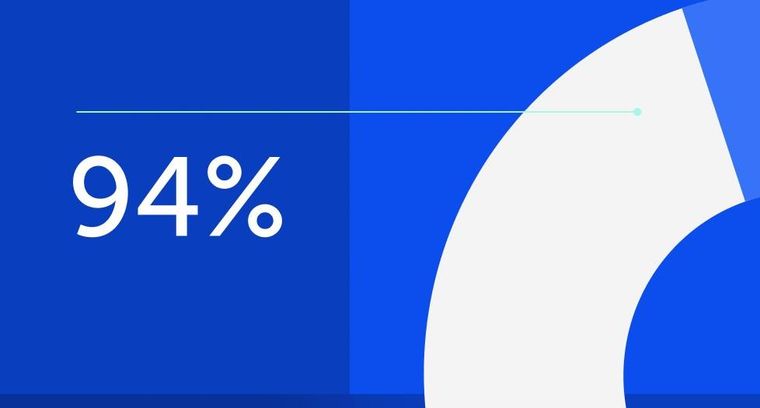
94% of researchers rate our articles as excellent or good
Learn more about the work of our research integrity team to safeguard the quality of each article we publish.
Find out more
ORIGINAL RESEARCH article
Front. Agron., 12 November 2021
Sec. Plant-Soil Interactions
Volume 3 - 2021 | https://doi.org/10.3389/fagro.2021.680466
This article is part of the Research TopicSoil-Plant-Microbe Interactions: An Innovative Approach Towards Improving Soil Health and Plant GrowthView all 26 articles
Cotton is a valuable fiber and cash crop in Telangana, India. This study examines how crop growth and fiber yield are affected by the uneven distribution of rainfall. Cotton phyllosphere bacterial isolates were gathered from the Rangareddy and Warangal Districts of Telangana, and in total, 31 phyllosphere bacterial isolates were obtained. These isolates were screened for drought tolerance and it was found that fifteen suitable isolates grew at −1.25 Mpa stress level with copious amounts of exopolysaccharides production. These isolates were further screened for ACC deaminase production and we observed 0.13–0.40 mM of α-ketobutyrate per milligram of cellular protein per hour. Five efficient bacterial isolates, namely Pseudomonas stutzeri, Acinetobacter sp., Bacillus mojavensis, Pseudomonas chlororaphis, and Enterobacter asburiae were found to produce ACC deaminase and were able to grow at −1.25 Mpa stress level. The cotton variety ADB-542 (drought susceptible) was treated with drought-tolerant five isolates. Acinetobacter sp. treated seeds had the highest seed germination and seedling vigor of 76.67 and 45.81%, respectively. The nutrient status of inoculated plots was considerably improved. The root length, fresh weight, proline content, and the number of bolls were increased by 28.52, 41.9, 28.78, and 12.99%, respectively, with the inoculation of Acinetobacter sp., to plants at −0.75 Mpa water potential. Overall the performance of cotton was improved significantly with the inoculation of phyllosphere bacteria to seeds; hence they can be recommended for the application of field crops as bio-inoculants.
Cotton is mainly cultivated as a rainfed crop in the Telangana districts of Warangal and Rangareddy due to a lack of irrigation facility. In recent years, cotton production was declined due to severe climate change biotic stress, increased temperature, inconsistent distribution of rainfall, decreased water availability, and crop yield (Gosal et al., 2009). As per Cotton Advisory Board (CAB) estimates, cotton production in India during 2017–18 was 377 lakh bales (170 kg) from 122 lakh hectares with a productivity of 524 kg lint/ha (CAB, AICRP for Cotton 2017–18). The quantity and quality of the fiber produced in cotton plant crops are directly related to water availability during the different phenological phases of development (Zakaria and Sabrina, 2017).
In many cases, the cotton economic yield was severely affected by drought stress in arid and semi-arid areas. Drought impedes germination, seedling growth, flower and bud drop, interferes with photosynthesis, and increases CO2 loss through transpiration, thus increasing the competition between vegetative and reproductive aspects for nutrients and carbohydrates (Qian et al., 2020). Moisture conservation practices have improved the crop stand by 10–15%; however, low yields were incurred due to peak moisture stress at the square formation stage. A lack of efficient moisture stress management technology makes the crops more vulnerable to drought conditions.
Developing climate-resilient varieties and hybrids takes several years and demands extensive labor and money. The potential for phyllosphere microorganisms to promote plant growth under various abiotic stress conditions, especially drought, has been of particular interest to researchers in recent years. Illustrating that the phyllosphere microflora is extremely useful for elucidating underlying plant stress mitigation pathways and identifying possible future candidates. However, there is a lack of detailed evidence on the phyllosphere bacterial population of cotton and their functions in abiotic stress mitigation. Beneficial phyllosphere microorganisms help plants manage abiotic stress more efficiently by interacting with roots and leaves (Glick et al., 2007; Chauhan et al., 2015; Ripa et al., 2019). Drought stress induces plants to produce more abscisic acids (ABA), which predisposes plant tissues to produce stress-related proteins and causes stomatal closure (Sah et al., 2016). The concentration of ABA in plants is influenced both favorably and adversely by bacterial interactions with plants. Inoculation of Bacillus pumilis, and Azospirillum lipoferum increased the ABA concentration several-fold in planta (Cohen et al., 2015; Yasmin et al., 2017). Several researchers have suggested using phyllosphere bacteria in drought stress mitigation in several crops (Timmusk and Wagner, 1999; Marulanda et al., 2008). Phyllosphere microorganisms tend to produce and share compatible solutes, amines, and sugars with plants and incite Induced Systemic Tolerance (IST) against drought stress (Yang et al., 2009).
It has also been reported that phyllosphere microorganisms produce exopolysaccharides (EPS), thus indirectly improving plants' water availability (Creus et al., 2004). In addition, they were capable of producing phytohormones like cytokinin and auxins with concomitant production of stress response enzyme-ACC deaminase under abiotic stress conditions (Madhaiyan et al., 2005; Chinnadurai et al., 2009). The phyllo-rhizosphere isolates, Pantoea agglomerans, and Azospirillum brasilience produce nitric oxide on leaves, a signaling molecule for IAA-producing pathways, and help with adventurous root formation which effectively checks the drought stress in plants (Molina-Favero et al., 2008). The phyllosphere bacteria are more competitive in stressed ecosystems because they are continually exposed to harsh conditions, as shown by the high microbial numbers. The common phyllosphere bacteria encountered are Pseudomonas sp., Xanthomonas sp., Bacillus sp., Erwinia sp. (Pantoea sp.), and Methylobacterium sp. (Holland et al., 2002; Kumar et al., 2019). In recent years, there has been raised concern about the possible role of phyllosphere microorganisms in foodborne illnesses and possible metabolic interaction with applied foliar microbes (Legein et al., 2020). Rhizosphere application of microbes potentially avoids foodborne illness through physical separation. Furthermore, because drought is primarily defined by a lack of moisture in the root zone, phyllosphere bacteria in the root zone have a more significant impact than phyllosphere application.
There is still a lack of research on the application of phyllosphere microorganisms to soil or the rhizosphere and their potential roles in drought mitigation (Saghafi et al., 2020). In response, this study aimed to find the prospective phyllobacteria for drought mitigation in the rhizosphere. The cotton-growing regions of Telangana (Warangal and Rangareddy districts) have witnessed intermittent rainfall or drought for the past few decades, and it is essential to find a suitable solution to the problem. In this view, the present study is focused on the isolation and characterization of drought-tolerant cotton phyllosphere-associated bacterial strains, which were screened for stress alleviating characters such as IAA, ACC deaminase, and exopolysaccharides production. They were then further evaluated for inducing drought tolerance in cotton under pot culture conditions.
Leaf samples were collected from cotton crop growing areas in the Rangareddy and Warangal Districts of Telangana State. All samples were separately bagged, brought to the laboratory in sterile polythene bags, and stored at 4°C to isolate phyllosphere bacteria later.
Different phyllosphere bacterial strains were isolated by leaf imprinting technique, using suitable media and serial dilution method. Ammonium Mineral Salts (AMS) medium (Whittenbury et al., 1970) amended with filter-sterilized solutions of cycloheximide (50 mg/ml), vitamins (Colby and Zatman, 1973), and 0.5% v/v methanol was used as a selective medium for isolating phyllosphere bacteria and methylotrophs, and the final pH of the medium was adjusted to pH 7.0. Fresh leaves of cotton were imprinted on the AMS medium so that each plate should have one side of the leaf (Corpe et al., 1985). Finally, the plates were incubated at 28 ± 2°C for 5 days in the incubator.
Trypticase Soy Broth (TSB) with different water potentials (0 Mpa, −0.25 Mpa, −0.50 Mpa, −0.75 Mpa, and −1.25 Mpa) was prepared by adding 2.4, 4.7, 6.5, and 9.4 g of polyethylene glycol (PEG-6000) in 100 ml of distilled water (Michel and Kaufmann, 1973; Sandhya et al., 2015). Pure bacterial isolates were inoculated into TSB and incubated at 30°C for 24 h at 120 rpm, and shaken in a rotary shaker. Growth measurements were recorded at 600 nm in a spectrophotometer for the treatments, including control (TSB without bacterial culture). Isolates with an increased optical density of 48 h incubation were considered drought-tolerant bacteria.
The production of exopolysaccharides by isolates at stress-induced conditions was estimated as per the procedure given by Ali and Kim (2018). In brief, isolates were inoculated in trypticase soy broth with −0.75 Mpa stress level and incubated at 30°C for 3 days in the shaker at 200 rpm. Bacterial cells were harvested with 500 μl of 1 mM EDTA and centrifuged at 9,000 rpm for 10 min. Then the supernatant was separated and added with cold acetone solution. Biomass deposited in exopolysaccharide was washed twice with distilled water and dried at 60°C for 24 h. Dry weights of biomass produced by the bacterial isolates were expressed as mg/ml.
Morphological characters such as shape, size, and gram responses were studied using standard protocols (Cappuccino and Sherman, 1992). The physiological/biochemical characters such as indole production (Isenberg and Sundheim, 1958), methyl red test (Macfaddin, 2000), Voges-Prausker test (Macfaddin, 2000), Citrate utilization test (Macfaddin, 2000), starch hydrolysis test (Macfaddin, 2000), catalase test (Rangaswami and Bagyaraj, 1993), and oxidase test (Collins and Lyne, 1970) were performed for all isolates. The production of HCN by bacterial isolates was tested on AMS medium amended with glycine at 4.4 g/l (Castric and Castric, 1983). Production of brown or reddish-brown was considered positive and scored as moderate (++) or strong (+++) based on color intensity.
The genomic DNA of bacteria was isolated by following the standard method given by Ausubel et al. (1999). The extracted DNA was initially quantified against the lambda DNA standard (1 Kb). The 16S rRNA gene of the target bacterial isolates was amplified by using universal primers as reported by Heddi et al. (1998). The reaction mixture was incubated in a thermal cycler (Eppendorf, Germany), and the conditions for PCR amplification were 94°C for 5 min for initial denaturation, followed by 35 cycles of 95°C for 1 min (denaturation), 60°C for 45 s (annealing), 72°C for 1 min 30 s (extension) and a final extension at 72°C for 7 min. Electrophoresis used 1% agarose gel to resolve the amplified PCR products in 1 X Tris-acetate EDTA buffer, a 1 kb DNA ladder (Bangalore Genei) marker. PCR products of the 16S rRNA gene of five efficient bacterial isolates were freeze-dried in a lyophilizer. The purified PCR products were sequenced using the forward primer 5′-AGA GTT TGA TCC TGG CTC AG- 3′ and reverse primer 5′-CGG TTA CCT TGT TAC GAC TT-3′ in Sanger dideoxy method. The partial 16S rRNA sequences of bacterial isolates were compared with the available sequences in GenBank-BLAST (Basic Local Alignment Search Tool) Database (http://www.ncbi.nih.gov/blast).
Isolates were tested for their ability to solubilize tri-calcium phosphate [Ca3(PO4)2], zinc phosphate, and potassium release on Pikovaskaya's agar medium (Pikovaskaya, 1948), Tris minimal medium (Saravanan et al., 2003), and Aleksandrov's medium supplemented with mica (Prajapati and Modi, 2012), respectively. The plates were incubated at 37°C for 3–5 days. The isolates showed a clear halo zone of solubilization around the colony were taken as solubilizers, and solubilization was expressed in mm.
Indole-Acetic Acid production by isolates was measured by the method given by Gordon and Weber (1951). In short, bacterial cultures were inoculated to Luria-Bertani broth containing 5 mM tryptophan and incubated for 3–4 days. They were followed by centrifugation at 10,000 rpm for 20 min. Two ml of the supernatant were mixed with two drops of orthophosphoric acid and 4 ml of Salkowski reagent and incubated at room temperature for 25 min. The pink colors developed after 30 min, which was considered positive for IAA production. The intensity of the pink color developed was measured at 530 nm in a spectrophotometer. A standard graph determined the quantity of IAA in the culture filtrate and expressed as μg/ml of the medium.
Isolates were tested for siderophore production on Chrome azurol sulfonate (CAS) agar medium (Schwyn and Neilands, 1987). CAS agar medium was prepared by dissolving 60.5 mg CAS in 50 ml of double-distilled water, mixed with 10 ml of iron (III) solution (1 mM FeCl3. 6H2O in 10 mM HCl) was added to 72.9 mg of hexadecyl trimethyl ammonium bromide (HDTMA) in 40 ml of distilled water. This reagent was added to the PIPES agar medium (30.24 g of PIPES buffer dissolved in 750 ml of distilled water + 15 g of agar, and its pH was adjusted to 6.8 using 1.06 g of NaOH pellets). The medium was then allowed to solidify in the plates, and isolates were spot inoculated and incubated at 30°C for 3–4 days. Siderophore-producing isolates produced an orange color around the colony.
The ACC deaminase production by isolates was assayed using the protocol laid down by Honma and Shimomura (1978). In this method, the amount of α-ketobutyrate produced after the cleavage of ACC by ACC deaminase was measured in mM/mg/h of α-ketobutyrate, which was compared to a standard curve of α-ketobutyrate based on the absorbance at 540 nm in a spectrophotometer.
The selected bacterial inoculum was prepared in the Tryptic Soya Broth (TSB) and incubated at 28 ± 2°C in an orbital shaking incubator at 160 rpm for 3–5 days. The cells were harvested at 15,000 × g for 10 min and then suspended in 0.03 M MgSO4 to obtain the requisite cell concentration (109 CFU/ml) at OD600. The compatibility of the five bacterial isolates was examined on TSA plates using the dual culture technique for microbial consortium preparation (Kunova et al., 2016). The bacterial consortium was prepared by inoculating TSB medium with equivalent quantities of 3-day old bacterial suspensions of CPB23, CPB24, CPB25, CPB27, and CPB31.
Cotton (G. hirsutum L.) variety ADB-542 seeds were surface sterilized in 70% ethanol for 30 s, then 0.1 per cent mercuric chloride for 3 min, followed by sterile distilled water rinses. The cotton seeds were incubated for 1 h in the appropriate suspension of bacterial cultures, then air-dried aseptically in the laminar airflow for bacterial inoculation. Cotton seeds that had been surface-sterilized but not primed were used in the control group and immersed in a 0.03 M MgSO4 solution for 5 min. Surface-sterilized seeds were aseptically placed on 0.8 percent seedling agar and incubated for 48–96 h to test germination (Islam et al., 2009).
A microcosm experiment was carried out at the Deptartment of Agricultural Microbiology and Bioenergy, the College of Agriculture, PJTSAU, Rajendranagar, Hyderabad during Rabi-2018 (September 20, to December, 20). The mean temperature during the crop growth period was ~22 ± 2°C. The soil was collected from College Farm, PJTSAU, Hyderabad, which had physiochemical properties, namely, red color with pH-7.25, Electrical Conductivity (EC)-0.498 (dS m−1), organic carbon (OC) 0.65%, available Nitrogen (N)-148.18 Kg ha−1, available phosphorous (P2O5)-68.22 Kg ha−1, available potassium (K2O)-270.12 Kg ha−1, and bulk density 1.7 mg m−3. This soil was autoclaved (121°C for 15 min at 15 psi) three times and cooled before filling into pots, and each pot was filled with 15 kg of soil pot−1. Seeds of ADB-542 variety were obtained from RRC, Rajendranagar, Hyderabad. The inoculum was prepared by growing selected isolates in LB broth for 24 h. Treatments were imposed by inoculating the drought susceptible cotton variety (ADB-542) seeds with 2 ml (109 CFU/ml) of efficient strains (Whittenbury et al., 1970); seeds without inoculation served as control. Three seeds per pot were sown at a depth of 5 cm, and the experiment was laid out in Complete Randomized Block Design (CRD) with eight treatments (five isolates, consortium, and two controls) and three replications. After 15 days of sowing, drought was imposed by adding PEG-6000 at 10, 20, and 30% in pots with 50% field capacity. After 20 days of sowing, cultures were sprayed on leaves with a hand sprayer at the rate of 25 ml/plant (Holland and Polacco, 1994). The recommended fertilizer (RDF) dose for cotton was 120-60-60 kg of NPK ha−1. Irrigations were given based on the soil field capacity status.
Biometric observations viz., germination percentage (20 DAS), seedling vigor index [Seedling vigor index = (Root length + shoot length) x germination %] as given by Abdulbaki and Andersons (1973) and shoot length (cm), root length (cm), fresh weight (g), dry weight (gm, after oven drying at 60–65°C for 1 h for 3 consequent days), number of leaves, and number of branches were recorded at 30, 60, and 90 days after sowing in five competitive plants selected at random from each treatment and the mean per plant was worked out. Yield attributes including the number of bolls, boll weight (g), and seed cotton yield (Kg ha−1).
The bacterial population count on Tryptic soya Agar (TSA) was recorded at 30, 60, and 90 DAS in soil and leaf samples. In brief, leaf samples and rhizosphere soil samples were collected from eight treatments. Leaf samples were processed as per the procedure given by Yadav et al. (2010), and soil samples were processed as per the procedure laid down by Parmar et al. (2016).
The amount of proline in cotton leaf samples was determined based on proline's reaction with ninhydrin (Bates et al., 1973) at the flowering stage in pot culture (60 Days After Planting). Fresh cotton leaves (0.5 g) were hand–homogenized in 5 ml of 3% (w/v) sulfosalicylic acid. The homogenate was filtered through Whatman no. 2 filter paper. In another test tube, 2 ml of acid ninhydrin and 2 ml of glacial acetic acid were added to the 2 ml of filtrated extract. The resulting mixture was incubated at 100°C in a hot water bath. The reaction was then stopped using an ice bath, and the contents were extracted with 4 ml of toluene and mixed vigorously using a stirrer for 15–20 s. The chromophore containing toluene was aspirated from the aqueous phase and thawed to room temperature, and the absorbance of the solution was measured at 520 nm using a spectrophotometer. The proline concentration was determined from a standard curve prepared with L—proline and expressed as μg/g FW (Fresh weight).
The data obtained in different treatments were statistically analyzed using a Completely Randomized Design (CRD) described by Yates (1937). Data on other characters viz., growth parameters, NPK analysis, bacterial population, proline estimation, yield attributes, and yield were subjected to analysis of variance (ANOVA) procedures outlined for CRD (wherever necessary). Statistical significance was tested by p-value at 0.01 level of probability, and the critical difference was worked out where the effects were significant.
A total of 31 isolates from the Telangana districts of Rangareddy (17 isolates) and Warangal (14 isolates) were isolated and purified based on variations in colony morphology on the AMS medium. In the Gram staining test, seventeen isolates retained crystal violet (Gram-positive) and fourteen isolates retained safranin (Gram-negative). Microscopic studies (Olympus) using an oil immersion lens (Numerical aperture-0.95) revealed that eighteen isolates were rods and thirteen were oval. At 0 Mpa (Megapascal) stress level, all the isolates grew well in TSB, but their growth was slowed as PEG−6000 concentration increased (Figure 1). Growth at −0.25, −0.50, −0.75, −1.25 Mpa stress levels was recorded in spectrophotometer at OD600, values were in the range of 0.2–1.09 (31 isolates), 0.14–0.99 (31 isolates), 0.09–0.62 (31 isolates), 0.02–0.39 (28 isolates), and 0.02–0.17 (15 isolates), respectively. Drought tolerant isolates had OD600 values >0.10 at a stress level of −1.25 Mpa. We also noticed that isolates grew well at stress levels ranging from −0.25 to −0.75 Mpa.
Figure 1. Growth of bacterial Isolates under drought stress. As the concentration of PEG-6000 increases, many isolates' growth was inhibited. Few isolates tolerated the higher concentrations of PEG-6000, which were labeled as Drought tolerant bacteria. *indicates that the isolate that doesn't fall in the mean values.
Furthermore, these isolates were tested for their ability to develop EPS at a stress level of −0.75 Mpa. Nine isolates were able to produce exopolysaccharides with concentrations ranging from 0.20 to 0.99 mg ml−1. Drought stress caused a significant amount of EPS demand. CPB 24 had the most EPS (0.99 mg ml−1) under similar conditions (Table 1). The isolates with the maximum in vitro drought tolerance and EPS output were selected for in vivo cotton growth promotion under drought stress.
Table 1. Biochemical and plant growth attributes: physiological/biochemical responses of efficient isolates and production of EPS, ACC deaminase, IAA, HCN under in vitro conditions.
The physiological or biochemical characteristics of isolates are illustrated in Table 1. Selective isolates such as CPB 23, CPB 24, CPB 25, CPB 27, and CPB 31 were characterized using 16S rRNA sequencing. BLAST analysis of 16S rRNA isolates showed homology Pseudomonas stutzeri, Acinetobacter sp., Bacillus mojavensis, Pseudomonas chlororaphis, and Enterobacter asburiae, respectively. These isolates were submitted to NCBI-GenBank and obtained accession numbers i.e., MZ614657, MZ614623, MZ614631, MZ614656, and MZ614653, respectively.
Phosphate solubilizers were characterized by forming a clear halo zone around the colony on Pikovoskay's media after 5 days of incubation. Fifteen isolates tested positive in this test, with CPB-24 (Acinetobacter sp.) having the maximum solubilization of 21 mm (Table 1). Solubilization zones ranged from 2 to 21 mm in diameter. Potassium release on Aleksandrov's medium was recorded in 13 isolates with solubilization zones ranging from 3 to 18 mm, isolate Acinetobacter sp., showed a maximum of 18 mm solubilization zone. Zinc phosphate solubilization assay was performed on Tris Minimal media Solubilization, and observation was recorded after 5 days of inoculation. Thirteen isolates were positive for this test. The diameter of solubilization zones was in the range of 3–22 mm. Highest zinc phosphate solubilized by Enterobacter asburiae (22 mm).
The production of IAA by 31 phyllosphere bacteria was quantified at 530 nm in a spectrophotometer. The isolates released different amounts of indole acetic acid, with 9 preferring to grow in L-tryptophan's presence. They produced significant amounts of IAA in the range of 3.81–17.93 μg ml−1. When combined with 0.1% of L-tryptophan, Acinetobacter sp. produced the maximum IAA of 17.93 μg/ml, followed by Enterobacter asburiae with 14.44 μg/ml. ACC deaminase activity test was performed on Dworkin and Foster (DF) salts minimal broth and found seven positive isolates viz., CPB 11, Pseudomonas stutzeri, Acinetobacter sp., Bacillus mojavensis, Pseudomonas chlororaphis, and Enterobacter asburiae. Quantification of ACC deaminase activity was performed based on the production of α-ketobutyrate through deamination reaction in DF minimal media. The observation was recorded at 540 nm in the spectrophotometer, and ACC deaminase activity was recorded in the range of 0.13–0.40 mM of α-ketobutyrate per milligram of cellular protein per hour. The highest ACC deaminase activity was reported in the bacterial isolate Acinetobacter sp. (0.40 mM α-ketobutyrate mg protein−1 h−1) followed by Pseudomonas stutzeri (0.32 mM α-ketobutyrate mg protein−1 h−1) (Table 1). The highest IAA production was reported in Acinetobacter sp. (17.93 μg ml−1), followed by Enterobacter asburiae (14.44 μg ml−1).
HCN production was observed in 21 isolates. Eight were classified as vigorous HCN producers and 13 isolates as moderate HCN producers based on the brown color intensity developed in picric acid filter paper. Siderophore production was observed among the 13 isolates; of these, 3 isolates were classified as the highest siderophore producers and 10 isolates as moderate producers based on the size of the yellow halo zone around the colony.
The influence of the five highest ACC deaminase, IAA producing and phosphate, zinc, and potassium solubilizing PGPR strains, namely, Pseudomonas stutzeri, Acinetobacter sp., Bacillus mojavensis, Pseudomonas chlororaphis, and Enterobacter asburiae, on growth promotion of cotton under no stress and drought stress conditions, were evaluated through pot trials. Soil nutrient status was improved over control plants. The soil nitrogen (224 kg/ha), phosphorous (99.8 kg/ha), and potassium (547.3 kg/ha) content were highest in Acinetobacter sp. treated soils (Table 2). The compatibility test between the isolates was satisfactory and did not find any inhibitory activity between the five efficient isolates.
The adverse effects of drought stress resulted in a reduction in growth and yield parameters such as germination percentage, seedling vigor index, number of bolls per plant, boll weight, seed cotton yield per plant, plant height, root length, number of leaves, number of branches, fresh and dry weight of root and shoot of cotton plants in uninoculated plants compared to non stressed cotton plants. However, the plant growth and yield parameters in root and shoot length, fresh and dry weight, boll weight, and cotton yield were significantly (p < 0.01) increased in bacterial inoculated plants under drought-stressed conditions. The isolate Acinetobacter sp. application enhanced cotton plant growth exposed to drought-stressed conditions (30% PEG-6000).
The seed germination was significantly higher in T4 (Acinetobacter sp., + 30% PEG-6000) 76.67%, which was 17% higher than the control treatment. The seedling vigor index increased by 45.81% in T4 compared to the control under drought stress conditions. The plant height had improved (p < 0.01) by 10% in T4 treatment than corresponding uninoculated control plants. However, no significant difference was found in the case of shoot length between individual strain applications.
Similarly, the isolate Acinetobacter sp. treatment had a tremendous influence on root length, number of leaves, and the number of secondary branches of cotton plants on exposure to drought-stressed conditions. The root length was increased by ~28.52% in T4 treatment than controls with an unstressed and stressed plants group. Furthermore, increased fresh and dry weights under drought stress were recorded in Acinetobacter sp. and Enterobacter asburiae treated cotton plants (Table 3). Fresh weight was significantly (p < 0.01) increased by 41.9 and 33.3%, while dry weight improved by 41.7 and 29.7% at T4 and T7 treatments when compared to the stressed control (T2). A significant increase in the proline content was reported in inoculated cotton plants over the control plants. The proline content in cotton plants under drought stress was increased by 28.78% in T4 treatment, followed by 25.39 at Treatment T7. The number of bolls and boll weight were significantly higher in Acinetobacter sp., treated cotton plants (Table 3). The number of bolls was markedly higher in T4 (12.66 per plant), followed by T7 (11.66 per plant). The boll weight in cotton was highest in T4 (5.36 g), followed by T7 (5.10 g). Similarly, the seed cotton yield was highest in T4 (1027.77 kg ha−1).
Table 3. Influence of phyllosphere bacteria on the cotton crop: significant increase in fresh and dry weights of cotton in bacteria primed treatments.
Cultivable bacterial populations in rhizosphere and phyllosphere samples were analyzed on Nutrient Agar plates. The bacterial population in the rhizosphere and phyllosphere samples were increased up to 60 days of sowing in all treatments; the maximum population was reported in Acinetobacter sp., treated cotton plants (Table 3). The cultivable bacterial population in the rhizosphere and phyllosphere were recorded at 60 DAS as 5.70 × 107 and 6.25 × 105 CFU gm−1.
Drought conditions are better controlled by the soil application of microorganisms as drought mainly refers to soil conditions. In addition, soil application will enhance their chances of survival in the rhizosphere, as the rhizosphere is a nutrient-rich environment and better coordinates with plants' defense mechanisms to control soil-borne pathogens. The phyllosphere microorganisms with ACC deaminase activity play a significant role in promoting plants grown under different stress conditions (Chandra et al., 2018). When the plant is under stress, an intermediate compound of ethylene called 1-aminocyclopropane-carboxylic acid (ACC) makes its way into the rhizosphere as a root exudate. Rhizosphere microbes produce a special kind of enzyme called ACC deaminase, which breaks ACC and produces ammonia and α-ketobutyrate (Zhang et al., 2010). Ultimately, the ethylene production in plants was interrupted by rhizosphere microorganisms and usually helped the plant function (Barnawal et al., 2014).
In the present work, 31 phyllosphere bacterial isolates were tested for drought tolerance under in vitro conditions. Five isolates grew at high moisture stress (−1.25 Mpa) and were identified as Pseudomonas stutzeri, Acinetobacter sp., Bacillus mojavensis, Pseudomonas chlororaphis, and Enterobacter asburiae based on BLAST analysis. The cotton plants inoculated with the highest EPS (0.99 mg ml−1) and ACC deaminase-producing (Figure 2) isolate Acinetobacter sp., (CPB 24) showed maximum nutrient uptake and soil nutrient status. Bashan et al. (2004) reported, inoculation with EPS producing Azospirillum sp. increased soil nutrient status, structure, and aggregation. Several strains of Pseudomonas sp., Bacillus sp., and Acinetobacter sp. were reported to produce EPS, IAA, ACC deaminase, and solubilize insoluble minerals under different strains (Verma et al., 2018; Nagaraju et al., 2020). The precursor (L-tryptophan) concentration directly affects IAA produced (Nagaraju et al., 2020). According to current research, isolates preferred to grow at a five mM concentration of L-tryptophan and generated variable amounts of IAA, which was confirmed spectrophotometrically at 600 nm optical density.
Figure 2. Exopolysaccharides (EPS) and ACC deaminase production. The production of both EPS and ACC was less commonly observed; few isolates were positive for exopolysaccharides production, and very few were able to produce ACC deaminase. The isolate CPB-24 was able to produce both exopolysaccharides and ACC deaminase.
In many cultivated plants, nutrient uptake was impeded by drought stress; however, in the current study, inoculated plants showed increased plant nutrient status and fresh and dry weights. The highest levels of soil nitrogen, phosphorous, and potassium were recorded in Acinetobacter sp. treated plots. Concomitant observations made under in vitro conditions showed that phosphorous and potassium solubilization were highest in the same treatment. Earlier reports suggested that phosphate solubilization and chelation by beneficial soil microorganisms are the primary reason for the higher nutrient status in soil (Kour et al., 2020). Several findings support early reports on zinc, phosphate, and potassium solubilization and confirm their universal soil occupancy (Kour et al., 2019). Solubilization of the minerals/chemicals are accomplished by lowering the pH of the surroundings, production of several organic acids by Pseudomonas sp., Enterobacter sp., and Bacillus sp., was reported by Nagaraju et al. (2016, 2020). In addition, several microbes produce siderophores when the available forms of iron and zinc are limited in soil (Priyanka et al., 2015). Several reports have highlighted the importance of siderophore production in promoting plant growth. However, little work has been carried out under drought-stress conditions. In the present investigation, we highlighted the production of siderophores by drought-tolerant phyllosphere bacteria.
Intergenic compatibility i.e., seed and microbes, were tested by inoculating drought-tolerant PGPR bacterial isolates to seeds under drought stress and found a significant improvement in germination percentage and seedling vigor, compared with the untreated control. The compatibility between the bacterial isolates was confirmed in the dual culture test and recorded their cumulative positive effects in cotton crop growth when applied as a consortium under a pot culture experiment (Figure 3). In nature, microbes work synergistically with some microbes (Morris et al., 2013). Thus, applying a combination of two or more beneficial microbes to higher populations may positively impact the growth and yield of crops under different stresses (Triveni et al., 2013; Barra et al., 2016; Nagaraju et al., 2020). However, several researchers have reported that individual cultures might perform on par or higher than consortium because of their variable inhibitory activity under in vivo conditions when compared to in vitro conditions (Kour et al., 2019). In the current investigation, mono-inoculation with Acinetobacter sp. individual culture inoculated plants outperformed the control. In the present research, control plants showed the lowest root and shoot length and recorded the lowest proline content. Plants produce stress hormones under drought stress, which result in decreased yields. Several researchers suggest that applying plant growth-promoting bacterial isolates manifests ethylene production in plants and thus confers drought tolerance and crop growth (Safari et al., 2018). In the pot culture experiment, seed bacterization of PGP bacterial isolates enhanced the root, shoot length, fresh and dry weights, boll weight, number of bolls per plant, number of leaves per plant, and secondary branches per plant because of their multifarious PGP traits (Figure 4). Our study emphasizes that using a single strain or a consortium enhances crop growth under stressed and non-stressed conditions. Therefore, the application of stress-bursting microbes induces tolerance and increases crop growth under harsh climatic conditions. Furthermore, these can be multiplied and commercialized as biofertilizers or bio inoculants after careful evaluation in pot and field trials.
Figure 3. Growth promoting by phyllosphere bacteria in cotton. A significant increase in plant height with the inoculation of CPB-24 was recorded in cotton crops under imposed drought conditions.
Figure 4. Effect of phyllosphere bacteria on cotton growth parameters under drought conditions. (A) Plant height (B) number and leaves, and number of branches per plant.
The current study reports on the isolation of drought-tolerant ACC deaminase-producing bacteria from the phyllosphere of cotton. Five potential strains, Pseudomonas stutzeri, Acinetobacter sp., Bacillus mojavensis, Pseudomonas chlororaphis, and Enterobacter asburiae, were found to have plant growth-promoting potentials such as siderophore production, IAA production, phosphate, zinc, and potassium solubilization. Screening for drought tolerance in pot culture conditions revealed that inoculating drought-tolerant isolates improved cotton crop growth and yield. It is also unclear if inoculating other plants with these drought-tolerant isolates has a comparable effect on plants other than cotton, allowing them to produce more grain or fruit under various stresses. As a result, more emphasis should be given to multi-location trails with multiple crops in future research.
The datasets presented in this study can be found in NCBI-GenBank online repositories. The names of the NCBI-GenBank and accession number(s)- MZ614657, MZ614623, MZ614631, MZ614656, and MZ614653 can be found in the article.
SS conducted all the experiments under the guidance of ST. ST, PL, and BV were involved in designing the experiment. YN analyzed the data and wrote the manuscript. All authors contributed to the article and approved the submitted version.
The authors declare that the research was conducted in the absence of any commercial or financial relationships that could be construed as a potential conflict of interest.
All claims expressed in this article are solely those of the authors and do not necessarily represent those of their affiliated organizations, or those of the publisher, the editors and the reviewers. Any product that may be evaluated in this article, or claim that may be made by its manufacturer, is not guaranteed or endorsed by the publisher.
Abdulbaki, A. A., and Andersons, J. D. (1973). Vigour determination in soybean by multiple criteria. Crop Sci. 13, 630–637. doi: 10.2135/cropsci1973.0011183X001300060013x
Ali, S., and Kim, W. C. (2018). Plant growth promotion under water: decrease of waterlogging-induced ACC and ethylene levels by ACC deaminase-producing bacteria. Front. Microbiol. 9:1096. doi: 10.3389/fmicb.2018.01096
Ausubel, F. M., Albright, L. M., and Jingyue, J. (1999). Current Protocols in Molecular Biology. John Wiley & Sons, Inc., Media, PA. 701–705.
Barnawal, D., Bharti, N., Maji, D., Chanotiya, C. S., and Kalra, A. (2014). ACC deaminase-containing Arthrobacter protophormiae induces NaCl stress tolerance through reduced ACC oxidase activity and ethylene production resulting in improved nodulation and mycorrhization in Pisum sativum. J. Plant Physiol. 171, 884–894. doi: 10.1016/j.jplph.2014.03.007
Barra, P. J., Inostroza, N. G., Acuña, J. J., Mora, M. L., Crowley, D. E., and Jorquera, M. A. (2016). Formulation of bacterial consortia from avocado (Persea americana Mill.) and their effect on growth, biomass and superoxide dismutase activity of wheat seedlings under salt stress. Appl. Soil Ecol. 102, 80–91. doi: 10.1016/j.apsoil.2016.02.014
Bashan, Y., Holguin, G., and De-Bashan, L. E. (2004). Azospirillum-plant relationships: physiological, molecular, agricultural, and environmental advances (1997–2003). Can. J. Microbiol. 50, 521–577. doi: 10.1139/w04-035
Bates, L. S., Waldern, R. D., and Teare, J. D. (1973). Rapid determination of free proline in water stresses studies. Plant Soil 38, 205–208. doi: 10.1007/BF00018060
Cappuccino, J. G., and Sherman, N. (1992). Microbiology: A Laboratory Manual. The Benjamin/Comings Publishing Company, Inc. California.
Castric, K. F., and Castric, P. A. (1983). Method for rapid detection of cyanogenic bacteria. Appl. Environ. Microbiol. 45, 700–702. doi: 10.1128/aem.45.2.701-702.1983
Chandra, D., Srivastava, R., Glick, B. R., and Sharma, A. K. (2018). Drought-tolerant Pseudomonas spp. Improve the growth performance of finger millet (Eleusine coracana (L.) Gaertn.) under nonstressed and drought-stressed conditions. Pedosphere 28, 227–240. doi: 10.1016/S1002-0160(18)60013-X
Chauhan, H., Bagyaraj, D. J., Selvakumar, G., and Sundaram, S. P. (2015). Novel plant growth promoting rhizobacteria prospects. Appl. Soil Ecol. 95:38–53. doi: 10.1016/j.apsoil.2015.05.011
Chinnadurai, C., Balachandar, D., and Sundaram, S. P. (2009). Characterization of 1- aminocyclopropane-1-carboxylate deaminase producing methylobacteria from phyllosphere of rice and their role in ethylene regulation. World J. Microbiol. Biotechnol. 25, 1403–1411. doi: 10.1007/s11274-009-0027-1
Cohen, A. C., Bottini, R., Pontin, M., Berli, F. J., Moreno, D., and Boccanlandro, H. (2015). Azospirillum brasilense ameliorates the response of Arabidopsis thaliana to drought mainly via enhancement of ABA levels. Physiol. Plant. 153, 79–90. doi: 10.1111/ppl.12221
Colby, J., and Zatman, L. J. (1973). Trimethylamine metabolism in obligate and facultative methylotrophs. Biochem. J. 132, 101–112. doi: 10.1042/bj1320101
Collins, C. H., and Lyne, P. M. (1970). Microbiological methods. Baltimore, MD: Butterworths London, University Park Press. 171–311.
Corpe, W. A., Jensen, T. E., and Baxter, M. (1985). Fine structure of cytoplasmic inclusions of some methylotrophic bacteria from plant surfaces. Arch. Microbiol. 145, 107–112. doi: 10.1007/BF00446765
Creus, C., Sueldo, R., and Barassi, C. (2004). Water relations and yield in Azospirillum- inoculated wheat exposed to drought in the field. Can. J. BOT. 82, 273–281. doi: 10.1139/b03-119
Glick, B. R., Cheng, Z., Czarny, J., and Duan, J. (2007). Promotion of plant growth by ACC deaminase-producing soil bacteria. Eur J Plant Pathol. 119, 329–339. doi: 10.1007/s10658-007-9162-4
Gordon, S. A., and Weber, R. P. (1951). Colorimetric estimation of indole acetic acid. Plant Physiol. 26, 192–195. doi: 10.1104/pp.26.1.192
Gosal, S. S., Wani, S. H., and Kang, M. S. (2009). Biotechnology and drought tolerance. J. Crop Improv. 23, 19–54. doi: 10.1080/15427520802418251
Heddi, A., Grenier, A. M., Khatchadourian, C., Charles, H., and Nardon, P. (1998). Four intracellular genomes direct weevil biology: nuclear, mitochondrial, principal endosymbiont, and Wolbachia. Proc. Natl. Acad. Sci. U.S.A. 96, 6814–6819. doi: 10.1073/pnas.96.12.6814
Holland, M. A., Long, R. L. G., and Polacco, J. C. (2002). “Methylobacterium spp.: phylloplane bacteria involved in cross-talk with the plant host?,” in Phyllosphere Microbiology, eds S. E. Lindow, E. I. Hecht-Poinar, and V. J. Elliot (St. Paul, MN: APS Press). p.125–35.
Holland, M. A., and Polacco, J. C. (1994). PPFMs and other contaminants: is there more to plant physiology than just plant. Ann. Rev. Plant Physiol. 45, 197–209. doi: 10.1146/annurev.pp.45.060194.001213
Honma, M., and Shimomura, T. (1978). Metabolism of 1-aminocyclopropane- 1-carboxylic acid. Agric. Biol. Chem. 42, 1825–1831. doi: 10.1080/00021369.1978.10863261
Isenberg, H. D., and Sundheim, L. H. (1958). Indole reactions in bacteria. J. Bacteriol. 75, 682–690. doi: 10.1128/jb.75.6.682-690.1958
Islam, M. S., Huda, K. M. K., Firoz, M., Sufara, A. B., and Myeong, H. W. (2009). Regeneration and genetic transformation of Tossa Jute (Corchorus olitorius L.). Aust. J. Crop Sci. 3, 287–293, doi: 10.3316/informit.037844546239425
Kour, D., Rana, K. L., Sheikh, I., Kumar, V., Yadav, A. N., Dhaliwal, H. S., et al. (2020). Alleviation of drought stress and plant growth promotion by Pseudomonas libanensis EU-LWNA-33, a drought-adaptive phosphorus-solubilizing bacterium. Proc. Natl. Acad. Sci. U.S.A. 90, 785–795. doi: 10.1007/s40011-019-01151-4
Kour, D., Rana, K. L., Yadav, A. N., Yadav, N., Kumar, V., Kumar, A., et al. (2019). “Drought-tolerant phosphorus-solubilizing microbes: biodiversity and biotechnological applications for alleviation of drought stress in plants,” in Plant Growth Promoting Rhizobacteria for Sustainable Stress Management: Volume 1: Rhizobacteria in Abiotic Stress Management, eds R. Z. Sayyed, N. K.Arora, M. S. Reddy (Springer: Singapore), 255–308. doi: 10.1007/978-981-13-6536-2_13
Kumar, M., Kour, D., Yadav, A. N., Saxena, R., Rai, P. K., Jyoti, A., et al. (2019). Biodiversity of methylotrophic microbial communities and their potential role in mitigation of abiotic stresses in plants. Biologia 74, 287–308. doi: 10.2478/s11756-019-00190-6
Kunova, A., Bonaldi, M., Saracchi, M., Pizzatti, C., Chen, X., and Cortesi, P. (2016). Selection of Streptomyces against soil borne fungal pathogens by a standardized dual culture assay and evaluation of their effects on seed germination and plant growth. BMC Microbiol. 16:272. doi: 10.1186/s12866-016-0886-1
Legein, M., Smets, W., Vandenheuvel, D., Eilers, T., Muyshondt, B., Prinsen, E., et al. (2020). Modes of action of microbial biocontrol in the phyllosphere. Front. Microbiol. 11:1619. doi: 10.3389/fmicb.2020.01619
Macfaddin, J. F. (2000). Biochemical Tests for Identification of Medical Bacteria. Media, PA; Philadelphia, PA: John Wiley & Sons, Inc; Lippincott Williams & Wilkins 912–928.
Madhaiyan, M., Poonguzhali, S., Sundaram, S. P., and Tongmin, S. A. (2005). A new insight into foliar applied methanol influencing phylloplane methylotrophic dynamics and growth promotion of cotton (Gossypium hirsutum) and sugarcane (Saccharum officinarum). Environ. Exp. Bot. 57, 168–176. doi: 10.1016/j.envexpbot.2005.05.010
Marulanda, A., Azcon, R., Ruiz-Lozano, J. M., and Aroca, R. (2008). Differential effects of a Bacillus megaterium strain on Lactuca sativa plant growth depending on the origin of the arbuscular mycorrhizal fungus coinoculated: physiologic and biochemical traits. J Plant Growth Regul. 27:10. doi: 10.1007/s00344-007-9024-5
Michel, B. E., and Kaufmann, M. R. (1973). The osmotic potential of polyethylene glycol 6000. Plant Physiol. 51, 914–916. doi: 10.1104/pp.51.5.914
Molina-Favero, C., Creus, C. M., Simontacchi, M., Puntarulo, S., and Lamattina, L. (2008). Aerobic nitric oxide production by Azospirillum brasilense Sp245 and its influence on root architecture in tomato. Mol. Plant Microb. Interact. 2, 1001–1009. doi: 10.1094/MPMI-21-7-1001
Morris, B. E., Henneberger, R., Huber, H., and Moissl-Eichinger, C. (2013). Microbial syntrophy: interaction for the common good. FEMS Microbiol. Rev. 37, 384–406. doi: 10.1111/1574-6976.12019
Nagaraju, Y., Gundappagol, R. C., and Mahadevaswamy. (2020). Mining saline soils to manifest plant stress-alleviating halophilic bacteria. Curr. Microbiol. 77, 2265–2278. doi: 10.1007/s00284-020-02028-w
Nagaraju, Y., Triveni, S., Subhashreddy, R., Vidyasagar, B., Kumar, B. P., Chari, K. D., et al. (2016). Screening of zinc solubilizing and potassium releasing bacterial and fungal isolates from different rhizosphere soils different rhizosphere soils. Bioscan. 11, 2187–2192.
Parmar, P., Nagesh, S., and Sindhu, S. S. (2016). Potassium solubilization by rhizosphere bacteria: influence of nutritional and environmental conditions. J. Microbiol Res. 3, 25–31. doi: 10.5923/j.microbiology.20130301.04
Pikovaskaya, R. I. (1948). Mobilization of phosphorus in soil connection with the vital activity of some microbial species. Microbiologia 17, 362–370.
Prajapati, K. B., and Modi, H. A. (2012). Isolation and characterization of potassium solubilizing bacteria from ceramic industry soil. J. Microbiol. 1, 8–14.
Priyanka, K., Nath, A., and Chaube, R. (2015). Identification of human drug targets using machine-learning algorithms. Comput. Biol. Med. 56, 175–181. doi: 10.1016/j.compbiomed.2014.11.008
Qian, L., Chen, X., Wang, X., Huang, S., and Luo, Y. (2020). The effects of flood, drought, and flood followed by drought on yield in cotton. Agronomy 10:555. doi: 10.3390/agronomy10040555
Rangaswami, G., and Bagyaraj, D. J. (1993). “Microbial biotechnology,” in Agricultural Microbiology (New Delhi: Prentice hall of india pvt. Ltd), 389–405.
Ripa, F. A., Cao, W. D., Tong, S., and Sun, J. G. (2019). Assessment of plant growth promoting and abiotic stress tolerance properties of wheat endophytic fungi. Biomed Res. Int. 27:6105865. doi: 10.1155/2019/6105865
Safari, D., Jamali, F., Nooryazdan, H. R., and Bayat, F. (2018). Evaluation of ACC deaminase producing 'Pseudomonas fluorescens' strains for their effects on seed germination and early growth of wheat under salt stress. Aust. J. Crop Sci. 12, 413–421. doi: 10.21475/ajcs.18.12.03.pne801
Saghafi, D., Asgari Lajayer, B., and Ghorbanpour, M. (2020). “Engineering bacterial ACC deaminase for improving plant productivity under stressful conditions,” in Molecular Aspects of Plant Beneficial Microbes in Agriculture, eds V. Sharma, R. Salwan, and L. K. Tawfeeq Al-ani (Amsterdam: Elsevier), 259–277. doi: 10.1016/B978-0-12-818469-1.00022-5
Sah, S. K., Reddy, K. R., and Li, J. (2016). Abscisic acid and abiotic stress tolerance in crop plants. Front. Plant Sci. 7:571. doi: 10.3389/fpls.2016.00571
Sandhya, V., Ali, Z., Grover, M., Reddy, G., and Bandi, V. (2015). Alleviation of drought stress effects in sunflower seedlings by the exopolysaccharides producing Pseudomonas putida strain gap-p45. Biol. Fertil. Soils 46, 17–26. doi: 10.1007/s00374-009-0401-z
Saravanan, V. S., Subramoniam, S. R., and Raj, S. A. (2003). Assessing in vitro solubilization potential of different zinc solubilizing bacterial (ZSB) isolates. Braz, J. Microbiol. 34, 121–125. doi: 10.1590/S1517-83822004000100020
Schwyn, B., and Neilands, J. B. (1987). Universal chemical assay for the detection and determination of siderophores. Anal. Biochem. 160, 47–56. doi: 10.1016/0003-2697(87)90612-9
Timmusk, S., and Wagner, E. G. H. (1999). The plant growth promoting rhizobacterium Paenibacillus polymyxa induces changes in Arabidopsis thalianana gene expression: a possible connection between biotic and abiotic stress responses. Mol. Plant Microbe. Interact. 12, 951–959. doi: 10.1094/MPMI.1999.12.11.951
Triveni, S., Prasanna, R., Shukla, L., and Saxena, A. K. (2013). Evaluating the biochemical traits of novel Trichoderma-based biofilms for use as plant growth-promoting inoculants. Ann. Microbiol. 63, 1147–1156. doi: 10.1007/s13213-012-0573-x
Verma, J. P., Jaiswal, D. K., Krishna, R., Prakash, S., Yadav, J., and Singh, V. (2018). Characterization and screening of thermophilic Bacillus strains for developing plant growth promoting consortium from hot spring of Leh and Ladakh region of India. Front. Microbiol. 9:1293. doi: 10.3389/fmicb.2018.01293
Whittenbury, R., Phillips, K. C., and Wilkinson, J. F. (1970). Enrichment, isolation and some properties of methane-utilizing bacteria. J. Gen. Microbiol. 61, 205–218. doi: 10.1099/00221287-61-2-205
Yadav, R. K., Karamanoli, K., and Vokou, D. (2010). Estimating bacterial population on the phyllosphere by serial dilution plating and leaf imprint methods. Ecol. Soc. 17, 47–52. doi: 10.3126/eco.v17i0.4105
Yang, J., Kloepper, J. W., and Ryu, C. M. (2009). Rhizosphere bacteria help plants tolerate abiotic stress. Trends Plant Sci.14:1–4. doi: 10.1016/j.tplants.2008.10.004
Yasmin, H., Nosheen, A., Naz, R., Bano, A., and Keyani, R. (2017). L-tryptophan-assisted PGPR-mediated induction of drought tolerance in maize (Zea mays L.). J. Plant Interact. 12, 567–578. doi: 10.1080/17429145.2017.1402212
Yates, F. (1937). The design and analysis of factorial experiments. Technical communication of the commonwealth bureau of soils. Commonwealth Agricul. Bureaux. 35:22.
Zakaria, M. S., and Sabrina, S. (2017). Cotton production and climatic factors: studying the nature of its relationship by different statistical methods. Cogent Biol. 3:1. doi: 10.1080/23312025.2017.1292882
Keywords: drought, water potential, ACC deaminase, phyllosphere, exopolysaccharides (EPS)
Citation: Sharath S, Triveni S, Nagaraju Y, Latha PC and Vidyasagar B (2021) The Role of Phyllosphere Bacteria in Improving Cotton Growth and Yield Under Drought Conditions. Front. Agron. 3:680466. doi: 10.3389/fagro.2021.680466
Received: 14 March 2021; Accepted: 29 September 2021;
Published: 12 November 2021.
Edited by:
Upendra Kumar, National Rice Research Institute (ICAR), IndiaReviewed by:
Durgesh Kumar Jaiswal, Banaras Hindu University, IndiaCopyright © 2021 Sharath, Triveni, Nagaraju, Latha and Vidyasagar. This is an open-access article distributed under the terms of the Creative Commons Attribution License (CC BY). The use, distribution or reproduction in other forums is permitted, provided the original author(s) and the copyright owner(s) are credited and that the original publication in this journal is cited, in accordance with accepted academic practice. No use, distribution or reproduction is permitted which does not comply with these terms.
*Correspondence: Y. Nagaraju, bmFnYXJhanVsdnJ0aDYyQGdtYWlsLmNvbQ==; bmFnYXJhanU1OUBob3RtYWlsLmNvbQ==
Disclaimer: All claims expressed in this article are solely those of the authors and do not necessarily represent those of their affiliated organizations, or those of the publisher, the editors and the reviewers. Any product that may be evaluated in this article or claim that may be made by its manufacturer is not guaranteed or endorsed by the publisher.
Research integrity at Frontiers
Learn more about the work of our research integrity team to safeguard the quality of each article we publish.