- 1Laboratory of Evolutive Entomology, Institute of Biology, University of Neuchâtel, Neuchâtel, Switzerland
- 2Laboratory of Entomology, Wageningen University, Wageningen, Netherlands
- 3Laboratory of Fundamental and Applied Research in Chemical Ecology, University of Neuchâtel, Neuchâtel, Switzerland
- 4Neuchâtel Platform of Analytical Chemistry, University of Neuchâtel, Neuchâtel, Switzerland
Plant domestication often reduces plant defenses by selection on chemical and physical defense traits. Thus, it is expected that herbivorous insects perform better on crop varieties than on their wild relatives. However, recent studies show that this pattern is not ubiquitous. We examined how varieties of squash (Cucurbita spp.) domesticated for different purposes (for consumption or as ornamentals), differ in plant defense traits and in their interactions with a leaf and a root herbivore. Two types of defenses were measured: cucurbitacins, which are toxic phytochemicals, and trichomes that are physical barriers for most herbivores. We addressed the following questions: (i) what is the variation in cucurbitacin content and leaf trichome density among varieties? (ii) does purpose of domestication explain differences in defense traits among varieties? and (iii) are herbivore feeding preferences and performance altered by the defense traits of squash varieties? We found great variation in cucurbitacin content among varieties, but not according to their purpose of domestication. Cucurbitacins were found mostly in cotyledons and roots and in very small quantities in the leaves. In contrast, trichome density was greater on the varieties selected for consumption than on the ornamental varieties. The performance of a leaf herbivore (Spodoptera latifascia) and a root herbivore (Diabrotica balteata), was not different among squash varieties. Moreover, in a choice experiment, larvae of the root herbivore preferred to feed on squash varieties with high cucurbitacin content. Whereas, in the field, native leaf herbivores preferred to feed on varieties selected for consumption. Our results contribute to a better understanding on how varietal selection may differentially affect plant defenses. This knowledge could help in the development of crop varieties with enhanced insect resistance.
Introduction
Plant domestication is an evolutionary process whereby wild plants are modified to meet human needs (Meyer and Purugganan, 2013; Cornille et al., 2014). The suite of morphological and physiological traits that distinguish crops from their wild ancestors is known as the domestication syndrome (Harlan and De Wet, 1972; Koinange et al., 1996). Domestication traits are common in many crop species and generally include those that facilitate cultivation and harvesting, as well as other desirable traits, such as an increase in fruit size, yield, number of seeds, or plant growth (Wink, 1988; Evans, 1993; Meyer et al., 2012).
A study comparing 203 crops from 68 families showed that in 66% of these crops, the most common trait altered by domestication was the plant's secondary chemistry (loss or reduction of toxic or bitter compounds), followed by changes in plant morphology (e.g., aerial vegetative part or fruit size) (Chen et al., 2015). In recent years, there has been a surge in research examining how these domesticated traits have affected the interactions between crop plants and their associated herbivores. Overall, these studies reveal that often a decrease in chemical and physical plant defense results in increased insect performance (Idris and Grafius, 1996; Benrey et al., 1998; Gols et al., 2008). However, these patterns are not ubiquitous. For example, Turcotte et al. (2014) compared the performance of a leaf chewing herbivore (Spodoptera exigua) and a phloem-feeding aphid (Myzus persicae) on 29 pairs of crop plants and their wild relatives. They found that while domestication reduced the levels of morphological and chemical defenses in most of the examined crops, it did not always result in increased insect performance. In another study, Shlichta et al. (2018) found that in lima bean, Phaseolus lunatus, domestication has reduced the content of cyanogenic glycosides in seeds but not in leaves. Accordingly, the performance of the seed beetle Zabrotes subfasciatus, was better on seeds of domesticated varieties than on seeds of wild plants, whereas the performance of the generalist Spodoptera exigua, was not affected by the levels of cyanogenic glycosides (Shlichta et al., 2018). The authors argue that the trade-off between lower levels of defense and increased insect performance is supported for the seeds because this is the organ targeted by the domestication process.
Several other factors might be important to consider regarding the trade-off between decreased plant defense and increased insect performance. These include factors associated with the plant, the insect, or their interaction. For example, patterns of plant resistance in crops may vary across locations, degree of domestication and the organ or life stage attacked by the herbivore (Newell-McGloughlin, 2008; Chen et al., 2017). Crop domestication can also have a strong impact on the plant's nutritional content (Newell-McGloughlin, 2008; Delgado-Baquerizo et al., 2016; Gaillard et al., 2018) and insects may preferentially select and perform better on domesticated crops because of their higher nutritional quality, which may not always correlate with a decrease in defense traits (Tallamy et al., 1998). From the insect's side, feeding mode, host range, and/or level of specialization are important factors that can also influence their performance (Chen et al., 2015; Whitehead et al., 2017).
One factor that has been neglected in studies that examine the relationship between plant domestication and resistance to herbivores is the crop's purpose of domestication. This refers to the particular use for which a crop or a specific variety were domesticated, for example, for human or livestock consumption, fiber, as ornamental or medicinal use, and various other purposes depending on the type of crop. A large number of our food plants were originally selected for purposes other than food consumption (Meyer et al., 2012). For example, carrots were first domesticated for their seeds that were used as both spice and medicine (Simon, 2000) and saffron was used as a body paint, dye and perfume (Mousavi and Bathaie, 2011). Meyer et al. (2012) hypothesized that shifts in the primary used organs of a crop would cause changes in other organs, and further, that there would be a correlation between some organs used for food and non-food uses of other organs. In such cases, changes in chemical and physical defenses in a crop compared to its wild ancestor may be the result of the original desire for selection and not the current use of that crop.
Squash, Cucurbita spp. represents a fascinating system to study the implications of domestication for ecological interactions (Lira et al., 2016). With 21 taxa, the genus has experienced independent domestication events in five species: C. pepo, C. ficifolia, C. moschata, C. maxima, and C. argyrosperma (Sanjur et al., 2002). Each domestication event occurred independently, sometimes on more than one occasion (Lira et al., 2016). Each variety was selected for specific traits, defined by the nutritional and cultural needs of early human populations in America (Zizumbo-Villarreal et al., 2010, 2012). Even though each species underwent a unique selection regime to meet local conditions and preferences, many domestication traits are common. One of the main traits altered with domestication is the content of a class of secondary metabolites called cucurbitacins. These molecules are oxygenated tetracyclic triterpenes, extremely bitter that render plants toxic or unpalatable to many invertebrates and vertebrate herbivores, including humans (Da Costa and Jones, 1971; Ferguson and Metcalf, 1985). However, cucurbitacins can also serve as feeding attractants for a number of specialized phytophagous beetles belonging to the Chrysomelidae family (tribe Luperini, Old World Aulacophora) (Chambliss and Jones, 1966; Castellanos-Morales et al., 2018). The second trait modified during domestication is the density of trichomes (Paris, 2016; Chomicki et al., 2020). Trichomes can develop on the surface of all plant organs and can be “glandular” or “non-glandular.” Non-glandular trichomes can serve as a physical defense against herbivores by interfering with their movement, causing physical entrapment or in some cases inflicting injury (Ashraf et al., 2018; Kaur and Kariyat, 2020). Whereas, glandular trichomes can synthesize and secrete toxic chemicals (Dimock et al., 1982; Wagner, 1991; Kang et al., 2010a; Mitchell et al., 2016). Indeed, high densities of foliar trichomes can increase plant resistance against herbivores (Tian et al., 2012; Vermeij, 2015).
Although detailed studies exist on the history of domestication of Cucurbita (Nee, 1990; Nigh and Diemont, 2013; Lira et al., 2016; Chomicki et al., 2020), the extent to which squash varieties selected for different purposes differ in physical and chemical traits associated with defense and affect the interaction with herbivores, is not yet known. In this study we examined how variation in defense traits in several squash varieties affects the interaction with two generalist herbivores, and whether plant resistance against these herbivores can be explained by the variety's purpose of domestication. To do this, we used several varieties of squash from the Cucurbita genus and investigated the relationship between levels of chemical (cucurbitacins) and physical defenses (trichomes), and the preference and performance of a leaf and a root herbivore. We addressed the following questions: (i) what is the level of variation in cucurbitacin content and leaf trichome density among varieties? (ii) does purpose of domestication (consumption vs. ornamental) explain differences in defense traits among varieties? and (iii) are herbivores feeding preferences and performance altered by defense traits of squash varieties? Therefore, we quantified the content of cucurbitacins in roots, cotyledons and leaves, and density of trichomes in leaves and cotyledons of several squash varieties. Then, we conducted performance bioassays with two generalist herbivores, a leaf-chewing caterpillar (Spodoptera latifascia) and a root-feeding beetle (Diabrotica balteata). As larvae of D. balteata can move freely in the soil where the adult female lays its eggs, we also conducted choice experiments to test whether beetle larvae showed differential preferences for squash varieties. Finally, we carried out a common garden experiment in Mexico, the place of origin of squash and quantified infestation and damage from native generalist and specialist herbivores.
Materials and Methods
Plants
We selected squash varieties across different species of Cucurbita within the same clade and with known wild ancestors (Castellanos-Morales et al., 2018) (Supplementary Figure 1). This clade includes Cucurbita varieties of the species pepo, maxima, ficifolia, and argyrosperma. Seeds were purchased from KCB-Samen (GmbH, Bottmingen, Switzerland) and Zollinger (biolgische Samengartenerei, Les Evouettes, Switzerland). In total, we used nine varieties selected for two different purposes (Table 1). Four varieties were domesticated for consumption, either of their fruits [Chilacayote (Fcf), Silver Edge (Fbg), or their seeds, Seed oil pumpkin (Scpo) and Lady Godiva (Slg)] and three varieties domesticated for ornamental use [Atlantic Giant (Oag), Griemlins (Ocp), and Turkish Turban (Ott)]. For the field experiment, two of the consumption varieties (Scpo and Slg) were replaced by two hybrid varieties, also used for consumption, collected in Mexico, Hsc and White Fall F1 (Hwf). For the laboratory experiments, seeds of each variety were individually germinated in 8-cm-diameter plastic pots filled with soil (Sinntal-Altengronau, Germany) mixed with 50% sand. Fifteen-day-old plants with cotyledons and two developed leaves were used for all experiments. Plants were grown under controlled conditions in a greenhouse (24 ± 5°C, 16:8 h L: D) and watered every other day. For the experiments with S. latifascia on leaves and D. balteata on roots, all the afore-mentioned varieties were used. For the experiments with S. latifascia on cotyledons the variety Slg was not used due to low germination rate at the time of the experiment.
Insects
Spodoptera latifascia, Walker, 1856 (Lepidoptera: Noctuidae), commonly known as the velvet armyworm, is a polyphagous insect whose caterpillars have been reported to feed on, and cause economic damage to cowpea, soybean, cotton and many other crops (Habib et al., 1982; Portillo et al., 1996). In our field site in Mexico, caterpillars are often found feeding on cultivated squash (Traine et al., 2020). Female moths lay their eggs in batches on the leaf surface and upon hatching, caterpillars feed gregariously before dispersing and individually feeding on the leaves throughout their later developmental stages. Colonies of S. latifascia were established in the lab in Switzerland from caterpillars collected mainly from wild bean or cultivated maize plants in a field site located at the experimental campus of the Universidad del Mar in Puerto Escondido, Oaxaca, Mexico (15°55′27.9″ N 97°09′04.3″ W). Caterpillars were reared on artificial diet (“beet armyworm diet,” Bio-Serv, Flemington, NJ, USA) (Cuny et al., 2018).
The banded cucumber beetle, Diabrotica balteata LeConte, 1865 (Coleoptera; Chrysomelidae), originates from the tropical Americas (Teng et al., 1984; Moreira et al., 2015). In this region it has been associated with wild and domesticated squash for thousands of years (Metcalf, 1979; Metcalf and Lampman, 1989). This species is a pest of several agricultural crops including cucurbits, beans and sweet potatoes (Pitre Jr and Kantack, 1962). Larvae feed on roots and tubers and adults eat leaves, cotyledons and flowers (Pitre Jr and Kantack, 1962). Eggs of D. balteata were obtained from Syngenta (Stein, Switzerland). Upon hatching, larvae were reared on maize roots (hybrid DFI 45321, DSP, Delley, Switzerland) until second instar when they were used for experiments. Both insect rearings were kept under quarantine conditions at the University of Neuchatel, Switzerland (25 ± 2°C, 16:8 h L: D and 60% RH ± 5%).
Adult beetles of the genus Acalymma (Chrysomelidae: Galerucinae) frequently visited our squash plants during the field experiments in Mexico. The genus Acalymma mainly occurs in the New World (Cabrera and Durante, 2001; Capinera, 2001), but it is mostly distributed in the tropical regions of Mexico (Smith, 1966). Some species are considered oligophagous (they feed on several species of Cucurbitaceae as adults and larvae) (Eben et al., 1997a), and major pests of cucurbits. Adults feed on pollen, leaves and flowers and larvae are root feeders (Eben et al., 1997a,b).
Plant Defense Measurements
Cucurbitacin Content
We measured cucurbitacin content in the plant tissues that are readily eaten by the two herbivore species. Leaves, roots and cotyledons of 2-week-old Cucurbita spp. were ground separately into powder in liquid nitrogen. We weighed 100 mg of powder on a microbalance to the nearest 0.1 mg (Mettler Toledo XP6, Columbus, Ohio, USA), mixed it with 1 ml of methanol (99.999%) (Kaushik et al., 2015) and added five glass beads in a 1.5 mL Eppendorf tube. Samples were placed in Retsch tissue lyser (Quiagen, Hilden, Germany) at 30 Hz for 4 min, then centrifuged during 5 min at 14,000 rpm, and 700 μl of supernatant were removed and diluted with 300 μl of water. The cucurbitacin analyses were performed by UHPLC-QTOFMS using an Acquity UPLC™ coupled to Synapt G2 high-resolution mass spectrometer (Waters, Milford, USA) and an Acquity UPLC BEH C18 1.7 μm, 2.1 × 50 mm (Waters) column. Two mobile phases were used in gradient mode; water plus 0.05% of formic acid and acetonitrile plus 0.05% of formic acid. The injection volume in the machine was 2.5 μl. Detection was performed in electrospray negative ionization (ESI) using the MSE acquisition mode (data-independent acquisition alternating between low and high collision energies). The source parameters were capillary voltage −2.0 kV, cone voltage −25 V, source temperature 120°C, desolvation gas flow and temperature 800 l/h, and 350°C, respectively, cone gas flow 20 l/h. The exact mass measurements (<2 ppm) were ensured by infusing a 500 ng/ml solution of leucine-enkephalin at 15 μl/min through the Lockspray™ probe. For the acquisition and data processing we used the software Masslynx™ v.4.1 (Waters). Cucurbitacins were identified based on their molecular formula and fragmentation patterns provided by mass measurements. Peaks corresponding to known cucurbitacins were automatically integrated using Quanlynx™ with a 0.1 min chromatographic window centered on the retention time of each component and a 0.02 Da mass window centered on the (M+HCOO) ion.
Quantification of all cucurbitacins was done either in a relative manner or by external calibration using cucurbitacin B as standard. The cucurbitacin concentration was expressed in μg per g of plant material. In a first step, cucurbitacins were expressed as relative content as we did not have the cucurbitacin B standard (Supplementary Figure 2). In a second step, we could calculate absolute cucurbitacins concentration using the standard. However, the hybrids seed from Mexico (Hsc, Hwf) and one variety selected for the consumption (Slg) were not available anymore.
Trichomes
A preliminary analysis revealed a significant positive correlation between trichomes present on adaxial and abaxial surfaces of the leaf. We counted the number of trichomes on the adaxial side of the leaves and cotyledons for all squash varieties using a stereoscopic microscope. For this, 1.14 cm2 circles were punched from the middle of the cotyledon and second leaf, at the base of the main vein. We counted the trichomes longer than 1.5 mm from seven plants of each variety.
Performance of S. latifascia Caterpillars on Leaves and Cotyledons of Squash Varieties
To test the effect of plant variety and domestication purpose on insect performance, we measured the relative growth rate of S. latifascia caterpillars feeding on leaves and cotyledons of different squash varieties. For the experiment on leaves, individual second instar caterpillars were weighed and randomly placed on the adaxial side of the third leaf of each plant, using a 1.13 cm2 clip cage (homemade). This method has been used in other studies to maintain caterpillars on a single leaf (Rostás and Wölfling, 2009; Shlichta et al., 2018). For the experiment on cotyledons, we used larger clip cages (4.52 cm2) adapted to the size of the cotyledons, then we placed two second instar caterpillars, previously weighed, inside each clip cage (BioQuip product, USA) on one random cotyledon. Therefore, we divided the weight by two to have a mean weight per caterpillar. After 2 days on leaves or cotyledons, the caterpillars were weighed again. Mean relative growth rate (RGR) was calculated as, RGR = (ln (final weight) – ln (initial weight))/number of days (Tammaru and Esperk, 2007; Lariviere et al., 2015). These experiments were done once with 10 replicates per variety. Dead larvae were removed from the analysis.
Performance of D. balteata Larvae on Roots of Squash Varieties
We conducted an experiment to test the effects of squash varieties and domestication purpose on the performance of larvae of D. balteata. Five second instars were randomly selected and placed on the roots of 21-day-old squash plants that were placed inside a small plastic bag. In order to calculate the initial weight, the five larvae were weighed together before the experiment and the total weight was divided by five to obtain an estimate of the individual mean weight. As larvae feed in the soil and their development cannot be monitored, they were again weighed only at the end of the experiment. After 6 days, larvae were collected and the final weight was calculated by weighing all the larvae together for each plant and dividing the total weight by the number of larvae recovered from each plastic bag. Plants were watered with 20 ml of tap water one time in the middle of the bioassay. The number of replicates for each variety was dependent on the availability of plants. This experiment was repeated three times with a total of 13 replicates for Fbg, 14 for Fcf, 10 for Scpo, 14 for Slg, 4 for Oag, 15 for Ocp, and 13 for Ott. Larval RGR was calculated as described above.
Choice-Experiment With D. balteata on Roots of Squash Varieties
The purpose of this experiment was to test the feeding preference of D. balteata larvae when offered squash varieties with different cucurbitacin content. We used five varieties with contrasting cucurbitacin content in the roots: Ott and Scpo with high content and Fcf, Fbg, and Ocp with low or no cucurbitacins. We conducted pairwise comparisons (Fcf × Ott, Fbg × Ott, and Ocp × Scpo), each time with one variety containing none or low content of cucurbitacins and a second variety with high cucurbitacin content. To avoid any bias in the choice of larvae for a particular variety, newly hatched larvae were reared on maize roots until second instar (~10 days). Larvae were starved for 12 h before the experiments. We used squared Petri dishes (12 cm × 12 cm, Sarstedt, Germany) with moistened filter paper and a wet cotton ball. We pierced two holes in one side of the Petri dish to place the roots of the two tested varieties. The Petri dishes were sealed with parafilm. Five D. balteata larvae were released in the middle through a hole in the lid. Petri dishes were covered with red cellophane paper to decrease the amount of light, which could disturb larval behavior (Petri dishes, Supplementary Figure 3). Larval choice, defined by the larval position on the roots was recorded every 10 min during the first hour, then every hour for 6 h and their final choice after 24 h. We performed 30 replicates for the combination Fcf × Ott, 10 replicates for the combination Fbg × Ott and 10 replicates for the combination Ocp × Scpo.
Herbivore Infestation and Plant Damage of Squash Varieties in the Field
We conducted a common garden experiment to examine the susceptibility of the different squash varieties to natural infestation by herbivores. The field was located in the coastal area of the state of Oaxaca, Mexico, 15 km northwest of Puerto Escondido in the UMAR (Universidad del Mar) experimental campus (15°55.596′ N, 97°9.118′ W) (Cuny et al., 2018; Bustos-Segura et al., 2020). The experimental field area is mainly used for cultivation of maize, and it is surrounded by native vegetation including wild cucurbits and wild lima bean plants (Cuny et al., 2018). The experiment was performed during the dry season, under a tropical climate from 9 December 2016 to 30 January 2017. At the time of our experiment, the field site was also used for a parallel experiment conducted with bean plants (Cuny et al., 2018). In this site we could find natural populations of herbivorous insects that feed on squash including Chrysomelidae beetles from the subtribe Diabroticina (Acalymma spp. and Diabrotica balteata) and lepidopterans (Spodoptera latifascia and Diaphania spp.) (Hernandez-Cumplido et al., 2016; Cuny et al., 2018; Moreira et al., 2018).
For this experiment, the varieties Scpo and Slg used in lab experiments did not grow well and were replaced by one Mexican hybrid (local variety crossed with a wild squash of C. argyrosperma, Hsc) and a hybrid between two species (cultivated C. pepo crossed with cultivated C. maxima, Hwf). These two varieties are commonly grown and used for their fruits by locals in the region.
Plants were grown in biodegradable pots (4 cm of diameter) with local soil. Pots were placed inside field tents (Lumite® 2 × 2 m, Bioquip) for 2 weeks to protect plants from insect's attack. In total, seven varieties (fruit: Fbg, Fcf, ornamental: Oag, Ott, Ocp and hybrids: Hwf, Hsc; see Table 1) were used for this experiment. Two-week-old plants were transplanted directly into the ground, in a common garden setting and randomly distributed among six rows separated by 1 meter. Each row contained one plant of each variety with six plants per variety, 35 plants in total (Supplementary Figure 4). The number and identity of insects on each plant and the damage level were recorded every 3–4 days. Plant damage was visually estimated in percentage of total surface damage (eaten/removed) for each plant. This experiment lasted 6 weeks until most plants (80%) that survived had produced fruits.
At the end of this period, end of January, very few herbivores remain in the field. Because many Diabroticina species look very similar, adult beetle samples were collected and kept in a solution of 95% EtOH for identification. A DNA barcoding was performed with two legs of five similar adult beetles and one extract from larvae collected on 30 January 2017 at the field site to confirm the identity of the insects. DNA was extracted with a “NucleoSpin Tissue” kit (Macherey-Nagel, Switzerland) following the manufacturer's protocol. The 658 base-pair-long barcoding fragment of the mitochondrial gene Cytochrome Oxidase I was amplified using the primers LepF and LepR (Hebert et al., 2004). PCR products were purified enzymatically using a mix of the enzymes Exonuclease and FastAP Thermosensitive Alkaline Phosphatase (Fermentas, MA, USA). Sequencing was performed bidirectionally by an external company (Microsynth AG, Switzerland) with the primers used for the PCR. Chromatograms were edited and assembled with GeneiousR6 (Kearse et al., 2012). The consensus sequences were submitted to Genbank (Genbank accession numbers MT773619–MT773624).
Statistical Analyses
We used the statistical software R (ver. 4.02.2, the R foundation for statistical computing, Vienna, Austria). The amount of cucurbitacins was log transformed before the analysis. The variation in cucurbitacin relative content and concentration in plant parts and the number of trichomes among cucurbit varieties were analyzed with a linear model. Differences for RGR of leaf caterpillars and root beetle larvae were analyzed with linear mixed models with the domestication purpose as fixed factor and plant variety as a random factor (lme4 package, ver. 0.99999-0). Replicates from the three experiments with D. balteata were pooled. Larval mortality was analyzed with a linear model. To test the effect of purpose of domestication, plant defense (cucurbitacins concentration and trichome density) and insect performance were analyzed with linear mixed models with plant variety as a random factor.
Results from the choice experiment with D. balteata were analyzed first for the whole experiment with a generalized linear model (glm) with time as a factor for quasibinomial data. Then for each timepoint, we constructed a glm for quasibinomial data and performed a Chi-squared test.
For the common garden experiment, although other herbivore species were frequently observed foraging and feeding on plants in the adjacent plots of beans and maize (including D. balteata, S. latifascia and unidentified Chrysomelidae species), 95% of the insects recorded visiting and feeding on the squash varieties were adults of Acalymma spp. Therefore, analyses were performed only with these insect species.
We tested the difference in number of insects and leaf damage among squash varieties with time point as fixed effect and the ID of the plant as random factor, to account for the repeated measurement structure. As the number of insects was not normally distributed, we used a negative binomial distribution. To test the effect of purpose of domestication on the insect abundance, we used a generalized linear mixed model (glmer) included “purpose,” “week” and the interactions between “purpose” and “week,” varieties, as fixed factors, replicate and time as random factors. FDR-corrected post-hoc tests were carried out in order to check differences among treatments. Finally, to test whether plant damage was associated with Acalymma beetles, using a linear mixed model, we performed a regression between amount of damage per plant and the number of Acalymma adults.
Results
Cucurbitacin Content in the Different Squash Varieties and Plant Tissues
The type of cucurbitacins found in the squash varieties were identified as putative cucurbitacins B. Cucurbitacins were present in leaves, cotyledons, and roots of squash varieties. The concentration in the different plant tissues followed the same trend across varieties; the highest concentration was found in the cotyledons, followed by the roots and a very low concentration was found in leaves (Figure 1). In leaves, we did not find any significant differences in cucurbitacin content among varieties [F(5, 11) = 2.259, P = 0.121; Figure 1A], nor among domestication purposes [F(1, 4) = 2.733, P = 0.173]. Differences were, however, significant among varieties in cotyledons [F(5, 12) = 116.4, P < 0.0001; Figure 1B] and roots [F(5, 11) = 7.742, P = 0.002; Figure 1C], but not according to the purpose of domestication [cotyledons: F(1, 4) = 1.866, P = 0.243 and roots: F(1, 4) = 0.896, P = 0.397]. The highest cucurbitacin content in the cotyledons was found in Fcf, and the lowest in Ocp (Figure 1B). The highest concentration in the roots was found in Ott, and the lowest in Fcf (Figure 1C). For the Slg and hybrid varieties, we only measured relative contents of cucurbitacins (Supplementary Figure 2) and not absolute concentrations, as these varieties were not available at the time of the second experiment with the cucurbitacin standard. The relative content of cucurbitacins in cotyledons and roots of the Slg variety was significantly different than Scpo, the other variety selected for seed consumption (Supplementary Figure 2), but similar to the content in the Ott, Fcf, and Hwf varieties. Hybrid fruit varieties (Hsc and Hwf) had similar cucurbitacin content relative to the other fruit consumption (Supplementary Figure 2).
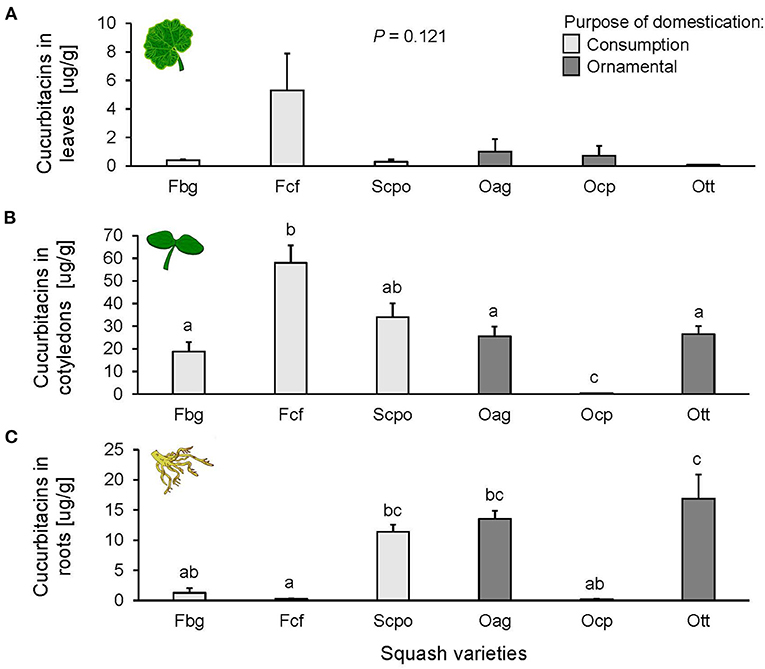
Figure 1. Cucurbitacin concentration in (A) leaves (n = 3), (B) cotyledons (n = 3), and (C) roots (n = 3) of the studied Cucurbita spp. varieties. The bars represent means (±SE). Light-colored bars are varieties domesticated for consumption and dark-colored bars are varieties domesticated for an ornamental purpose. P-values are given for treatment comparisons with log transformed data [linear model], followed by pairwise comparisons of Least Squares Means (LS means).
Number of Trichomes
The foliar trichomes identified on all of the tested varieties were simple and non-glandular (Supplementary Figure 5). No trichomes were found on cotyledons. Leaf trichome density on the adaxial side of the leaf was significantly different among cucurbit varieties [F(6, 40) = 4.379, P = 0.001; Figure 2] and between the two categories of purpose of domestication. Varieties selected for ornamental use had fewer trichomes than varieties selected for consumption [ = 5.709, P = 0.01]. The highest trichome density was found in Scpo (variety domesticated for consumption) and the lowest in the ornamental varieties (Oag and Ott).
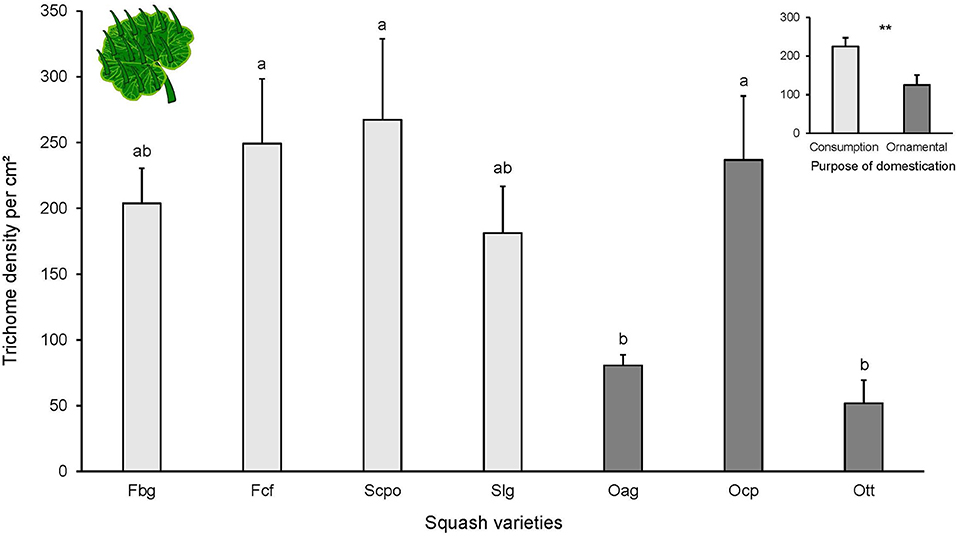
Figure 2. Number of trichomes per cm2 on adaxial side of the leaves from different squash varieties (n = 7). Bars represent means (±SE). Light-colored bars are varieties domesticated for consumption and dark-colored bars are varieties domesticated for an ornamental purpose. P-values are given for treatment comparisons with log transformed data [linear model], followed by pairwise comparisons of Least Squares Means (LS means). Upper graph is number of trichomes per purpose of domestication (consumption or ornamental). P-values are given for treatment comparison with glmer model with varieties as random factor. **P < 0.01.
Performance of S. latifascia Caterpillars on Leaves and Cotyledons of Squash Varieties
Larval survival at the end of the experiment was almost 100%, only 2 larvae died on the Ocp variety and one on Ott. We did not find significant differences among squash varieties in the RGR of S. latifascia caterpillars fed on leaves [F(6, 57) = 1.298, P = 0.268; Figure 3A]. However, when varieties were grouped by purpose of domestication, the RGR was significantly different, with the higher RGR on the ornamental varieties [F(1, 57) = 5.787, P = 0.01, Figure 3A]. Larval survival on cotyledons at the end of the experiment was 100% on all varieties. The RGR on the cotyledons was significantly affected by the plant variety [F(5, 54) = 3.015, P = 0.018; Figure 3B]. Caterpillar growth was higher on Oag compared to the other varieties, but the difference was only significant when compared with Fcf (P = 0.005; Figure 3B). Finally, no significant effect on the RGR of the caterpillars was found when varieties were grouped according to their domestication purpose [F(1, 60) = 4.328, P = 0.106], likely due to the great variation between varieties of the same group.
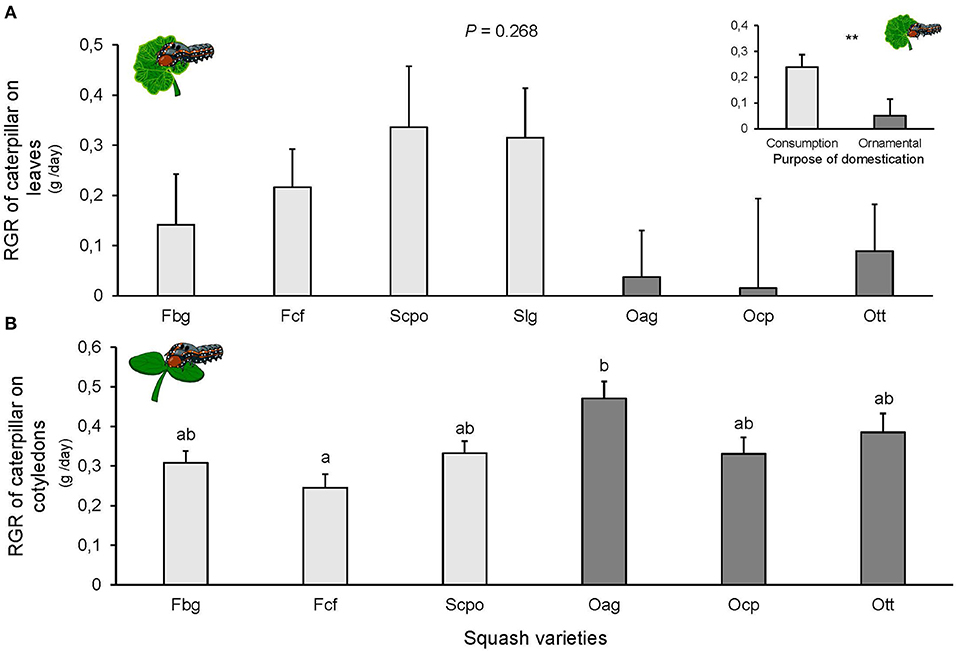
Figure 3. Relative growth rate (RGR) of Spodoptera latifascia caterpillars (A) on leaves (n = 10) and (B) cotyledons (n = 10) of squash varieties (Cucurbita spp.). Bars represent means (±SE). Light-colored bars are varieties domesticated for consumption and dark-colored bars are varieties domesticated for an ornamental purpose. RGR was calculated for each individual caterpillar as the weight difference between initial and final weight after 48 h of feeding. P-values are given for treatment [linear mixed model], followed by pairwise comparisons of Least Squares Means (LS means). Upper graph is RGR of caterpillars on leaves per purpose of domestication (consumption or ornamental). P-values are given for treatment comparison with glmer model with varieties as random factor. **P < 0.01.
Performance of D. balteata on Roots of Squash Varieties With Different Cucurbitacin Content
Diabrotica balteata larvae did not survive on the Oag variety (which had fewer replicates) and was not included in the analysis. For all other varieties, larval mortality at the end of the experiment varied between 40 and 60% with no significant differences among varieties [F(6, 76) = 0.774, P = 0.11]. These percentages reflect the number of larvae recovered from the soil at the end of the experiment and it is possible that mortality was overestimated. Notably, for the analysis of RGR only live larvae were used and all replicates had surviving larvae. No significant differences were found in the RGR of D. balteata larvae fed on roots of the different squash varieties [F(6, 58) = 1.824, P = 0.11; Figure 4], nor according to their domestication purpose [F(1, 6) = 0.24, P = 0.64].
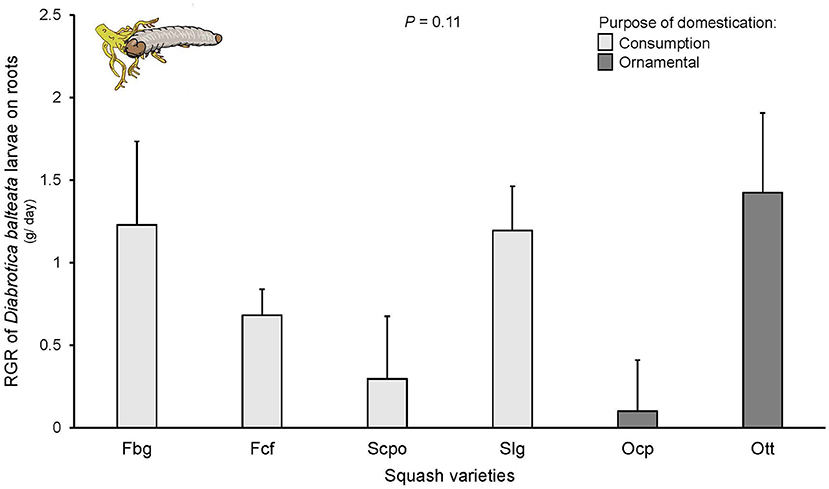
Figure 4. Relative growth rate (RGR) of Diabrotica balteata larvae on roots of squash varieties (Cucurbita spp.) n = 10–15. Bars represent means (±SE). Light-colored bars are varieties domesticated for consumption and dark-colored bars are varieties domesticated for an ornamental purpose. RGR was calculated for groups of larvae as the weight difference between initial and final weight (ln) after 6 days of feeding. P-values are given for treatment [linear mixed model].
Preference of D. balteata on Roots of Squash Varieties With Different Cucurbitacin Content
Consistently, significantly more larvae were observed on roots of varieties containing high cucurbitacin content over varieties with low or no cucurbitacins (Figure 5A) [ = 47.87, P < 0.0001], Figure 5B [ = 39.46, P < 0.0001], Figure 5C [ = 16.42, P < 0.001]. Larval choice was significantly different already after 1-h of the start of the experiment in the first two comparisons and highly significant after 24 h in all three comparisons.
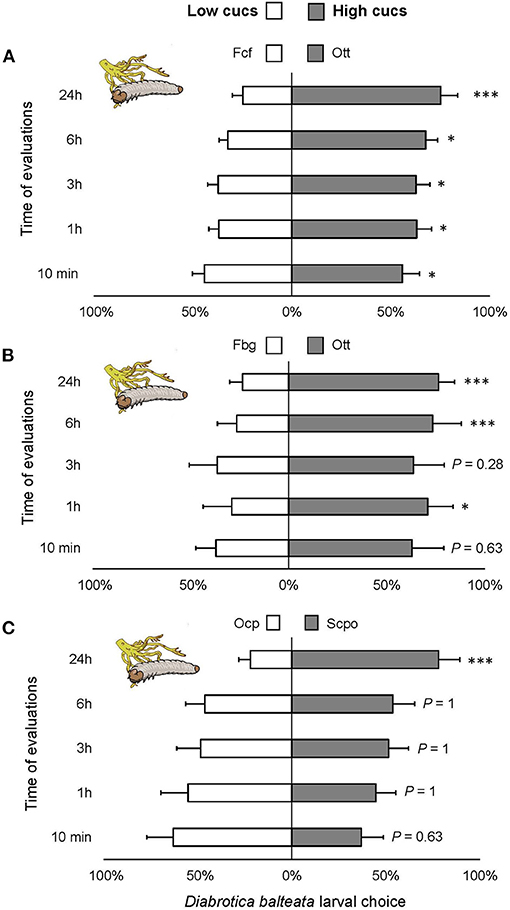
Figure 5. Feeding preference of Diabrotica balteata larvae on roots of domesticated varieties of squash with high cucurbitacin content. (A–C) Five larvae of D. balteata were allowed to choose between two varieties with low and high levels of cucurbitacins. Larval choice was recorded as number of larvae on roots after 10 min, 1, 3, 6, and 24 h after the start of the experiment. Sample sizes: (A) n = 30, (B,C) n = 10. Bars represent means (±SE) express the percentage of larvae that chose a treatment per time point (x axis). Bonferroni corrected P-values are given for treatment comparisons [generalized linear model (family, Binomial)], followed by pairwise comparisons of Least Squares Means (LS means). *P < 0.05, ***P < 0.001.
Herbivore Infestation and Plant Damage of Squash Varieties in a Common Garden
Although D. balteata was abundant at the field site, it was mainly observed on bean and maize plants adjacent to the squash common garden. Approximately, 95% of the insects that were surveyed visiting and feeding on the squash plants belonged to the genus Acalymma spp., which was confirmed by the later sequencing and identification in the lab. Blast searches on GenBank found matches (more than 99% similarity) for Acalymma blomorum and A. innubum. As the accurate identification of these two species was not possible in the field, for the analysis on abundance we pooled both Acalymma species.
The number of insects on the plants was different depending on the purpose of domestication [ = 7.462, P = 0.006, Figure 6A]. Overall, the varieties selected for consumption attracted more Acalymma beetles than ornamental varieties, especially after the fourth week [ = 15.752, P < 0.001, Figure 6A]. The variation in Acalymma beetle abundance in the common garden was explained by the time [ = 204.41, P < 0.001, Figure 6A]. The population of Acalymma spp. peaked in the middle of the season and decreased toward the end.
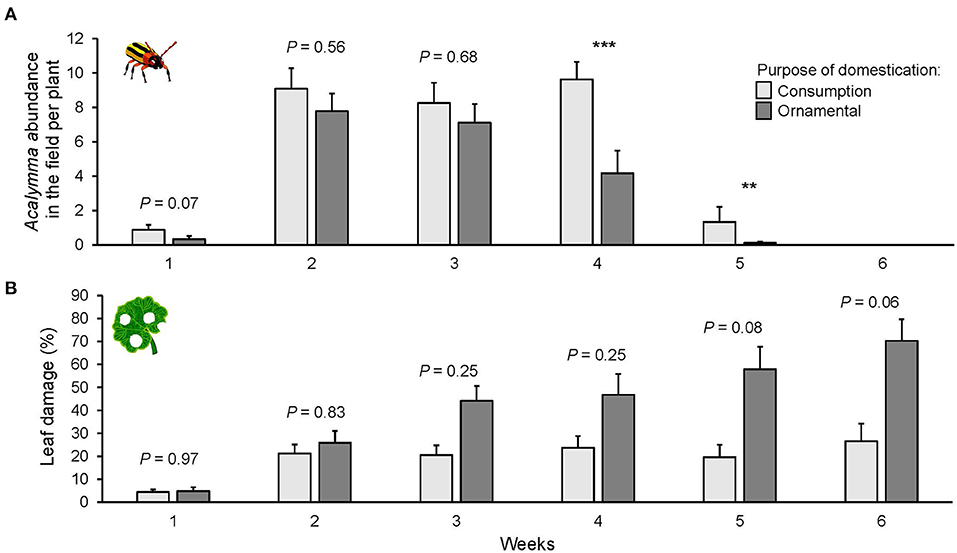
Figure 6. Mean number of Acalymma spp. beetles per plant (A) and percent of leaf damage (B) on squash varieties domesticated for different purposes in a common garden field experiment in Puerto Escondido, Oaxaca, Mexico. Data were recorded once per week for 6 weeks. Bars represent means (±SE). Light-colored bars are varieties domesticated for consumption and dark-colored bars are varieties domesticated for an ornamental purpose. P-values are given for treatment [linear mixed model] per week and followed by pairwise comparisons of Least Squares Means (LS means). **P < 0.01, ***P < 0.001.
Leaf damage was significantly different among varieties [ = 81.424, P < 0.001, Supplementary Figure 6B], and only marginally different for purpose of domestication [ = 2.71, P = 0.09, Figure 6B]. However, the analysis revealed some trends; the ornamental varieties suffered the highest herbivore damage and overall, the lowest damage was on the hybrid Mexican varieties suggesting some degree of resistance.
Leaf damage also increased through time [ = 81.424, P < 0.001, Figure 6B]. After the third week, the percentage of damage was higher for the variety Ott compared to the hybrid varieties Hsc and Hwf (P = 0.0005, Supplementary Figure 6B), but also compared to one fruit variety (Fbg). In the last week of the field census, only the fruit variety (Fbg) and the hybrid variety (Hsc) suffered <20% of leaf damage. Plant damage was not correlated with the abundance of Acalymma beetles [ = 0.193, P = 0.660].
Discussion
We found great variation in cucurbitacin content and leaf trichome density across all squash varieties. Overall, we did not find support for our hypothesis on reduced defenses in consumption varieties. Our results also show that herbivore performance is not negatively affected by high cucurbitacin content nor by trichome density. Instead, larvae of the root herbivore, D. balteata preferred to feed on varieties with high levels of cucurbitacins.
The content of cucurbitacins in leaves for all varieties was very low, and relatively high in cotyledons and roots as has been shown in other studies (Metcalf et al., 1982; Hirsh and Barbercheck, 1996; Tallamy and Gorski, 1997; Brzozowski et al., 2019). The highest content was in cotyledons. Greater levels of chemical defense in young tissues are often found in plants (Zangerl and Rutledge, 1996; McCall and Fordyce, 2010). If cotyledons, the first photosynthetic organ, are damaged, the consequences for plant development will be more detrimental than if older organs are damaged (McCall and Fordyce, 2010; Barton and Hanley, 2013). For example, glucosinolates in Arabidopsis thaliana vary among plant tissues and the highest concentrations are found in seedlings (Hopkins et al., 2009). Similarly, in lima bean (Phaseolus lunatus), cyanogenic glycosides are found in higher quantity in cotyledons than in other plant vegetative tissues (Cuny et al., 2019).
The differences in defense traits, cucurbitacins vs. trichomes, between tissues are in line with plant defense theories that predict a trade-off in resource investment for chemical and physical defenses (Freeman and Beattie, 2008; Moles et al., 2013). Similar trade-offs in plant tissues have been found in several wild populations of C. argyrosperma, in which roots and cotyledons have a high content of cucurbitacins, while leaves have high trichome densities and low levels of cucurbitacins (Jaccard et al., 2021). This suggests that although domestication reduced plant defense in squash (Metcalf et al., 1982; Nee, 1990; Brzozowski et al., 2019; Chomicki et al., 2020), it did not alter the trade-off between physical and chemical defense in different plant tissues. The different concentration of cucurbitacins and trichome density in cotyledons and leaves could indicate that plants switch defense strategies during their ontogeny toward the production of trichomes in leaves (Boege and Marquis, 2005; Barton and Koricheva, 2010). Brzozowski et al. (2020) found that, in one variety of Cucurbita pepo, there is a peak of cucurbitacin content in seedlings very early in the development, starting at the radical emergence, but cucurbitacins diminished once cotyledons were fully expanded. However, in a different variety of the same species containing less cucurbitacins, they did not detect changes in cucurbitacin levels throughout plant development. Thus, it would be interesting to further examine changes in defense traits throughout plant ontogeny for different species and cultivated varieties of squash.
Contrary to our expectations, we did not find lower levels of cucurbitacins or fewer trichomes in varieties domesticated for consumption compared to ornamental varieties. Several factors may explain these findings. First, other defense traits not considered in this study could have been reduced or selected during the domestication process. Alternatively, as fruits are the main edible part of the squash plant, it is possible that levels of cucurbitacins in leaves, cotyledons and roots do not reflect the direct target of domestication. In retrospective, it would have been informative to examine cucurbitacin levels in fruits and seeds. But this was not initially considered as we only examined the tissues eaten by the studied insects. However, because of the extreme bitterness of cucurbitacins, fruits of consumed cucurbit plants usually are void of these compounds. This is due to the original selection of non-bitter plants for food use by our ancestors and later by new breeders (Rymal et al., 1984; Gry, 2006). Yet, further studies should examine the levels of cucurbitacins present in the seeds and their effect on seed feeding insects.
Secondly, we found great variation in trichome density and cucurbitacin content among varieties domesticated for the same purpose, particularly among ornamental varieties and this variation may override differences resulting from the domestication purposes. Finally, the varieties used in our study belong to different Cucurbita species and it could be that the differences caused by speciation are higher than the putative effect of purpose of domestication. Ideally, to control for a phylogenetic signal, we should evaluate varieties of the same squash species. However, in this study we chose to use different species from the same clade that allowed us to test various uses of squash, which are not readily available within a single species. In the future, we expect to elucidate more questions regarding this aspect as we are currently exploring the relationship between purpose of domestication and plant resistance against herbivores with varieties of the species C. argyrosperma that have the same wild ancestor (Jaccard et al., 2021).
Changes in plant defense as a result of domestication are expected to alter the interaction with the plant's natural enemies (Levin, 1973; Chaudhary, 2013). Accordingly, we expected the performance of the two generalist herbivores to be lower on varieties with higher trichome densities and cucurbitacin levels. Cucurbitacins are among the bitterest plant secondary compounds found in plants (Rehm et al., 1957; Metcalf, 1986) and their roles are diverse (Hill et al., 1991), including protection against plant pathogens (Tallamy et al., 1998; Smyth et al., 2002) and deterrence of generalist insects and other arthropods (Tallamy et al., 1998). But they can also act as attractants and feeding stimulants for specialist insects, like Acalymma (Tallamy and Krischik, 1989; Tallamy and Gorski, 1997; Smyth et al., 2002). Interestingly, although S. latifascia and D. balteata are known to be very polyphagous insects (Howe et al., 1976; Habib et al., 1982), the content of cucurbitacins in cotyledons and roots did not affect larval performance. Moreover, choice experiments with D. balteata revealed that consistently, larvae preferred to feed on roots with high cucurbitacin content, which suggests that this species is adapted to these compounds. A likely explanation may lie in the common origin of genus Cucurbita and D. balteata, Mexico, where both plants and insects have been interacting for thousands of years probably well before the domestication of squash (Bach, 1980; Metcalf, 1986). Also in this region, squash is commonly grown in traditional mixed cropping with maize and beans (both crops readily consumed by D. balteata larvae). Thus, it is possible that during the generalist's long-time association with squash it has evolved to tolerate specific secondary defenses leading to an adaptation to the plant without being specialized on it. In addition, D. balteata belongs to the subtribe Diabroticina, which includes the specialist Acalymma, thus the preference toward cucurbitacins could be a shared trait among the Diabroticina. Because they can readily tolerate cucurbitacins the plants may provide a nutritional niche for the larvae, without much competition.
We also consider it possible that the larval feeding choices are the consequence of physiological adaptations in the adult Diabrotica beetles, which are known to be attracted to and feed on Cucurbita plants. Yet, it is not excluded that feeding on plants with cucurbitacins provides benefits that we have not yet uncovered.
Caterpillars of S. latifascia grew better on the consumption varieties, despite the higher density of trichomes. One reason may be the size and form of these trichomes, or the larval instar used in our study. Indeed, non-glandular trichomes only physically impede the movement of small arthropod herbivores on the plant surface (Dalin et al., 2008; Kang et al., 2010b). Moreover, like most caterpillars of the genus Spodoptera, S. latifascia have strong mandibles (Ma, 1976) which likely facilitated the chewing of the hairy leaves. Alternatively, selection on these varieties might have increased the nutritional quality of the leaves. This hypothesis remains to be tested.
Due to the low germination rate of the seeds in the field, the number of varieties and of plants of each variety that comprised the common garden experiment was small. Therefore, the conclusions that we can draw from this experiment are limited. Nevertheless, this experiment yielded interesting results. Overall, the ornamental varieties suffered the highest herbivore damage, particularly the variety Ott that did not grow well. However, we believe that these results do not necessarily reflect that insects preferred these plants. Rather, their poor growth might have compromised their ability to resist herbivore damage. In contrast, the Mexican hybrid varieties grew well and were less damaged by the natural herbivore populations, suggesting some degree of resistance, same for one variety selected for the fruit consumption (Fbg). One interesting and unexpected result was the low number of D. balteata adults that visited the plants, despite their high numbers in the field site. Instead, they preferred other crops in the site, such as beans, peanuts and maize. Individuals of Acalymma spp. on the other hand, were highly abundant in our experimental plots, but their abundance and amount of damage on the plants were not correlated with cucurbitacin levels or trichome density. This is not surprising as species in the genus Acalymma are known to be specialists on squash, adapted and attracted to cucurbitacins which they use as kairomones to locate their host plants and capable of sequestering them for their own defense (Bach, 1980; Batch, 1984; Benitez et al., 2014). Our results are in accordance with those from Theis et al. (2014). They found that the abundance of Acalymma vittatum in a squash field site in Massachusetts (USA), was not correlated with the cucurbitacin content of 20 cucurbit varieties, including cultivated and wild species. They suggest that the damage could be predicted by other plant traits and not by beetle presence and that floral sesquiterpenoids were driving these interactions. Similarly, in an ongoing study with wild and domesticated plants of C. argyrosperma, we found that larvae of the specialist A. vittatum were strongly attracted to cucurbitacins present in roots, but cucurbitacin content was not correlated with larval performance (Jaccard et al., 2021). Interestingly, these plants do not contain any cucurbitacins in the leaves which supports the hypothesis that other traits are likely involved in the attraction of adult beetles. Future work should explore the role of leaf and flower volatiles in this attraction.
In conclusion, our study suggests that varietal selection, but not the purpose of domestication in squash has altered cucurbitacin content and leaf trichome density, but the performance of the leaf and root herbivore was not affected by these defense traits. Further, the preference of D. balteata larvae to cucurbitacins may be likely due to the evolutionary history that this species has shared with wild and domesticated squash plants in their region of origin and domestication. Future efforts should be directed at elucidating the effects of domestication of this and other crops on their susceptibility to herbivorous pests. A better understanding of these effects could help in the development of crop varieties with enhanced insect and disease resistance.
Data Availability Statement
The datasets generated for this study can be found in online repositories. The names of the repository/repositories and accession number(s) can be found in the article/Supplementary Materials.
Author Contributions
CJ and BB originally formulated the idea. CJ, BB, and MC designed the experiments. CJ performed all the experiments and wrote the first version of the manuscript. GG developed the cucurbitacin extraction method. CJ, MC, and CB-S worked in the field in Mexico. CJ and CB-S did the DNA barcoding for field insect determination. LG performed the experiment on cotyledons. CJ, CB-S, and CA analyzed the data. All authors contributed to the elaboration of the design and to the writing of the last version of the manuscript.
Funding
This research was financially supported by the Swiss National Science Foundation (Project No. 31003A-162860) awarded to BB. Field work was partially financed by a travel grant to CJ from the Wuthrich et Mathey-Dupraz Foundation, University of Neuchatel.
Conflict of Interest
The authors declare that the research was conducted in the absence of any commercial or financial relationships that could be construed as a potential conflict of interest.
Acknowledgments
We thank Olivier Kindler from Syngenta Crop protection for providing eggs of Diabrotica balteata and Nicolas Marguier for the drawings. We are grateful to the Universidad del Mar (Puerto Escondido, Oaxaca, Mexico) for technical and logistic support during the field study and special thanks to Alfredo López-Rojas for assistance during field work. We thank the editor and reviewers' whose suggestions were very helpful in improving the manuscript.
Supplementary Material
The Supplementary Material for this article can be found online at: https://www.frontiersin.org/articles/10.3389/fagro.2021.683936/full#supplementary-material
References
Ashraf, M. A., Akbar, A., Askari, S. H., Iqbal, M., Rasheed, R., and Hussain, I. (2018). Recent advances in abiotic stress tolerance of plants through chemical priming: an overview. Adv. Seed Priming 51–79. doi: 10.1007/978-981-13-0032-5_4
Bach, C. E. (1980). Effects of plant density and diversity on the population dynamics of a specialist herbivore, the striped cucumber beetle, Acalymma vittata (Fab). Ecology 61, 1515–1530. doi: 10.2307/1939058
Barton, K. E., and Hanley, M. E. (2013). Seedling–herbivore interactions: insights into plant defence and regeneration patterns. Ann. Bot. 112, 643–650. doi: 10.1093/aob/mct139
Barton, K. E., and Koricheva, J. (2010). The ontogeny of plant defense and herbivory: characterizing general patterns using meta-analysis. Am. Nat. 175, 481–493. doi: 10.1086/650722
Batch, C. E. (1984). Plant spatial pattern and herbivore population dynamics: plant factors affecting the movement patterns of a tropical cucurbit specialist (Acalymma innubum). Ecology 65, 175–190. doi: 10.2307/1939469
Benitez, M., Fornoni, J., Garcia-Barrios, L., and López, R. (2014). Dynamical networks in agroecology: the milpa as a model system. Front. Ecol. Evol. Complexity.
Benrey, B., Callejas, A., Rios, L., Oyama, K., and Denno, R. F. (1998). The effects of domestication of brassica and phaseoluson the interaction between phytophagous insects and parasitoids. Biol. Control 11, 130–140. doi: 10.1006/bcon.1997.0590
Boege, K., and Marquis, R. J. (2005). Facing herbivory as you grow up: the ontogeny of resistance in plants. Trends Ecol. Evol. 20, 441–448. doi: 10.1016/j.tree.2005.05.001
Brzozowski, L. J., Gore, M. A., Agrawal, A. A., and Mazourek, M. (2020). Divergence of defensive cucurbitacins in independent Cucurbita pepo domestication events leads to differences in specialist herbivore preference. Plant, Cell Environ. 43, 2812–2825. doi: 10.1111/pce.13844
Brzozowski, L. J., Mazourek, M., and Agrawal, A. A. (2019). Mechanisms of resistance to insect herbivores in isolated breeding lineages of Cucurbita pepo. J. Chem. Ecol. 45, 313–325. doi: 10.1007/s10886-019-01046-8
Bustos-Segura, C., Cuny, M. A., and Benrey, B. (2020). Parasitoids of leaf herbivores enhance plant fitness and do not alter caterpillar-induced resistance against seed beetles. Funct. Ecol. 34, 586–596. doi: 10.1111/1365-2435.13478
Cabrera, N., and Durante, S. (2001). Description of mouthparts of the genus Acalymma Barber (Coleoptera: Chrysomelidae: Galerucinae). Trans. Am. Entomol. Soc. 127, 371–379.
Castellanos-Morales, G., Paredes-Torres, L. M., Gámez, N., Hernández-Rosales, H. S., Sánchez-de la Vega, G., Barrera-Redondo, J., et al. (2018). Historical biogeography and phylogeny of Cucurbita: insights from ancestral area reconstruction and niche evolution. Mol. Phylogenet. Evol. 128, 38–54. doi: 10.1016/j.ympev.2018.07.016
Chambliss, O. L., and Jones, C. M. (1966). Cucurbitacins: specific insect attractants in Cucurbitaceae. Science 153, 1392–1393. doi: 10.1126/science.153.3742.1392
Chaudhary, B. (2013). Plant domestication and resistance to herbivory. Int. J. Plant Genomics 2013:572784. doi: 10.1155/2013/572784
Chen, Y. H., Gols, R., and Benrey, B. (2015). Crop domestication and its impact on naturally selected trophic interactions. Annu. Rev. Entomol. 60, 35–58. doi: 10.1146/annurev-ento-010814-020601
Chen, Y. H., Shapiro, L. R., Benrey, B., and Cibrián-Jaramillo, A. (2017). Back to the origin: in situ studies are needed to understand selection during crop diversification. Front. Ecol. Evol. 5:125. doi: 10.3389/fevo.2017.00125
Chomicki, G., Schaefer, H., and Renner, S. S. (2020). Origin and domestication of Cucurbitaceae crops: insights from phylogenies, genomics and archaeology. New Phytol. 226, 1240–1255. doi: 10.1111/nph.16015
Cornille, A., Giraud, T., Smulders, M. J., Roldán-Ruiz, I., and Gladieux, P. (2014). The domestication and evolutionary ecology of apples. Trends Genet. 30, 57–65. doi: 10.1016/j.tig.2013.10.002
Cuny, M. A., Gendry, J., Hernández-Cumplido, J., and Benrey, B. (2018). Changes in plant growth and seed production in wild lima bean in response to herbivory are attenuated by parasitoids. Oecologia 187, 447–457. doi: 10.1007/s00442-018-4119-1
Cuny, M. A., La Forgia, D., Desurmont, G. A., Glauser, G., and Benrey, B. (2019). Role of cyanogenic glycosides in the seeds of wild lima bean, Phaseolus lunatus: defense, plant nutrition or both? Planta 250, 1281–1292. doi: 10.1007/s00425-019-03221-3
Da Costa, C. P., and Jones, C. (1971). Cucumber beetle resistance and mite susceptibility controlled by the bitter gene in Cucumis sativus L. Science 172, 1145–1146. doi: 10.1126/science.172.3988.1145
Dalin, P., Ågren, J., Björkman, C., Huttunen, P., and Kärkkäinen, K. (2008). Induced Plant Resistance to Herbivory. Santa Barbara, CA: Springer, 89–105.
Delgado-Baquerizo, M., Reich, P. B., García-Palacios, P., and Milla, R. (2016). Biogeographic bases for a shift in crop C: N: P stoichiometries during domestication. Ecol. Lett. 19, 564–575. doi: 10.1111/ele.12593
Dimock, M. B., Kennedy, G. G., and Williams, W. G. (1982). Toxicity studies of analogs of 2-tridecanone, a naturally occurring toxicant from a wild tomato. J. Chem. Ecol. 8, 837–842. doi: 10.1007/BF00994783
Eben, A., Barbercheck, M. E., and Martín, A. S. (1997a). Mexican diabroticite beetles: I. Laboratory test on host breadth of Acalymma and Diabrotica spp. Entomol. Exp. Appl. 82, 53–62. doi: 10.1046/j.1570-7458.1997.00113.x
Eben, A., Barbercheck, M. E., and Martín, A. S. (1997b). Mexican diabroticite beetles: II. Test for preference of cucurbit hosts by Acalymma and Diabrotica spp. Entomol. Exp. Appl. 82, 63–72. doi: 10.1046/j.1570-7458.1997.00114.x
Evans, L. (1993). Crop Evolution, Adaptation and Yield. New York, NY: Cambridge University Press, 24.
Ferguson, J., and Metcalf, R. (1985). Cucurbitacins. J. Chem. Ecol. 11, 311–318. doi: 10.1007/BF01411417
Freeman, B. C., and Beattie, G. A. (2008). An overview of plant defenses against pathogens and herbivores. Plant Health Instructor. doi: 10.1094/PHI-I-2008-0226-01
Gaillard, M. D., Glauser, G., Robert, C. A., and Turlings, T. C. (2018). Fine-tuning the ‘plant domestication-reduced defense’hypothesis: specialist vs generalist herbivores. New Phytol. 217, 355–366. doi: 10.1111/nph.14757
Gols, R., Bukovinszky, T., Van Dam, N. M., Dicke, M., Bullock, J. M., and Harvey, J. A. (2008). Performance of generalist and specialist herbivores and their endoparasitoids differs on cultivated and wild Brassica populations. J. Chem. Ecol. 34, 132–143. doi: 10.1007/s10886-008-9429-z
Habib, M., Paleari, L., and Amaral, M. (1982). Effect of three larval diets on the development of the armyworm, Spodoptera latifascia Walker, 1856 (Noctuidae, Lepidoptera). Rev. Brasil. Zool. 1, 177–182. doi: 10.1590/S0101-81751982000300007
Harlan, J., and De Wet, J. (1972). A simplified classification of cultivated sorghum 1. Crop Sci. 12, 172–176. doi: 10.2135/cropsci1972.0011183X001200020005x
Hebert, P. D., Penton, E. H., Burns, J. M., Janzen, D. H., and Hallwachs, W. (2004). Ten species in one: DNA barcoding reveals cryptic species in the neotropical skipper butterfly Astraptes fulgerator. Proc. Nat. Acad. Sci. U.S.A. 101, 14812–14817. doi: 10.1073/pnas.0406166101
Hernandez-Cumplido, J., Glauser, G., and Benrey, B. (2016). Cascading effects of early-season herbivory on late-season herbivores and their parasitoids. Ecology 97, 1283–1297. doi: 10.1890/15-1293.1
Hirsh, I., and Barbercheck, M. E. (1996). Effects of host plant and cucurbitacin on growth of larval Diabrotica undecimpunctata howardi. Entomol. Exp. Appl. 81, 47–51. doi: 10.1111/j.1570-7458.1996.tb02013.x
Hopkins, R. J., van Dam, N. M., and van Loon, J. J. (2009). Role of glucosinolates in insect-plant relationships and multitrophic interactions. Annu. Rev. Entomol. 54, 57–83. doi: 10.1146/annurev.ento.54.110807.090623
Howe, W., Sanborn, J., and Rhodes, A. (1976). Western corn rootworm adult and spotted cucumber beetle associations with Cucurbita and cucurbitacins. Environ. Entomol. 5, 1043–1048. doi: 10.1093/ee/5.6.1043
Idris, A., and Grafius, E. (1996). Effects of wild and cultivated host plants on oviposition, survival, and development of diamondback moth (Lepidoptera: Plutellidae) and its parasitoid Diadegma insulare (Hymenoptera: Ichneumonidae). Environ. Entomol. 25, 825–833. doi: 10.1093/ee/25.4.825
Jaccard, C., Bustos-Segura, C., Ye, W., Glauser, G., Kaplan, I., and Benrey, B. (2021). The domestication of Cucurbita argyrosperma altered plant defense and differentially affected the performance of a specialist and a generalist herbivore (Unpublished Results).
Kang, J.-H., Liu, G., Shi, F., Jones, A. D., Beaudry, R. M., and Howe, G. A. (2010b). The tomato odorless-2 mutant is defective in trichome-based production of diverse specialized metabolites and broad-spectrum resistance to insect herbivores. Plant Physiol. 154, 262–272. doi: 10.1104/pp.110.160192
Kang, J.-H., Shi, F., Jones, A. D., Marks, M. D., and Howe, G. A. (2010a). Distortion of trichome morphology by the hairless mutation of tomato affects leaf surface chemistry. J. Exp. Bot. 61, 1053–1064. doi: 10.1093/jxb/erp370
Kaushik, U., Aeri, V., and Mir, S. R. (2015). Cucurbitacins–an insight into medicinal leads from nature. Pharmacogn. Rev. 9, 12. doi: 10.4103/0973-7847.156314
Kearse, M., Moir, R., Wilson, A., Stones-Havas, S., Cheung, M., Sturrock, S., et al. (2012). Geneious Basic: an integrated and extendable desktop software platform for the organization and analysis of sequence data. Bioinformatics 28, 1647–1649. doi: 10.1093/bioinformatics/bts199
Koinange, E. M., Singh, S. P., and Gepts, P. (1996). Genetic control of the domestication syndrome in common bean. Crop Sci. 36, 1037–1045. doi: 10.2135/cropsci1996.0011183X003600040037x
Lariviere, A., Limeri, L. B., Meindl, G. A., and Traw, M. B. (2015). Herbivory and relative growth rates of Pieris rapae are correlated with host constitutive salicylic acid and flowering time. J. Chem. Ecol. 41, 350–359. doi: 10.1007/s10886-015-0572-z
Levin, D. A. (1973). The role of trichomes in plant defense. Q. Rev. Biol. 48, 3–15. doi: 10.1086/407484
Lira, R., Eguiarte, L., Montes, S., Zizumbo-Villarreal, D., Marín, P. C. G., and Quesada, M. (2016). “Homo sapiens–Cucurbita interaction in mesoamerica: domestication, dissemination, and diversification,” in Ethnobotany of Mexico, 389–401. doi: 10.1007/978-1-4614-6669-7_15
Ma, W.-C. (1976). The Host-Plant in Relation to Insect Behaviour and Reproduction Boston, MA: Springer, 139–151. doi: 10.1007/978-1-4613-4274-8_23
McCall, A. C., and Fordyce, J. A. (2010). Can optimal defence theory be used to predict the distribution of plant chemical defences? J. Ecol. 98, 985–992. doi: 10.1111/j.1365-2745.2010.01693.x
Metcalf, R., and Lampman, R. (1989). The chemical ecology of diabroticites and cucurbitaceae. Experientia 45, 240–247. doi: 10.1007/BF01951810
Metcalf, R. L. (1979). Plants, chemicals, and insects: some aspects of coevolution. Bull. ESA 25, 30–35. doi: 10.1093/besa/25.1.30
Metcalf, R. L. (1986). Coevolutionary adaptations of rootworm beetles (Coleoptera: Chrysomelidae) to cucurbitacins. J. Chem. Ecol. 12, 1109–1124. doi: 10.1007/BF01638999
Metcalf, R. L., Rhodes, A. M., Metcalf, R. A., Ferguson, J., Metcalf, E. R., and Lu, P. Y. (1982). Cucurbitacin contents and diabroticite (Coleoptera: Chrysomelidae) feeding upon Cucurbita spp. Environ. Entomol. 11, 931–937. doi: 10.1093/ee/11.4.931
Meyer, R. S., DuVal, A. E., and Jensen, H. R. (2012). Patterns and processes in crop domestication: an historical review and quantitative analysis of 203 global food crops. New Phytol. 196, 29–48. doi: 10.1111/j.1469-8137.2012.04253.x
Meyer, R. S., and Purugganan, M. D. (2013). Evolution of crop species: genetics of domestication and diversification. Nat. Rev. Genet. 14, 840–852. doi: 10.1038/nrg3605
Mitchell, C., Brennan, R. M., Graham, J., and Karley, A. J. (2016). Plant defense against herbivorous pests: exploiting resistance and tolerance traits for sustainable crop protection. Front. Plant Sci. 7:1132. doi: 10.3389/fpls.2016.01132
Moles, A. T., Peco, B., Wallis, I. R., Foley, W. J., Poore, A. G., Seabloom, E. W., et al. (2013). Correlations between physical and chemical defences in plants: tradeoffs, syndromes, or just many different ways to skin a herbivorous cat? New Phytol. 198, 252–263. doi: 10.1111/nph.12116
Moreira, X., Abdala-Roberts, L., Gols, R., and Francisco, M. (2018). Plant domestication decreases both constitutive and induced chemical defences by direct selection against defensive traits. Sci. Rep. 8, 1–11. doi: 10.1038/s41598-018-31041-0
Moreira, X., Abdala-Roberts, L., Hernández-Cumplido, J., Cuny, M. A., Glauser, G., and Benrey, B. (2015). Specificity of induced defenses, growth, and reproduction in lima bean (Phaseolus lunatus) in response to multispecies herbivory. Am. J. Bot. 102, 1300–1308. doi: 10.3732/ajb.1500255
Mousavi, S. Z., and Bathaie, S. Z. (2011). Historical uses of saffron: identifying potential new avenues for modern research. Avicenna J. Phytomed. 1, 57–66. doi: 10.22038/AJP.2011.122
Nee, M. (1990). The domestication of cucurbita (Cucurbitaceae). Econ. Bot. 44:56. doi: 10.1007/BF02860475
Newell-McGloughlin, M. (2008). Nutritionally improved agricultural crops. Plant Physiol. 147, 939–953. doi: 10.1104/pp.108.121947
Nigh, R., and Diemont, S. A. (2013). The Maya milpa: fire and the legacy of living soil. Front. Ecol. Environ. 11, e45–e54. doi: 10.1890/120344
Pitre Jr, H. N., and Kantack, E. J. (1962). Biology of the banded cucumber beetle, Diabrotica balteata, in Louisiana. J. Econ. Entomol. 55, 904–906. doi: 10.1093/jee/55.6.904
Portillo, H. E., Pitre, H. N., Meckenstock, D. H., and Andrews, K. L. (1996). Oviposition preference of Spodoptera latifascia (Lepidoptera: Noctuidae) for sorghum, maize and non-crop vegetation. Florida Entomol. 552–562. doi: 10.2307/3496068
Rehm, S., Enslin, P., Meeuse, A., and Wessels, J. (1957). Bitter principles of the Cucurbitaceae. VII.—the distribution of bitter principles in this plant family. J. Sci. Food Agricul. 8, 679–686. doi: 10.1002/jsfa.2740081203
Rostás, M., and Wölfling, M. (2009). Caterpillar footprints as host location kairomones for Cotesia marginiventris: persistence and chemical nature. J. Chem. Ecol. 35, 20–27. doi: 10.1007/s10886-009-9590-z
Rymal, K., Chambliss, O., Bond, M., and Smith, D. (1984). Squash containing toxic cucurbitacin compounds occurring in California and Alabama. J. Food Prot. 47, 270–271. doi: 10.4315/0362-028X-47.4.270
Sanjur, O. I., Piperno, D. R., Andres, T. C., and Wessel-Beaver, L. (2002). Phylogenetic relationships among domesticated and wild species of Cucurbita (Cucurbitaceae) inferred from a mitochondrial gene: implications for crop plant evolution and areas of origin. Proc. Natl. Acad. Sci. U.S.A. 99, 535–540. doi: 10.1073/pnas.012577299
Shlichta, J. G., Cuny, M. A., Hernandez-Cumplido, J., Traine, J., and Benrey, B. (2018). Contrasting consequences of plant domestication for the chemical defenses of leaves and seeds in lima bean plants. Basic Appl. Ecol. 31, 10–20. doi: 10.1016/j.baae.2018.05.012
Simon, P. W. (2000). Domestication, historical development, and modern breeding of carrot. Plant Breed. Rev. 19, 157–190. doi: 10.1002/9780470650172.ch5
Smith, R. (1966). F. Distributional patterns of selected western North American insects: the distribution of diabroticites in western North America. Bull. ESA 12, 108–110. doi: 10.1093/besa/12.2.108
Smyth, R. R., Tallamy, D. W., Renwick, J. A. A., and Hoffmann, M. P. (2002). Effects of age, sex, and dietary history on response to cucurbitacin in Acalymma vittatum. Entomologia 104, 69–78. doi: 10.1046/j.1570-7458.2002.00992.x
Tallamy, D. W., and Gorski, P. M. (1997). Long-and short-term effect of cucurbitacin consumption on Acalymma vittatum (Coleoptera: Chrysomelidae) fitness. Environ. Entomol. 26, 672–677. doi: 10.1093/ee/26.3.672
Tallamy, D. W., and Krischik, V. A. (1989). Variation and function of cucurbitacins in Cucurbita: an examination of current hypotheses. Am. Nat. 133, 766–786. doi: 10.1086/284952
Tallamy, D. W., Whittington, D. P., Defurio, F., Fontaine, D. A., Gorski, P. M., and Gothro, P. W. (1998). Sequestered cucurbitacins and pathogenicity of Metarhizium anisopliae (Moniliales: Moniliaceae) on spotted cucumber beetle eggs and larvae (Coleoptera: Chrysomelidae). Environ. Entomol. 27, 366–372. doi: 10.1093/ee/27.2.366
Tammaru, T., and Esperk, T. (2007). Growth allometry of immature insects: larvae do not grow exponentially. Fuct. Ecol. 21, 1099–1105. doi: 10.1111/j.1365-2435.2007.01319.x
Teng, H., Waddill, V., Slansky, F., and Strayer, J. (1984). Performance and host preference of adult banded cucumber beetles, Diabrotica balteata, when offered several crops. J. Agric. Entomol. 1, 330–338.
Theis, N., Barber, N. A., Gillespie, S. D., Hazzard, R. V., and Adler, L. S. (2014). Attracting mutualists and antagonists: plant trait variation explains the distribution of specialist floral herbivores and pollinators on crops and wild gourds. Am. J. Bot. 101, 1314–1322. doi: 10.3732/ajb.1400171
Tian, D., Tooker, J., Peiffer, M., Chung, S. H., and Felton, G. W. (2012). Role of trichomes in defense against herbivores: comparison of herbivore response to woolly and hairless trichome mutants in tomato (Solanum lycopersicum). Planta 236, 1053–1066. doi: 10.1007/s00425-012-1651-9
Traine, J., Cuny, M. A., Bustos-Segura, C., and Benrey, B. (2020). The interaction between host and host plant influences the oviposition and performance of a generalist ectoparasitoid. Entomol. Exp. Appl. 169, 133–144. doi: 10.1111/eea.12949
Turcotte, M. M., Turley, N. E., and Johnson, M. T. (2014). The impact of domestication on resistance to two generalist herbivores across 29 independent domestication events. New Phytol. 204, 671–681. doi: 10.1111/nph.12935
Vermeij, G. J. (2015). Plants that lead: do some surface features direct enemy traffic on leaves and stems? Biol. J. Linnean Soc. 116, 288–294. doi: 10.1111/bij.12592
Wagner, G. J. (1991). Secreting glandular trichomes: more than just hairs. Plant Physiol. 96, 675–679. doi: 10.1104/pp.96.3.675
Whitehead, S. R., Turcotte, M. M., and Poveda, K. (2017). Domestication impacts on plant-herbivore interactions: a meta-analysis. Philos. Trans. R. Soc. Lond. B. Biol. Sci. 372:20160034. doi: 10.1098/rstb.2016.0034
Wink, M. (1988). Plant breeding: importance of plant secondary metabolites for protection against pathogens and herbivores. Theor. Appl. Genet. 75, 225–233. doi: 10.1007/BF00303957
Zangerl, A. R., and Rutledge, C. (1996). The probability of attack and patterns of constitutive and induced defense: a test of optimal defense theory. Am. Nat. 147, 599–608. doi: 10.1086/285868
Zizumbo-Villarreal, D., Colunga-GarcíaMarín, P., and Evolution, C. (2010). Origin of agriculture and plant domestication in West Mesoamerica. Genet. Resour. Crop Evol. 57, 813–825. doi: 10.1007/s10722-009-9521-4
Keywords: domestication, squash, plant defenses, cucurbitacins, herbivores, trichomes
Citation: Jaccard C, Cuny MAC, Bustos-Segura C, Arce CCM, Giollo L, Glauser G and Benrey B (2021) Squash Varieties Domesticated for Different Purposes Differ in Chemical and Physical Defense Against Leaf and Root Herbivores. Front. Agron. 3:683936. doi: 10.3389/fagro.2021.683936
Received: 22 March 2021; Accepted: 16 June 2021;
Published: 16 July 2021.
Edited by:
Cesar Rodriguez-Saona, Rutgers, The State University of New Jersey, United StatesReviewed by:
Rupesh Kariyat, The University of Texas Rio Grande Valley, United StatesIan Pearse, United States Geological Survey (USGS), United States
Copyright © 2021 Jaccard, Cuny, Bustos-Segura, Arce, Giollo, Glauser and Benrey. This is an open-access article distributed under the terms of the Creative Commons Attribution License (CC BY). The use, distribution or reproduction in other forums is permitted, provided the original author(s) and the copyright owner(s) are credited and that the original publication in this journal is cited, in accordance with accepted academic practice. No use, distribution or reproduction is permitted which does not comply with these terms.
*Correspondence: Betty Benrey, betty.benrey@unine.ch