Schwander et al. (2012) did demonstrate an additive effect of Rpv10 and Rpv3.1 on the degree of resistance, but both Rpv3- and Rpv10-dependent responses have been demonstrated to attenuate with backcrossing (Foria et al., 2018; Possamai et al., 2020). Progeny of Solaris carrying only Rpv10 has even been shown to exhibit a disease severity similar to vines not carrying Rpv loci (Possamai et al., 2020). Although the availability of molecular markers facilitates the screening of QTL carriers (Eibach et al., 2007), the presence of the QTLs is insufficient to predict the strength of the disease resistance. A general phenotyping assay using Rpv-breaking isolates would allow easy classification of resistant phenotypes. Most phenotyping studies focus only on the characterization of the susceptibility of European cultivars and resistant cultivars against a field population of P. viticola (Boso and Kassemeyer, 2008; Boso et al., 2014). When performed against a characterized set of isolates, the phenotyping of resistant cultivars would become more informative and would provide sufficient power to discriminate partial and isolate-specific resistance. We observed a large variability between cultivars, not only in sporulation, but also in necrosis. Ideally, the assay would combine a measure for sporulation with necrosis, as proposed by Gómez-Zeledón et al. (2017). The phenotyping assay can also be applied as an empirical method for screening of interesting genetic resources. Seyve Villard 39-639 is currently only known to carry Rpv3.1. However, its strong resistance and isolate-specific susceptibility in our phenotyping assay implies another factor might be influencing the resistance to P. viticola, which was not inherited by Calardis Blanc.
While the host population is relatively well-described, at least in terms of parentage and QTLs, many aspects of the pathogen population remain undisclosed. The existence of different pathotypes could offer an explanation for the strong variability of downy mildew resistance, observed between years (Savary et al., 2009; Calonnec et al., 2013; Vezzulli et al., 2018; Zini et al., 2019) and between studies (Lisek, 2010; Pavloušek, 2012; Vezzulli et al., 2018; Zini et al., 2019). Contrary to our results, for example, the resistance of Lela (Rpv12) was reported only to be mid-level, below Rpv3.1 resistance (Pavloušek, 2012), while Seyval and Regent were found highly resistant (Lisek, 2010; Vezzulli et al., 2018). Especially for partially resistant genotypes, the pathogen strain is of great importance for the resistance outcome (Possamai et al., 2020). However, many resistance studies have been using field mixtures, yearly collected in the field. The determination of pathotypes and the use of genetically homogeneous P. viticola material would allow comparing studies in time and space and future-proofing these results for the dynamic pathogen population. The increasing deployment of Rpv-loci and the ability of P. viticola to recombine each year could facilitate the breakdown of Rpv-mediated resistances.
The diversity of P. viticola has mainly been studied with regards to population studies and fungicide resistance, for which genotyping was facilitated by molecular markers, such as RAPD (Stark-Urnau et al., 2000), microsatellites (Gobbin et al., 2003; Delmotte et al., 2006; Rouxel et al., 2012), AFLP (Scherer and Gisi, 2006) and SNP (Chen et al., 2007; Delmotte et al., 2011). However, these molecular markers have not been designed to describe the pathotypes prevalent in current populations (Gessler et al., 2011). Pathotypes are often outlined by a unique pattern of reactions on different resistant cultivars (Crute and Johnson, 1976). Although described for many other downy mildews, such as Plasmopara halstedii (Trojanová et al., 2017), a uniform bioassay-based differentiation of pathotypes has not yet been established for P. viticola. The perennial nature of grapevine complicates the conduction of this assay and its practical use, since crop rotation in response to the findings is hard. The pathotyping system is also strongly dependent on the use of single isolates, experimental conditions, and fitness of plant and pathogen. Even so, a global characterization system of standardized host genotypes, using a nomenclature system as defined by Limpert and Müller (1994), could be very useful for those interested in P. viticola pathotypes and for plant breeding programs in particular. Moreover, an identification of the prevalent races within a local, regional, or large-scale population using a small set of differential cultivars could be an important advisory tool. Finally, such a system could be paramount to understanding how a pathotype is overcoming the resistance of its host. A pathotyping system and an increased understanding of the pathosystem might be necessary for the reliable, durable control of the pathogen.
To evaluate the durability of some of the commonly used Rpv loci, the influence of stacking, and the virulence diversity of P. viticola, a set of 16 grapevine cultivars, carrying different Rpv loci, was challenged with five P. viticola isolates. The cultivars and isolates were grouped according to the evaluation of the severity of sporulation. The level of necrosis provided additional, valuable information about the activation of the resistance mechanism. To a great extent, the specific symptomatologies could be related to the presence of major Rpv loci. Pyramiding of resistances did not always result in durable or increased disease resistance. More than the presence of QTLs, this phenotyping assay can predict the strength of the host resistance to P. viticola. When performed against a characterized set of isolates, a phenotyping assay based on sporulation and necrosis would provide sufficient power to discriminate partial and isolate-specific resistances in cultivars. However, this requires P. viticola isolates to be characterized. Conversely, using standardized host genotypes, such a bio-assay could also be employed to identify pathotypes of P. viticola. Currently, no uniform characterization system has been established for P. viticola. The determination of pathotypes would allow comparing studies of this pathosystem in time and space. Moreover, the characterization of pathotypes could serve as an advisory tool informing about the prevalent races and help to assess how the host's resistance is overcome. An increased understanding of both members of the pathosystem is necessary for the reliable, durable control of the pathogen.
An efficient resistance was observed for Rpv12, Rpv10, and Rpv3.1 against the isolates obtained from susceptible V. vinifera cultivars. Rpv1 only conferred partial resistance and Rpv3.2 and Rpv3.3 failed to restrict sporulation. The sporulation and necrosis on Rpv3.1+ and Rpv10+ hosts, however, were found to be isolate-specific. The isolates derived from Rpv3.1+ hosts, GRE1 and GPH1, were able to sporulate intensely on cultivars carrying Rpv3.1, without triggering necrosis. Moreover, Rpv10 was not able to efficiently halt the development of the Rpv3.1-breaking isolate GPH1. This isolate could have developed an adaptation to Rpv10, during an earlier encounter with a Rpv10+ cultivar, or might have gained virulence on Rpv10+ cultivars because of its adaptation to Rpv3.1. This suggests that the loci Rpv3 and Rpv10, single of stacked, do not result in the most sustainable resistance. To assess the resistance mechanisms underlying Rpv3.1, Rpv10, and Rpv12 and the infection mechanisms of the different isolates, the hormonal responses in differential interactions were compared. Phytohormones are known to play a role in resistance to P. viticola and might be implicated in the basal resistance of a cultivar against this pathogen. In the defense response, however, the role of phytohormones remains to be elucidated. No significant differences were observed in the levels of ABA, IAA, JA or SA levels in response to the isolates during the first 24 hpi. Nevertheless, this does not preclude their involvement in the defense response.
The knowledge gained in this paper may help breeders assess the durability of resistance in their varieties and to communicate recommendations for effective plant protection measurements to the wine growers. Furthermore, it may help grapevine breeders choose the most suitable parents for their crossings in order to develop new varieties with pyramidized resistances against a broad range of P. viticola isolates.
The original contributions presented in the study are included in Supplementary Table 1. Further inquiries can be directed to the corresponding author.
LH, RH, AK, RT, and MH contributed to the conceptualization and design of the study. Resources were provided by RH, AK, EA, RT, and MH. LH conducted the experiments and formal analysis. LH wrote the first draft of the manuscript, supervised by MH. EA, RT, and MH contributed to the funding acquisition. All authors contributed to manuscript revision, read, and approved the submitted version.
This research was co-financed by the European Union (ERA-NET FACCE SURPLUS), the fund for scientific research-Flanders (FWO grant G0D8615N), BMBF-Germany, and ANR-France in the framework of the collaborative international VITISMART consortium.
The authors declare that the research was conducted in the absence of any commercial or financial relationships that could be construed as a potential conflict of interest.
The Supplementary Material for this article can be found online at: https://www.frontiersin.org/articles/10.3389/fagro.2021.684023/full#supplementary-material
Supplementary Figure 1. Simplified pedigree showing the biological relationship between the different cultivars used in this study (in gray boxes). The cultivars of origin of the P. viticola isolates are indicated with a bold outline. Full and dashed lines mark direct and distant progeny, respectively. For resistant cultivars, the ancestral species that are known to be crossed with V. vinifera are shown on the left. If none are indicated, the cultivar is expected to belong to V. vinifera subsp. vinifera. The presence of the major loci conferring resistance to P. viticola (Rpv) carried by the cultivars used in this study is indicated underneath the corresponding cultivar or species group. The overview of the resistance traits and the species of origin is limited to the information currently available.
Supplementary Figure 2. Overview of the symptoms observed in the phenotyping assay. One representative leaf disk is shown for each cultivar-isolate interaction.
Supplementary Figure 3. Disease severity caused by five different isolates of P. viticola on a set of cultivars. For each interaction, leaf disks were assigned to disease classes ranging from 0 (no sporulation) to 4 (numerous sporangiophores). Different letters indicate significant differences in the interaction between isolates with the same cultivar (Kruskal-Wallis followed by Dunn test; p = 0.05).
Supplementary Figure 4. Course of the levels of ABA, IAA, SA, and JA in detached leaves of the resistant cultivars Regent, Solaris, and Lela during the interaction with P. viticola. Sampling occurred 6, 12, and 24 h after spray-inoculation with water or with the isolates GPN1, GRE1 or GPH1. No significant differences were found in the interaction between isolates with the same cultivar at the same time point (Kruskal-Wallis followed by Dunn test; p = 0.05).
Supplementary Figure 5. Levels of ABA, IAA, SA, and JA in young plantlets of Chardonnay and the resistant cultivars Regent, Solaris, and Lela during the interaction with P. viticola. Sampling occurred 12 h after spray-inoculation with water or with the isolates GPN1, GRE1 or GPH1. Different letters indicate significant differences in the interaction between isolates with the same cultivar (Kruskal-Wallis followed by Dunn test; p = 0.05). When statistical differences are absent, no letters are shown.
Supplementary Table 1. Overview of the data presented in this study.
Ait Barka, E., Nowak, J., and Clément, C. (2006). Enhancement of chilling resistance of inoculated grapevine plantlets with a plant growth-promoting rhizobacterium, Burkholderia phytofirmans strain PsJN. Appl. Environ. Microbiol. 72, 7246–7252. doi: 10.1128/AEM.01047-06
Alonso-Villaverde, V., Voinesco, F., Viret, O., Spring, J., and Gindro, K. (2011). The effectiveness of stilbenes in resistant Vitaceae: ultrastructural and biochemical events during Plasmopara viticola infection process. Plant Physiol. Biochem. 49, 265–274. doi: 10.1016/j.plaphy.2010.12.010
Anderson, R. G., Deb, D., Fedkenheuer, K., and McDowell, J. M. (2015). Recent progress in RXLR effector research. Mol. Plant Microbe Interact. 28, 1063–1072. doi: 10.1094/MPMI-01-15-0022-CR
Bellin, D., Peressotti, E., Merdinoglu, D., Wiedemann-Merdinoglu, S., Adam-Blondon, A.-F., Cipriani, G., et al. (2009). Resistance to Plasmopara viticola in grapevine ‘Bianca’ is controlled by a major dominant gene causing localised necrosis at the infection site. Theor. Appl. Genet. 120, 163–176. doi: 10.1007/s00122-009-1167-2
Blum, M., Waldner, M., and Gisi, U. (2010). A single point mutation in the novel PvCesA3 gene confers resistance to the carboxylic acid amide fungicide mandipropamid in Plasmopara viticola. Fungal Genet. Biol. 47, 499–510. doi: 10.1016/j.fgb.2010.02.009
Boso, S., Alonso-Villaverde, V., Gago, P., Santiago, J. L., and Martínez, M. C. (2014). Susceptibility to downy mildew (Plasmopara viticola) of different Vitis varieties. Crop Prot. 63, 26–35. doi: 10.1016/j.cropro.2014.04.018
Boso, S., and Kassemeyer, H. H. (2008). Different susceptibility of European grapevine cultivars for downy mildew. Vitis 47, 39–49. doi: 10.5073/vitis.2008.47.39-49
Busam, G., Kassemeyer, H. H., and Matern, U. (1997). Differential expression of chitinases in Vitis vinifera L. responding to systemic acquired resistance activators or fungal challenge. Plant Physiol. 115, 1029–1038. doi: 10.1104/pp.115.3.1029
Calonnec, A., Wiedemann-Merdinoglu, S., Delière, L., Cartolaro, P., Schneider, C., and Delmotte, F. (2013). The reliability of leaf bioassays for predicting disease resistance on fruit: a case study on grapevine resistance to downy and powdery mildew. Plant Pathol. 62, 533–544. doi: 10.1111/j.1365-3059.2012.02667.x
Casagrande, K., Falginella, L., Castellarin, S. D., Testolin, R., and Di Gaspero, G. (2011). Defence responses in Rpv3-dependent resistance to grapevine downy mildew. Planta 234, 1097–1109. doi: 10.1007/s00425-011-1461-5
Chen, W., Delmotte, F., Richard-Cervera, S., Douence, L., Greif, C., and Corio-Costet, M.-F. (2007). At least two origins of fungicide resistance in grapevine downy mildew populations. Appl. Environ. Microbiol. 73, 5162–5172. doi: 10.1128/AEM.00507-07
Chitarrini, G., Riccadonna, S., Zulini, L., Vecchione, A., Stefanini, M., Larger, S., et al. (2020). Two-omics data revealed commonalities and differences between Rpv12- and Rpv3-mediated resistance in grapevine. Sci. Rep. 10:12193. doi: 10.1038/s41598-020-69051-6
Crute, I. R., and Johnson, A. G. (1976). The genetic relationship between races of Bremiae lactucae and cultivars of Lactuca sativa. Ann. Appl. Biol. 83, 125–137. doi: 10.1111/j.1744-7348.1976.tb01701.x
Delmotte, F., Chen, W. J., Richard-Cervera, S., Greif, C., Papura, D., Giresse, X., et al. (2006). Microsatellite DNA markers for Plasmopara viticola, the causal agent of downy mildew of grapes. Mol. Ecol. Notes 6, 379–381. doi: 10.1111/j.1471-8286.2005.01240.x
Delmotte, F., Machefer, V., Giresse, X., Richard-Cervera, S., Latorse, M. P., and Beffa, R. (2011). Characterization of single-nucleotide-polymorphism markers for Plasmopara viticola, the causal agent of grapevine downy mildew. Appl. Environ. Microbiol. 77, 7861–7863. doi: 10.1128/AEM.05782-11
Delmotte, F., Mestre, P., Schneider, C., Kassemeyer, H.-H., Kozma, P., Richart-Cervera, S., et al. (2014). Rapid and multiregional adaptation to host partial resistance in a plant pathogenic oomycete: evidence from European populations of Plasmopara viticola, the causal agent of grapevine downy mildew. Infect. Genet. Evol. 27, 500–508. doi: 10.1016/j.meegid.2013.10.017
Di Gaspero, G., Copetti, D., Coleman, C., Castellarin, S. D., Eibach, R., Kozma, P., et al. (2012). Selective sweep at the Rpv3 locus during grapevine breeding for downy mildew resistance. Theor. Appl. Genet. 124, 277–286. doi: 10.1007/s00122-011-1703-8
Eibach, R., Zyprian, E., Welter, L., and Töpfer, R. (2007). The use of molecular markers for pyramiding resistance genes in grapevine breeding. Vitis 46, 120–124. doi: 10.17660/ActaHortic.2009.827.96
Eisenmann, B., Czemmel, S., Ziegler, T., Buchholz, G., Kortekamp, A., Trapp, O., et al. (2019). Rpv3-1 mediated resistance to grapevine downy mildew is associated with specific host transcriptional responses and the accumulation of stilbenes. BMC Plant Biol. 19:343. doi: 10.1186/s12870-019-1935-3
Feechan, A., Anderson, C., Torregrosa, L., Jermakow, A., Mestre, P., Wiedemann-Merdinoglu, S., et al. (2013). Genetic dissection of a TIR-NB-LRR locus from the wild North American grapevine species Muscadinia rotundifolia identifies paralogous genes conferring resistance to major fungal and oomycete pathogens in cultivated grapevine. Plant J. 76, 661–674. doi: 10.1111/tpj.12327
Figueiredo, A., Martins, J., Sebastiana, M., Guerreiro, A., Silva, A., Rita, A., et al. (2017). Specific adjustments in grapevine leaf proteome discriminating resistant and susceptible grapevine genotypes to Plasmopara viticola. J. Proteomics 152, 48–57. doi: 10.1016/j.jprot.2016.10.012
Fischer, B. M., Salakhutdinov, I., Akkurt, M., Eibach, R., Edwards, K. J., Töpfer, R., et al. (2004). Quantitative trait locus analysis of fungal disease resistance factors on a molecular map of grapevine. Theor. Appl. Genet. 108, 501–515. doi: 10.1007/s00122-003-1445-3
Fontaine, M. C., Austerlitz, F., Giraud, T., Labbé, F., Papura, D., Richard-Cervera, S., et al. (2013). Genetic signature of a range expansion and leap-frog event after the recent invasion of Europe by the grapevine downy mildew pathogen Plasmopara viticola. Mol. Ecol. 22, 2771–2786. doi: 10.1111/mec.12293
Foria, S., Copetti, D., Eisenmann, B., Magris, G., Vidotto, M., Scalabrin, S., et al. (2020). Gene duplication and transposition of mobile elements drive evolution of the Rpv3 resistance locus in grapevine. Plant J. 101, 529–542. doi: 10.1111/tpj.14551
Foria, S., Magris, G., Morgante, M., and Di Gaspero, G. (2018). The genetic background modulates the intensity of Rpv3-dependent downy mildew resistance in grapevine. Plant Breed. 137, 220–228. doi: 10.1111/pbr.12564
Fröbel, S., Dudenhöffer, J., Töpfer, R., and Zyprian, E. (2019). Transcriptome analysis of early downy mildew (Plasmopara viticola) defense in grapevines carrying the Asian resistance locus Rpv10. Euphytica 215:28. doi: 10.1007/s10681-019-2355-z
Genet, J.-L., and Jaworska, G. (2013). Characterization of European Plasmopara viticola isolates with reduced sensitivity to cymoxanil. Eur. J. Plant Pathol. 135, 383–393. doi: 10.1007/s10658-012-0094-2
Gessler, C., Pertot, I., and Perazzolli, M. (2011). Plasmopara viticola: a review of knowledge on downy mildew of grapevine and effective disease management. Phytopathol. Mediterr. 50, 3–44. doi: 10.14601/Phytopathol_Mediterr-9360
Gindro, K., Pezet, R., and Viret, O. (2003). Histological study of the responses of two Vitis vinifera cultivars (resistant and susceptible) to Plasmopara viticola infections. Plant Physiol. Biochem. 41, 846–853. doi: 10.1016/S0981-9428(03)00124-4
Gisi, U., Waldner, M., Kraus, N., Dubuis, P. H., and Sierotzki, H. (2007). Inheritance of resistance to carboxylic acid amide (CAA) fungicides in Plasmopara viticola. Plant Pathol. 56, 199–208. doi: 10.1111/j.1365-3059.2006.01512.x
Gobbin, D., Pertot, I., and Gessler, C. (2003). Identification of microsatellite markers for Plasmopara viticola and establishment of high throughput method for SSR analysis. Eur. J. Plant Pathol. 109, 153–164. doi: 10.1023/A:1022565405974
Gobbin, D., Rumbou, A., Linde, C. C., and Gessler, C. (2006). Population genetic structure of Plasmopara viticola after 125 years of colonization in European vineyards. Mol. Plant Pathol. 7, 519–531. doi: 10.1111/j.1364-3703.2006.00357.x
Gómez-Zeledón, J., Kaiser, M., and Spring, O. (2016). An extended leaf disc test for virulence assessment in Plasmopara viticola and detection of downy mildew resistance in Vitis. J. Plant Pathol. Microbiol. 7:5. doi: 10.4172/2157-7471.1000353
Gómez-Zeledón, J., Kaiser, M., and Spring, O. (2017). Exploring host-pathogen combinations for compatible and incompatible reactions in grapevine downy mildew. Eur. J. Plant Pathol. 149, 1–10. doi: 10.1007/s10658-017-1156-2
Gómez-Zeledón, J., and Spring, O. (2018). Up-regulated RxLR effector genes of Plasmopara viticola in synchronized host-free stages and infected leaves of hosts with different susceptibility. Fungal Biol. 122, 1125–1133. doi: 10.1016/j.funbio.2018.07.006
Gómez-Zeledón, J., Zipper, R., and Spring, O. (2013). Assessment of phenotypic diversity of Plasmopara viticola on Vitis genotypes with different resistance. Crop Prot. 54, 221–228. doi: 10.1016/j.cropro.2013.08.015
Gu, Z., Eils, R., and Schlesner, M. (2016). Complex heatmaps reveal patterns and correlations in multidimensional genomic data. Bioinformatics 32, 2847–2849. doi: 10.1093/bioinformatics/btw313
Guerreiro, A., Figueiredo, J., Sousa Silva, M., and Figueiredo, A. (2016). linking jasmonic acid to grapevine resistance against the biotrophic oomycete Plasmopara viticola. Front. Plant Sci. 7:565. doi: 10.3389/fpls.2016.00565
Haeck, A., Van Langenhove, H., Harinck, L., Kyndt, T., Gheysen, G., Höfte, M., et al. (2018). Trace analysis of multi-class phytohormones in Oryza sativa using different scan modes in high-resolution Orbitrap mass spectrometry: method validation, concentration levels, and screening in multiple accessions. Anal. Bioanal. Chem. 410, 4527–4539. doi: 10.1007/s00216-018-1112-9
Hamiduzzaman, M. M., Jakab, G., Barnavon, L., Neuhaus, J. M., and Mauch-Mani, B. (2005). β -aminobutyric acid-induced resistance against downy mildew in grapevine acts through the potentiation of callose formation and jasmonic acid signaling. Mol. Plant Microbe Interact. 18, 819–829. doi: 10.1094/MPMI-18-0819
Heyman, L., Chrysargyris, A., Demeestere, K., Tzortzakis, N., and Höfte, M. (2021). Responses to drought stress modulate the susceptibility to Plasmopara viticola in Vitis vinifera self-rooted cuttings. Plants 10:273. doi: 10.3390/plants10020273
Johnson, R. (1984). A critical analysis of durable resistance. Annu. Rev. Phytopathol. 22, 309–330. doi: 10.1146/annurev.py.22.090184.001521
Jürges, G., Kassemeyer, H.-H., Dürrenberger, M., Düggelin, M., and Nick, P. (2009). The mode of interaction between Vitis and Plasmopara viticola Berk. & Curt. Ex de Bary depends on the host species. Plant Biol. 11, 886–898. doi: 10.1111/j.1438-8677.2008.00182.x
Kamoun, S., Furzer, O., Jones, J. D. G., Judelson, H. S., Ali, G. S., Dalio, R. J. D., et al. (2015). The top 10 oomycete pathogens in molecular plant pathology. Mol. Plant Pathol. 16, 413–434. doi: 10.1111/mpp.12190
Kast, W. K., Stark-Urnau, M., Seidel, M., and Gemmrich, A. R. (2000). Inter-isolate variation of virulence of Plasmopara viticola on resistant vine varieties. Mitteilungen Klosterneuburg Rebe Wein Obs. Früchteverwertung 5, 38–42.
Kortekamp, A., Wind, R., and Zyprian, E. (1998). Investigation of the interaction of Plasmopara viticola with susceptible and resistant grapevine cultivars. Zeitschrift fur Pflanzenkrankheiten und Pflanzenschutz 105, 475–488.
Kortekamp, A., and Zyprian, E. (2003). Characterization of Plasmopara-resistance in grapevine using in vitro plants. J. Plant Physiol. 160, 1393–1400. doi: 10.1078/0176-1617-01021
Lazazzara, V., Bueschl, C., Parich, A., Pertot, I., Schuhmacher, R., and Perazzolli, M. (2018). Downy mildew symptoms on grapevines can be reduced by volatile organic compounds of resistant genotypes. Sci. Rep. 8:1618. doi: 10.1038/s41598-018-19776-2
Le Comité Champagne (2020). The Economy of Champagne: Key Market Statistics 2019. Available online at: https://www.champagne.fr/en/champagne-economy/key-market-statistics (accessed January 5, 2021).
Li, X., Wu, J., Yin, L., Zhang, Y., Qu, J., and Lu, J. (2015). Comparative transcriptome analysis reveals defense-related genes and pathways against downy mildew in Vitis amurensis grapevine. Plant Physiol. Biochem. 95, 1–14. doi: 10.1016/j.plaphy.2015.06.016
Limpert, E., and Müller, K. (1994). Designation of pathotypes of plant pathogens. J. Phytopathol. 140, 346–358. doi: 10.1111/j.1439-0434.1994.tb00617.x
Lisek, J. (2010). Yielding and healthiness of selected grape cultivars for processing in central Poland. J. Fruit Ornam. Plant Res. 18, 265–272. Available online at: http://www.inhort.pl/files/journal_pdf/journal_2010_2/full26%202010(2).pdf
Liu, R., Wang, L., Zhu, J., Chen, T., Wang, Y., and Xu, Y. (2015). Histological responses to downy mildew in resistant and susceptible grapevines. Protoplasma 252, 259–270. doi: 10.1007/s00709-014-0677-1
Liu, S., Wu, J., Zhang, P., Hasi, G., Huang, Y., Lu, J., et al. (2016). Response of phytohormones and correlation of SAR signal pathway genes to the different resistance levels of grapevine against Plasmopara viticola infection. Plant Physiol. Biochem. 107, 56–66. doi: 10.1016/j.plaphy.2016.05.020
Malacarne, G., Vrhovsek, U., Zulini, L., Cestaro, A., Stefanini, M., Mattivi, F., et al. (2011). Resistance to Plasmopara viticola in a grapevine segregating population is associated with stilbenoid accumulation and with specific host transcriptional responses. BMC Plant Biol. 11:114. doi: 10.1186/1471-2229-11-114
Martin, C., Vernoy, R., Carr,é, M., Vesselle, G., Collas, A., Bougerey, C., et al. (1987). The vine and techniques of in vitro cultivation. Bull. l'O.I.V. 675–676, 447–458.
Maul, E., Töpfer, R., Röckel, F., Brühl, U., Hundemer, M., Mahler-Ries, A., et al. (2020). Vitis International Variety Catalogue. Available online at: www.vivc.de (accessed January 5, 2021).
Merdinoglu, D., Wiedeman-Merdinoglu, S., Coste, P., Dumas, V., Haetty, S., Butterlin, G., et al. (2003). Genetic analysis of downy mildew resistance derived from Muscadinia rotundifolia. Acta Hortic. 603, 451–456. doi: 10.17660/ActaHortic.2003.603.57
Pavloušek, P. (2012). Evaluation of foliar resistance of grapevine genetic resources to downy mildew (Plasmopara viticola). Acta Univ. Agric. Silvic. Mendelianae Brun. 60, 191–198. doi: 10.11118/actaun201260080191
Peressotti, E., Wiedemann-Merdinoglu, S., Delmotte, F., Bellin, D., Di Gaspero, G., Testolin, R., et al. (2010). Breakdown of resistance to grapevine downy mildew upon limited deployment of a resistant variety. BMC Plant Biol. 10:147. doi: 10.1186/1471-2229-10-147
Pezet, R., Gindro, K., Viret, O., and Spring, J.-L. (2004). Glycosylation and oxidative dimerization of resveratrol are respectively associated to sensitivity and resistance of grapevine cultivars to downy mildew. Physiol. Mol. Plant Pathol. 65, 297–303. doi: 10.1016/j.pmpp.2005.03.002
Polesani, M., Bortesi, L., Ferrarini, A., Zamboni, A., Fasoli, M., Zadra, C., et al. (2010). General and species-specific transcriptional responses to downy mildew infection in a susceptible (Vitis vinifera) and a resistant (V. riparia) grapevine species. BMC Genomics 11:117. doi: 10.1186/1471-2164-11-117
Possamai, T., Migliaro, D., Gardiman, M., Velasco, R., and De Nardi, B. (2020). Rpv mediated defense responses in grapevine offspring resistant to Plasmopara viticola. Plants 9:781. doi: 10.3390/plants9060781
R Core Team (2019). R: A Language and Environment for Statistical Computing. Vienna, Austria: R Found. Stat. Comput. Available online at: https://www.r-project.org/
Rouxel, M., Papura, D., Nogueira, M., Machefer, V., Dezette, D., Richard-Cervera, S., et al. (2012). Microsatellite markers for characterization of native and introduced populations of Plasmopara viticola, the causal agent of grapevine downy mildew. Appl. Environ. Microbiol. 78, 6337–6340. doi: 10.1128/AEM.01255-12
Sánchez-Mora, F. D., Saifert, L., Zanghelini, J., Assumpção, W. T., Guginski-Piva, C. A., Giacometti, R., et al. (2017). Behavior of grape breeding lines with distinct resistance alleles to downy mildew (Plasmopara viticola). Crop Breed. Appl. Biotechnol. 17, 141–149. doi: 10.1590/1984-70332017v17n2a21
Savary, S., Delbac, L., Rochas, A., Taisant, G., and Willocquet, L. (2009). Analysis of nonlinear relationships in dual epidemics, and its application to the management of grapevine downy and powdery mildews. Phytopathology 99, 930–942. doi: 10.1094/PHYTO-99-8-0930
Scherer, E., and Gisi, U. (2006). Characterization of genotype and mating type in european isolates of Plasmopara viticola. J. Phytopathol. 154, 489–495. doi: 10.1111/j.1439-0434.2006.01136.x
Schwander, F., Eibach, R., Fechter, I., Hausmann, L., Zyprian, E., and Töpfer, R. (2012). Rpv10: a new locus from the Asian Vitis gene pool for pyramiding downy mildew resistance loci in grapevine. Theor. Appl. Genet. 124, 163–176. doi: 10.1007/s00122-011-1695-4
Stark-Urnau, M., Seidel, M., Kast, W. K., and Gemrich, A. R. (2000). Studies on the genetic diversity of primary and secondary infections of Plasmopara viticola using RAPD/PCR. Vitis 39, 163–166. doi: 10.5073/vitis.2000.39.163-166
Statistisches Landesamt Rheinland-Pfalz (2020). Bestockte Rebfläche der Keltertrauben 1989 bis 2019 nach ausgewählten Rebsorten und Anbaugebieten. Landwirtschaft: Zeitreihen regional. Available online at: https://www.statistik.rlp.de/de/wirtschaftsbereiche/landwirtschaft/zeitreihen-regional (accessed January 5, 2021).
Töpfer, R., Hausmann, L., Harst, M., Maul, E., Zyprian, E., and Eibach, R. (2011). New horizons for grapevine breeding. Fruit Veg. Cereal Sci. Biotechnol. 5, 79–100.
Trojanová, Z., Sedlárov,á, M., Gulya, T. J., and Lebeda, A. (2017). Methodology of virulence screening and race characterization of Plasmopara halstedii, and resistance evaluation in sunflower – a review. Plant Pathol. 66, 171–185. doi: 10.1111/ppa.12593
van Heerden, C. J., Burger, P., Vermeulen, A., and Prins, R. (2014). Detection of downy and powdery mildew resistance QTL in a ‘Regent’ × ‘RedGlobe’ population. Euphytica 200, 281–295. doi: 10.1007/s10681-014-1167-4
Venuti, S., Copetti, D., Foria, S., Falginella, L., Hoffmann, S., Bellin, D., et al. (2013). Historical introgression of the downy mildew resistance gene Rpv12 from the Asian species Vitis amurensis into grapevine varieties. PLoS ONE 8:e61228. doi: 10.1371/journal.pone.0061228
Vezzulli, S., Malacarne, G., Masuero, D., Vecchione, A., Dolzani, C., Goremykin, V., et al. (2019). The Rpv3-3 haplotype and stilbenoid induction mediate downy mildew resistance in a grapevine interspecific population. Front. Plant Sci. 10:234. doi: 10.3389/fpls.2019.00234
Vezzulli, S., Vecchione, A., Stefanini, M., and Zulini, L. (2018). Downy mildew resistance evaluation in 28 grapevine hybrids promising for breeding programs in Trentino region (Italy). Eur. J. Plant Pathol. 150, 485–495. doi: 10.1007/s10658-017-1298-2
Wang, C., Wu, J., Zhang, Y., and Lu, J. (2018). Muscadinia rotundifolia ‘Noble’ defense response to Plasmopara viticola inoculation by inducing phytohormone-mediated stilbene accumulation. Protoplasma 255, 95–107. doi: 10.1007/s00709-017-1118-8
Welter, L. J., Göktürk-Baydar, N., Akkurt, M., Maul, E., Eibach, R., Töpfer, R., et al. (2007). Genetic mapping and localization of quantitative trait loci affecting fungal disease resistance and leaf morphology in grapevine (Vitis vinifera L). Mol. Breed. 20, 359–374. doi: 10.1007/s11032-007-9097-7
Zini, E., Dolzani, C., Stefanini, M., Gratl, V., Bettinelli, P., Nicolini, D., et al. (2019). R-loci arrangement versus downy and powdery mildew resistance level: a Vitis hybrid survey. Int. J. Mol. Sci. 20:3526. doi: 10.3390/ijms20143526
Keywords: Rpv, downy mildew, resistance genes, Vitis vinifera, characterization, phenotyping, virulence, breakdown
Citation: Heyman L, Höfle R, Kicherer A, Trapp O, Ait Barka E, Töpfer R and Höfte M (2021) The Durability of Quantitative Host Resistance and Variability in Pathogen Virulence in the Interaction Between European Grapevine Cultivars and Plasmopara viticola. Front. Agron. 3:684023. doi: 10.3389/fagro.2021.684023
Received: 22 March 2021; Accepted: 18 May 2021;
Published: 11 June 2021.
Edited by:
Anne-Katrin Mahlein, Institut für Zuckerrübenforschung (IfZ), GermanyReviewed by:
Joana Figueiredo, University of Lisbon, PortugalCopyright © 2021 Heyman, Höfle, Kicherer, Trapp, Ait Barka, Töpfer and Höfte. This is an open-access article distributed under the terms of the Creative Commons Attribution License (CC BY). The use, distribution or reproduction in other forums is permitted, provided the original author(s) and the copyright owner(s) are credited and that the original publication in this journal is cited, in accordance with accepted academic practice. No use, distribution or reproduction is permitted which does not comply with these terms.
*Correspondence: Monica Höfte, TW9uaWNhLkhvZnRlQFVHZW50LmJl
Disclaimer: All claims expressed in this article are solely those of the authors and do not necessarily represent those of their affiliated organizations, or those of the publisher, the editors and the reviewers. Any product that may be evaluated in this article or claim that may be made by its manufacturer is not guaranteed or endorsed by the publisher.
Research integrity at Frontiers
Learn more about the work of our research integrity team to safeguard the quality of each article we publish.